- 1Department of Geography, University of Innsbruck, Innsbruck, Austria
- 2alpS GmbH, Innsbruck, Austria
Latest findings of the IPCC highlight the fact that there is an urgent need for climate action on both individual and societal levels, because political regulations and technical advances just would not be enough to counter climate change. Acknowledging young people’s role as present and future decision-makers, their engagement is absolutely imperative in order to achieve Sustainable Development Goal 13, “Climate Action.” Therefore, new methods of teaching and learning are necessary, and they need to encourage transformative learning, which, it is assumed, will lay foundations for transformative engagement. Research in the field of science education credits scientific literacy vision III as fostering transformative learning and engagement. In this study, transdisciplinary education is analyzed as a promising concept that enables exchange of knowledge, experiences, and perspectives between students and scientific partners while they jointly complete research on real-world issues. A quantitative analysis capturing scientific literacy and transformative engagement for climate action of Austrian and German secondary school students (N = 162) is carried out alongside a literature review. This study reveals that the didactical concept of transdisciplinary education notably contributes to the implementation of scientific literacy vision III as well as vision II. According to the results, the three visions of scientific literacy are predictors for transformative engagement for climate action, assuming to be preceded by a transformative learning process. These encouraging findings need to be replicated by further scholars in other contexts.
Introduction
Anthropogenic climate change (CC) is one of the Grand Challenges of the 21st century and the most prominent Planetary Boundary world society has to tackle (Steffen et al., 2015, 3–6; IPCC, 2018, V–VI). Visible CC consequences like increase of extreme weather events, such as drought and extreme heat in North America and Siberia, and heavy rains and flooding in Asia and Europe during summer 2021, demonstrate that CC is not only a future issue (Ciavarella et al., 2020, 21; Schäfer et al., 2021, 14; Philip et al., 2021, 1–2). Increase of human interference with nature has become so intense that the age we live in has been designated the “Anthropocene,” the age of mankind (Crutzen, 2006, 13; Zalasiewicz et al., 2017, 56). Nevertheless, world societies not only share responsibility for the climate crisis but also have a significant role in mitigating and adapting to CC (IPCC, 2018, V; O’Brien, 2018, 154). Young people, in particular, hold an important position, since they are the ones who will be increasingly affected by CC consequences during their lifetime. Their role as decision-makers of today and tomorrow, as well as their active engagement is critical to sustainable development (Corner et al., 2015, 523). In order to “limit global warming to well below 2, preferably to 1.5 degrees Celsius,” the aim of the community of states in the Paris Agreement 2015 (UNFCCC, 2015), political regulations and financial investments, as well as scientific and technological breakthroughs, are necessary, but they alone are not enough (UNFCCC, 2015; Barnosky et al., 2016, 1). To maximize the success of meeting all these requirements, there is an urgent need for change, a transformation within the personal sphere, including beliefs, values, world views, and, more than anything, individual and collective actions for a climate-friendly future (Rockström et al., 2017, 1,269; O’Brien et al., 2018, 155–57).
Recently, there has been yet another call for education to not only produce knowledge but to also raise awareness on today’s challenges and sustainable actions as well as to empower learners to develop key competencies like critical thinking, systems thinking, integrated problem-solving, and collaboration competency, as well as values to engage for sustainable development (WBGU, 2011, 352–58; UNESCO, 2017, 2021, 17). In this context, the role of transformative learning, which can be defined as “learning that transcends habitual thought patterns and behavior through deep learning that changes frames of reference, assumptions, and habits of mind” (Klein, 2018, 13) or, in Mezirow’s words, learning “that transforms problematic frames of reference—sets of fixed assumptions and expectations […]—to make them more inclusive, discriminating, open, reflective, and emotionally able to change” is discussed (Mezirow, 2003, 58). Consequently, this kind of learning is considered to be a powerful tool to create change and to promote individuals’ transformative engagement (Mitchell et al., 2015, 88 and 92–93; Klein, 2018, 21). Transformative engagement, in this study, is understood in a more comprehensive understanding of Barnes et al.’s (2020, 824) definition of transformative and Grabau and Ma’s (2017, 1,046–47) definition of science engagement, as being a fundamental change in individuals’ cognitive, emotional and behavioral characteristics encouraging alteration of social-ecological relationships toward a climate-friendly future.
Sustainable Development Goal (SDG) 4, “Quality Education,” plays a significant role in fostering this type of learning for achieving SDG 13, “Climate Action” (Boeren, 2019, 279; UN, 2021, 27). In this regard, the potential of science education with its major goal of raising scientific literacy (SL) has been thoroughly discussed (Laugksch, 2000, 84; Yacoubian, 2018, 308–9). However, can teacher-centered, knowledge-based science education really fulfill these challenges? A growing body of literature claims that traditional pathways of teaching have to be rethought in order to foster transformative learning and engagement, enabling participation, integration, and reflection (Singer-Brodowski, 2016, 15–16; Balsiger et al., 2017, 359; Hindley and Wall, 2018, 263–66; Kyle, 2020, 1; Leichenko and O’Brien, 2020, 1). Leichenko et al. (2021, 1), for example, stated that most climate change education (CCE) programs pay attention to the physical dimension of CC and evaluation of political, technological, and behavioral solutions without practically supporting students to adopt and sustain their active role in the necessary transformation. Kyle (2020, 4–5) argued that students frequently experience a kind of science education disassociated from real-world topics. He suggested that science education should empower students to actively deal with societal issues in their lifeworld. Moreover, many approaches fail to consider the relevance of emotions, values, and worldviews, which are important in provoking personal change (Leichenko et al., 2021, 2–3) [for more details, see Verlie et al. (2021) and O’Brien and Sygna (2013)]. In a literature review about effective CCE strategies, Monroe et al. (2019, 804–6) pointed out the importance of personal meaningfulness and engaging teaching methods by means of field trips, community action projects, or collaboration with scientists or affected persons. These claims were also addressed by the concept of SL vision III (Sjöström and Eilks, 2018, 67; Valladares, 2021, 581–82).
Moving beyond traditional pathways of (teaching and) learning, we argue that transdisciplinary education (TE) is a promising concept in science education, enabling students to perform research together with out-of-school (scientific) partners in real-world learning settings (Kubisch et al., 2021b, 3–5). TE is assumed to raise students’ SL and transformative learning, foster personal meaningfulness and generate competencies via active engagement and exchange of experience as well as different perspectives and forms of knowledge (Thomas, 2009, 245; Mitchell et al., 2015, 86), and, finally, contribute to a climate-friendly future by transformative engagement (Kubisch et al., 2021b, 1). TE should not be considered as an isolated approach but rather a frame for active and constructivist learning approaches like inquiry-based learning (Riemeier, 2007; Pedaste et al., 2015).
The objective of this publication is fourfold:
(1) To provide an insight into the scholarly discussion about science education and SL for transformative engagement for climate action.
(2) To point out the relevance of TE by means of a literature review and good practice examples, addressing demands on science education in the field of CC.
(3) To quantitatively evaluate the change of predictive quality of the three dimensions of SL (vision I to III) on transformative engagement for climate action and assessing the single contribution of the visions (via pre-post comparison of secondary school students’ answers in a TE project).
(4) To derive from the findings how and in which form TE can contribute to scientific literacy and transformative learning for transformative engagement for climate action.
The study is structured as follows: first, we give a brief insight into the role of science education, its limitations and visions, and significance of student’s scientific literacy; this is followed by a literature review and good practice examples of transdisciplinarity (in education), and is continued by study design and results. We finish with discussion and conclusion.
Visions and Limitations of Today’s Science Education and the Role of Scientific Literacy
In contrast with the broad consensus among scientists that anthropogenic CC is happening and that there is an urgent need for more actions (IPCC, 2018, V–VI), doubts about and denial of CC still exist in societies (Maibach et al., 2014, 295; Corner et al., 2015, 529; Jylhä, 2018, 487; Hahnel et al., 2020, 16). Since awareness of CC causes and consequences is critical for engagement and action (Poortinga et al., 2019, 25), there is still lack of individual and collective engagements (Maibach et al., 2014, 295–96; Jylhä, 2018, 487). Literature reviews of young peoples’ CC awareness, knowledge, and action found that vague knowledge and concepts, as well as misconceptions about CC causes, impacts, and solutions persist among young people aged between 8 and 19 years (Corner et al., 2015, 529; Lee et al., 2020, 7–9). According to Lee et al. (2020, 7–9), scientifically accurate knowledge of the causes of CC tended to increase with age. The need for climate protection was proposed without the acknowledgment of personal responsibility or awareness of time lags of the usefulness of measures (Lee et al., 2020, 7–9). These findings are in contrast to the ongoing Fridays For Future movements (Hagedorn et al., 2019, 79) and general high levels of CC concern among young adults (Corner et al., 2015, 529).
