- 1Department of Psychology, Education and Child Studies, Erasmus University Rotterdam, Rotterdam, Netherlands
- 2Roosevelt Center for Excellence in Education, University College Roosevelt, Utrecht University, Middelburg, Netherlands
- 3Delft Institute of Applied Mathematics, Delft University of Technology, Delft, Netherlands
- 4School of Education/Early Start, University of Wollongong, Wollongong, NSW, Australia
We investigated the effects of active workstations on cognitive control functions in individuals diagnosed with Attention Deficit Hyperactivity Disorder (ADHD). In a fully counterbalanced randomized control design, we examined the effects of cycling on a desk bike on phonological working memory (WM) in 18 adolescents with ADHD. Adolescents performed a phonological WM test across two separate sessions during which they either cycled or not. It was hypothesized that participants would perform better on the WM task while cycling as compared to seated-rest. Results showed that total WM performance was not affected by desk-bike cycling. Exploratory analyses suggested that cycling during more difficult trials (i.e., high WM demands) is beneficial for WM performance. More research is needed to shed light on how task difficulty moderates the potential compensatory effect of desk-bike cycling on WM performance in adolescent ADHD participants.
Introduction
Effects of Desk-Bike Cycling on Phonological Working Memory (WM) Performance in Adolescents With ADHD.
Despite evidence that points to positive associations between physical activity, cognitive function (Hillman et al., 2008), academic performance (Castelli et al., 2007; Donnelly et al., 2016), and overall health (Butte et al., 2007) in school-aged youth, children’s opportunities to engage in physical activities during the school day have significantly decreased during the last two decades (Institute of Medicine, 2013). This trend has co-occurred with the increasing prevalence of sedentary behaviors in western society due to advances in technology and environmental changes (Ng and Popkin, 2012) and contributes to chronic health problems across the life span, such as obesity and cardiovascular diseases (Hillman et al., 2008). As a response to these negative developments, organizations have begun to promote physical activity in the classroom (National Association for Sport and Physical Education et al., 2008). In addition, there has been a gradual increase in studies investigating the impact of active teaching lessons or active workstations on health and cognition (see also Kibbe et al., 2011; Donnelly et al., 2016; for a review see Mavilidi et al., 2018; Ruiter et al., 2019).
Recently, it has been suggested that physical activity interventions are especially beneficial for those children characterized by lower cognitive control and self-regulatory behavior1 (Drollette et al., 2014). That is, overall findings revealed that a single, acute bout of moderate-intensity aerobic exercise facilitates cognitive performance, with more extensive effects observed for the lower-performing group than the higher-performing group on a flanker task. Similarly, Mahar et al. (2006) reported that increasing physical activity opportunities in the classroom to improve on-task behavior were especially beneficial for children who demonstrated poor on-task behavior at baseline. One specific class of individuals with cognitive control impairments is children diagnosed with attention deficit hyperactivity disorder (ADHD). Attention deficit hyperactivity disorder is a psychological outcome that manifests itself during childhood and is characterized by developmentally inappropriate levels of inattention, impulsivity, and hyperactivity (Rapport et al., 2009). Contemporary leading theories have explained the mechanism underlying ADHD-related deficits as an imbalance in the catecholaminergic systems in the brain, leading to dysfunction of attentional circuits (Rapport et al., 2009). Specifically, it has been hypothesized that attentional impairments may be due to depleted levels of dopamine in the prefrontal cortex. The efficacy of psychostimulants in the treatment of ADHD supports this notion (Yu-Kai et al., 2012).
Pontifex et al. (2013) investigated the effect of a single bout of moderate-intensity aerobic exercise on children with and without ADHD using objective measures of attention and brain neurophysiology. Although both groups improved performance on the flanker task following exercise, acute exercise appeared to have an additional benefit for children with ADHD. The ADHD group exhibited an increase in a neuroelectric component (i.e., error-related negativity, ERN) that has been linked to the upregulation of processes involved in action monitoring. Importantly, these exercise-induced facilitations in action monitoring processes resulted in increased task performance following errors in the ADHD group only.
Apart from the reported benefits of physical activities, such as aerobic exercise, recent studies also suggest that self-initiated spontaneous motor activity seems to serve as a positive function for children with ADHD. Rapport et al. (2009) noticed that the relationship between increased rates of activity and ADHD is pervasive, as the disorder is associated with excessive gross motor activity and fidgeting from early childhood (Corkum et al., 1998; Rapport et al., 2009), in both children and adults (Teicher et al., 2012). Moreover, also during the performance of cognitively demanding tasks (i.e., WM, stop-signal, and choice tasks), increased levels of activities among children and adults diagnosed with ADHD have been reported (Rapport et al., 2009; Alderson et al., 2012; Hudec et al., 2014). Rapport et al. (2009) hypothesized that increased movement may serve as a compensatory function to augment chronic cortical under-arousal associated with ADHD (Dickstein et al., 2006). In other words, hyperactivity is a functional and secondary symptom reflective of unconscious efforts to increase arousal to meet cognitive WM demands. This model therefore predicts a positive relation between gross motor activity and cognitive performance for persons with ADHD. Indeed, this functional WM model was substantiated with recent findings showing that higher rates of spontaneous motor activity predicted significantly better phonological WM performance (Sarver et al., 2015) and better cognitive control performance (Hartanto et al., 2016) for children with ADHD. However, findings also showed that increasing set size demands within the context of a phonological WM task was not significantly related to an activity level as one should expect when adhering to the functional model (Rapport et al., 2009; Sarver et al., 2015).
