- Faculty of Education, University of Oulu, Oulu, Finland
Interests in Maker Education have been increasing among K-12 educators. In this study, we focused on one of the contexts of Maker Education, digital fabrication activities, at a makerspace in Finland. We aimed to explore: (1) the potentials and the factors to develop twenty-first century skills and computational thinking practices through digital fabrication activities, and (2) challenges of utilizing digital fabrication in K-12 Maker Education. We examined perspectives of teachers and facilitators who have roles to support pupils in Maker Education. We presented three cases of school visits (3–5 days), where the pupils (7–9th grades) created tangible artifacts with digital fabrication facilities at the makerspace. We collected data through participants' observation, informal interviews and focus group interviews with teachers and facilitators. For data analysis, we employed theory-driven and data-driven approaches. The results showed that digital fabrication activities can provide learning opportunities for twenty-first century skills and computational thinking practices. The teachers and the facilitators discussed the six factors of digital fabrication activities which influenced pupils' learning. However, the result also indicated the possibility that the teachers and the facilitators might not be familiar with the concepts of computational thinking. Also, different perspectives between the teachers and the facilitators toward the structure of the current activities surfaced. By identifying potentials and challenges of the current practices, the study has implications to advance Maker Education to be better integrated into K-12 school contexts.
Introduction
Making is described as “a class of activities focused on designing, building, modifying, and/or repurposing material objects, for playing or useful ends, oriented toward making a ‘product’ of some sort that can be used, interact with, or demonstrated” (Martin, 2015, p. 31). Katterfeldt (2013, p. 139–141) describes the maker culture phenomenon is built upon a long-run do-it-yourself (DIY) culture where people have been expressing themselves by designing and creating things by themselves. Martin (2015, p. 35) explains that maker mindset includes playful, growth-oriented, failure-positive, and collaborative values, beliefs, and dispositions that are shared in the community. The current maker culture is strongly influenced by digital technologies (Katterfeldt, 2013, p. 139; Martin, 2015, p. 32) and involves both traditional crafts and digital technologies in manufacturing and designing (Martin, 2015, p. 31). Especially, making artifacts with computer-controlled fabrication processes (and digital materials) is commonly described as digital fabrication (Gershenfeld, 2012, p. 50). Digital fabrication activity can be used as one of the contexts of Maker Education.
Previous studies have emphasized the benefits of applying making in education (e.g., Halverson and Sheridan, 2014, p. 497–501; Kafai et al., 2014; Näykki et al., 2019, p. 7–9; Papavlasopoulou et al., 2017, p. 61–63). Blikstein (2013, p. 4) illustrates constructionism as one of the theoretical and pedagogical pillars which underlies “at the very core of what making and digital fabrication mean for education” (p. 5). Constructionism was introduced by Seymour Papert, built upon Jean Piaget's constructivism (Papert and Harel, 1991). Constructionism emphasizes an individual constructs knowledge effectively in interactions with the physical and social environments, such as building and making and publicly sharing personally meaningful artifacts (Blikstein, 2013, p. 5).
Although Maker Education is often associated with STEM/STEAM (Science, Technology, Engineering, Art, Mathematics) education (e.g., Vossoughi and Bevan, 2014; Taylor, 2016; Vartiainen and Kumpulainen, 2020), opportunities which Maker Education provides are not limited to subject studies. Prototyping in ill-structured making activities enhances refining the idea, constraining materials, and getting inspiration (Yrjönsuuri et al., 2019, p. 150–157). In digital fabrication, fast prototyping enhances iterative cycles of failure and redesigning during the design processes (Blikstein, 2013, p. 13; Smith et al., 2015, p. 16). Hynes and Hynes (2018, p. 868) explain that makerspaces have the potential to provide the environments for interdisciplinary collaboration and self-directed learning, as well as fostering creativity, problem solving and design thinking skills. In other words, makerspaces place pupils' needs, interests and experiences as the starting point for their explorations. This type of student-centered approach creates a context that is built around creativity and allows affective learning processes, such as experiencing and expressing emotions (Näykki et al., 2019, p. 10).
Making activities are often ill-structured and open-ended (Pitkänen et al., 2019, p. 3). In fact, designing is not an easy process to straightforwardly implement ideas into practice (Kangas et al., 2013, p. 162). Previous studies have used frameworks, such as design thinking, to structure messy design processes in making activities (e.g., Smith et al., 2015; Andersen and Pitkänen, 2019; Montero et al., 2020). Hughes et al. (2019, p. 3–4) used design thinking as theoretical framework to examine primary school pupils' making activities. They conclude that design thinking potentially develop (1) problem-solving skills in design processes by guiding pupils to solve real-world problems and (2) knowledge creation by providing opportunities for challenging and questioning of existing knowledge (Hughes et al., 2019, p. 7–10).
While the benefits of Maker Education are emphasized, there are issues when it comes to integrating making and digital fabrication activities in K-12 education. For instance, Nemorin (2017) critically examined the attempt to introduce digital fabrication, specifically 3D modeling and printing, in a school context, and identified pragmatic issues which can arise in Maker Education. The issues include: (1) When digital fabrication activities are recognized as a school activity, it may hinder engagement. (2) Continuous experience of failure in the process of digital fabrication activities may bring negative emotions. (3) Lack of understanding and skills in operating technologies, such as 3D modeling software, may limit creativity (Nemorin, 2017, p. 15–18). While making and digital fabrication activities are often implemented as student-centered and ill-structured DIY activities, pupils need support and guidance during the activities (Pitkänen et al., 2019, p. 6–7).
Smith et al. (2016, p. 46) highlight the challenges in Maker Education due to the contradictions between curriculum-based education and explorative maker culture. When an out-of-school makerspace becomes a context to implement Maker Education, makerspace facilitators, who facilitate the activities and provide guidance to operate tools and machines, have as important role in pupils' learning as teachers. In the contexts of Maker Education, and digital fabrication in particular, both pedagogical and technological support are needed in order to enhance pupils' learning experience during the activities (Näykki et al., 2019, p. 10; Pitkänen et al., 2019, p. 6–7). However, currently, there are only a few studies, such as Pitkänen et al. (2019), closely examined different perspectives between school teachers (pedagogy experts) and makerspace facilitators (technology experts), toward Maker Education. To identify potentials and challenges in Maker Education and successfully implement at schools, it is essential to highlight both teachers' and facilitators' views.
Transversal Competences in Maker Education
In this study, we focus on potential of digital fabrication activities as contexts to develop non-subject transversal competences. Finnish National core Curriculum for basic education defines transversal competence as “an entity consisting of knowledge, skill, values, attitudes, and will,” which can be applied in a given situation (Finnish National Board of Education, 2016, p. 21). The curriculum emphasizes that competences that cross the boundaries and link different fields of knowledge and skills are a precondition in the future society (Finnish National Board of Education, 2016, p. 21). In the following sections, we introduce two non-subject transversal competences used in this study and their connections with Maker Education.
Twenty-First Century Skills
One of the non-subject transversal competences on which we focus in this study is twenty-first century skills. Several studies have examined the potential for learning twenty-first century skills in digital fabrication. Based on the survey, Peppler et al. (2015, p. 1–6) state that twenty-first century skills are highly aligned with daily activities in makerspaces. In addition, Taylor (2016) concludes the activities in makerspaces can be transformed into classroom projects that match the goals of twenty-first century education.
Various organizations, such as UNESCO, OECD, European Council, have emphasized importance to develop the skills which are required in twenty-first century. The Assessment and Teaching of Twenty-First Century Skills (ACT21S) project was launched in 2009, sponsored by three of the world's major technology companies, Cisco, Intel and Microsoft. It aimed to provide a guideline for educational institutes in many countries which are transforming from an industrial-based economy to information-based economy (Griffin et al., 2012, p. 2). The framework divides twenty-first century skills into four main categories and 10 subcategories (see Table 1).