Against the backdrop of findings pointing at various possible manifestations of young people’s knowledge, awareness, and action, the importance of science education becomes apparent. Liu (2013, 26) states, that formerly mentioned challenges are also reflected in school, culminating in students’ disinterest in science and decreasing interest in science careers. According to an international group of scholars, school science is disconnected from reality, failing to address the role of science in students’ everyday lives (Linder et al., 2007, 2–3). Moreover, relevant topics like CC and the role of science for social, economic, and political actions are neglected. Consequently, recent calls from scholars state the need for transformative science education– moving away from simply teaching and learning disciplinary knowledge to “science-in-context” (Bencze and Alsop, 2014, 827–29). This is captured by the concept of socioscientific issues (SSIs) (Zeidler et al., 2005, 357; Zeidler et al., 2019, 1), contextualizing science knowledge and connecting science knowledge to issues of social significance (Bencze and Alsop, 2014, 833). This kind of science teaching and learning tends “to mold sustainable development education by developing general educational skills in the area of an individual’s actions as a responsible member of society” (Eilks, 2015, 154–55). Thus, SSIs are part of an educational philosophy that is built on moral intelligence and social responsibility (Bencze and Alsop, 2014, 833). Moral intelligence and social responsibility also reflect in Valladares’s (2021, 582) requests of a type of science education “that broadens the agency capacity of individuals and communities to take advantage of science in the generation of adaptative, resilient, and sustainable responses to unpredictable changes of today.” Valladares (2021, 581) further states that in order to achieve transformative science education, students need to experience education that allows them to “understand, value, and relate to the world differently in their everyday lives, not only through canonic scientific ideas, but also through a dialog exchange of different perspectives (1), of alternative forms of knowledge (2) and by fostering student’s engagement with science and with their community and culture (3).”
This notion is captured by the concept of SL, which is considered to be one of the major goals of science education, which is backed by scholars of science education, science curricula, and policy documents, as it is considered to be equally beneficial for both individuals and societies as a whole (Laugksch, 2000, 84; OECD, 2017, 21; Yacoubian, 2018, 308–9). According to science educationists, a scientifically literate person should be able to make informed decisions that are based on scientific knowledge, which requires factual and procedural knowledge of science. Scientifically literate people know about the benefits of science while simultaneously taking a critical perspective on science (Norris and Phillips, 2003, 225; OECD, 2017, 21; Yacoubian, 2018, 308). In particular, SL vision III, which can be designated as science for transformation (Sjöström and Eilks, 2018, 78) or critical SL (Hodson, 2011) in Sjöström and Eilks (2018, 66), touches on this idea, targeting to encourage critical engagement with science (Liu, 2013, 25) and sociopolitical actions (Hodson, 2003, 645). While aiming to contribute to critical citizenship and sustainability (Sjöström and Eilks, 2018, 78), vision III is closely associated with transformative learning (Mezirow, 2003, 58–59) and transdisciplinarity, fostering student’s dialogical emancipation and participation (Sjöström and Eilks, 2018, 77; Valladares, 2021, 557). According to Simonneaux (2014, 51), critical reflexivity, participation, and action in scientific activities are significant for transformative science education. According to Choi et al. (2011, 681), a concept of SL for the 21st century is required, which includes competencies, which echo the competencies demanded by Education for Sustainable Development (ESD) (UNESCO, 2017, 10). Wiek et al. (2011, 204) propose a definition of competencies contributing to sustainability as “complexes of knowledge, skills, and attitudes that enable successful task performance and problem solving with respect to real-world sustainability problems, challenges, and opportunities.” Besides SL vision III, their definition also highlights the importance of visions I and II of SL. While vision I is focused on knowledge of scientific content and processes, vision II creates a link to students’ lifeworld, focusing on the relevance of scientific knowledge and processes in society (Roberts, 2007, 2011). Consequently, vision I, “within science,” is focused on pure science learners aiming to develop mental capacity and preparing for a science career, whereas vision II targets bridging science and society. Meanwhile, vision III, “science within society,” aims at encouraging students to engage in complex SSIs for seeking informed and responsible solutions (Liu, 2013, 29). Liu (2013, 31) argues that “focusing on one vision while ignoring others is undesirable” (Liu, 2013, 31), since the three visions of SL are interdependent and reinforce each other. Balanced didactical implementation of the three visions of SL might also face challenges similar to those encompassed in the concept of ESD. ESD is now an educationally well-justified concept (Burmeister and Eilks, 2012, 93–94; Burmeister et al., 2013, 169), however, its implementation in formal school education still remains rare (Eilks, 2015, 156). Despite the demand for competencies, educating responsible and critical citizens, which is significant for achieving SL vision III, teaching and learning, in many cases, still remains content-driven (Eilks, 2015, 156). According to Burmeister et al. (2013, 173–74), reasons for this are lack of teacher’s knowledge of the ESD philosophy and pedagogy and limited resources. However, Sjöström and Rydberg (2018, 9) point out possible didactical dilemmas that might arise if teachers aim to balance knowledge transfer, competency generation, and students’ sociopolitical participation. This might also be true for balanced implementation of the three visions of SL.
As a consequence of the reflections of expanding SL to vision III, the vision of knowing-in-action (Aikenhead, 2007, 68), subject teaching, and learning will need to transcend school boundaries and open up students’ lifeworld, acknowledging the importance of transdisciplinary perspectives and fostering active engagement with SSIs (Hodson, 2003, 666; Sjöström and Eilks, 2018, 77; Zeidler et al., 2019, 1) while simultaneously considering possible didactical dilemmas (Sjöström and Rydberg, 2018, 9).
Transdisciplinarity in Education: Literature Review and Good Practice Examples
Literature Review and Good Practice Examples of Transdisciplinarity
In this section, the aims, procedures, and further outcomes of transdisciplinary collaborations are discussed. Firstly focusing on transdisciplinarity in science education, Sjöström and Rydberg (2018, 5 and 10) define transdisciplinary Didaktik as partnerships between teachers of different school subjects, who teach about SSI. Further studies on formal school education that discuss transdisciplinarity share a similar definition (e.g., Nordén, 2018, 666–67). In this study, we go beyond this understanding, arguing that transdisciplinarity in school education is rare (Kubisch et al., 2021b, 2); focusing predominantly on higher education [e.g., Clarke and Ashhurst (2018); Beecroft (2019), Pohl et al. (2018), and Clark and Button (2011)], we draw on literature discussing transdisciplinary research and transdisciplinarity in higher education. However, before we clarify how transdisciplinarity and TE are understood in this study, we will explore Lang et al.’s (2012) understanding of transdisciplinary research in the realm of sustainability science and how we share these views.
Transdisciplinary research involves high levels of integration and reflection. Partners from science and societies work together tackling socially relevant scientific issues like CC. Societal partners tend to be those who are affected by a problem and who have different experiences and expertise on the issue. The process of dealing with these challenges is based on scientific research processes and draws on both diverse interdisciplinary methods and mutual exchange of knowledge and experiences, aims, interests, and visions of science and society (Lang et al., 2012, 28–35). All partners are continuously involved in the scientific knowledge production process, co-producing knowledge for sustainable solutions as well as generating societal (e.g., community well-being) and scientific effects (e.g., new scientific insights) (Walter et al., 2007, 326–28). Aligning with transdisciplinary research, an SSI is the starting point of TE, being addressed in a dialogical manner between students and diverse partners from science and outside academia exchanging scientific and everyday life knowledge and experience (Schmohl and Philipp, 2021, 14; Kubisch et al., 2021b, 3–4). This exchange enables mutual learning among all those involved (Clark and Button, 2011, 50; Mitchell et al., 2015, 93). “Learning in this sense is a process that collaboratively generates new rich insights that remain undetectable from a single disciplinary or purpose-less (in Jantsch’s terms) perspective” (Mitchell et al., 2015, 93). In addition to improving students’ research skills (Currie et al., 2005, 405), knowledge production is one of the outcomes of transdisciplinary collaboration. According to the ProClim (1997, 15) report, three kinds of knowledge are required to foster sustainable development, i.e., system knowledge (knowledge of structures and processes and the current status quo), target knowledge (visions and goals of society), and transformation knowledge (knowledge of how to achieve targets) (ProClim, 1997, 15). The design of TE study programs [e.g., by Pearce et al. (2018)] aims to enable students to acquire these types of knowledge. Drawing on a conceptual assessment and the experience of the authors of that study, active involvement in scientific processes and collaboration with scientists and societal partners are assumed to promote these kinds of knowledge and generate diverse kinds of competencies (Pearce et al., 2018, 168–171 and 179). Building competencies and environmental awareness are also strengths of TE. Clark and Button (2011, 48) point out the development of critical thinking competency, and Young et al. (2015, 72) name communicative competency and environmental awareness, linking their TE approach science, art, and community. The development of these competencies ascribed to TE is in line with the demands of SDG 4, “Quality Education” for competency generation according to the goals of ESD (UNESCO, 2017, 10). Furthermore, Vilsmaier and Lang (2015, 51) draw attention to the increase of reflective thinking by TE. “Thereby students learn how to change perspectives and create mutual understanding […]. The exploration of the otherness of the other further enhances the understanding of their own perspective, knowledge […] as well as of their social background. This exploration also allows for reflection of the students’ own values, interests, objectives and culturality” (Vilsmaier and Lang, 2015, 51). Consequently, TE creates an environment of deep reflection, which, in turn, facilitates conceptual change of misconceptions and restructures existing mental models (Mitchell et al., 2015, 93; Thomas and Kirby, 2020, 223). According to Mitchell et al. (2015, 93) and Klein (2018, 16 and 20–21), this kind of transformative learning enables reflection and evolution of thought patterns, norms, values, and behaviors, which promote change toward sustainability. This is confirmed by Walter et al. (2007, 333–34) by demonstrating that transdisciplinary collaboration not only fosters the decision-making capacity of partners involved but also supports the generation of transformational knowledge, which is acknowledged to be relevant for action.