With these recent findings, the widespread assumption that excess gross motor activity interferes with children’s ability to actively engage in learning-related activities that challenge executive functions, such as WM, and the subsequent recommendations or measures to target this hyperactivity in the classroom, should be reconsidered. Instead of decreasing the activity levels of children and adolescents diagnosed with ADHD, research should investigate how opportunities for physical activities, particularly, ones that are neither disruptive nor stigmatizing, can be used in academic settings to help children perform cognitively demanding tasks. One promising strategy for increasing physical activity in the classroom is through the use of active workstations, such as desk bikes (Torbeyns et al., 2014; Ruiter et al., 2017).
Given the role of physical activity on cognitive function and academic performance, the aim of the present study was to investigate whether an exercise that is concurrent and of light intensity (i.e., self-paced cycling on a desk bike) could increase cognitive control of adolescents diagnosed with ADHD. Most research in the acute exercise literature has found positive effects on cognitive control processes after at least 20 min of moderate exercise (e.g., Hillman et al., 2003) in adults. To our knowledge, very few studies have investigated the effectiveness of a seated concurrent light intensity physical activity for preadolescents or adolescents diagnosed with ADHD (Sarver et al., 2015; Ruiter et al., 2019). Therefore, in the present study, the effects of structured motor activity on a desk bike on phonological WM performance in adolescents diagnosed with ADHD were examined. Desk-bike cycling was used in the study given its feasibility (i.e., allows students to stay seated and is relatively quiet) and affordability to be used in a classroom setting. Based on findings in previous work in which higher rates of spontaneous motor activity predicted significantly better phonological WM performance for children with ADHD (Sarver et al., 2015), it was hypothesized that adolescents with ADHD would benefit from cycling on the desk bike (i.e., concurrent light-intensity activity) and consequently show higher WM performance during cycling than during seated-rest. Since cycling on a desk bike is considered a light intensity activity in this study, no hypothesis was formulated for effects after cycling. We also explored whether WM performance during cycling would be differentially affected by WM load. In addition, we explored the effects of desk-bike cycling on subjective measures of mental effort, enjoyment, and difficulty.
Materials and Methods
Participants
Participants were 18 students of a special education school in the Netherlands (age, 15.62 ± 2.20; gender, 17 men), who were recruited through a newsletter and a special participation request letter sent via the school to the parents of 49 eligible adolescents. All participants had received the formal diagnosis of ADHD by their psychologist or psychiatrist using the Diagnostic and Statistical Manual of Mental Disorders, Fifth Edition (DSM-V) criteria. Given generalizability concerns, adolescents with comorbidities were included. Out of the 18 participants, 10 had a comorbid diagnosis with an Autism Spectrum Disorder, and four with other comorbid diagnoses (i.e., persistent depressive disorder, attachment disorder, adjustment disorder, developmental coordination disorder, and Tourette syndrome). Twelve children were prescribed psychostimulants at the time of assessment. Parents of participants who were taking psychostimulants were asked to withhold these for a minimum of 24 h prior to participating in all assessment sessions. Furthermore, participants were asked to refrain from drinking coffee or high-sodium drinks. This study was approved by the university’s Institutional Review Board.
Apparatus
One height-adjustable desk, one desk bike (Worktrainer), and one laptop (Lenovo B590, 15.6 in.) was used in the study. The cycling resilience was kept equal across participants.
Design and Procedure
The present study employed a fully counter-balanced, within-subjects repeated measures design. Each participant took part in two assessment sessions with repeating phonological WM tasks under different conditions. Each session was separated by approximately 8 days (range 5–16). Participants were tested in the same order and in the same room on both occasions. Randomization and counterbalancing of participants occurred before the first session to minimize learning and order effects (i.e., seated-rest–cycling vs. cycling–seated-rest). The students were individually taken out of class, brought to a quiet room, and were seated on a desk bike approximately 0.6 m from a laptop placed on a height-adjustable desk. The resistance rate was kept equal across students and sessions. Participants were given instructions about the task and had to practice several trials. In the cycling condition, they were asked to ride the desk bike for 2 min in order to familiarize themselves with the apparatus and to find a stable, endurable pace of their preference. In the non-cycling condition, participants were asked to sit still for 2 min on the desk bike. After this, the task started and participants completed it while cycling or engaging in seated-rest. Performance was monitored at all times by two trained research assistants who were seated in the same room, but outside participants’ view and who wrote down the participants’ oral answers. During both sessions, participants were recorded with a camera to register their responses. A session lasted for 30 min.