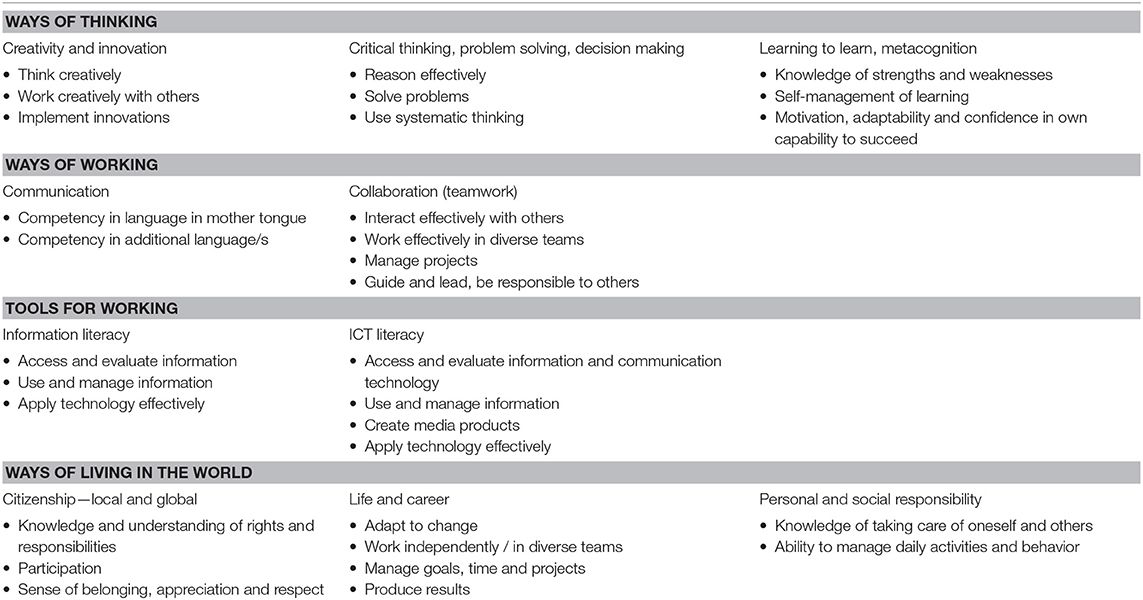
Table 1. Categorization of twenty-first Century Skills in ATC21S framework (Griffin et al., 2012, p. 18–19).
It defines each subcategory from three dimensions: knowledge, skills, and attitudes/values/ethics (Griffin et al., 2012, p. 2). Ways of thinking include skills which require greater focus and reflection to think systematically. Ways of working emphasize globalization where communication needs to be rapid, concise and aware of cultural differences. Tools for working involve accessing and evaluating new information efficiently to effectively utilize through skilled use of ICT to encounter information explosion. Ways of living in the world are based on the presumption that individuals are going to compete, connect and collaborate beyond the cultural differences.
The definition of twenty-first century skills in ATC21S framework from Griffin et al. (2012) and the seven transversal competences described in the Finnish National core curriculum for basic education from Finnish National Board of Education (2016) share core elements (Pitkänen and Iwata, 2019, p. 16). The seven transversal competences include: (1) Thinking and learning to learn, (2) Cultural competence, interaction and self-expression, (3) Taking care of oneself and managing daily life, (4) Multiliteracy, (5) ICT competence, (6) Working life competence and entrepreneurship, and (7) Participation, involvement and building a sustainable future (Finnish National Board of Education, 2016, p. 21–26). We chose twenty-first century skills as a bridge between pupils' learning and digital fabrication activities in their makerspace visit to provide grounds for utilizing such activities as part of the school curriculum.
Computational Thinking
Another non-subject transversal competence in this study is computational thinking (CT). Previous studies (e.g., Borges et al., 2017; Iwata et al., 2019; Laru et al., 2019) have discussed connections between digital fabrication and CT. By examining the making activities, Rode et al. (2015, p. 5–7) indicate that CT can be fostered in making. Montero (2018, p. 1–2) explains that digital fabrication activities are beneficial to introduce CT, rather than through programming alone, since such activities reduce the negative bias toward programming or coding. Digital fabrication may enhance learning the concepts of CT by providing hands-on experiences connected with personal interests.
CT refers to a way of solving complex problems by applying the set of thinking skills, practices and approaches which are fundamental to computing (Wing, 2006, p. 33). CT is built upon the fundamental concepts of computing: abstraction (“mental” tool of computing) and automation (operation of abstractions) (Wing, 2008, p. 3,717). Denning and Tedre (2019, p. xi) note that CT leads to understanding how computer works as well as possibilities and limitations of technologies, which is vital for taking advantage of technology-infused social world. Brennan and Resnick (2012) introduce broader understanding of CT, which concerns one's knowledge and actions as well as the world around him/ her. They illustrate three key dimensions of CT: (sequences, loops, events, parallelism, conditionals, operators, data), computational practices (being incremental and iterative, testing and debugging, reusing and remixing, abstracting, and modularizing), and computational perspectives (expressing, connecting, questioning) (Brennan and Resnick, 2012, p. 3–11). With the broader understanding of CT, Gretter and Yadav (2016) introduce an integrated approach to connect CT and twenty-first century skills, which concerns important knowledge, skills, and attitudes in digitalized, and globally connected world. In this study, we focused on CT to illustrate the potential of digital fabrication activities to develop one of the important skills in the modern society.
Since CT was described as “a fundamental skill for everyone, not just for computer scientists” (Wing, 2006, p. 33), interests in integrating CT in K-12 education have been increasing. Mannila et al. (2014) made the investigations of how CT is implemented in K-9 education. They conclude that some teachers already began integrating CT into schools activities even if the concepts of CT are not clearly defined in the national curriculums (Mannila et al., 2014, p. 24). However, Mäkitalo et al. (2019, p. 105–106) indicated more research is needed for developing teachers' understanding of CT and professional development in CT.
There have been several practical definitions to utilize the concepts of CT in K-12 education (e.g., Astrachan and Briggs, 2012, p. 39–41; Barr et al., 2011, p. 20–21; Grover and Pea, 2013, p. 39–40).
We used the operational definition of CT introduced by Barr et al. (2011) based on the joint project by International Society for Technology in Education (ISTE) and the Computer Science Teachers Association (CSTA). The definition was created for teachers to integrate CT with educational objectives and classroom practices. It is a set of skills, actions and practices which demonstrate the principles of CT emphasizing such CT practices as problem-solving process. The definition involves the following steps: (1) Formulating problems in a way that enables us to use a computer and other tools to help solve them; (2) Logically organizing and analyzing data; (3) Representing data through abstractions, such as models and simulations; (4) Automating solutions through algorithmic thinking (a series of ordered steps); (5) Identifying, analyzing, and implementing possible solutions with the goal of achieving the most efficient and effective combination of steps and resources; and (6) Generalizing and transferring this problem-solving process to a wide variety of problems (Barr et al., 2011, p. 21).
Aims of the Study and Research Questions
The aim of the study is two-folded: (1) to explore the potentials and the factors to develop transversal competences through digital fabrication activities. Among transversal competences, we especially focus on twenty-first century skills and CT; (2) to explore challenges of utilizing digital fabrication activities in K-12 education. We examine school teachers' and makerspace facilitators' perspectives toward the current practices of digital fabrication activities. To achieve the aim, we pursue the following three research questions:
(1) To what extent are twenty-first century skills, such as ways of thinking, ways of working, tools for working and ways of living in the world, recognized in digital fabrication activities?
(2) To what extent are CT practices recognized in digital fabrication activities?
(3) What are teachers' and facilitators' conceptions of the factors which influence pupils' learning of twenty-first century skills and CT practices in digital fabrication activities?
By identifying potentials and challenges of the current practices, the study has implications to advance Maker Education to be better integrated in K-12 school contexts.
Research Methods
This study has characteristics of ethnographic methodology. Ethnography is a term which describe both method and the written product of research that researchers immersing themselves in the contexts of the study (Bryman, 2012, p. 432). Although there are claims that observation is a primary source of information in ethnography (Gobo, 2008, p. 4), an ethnographic study entails a wide range of data collection and sources, such as interviews (Bryman, 2012, p. 432). The setting of an ethnography can be open (such as a public community) or closed (such as an organization), and the researcher can have covert role (not disclosing the fact that s/he is a researcher) or overt role (disclosing the fact that s/he is a researcher) (Bryman, 2012, p. 433–434). We chose ethnography as a research method because it allowed us to get close to the people involved in the context and thereby to collect more complete and intense understanding of their culture and values.
In this study, first two authors, who were the students at the Faculty of Education at the university, participated into daily activities of the makerspace (shared context with participants) for more than three years through various activities (e.g., internships at makerspaces, observing, and facilitating workshops for pupil groups at makerspaces, organizing, and facilitating workshops for pre-service and in-service teachers, university courses about digital fabrication, and Fab Academy1 One of the potential risks in an ethnographic study is that as the ethnographer immerse him-/ herself in the context, s/he becomes a native and forgets to see the context from his/ her own eyes (Bryman, 2012, p.445; Gobo, 2008, p. 7). We addressed this risk by the regular discussion among the authors where we discussed issues in the context with scientific point of views. In this way, we kept perspective as researchers while participating in the context as observers.
