- 1Institute of Education, Primary Initial Teacher Education Department, University of Worcester, Worcester, United Kingdom
- 2Department of Education, Faculty of Humanities, Social Sciences and Education, UiT the Arctic University of Norway, Tromsø, Norway
This three-year longitudinal case study focused on the deployment of mobile technology in the form of tablet computers (iPads), during Inquiry Based Science Education (IBSE). The research took place in a larger than average primary school in the West Midlands, UK, which showed a strong commitment to Technology Enhanced Learning (TEL) resulting in iPads being used as an integral learning tool, across the entire curriculum. During the research, pupils in Upper Key Stage Two (10–11 year olds) were observed taking part in science weeks which consisted of intense periods of science inquiry, much of which was child-led. The impact of the embedded use of iPads was monitored by scrutinising pupils’ work in the form of multimedia presentations and experimental reports. Pupils’ learning behaviours and attitudes to mobile technology were explored through observations and paired interviews. The embedded use of iPads during IBSE was shown to increase science knowledge acquisition and support scientific literacy, recording of processes and aid understanding of working scientifically. Furthermore, iPads were shown to afford opportunities for personalisation of scientific learning experiences and foster collaboration at several levels, factors which were highly valued by the pupils. The outcomes of this study can be used to further inform the refinement of m-learning strategies in primary science and illuminate opportunities for developing the practice of science pedagogues.
Introduction
Pupils who are being educated now in primary schools will contribute to a legacy of scientific knowledge and societal judgements in the 22nd century, and as such will require strong scientific literacy skills and a deep understanding of all three disciplines. This aspiration is somewhat problematic in England given that the profile of primary science in the curriculum is undoubtedly diminished (Wellcome Trust, 2011, 2014; OFSTED, 2011, 2014). Hence, UK stakeholders have argued that this situation requires urgent remediation in terms of policy change in order to improve opportunities within primary science education (Economic and Social Research Council, 2013; Science Community Representing Education [SCORE], 2013; Wellcome Trust, 2013) and encourage learners to see themselves as potential scientists of the future. Since science capital (based on social capital theory and defined as the tendency of pupils to believe they can make a scientific contribution to society), is thought to build during the formative primary stages of education (STEM Learning, 2019); it is essential to commit to further refine the effectiveness of science pedagogy throughout early formal education for all learners (Harlen, 2010; Lievesley, 2014). It is argued (Duschl et al., 2007) that in a technologically advanced society, learning should not be focused merely on the recall of facts but rather the development of a deep understanding of the nature of science and its associated methodology. Therefore, the emphasis should be placed firmly on exploration and research skill development, enabling future generations of scientists to create global solutions (United Nations Education Scientific and Cultural Organisation [UNESCO], 2017).
There is a consensus of opinion that in order for science education to be effective it needs to be undertaken from the perspective of pupils’ experiences of the world around them (Worth, 2010) and to some degree be responsive to the findings that pupils value both guided and independent means to study (Lau et al., 2017). In this way, pupils’ natural curiosity can be harnessed during exploratory inquiry, which can lead to more formalised learning. Following the initial phase of scientific curiosity, it is necessary for pupils to plan, record, analyse and share their inquiries, traditionally this was achieved using paper and pen methods. In the last decade there has been a change in the way pupils communicate with each other and teachers in the primary classroom, with the prevalent use of mobile technology (m-technology). Whilst many studies across the primary curriculum including mathematics (Hilton, 2018), literacy (Lynch and Redpath, 2014; Browder et al., 2017; Bergeson and Rosheim, 2018) and the humanities (Monem et al., 2018) have highlighted the possibilities of deploying m-technology, in the majority of cases these have focused on realising learning outcomes and teacher perspectives (Boon et al., 2021).
Studies specifically focusing on the pedagogical affordances of m-technology during science inquiry are less prevalent and generally do not concern the attitudes of the pupils themselves to this mode of learning (Wang et al., 2022). Hence, this study focuses on primary age pupils in upper Key Stage Two (years 5 and 6, pupils aged 10–11 years old) where they are establishing the beginnings of scientific inquiry, and in addition experiencing a crucial formative stage during which they may start to regard themselves as scientists of the future.
Pedagogical framework: Inquiry Based Science Education (IBSE) and an inquiry-based approach
The key focus of this study relies on the use of an established pedagogy, namely Inquiry Based Science Education (IBSE), supplemented by the deployment of mobile technology (m-technology). Specifically, the research aims to explore the potential synergies between IBSE as facilitated by m-technology, to enable deeper understanding of scientific inquiry in the primary classroom, from the perspective of the learner.
There is a large body of work devoted to IBSE, and in general the evidence suggests it is a highly effective method of engaging learners with scientific discovery and key scientific concepts (Berg et al., 2003; Leonard et al., 2009; Furtak et al., 2012; van Uum et al., 2016). However, closer examination of the literature reveals that the situation is not totally clear-cut (Rönnebeck et al., 2016). In his meta-analysis, Anderson (2002) highlights whilst the effectiveness of this type of pedagogy is generally agreed, researchers tend to define IBSE with respect to their own particular research context. Therefore, there can be subtle differences in what researchers believe constitutes inquiry-based learning opportunities. For this study the definition honed by Linn et al. (2004), p. 4 is adopted, since it concisely summarises the key features of IBSE as “The intentional process of diagnosing problems, critiquing experiments, and distinguishing alternatives, planning investigations, researching conjectures, searching for information, constructing models, debating with peers, and forming coherent arguments.”
This is an extensive delineation which adheres to the epistemology of advanced scientific study and research. Initial contemplation might suggest this as an overly ambitious approach for primary education but in fact, both the nature and process associated with science have already been demonstrated to be effectively explored within inquiry-based approaches (Bianchini and Colburn, 2000). In breaking down the definition further, it can be seen that the first four components: problematising, critiquing experiments, distinguishing alternatives and subsequent planning investigations, are all integral elements of the “working scientifically” portion of the National Curriculum for Science in England (DfE, 2013, 2015).
Such are the benefits of this pedagogic approach, that inquiry-based approaches have been adopted by curriculum designers in Australia, USA, Middle East, Asia, and Europe (Abd-El-Khalick, 2003; National Curriculum Board of Australia, 2009; National Research Council, 2012), as a means of fostering and developing scientific knowledge and skills. Inquiry-based science teaching affords learners opportunities to not only engage in valuable hands-on/minds on learning experiences (Minner et al., 2010) but also develop science specific content knowledge (Sandoval, 2005) and cultivate a far-reaching appreciation of the nature of scientific discovery (Schwartz et al., 2004).