Measures
Phonological Working Memory Task
The phonological WM task is similar to the Letter-Number Sequencing subtest on the Wechsler Intelligence Scale for Children, 4th Edition (WISC-IV; Wechsler, 2003) and assesses phonological WM based on Baddeley’s (2007) model by requiring both central executive processing/manipulation and phonological storage-rehearsal. This cognitive task has often been used in ADHD research (e.g., Rapport et al., 2008; Sarver et al., 2015). Participants were presented with a series of jumbled numbers and a capital letter on a laptop. Each number and letter (4 cm height, font Arial) appeared on the screen for 800 ms, followed by a 200 ms interstimulus interval. The letter was never presented in the first or last position of the sequence to minimize potential primacy and recency effects and was counterbalanced across trials to appear an equal number of times in the other serial positions (i.e., position 2, 3, 4, or 5). Participants were instructed to verbally recall the numbers in order from smallest to largest and ending with the letter (e.g., the sequence 3 H 5 8 is correctly recalled as 3 5 8 H). See Figure 1 for a graphical representation of the phonological WM task.
Each participant was administered the phonological task at four different cognitive loads (i.e., phonological set sizes consisting of 3, 4, 5, and 6 stimuli) across the two testing sessions. The four WM set size conditions, each contained 24 unique trials of the same stimulus set size, were counterbalanced across the two testing sessions to control for order effects and potential proactive interference effects across set size conditions (Conway et al., 2005). Before the start of the task, five practice trials with four stimuli were given to the participants. The research assistant would let the student practice until the 80% correct criteria were achieved.
Two trained research assistants, shielded from the participant’s view, recorded the oral responses. Uncertainties and discrepancies regarding the oral responses were overcome via audio-video review. Performance data were calculated using partial-credit unit scoring (proportion of stimuli correct per trial) due to its preferred psychometric properties relative to all-or-nothing scoring (Conway et al., 2005) and control for differences in the number of stimuli available for recall across the set sizes.
Task Evaluation
After task completion, participants were asked to rate their subjective task experience on aspects of difficulty (“How difficult was the task?”), enjoyment (“How much did you enjoy the task?”), and effort (“How much effort did you invest during the task?”) on a nine-point Likert scale varying from 1 (very, very low) to 9 (very, very high).
Results
Preliminary Analyses
To check for potential confounding effects of counterbalancing the order of the cycling condition, we ran an additional 2 × 4 mixed ANOVA on proportion correct score where session order (counterbalancing order: seated-rest first, cycling first) was included in addition to the factors of our main analysis. Results showed no significant interaction effects on proportion correct of counterbalancing order with cycling condition, F(1, 16) = 0.075, p = 0.788, η2partial = 0.005, indicating that counterbalancing order did not impact the effect of our experimental manipulation.
Phonological Working Memory
Descriptive statistics are shown in Table 1. The hypothesis that participants would perform significantly better on the WM task while cycling as compared to seated-rest was tested with a one-way repeated measures ANOVA. The dependent variable was task performance (i.e., total test scores), whereas the independent variable was test condition (i.e., cycling vs. seated-rest). When compared task performance while cycling (M = 0.775, SD = 0.147) with task performance while sitting (M = 0.763, SD = 0.139), results indicated that there was no statistically significant difference and thus no statistically significant effect of cycling on task performance compared to sitting; t(17) = − 0.614, p = 0.548, d = 0.08. This result demonstrates that students performed on a very similar level on the cognitive task despite the change in test conditions.
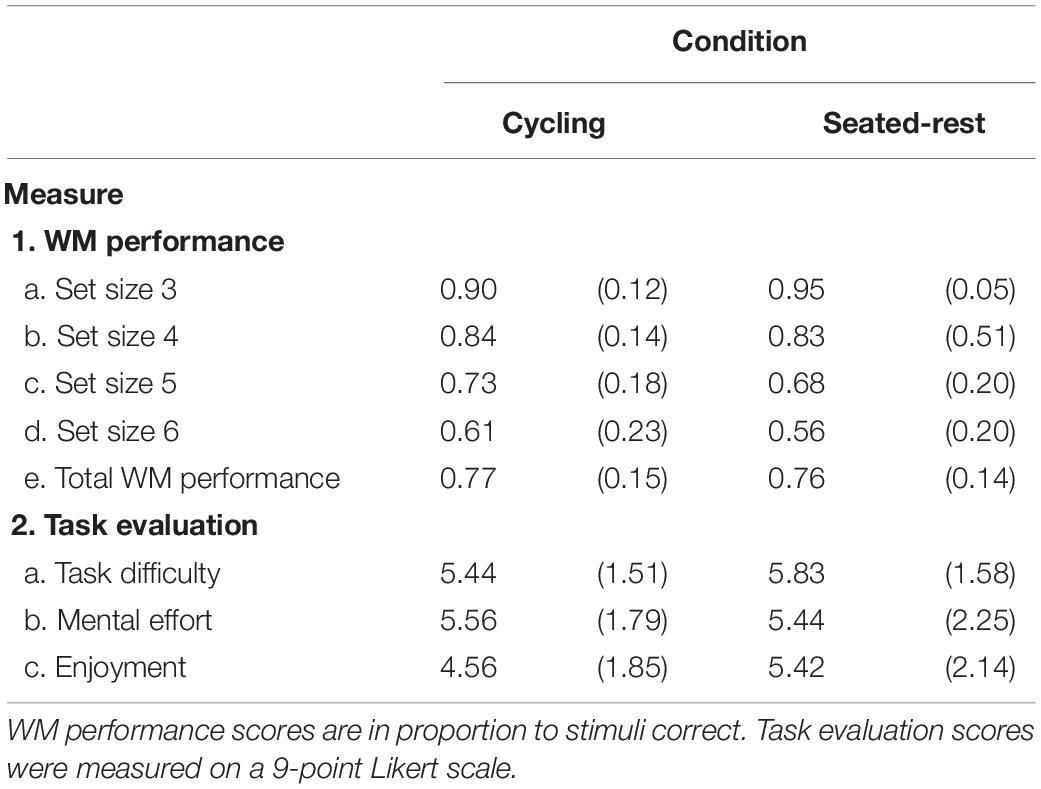
Table 1. Means (SDs) of working memory (WM) performance and task evaluation measures across conditions.