The role of the participating authors had changed throughout the study according as they participated in those activities as “Overt Full Members” (participating in the activities as members disclosing the fact that we are researchers) or as “Participating Observers” (participating in the activities as observers) (Bryman, 2012, p. 441–444). While participating in the activities, they interacted with other participants in the context. From these experiences, we have gained deep understanding of the practices, visiting school groups, tools, and other characteristics of the research context, which was used for overall research design, such as developing the research questions, forming interview themes and questions, and understanding and interpreting the data.
Participants and Context of the Study
The participants were five teachers from three local area schools and their classes (N = 41 pupils) as well as two makerspace facilitators. The context of the study is a makerspace in Finland. The makerspace offers digital fabrication facilities, such as 3D printers, a laser cutter, a high-resolution milling machine, a large CNC milling machine, a vinyl cutter, an electronics workbench, and a suite of tooling and materials. The makerspace is open for public providing visitors with opportunities to use the facilities. In addition, the makerspace has arranged different type of digital fabrication activities for school visitors. Current activities at the makerspace typically include: (1) 2D- and 3D-designing and manufacturing, (2) prototyping with electronics, (3) programming incorporating the basic programming of embedded systems with a high-level programming language, or (4) utilizing the tools and machines.
In this study, we focused on three cases of school visit. Table 2 describes the details of activities of the three cases. We chose those three cases because the cases have both similarities and differences, which allowed us to compare the cases and to examine the phenomenon from different perspectives. One of the similarities was that in all the three cases, participants visited the makerspace as part of multidisciplinary learning module. Multidisciplinary learning module is a learning approach, manifested in Finnish National core Curriculum for basic education, advocating to learn a phenomenon from multiple perspectives beyond the boarder of the subjects (Finnish National Board of Education, 2016). Another similarity is that the activities in the three cases followed the typical procedure of a digital fabrication activity for school visitors at this makerspace. The procedure included introduction of the environment, introduction of project objectives, planning, and working on the projects. In addition, the activities were implemented as group activities and as ill-structured problem-solving without strict instructions and schedule. In all the three cases, the activities were run by two facilitators who had technological background and worked at the makerspace. The facilitators' main role was to provide instructions of the basic operations of facilities and digital tools, and to help pupils when they had problems in the process rather than providing exact instructions. The teachers' role was mainly to observe the activities and manage general schedules of their students.
One the other hand, the three cases had differences in activity design, especially regarding origin of the projects (see Table 2). In the case I (School A), the pupils had total freedom of what they made with a few requirements, such as using a microcontroller. They had presentations after the end of the activity. In the case II (School B), the pupils decided what to make according to the theme provided by the teachers. Two projects were implemented as collaborative projects where two groups worked on the same project with different responsibility of the tasks: one group was taking care of physical design, while another dealt with mechanical design. One project was implemented individually by one group. In the case III (School C), the pupils visited the makerspace as a part of ongoing project: designing a model of a playhouse at the school. They had presentations in the middle and end of the activity.
Data Collection
We collected qualitative data of the three cases of digital fabrication activities through participants observation, informal interviews, and two focus group interviews (see Table 3). First, we observed the visiting schools' digital fabrication activities at the makerspace. We recorded data from the observations as field notes and photographs. In order to reduce the impact of the potential bias of the participating observers, we also collected the data from participants through interviews. During and after the activities, we conducted semi-structured informal interviews, in order to gain extended understanding of the context and the participants' first impressions on the activities. The interviewees of the informal interviews were the five teachers, and 13 pupils out of 41 in total. We used the data from the observations and informal interviews as pilot data to prepare for the main data collection.
After the observations, we conducted the semi-structured focus group interviews to examine the activities further together with teachers and facilitators and to use as main data for the study. In focus group interview I, the interviewees were three teachers from two secondary schools (School A, C), who participated in the three cases of the activities with their pupils. We collected the teachers' opinions on the following three themes: (1) preparation for the activities in the makerspace, (2) potential for learning twenty-first century skills and CT practices and the notable aspects that enhanced learning during the activities, and (3) applying activities in school contexts. In focus group interview II, we invited the makerspace facilitators who ran the activities. We asked the questions regarding following themes: (1) implementation of twenty-first century skills and CT practices in digital fabrication activities and (2) development of the activities. The focus group interviews were recorded as audio and videos. We conducted both informal interviews and focus group interviews in English, and later transcribed them.
Data Analysis
We familiarized ourselves with the pilot data (observations and informal interviews) and used it to understand the research context and to formulate the interview questions for the main data collection (focus group interviews). We conducted theory- and data-driven analyses of the focus group interviews data following the analysis strategies introduced by Krueger and Casey (2000, p. 132–138). To answer the first and the second research questions, we applied theory-driven analysis, in which we coded the transcripts based on the definitions from previous studies. We used the 10 subcategories defined by Griffin and colleagues (2012, p. 18–19) as twenty-first century skills, and looked for the parts which match the listed explanations of each subcategory (see Table 1). For instance, in the case of subcategory: Creativity and Innovation in Ways of Thinking, we looked for parts in the sentences in the transcribed interview data which match the explanations: think creatively, work creatively with others, and implement innovations, and coded the parts as Creativity and Innovation. For CT practices, we looked for the six concepts of CT practices defined by Barr and colleagues (2011, p. 21). The six concepts were already concrete and self-explanatory, thus we used the six concepts to look for the parts which should be coded. For the third research question, we performed data-driven analysis where we generated codes and categories inductively implementing different coding phases, such as initial coding and focused coding (Bryman, 2012, p. 568–570).
The unit of analysis of this study was institutional level. According to Lewis-Beck et al. (2004, p. 1,158), a unit of analysis is the subject of the study about which an analyst may generalize. In this study, we intended to highlight perspectives of teachers and makerspace facilitators toward the current activities. We were interested in perspectives of groups of people: teachers and facilitators, rather than perspectives of individual participants. Moreover, to avoid the possibility to identify individual participants, in the following section, we limited to specify individual participants and kept them general, such as “one teacher” and “one facilitator.”
We collaboratively carried out coding and data analysis. First, the primary coder designated the initial categories. For developing and refining the categories, the co-coder read the unmarked transcript of the focus group interviews and coded with the initial codes and categories created by the primary coder. Then we discussed the disagreements to modify the codes and the categories. Finally, based on the modified codes and categories, both the primary coder and the co-coder conducted coding again. We used NVivo in the coding process and to test the reliability of coding. The score of overall inter-rater agreement, Cohen's Kappa coefficient (Cohen, 1960), is k = 0.73.
Results
In this section, we answer to our three research questions: (1) To what extent are twenty-first century skills recognized in digital fabrication activities? (2) To what extent are CT practices recognized in digital fabrication activities? (3) What are teachers' and facilitators' conceptions of the factors which influence pupils' learning of twenty-first century skills and CT practices in digital fabrication activities?
Twenty-First Century Skills in Digital Fabrication Activities
Here, we present the results on to what extent twenty-first century skills are recognized by the teachers and the facilitators. Both the teachers and the facilitators discussed frequently about twenty-first century skills. Table 4 summarizes the identified twenty-first century skills from the two focus group interviews.
Ways of Thinking
Among the four categories of twenty-first century skills, both the teachers and the facilitators most frequently discussed about the category of ways of thinking (see Table 4). Both the teachers and the facilitators illustrated digital fabrication activities enhanced the pupils' creativity. They explained that the pupils engaged in the creative fabrication process with freedom and autonomy of ideation and designing. Also, the lively working environment with the machines and the tools increased the pupils' creativity. One teacher explained as follows:
“The environment at makerspace was very. makes you pretty creative, because all those staff and all the machines were around you, and you have just free hands do anything, do everything.”
The teachers and the facilitators recognized the opportunities of presentations during the activities as one of the possibilities to develop pupils' metacognitive skills. One facilitator said:
“This [presentations] helped them to understand what they have learnt.”
In the presentations, the pupils explained the idea and the progress of their project. The teachers noted reflecting on their own learning during the activities allowed the pupils to have metacognitive skills. In addition, the teachers found the possibility for the pupils to understand and utilize own strength during the activities. One teacher described as:
“Students really have to think what is my area, what is my best skills and use those to that project.”