For an inquiry based approach to be effective, scientific discovery needs to be made accessible to all pupils and not perceived to be only undertaken by people of certain cultural or educational backgrounds (Gibbs, 2014). Learners also need to be able to engage with a range of development opportunities to hone their scientific skills or in simplistic terms work like a scientist (Archer et al., 2010; DfE, 2013, 2015). A range of inquiries, including fair or comparative testing, pattern recognition, secondary research and problem-solving should be undertaken to allow pupils to improve these key skills which represent “working scientifically” (Turner et al., 2011) in a similar way to a scientist. García-Carmona (2020, p. 448) citing National Research Council (1996, pp. 137–138), develops this idea further and advocates positioning inquiry-based approaches as being explicitly linked to “what scientists do” and highlights “students should evaluate their own results or solutions to problems, as well as those of other children” and consider “alternative explanations” both of which, mirror the processes that scientists undertake during dissemination of their ideas and peer review. Encouraging children to see themselves as scientists, also confers the added advantage of increasing pupils’ science capital which can be a positive indicator for sustained science study in post-16 education (Archer et al., 2015).
A significant number of components of the outlined process, also represent typical constructivist-based learning activities within the primary classroom (Hackling and Prain, 2008; OFSTED, 2011). In addition, proponents of this approach point out that an inquiry based science is highly accessible to primary school pupils and can be carried out almost everywhere: in non-specialist classrooms, outdoors and even at home with parental supervision at a low cost (Blacklock, 2012; Lee, 2012). It is important to note however that practical experience alone is not enough to secure deep understanding of methodology or scientific principles (Bransford et al., 2000) but rather “wrap around” thinking as a result of initial research, trialing, analysis and discussion is required to substantiate learning during inquiry (Pedaste et al., 2015).
Potential barriers during investigational primary science
In England, upper KS 2 pupils (10–11-year olds) are introduced to the scientific method through the concept of “fair testing” which encourages the development of a logical and systematic approach (DfE, 2013) as a component of IBSE. Teachers can act as mediators of this approach by encouraging careful observation and the formulation of authentic child-led questions (Keys and Bryan, 2001; OFSTED, 2011, p. 15). They can also model a collaborative approach to inquiry in their classrooms, supported by extensive opportunities for in-depth discussion and peer review (OFSTED, 2011, p. 638). Teachers arguably have a pivotal role in sustaining pupil interest and engagement by prompting deep thinking using extensive classroom discourse (Crawford, 2000).
Whilst IBSE teaching approaches have been judged to be efficacious (Chang and Mao, 1999; Anderson, 2002; Wilson et al., 2009; Minner et al., 2010) there are inherent practical problems associated with such methodologies with young learners. Central to these challenges is the fact that primary aged pupils are often engrossed in the practical elements of inquiries and hence reluctant to pause and document their observations. To facilitate effective learning, these outcomes need to be captured in a time efficient manner, so that pupils do not miss key events. They also need to assimilate the process of planning and carrying out investigations, so they can develop confidence in setting up future inquiries. Often in the overriding quest to see what happens, the implementation of a systematic and fair testing regime is overlooked and potentially forgotten. Mobile technologies that are able to capture in-the-moment scientific phenomena and processes (Clark and Luckin, 2013) could therefore be valuable tools in improving effective metacognition of operational scientific experimentation. Additionally, they afford learners endless opportunities for research and analysis, by collating and processing a range of data including multi-media outputs (Clark and Luckin, 2013) and acting as a vital conduit for the requirement to communicate their ideas confidently (van der Graaf, 2020). M-technology deployment during science inquiry may also afford teachers the opportunity to share key experimental outcomes with children after the investigation itself, when there is less time pressure (Burden et al., 2016).
Technology enhanced learning using mobile technologies
Classic TEL approaches have been used to support scientific inquiry for some time according to Kim et al. (2007, p. 1017) who describe their potential as being able to provide: ‘technology-enhanced, student-centred, flexible opportunities’ during inquiry processes. However, due to the physical space required by stand-alone computers and laptops, they are challenging to integrate closely into scientific investigations, resulting in their use being restricted to initial research phases or subsequent analysis. In contrast, there is the more recent phenomenon of rapid adoption of mobile learning (m-learning) in primary schools, much of it mediated by the use of tablet computers (Boon et al., 2021). Research in different age phases including early years education, Lynch and Redpath (2014); Burden and Kearney (2016); Song and Wen (2018) suggests that due to their portability, multi-modal nature and ease of use, m-technologies may give rise to additional unique learning opportunities. Such learning prospects have been described by the iPAC (i Personalise, Authenticity and Collaboration) framework as developed by Burden and Kearney (2016). This model describes how m-technologies can be used by learners to personalise experiences, improve or make explicit the authenticity of operation and encourage effective collaboration during experiential learning.
Each of the three main constructs (P, A, and C) are characterised by sub constructs e.g., personalisation of the learning experience, which may give rise to an increase in agency; where pupils record and later discuss outcomes in their own way. This positive psychological aspect in conjunction with the improved motivation associated with inquiry-based approaches (Justice et al., 2009) may act as a powerful enabler in science learning. Through multimedia experiences (documentaries, YouTube video-clips, virtual reality laboratories) pupils are becoming more aware of what real everyday science looks like. Arguably they are beginning to be critical and make judgements about the legitimacy of scientific experimentation and as such becoming more discerning in experimental design. By giving pupils the choice of embedding m-technologies during the scientific processes, they may consider their learning experiences more credible and from their perspective, authentic.
M-technology as a means of fostering scientific collaboration
In terms of collaboration, scientific discovery and development has always been associated with discourse and peer review. Even at a young age, learners are capable and open to social constructivist learning mechanisms via the processes of data gathering and sharing in science (Dunn et al., 2016 and Furman et al., 2018). Several science pedagogues (Ford and Forman, 2006; Allen, 2010; Harlen, 2010) highlight how crucial it is for learners to be afforded opportunities to engage in high quality learning conversations with their peers and teachers about their scientific discoveries. During, and as a result of these conversations misconceptions may be revealed and challenged, resulting in improved understanding and remodelling of conceptually difficult ideas (Allen, 2010). A systematic review of the use of m-technology during science learning by Afikah et al. (2022) highlights the increased social interactions evident using such an approach and enhanced opportunities for pupils to develop their problem-solving skills.
Research questions
Since the aforementioned literature suggests that pupils are able to take the lead in the classroom, collaborate together and socially construct their emerging scientific ideas, this study seeks to explore in depth the enactment of IBSE using m-technology from a learner perspective and focuses on the following research questions:
(i) In what ways may iPads be used to support inquiry-based learning including planning, recording and working scientifically?
(ii) What learning behaviours and attitudes do primary aged pupils display toward iPad use during IBSE?
Materials and methods
Research context
The research setting was a larger than average primary school (400+ pupils, taught by approximately 40 teaching and support staff) in the West Midlands region of England. The school was newly built prior to the study and as a result, pupils had access to a wide range of high-quality information and communication technology (ICT) equipment, including tablet computers (iPads). The research focused on Upper Key Stage 2 (UKS2) pupils (10–11 year olds) where each of the 60 pupils in year 5 (10-year olds) had access to their own iPad tablet computer and each of the 60 pupils in year six (11-year olds) shared an iPad between two learners. Since the pupils used iPads every day in a range of lessons, they were very familiar with operating them and aware of the options to use different apps for writing, video capture and drawing etc.