Returning to the argument mooted at the beginning of this chapter, namely, that transdisciplinarity in formal school education is rare (Kubisch et al., 2021b, 2), we would finally like to refer to research in the field of place-based SSI approaches. According to Semken and Butler Freeman (2008, 1,043–44), these approaches variously address transdisciplinary knowledge exchange between students and various out-of-school partners (e.g., researchers and community members). Herman et al. (2019, 347–57) demonstrate that students’ explanations of an SSI became more discriminating and representative of complex interactions within a system during a place-based intervention, which fostered the exchange of different perspectives with out-of-school partners (Herman et al., 2019, 347–57). Beyond this study, Zeidler et al. (2019, 7) argue that place-based SSI approaches are proven to be effective even if topics to be discussed are detached from student’s lifeworld.
Example of SEAS and k.i.d.Z.21: A Good Practice Example
The EU funded project Science Education for Action and Engagement toward Sustainability (SEAS) compares teaching and learning approaches within so-called open schooling networks in different European countries (Austria, Belgium, Estonia, Italy, Norway, and Sweden). Building on a transdisciplinary approach, schools opened to their environment, allowing students and teachers to collaborate with diverse out-of-school partners on finding solutions for real-world sustainability issues within their community. The aim of SEAS is to increase students’ SL and to trigger transformative engagement in order to contribute to a sustainable present and future (Kubisch et al., 2021b, 9).
The Austrian research education collaboration on CC, k.i.d.Z.21—competent toward the future, is an exemplary representation of a successful TE project within the project SEAS (see, e.g., Keller et al., 2019; Deisenrieder et al., 2020; Kubisch et al., 2021a). k.i.d.Z.21 was founded in the year 2012 with the purpose of increasing young people’s awareness of CC and its consequences to generate acceptance of necessary socio-ecological transformation and prepare students not just for today’s but also future challenges. Over the course of one school year, Austrian and German students aged between 13 and 16 years deal with the topic of CC in different inter-and transdisciplinary modules (Oberrauch et al., 2015, 19–26; Stötter et al., 2016, 214–15). Since the beginning of k.i.d.Z.21, more than 3,500 students and 100 scientific partners have worked in collaboration. Figure 1 shows the modules of k.i.d.Z.21 during one school year. These are based on didactical approaches of moderate constructivism and inquiry-based learning, and are embedded in an inter- and transdisciplinary setting (red framed modules in Figure 1) (Oberrauch et al., 2015, 19–26; Kubisch et al., 2021b, 5–6).
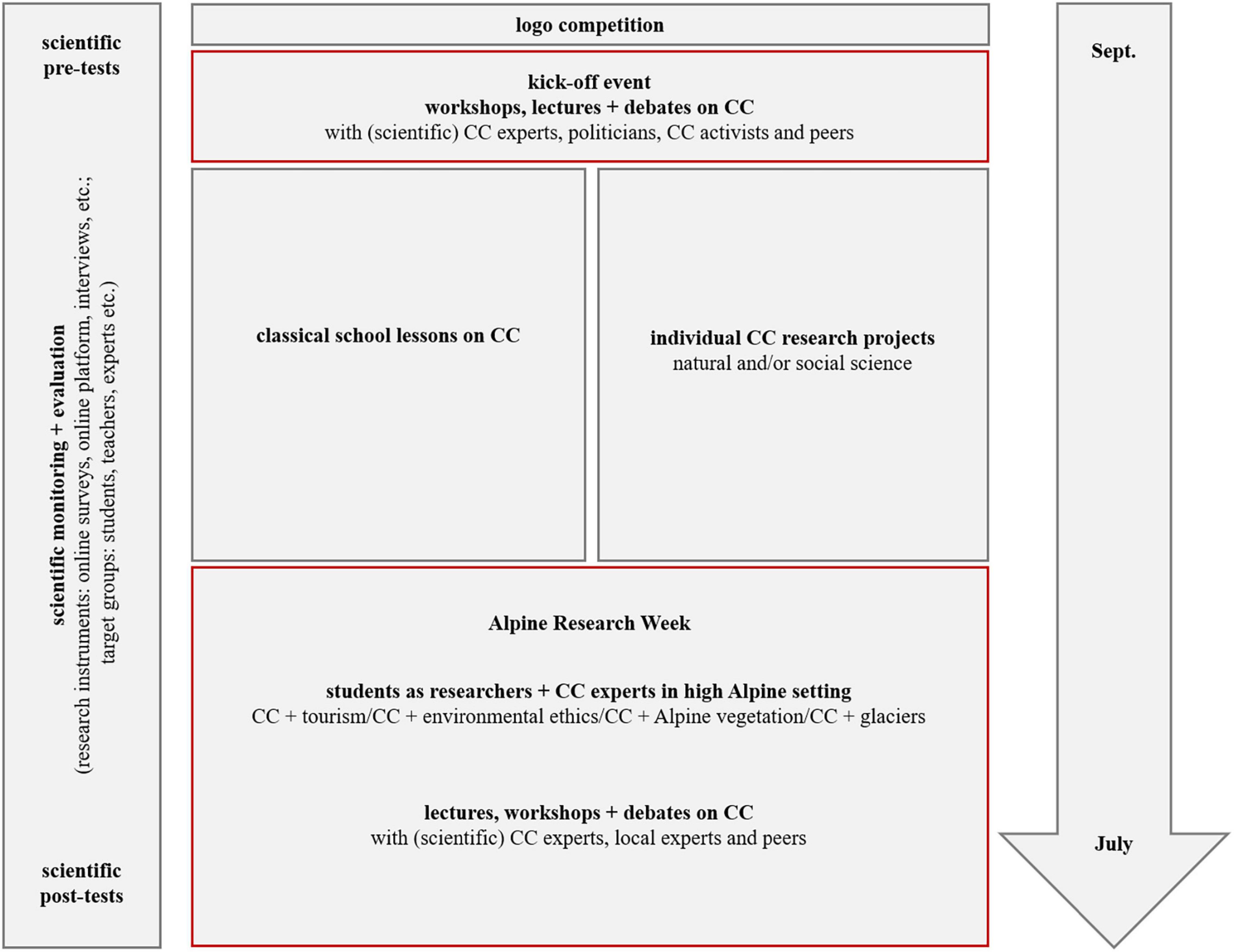
Figure 1. Modules of the Austrian transdisciplinary education (TE) project k.i.d.Z.21, with the red-framed modules as transdisciplinary modules [source: modified according to Kubisch et al. (2021b, 6)].
The moderate constructivist understanding of learning originates from the idea that learning is an active, situational, emotional, social, and self-regulated process. Therefore, learning is considered as a constructive process, which builds on available conceptions originating from individual experiences (Riemeier, 2007, 69–70). In order to facilitate individual knowledge construction processes, students need both the space to follow their interests and to tie up with their preconceptions and experiences (Widodo and Reinders, 2004, 237–38), which are implemented with inquiry-based learning modules. Inquiry-based learning enables students to identify problems, build hypotheses, formulate their own research questions, collect data, and construct answers to their individual questions. From a pedagogical point of view, students are guided through this complex scientific process, which is didactically reduced. Research studies in the field of inquiry-based learning demonstrate its potential in comparison to traditional teaching styles of direct instruction (Pedaste et al., 2015, 48). Furtak et al. (2012, 315–16) indicate a positive effect of inquiry-based teaching on students’ learning in a meta-analysis, while Pedaste and Sarapuu (2006, 48) show the application and generation of problem-solving competencies during inquiry processes. Furthermore, the European Commission acknowledged inquiry-based learning as an important and effective learning approach to build a scientifically literate society (European Commission, 2007, 2). The expansion of SL and a higher learning effect are also proven by studies in the field of moderate constructivist teaching and learning (Widodo and Reinders, 2004, 233).
The moderate constructivist approach of learning cuts across all k.i.d.Z.21 modules, while the inquiry-based learning approach is applied in the individual CC research projects module and the Alpine Research Weeks. The k.i.d.Z.21 project begins with a logo competition at the start of a school year. Based around the area of arts education, students deal with CC for the first time and capture their association with CC in a logo, which accompanies them through the full-year collaboration. The starting point of the transdisciplinary collaboration is the subsequent kick-off event. In this module, students and teachers start a dialog with scientific experts, politicians, and peers to discuss climate change, its consequences, and possible solutions in different fields of everyday life. Besides lectures about CC, students pass through different workshops where, for example, they discuss different keywords such as “youth engagement,” “sustainability,” and “my city” at the global and local levels in the context of CC with scientific experts, politicians, and peers. Consequently, students’ pre-concepts of and experiences with CC are captured and included in the discussions. During the school year, students have classical school lessons on CC in different subjects (e.g., in musical education and mathematics education). This module is guided by participating teachers themselves, connecting and expanding the learning objectives of their subject with the topic of CC. Moreover, students generate their own questions about CC and individually develop CC research projects that are presented at the end of the school year. Based on the approach of inquiry-based learning and a moderate constructivist understanding of learning, students have total freedom to design their projects. They are able to follow their interests in generating a research question and are free in collecting and analyzing the data as well as in the way they present their findings. They are supported by their teacher, whenever necessary. Students have developed innovative research projects in the past. For example, some students measured the temperature over a period of time in the village in which they lived and compared it to the location of their school within the city. Others analyzed the travel behavior of classmates and teachers and developed an initiative, motivating them to act climate-friendly by going to school by bike. During the inter- and transdisciplinary Alpine Research Week, students and scientific experts meet in a high Alpine Mountain area to do research on CC in different fields (e.g., tourism, environmental ethics, glacier, and Alpine vegetation) in a real-world learning setting. According to the moderate constructivist approach to learning, students are only guided by necessary instructions and have the opportunity to develop their own research questions and hypotheses, collect data, and present their findings in groups. Scientific experts and the k.i.d.Z.21 team take the role of coaches: they support the students whenever necessary in their research process and discuss and interact with them as equal partners (Kubisch et al., 2021b, 5–6). The tourism module, for example, starts with capturing students’ pre-concepts about CC and tourism in a concept map. The concept map is continuously expanded during the module. After capturing first associations of CC, students and scientific experts go to an elevation and discuss the change of the city and landscape, comparing a photograph of the past with the reality in front of them. From this discussion, they develop their own research questions in small groups and do research, collecting and analyzing data to finally report their findings in a presentation. Ultimately, the entire group meets again to present and discuss each groups’ findings. The module finishes with an expansion of the concept maps. The other modules also offer pre-defined tasks (e.g., an albedo experiment in the glacier module and emotional mapping in the environmental ethic module), and students have the opportunity to conduct research on climate change based on their individual research questions.