The facilitators especially highlighted problem solving, critical thinking and decision making during the activities. One facilitator explained:
“Critical thinking, problem-solving, and decision making is part of the whole process of [the name of makerspace].”
Especially they emphasized decision making is embedded in every step in the process of the activity.
Ways of Working
Both the teachers and the facilitators noted the pupils learned collaborative skills through the activities where they worked as a team. One teacher reflected:
“Sometimes they had to divide the work and while one person is doing one thing, the other person is doing another thing, so you need to trust in what the other is doing.”
Regarding communication skills, both the teachers and the facilitators stressed the opportunities to develop competency in English. Although the instructions during the activities were mainly in Finnish, the pupils had to use English to search relevant information from the online platforms where people share instructions. One teacher reflected as follows:
“One benefit…. was the speaking in English. The ninth graders was first very. kind of covered in own cave but then, during that week they started to speak. And I was very surprised in Thursday-Friday, that all of those guys were speaking, very freely, in English!”
Tools of Working
The facilitators discussed frequently about the category of tools for working (see Table 4). Both the teachers and the facilitators indicated the opportunities of learning ICT literacy with emphasis of programming. The pupils, who used microcontrollers (see Table 2), searched the relevant codes from the online platforms and modified the existing codes to utilize them for own project. One facilitator explained as follows:
“Perhaps in some of the cases, it was just taking a code, copy, and paste it, but they need more or less to understand, what the code was doing. So, I think in that sense we are dealing with many of the aspects of information and communication technology.”
The facilitators emphasized information literacy. The pupils needed to find relevant information by themselves to apply to their project. One facilitator described:
“I think one of the most important things is that how to find information, how to select the correct keywords to find a relevant information.”
The facilitators explained that instead of providing answers, they gave the pupils the keywords, such as specific technical terms, which helped them to reach relevant information. One facilitator illustrated:
“To understand where they have to search results, they have to understand what they need, what is relevant, what is the best answer, what is the example that you could then start developing from.”
Ways of Living in the World
The teachers frequently discussed about the category of ways of living in the world (see Table 4). The teachers highlighted the pupils had the opportunities to develop skills of management of goal, time and project which is one of the skills regarding life and career (see Table 1).
The teachers noticed some of the pupils were not used to the different working style. They emphasized the needs to develop working styles and the attitudes in the real working places to avoid being used to the working style at schools. One teacher illustrated:
“That is something that what we should start implementing, this kind of working, much earlier on. So that they kind of learn the right way of working, not just give me a focus that we need.”
Moreover, the teachers found the concepts of citizenship can be learnt through the activities. In the case of School C, when the pupils worked on the project related to their community, designing new playhouse for the school, they had the opportunities to think community's needs to develop the project (see Table 2).
Computational Thinking Practices in Digital Fabrication Activities
Second research question was focusing on CT practices in digital fabrication activities and how teachers and facilitators recognized those practices. In the Table 5, the results of identification of CT practices in digital fabrication activities based on the teachers' and the facilitators' focus group interviews are presented.
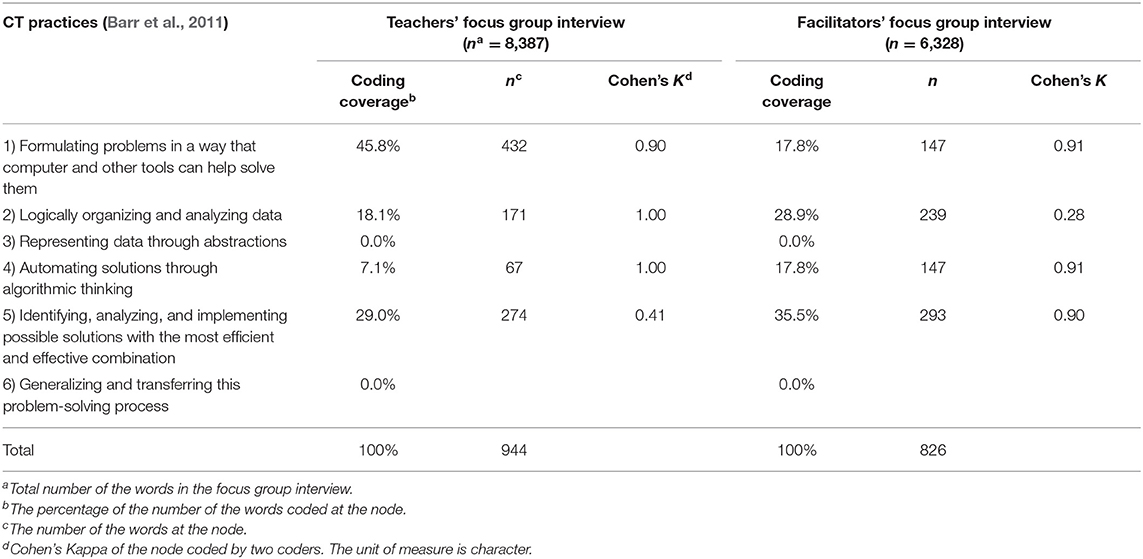
Table 5. Summary of the aspects of CT practices identified in focus group interviews (developed based on Iwata et al., 2019).
The facilitators reflected digital fabrication processes involve CT which engineers use regularly while working. One facilitator explained as follows:
“Any process in [name of the makerspace] requires this way of thinking: go through these logical steps. For example, if you want to make a printed circuit board or milled circuit board, you have to follow these certain steps, and it is computational thinking, it's an algorithm you have to follow.”
Among the six aspects of CT practices, the teachers discussed most frequently about “Formulating problems in a way that computer and other tools can help solve them.” During the activities, the pupils used computers for designing and fabrication. For instance, the pupils drew design for an artifact on the computer to fabricate with a laser cutter, or they wrote the codes for a microcontroller to add specific functions on an artifact.
Additionally, both the teachers and the facilitators discussed frequently about “Logically organizing and analyzing data” and “Identifying, analyzing, and implementing possible solutions with the most efficient and effective combination.” In the activities the pupils needed to logically organize and analyze information and identify each step required in the process. They considered the most efficient way to make their artifacts function as they wished and the most effective procedure to build it. One facilitator illustrated as follows:
“You need to think first the external design, then how you are going to put inside the mechanics, and after that how the mechanics is going to work, so when you press the button you have servo that moves and opens the leak and switch off the button again and then go back, so whole this process is step by step process, so I think that sense is computational thinking.”
Although the teachers and the facilitators identified the several aspects of CT practices, neither of them discussed about CT practices frequently. One facilitator mentioned that CT is not the most familiar concepts for schools as follows:
“It's basically engineering is computational thinking. But at schools I don't think teachers have this build-in curriculum to follow.”
In fact, although ideas of CT are embedded in school curriculum in Finland, it is not named as CT.
However, also the facilitators were not fully aware of the concepts of CT. One facilitator expressed:
“I don't know. We don't know actually, we guess that they are learning CT. But we don't know they are.”
Teachers' and Facilitators' Conceptions of the Factors Which Influence Pupils' Learning in Digital Fabrication Activities
The teachers and the facilitators discussed six factors which had influences on pupils' learning twenty-first century skills and CT practices. In the following sections, we explain the six factors under three categories: task characteristics of the digital fabrication activities, facilitation of the digital fabrication activities, and learning environment and the structure of the digital fabrication activities.
Task Characteristics of the Digital Fabrication Activities
The teachers and the facilitators raised two factors regarding task characteristics of the digital fabrication activities and their influence on the potential for learning of twenty-first century skills and CT practices identified in the two focus group interviews (see Figure 1).
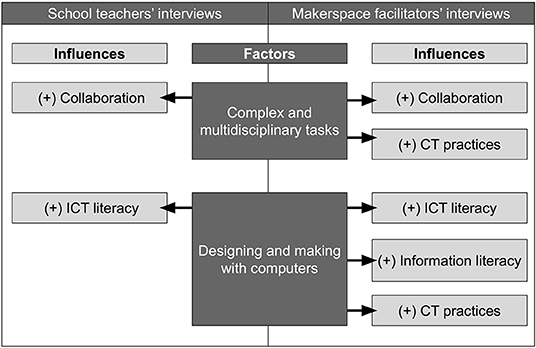
Figure 1. The factors regarding task characteristics of the activities and their positive influence (+) on learning 21st century skills and CT practices.