We also looked at the effect of condition on the WM task at four different cognitive loads (i.e., phonological set sizes consisting of 3, 4, 5, and 6 stimuli). Mauchly’s test indicated that the assumption of sphericity had been violated for the main effect of WM scores, χ2 (5) = 13.94, p = 0.016. Therefore, degrees of freedom were corrected using Greenhouse-Geisser estimates of sphericity (ε = 0.66). There was a significant main effect of WM set size on task performance, F(1.98, 33.64) = 58.06, p < 0.001, η2partial = 0.77, with higher set sizes corresponding with decreased performance (see Table 1). There was no significant main effect of condition on task performance, F(1, 17) = 0.68, p = 0.421, η2partial = 0.04. Mauchly’s test also indicated that the assumption of sphericity had been violated for the interaction effect of WM Scores x Condition, χ2 (5) = 18.51, p = 0.002. Again, degrees of freedom were corrected using Greenhouse-Geisser estimates of sphericity (ε = 0.62). There was a significant interaction effect between condition and WM set size scores, F(1.86, 31.71) = 3.50, p = 0.045, η2partial = 0.17. This suggests that conditions had different effects on participants’ scores depending on which set size was given.
To explore this interaction as depicted in Figure 2, two paired sample t-tests were performed comparing the low (i.e., set sizes 3 and 4) and high (i.e., set sizes 5 and 6) WM set sizes across different conditions. The first paired sample t-test comparing cycling and seated-rest during WM set sizes 3 and 4 revealed no difference between the scores for seated-rest (M = 1.78, SD = 0.19) and cycling (M = 1.74, SD = 0.26), t(17) = 1.11, p = 0.284, d = 0.02. Another t-test compared the scores at WM set sizes 5 and 6 revealed a marginally significant difference between cycling (M = 1.35, SD = 0.36) and seated-rest (M = 1.24, SD = 0.38), t(17) = 2.07, p = 0.054, d = 0.08. Taken together, these results point in the direction of a specific trend: at low levels of load, WM performance is unaffected by cycling, but when set sizes increase, cycling seems to result in higher WM scores as compared to seated-rest.
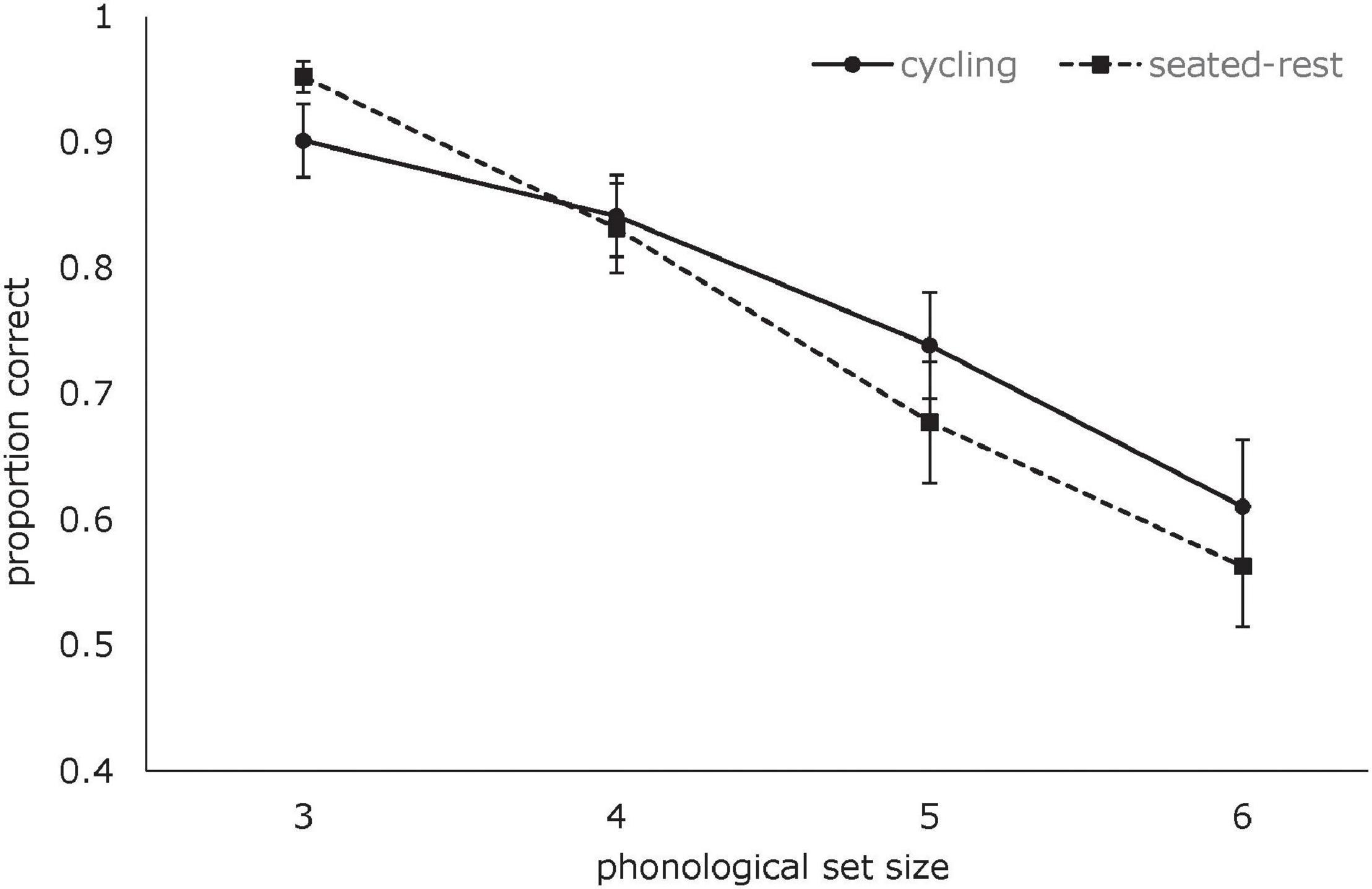
Figure 2. Proportion correct at 4 phonological set sizes. Error bars represent standard error of the mean.
Task Evaluation
Due to the ordinal nature of the task evaluation scores, a Wilcoxon Signed Ranks Test was conducted to compare the effects of both conditions on the dependent variables “task difficulty,” “enjoyment,” and “mental effort.” No significant differences were found between conditions for task difficulty (Z = −0.960, p = 0.337), enjoyment (Z = −1.745, p = 0.081), or mental effort (Z = −0.086, p = 0.932). Consequently, cycling during the WM task did not make a statistically significant difference for participants’ rating of task difficulty, enjoyment, and effort as compared to seated-rest. Hence, the task evaluation scores do not offer an explanation for the results.