The collaboration with participating teachers, who are representatives for their respective schools, starts with a teacher training course, which is offered once a year. During the one-week training, the teachers are introduced to the didactical concept and the modules of k.i.d.Z.21, and organizational tasks are discussed. Collaboration with schools is usually through one contact person, namely, one teacher who takes part in the teacher training. This person is responsible for cascading the objectives of the project, in-school modules, and tasks involved to other subject teachers who will be delivering them in their respective lessons, e.g., the module logo competition or classical school lessons on CC. In the past, it has been seen that many teachers eventually chose to take part in the teacher training themselves. The transdisciplinary modules are guided by the k.i.d.Z.21 team and scientific experts who voluntarily support the project.
The didactical approach of k.i.d.Z.21 appears to be very effective with regard to both learning outcome and potential to increase an environmental-friendly behavior. It also meets the demands of Agenda 2030 and ESD (UN, 2015, 21; UNESCO, 2017, 10). At the same time, it embodies the same claims as transformative science education, being participative, engaging, and allowing the exchange of different expertise (Valladares, 2021, 581). This is reinforced by the findings of accompanying scientific evaluation of k.i.d.Z.21 so far. Keller et al. (2019) demonstrate that during the module with the highest degree of transdisciplinarity and active involvement in an inquiry-process, the Alpine Research Week, students learned the most about CC and its consequences, in second place were the individual CC research projects and in third place the kick-off event. Classical school lessons obtained the lowest results. Moreover, students felt better prepared to face the challenges of CC after taking part in k.i.d.Z.21 (Keller et al., 2019, 40–42; Kubisch et al., 2021a, 6–8). The latter may be explained by the simultaneous increase in knowledge of CC, its causes and consequences, and how to act climate-friendly, as unpublished findings in the research education-collaboration demonstrate. Analyzing pre- and post-changes in knowledge, attitudes, personal concern, climate-friendly behavior, and multiplicative action among different types of awareness, Kuthe et al. (2019, 382–83) show that by means of diverse didactical concepts of k.i.d.Z.21, weaknesses in students’ climate literacy could be addressed. Deisenrieder et al. (2020, 8–11) and Kubisch et al. (2021a, 6–8) indicate that in addition to self-efficacy and future concern, students’ climate-friendly behavior in different dimensions (everyday behavior, information seeking, and dissemination as well as engagement) could be significantly raised during the course of one school year. Furthermore, the findings of Kubisch et al. (2021a, 6–8) show that the collaboration during the Alpine Research Week resulted in a mutual learning effect among students, teachers, and scientific experts, as opposed to a one-dimensional knowledge transfer. Both teachers and students prefer more frequent collaboration with scientific experts when working on the solution of real-world problems. Additionally, statistical analysis by means of SPSS IBM Statistic 26 of unpublished findings of a standardized questionnaire in the TE project k.i.d.Z.21 demonstrates an increase in students’ understanding of scientific processes, how to argue scientifically, and how to reflect on problems from different perspectives. Students acquired an understanding of why science is important in everyday life and how to apply scientific knowledge in everyday life after collaborating with scientific experts in the Alpine Research Week in 2021.
These findings show the enormous potential that the didactical concept of k.i.d.Z.21 has to contribute to SL and transformative engagement, combining the moderate constructivist approach and inquiry-based learning in inter- and transdisciplinary settings.
Study Design and Method
The study was carried out in the project SEAS with N = 162 secondary school students from Austria and Germany, aged between 13 and 16 years who were part of the research-education cooperation k.i.d.Z.21. In total, 27.8% of the students were female, while 31.6% of the students were male; 2.3% were diverse; 38.4% of the students preferred not to answer the question. The students were consulted by means of a standardized online questionnaire before (pre-test) and after (post-test) being part of the TE project. The collaboration started at the beginning of the school year in September 2020 and ended in July 2021. Besides SL (visions I to III), students’ climate-friendly behavior, their CC concern, their feeling of responsibility, and their locus of control regarding CC were queried (see Table 1). These items were derived and modified in a further k.i.d.Z.21 standardized questionnaire. The questionnaire has been applied since the beginning of the project in 2012. The items of SL were derived and modified via the following assessment instruments (Pugh et al., 2010; Reeve and Tseng, 2011; Heddy and Sinatra, 2017; Patall et al., 2018) and co-developed by SEAS partners. All the questions were closed questions using a five-point assessment Likert scale, in which the dimensions ranged from “I disagree” to “I agree.” The standardized questionnaire asked for students’ self-assessment, possibly biasing the data, which can be declared as a limitation of this study. The reliability of the questionnaire was tested with Austrian secondary school students independently of the TE project.
The statistical relationship between students’ SL and their transformative engagement for climate action was analyzed before and after participating in the TE project k.i.d.Z.21. According to the definition in the introduction, transformative engagement for climate action consists of students’ awareness of CC and the need to act, their feeling of responsibility, their locus of control, and their climate-friendly behavior (see Table 1). Subsequently, the single contribution of the three visions of SL to transformative engagement was assessed in more detail.
The data were analyzed using SPSS IBM Statistics 26. In order to distinguish the three dimensions of SL a factor analysis, more specifically, a principal component analysis with varimax rotation was used (Sedlmeier and Renkewitz, 2013, 684). An analysis of scale reliability (SR) by means of Cronbach’s alpha ensures that the constructs analyzed are well represented by the single items. According to Kuckartz et al. (2013, 247), values for SR are interpreted to be acceptable if SR > 0.7. In order to assess the predictive quality of the three visions of SL for transformative engagement for climate action, a regression analysis was conducted. Both pre- and post-test data were analyzed by multiple regression analysis in order to detect changes in the predictive power of SL before and after taking part in the TE project. Variance inflation factor (VIF) was calculated to prevent multicollinearity of the predictors, and values for VIF < 10 were acknowledged as acceptable (Kuckartz et al., 2013, 271–72). The effect size of the adjusted R2 was calculated to indicate the practical importance of the results beyond statistical significance (p < 0.05). A low effect size is defined for values between 0.1 ≤ f < 0.25, a medium one for 0.25 ≤ f < 0.40, and a high effect size for f > 0.4 (Cohen, 1988, 410–14). A test for normal distribution was waived, since the sample size is sufficiently high, preventing major effects on the results of the analysis (Sedlmeier and Renkewitz, 2013, 325).
Results
Preparation of the Data
Before beginning the main evaluation of the data, a principal component analysis was conducted comprising the SL items. The results opt for a three-factorial solution, resolving 61.53% of the total variance. The items, being allocated to the respective factor, substantively fit the definition of the SL visions. Items belonging to SL visions I and II queried students’ concepts about science and scientific research; however, vision II was focused more on scientific processes and linked those to student’s lives. SL vision III was focused on students’ practical and transformative engagement with science inside and outside the classroom (see section “Visions and Limitations of Today’s Science Education and Role of Scientific Literacy”). Cronbach’s alpha was calculated for the three factors of SL and for transformative engagement for climate action to guarantee the reliability of the factors (for more information, see Table 1).
Multiple Regression Analysis Pre- and Post-tests
A multiple regression analysis was performed to analyze the predictive quality of the model. The pre- and post-test comparisons of the multiple regression aimed to show the change in predictive quality of SL on transformative engagement before and after participation in the TE project. The three visions of SL were treated as predictors, while transformative engagement for climate action was treated as criterion variable. In order to adequately determine the contribution of the TE project, change in the predictive quality of the SL model was analyzed. The status quo of students’ SL visions both at the beginning of the school year (pre-test data) and at the end of the school year (post-test data) were used to predict students’ transformative engagement for climate action at the end of the school year (post-test data).
Scientific literacy (SL) visions I to III were able to statistically significantly predict transformative engagement both in the pre-test [F(3, 73) = 5.777, p < 0.01] and the post-test [F(3, 168) = 50.86, p < 0.01]. The findings revealed that the predictive quality of the model increased after the project (adjusted R2pre–test < adjusted R2post–test), explaining more of the variance of transformative engagement. While the pre-test model explained 16.4% of the variance, the post-test model explained 47.1% of the variance of transformative engagement. In the pre-test, considering the standardized ß coefficient, only SL vision I had a significant influence on transformative engagement (ß = 0.413, p < 0.01), whereas in the post-test model all the three visions significantly predicted transformative engagement. The standardized ß coefficient of SL vision I, however, decreased in the post-evaluation (ß = 0.152, p < 0.05), also in comparison to the standardized coefficients of SL vision II (ß = 0.311, p < 0.01) and III (ß = 0.34, p < 0.01) in the post-evaluation. Consequently, in the post-evaluation, SL vision III had a higher influence on transformative engagement for climate action than vision I and a slightly higher influence than vision II (see Table 2). Referring to Cohen (1988), the effect sizes of both the pre-test model (f = 0.442) and the post-test model (f = 0.944) were high.