Factor 1: Complex and multidisciplinary tasks
In the three cases, the projects were complex and required knowledge and skills of multiple subjects, such as mathematics, physics and art. The projects were difficult for them to complete without dividing and sharing the tasks. One teacher reflected as follows:
“One girl said that if you have been sort of normal group activities in school, she would have taken like the whole control, but this one was so huge, and she realized that she couldn't do that. So, she had to delegate. That was precious that she had to, but also about trusting the team and can't control everything.”
In the case of school B, the pupils worked collaboratively dividing the tasks of one project between two groups (See participants and contexts of the study). To work efficiently and integrate the divided tasks, the groups had to communicate and build shared understanding of their project.
In addition, the facilitators emphasized the complex problem-solving positively influenced learning and utilizing CT practices. One facilitator explained:
“Computational thinking it's best applied to little bit larger design problems, really have to divide your work into pieces that you have to solve piece by piece.”
Factor 2: Designing and making with computers
The pupils needed to design on the computers and create the design files with a certain format to use digital fabrication machines. The teachers and the facilitators found using the computers to fabricate artifacts enhanced pupils' ICT skills. One facilitator mentioned:
“I think that ICT literacy is clear. I mean they had to use computers to do all the designs before using, for example, the laser cutter.”
The pupils applied digital technology effectively to their fabrication process.
Another aspect of use of computers during the activity was recognized as a factor to enhance information literacy. The facilitators emphasized searching information is important to get ideas and inspiration for the projects and find the relevant instructions from documentations of other makers' projects in the online platforms. One facilitator said:
“It was especially important during the design phase, when they had to decide what to do, so they were looking for some ideas on the internet, for example.”
Facilitation of the Digital Fabrication Activities
The teachers and the facilitators discussed two factors regarding facilitation of the digital fabrication activities and their influence on the potential for learning of twenty-first century skills and CT practices (see Figure 2).
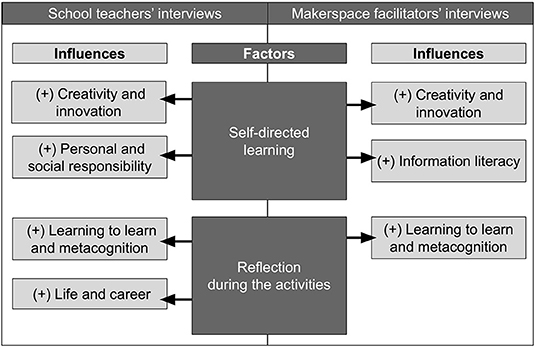
Figure 2. The factors regarding facilitation of the activities and their positive influence (+) on learning 21st century skills and CT practices.
Factor 3: Self-directed learning
The facilitators reflected they tried not to interfere too much in the pupils' work and fabrication processes. They described themselves as “older colleagues, with expertise” and let the pupils work without controlling. The facilitators emphasized the pupils can be creative because they have autonomy in designing and making process. One facilitator illustrated as follows:
“We gave the kids total full freedom for creativity and innovation, they decided by themselves what they want to create, what kind of device they want to make.”
The teachers recognized that the facilitators give the pupils large autonomy during the activities. One teacher reflected as follows:
“One of our students was saying in QRIDI-program2, this evaluation, that it was so nice that teacher keep us responsibility, that they didn't advice every second what to do, just be around and help if they needed.… they knew that they can have a help but they also have time to think themselves and solve the problems themselves and do the different kind of…. make these all the situation they own way.”
Factor 4: Reflection during the digital fabrication activities
School C had the mid-term presentations where the pupils explained the ideas of the projects and the progress of the fabrication process (see Table 2). The teachers and the facilitators found the presentations enhanced pupils' metacognitive skills through reflecting on their learning. In addition, the teachers found the reflective practice enhanced the skills of management of goal, time and project which is one of the skills of life and career (see Table 1). One of the teachers, who witnessed the mid-term presentations, illustrated as follows:
“After the presentation. they had clear focus what to do, what we have to do next and so on. We have a huge to do so much tasks. We have certain time to do that. so they are very focus to do that job.”
Learning Environment and the Structure of the Digital Fabrication Activities
The teachers and the facilitators mentioned to two factors regarding learning environment and the structure of the activities and their influence on the potential for learning of twenty-first century skills and CT practices identified in the two focus group interviews (see Figure 3).
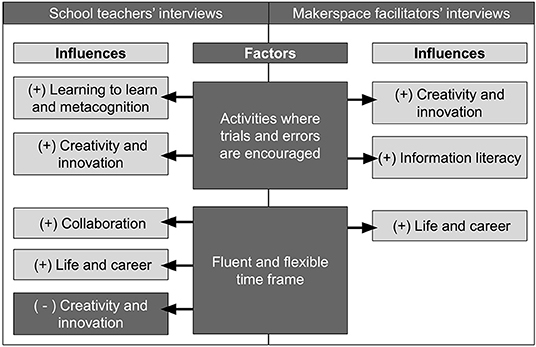
Figure 3. The factors regarding learning environment and the structure of the activities and their positive influence (+) and negative influence (−) on learning 21st century skills and CT practices.
Factor 5: Digital fabrication activities where trials and errors are encouraged
The teachers explained digital fabrication activities as the environment where trials and errors are encouraged. One teacher explained as follows:
“You go and just try and error and it doesn't even matter if you totally succeed or fail on the product.… important thing is what kind of cognitive skills and how you reflect, what you learn in the process, and if you came back, what would you do better.”
The teachers found that with digital tools, it is possible to see the result of a trial instantly. One teacher said:
“The feedback is instant: oh, this doesn't work, doesn't fit.”
In addition, the teachers found repeating trials and errors can increase pupils' confidence in own capability to succeed which is one of the skills regarding metacognition (see Table 1). One teacher described as follows:
“I think it's very beneficial to see that, I think that's one thing that they are missing at school that when you are learning something, it's a process, that you might have figure through kind of the same thing in many different cycles. And with every cycle, you'll get little bit higher, and it's kind of, it's very slow process, and it takes time…. You do it, then you look at it, then you go like ‘Oh, I would have done that better.’ I think that applies to within very generally in our lives.”
Factor 6: Fluent and flexible time frame
Both the teachers and the facilitators found the positive effects of fluent and flexible time frame on pupils' continuous thinking and working process. The pupils focused on one project all time during the activities, thus their thinking was not interrupted by time, as it often happens at schools. One teacher explained:
“One girl said that usually school you do something for maybe two hours and have to change head and they need to do something else.”
However, the teachers recognized limited time for the project also as a factor to possibly hinder pupils' learning and creativity. The teachers indicated a negative aspect of activities without strict time frame. To complete the project in the limited time, the pupils had to rush in the end. One of the teachers explained:
“When you have limited time and you have lots do. and busses come [the time to leave comes], you have stress, and panic, and pressure comes more and more, and then you are not so maybe creative.”
Also, one teacher explained working for a long time without taking a break can hinder creativity as follows:
“If you are just 14 or 15 years old, and you are working two and a half hours, and then one break, and then two and a half hours again. If you want to make some creative, then if you need some break or if you want to go outside and walk a little bit. I think it's nice.”
Moreover, the teachers mentioned the needs for having time to think quietly to be more creative, especially because the makerspace is a lively environment. One teacher illustrated:
“Some of them are maybe be bit more introverted, and when you are in creative process, you might want to have sort of more quiet time.”
Discussion
In this study, we aimed to explore: (1) the potentials and the factors to develop non-subject transversal competences through digital fabrication activities, and (2) the challenges of utilizing digital fabrication in K-12 Maker Education. We focused on twenty-first century skills, which share the elements with Finnish National core Curriculum for basic education, and CT practices, which are important problem-solving skills to be developed in the digitalized society. The results suggest that digital fabrication activities have the potential for learning of the skills covering all four categories of twenty-first century skills and several aspects of CT practices. The teachers and the facilitators discussed the six factors which had influence on the pupils' learning regarding task characteristics, facilitation, and environment and the structure of the digital fabrication activities. However, the potential challenges of utilizing digital fabrication activities in K-12 Maker Education were also uncovered. The results indicate the possibility that the teachers and the facilitators are not fully aware of the concepts of CT. Also, different perspectives between the teachers and the facilitators toward the structure of the digital fabrication activities surfaced.