Discussion
This study is the first one to examine whether physical activity in the form of stationary cycling can be used in the classroom to help adolescents diagnosed with ADHD perform cognitively demanding tasks. Based on literature pointing toward a positive association between motor activity and cognitive control for adolescents with ADHD (Drollette et al., 2014; Sarver et al., 2015; Hartanto et al., 2016), we hypothesized that exercise in form of cycling during a phonological WM task would result in increased WM performance. Yet, no statistically significance of cycling was found on total WM performance. The present study therefore fails to substantiate the assumption that facilitating physical activity helps adolescents with ADHD improve their WM performance. This result is in line with several previous studies failing to find a beneficial effect of active workstations on cognitive functioning (Bantoft et al., 2016; Torbeyns et al., 2017). It is important to note, however, that these earlier results have arrived with a different population, namely, adults or typically developing children and not children or adolescents diagnosed with ADHD.
As an additional exploratory analysis, we also looked at the effect of condition on WM task performance at low and high levels of the cognitive load instead of total WM performance. Data revealed that at low levels of load (i.e., set sizes 3 and 4), seated-rest and cycling resulted in comparable WM scores, whereas cycling resulted in better WM performance when set sizes increase (i.e., set sizes 5 and 6).
This result is in line with the study of Hartanto et al. (2016) who found that higher rates of spontaneous motor activity predicted significantly better performance on inhibitory control. In their study, participants’ movements were measured by affixing an actometer to their ankles that measured their level of activity while completing the flanker test. The accuracy of the participants with ADHD was significantly improved when they were moving. In other words, correct answers were associated with more motion than incorrect answers. This result suggests that accuracy in ADHD may be enhanced by more intense activities or that when a child with ADHD is using more cognitive resources they are more likely to be engaging in physical activity. One possible mechanism for a relationship between movement and cognitive performance is that children with ADHD use movement to self-regulate alertness. An optimal level of arousal is required for peak cognitive performance (Yerkes and Dodson, 1908). The results of the present investigation and the study of Hartanto et al. (2016) support Rapport et al.’s (2009) model that hyperactivity may serve to compensate for underarousal and acts to improve cognitive performance.