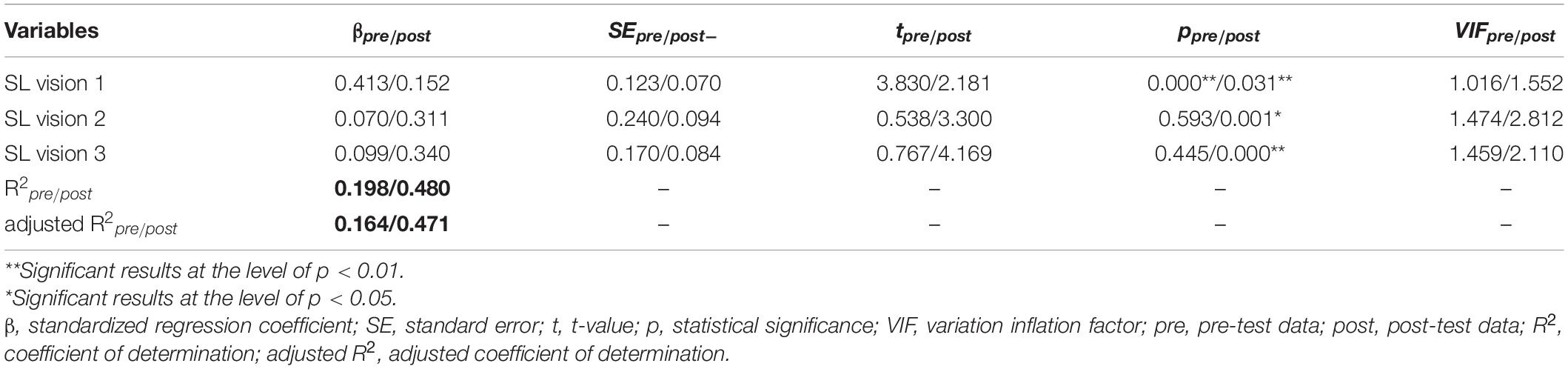
Table 2. Pre-post comparison regression analysis—scientific literacy for transformative engagement for climate action.
Discussion and Recommendations: TE for Transformative Science Education
The concept of SL is thoroughly discussed by scholars (Norris and Phillips, 2003, 225), being defined as a major goal of science education (Yacoubian, 2018, 308) and as a prerequisite for a scientifically literate and responsible society (OECD, 2017, 39). Current scholarly debates draw on a more comprehensive view and emphasize the need for science education and, thus, SL to address the pressing socio-ecological challenges of our time. Initial definitions were based on a narrower understanding of the term “scientifically literate,” focusing on the understanding of scientific knowledge and processes [Showalter (1974) in Laugksch (2000, 74)]. Today’s science education is meant to foster change in values, attitudes, and behaviors at individual and societal levels, supporting sustainable development (Laugksch, 2000, 84; OECD, 2017, 39; Sjöström and Eilks, 2018, 78; Valladares, 2021, 581–82). Beyond SL visions I and II, which relate to scientific knowledge and processes for later application and understanding of the usefulness of scientific knowledge in life and society (Sjöström and Eilks, 2018, 65–66), SL vision III is assumed to contribute to transformative engagement by means of engaging teaching methods and knowing-in-action (Aikenhead, 2007, 68; Liu, 2013, 29).
For our research, we collected data by means of a standardized questionnaire from secondary school students in Austria and Germany. The data were evaluated by regression analysis, taking a closer look at the contribution of SL and the single SL visions to student’s transformative engagement for climate action, both before and after taking part in the TE project k.i.d.Z.21 (Table 2). The regression analysis of the post-test model reveals that all the three visions of SL statistically significantly predict transformative engagement for climate action, with SL vision III measuring practical and transformative engagement in science and having a slightly higher influence than vision II and greater influence than vision I. Visions I and II, which capture knowledge and concepts of science and scientific processes and practical implications of science, have a (slightly) minor influence on transformative engagement. Despite increasing calls for (science) education to be transformative (Balsiger et al., 2017, 359; Kyle, 2020, 1; Valladares, 2021, 581–82), the results are in contrast to a wide range of school curricula, which are predominantly based on knowledge production and are not connected to students’ lifeworld (Balsiger et al., 2017, 359; Kyle, 2020, 1–4). Moreover, the majority of scholarly debates is still focused on knowledge, e.g., improvement of CC knowledge (Monroe et al., 2019, 796–98). Of course, the significance of knowledge should not be underestimated, since the exchange of knowledge and knowledge production is one of the leading aims and outcomes of transdisciplinary collaborations (Lang et al., 2012, 26). We concur with Liu (2013, 31) that all the three visions of SL are important, including knowledge of scientific concepts and processes. Consistent with the emphasis on the importance of (science) education in sustainability discourse (UN, 2015; UNESCO, 2017; Kyle, 2020, 1), we propose a more visionary approach to science education considering all the three visions of SL. However, we emphasize the need for didactical concepts, which foster the transformative potential of SL, especially vision III. Hence, returning to the initial question, whether teacher-centered, knowledge-based science education can meet the claims for SL vision III, in accordance with other scholars in the realm of transformative education (Singer-Brodowski, 2016, 15–16; Balsiger et al., 2017, 359; Leichenko and O’Brien, 2020, 1; Valladares, 2021, 581–82) and underscored by missing climate action at a societal level (Maibach et al., 2014, 295–96; Jylhä, 2018, 487), our answer is no.
Since knowledge of climate change and sustainability is, to a large degree, under constant change and context-dependent (Riemeier, 2007, 70–71; Künzli, 2019, 8), knowledge production needs to be a co-production process among students, scientists, and other out-of-school partners who jointly research topics related to students’ lifeworld and everyday life (Kubisch et al., 2021b, 1). This is not only consistent with calls for transdisciplinarity (Lang et al., 2012, 25) but also with contemporary approaches to learning, like moderate constructivism (Riemeier, 2007, 69–70), which are frequently discussed in scholarly debates. As shown in the literature review of transdisciplinarity and TE, mutual learning occurs during the collaboration process that fosters knowledge production and provides both new scientific and societal insights (Walter et al., 2007, 326–28; Clark and Button, 2011, 50; Mitchell et al., 2015, 93; Kubisch et al., 2021a). Consequently, the concept of TE captures all the three visions of SL (see Table 2). From a didactical point of view, the collaboration needs to be carefully designed, taking into consideration students’ interest and allowing them to tie up with their preconceptions and experience, hence fostering an equal exchange (Widodo and Reinders, 2004, 237–38). While jointly researching some real-world issues, students construct knowledge of scientific processes and research skills, and generate competencies like critical, system, and reflective thinking, and communicative and problem-solving competency (Currie et al., 2005, 405; Pedaste and Sarapuu, 2006, 48; Clark and Button, 2011, 48; Furtak et al., 2012, 315–16; Young et al., 2015, 72). This is in line with the didactical approach of inquiry-based learning (Pedaste et al., 2015, 51–54) and claims of ESD (UNESCO, 2017, 10).
In order to demonstrate the success of the TE concept, we conducted a pre-post comparison of the regression analysis of the pre- and post-test data, analyzing change in the predictive quality of SL on transformative engagement for climate action (Table 2). The predictive quality of the model is higher after students’ have taken part in the TE project, being explained by a higher influence of the SL visions, in particular visions III and II, gained in importance, reflected by a higher standardized ß coefficient. The change in predictive quality comparing the pre- and post-test models is 30.7%. Moreover, the pre- and post-test comparison demonstrates that SL visions II and III, which did not significantly predict transformative engagement in the pre-test, did so in the post-test, with SL vision III having a slightly higher influence than vision II and especially vision I. Hence, these findings give reason to assume that the TE concept supports the didactical realization of vision III and that of vision II. The influence of vision I on transformative engagement decreased after the students took part in the TE project. These findings support the assumption that by promoting the three visions of SL via the TE concept (see Figure 2), connecting knowledge of scientific content and processes (vision I) to a meaningful real-world context (vision II) through students’ active engagement in science with scientists (vision III), the significance of pure knowledge decreases. At the same time, the synergy of knowledge, meaningful real-world context, and active engagement in science fosters the significance of vision II and, in particular vision, III for transformative engagement for climate action.
Therefore, the contribution of the TE concept to the didactical realization of SL should be explained in more detail (see also Figure 2). The equal exchange of all partners involved, in the form of an inquiry process, fosters deep reflection, allowing for an opportunity to get to know not only different perspectives but also to gain awareness of one’s own perspective. This deep reflection is associated with transformative learning, facilitating the restructuring of mental models and enabling conceptual change through change in thought patterns, values, and behaviors (Mitchell et al., 2015, 93; Klein, 2018, 20–21) for transformative engagement (Deisenrieder et al., 2020, 8–11; Kubisch et al., 2021a, 6–8). Moreover, active engagement in a joint inquiry process not only increases scientific skills (Currie et al., 2005, 405), awareness (Young et al., 2015, 72; Kuthe et al., 2019, 382–83), and personal meaningfulness of socio-ecological issues (Monroe et al., 2019, 804–6), but it also draws on all the three kinds of knowledge, system, target, and transformation, which are relevant for action (Walter et al., 2007, 333–34).
Conclusion and Future Research
In order to achieve the 1.5-degree Celsius target, the need for social transformation has been acknowledged in the Paris Agreement (UNFCCC, 2015), requesting changes in values and world-views and, even more importantly, demanding for individual and collective actions (O’Brien et al., 2018, 155–57). SDG4, “Quality Education” plays a key role in this regard and aims to contribute by means of awareness building, and knowledge and competency generation to transformative engagement (WBGU, 2011, 352–58; UNESCO, 2021). In that discourse, science education is understood as making a major contribution to a scientifically literate society, which makes informed and responsible decisions (OECD, 2017, 39).