The Potential for Learning of Twenty-First Century Skills Through Digital Fabrication Activities
The results suggest the digital fabrication activities can be possibly used to foster the skills covering all the four categories of twenty-first century skills defined by Griffin et al. (2012, p. 18–19; see Table 4). Thus, digital fabrication activities may enhance the seven transversal competences described in the Finnish National core curriculum, which share the elements with the definition of the twenty-first century skills. The teachers and the facilitators similarly described the skills in ways of thinking and ways of working, while they emphasized different skills in other two categories.
Based on the results, it can be said that the digital fabrication activities enhanced the pupils' creativity. It supports Martin (2015, p. 32), which illustrates that democratized digital fabrication tools and machines to design and fabricate artifacts allow pupils to be creative by helping them to visualize their imagination and expanding options for production. Exploratory approach, such as the facilitation without giving explicit instructions would foster pupils' creativity and out-of-box thinking. Multidisciplinary digital fabrication activities which require knowledge and skills from various fields may encourage the pupils to find and utilize own strength in a team. In addition, through digital fabrication activities, pupils can strengthen their belief in own capability to succeed. Applying existing expertise in practice and repeating the process of design cycles increase a learner's self-esteem (Blikstein, 2013, p. 7). In the process of digital fabrication where digital tools allow fast prototyping and fasten design cycles including trials and errors, pupils may have opportunities to build confidence in own capability to success.
The results indicate that digital fabrication activities encourage intensive collaboration toward complex problem solving. According to Blikstein (2013, p. 7), pupils can reach deeper level of collaboration through several cycles of failure and redesigning. In this study the pupils needed to work and communicate effectively as a team to complete a complex problem-solving activity. In addition, as sharing is one of the characteristics of maker culture (e.g., Halverson and Sheridan, 2014, p. 496–497; Martin, 2015, p. 36; Papavlasopoulou et al., 2017, p. 57; Vossoughi and Bevan, 2014, p. 25–27), often makers document their work on the online platforms in English to share them worldwide. The pupils needed to search, understand and assess information written in English to apply it to their projects. Necessity of using English may encourage the Finnish speaking pupils to use English actively during the activities and may help developing confidence in additional language.
The teachers and the facilitators emphasized different aspects of twenty-first century skills (see Table 4). The facilitators indicated that the pupils developed information literacy, which is a subcategory of tools for working, by finding, judging, and modifying relevant information on the online platforms to apply to their projects. This result is aligned with Gravel et al. (2018, p. 933–935) that experienced makers are used to identify, organize, and integrate information from text. The facilitators, who are the experienced makers and used to the way of sharing and finding information in maker culture, recognized the important literacy. This kind of way of finding information may not be implemented often at schools, where pupils might be used to wait for information and instructions to be provided by teachers.
On the other hand, the teachers discussed frequently about ways of living in the world. Working style in the makerspace was different from the one in schools. The pupils had more freedom and responsibility to manage time and project. The mid-term presentations helped the pupils to reflect on their goal and remaining tasks to complete their project. In addition, the teachers also mentioned to community and participation regarding the topic of the activity of School C: creating a model of new playhouse in the school (see Table 2). To design the suitable playhouse for their school community, they had to visualize the community's need and the users of the playhouse in detail. This category does not necessary share its elements with nature of digital fabrication, maker culture or constructionist approach. Rather, it shares elements with the transversal competences in Finnish National core curriculum (Finnish National Board of Education, 2016, p. 22–25), with which the teachers are familiar. These skills which are not naturally involved in the process of digital fabrication activities may better to be included as a topic of the activities.
Needs for Recognizing CT
We found the potentials for learning CT practices through digital fabrication activities (see Table 5). The results suggest that digital fabrication activities, where pupils solve complex problems using computers, can develop the aspects of CT practices defined by Barr et al. (2011, p. 21). During the activities the pupils solved the complex problems assisted by the computers. They arranged the problems in the specific formats to utilize digital fabrication tools, such as laser cutters. Also, they needed to decompose the complex fabrication process into small manageable steps. In the process of decomposing, the pupils logically organized the whole process, identified each step and implemented in the most efficient order.
However, the results indicate the possibility that the teachers and the facilitators may not be fully aware of the concepts of CT. The teachers' discussion touched only the surface of CT, such as using computers to solve problems, and CT practices which involve the fundamental concepts of CT, such as abstractions and automation, were not intensively discussed. Overall, compared to twenty-first century skills intensiveness and quantity of description of CT during the teachers' focus group interviews were lower (see Table 5). This result supports Mannila et al. (2014, p. 14), illustrating that very few teachers implement the concepts of abstraction and automation in classroom practice. The teachers might not be familiar enough with the concepts of CT and its practices to implement classroom activities which emphasize CT. The ideas of CT are embedded in Finnish National core curriculum for basic education without naming CT. For instance, key content areas related to the objectives of mathematics in grade 7–9th include: (1) thinking skills and methods, such as logical thinking and discovering rules and dependencies, (2) examining and applying functions, (3) data processing, such as collecting, and structuring, and analyzing data (Finnish National Board of Education, 2016, p. 402–405). Connecting those mentioned skills with problem-solving which applies how computer works may enhance teachers' understanding of holistic views of CT (Denning and Tedre, 2019, p. xi).
On the other hand, the results reveal the possibility that the facilitators had not thoroughly defined the concepts of CT even though, as they described during the interviews, it is a fundamental thinking process for engineers. Because the concepts of CT are already built-in thinking and working methods for them, the facilitators may have not defined CT particularly as something that pupils can learn. Teachers' and facilitators' awareness of the concepts of CT may be essential to provide opportunities for pupils to understand and apply CT practices in digital fabrication activities. Insufficient understanding of CT concepts and practices might diminish the potentials to develop CT in digital fabrication activities.
Teachers' and Facilitators' Conceptions of the Potential Influence of the Current Digital Fabrication Activities on Pupils' Learning of Twenty-First Century Skills and CT Practices
The teachers and the facilitators discussed the six factors which influenced pupils learning in the context of the makerspace. In the following section, we explain to what extent the teachers and the facilitators considered these factors influenced learning. The results indicate that the teachers and the facilitators have similar views toward the current practices of digital fabrication activities. However, the teachers also expressed the concerns about potential negative influence on pupils' learning due to the limited time.
Complex and Multidisciplinary Tasks
Complex problem-solving activities which require knowledge and skills in different fields may enhance collaboration. The pupils needed to divide tasks of the project to complete in a limited time. When a project requires high cognitive load, information need to be divided among group members which has larger capacity of processing information than an individual (Kirschner et al., 2009, p. 35–38). To integrate the divided tasks for making a tangible and physical artifact, pupils need to communicate in detail and build shared understanding. For instance, in the case of School B, one group which took care of designing of the outer part of the artifact had to consider the mechanical part which the other group handled, in order to fit all the part in it (see participants and contexts of the study). The pupils collaborated as a team communicating and building shared understanding to well integrate the divided tasks.
Frequent Use of Computers and Digital Tools
Additionally, the pupils used the computers frequently during the process. They used the computers especially while developing ideas, designing an artifact and finding relevant information which they can use for their project. In digital fabrication, using computers is not a purpose but a method. The pupils used the computers to achieve the project which they were interested in.
Facilitation Designed as Self-Directed Learning
The pupils' freedom during the self-directed activities might encourage them to be creative. In the environments where there was no strict rules and explicit instructions for the procedure, the pupils decided freely and creatively what to make based on their interests. They discussed with the peers and developed ideas through trials and errors. Ways of facilitation, such as providing only hints to provoke the pupils' imagination and introducing the tools to realize the artifacts, might increase the pupils creativity.
Supporting Opportunities of Reflection
Reflection during the activities may positively influence pupils' metacognitive skills. Utilizing reflective practices is beneficial in constructionist approach in order to understand the continuously developing ideas, challenges, and procedures (Vossoughi and Bevan, 2014, p. 35). Reflecting and evaluating own performance may make pupils aware of what they have learned and the remaining steps to reach the goal while engaging in iterative cycles of prototyping where they might fail to follow their cognitive development.