The study took place during science weeks, which were characterised by periods of intense scientific inquiry by all pupils. During these weeks the normal school timetable was suspended and rather than it represent the usual mix of curriculum subjects including core subjects (English and mathematics) and foundations subject (e.g., geography, history or religious education), the pupils exclusively studied science for approximately 25 h per week. These science weeks involving at least eight different inquiries, took place in addition to the usual science lessons at six monthly intervals over the space of three consecutive academic years. This initiative aimed to enable pupils to improve their scientific skills and knowledge bases, in a subject which has seen a reduced profile in primary schools since the removal of statutory testing in 2009 (Wellcome Trust, 2014).
The analysis of pupils’ work was aided by the school’s sophisticated data management system, which allowed tracking of attainment within a large database of pupils’ work. Due to the integrated learning and assessment infrastructure using m-technology, this school was judged to be highly suitable for this case study.
Expectations of pupils’ learning during the science weeks
In addition to learning appropriate content knowledge during their science study across all three science disciplines, pupils were required to acquire disciplinary knowledge in terms of working scientifically. According to the requirements of the National Curriculum for England: Science Programmes of Study (DfE, 2015, p. 25) pupils learn to follow the scientific method. Hence the pupils worked logically and sequentially through the scientific processes of planning, taking measurements, recording data and results, making predictions and reporting and presenting findings. In addition, with respect to their developing scientific literacy, they were required to use appropriate scientific language and illustrations to communicate their ideas. In summary, during the science weeks pupils were expected to engage with all the science inquiries by working practically and documenting and sharing their investigational outcomes using the iPads.
Expectations of teachers and leaders during science weeks
Teachers and science/phase leaders adopted an inclusive, child led (Siry et al., 2012) stance within their classrooms, in keeping with the ethos of the school. Specifically, they aimed to articulate and model that anyone can act like and become a scientist, and that all perspectives on approaches and modes of IBSE were valued. Teachers encouraged pupils to ask questions of themselves and others, discuss their points of view and work carefully and methodically. If a pupil required help or guidance that was given by a teacher but they strived to enable progress whilst encouraging independent learning. During the science weeks the teachers and leaders liaised extensively with the researchers to exchange their ideas on what was working well and what if anything, needed development. They also gave feedback to pupils whilst showcasing their work, using interactive white boards to share inquiry reports and presentations.
Research ethics
The research was undertaken from a perspective of minimising the researcher footprint and according to best practice as defined by British Educational Research Association (2018). In short, participants were approached via the deputy head of school who acted as a gatekeeper for the study and issued effective communication of the purpose and expectations of the study. Both parental written consent and on-going pupil assent to take part was sought. Participants were regularly reminded of their right to withdraw from the study, although none chose to do so. Confidentiality and anonymity were preserved at all times.
Participants and sampling
During each phase of the study across three academic years, samples of work were taken from thirty pupils from each year group (sixty pupils in total), which represented data from half of all learners in UKS2. The purposive sample represented a mix of gender, ethnicity and academic attainment. In addition, twelve pairs of pupils were interviewed from each cohort, each year to determine their perspectives and attitudes to learning. The data from pupils who worked both in pairs or singly were used for data analysis purposes.
Data collection instruments
(i) Semi-structured observations of pupils and teachers during the scientific inquiries
Semi-structured observations took place during all phases of scientific inquiry for both year groups, resulting in over forty hours of observational material being acquired. The schedule was designed using key learning facilitators identified by the seminal work of Burden and Younie (2014) from the Mapping Educationalist Specialist Know-How (MESH) guide in using iPads in the classroom. Such facilitators were: undertaking scientific processes and social interaction, initiating own enquiry strategies, exploiting the mobility of iPads and engagement and motivation during the scientific inquiry. Descriptions of each type of learning behaviour were produced and discussed at length prior to observation to ensure reliability of the measure. Piloting in another school with similar aged pupils and class sizes was used to check for validity.
(ii) Semi-structured interviews with pupils
These were designed according to the strategy outlined by Kvale (1996). Initial general questions were asked about the scientific enquiry as a means of creating rapport and ensuring pupil comfort, for example: “Can you tell me about your work in science this week?” These questions were followed by more probing questions exploring in-depth the use of iPads by the pupils. For example: “Did the iPad help you understand the science? Can you give me an example please?” Pupils were also asked if they used more traditional methods to process and record data, for example handwritten tables.
The interviews which lasted approximately 30 min, were completed by asking questions to discern attitudes toward using iPads during work in science. Frequently during the interviews, responses were read back to the pupils to check for meaning and understanding on the behalf of the researcher. In addition, the majority of pupils chose to bring their iPad to the interviews and illustrated their answers by demonstrating their learning activities recorded as multimodal documents. Notes were taken to record these responses and screen shots taken of the pupils’ work.
Data analysis
The pupil interviews (with accompanying notes detailing when pupils had referred to specific examples of their work on the iPads) were recorded on an additional iPad, transcribed verbatim and, together with the classroom observations, the data were analysed separately using thematic analysis. Systematic analysis was achieved by using NVivo 11 software to support a five-stage process according to the premises of Braun and Clarke (2006). The first phase involved familiarisation of the data by reading and re-reading multiple times. This stage was followed by the generation of initial codes (termed nodes in NVivo 11) from across the entire dataset. Memos were made to ensure the contexts of the codes were retained. Cluster analysis was then undertaken to create thematic maps and highlight potential themes which were then reviewed. A round of confirming codes and then re-coding was undertaken to confirm emergent themes. In some cases, themes were collated into sub themes or merged leading to the definition of final themes. Internal reliability of the data was improved by another independent researcher being involved in the analysis and rechecking codes and themes. The trustworthiness of the findings was enhanced by triangulation (Guba and Lincoln, 1994) across the data from observations, pupils’ work and paired interviews. This process is summarised in Figure 1.