However, as pointed out in this study and acknowledged by many other scholars discussing the concept of SL, teacher-centered and knowledge-based science education is not the appropriate methodology to reach these goals. TE is a promising didactical concept working toward the claims of SL vision III, which can be described as science for transformation (Sjöström and Eilks, 2018, 78). TE is considered to be a frame, enabling students’ active, situational, emotional, social, and self-regulated learning (Riemeier, 2007, 69–70), as well as identification and pursuit of (scientific) issues within students’ lifeworld (Pedaste et al., 2015, 55–57). Active engagement in a research process and exchange of different perspectives and forms of knowledge between students and scientific experts are assumed to contribute to transformative learning (Mezirow, 2003, 58), which enables change in habitual thought patterns, norms, values, and, finally, behaviors (Mitchell et al., 2015, 93; Klein, 2018, 20–21). The findings of this study give first indications, demonstrating the influence of SL on transformative engagement for climate action in a TE project.
These promising results need to be replicated and proven by future research also taking into account the limitations of this study. In particular, the SL visions need to be replicated and the constitutive items should be further expanded in terms of their content. SL vision I, in particular, needs to be considered critically. In this study, SL vision I is not specifically focused on “scientific content and scientific processes” (Sjöström and Eilks, 2018, 65) but on a fundamental understanding of the nature of science, capturing students’ (mis)conceptions of science. The reason is that operationalizing SL vision I on the basis of disciplinary knowledge does not allow for the replication of the findings and, thus, transfer to other contexts (e.g., different school types, age groups, and countries) because of student’s diverse prior knowledge. Consequently, future research needs to rethink the operationalization of SL vision I. Considering the research frame, which was determined in advance in this TE project, we propose to replicate this study allowing students to co-design the whole research process and collaborating with scientists within students’ local community.
Data Availability Statement
The raw data supporting the conclusions of this article will be made available by the authors, without undue reservation.
Ethics Statement
The studies involving human participants were reviewed and approved by Institutional Review Board and Ethics Committee of the University of Innsbruck. Written informed consent to participate in this study was provided by the participants’ legal guardian/next of kin.
Author Contributions
SK, HK, LK, VD, MF, KO, NL, SP, and JS: conceptualization. HK and SK: investigation and data evaluation. SK, HK, and LK: writing original draft preparation. LK, VD, MF, KO, NL, SP, and JS: writing review and editing. SK: visualization. LK and JS: supervision, project administration, and funding acquisition. All authors have read and agreed to the published version of the manuscript.
Funding
This research received funding from the European Union’s Horizon 2020 Research and Innovation Program under grant agreement no. 824522.
Conflict of Interest
HK is employed by alpS GmbH which is part of the project Science Education for Action and Engagement toward Sustainability.
The remaining authors declare that the research was conducted in the absence of any commercial or financial relationships that could be construed as a potential conflict of interest.
Publisher’s Note
All claims expressed in this article are solely those of the authors and do not necessarily represent those of their affiliated organizations, or those of the publisher, the editors and the reviewers. Any product that may be evaluated in this article, or claim that may be made by its manufacturer, is not guaranteed or endorsed by the publisher.
Acknowledgments
We would like to thank Lisa Thompson for the help with style and language.
References
Aikenhead, G. (2007). Promoting Scientific Literacy: Science Education Research in Transaction. Uppsala: Geotryckeriet, 64–71.
Balsiger, J., Förster, R., Mader, C., Nagel, U., Sironi, H., Wilhelm, S., et al. (2017). Transformative learning and education for sustainable development. GAIA Ecol. Perspect. Sci. Soc. 26, 357–359. doi: 10.14512/gaia.26.4.15
Barnes, M. L., Wang, P., Cinner, J. E., Graham, N. A. J., Guerrero, A. M., Jasny, L., et al. (2020). Social determinants of adaptive and transformative responses to climate change. Nat. Clim. Chang. 10, 823–828. doi: 10.1038/s41558-020-0871-4
Barnosky, A. D., Ehrlich, P. R., and Hadly, E. A. (2016). Avoiding collapse: grand challenges for science and society to solve by 2050. Elem. Sci. Anth. 4, 1–9. doi: 10.12952/journal.elementa.000094
Beecroft, R. (2019). “Das transformative projektseminar – didaktische ansätze und methodische umsetzung,” in Transdisziplinär Und Transformativ Forschen, Band 2, eds R. Defila and A. Di Giulio (Wiesbaden: Springer Fachmedien Wiesbaden), 293–333.
Bencze, J., and Alsop, S. (eds) (2014). Activist Science and Technology Education. Dordrecht: Springer.
Boeren, E. (2019). Understanding Sustainable Development Goal (SDG) 4 on quality education from Micro, Meso and Macro perspectives. Int. Rev. Educ. 65, 277–294. doi: 10.1007/s11159-019-09772-7
Burmeister, M., and Eilks, I. (2012). An example of learning about plastics and their evaluation as a contribution to education for sustainable development in secondary school chemistry teaching. Chem. Educ. Res. Pract. 13, 93–102. doi: 10.1039/C1RP90067F
Burmeister, M., Schmidt-Jacob, S., and Eilks, I. (2013). German chemistry teachers’ understanding of sustainability and education for sustainable development—an interview case Study. Chem. Educ. Res. Pract. 14, 169–176. doi: 10.1039/C2RP20137B
Choi, K., Lee, H., Shin, N., and Won Kim, S. -, and Krajcik, J. (2011). Re-Conceptualization of Scientific Literacy in South Korea for the 21st Century. J. Res. Sci. Teach. 48, 670–697. doi: 10.1002/tea.20424
Ciavarella, A., Cotterill, S., Scott, P., Kew, S., Philip, S., van Oldenborgh, G. J., et al. (2020). Prolonged Siberian Heat of 2020. Available online at: https://www.worldweatherattribution.org/siberian-heatwave-of-2020-almost-impossible-without-climate-change/ (accessed July 12, 2021).
Clark, B., and Button, C. (2011). Sustainability transdisciplinary education model: interface of arts, science, and community (STEM). Int. J. Sustain. High. Educ. 12, 41–54. doi: 10.1108/14676371111098294
Clarke, E., and Ashhurst, C. (2018). Making collective learning coherent: an adaptive approach to the practice of transdisciplinary pedagogy. Fam. Neuhauser. Gibbs 2018, 151–165.
Cohen, J. (1988). Statistical Power Analysis for the Behavioral Sciences. Mahwah, NJ: Lawrence Erlbaum Associates.
Corner, A., Roberts, O., Chiari, S., Völler, S., Mayrhuber, E. S., Mandl, S., et al. (2015). How do young people engage with climate change? The role of knowledge, values, message framing, and trusted communicators. WIREs Clim. Change 6, 523–534. doi: 10.1002/wcc.353
Crutzen, P. J. (2006). “The anthropocene,” in Earth System Science in the Anthropocene, eds E. Ehlers and T. Krafft (Berlin: Springer), 13–18.
Currie, M., King, G., Rosenbaum, P., Law, M., Kertoy, M., and Specht, J. (2005). A model of impacts of research partnerships in health and social services. Eval. Progr. Plann. 28, 400–412. doi: 10.1016/j.evalprogplan.2005.07.004
Deisenrieder, V., Kubisch, S., Keller, L., and Stötter, J. (2020). Bridging the action gap by democratizing climate change education—the case of K.I.D.Z.21 in the context of fridays for future. Sustainability 12, 1–19. doi: 10.3390/su12051748
Eilks, I. (2015). Science education and education for sustainable development – justifications, models, practices and perspectives. Eurasia J. Math. Sci. Technol. Educ. 11, 149–158. doi: 10.12973/eurasia.2015.1313a
European Commission (2007). Science Education Now: A Renewed Pedagogy for the Future of Europe. Available online at: https://www.eesc.europa.eu/en/documents/rocard-report-science-education-now-new-pedagogy-future-europe (accessed October 10, 2021).
Furtak, E. M., Seidel, T., Iverson, H., and Briggs, D. C. (2012). Experimental and quasi-experimental studies of inquiry-based science teaching. Rev. Educ. Res. 82, 300–329. doi: 10.3102/0034654312457206
Grabau, L. J., and Ma, X. (2017). Science engagement and science achievement in the context of science instruction: a multilevel analysis of U.S. Students and Schools. Int. J. Sci. Educ. 39, 1045–1068. doi: 10.1080/09500693.2017.1313468
Hagedorn, G., Loew, T., Seneviratne, S. I., Lucht, W., Luise Beck, M. -, Hesse, J., et al. (2019). The concerns of the young protesters are justified: a statement by scientists for future concerning the protests for more climate protection. GAIA Ecol. Perspect. Sci. Soc. 28, 79–87. doi: 10.14512/gaia.28.2.3
Hahnel, U. J. J., Mumenthaler, C., and Brosch, T. (2020). Emotional foundations of the public climate change divide. Clim. Change 161, 9–19. doi: 10.1007/s10584-019-02552-0
Heddy, B. C., and Sinatra, G. M. (2017). Transformative parents: facilitating transformative experiences and interest with a parent involvement intervention. Sci. Educ. 101, 765–786. doi: 10.1002/sce.21292
Herman, B. C., Owens, D. C., Oertli, R. T., Zangori, L. A., and Newton, M. H. (2019). Exploring the complexity of students’ scientific explanations and associated nature of science views within a place-based socioscientific issue context. Sci. Educ. 28, 329–366. doi: 10.1007/s11191-019-00034-4
Hindley, A., and Wall, T. (2018). “A unifying, boundary-crossing approach to developing climate literacy,” in Implementing Sustainability in the Curriculum of Universities, ed. W. L. Filho (Cham: Springer International Publishing), 263–278. doi: 10.1007/978-3-319-70281-0_16
Hodson, D. (2003). Time for action: science education for an alternative future. Int. J. Sci. Educ. 25, 645–670. doi: 10.1080/09500690305021
Hodson, D. (2011). Looking to the Future: Building a Curriculum for Social Activism. Rotterdam: Sense.