Encouraging Trials and Errors
The teachers indicated repeating trials and errors can help pupils to develop metacognitive skills, especially the skills to confront uncertainty. During the activities the pupils tested possible solutions and making modification based on analysis of the result. One of the notable characteristics of maker culture is “failure positive” (Martin, 2015, p. 35–36; Vossoughi and Bevan, 2014, p. 23–24). In the process of digital fabrication activities, which consists of iterative cycles of experiment, analysis and reflection, pupils may continuously improve the project by analyzing and reflecting on the result each time. In digital fabrication activities, where digital tools help pupils to rapidly iterate the cycle of trials and errors through quick prototyping (Blikstein, 2013, p. 13; Smith et al., 2015, p. 16), pupils may be able to gain confidence in own capability to succeed.
Time Frame Embracing an Appropriate Range of Flexibility
In digital fabrication activities, the pupils focused on one task without being interfered by time. They were responsible on completing their project in a limited time. Compared to secondary schools where most of the classes are implemented in the time span of 45 min or so, in the makerspace the pupils had more chances to immerse themselves in the project and achieve cognitive engagement. Also, at the makerspace, the facilitators did not provide strict time schedule. Thus, the pupils needed to plan how to proceed the project by themselves which may develop their skills of management of time and project.
However, the teachers highlighted possible negative impacts of not providing a strict time frame. The teachers indicated that when pupils are engaged in the activities and there is no time frame, it may fatigue pupils and result in giving negative influences on pupils' performance. Also, they concerned about pupils' stress which would be caused by rushing to complete the project in a short time. Pitkänen et al. (2019, p. 6–7) emphasize that even though freedom and flexibility are regarded as positive characteristics of digital fabrication activities, there are needs for scaffolding pupils' learning.
Limitations and Implications of the Study
Firstly, we are aware that the sample size was small. The number of the participants of the first focus group interview was three and the second was two. In order to avoid identifying individual participants, we limited to describe participants of the focus group interviews in detail. It may affect the trustworthiness of the study. Secondly, we acknowledge the fact that we did not use the data from observations and informal interviews in the analysis phase for triangulation. We used the data from observations and informal interviews as the pilot data of the study to understand the context and to design the main data collection. Based on the pilot data, we were able to design better setup for the focus group interviews, such as formulating significant interview questions about the issues which we found from the pilot data and facilitating effective and fruitful discussion considering participants' background and understanding of the discussion topics. Thirdly, the concepts of CT could have been described in more detailed manner during the focus group interviews. Although we provided an explanation of CT practices during the interviews, there was a possibility that the participants did not fully understood the concepts. We came to know these limitations while participating and developing our understanding toward culture and people in the context throughout this long-term ethnographic study. Although the limitations may affect the results, we consider that these experiences of participation were necessary to interpret data with thorough understanding of the context and the participants' perspectives.
To conclude, digital fabrication activities are potential contexts for developing non-subject transversal competences. The factors, such as complex tasks which require frequent use of computers, student-centered facilitation and ill-structured activities, may enhance learning of twenty-first century skills and CT practices, both of which are important skills to be developed for digitalized and globally connected world. However, there may be challenges in the current practices of digital fabrication activities to be successfully implemented in K-12 education, such as teachers' concerns about flexible time frame and insufficient understanding of CT. Future studies will focus on developing teachers' and facilitators' understanding of CT and designing digital fabrication activities which enhance learning experiences, in order to effectively integrate digital fabrication into K-12 Maker Education.
Data Availability Statement
The datasets generated for this study will not be made publicly available in order to prevent identifying individual participants. Requests to access the datasets should be directed to Megumi Iwata, bWVndW1pLml3YXRhQG91bHUuZmk=.
Ethics Statement
Ethical review and approval was not required for the study on human participants in accordance with the local legislation and institutional requirements. Written informed consent was obtained from the individual participants of the main data collection for the publication of any potentially identifiable images or data included in this article. For the pilot data (observation and informal interviews), written informed consent was not obtained. The pilot data was collected as part of ethnographic study procedure and only used to develop the research design of this study.
Author Contributions
MI and KP contributed to develop research design, performed data collection and data analysis, and developed the first draft of the manuscript under the supervision of JL. All authors contributed to the manuscript revision, read, and approved the submitted version.
Conflict of Interest
The authors declare that the research was conducted in the absence of any commercial or financial relationships that could be construed as a potential conflict of interest.
Acknowledgments
The authors want to thank the teachers and the facilitators who participated in the focus group interviews. Preliminary results of this study were presented at the International Conference on Computational Thinking Education 2019. Additionally, this study was reported in the MI and KP's collaborative master's thesis with two journal article manuscripts, which is currently unpublished in order to protect the copyright of the manuscripts.
Footnotes
1. ^A distributed educational model providing learning opportunities of digital fabrication processes through hands-on experience. (https://fabacademy.org/).
2. ^A formative evaluation and learning analytics software program for comprehensive schools.
References
Andersen, H. V., and Pitkänen, K. (2019). Empowering educators by developing professional practice in digital fabrication and design thinking. Int. J. Child-Computer Interaction 21, 1–16. doi: 10.1016/j.ijcci.2019.03.001
Astrachan, O., and Briggs, A. (2012). The CS principles project. ACM Inroads 3, 38–42. doi: 10.1145/2189835.2189849
Barr, D., Harrison, J., and Conery, L. (2011). Computational thinking: a digital age skill for everyone. Learning Leading Technol. 38, 20–23. Retrieved from: https://id.iste.org/docs/learning-and-leading-docs/march-2011-computational-thinking-ll386.pdf
Blikstein, P. (2013). “Digital fabrication and “making” in education: the democratization of invention,” in FabLabs: Of Machines, Makers and Inventors, eds J. Walter-Herrmann and C. Büching (Bielefeld: Transcript Publishers), 203–222.
Borges, K. S., de Menezes, C. S., and da Cruz Fagundes, L. (2017). “The use of computational thinking in digital fabrication projects a case study from the cognitive perspective,” in 2017 IEEE Frontiers in Education Conference (FIE) (Indianapolis, IN: IEEE), 1–6. doi: 10.1109/FIE.2017.8190654
Brennan, K., and Resnick, M. (2012). “New framework for studying and assessing the development of computational thinking,” in Proceeding of the 2012 Annual Meeting of the American Educational Research Association (Vancouver, BC).
Cohen, J. (1960). A coefficient of agreement for nominal scales. Educ. Psychol. Measurement 20, 37–46. doi: 10.1177/001316446002000104
Finnish National Board of Education (2016). National Core Curriculum for Basic Education 2014. Helsinki: Finnish National Board of Education.
Gershenfeld, N. (2012). How to make almost anything: the digital fabrication revolution. Foreign Aff. 91, 43–57. Retrieved from: http://cba.mit.edu/docs/papers/12.09.FA.pdf
Gravel, B. E., Tucker-Raymond, E., Kohberger, K., and Browne, K. (2018). Navigating worlds of information: STEM literacy practices of experienced makers. Int. J. Technol. Design Educ. 28, 921–938. doi: 10.1007/s10798-017-9422-3
Gretter, S., and Yadav, A. (2016). Computational thinking and media and information literacy: an integrated approach to teaching twenty-first century skills. TechTrends 60, 510–516. doi: 10.1007/s11528-016-0098-4
Griffin, P. E., McGaw, B., and Care, E. (2012). Assessment and Teaching of 21st Century Skills. Dordrecht; New York, NY: Springer. doi: 10.1007/978-94-007-2324-5
Grover, S., and Pea, R. (2013). Computational thinking in K−12: a review of the state of the field. Educ. Res. 42, 38–43. doi: 10.3102/0013189X12463051
Halverson, E. R., and Sheridan, K. (2014). The maker movement in education. Harvard Educ. Rev. 84, 495–504. doi: 10.17763/haer.84.4.34j1g68140382063
Hughes, J., Morrison, L., Kajamaa, A., and Kumpulainen, K. (2019). Makerspaces promoting students' design thinking and collective knowledge creation: examples from Canada and Finland. LNICST 265, 343–352. doi: 10.1007/978-3-030-06134-0_38
Hynes, M. M., and Hynes, W. J. (2018). If you build it, will they come? Student preferences for Makerspace environments in higher education. Int. J. Technol. Design Educ. 28, 867–883. doi: 10.1007/s10798-017-9412-5
Iwata, M., Pitkänen, K., Laru, J., and Mäkitalo, K. (2019). “Developing computational thinking practices through digital fabrication activities,” in Proceedings of International Conference on Computational Thinking Education 2019 (Hong Kong: The Education University of Hong Kong), 223–228.