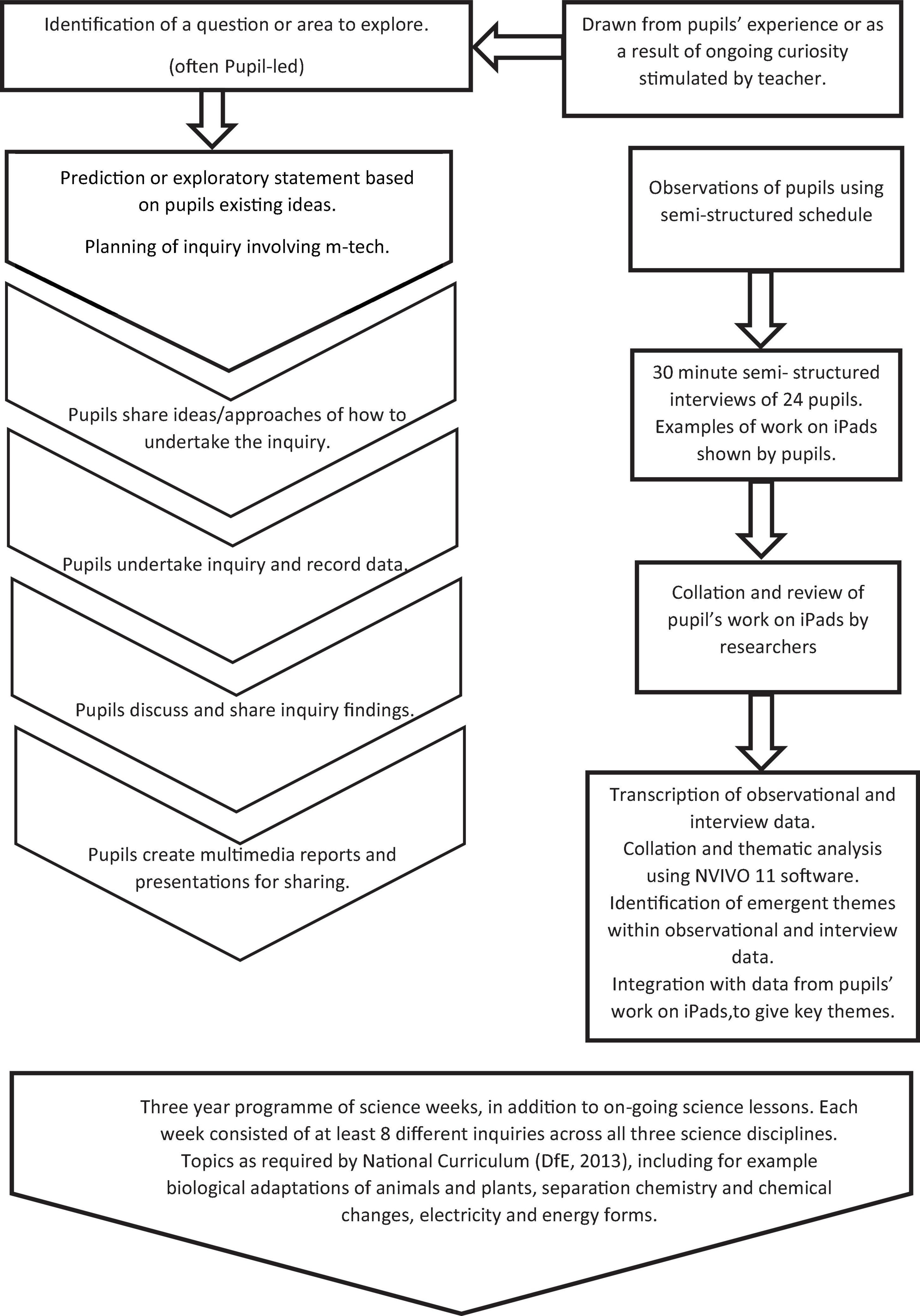
Figure 1. The methodological flow and timeline of the research (informed by Harlen, 2021).
Results
Using the data from the pupil and teacher observations and interviews, three key themes emerged with respect to the first research question as follows:
• Use of apps on iPads to increase science knowledge acquisition and scientific literacy
• Use of iPads to support planning and recording of inquiry processes
• Use of iPads to increase understanding of working scientifically
Each theme will be described in detail in turn using illustrative observations, examples of pupils’ work and quotes in the following section.
Use of apps on iPads to increase science knowledge acquisition and scientific literacy
One of the key strategies pupils used to increase their science knowledge during science weeks and beyond, was the use of small stand-alone applications (apps) on the iPads, with the types of apps used, falling into two broad categories. The first type was science specific and encompassed background knowledge on each topic for example plant and human biology. The second type of app was more generic and allowed processing and reporting of the investigational data, examples include Popplet, iMovie, Comic Strip, Explain Everything, and Book Creator. Pupils were given the opportunity to choose from a range of apps prior to the science weeks and were able to access the apps at any time during their learning. Interview data revealed approximately one third valued this opportunity to address their own learning needs, as illustrated by the following quote from a year 5 girl:
I sometimes like to use science apps when I don’t understand something, I ask my teacher if that is OK, and I use time after or before lessons. I liked the Trees are Best app as it helped
me learn a lot of interesting things about trees. I also used Plant Parts to help me become more confident with naming the parts of a flower.
All pupils confirmed at interview that they were self-reliant in terms of accessing information using science specific apps, they were often observed before and after investigational sessions sharing science-based facts and demonstrating different aspects of the apps to each other.
Whilst apps containing science specific material were undoubtedly useful for a significant proportion of learners, the vast majority of app usage centred around the use of generic apps. The first of these apps, iMovie, was used extensively to video capture real time recordings of specific experimental outcomes. The material was then reviewed and edited by the pupils prior to inclusion in their reports. For example, in the case of producing a Poly Vinyl Acetate (PVA) polymer, termed by the pupils “Flubber,” they were observed to video record the bouncing of “Flubber” balls alongside a 1 m ruler, in order to determine both the height of bounce and the number of bounces. Pupils were then observed to calculate mean averages of both variables which demonstrated links with their scientific knowledge of measurements.
Generic apps were also used at a deeper level to collate and process information from the scientific investigations. Both Book Creator and Comic Strip were used in a narrative manner to detail and record experimental methodology and results. High quality chronicling of both the experimental processes and results was evident. For example, analysis of an experimental write-up using the app Comic Strip contained photographs of the red cabbage pigment extraction step followed by filtration and the subsequent use of the extract as a pH indicator. Similarly, pupils used the app Explain Everything to produce an e-book logically and systematically detailing an investigation into the action of plant enzymes, on a jelly protein layer. Video clips obtained using the app iMovie were embedded into the experimental report to supplement the pupils’ observations.
It was observed on multiple occasions that the pupils appeared confident to manipulate and process a wide range of material in order to construct mind-maps, e-books, and comic strips to illustrate their scientific knowledge acquisition. One aspect of this knowledge acquisition was strong scientific literacy, supported by online resources. It was frequently observed that during the initial research phases of the inquiry, pupils often used online dictionaries during the construction of their investigational reports. For example the significance of the phrase “the liver detoxifies and metabolises” was explored for meaning by a year 6 girl, who immediately shared her understanding with the whole class. She emphasised the meaning of both the words detoxify and metabolism in a manner which suggested she understood two of these key functions of the liver.