Another explanation for this result can be found in the fact that cycling on a desk bike is a very specific form of structured, continuous movement with the lower body. Possibly, this type of movement will prevent adolescents with ADHD from making more uncontrolled movements with the upper body (e.g., arm movements) that are unrelated to the task at hand and that negatively affect task performance, since they take away the attention necessary for well-performing a task under high WM load conditions (Engle, 2002). More specifically, cycling movements have the potential to channel the surplus of motor activity common to adolescents with ADHD, while keeping a focus on the challenging cognitive task at hand. As a result, performance under high WM load conditions will be increased, while no such performance gains are obtained in tasks under low WM conditions. This explanation resonates well with findings that continuous body movements, as opposed to discrete movements, increase performance on WM tasks under conditions of high cognitive load (Maes et al., 2015). More research is needed to shed light on how task difficulty moderates the potential compensatory effect of cycling on task performance, especially, in children with ADHD. These findings have important implications for the educational field or clinical practice. Desk bikes can be implemented in the classroom to help manage students’ hyperactivity symptoms and boost their performance in the classroom on cognitively demanding tasks, without interrupting, or distracting peers or teachers in the classroom.
Limitations and Future Studies
In the present study, cycling was self-paced and mandatory. While the small sample size with the presence of comorbid diagnosis may limit the study’s ability to draw robust conclusions, the study is a first step toward examining the effect of concurrent light-intensity exercise on cognitive control. Future studies should replicate the study using a cadence sensor and multiple actigraph devices to gain a deeper insight into activity patterns of the upper and lower body associated with adolescents with ADHD. In addition, the inclusion of a cadence sensor in future studies can also shed light on the complex interplay between motor activity and different WM demands both during conditions of seated-rest and cycling. It would also be interesting to investigate whether mandatory or self-regulated cycling would differentially affect WM performance, since in the latter situation one can manage one’s own cognitive load.
Another limitation of the present study is that we exclusively looked at one single phonological WM task and we did not employ pre- and post-test experimental designs. Due to the diffuse nature of the WM construct, multiple tasks tapping the same construct but differing in other respects (e.g., design, modality) would provide a more robust outcome measure than using a single test alone. For instance, one can use the Digit span task, another common neuropsychological test in the WISC-IV battery to assess auditory WM. This task involves repeating a sequence of numbers in the same order right after they have been read aloud. This test differs from the phonological WM test, in which a screen was used to present the numbers and letters. Another essential step would be to investigating the relationship between visuospatial WM and physical activity. This is especially important, since evidence points out that concurrent motor movements, such as finger tapping (e.g., Della Sala et al., 1999), have been shown to produce interference with this aspect of WM. In addition, by using pre- and post-test experimental designs, future studies could also verify if desk-bike cycling increases cognitive performance at an individual level. By doing so, we will be able to investigate different parameters, such as intensity, type of task, individual characteristics, and self-initiation, to understand the mechanism underlying the effect of spontaneous physical activity.
This study was conducted in a controlled classroom setting with limited distractions over a short time period. We recommend follow-up studies with a larger sample size to investigate the long-term effects of desk bikes in a more naturalistic environment. It could not only be investigated whether movement on a desk bike translates to improved WM, which is critical for several academic tasks (Alloway and Alloway, 2010), but more importantly, whether it leads to improved academic attainment among adolescents with ADHD.
Data Availability Statement
The raw data supporting the conclusions of this article will be made available by the authors, without undue reservation.
Ethics Statement
The studies involving human participants were reviewed and approved by the Ethics Review Committee at the Department of Psychology, Education and Child Studies of Erasmus School of Social and Behavioral Sciences. Written informed consent to participate in this study was provided by the participants’ legal guardian/next of kin.
Author Contributions
MR, EG, SL, and FP conceived and planned the experiments. MR and EG carried out the experiments. MR, SL, JW, and FP contributed to the writing of the manuscript. SL and FP supervised the project. All authors provided critical feedback and helped shape the research, analysis, and manuscript.
Conflict of Interest
The authors declare that the research was conducted in the absence of any commercial or financial relationships that could be construed as a potential conflict of interest.
Publisher’s Note
All claims expressed in this article are solely those of the authors and do not necessarily represent those of their affiliated organizations, or those of the publisher, the editors and the reviewers. Any product that may be evaluated in this article, or claim that may be made by its manufacturer, is not guaranteed or endorsed by the publisher.
Footnotes
- ^ The term “cognitive control” refers to processes that allow information processing and behavior to vary adaptively depending on current goals and include interrelated processes of inhibition, working memory (WM), and cognitive flexibility (Diamond, 2013).
References
Alderson, R. M., Rapport, M. D., Kasper, L. J., Sarver, D. E., and Kofler, M. J. (2012). Hyperactivity in boys with attention deficit/hyperactivity disorder (ADHD): the association between deficient behavioral inhibition, attentional processes, and objectively measured activity. Child Neuropsychol. 18, 487–505. doi: 10.1080/09297049.2011.631905
Alloway, T. P., and Alloway, R. G. (2010). Investigating the predictive roles of working memory and IQ in academic attainment. J. Exp. Child Psychol. 106, 20–29. doi: 10.1016/j.jecp.2009.11.003
Baddeley, A. (2007). Working Memory, Thought, and Action, Vol. 45. New York, NY: Oxford University Press Inc.