IPCC (2018). “Global Warming of 1.5°C. an IPCC special report on the impacts of global warming of 1.5°c above pre-industrial levels and related global greenhouse gas emission pathways,” in The Context of Strengthening the Global Response to the Threat of Climate Change, Sustainable Development, and Efforts to Eradicate Poverty, eds V. Masson-Delmotte, P. Zhai, H.-O. Pörtner, D. Roberts, J. Skea, P. R. Shukla, et al. (Geneva: IPCC).
Jylhä, K. M. (2018). “Denial versus reality of climate change,” in Encyclopedia of the Anthropocene, eds D. A. Dellasala and M. I. Goldstein (Oxford: Elsevier Inc), 487–492. doi: 10.1016/b978-0-12-809665-9.09762-7
Keller, L., Stötter, J., Oberrauch, A., Kuthe, A., Körfgen, A., and Hüfner, K. (2019). Changing climate change education: exploring moderate constructivist and transdisciplinary approaches through the research-education co-operation K.I.D.Z.21. GAIA Ecol. Perspect. Sci. Soc. 28, 35–43. doi: 10.14512/gaia.28.1.10
Klein, J. T. (2018). “Learning in transdisciplinary collaborations: a conceptual vocabulary,” in Transdisciplinary Theory, Practice and Education: The Art of Collaborative Research and Collective Learning, eds D. Fam, L. Neuhauser, and P. Gibbs (Cham: Springer International Publishing), 11–23. doi: 10.1007/978-3-319-93743-4_2
Kubisch, S., Parth, S., Deisenrieder, V., Oberauer, K., Stötter, J., and Keller, L. (2021b). From transdisciplinary research to transdisciplinary education—the role of schools in contributing to community well-being and sustainable development. Sustainability 13, 1–13. doi: 10.3390/su13010306
Kubisch, S., Oberauer, K., Fritz, M. M., and Keller, L. (2021a). Transdisziplinäre klimawandelbildung in der forschungs-bildungs-kooperation k.i.d.Z.21. Haushalt Bildung Forschung 4, 1–12.
Kuckartz, U., Rädiker, S., Ebert, T., and Schehl, J. (2013). Statistik – Eine Verständliche Einführung. Cham: Springer Fachmedien.
Künzli, R. (2019). Disziplinarität und transdisziplinarität. ITDB 1, 8–15. doi: 10.5281/ZENODO.2557601
Kuthe, A., Körfgen, A., Stötter, H., and Keller, L. (2019). Strengthening their climate change literacy: a case study addressing the weaknesses in young people’s climate change awareness. Appl. Environ. Educ. Commun. 47, 375–388. doi: 10.1080/1533015X.2019.1597661
Kyle, W. C. (2020). Expanding our views of science education to address sustainable development, empowerment, and social transformation. Discip. Interdscip. Sci. Educ. Res. 2, 1–9. doi: 10.1186/s43031-019-0018-5
Lang, D. J., Wiek, A., Bergmann, M., Stauffacher, M., Martens, P., Moll, P., et al. (2012). Transdisciplinary research in sustainability science: practice, principles, and challenges. Sustain. Sci. 7, 25–43. doi: 10.1007/s11625-011-0149-x
Laugksch, R. C. (2000). Scientific literacy: a conceptual overview. Sci. Educ. 84, 71–94. doi: 10.1002/(sici)1098-237x(200001)84:1<71::aid-sce6>3.0.co;2-c
Lee, K., Gjersoe, N., O’Neill, S., and Barnett, J. (2020). Youth perceptions of climate change: a narrative synthesis. WIREs Clim. Change 11, 1–24. doi: 10.1002/wcc.641
Leichenko, R., Gram-Hanssen, I., and O’Brien, K. (2021). Teaching the how of transformation. Sustain. Sci. [Epub ahead of print]. doi: 10.1007/s11625-021-00964-5
Leichenko, R., and O’Brien, K. (2020). Teaching climate change in the anthropocene: an integrative approach. Anthropocene 30, 1–4. doi: 10.1016/j.ancene.2020.100241
Linder, C., Östman, L., and Wickman, P.-O. (eds) (2007). Promoting Scientific Literacy: Science Education Research in Transaction. Uppsala: Geotryckeriet.
Liu, X. (2013). “Expanding notions of scientific literacy: a reconceptualization of aims of science education in the knowledge society,” in Science Education for Diversity, eds N. Mansour and R. Wegerif (Dordrecht: Springer Netherlands), 23–39. doi: 10.1007/978-94-007-4563-6_2
Maibach, E., Myers, T., and Leiserowitz, A. (2014). Climate scientists need to set the record straight: there is a scientific consensus that human-caused climate change is happening. Earth’s Future 2, 295–298. doi: 10.1002/2013EF000226
Mezirow, J. (2003). Transformative learning as discourse. J. Transf. Educ. 1, 58–63. doi: 10.1177/1541344603252172
Mitchell, C., Cordell, D., and Fam, D. (2015). Beginning at the end: the outcome spaces framework to guide purposive transdisciplinary research. Futures 65, 86–96. doi: 10.1016/j.futures.2014.10.007
Monroe, M. C., Plate, R. R., Oxarart, A., Bowers, A., and Chaves, W. A. (2019). Identifying effective climate change education strategies: a systematic review of the research. Environ. Educ. Res. 25, 791–812. doi: 10.1080/13504622.2017.1360842
Nordén, B. (2018). Transdisciplinary teaching for sustainable development in a whole school project. Environ. Educ. Res. 24, 663–677. doi: 10.1080/13504622.2016.1266302
Norris, S. P., and Phillips, L. M. (2003). How literacy in its fundamental sense is central to scientific literacy. Sci. Educ. 87, 224–240. doi: 10.1002/sce.10066
Oberrauch, A., Keller, L., Riede, M., Mark, S., Kuthe, A., Körfgen, A., et al. (2015). ,,K.I.D.Z.21 – Kompetent in Die Zukunft – Grundlagen Und Konzept Einer Forschungs-Bildungs-Kooperation Zur Bewältigung Der Herausforderungen Des Klimawandels Im 21, Jahrhundert. GW Unterricht 3, 19–31.
O’Brien, K. (2018). Is the 1.5°C Target Possible? Exploring the three spheres of transformation. Curr. Opin. Environ. Sustain. 31, 153–160. doi: 10.1016/j.cosust.2018.04.010
O’Brien, K., Selboe, E., and Hayward, B. M. (2018). Exploring youth activism on climate change: dutiful, disruptive, and dangerous dissent. Ecol. Soc. 23:42. doi: 10.5751/ES-10287-230342
O’Brien, K., and Sygna, L. (eds) (2013). “Responding to climate change: the three spheres of transformation,” in Proceedings of Transformation in a Changing Climate, (Oslo: University of Oslo), 16–23.
OECD (2017). PISA 2015 Assessment and Analytical Framework: Science, Reading, Mathematic, Financial Literacy and Collaborative Problem Solving, Revised Edition. Paris: OECD.
Patall, E. A., Steingut, R. R., Freeman, J. L., Pituch, K. A., and Vasquez, A. C. (2018). Gender disparities in students’ motivational experiences in high school science classrooms. Sci. Educ. 102, 951–977. doi: 10.1002/sce.21461
Pearce, B., Adler, C., Senn, L., Krütli, P., Stauffacher, M., and Pohl, C. (2018). Making the link between transdisciplinary learning and research. Fam. Neuhauser. Gibbs 2018, 167–183.
Pedaste, M., Mäeots, M., Siiman, L. A., de Jong, T., van Riesen, S. A. N., Kamp, E. T., et al. (2015). Phases of inquiry-based learning: definitions and the inquiry cycle. Educ. Res. Rev. 14, 47–61. doi: 10.1016/j.edurev.2015.02.003
Pedaste, M., and Sarapuu, T. (2006). Developing an effective support system for inquiry learning in a web-based environment. J. Comput. Assist. Learn. 22, 47–62.
Philip, S. Y., Kew, S. F., van Oldenborgh, G. J., Yang, W., Vecchi, G. A., Anslow, F. S., et al. (2021). Rapid Attribution Analysis of the Extraordinary Heatwave on the Pacific Coast of the US and Canada June 2021. Available online at: https://www.worldweatherattribution.org/western-north-american-extreme-heat-virtually-impossible-without-human-caused-climate-change/ (accessed July 12, 2021).
Pohl, C., Krütli, P., and Stauffacher, M. (2018). Teaching transdisciplinarity appropriately for students’ education level. GAIA Ecol. Perspect. Sci. Soc. 27, 250–252. doi: 10.14512/gaia.27.2.14
Poortinga, W., Whitmarsh, L., Steg, L., Böhm, G., and Fisher, S. (2019). Climate change perceptions and their individual-level determinants: a cross-european analysis. Glob. Environ. Change 55, 25–35. doi: 10.1016/j.gloenvcha.2019.01.007
ProClim (1997). Research on Sustainability and Global Change – Visions in Science Policy by Swiss Researchers. Available online at: https://scnat.ch/en/uuid/i/6fc6028b-5a36-53a3-b259-48cfcfa10753-Visions_of_Swiss_scientists (accessed November 16, 2021).