Another good example of how pupils approached brand new terminology, was the use of the scientific term “enzymes” which several pupils found problematic. In this case pupils engaged with their teachers in modelling the actions of digestive enzymes on fat and protein molecules. They photographed the simplified cardboard molecules the teacher had created of the food molecules and how they were broken down by enzymes (modelled by scissors). This resulted in extensive discussion between groups of pupils who went on to use other multimedia apps to explore the meaning of new term. As a result, one year five pupil drew a cartoon of an enzyme molecule breaking down a long polymer to illustrate his understanding of the catabolic nature of enzymes.
The pupils also understood the importance of using text to describe the key scientific evidence contained within the multimedia and frequently ascribed each legend to a particular graphic, e.g. “The hummingbird does not have to suck up liquid into its beak, instead there are two grooves called troughs which draw the liquid through them.”
Similarly, key words such as “magma”, “pressure”, “lava,” and “eruption” were used to correctly annotate and narrate an animation showing volcanic activity in a pupil’s e-book.
Use of iPads to support planning and recording of inquiry processes
Three quarters of the pupils routinely used the mind mapping app Popplet to plan and analyse their data. This tool allows the user to build up the mind map by quickly creating many separate “bubbles” (termed popples) capable of storing data or ideas. The pupils were observed to create highly detailed planning frameworks consisting of multiple steps and large arrays of data organised in tables, using this app. For example, during the water transpiration investigation using celery and dyed water, pupils created a table using popples to record each celery sample in each environmental condition (low temperature/light, room temperature, and moderate temperature/light and darkness). The following day, when transpiration had taken place, pupils were observed to photograph each celery sample and place the image in their pre-prepared recording grid.
Pupils also described at interview how they referred to and refined mind maps during the whole inquiry, as articulated by a year 5 boy as follows:
I really like Popplet, it helps me plan what I am going to do. If I forget, I go back and check through the Popples…. Let me show you, this is the plan I did for my science experiment and here are all the Popples I used to make my results table.
Another feature of app usage by the pupils was the very proficient use of photographs, graphics and video capture material to illustrate scientific reports. Over three quarters of the pupils demonstrated proficient use of the app Book Creator to record in great detail experimental outcomes. For example, this was seen in a digestion investigation, where the e-books created by the pupils contained predictions pertaining to how the fruit slices would affect the consistency of the jelly. In order to fully record the digestion process over the course of the experiment, pairs of pupils used the iPad time lapse photography feature to record the experimental outcomes. An edited video clip of this process was then observed to be embedded in the resultant e-book alongside a well formulated narrative of the experiment. The pupils were abundantly aware of the need to be systematic in their recording as illustrated by the following exchange:
Researcher: “How did you know what to take the photographs of?”
Pupil: “Well some of our friends told us and others were just common sense basically. If you’re going to put it in the method, take a picture of it. This is the red cabbage at the start; these are all the pictures we took when we went through the process to get the purple juice out.”
Observational and interview data confirmed that pupils were able to effectively use the apps on the iPads to record a range of static and dynamic experimental outcomes and capture data in a way that they could then include in their scientific reports and presentations.
Use of iPads to increase understanding of working scientifically
It was clear that the use of iPads during inquiry had been facilitative for underpinning the scientific inquiry process. Analysis of results and scientific discussion seemed to be particularly supported by this approach. Pairs of pupils described how important it was when analysing a chemical sample to compare the result with that of a control and they achieved this by using the camera app. This was illustrated by the following exchange during interview:
Pupil: “The one at the back is a control one” [shows researcher photograph of test tube containing plant extract].
Researcher: “Oh okay, why do you need a control tube?”
Pupil: “To see if the extract has changed colour or not.”
In addition, the pupils were observed to compare their experimental outcomes embedded within multimedia files with other pupils, prompting scientific dialogue. Subsequent discussion appeared to prompt a host of higher order questions for example:
Pupil: “Mine dissolved faster than yours, did you stir faster?”
Pupil: “Did you use the same amount of water as us?. Yours is darker red?”
During the seed inquiry it was evident that the pupils were comparing their prediction for each fruit in terms of number and appearance of the seeds (which they had inserted in a single popple), with the actual findings following cutting open the fruit. This demonstrated they knew it was important to compare and contrast scientific predictions with actual results.
In terms of integrated iPad use facilitating in-depth understanding of scientific inquiry, the following example is illustrative:
Researcher: “How did you use the iPad to learn about animal adaptations?”
Pupil: “I’ve done a Popplet about the bird beaks” [shows the researcher a mind map showing the initial planning of the bird beak investigation together with Popples to record how much food was collected over which time periods, for each beak].
Researcher: “Then what did you do?”
Pupil: “Then we got to do an investigation … with tools that were like actual beaks … and then we had some stars in the water we had to time ourselves …. to see how many we could get into the cup with different tools. We then watched a video clip about the adaptation of the bird beaks.”
Pupil: “After our experiment I researched different bird diets and beaks and found out about humming birds having long beaks so they can get nectar out of plants.”
Researcher: “And what have you learnt about bird beaks?”
Pupil: “How the birds would like survive, with the limited food resources.”
These exchanges illustrate the strong understanding pupils had derived of working scientifically using iPads during their inquiries and subsequently carrying out additional research using online resources.
Learning behaviours and attitudes to the use of iPads in science inquiry
Three themes emerged in response to the second research question from the semi-structured observations, interviews and pupil work as follows:
• Personalisation of the science learning experience
• Positive attitudes to the benefits of using the affordances of mobile technology
• Collaboration with peers and teachers during scientific inquiries
Personalisation of the science learning experience
The majority of pupils took the opportunities afforded them by iPads to customise their learning experience during the science weeks. One of the pair of year five pupils described how they worked together initially when researching and planning and then went on to document their own inquiries individually:
Pupil: “At the beginning we made a little mind-map about what we would like to know about this particular project. We did lots of research on the internet about different plants in different places, this was interesting.”
The pair then proceeded to describe how they had each customised their own ebook by using different backdrops, fonts, colours and arrangements of images and texts to create their own unique record. They then revealed when they had finished, they used the iPads to peer-review.
Some pupils made unusual app choices; one girl described how she used PicCollage, an app usually reserved for social sharing of photographs, to compare images from the detergent investigation:
Pupil: “It can help you because you can screenshot it [test sample] … you can write a caption to it as well… then you’ll have a page of pictures.”
She then proceeded to explain how she carefully compared each test sample after washing with the control sample, to give a score to each detergent in terms of cleaning capacity.
The pupils were also articulate in describing how they liked to demonstrate their agency as learners. They were honest and reflective in expressing their preferences to use mobile technology as exemplified by the following year 6 pupil:
“I know some schools or some places they don’t use iPads.… you have to write it all down on paper and I’m really slow at writing all of it down on paper.”
Another year 6 girl commented how much easier it was to edit her work efficiently using the iPads:
“It was a lot easier to make changes when I reviewed the writing, and I could add pictures and make a really good document.”