Bantoft, C., Summers, M. J., Tranent, P. J., Palmer, M. A., Cooley, P. D., and Pedersen, S. J. (2016). Effect of standing or walking at a workstation on cognitive function: a randomized counterbalanced trial. Hum. Factors 58, 140–149. doi: 10.1177/0018720815605446
Butte, N. F., Christiansen, E., and Sørensen, T. I. (2007). Energy imbalance underlying the development of childhood obesity. Obesity 15, 3056–3066. doi: 10.1038/oby.2007.364
Castelli, D. M., Hillman, C. H., Buck, S. M., and Erwin, H. E. (2007). Physical fitness and academic achievement in third-and fifth-grade students. J. Sport Exerc. Psychol. 29, 239–252. doi: 10.1123/jsep.29.2.239
Conway, A. R., Kane, M. J., Bunting, M. F., Hambrick, D. Z., Wilhelm, O., and Engle, R. W. (2005). Working memory span tasks: a methodological review and user’s guide. Psychon. Bull. Rev. 12, 769–786. doi: 10.3758/bf03196772
Corkum, P., Tannock, R., and Moldofsky, H. (1998). Sleep disturbances in children with Attention-Deficit/Hyperactivity Disorder. J. Am. Acad. Child Adolesc. Psychiatry 37, 637–646.
Della Sala, S., Gray, C., Baddeley, A., Allamano, N., and Wilson, L. (1999). Pattern span: a tool for unwelding visuo–spatial memory. Neuropsychologia 37, 1189–1199. doi: 10.1016/s0028-3932(98)00159-6
Dickstein, S. G., Bannon, K., Xavier Castellanos, F., and Milham, M. P. (2006). The neural correlates of attention deficit hyperactivity disorder: an ALE meta-analysis. J. Child Psychol. Psychiatry 47, 1051–1062. doi: 10.1111/j.1469-7610.2006.01671.x
Donnelly, J. E., Hillman, C. H., Castelli, D., Etnier, J. L., Lee, S., Tomporowski, P., et al. (2016). Physical activity, fitness, cognitive function, and academic achievement in children: a systematic review. Med. Sci. Sports Exerc. 48, 1197–1222.
Drollette, E. S., Scudder, M. R., Raine, L. B., Moore, R. D., Saliba, B. J., Pontifex, M. B., et al. (2014). Acute exercise facilitates brain function and cognition in children who need it most: an ERP study of individual differences in inhibitory control capacity. Dev. Cogn. Neurosci. 7, 53–64. doi: 10.1016/j.dcn.2013.11.001
Engle, R. W. (2002). Working memory capacity as executive attention. Curr. Dir. Psychol. Sci. 11, 19–23.
Hartanto, T. A., Krafft, C. E., Iosif, A. M., and Schweitzer, J. B. (2016). A trial-by-trial analysis reveals more intense physical activity is associated with better cognitive control performance in attention-deficit/hyperactivity disorder. Child Neuropsychol. 22, 618–626. doi: 10.1080/09297049.2015.1044511
Hillman, C. H., Erickson, K. I., and Kramer, A. F. (2008). Be smart, exercise your heart: exercise effects on brain and cognition. Nat. Rev. Neurosci. 9, 58–65. doi: 10.1038/nrn2298
Hillman, C. H., Snook, E. M., and Jerome, G. J. (2003). Acute cardiovascular exercise and executive control function. Int. J. Psychophysiol. 48, 307–314. doi: 10.1016/s0167-8760(03)00080-1
Hudec, K. L., Alderson, R. M., Kasper, L. J., and Patros, C. H. G. (2014). Working memory contributes to elevated motor activity in adults with ADHD: an examination of the role of central executive and storage/rehearsal processes. J. Atten. Disord. 18, 357–368. doi: 10.1177/1087054713497398
Institute of Medicine (2013). Educating the Student Body: Taking Physical Activity and Physical Education to School. Washington, DC: The National Academies Press.
Kibbe, D. L., Hackett, J., Hurley, M., McFarland, A., Schubert, K. G., Schultz, A., et al. (2011). Ten Years of TAKE 10!® : integrating physical activity with academic concepts in elementary school classrooms. Prev. Med. 52, S43–S50. doi: 10.1016/j.ypmed.2011.01.025
Maes, P. J., Wanderley, M. M., and Palmer, C. (2015). The role of working memory in the temporal control of discrete and continuous movements. Exp. Brain Res. 233, 263–273. doi: 10.1007/s00221-014-4108-5
Mahar, M. T., Murphy, S. K., Rowe, D. A., Golden, J., Shields, A. T., and Raedeke, T. D. (2006). Effects of a classroom-based program on physical activity and on-task behavior. Med. Sci. Sports Exerc. 38, 2086–2094. doi: 10.1249/01.mss.0000235359.16685.a3
Mavilidi, M., Ruiter, M., Schmidt, M., Okely, A. D., Loyens, S., Chandler, P., et al. (2018). A narrative review of school-based physical activity for enhancing cognition and learning: the importance of relevancy and integration. Front. Psychol. 9:2079. doi: 10.3389/fpsyg.2018.02079
National Association for Sport and Physical Education (2008). Comprehensive School Physical Activity Programs, Position Statement. Reston, VA: National Association for Sport and Physical Education.