Pugh, K. J., Linnenbrink-Garcia, L., Koskey, K. L. K., Stewart, V. C., and Manzey, C. (2010). Motivation, learning, and transformative experience: a study of deep engagement in science. Sci. Educ. 94, 1–28. doi: 10.1002/sce.20344
Reeve, J., and Tseng, C. M. (2011). Agency as a fourth aspect of students’ engagement during learning activities. Contemp. Educ. Psychol. 36, 257–267. doi: 10.1016/j.cedpsych.2011.05.002
Riemeier, T. (2007). “Moderater konstruktivismus,” in Theorien in Der Biologiedidaktischen Forschung, eds D. Krüger and H. Vogt (Berlin: Springer-Verlag), 69–79.
Roberts, D. A. (2007). “Scientific literacy/science literacy,” in Handbook of Research on Science Education, eds S. K. Abell and N. G. Lederman (Mahwah, NJ: Lawrence Erlbaum), 729–780.
Roberts, D. (2011). “Competing visions of scientific literacy,” in Exploring the Landscape of Scientific Literacy, eds C. Linder, L. Ostman, D. Roberts, P. Wickman, G. Erickson, and A. MacKinnon (New York, NY: Routledge), 11–27.
Rockström, J., Gaffney, O., Rogelj, J., Meinshausen, M., Nakicenovic, N., and Joachim Schellnhuber, H. (2017). A Roadmap for Rapid Decarbonization. Science 355, 1269–1271. doi: 10.1126/science.aah3443
Schäfer, A., Mühr, B., Daniell, J., Ehret, U., Ehmele, F., Küpfer, K., et al. (2021). Hochwasser Mitteleuropa, Juli 2021 (Deutschland) : 21. Juli 2021 – Bericht Nr. 1 ,,Nordrhein-Westfalen & Rheinland-Pfalz. Available online at: https://www.cedim.kit.edu/2850.php (accessed July 12, 2021).
Schmohl, T., and Philipp, T. (2021). “Transdisziplinäre didaktik: eine einführung,” in Handbuch Transdisziplinäre Didaktik, eds T. Schmohl and T. Philipp (Bielefeld: Transcript Verlag), 13–23.
Sedlmeier, P., and Renkewitz, F. (2013). Forschungsmethoden Und Statistik: Ein Lehrbuch Für Psychologen Und Sozialwissenschaftler. Hallbergmoos: Pearson Studium.
Semken, S., and Butler Freeman, C. (2008). Sense of place in the practice and assessment of place-based science teaching. Sci. Educ. 92, 1042–1057. doi: 10.1002/sce.20279
Showalter, V. M. (1974). What is united science education? Part 5. Program objectives and scientific literacy. PRISM II 2:34.
Simonneaux, L. (2014). “Questions socialement vives and socio-scientific issues: new trends of research to meet the training needs of postmodern society,” in Topics and Trends in Current Science Education, eds C. Bruguière, A. Tiberghien, and P. Clément (Dordrecht: Springer), 37–54.
Singer-Brodowski, M. (2016). Transformative bildung durch transformatives lernen. zur notwendigkeit der erziehungswissenschaftlichen fundierung einer neuen idee. Z. Int. Bildungsforschung Entwicklungspädagogik 39, 13–17.
Sjöström, J., and Eilks, I. (2018). “Reconsidering different visions of scientific literacy and science education based on the concept of bildung,” in Cognition, Metagcognition, and Culture in STEM Education: Learning, Teaching and Assessment, eds Y. J. Dori, Z. R. Mevarech, and D. R. Baker (Cham: Springer), 65–88.
Sjöström, J., and Rydberg, C. (2018). “Towards transdisciplinary didaktik – didactic modelling of complex controversial issues teaching for reflexive bildung and sustainability,” in Building Bridges Across Disciplines, eds I. Eilks, S. Markic, and B. Ralle (Aachen: Shaker), 3–16.
Steffen, W., Richardson, K., Rockström, J., Cornell, S. E., Fetzer, I., Bennett, E. M., et al. (2015). Planetary boundaries: guiding human development on a changing planet. Sci. Educ. 347, 1–10. doi: 10.1126/science.1259855
Stötter, J., Keller, L., ütke-Spatz, L. L., Oberrauch, A., Körfgen, A., and Kuthe, A. (2016). Kompetent in die zukunft : die forschungs-bildungs-kooperation zur klimawandelbildung K.I.D.Z.21 Und K.I.D.Z.21-Austria. GAIA Ecol. Perspect. Sci. Soc. 25, 214–216. doi: 10.14512/gaia.25.3.19
Thomas, C. L., and Kirby, L. A. J. (2020). Situational interest helps correct misconceptions: an investigation of conceptual change in University Students. Instr. Sci. 48, 223–241. doi: 10.1007/s11251-020-09509-2
Thomas, I. (2009). Critical thinking, transformative learning, sustainable education, and problem-based learning in universities. J. Transf. Educ. 7, 245–264. doi: 10.1177/1541344610385753
UN (2015). Transforming Our World: The 2030 Agenda for Sustainable Development. Available online at: https://sdgs.un.org/2030agenda (accessed August 10, 2021).
UN (2021). The 17 Goals. Available online at: https://sdgs.un.org/goals (Accessed July 21, 2021).
UNESCO (2021). Education for Sustainable Development. Available online at: https://en.unesco.org/themes/education-sustainable-development (Accessed July 21, 2021).
UNFCCC (2015). The Paris Agreement. Available online at: https://unfccc.int/process-and-meetings/the-paris-agreement/the-paris-agreement (accessed July 29, 2021).
Valladares, L. (2021). Scientific literacy and social transformation: critical perspectives about science participation and emancipation. Sci. Educ. 30, 557–587. doi: 10.1007/s11191-021-00205-2
Verlie, B., Clark, E., Jarrett, T., and Supriyono, E. (2021). Educators’ experiences and strategies for responding to ecological distress. Aust. J. Environ. Educ. 37, 132–146. doi: 10.1017/aee.2020.34
Vilsmaier, U., and Lang, D. J. (2015). Making a difference by marking the difference: constituting in-between spaces for sustainability learning. Curr. Opin. Environ. Sustain. 16, 51–55. doi: 10.1016/j.cosust.2015.07.019
Walter, A. I., Helgenberger, S., Wiek, A., and Scholz, R. W. (2007). Measuring societal effects of transdisciplinary research projects: design and application of an evaluation method. Eval. Progr. Plann. 30, 325–338. doi: 10.1016/j.evalprogplan.2007.08.002
Widodo, A., and Reinders, D. (2004). Konstruktivistische Sichtweisen Vom Lehren Und Lernen Und Die Praxis Des Physikunterrichts. Z. Didaktik Naturwissenschaften 10, 233–255.
Wiek, A., Withycombe, L., and Redman, C. L. (2011). Key competencies in sustainability: a reference framework for academic program development. Sustain. Sci. 6, 203–218. doi: 10.1007/s11625-011-0132-6
Yacoubian, H. A. (2018). Scientific literacy for democratic decision-making. Int. J. Sci. Educ. 40, 308–327. doi: 10.1080/09500693.2017.1420266
Young, C., Reid, J., and Meehan, B. (2015). Taking action: researching an innovative pedagogy for an aesthetic visual approach to environmental issues. Curr. Opin. Environ. Sustain. 16, 64–72. doi: 10.1016/j.cosust.2015.07.002
Zalasiewicz, J., Waters, C. N., Summerhayes, C. P., Wolfe, A. P., Barnosky, A. D., Cearreta, A., et al. (2017). The working group on the anthropocene: summary of evidence and interim recommendations. Anthropocene 19, 55–60.
Zeidler, D. L., Herman, B. C., and Sadler, T. D. (2019). New directions in socioscientific issues research. Discip. Interdscip. Sci. Educ. Res. 1, 1–9. doi: 10.1186/s43031-019-0008-7
Keywords: climate action, quality (science) education, sustainable development goals, transdisciplinary education, scientific literacy, transformative learning, transformative engagement
Citation: Kubisch S, Krimm H, Liebhaber N, Oberauer K, Deisenrieder V, Parth S, Frick M, Stötter J and Keller L (2022) Rethinking Quality Science Education for Climate Action: Transdisciplinary Education for Transformative Learning and Engagement. Front. Educ. 7:838135. doi: 10.3389/feduc.2022.838135
Received: 17 December 2021; Accepted: 08 February 2022;
Published: 23 March 2022.
Edited by:
Antti Laherto, University of Helsinki, FinlandReviewed by:
Jesper Sjöström, Malmö University, SwedenRosemary Hipkins, New Zealand Council for Educational Research, New Zealand
Copyright © 2022 Kubisch, Krimm, Liebhaber, Oberauer, Deisenrieder, Parth, Frick, Stötter and Keller. This is an open-access article distributed under the terms of the Creative Commons Attribution License (CC BY). The use, distribution or reproduction in other forums is permitted, provided the original author(s) and the copyright owner(s) are credited and that the original publication in this journal is cited, in accordance with accepted academic practice. No use, distribution or reproduction is permitted which does not comply with these terms.
*Correspondence: Susanne Kubisch, U3VzYW5uZS5LdWJpc2NoQHVpYmsuYWMuYXQ=