Pupils were highly organised during the analysis stage with pairs critiquing the overall shape and content of the mind maps during learning. For example, during the sheep’s lung dissection, one pair arranged the popples in a zigzag formation in order to put the respiratory system organs in a logical space and add more information. They described how this choice had enabled them to effectively handle their data:
Pupil: “We found it easier to spread our ideas out, instead of just writing one massive paragraph about it.”
Overall, these quotes illustrate how personal learning preferences could be accommodated by using the m-technology.
Positive attitudes to the benefits of using the affordances of mobile technology
Often during interviews, the pupils described how they used the mobility of the iPads to great effect, for example during experimentation outside:
Pupil: “I took pictures when we were outside for science … we brought them inside and I could use the pictures to remind me what we had found.”
Others commented on how the ease with which the iPad could be positioned to take photographs was very helpful:
Pupil: “I took it from that angle…because you could see it had sunk instead of being on top.”
Due to the portability of the iPads, photographs of scientific processes were taken quickly and efficiently. One group used the mobility of the iPads to photograph up close, how the Poly Vinyl Acetate (PVA) glue polymer was used to fill a mould, and changed in shape over the course of the investigation. The pupils also used the apps to improve the accuracy of their observations, e.g., when polymer testing, they video-captured a ruler alongside the sample to record the snapping point.
This was also the case when recording outcomes which were technically difficult to see in great detail, as in the case of the static electricity investigation. Several observations indicated the pupils appeared fascinated to see the small plastic pieces attracted to the charged rod and used iMovie to film the pieces being lifted into the air. They then used the iMovie clip to illustrate their experimental results.
The only rarely reported dissatisfaction was pupils describing being “disappointed” when work was lost due to software updating processes or network problems.
Collaboration of peers and teachers during scientific inquiries
Collaboration was a strong theme identified within the pupils’ mode of learning when using iPads. Throughout the entire science weeks, they used material stored on the iPads and the class cloud server to initiate scientific discussion, gather and share data.
Conversations were often initiated by pupils exchanging research or their own experimental data. Overall, the collaborative approach was highly valued by the pupils as illustrated from the following quotes from year 5 and 6 Pupils.
Year 5 pupil: “We share ideas. Sometimes when I am struggling, I ask someone else and they show me what they did with the iPad.”
Year 5 pupil: “We use them all the time; we show our teacher our data or others.
And from a year 6 girl:
“I love sharing the work when it is mirrored on the board. When my work is shown I feel very happy, and I like to get ideas from other people.”
Some pupils appreciated the support of their partner during data gathering, a year 5 girl, new to the school said:
“We used the iPads to record scores for the bird beak experiment. I am not confident in working by myself, I like working with someone just to make sure that I’ve got all my stuff right.”
It was also clear that the pupils enjoyed viewing each other’s iMovie video clips of experimental findings and discussing outcomes:
Pupil: “I like listening to my friend’s recordings on their iPads and then we share ideas.”
During the explorations into circuits, lots of small group interactions were observed, for example between three pairs of pupils who showed each other video clips of trial and error type experiments. The pupils appeared happy to accept differences in approaches and outcomes. Indeed, some went as far as saying they valued critique from their peers.
The pupils saw data sharing in this way as a win-win situation and termed the phrase “Magpie” to indicate using someone else’s approach when they liked it:
Pupil: “When you’ve done some work, you can show it to everyone. If you’re stuck, you can Magpie … you can be shown what to do, not told.”
In the case of formative assessment, the teachers used Apple TV on the interactive white board [IWB] to share the pupil reports containing photographs, videos and diagrams. The pupils were observed to frequently collaborate together during this time of lesson plenaries and to maintain focus by discussing their results and approaches of others.
In terms of collaboration with the teachers and leaders, scientific inquiry reports were deposited by pupils on the shared cloud, used for cohort assessment purposes. The pupils valued the resulting additional feedback on their experimental work.
Year 5 boy: “Sometimes, the year five leader organises our work and gives us feedback so then we can get better and learn a bit more.”
This quote demonstrates the pupils understood how sharing their work facilitated understanding of their learning not just with their peers but with teachers and year group leaders.
Conclusion to findings
In summary, integration of the qualitative data from observations, interviews, and pupils’ work confirmed that the use of iPads within the science inquiries appeared to significantly enhance learning opportunities. Pupils were seen to move seamlessly from initial research, to planning, to experimental phases, whilst simultaneously adding to and refining their ideas. They used the large capacity of iPads to store, analyse data and present their findings, using an array of multimedia modes. Overall, the pupils exhibited highly positive attitudes and collaborative learning behaviours when using m-learning approaches.
Discussion
Reviewing the findings, it is clear that the researchers, teachers and pupils believed there were significant advantages to m-learning during scientific inquiry. This approach undoubtedly gave rise to innovative opportunities to undertake authentic scientific learning across all three science disciplines. Echoing the future scenarios of science learning and skill acquisition, proposed by Kearney et al. (2022) and originally advocated by Duschl et al. (2007) and United Nations Education Scientific and Cultural Organisation [UNESCO] (2017), this study foregrounds both the autonomous and connective affordances conferred by m-learning. Specifically, it highlights the capacity to deploy m-learning strategies during all phases of scientific inquiry in contrast to the use of classical stand-alone computers, which were restricted to the initial phases of research and planning and then analysis (Kim et al., 2007).
Bespoke learning resources in the form of specific apps were shown to be effective in affording pupils’ opportunities to research and develop their own science subject knowledge in a pupil-centred manner as advocated by Worth (2010). This echoes the work of Pedaste et al. (2015) who asserted that practical inquiry alone could not ensure deep learning but rather initial research was an important component of pupil metacognition and sense making in science.
The findings show clear resonances with the work of Hilton (2018) with respect to iPads facilitating engagement with mathematical processing. There were several occasions where pupils were observed to use the unique capabilities of the m-technology; for example, in videorecording followed by in-depth measurements, to refine the accuracy and analysis of their inquiries. Since the future of many aspects of scientific work rely on the deployment of mobile and remote technologies for measurements, this bodes well for the skills of the upcoming workforce.
In terms of overall effectiveness, an important point of interest was the large positive impact on the development of pupils’ digital scientific literacy through the use of both generic and specific apps, during all phases of the scientific inquiry. The pupils were able to move easily from one mode of scientific representation (e.g., text, graphics, or video material) to another and incorporate a range of data into their reports. Prain and Waldrip (2010):2 argue that it is a great advantage for learning, when students are able to interpret “modal diversity in representations of science concepts.” Indeed, it is not just different modes of representation that are of importance in fostering understanding, but rather the development of multiple ways of communicating and believing in science (Moje, 2008).