Ng, S. W., and Popkin, B. M. (2012). Time use and physical activity: a shift away from movement across the globe. Obes. Rev. 13, 659–680. doi: 10.1111/j.1467-789X.2011.00982.x
Pontifex, M. B., Saliba, B. J., Raine, L. B., Picchietti, D. L., and Hillman, C. H. (2013). Exercise improves behavioral, neurocognitive, and scholastic performance in children with attention-deficit/hyperactivity disorder. J. Pediatr. 162, 543–551. doi: 10.1016/j.jpeds.2012.08.036
Rapport, M. D., Alderson, R. M., Kofler, M. J., Sarver, D. E., Bolden, J., and Sims, V. (2008). Working memory deficits in boys with attention-deficit/hyperactivity disorder (ADHD): the contribution of central executive and subsystem processes. J. Abnorm. Child Psychol. 36, 825–837. doi: 10.1007/s10802-008-9215-y
Rapport, M. D., Bolden, J., Kofler, M. J., Sarver, D. E., Raiker, J. S., and Alderson, R. M. (2009). Hyperactivity in boys with attention-deficit/hyperactivity disorder (ADHD): A ubiquitous core symptom or manifestation of working memory deficits? J. Abnorm. Child Psychol. 37, 521–534. doi: 10.1007/s10802-008-9287-8
Ruiter, M., Eielts, C., Loyens, S., and Paas, F. (2019). Comparing cognitive control performance during seated rest and self-paced cycling on a desk bike in preadolescent children. J. Phys. Act. Health 16, 533–539. doi: 10.1123/jpah.2017-0437
Ruiter, M., Görlich, E., Loyens, S., and Paas, F. (2017). The effects of cycling on a desk bike on attention, retention and mood during a video lecture. Appl. Cogn. Psychol. 31, 593–603.
Sarver, D. E., Rapport, M. D., Kofler, M. J., Raiker, J. S., and Friedman, L. M. (2015). Hyperactivity in attention-deficit/hyperactivity disorder (ADHD): Impairing deficit or compensatory behavior? J. Abnorm. Child Psychol. 43, 1219–1232. doi: 10.1007/s10802-015-0011-1
Teicher, M. H., Polcari, A., Fourligas, N., Vitaliano, G., and Navalta, C. P. (2012). Hyperactivity persists in male and female adults with ADHD and remains a highly discriminative feature of the disorder: a case-control study. BMC Psychiatry 12:190. doi: 10.1186/1471-244X-12-190
Torbeyns, T., Bailey, S., Bos, I., and Meeusen, R. (2014). Active workstations to fight sedentary behaviour. Sports Med. 44, 1261–1273. doi: 10.1007/s40279-014-0202-x
Torbeyns, T., de Geus, B., Bailey, S., Decroix, L., Van Cutsem, J., De Pauw, K., et al. (2017). Bike desks in the classroom: energy expenditure, physical health, cognitive performance, brain functioning, and academic performance. J. Phys. Act. Health 14, 429–439. doi: 10.1123/jpah.2016-0224
Wechsler, D. (2003). Wechsler Intelligence Scale for Children–Fourth Edition (WISC-IV). San Antonio, TX: The Psychological Corporation.
Yerkes, R., and Dodson, J. (1908). The relation of strength of stimulus to rapidity of habit-formation. J. Comp. Neurol. Psychol. 18, 459–482.
Keywords: desk bikes, active workstations, phonological working memory, ADHD, adolescents
Citation: Ruiter M, Görlich E, Loyens S, Wong J and Paas F (2022) Effects of Desk-Bike Cycling on Phonological Working Memory Performance in Adolescents With Attention Deficit Hyperactivity Disorder. Front. Educ. 7:841576. doi: 10.3389/feduc.2022.841576
Received: 22 December 2021; Accepted: 14 February 2022;
Published: 11 March 2022.
Edited by:
Natalie Lander, Deakin University, AustraliaReviewed by:
Antonio Prieto, National University of Distance Education (UNED), SpainVanessa Arán Filippetti, Consejo Nacional de Investigaciones Científicas y Técnicas (CONICET), Argentina
Copyright © 2022 Ruiter, Görlich, Loyens, Wong and Paas. This is an open-access article distributed under the terms of the Creative Commons Attribution License (CC BY). The use, distribution or reproduction in other forums is permitted, provided the original author(s) and the copyright owner(s) are credited and that the original publication in this journal is cited, in accordance with accepted academic practice. No use, distribution or reproduction is permitted which does not comply with these terms.
*Correspondence: Jacqueline Wong, bC55Lmoud29uZ0B0dWRlbGZ0Lm5s