Kafai, Y., Fields, D., and Searle, K. (2014). Electronic textiles as disruptive designs: supporting and challenging maker activities in schools. Harvard Educ. Rev. 84, 532–556. doi: 10.17763/haer.84.4.46m7372370214783
Kangas, K., Seitamaa-Hakkarainen, P., and Hakkarainen, K. (2013). Design expert's participation in elementary students' collaborative design process. Int. J. Technol. Design Educ. 23, 161–178. doi: 10.1007/s10798-011-9172-6
Katterfeldt, E. (2013). “Maker culture, digital tools and exploration support for fablabs,” in FabLabs: Of Machines, Makers and Inventors, eds J. Walter-Herrmann and C. Büching (Bielefeld: Transcript Publishers), 139–147.
Kirschner, F., Paas, F., and Kirschner, P. A. (2009). A cognitive load approach to collaborative learning: united brains for complex tasks. Educ. Psychol. Rev. 21, 31–42. doi: 10.1007/s10648-008-9095-2
Krueger, R. A., and Casey, M. A. (2000). Focus Groups: A Practical Guide for Applied Research. 3rd ed. Thousand Oaks, CA: Sage.
Laru, J., Vuopala, E., Iwata, M., Pitkänen, K., Sánchez, M. I., Mäntyniemi, A., et al. (2019). “Designing seamless learning activities for school visitors in the context of Fab Lab Oulu,” in Seamless Learning: Perspectives, challenges and opportunities. Lecture Notes in Educational Technology, eds C. K. Looi, L. H. Wong, C. Glahn, and S. Cai (Singapore: Springer), 153–169. doi: 10.1007/978-981-13-3071-1_8
Lewis-Beck, M. S., Bryman, A., and Liao, F. T. (2004). The SAGE Encyclopedia of Social Science Research Methods. Thousand Oaks, CA: Sage Publications, Inc. doi: 10.4135/9781412950589
Mäkitalo, K., Tedre, M., Laru, J., and Valtonen, T. (2019). “Computational thinking in Finnish pre-service teacher education,” in Proceedings of International Conference on Computational Thinking Education 2019 (Hong Kong: The Education University of Hong Kong), 105–107.
Mannila, L., Dagiene, V., Demo, B., Grgurina, N., Mirolo, C., Rolandsson, L., et al. (2014). “Computational thinking in K-9 education,” in Proceedings of the Working Group Reports of the 2014 on Innovation and Technology in Computer Science Education Conference (New York, NY: ACM), 1–29. doi: 10.1145/2713609.2713610
Martin, L. (2015). The promise of the maker movement for education. J. Pre-College Eng. Educ. Res. 5, 30–39. doi: 10.7771/2157-9288.1099
Montero, C. S. (2018). “Facilitating computational thinking through digital fabrication,” in Proceedings of the 18th Koli Calling International Conference on Computing Education Research (New York, NY: ACM), 32. doi: 10.1145/3279720.3279750
Montero, C. S., Voigt, C., and Makitalo, K. (2020). “From digital fabrication to meaningful creations: pedagogical perspectives,” in Educational Robotics in the Context of the Maker Movement. Edurobotics 2018. Advances in Intelligent Systems and Computing, eds M. Moro, D. Alimisis, and L. Iocchi (Cham: Springer), 69–82. doi: 10.1007/978-3-030-18141-3_6
Näykki, P., Laru, J., Vuopala, E., Siklander, P., and Järvelä, S. (2019). Affective learning in digital education—case studies of social networking systems, games for learning, and digital fabrication. Front. Educ. 4:128. doi: 10.3389/feduc.2019.00128
Nemorin, S. (2017). The frustrations of digital fabrication: an auto/ethnographic exploration of ‘3D Making' in school. Int. J. Technol. Design Educ. 27, 517–535. doi: 10.1007/s10798-016-9366-z
Papavlasopoulou, S., Giannakos, M. N., and Jaccheri, L. (2017). Empirical studies on the Maker Movement, a promising approach to learning: a literature review. Entertainment Computing 18, 57–78. doi: 10.1016/j.entcom.2016.09.002
Papert, S., and Harel, I. (1991). “Situating constructionism,” in Constructionism, eds I. Harel and S. Papert (Westport, CT: Ablex Publishing Corporation), 1–11.
Peppler, K., Maltese, A., Keune, A., Chang, S., Regalla, L., and Initiative, M. E. (2015). Survey of Makerspaces, Part II. Open Portfolios Maker Education Initiative. Berkeley, CA: Maker Education Initiative. Retrieved from: https://makered.org/wp-content/uploads/2015/02/OPP_ResearchBrief7_SurveyofMakerspacesPart2_final.pdf
Pitkänen, K., and Iwata, M. (2019). What are the premises and the essential elements of activity design for applying digital fabrication in formal education? (master's thesis). University of Oulu, Oulu, Finland.
Pitkänen, K., Iwata, M., and Laru, J. (2019). “Supporting Fab Lab facilitators to develop pedagogical practices to improve learning in digital fabrication activities,” in Proceedings of Fablearn Europe 2019 Conference, Article No.6 (New York, NY: ACM). doi: 10.1145/3335055.3335061
Rode, J. A., Weibert, A., Marshall, A., Aal, K., von Rekowski, T., El Mimouni, H., et al. (2015). “From computational thinking to computational making,” in Proceedings of the 2015 ACM International Joint Conference on Pervasive and Ubiquitous Computing (New York, NY: ACM), 239–250. doi: 10.1145/2750858.2804261
Smith, R. C., Iversen, O. S., and Hjorth, M. (2015). Design thinking for digital fabrication in education. Int. J. Child-Computer Interaction 5, 20–28. doi: 10.1016/j.ijcci.2015.10.002
Smith, R. C., Iversen, O. S., and Veerasawmy, R. (2016). Impediments to digital fabrication in education: a study of teachers' role in digital fabrication. Int. J. Digital Literacy Digital Competence 7, 33–49. doi: 10.4018/IJDLDC.2016010103
Taylor, B. (2016). Evaluating the benefit of the maker movement in K-12 STEM education. Electronic Int. J. Educ. Arts Sci. 2, 1–22. Retrieved from: http://www.eijeas.com/index.php/EIJEAS/article/view/72/85
Vartiainen, J., and Kumpulainen, K. (2020). “Makerspaces, multiliteracies and early science education: The Finnish approach,” in Enhancing Digital Literacy and Creativity: Makerspaces in the Early Years Routledge, eds A. Blum-Ross, K. Kumpulainen, and J. Marsh (Abingdon: Routledge), 38–52. doi: 10.4324/9780429243264-4
Vossoughi, S., and Bevan, B. (2014). Making and tinkering: a review of the literature. Natl. Res. Council Committee Out School Time STEM, 1–55. Retrieved from: https://www.informalscience.org/making-and-tinkering-review-literature
Wing, J. M. (2008). Computational thinking and thinking about computing. Philos. Trans. Royal Soc. London A 366, 3717–3725. doi: 10.1098/rsta.2008.0118
Yrjönsuuri, V., Kangas, K., Hakkarainen, K., and Seitamaa-Hakkarainen, P. (2019). The roles of material prototyping in collaborative design process at an elementary school. Design Technol. Educ. 24, 141–162. Retrieved from: https://helda.helsinki.fi/handle/10138/304487
Keywords: maker education, digital fabrication, makerspace, twenty-first century skills, computational thinking
Citation: Iwata M, Pitkänen K, Laru J and Mäkitalo K (2020) Exploring Potentials and Challenges to Develop Twenty-First Century Skills and Computational Thinking in K-12 Maker Education. Front. Educ. 5:87. doi: 10.3389/feduc.2020.00087
Received: 24 February 2020; Accepted: 22 May 2020;
Published: 26 June 2020.
Edited by:
Kristiina Kumpulainen, University of Helsinki, FinlandReviewed by:
Jari Kukkonen, University of Eastern Finland, FinlandKim Koh, University of Calgary, Canada
Copyright © 2020 Iwata, Pitkänen, Laru and Mäkitalo. This is an open-access article distributed under the terms of the Creative Commons Attribution License (CC BY). The use, distribution or reproduction in other forums is permitted, provided the original author(s) and the copyright owner(s) are credited and that the original publication in this journal is cited, in accordance with accepted academic practice. No use, distribution or reproduction is permitted which does not comply with these terms.
*Correspondence: Megumi Iwata, bWVndW1pLml3YXRhQG91bHUuZmk=; Kati Pitkänen, a2F0aS5waXRrYW5lbkBzdHVkZW50Lm91bHUuZmk=