In order to (re)-construct scientific beliefs, learners need opportunities to engage in discussion with teachers, in addition to learning through sharing and critically evaluating their own and peers’ scientific inquiry (Ford and Forman, 2006; Yacoubian and BouJaoude, 2010). This involves the development of scientific literacy skills (Lee et al., 2013; Bergeson and Rosheim, 2018) and the correct usage of key science terminology (Shanahan and Shanahan, 2008), a component highlighted in this study, by the sharing and meaning making of key scientific terms using on-line dictionaries and the extensive deployment of appropriate vocabulary in the pupil presentations and reports. Overall, the use of iPads during inquiry afforded the pupils opportunities to develop their scientific literacy toward the end of KS 2, prior to transition to secondary education. It could be argued that this is of a particular significance, since the confident use of scientific language during argument making has been shown to be pivotal (van der Graaf, 2020).
From an inclusive practice perspective, in agreement with several studies (Miller et al., 2013; Knight and Davies, 2016; Browder et al., 2017), the use of iPads during scientific inquiry fostered accessibility for a range of learners, especially those with specific literacy needs. Such personalised strategies appeared to benefit learners who voiced the opinion that they liked to learn in their own way. Pupils with specific literacy needs described how m-learning allowed them to approach the documentation of the inquiries positively and enable them to make a prompt start and not lag behind the rest of the class. The science specific apps also allowed for effective differentiation of learning opportunities; with more able pupils accessing detailed information on specific topics e.g., evolutionary change, whilst others used the apps to review and reinforce their learning of conceptually challenging concepts (Allen, 2010) e.g., electrical circuits. Several pupils highlighted how the iPads allowed them to keep up with the pace of the lesson. This is wholly appropriate given evidence that pupils appreciate both independent and guided means of study (Lau et al., 2017) and echoes the study by Furman et al. (2018), who revealed even younger learners were pleased by opportunities to display autonomy, when using tablet computers during IBSE.
The pupils’ attitudes to using tablet computers during IBSE were overwhelmingly positive, one pair described how working with iPads enabled them to work at a different level and how they became excited when designing their own multimedia reports. Several pairs expressed how they preferred using iPads as they helped them learn more efficiently and registered disappointment that their diminished use at the culmination of upper KS2. These positive attitudes are largely in agreement with the findings of a systematised literature review into m-learning using iPads, undertaken by Boon et al. (2021), which explored the experiences of primary age pupils using m-technology to access the curriculum overall. In the specific case of science learning, the findings confirm those of others (Looi et al., 2011; Dunn et al., 2016; van Deursen et al., 2016) that m-technology is a valuable tool to facilitate ISBE. When utilising iPads during inquiry, pupils particularly valued using them to exchange ideas and undertake collaborative research together in agreement with Rønningsbakk (2020), arguably two of the most important aspects of the ways scientists work. Since pupils are motivated to use iPads, an important consideration during IBSE (Justice et al., 2009) and this pedagogical approach has been shown to be broadly effective (Clark and Luckin, 2013; Burden and Kearney, 2016; Hong et al., 2017), it would be advisable for teachers and curriculum designers to integrate m-technology into future scientific inquiry. Indeed, it is advocated that pupils’ voices should be given primacy even at the expense of teachers’ concerns when designing effective m-learning opportunities (Dunn et al., 2016), since they are agents of their own learning both in and beyond the classroom.
The exact role of teachers in inquiry based approaches is flexible however it has been suggested that teachers should afford their learners opportunities to explore “conceptual, epistemic, social and/or procedural domains of scientific knowledge” (van Uum et al., 2016, p. 450). In other words, pupils need to access the key scientific ideas, understand how scientific knowledge is constructed and appreciate how the nature of working scientifically and scientific debate, supports the process. Key to all these domains is the process of questioning, a skill highly represented in primary science teaching practice (Earle, 2014). This study has shown m-learning can be used to support pupils formulate and share questions with their pers and teachers. Teachers can also provide further exciting stimuli for pupils to illicit their ideas and prompt further questioning and discussion, which can be part of a formative assessment strategy (Constantinou et al., 2018). This could well be achieved by deploying mobile technology as a means of accessing engaging resources. In terms of effective teacher practice during IBSE, it has been suggested that primary school teachers may not see themselves substantively as “science teachers” and consequently may exhibit concerns about their science content knowledge (Harlen, 2021). However, research (Mellander and Svärdh, 2018) has shown that teachers who display strong pedagogic content knowledge of inquiry, deploy approaches associated with improved pupil learning. These arguments have clear implications for IBSE to be explicitly explored in teacher education programmes (Strat and Jegstad, 2022) in conjunction with the affordances of m-learning, as part of an inquiry based approach.
Since pupils articulated eloquently their preference for close collaboration and sharing of their scientific findings and expertise with peers and teachers alike, these findings can be used to inform future pedagogical approaches. They resonate significantly with the metastudy undertaken by Afikah et al. (2022) across all phases of the science curriculum, where collaboration was shown to be a key element of engagement and shared problem-solving. Specifically, this paper argues for the empowerment of individual pupils to realise their full potential within scientific inquiry and build their science capital, by using m-learning to enable them to collaborate with their peers and in turn, contribute to wider scientific discourse.
This socio-cultural potential of m-learning echoes Dunlop et al. (2015) arguments for building “communities of enquiry” during IBSE and builds on the work of Felix et al. (2005) who advocates addressing a question of common concern by harnessing different points of view. The added advantage of social constructivist learning is that it models many of the processes adopted during authentic scientific discovery in the real world (Burden and Kearney, 2016). Indeed, many features of the science learning opportunities afforded by this approach are compatible with the creation of technology enhanced multi-modalities as advocated by Zhang et al. (2010) and Lynch and Redpath (2014). These result in learners being made more aware of their metacognition and allow potential scientists of the future, to see for themselves how knowledge is constructed in a scientifically robust and collaborative manner.
Limitations
There were some limitations, arguably the most prominent being the use of a single, longitudinal, school case study. It is accepted that this school was at the forefront of good practice with respect to the use of iPads across the curriculum and therefore findings cannot be considered to be wholly generalisable. However, with the proliferation of Apple Distinguished Schools and Multi Academy Trusts in England, where pedagogical approaches are often shared within clusters of schools (Apple, 2021; Baxter and John, 2021), this study aimed to illustrate what is possible in terms of the affordances of m-learning supported IBSE and hence share effective practice. With respect to inherent limitations of the data collection tools, these are well known; pupils may behave differently when they know they are being observed and during interview may seek to provide answers which they believe the researcher will value (Einarsdóttir, 2007). However, the researchers feel both these limitations were reduced by the fact that an insider/outsider positionality (Moore, 2012) was adopted due to the strong relationships developed with the staff and learners over a three-year period. The limitations of work scrutiny are that it is partly subjective and open to bias. However, in this case, since the pupils brought their work on iPads to the interviews and illustrated their evolving ideas, it is believed the researchers gained a robust understanding of pupils’ thinking processes as advocated by Kellett (2006).
Conclusion
It is highly evident that m-learning is becoming wider spread in primary schools (Boon et al., 2021) and it is hoped that the key findings of this study will inform discourse around the use of m-technology to enhance primary science pedagogy from the perspectives of the learners. Further research would include the exploration of key findings, for example improved scientific recording and attitudes of pupils to their own metacognition, in a range of schools both within the U.K and internationally. In a global scientific community where an increasingly diverse range of modalities are being used to document and analyse during scientific enquiry (Asch et al., 2018), this approach could make a significant contribution to the engagement and education of the scientists of the future.
Data availability statement
The datasets presented in this article are not readily available because of confidentiality. Requests to access the datasets should be directed to k.blackmore@worc.ac.uk.
Ethics statement
The studies involving human participants were reviewed and approved by College for Arts, Humanities and Education Ethics Committee, University of Worcester. Written informed consent to participate in this study was provided by the participants’ legal guardian/next of kin.
Author contributions
KB wrote the first draft of the manuscript. LR discussed research and publications as a lens through which to view the manuscript, critiqued and wrote specific sections of the second draft, and contributed additional current literature. Both authors edited subsequent drafts together and contributed to manuscript revision.
Acknowledgments
We thank all the participants in this study who generously shared their knowledge and views of learning in primary science.
Conflict of interest
The authors declare that the research was conducted in the absence of any commercial or financial relationships that could be construed as a potential conflict of interest.
Publisher’s note
All claims expressed in this article are solely those of the authors and do not necessarily represent those of their affiliated organizations, or those of the publisher, the editors and the reviewers. Any product that may be evaluated in this article, or claim that may be made by its manufacturer, is not guaranteed or endorsed by the publisher.
References
Abd-El-Khalick, F. (2003). Inquiry in science education: International Perspectives. Cult. Comp. Stud. 88, 397–419.
Afikah, A., Astuti, S., Suyanta, S., Jumadi, J., and Rohaeti, E. (2022). Mobile Learning in Science Education to Improve Higher-Order Thinking Skills (HOTS) and Communication Skills: A Systematic Review. Int. J. Adv. Comp. Sci. Applic. 13, 698–704.
Anderson, R. (2002). Reforming Science Teaching: What Research Says about Inquiry. J. Sci. Teach. Educ. 13, 1–12.
Apple (2021). Apple Distinguished Schools: Centres of Leadership and Educational Excellence 2021–2024 Overview. Cupertino, CA: Apple.
Archer, L., Dawson, E., DeWitt, J., Seakins, A., and Wong, B. (2015). “Science capital”: A conceptual, methodological, and empirical argument for extending bourdieusian notions of capital beyond the arts. J. Res. Sci. Teach. 52, 922–948.
Archer, L., DeWitt, J., Osbourne, J., Dillon, J., Willis, B., and Wong, B. (2010). ‘Doing’ science versus ‘being’ a scientist: Examining 10/11-year-old school pupils’ constructions of science through the lens of identity. Sci. Educ. 94, 617–639.
Asch, M., Moore, T., Badia, R., Beck, M., Beckman, P., Bidot, T., et al. (2018). Big data and extreme-scale computing: Pathways to Convergence-Toward a shaping strategy for a future software and data ecosystem for scientific inquiry. Int. J. High Perform. Comput. Applic. 32, 435–479.
Baxter, J., and John, A. (2021). Strategy as learning in multi-academy trusts in England: strategic thinking in action. Sch. Leadersh. Manage. 41, 290–310.
Berg, C., Bergendahl, V., Lundberg, B., and Tibell, L. (2003). Benefiting from an open-ended experiment? A comparison of attitudes to, and outcomes of, an expository versus an open-inquiry version of the same experiment. Int. J. Sci. Educ. 25, 351–372.
Bergeson, K., and Rosheim, K. (2018). Literacy, equity, and the employment of iPads in the classroom: A comparison of secure and developing readers. Int. J. Educ. Math. Sci. Technol. 6, 173–181.
Bianchini, J., and Colburn, A. (2000). Teaching the nature of science through inquiry to prospective elementary teachers: A tale of two researchers. J. Res. Sci. Teach. 37, 177–209.
Boon, H., Boon, L., and Bartle, T. (2021). Does iPad use support learning in students aged 9–14 years? A systematic review. Aust. Educ. Res. 48, 525–541. doi: 10.1007/s13384-020-00400-0
Bransford, J., Brown, A., and Cocking, R. (eds) (2000). How people learn: brain, mind, experience, and school. Washington, DC: National Academy Press.
Braun, V., and Clarke, V. (2006). Using thematic analysis in psychology. Qual. Res. Psychol. 3, 77–101.
British Educational Research Association (2018). Ethical Guidelines for Educational Research. London: British Educational Research Association.
Browder, D., Root, J., and Wood, L. (2017). Effects of a story-mapping procedure using the iPad on the comprehension of narrative texts by students with autism spectrum disorder. Focus Autism Other Dev. Disabil. 32, 243–255. 611387 doi: 10.1177/1088357615
Burden, K., Aubusson, P., Brindley, S., and Schuck, S. (2016). Changing knowledge, changing technology: Implications for Teacher Education Futures. J. Educ. Teach. 42, 4–16.
Burden, K., and Kearney, M. (2016). Future Scenarios for Mobile Science Learning. Res. Sci. Educ. 46, 287–308.
Burden, K., and Younie, S. (2014). Using iPads effectively to enhance learning in schools. London: MESHGuides.
Chang, C., and Mao, S. (1999). Comparison of Taiwan science students’ outcomes with inquiry- group versus traditional instruction. J. Educ. Res. 92, 340–346. doi: 10.1186/s13054-016-1208-6
Clark, W., and Luckin, R. (2013). What the research says: iPads in the classroom. London Knowledge Lab. London: Institute of Education University of London.
Constantinou, C., Tsivitanidou, O., and Rybska, E. (2018). “What Is Inquiry-Based Science Teaching and Learning?,” in Professional Development for Inquiry-Based Science Teaching and Learning, eds O. E. Tsivitanidou, P. Gray, E. Rybska, L. Louca, and C. P. Constantinou (Cham: Springer), doi: 10.1007/978-3-319-91406-0_1
Crawford, B. (2000). Embracing the essence of inquiry: New roles for science teachers. J. Res. Sci. Teach. 37, 916–937. doi: 10.1002/1098-2736(200011)37:9<916::AID-TEA4>3.0.CO;2-2
DfE (2015). National Curriculum in England: Science Programmes of Study: Statutory guidance. England: DfE.
Dunlop, L., Compton, K., Clarke, L., and McKelvey-Martin, V. (2015). Child-led enquiry in primary science. Education 43, 462–481. doi: 10.1080/03004279.2013.822013
Dunn, J., Gray, C., Moffett, P., and Mitchell, D. (2016). ‘It’s more funner than doing work’: pupils’ perspectives on using tablet computers in the early years of school. Early Child Dev. Care 188, 819–831.
Duschl, R., Schweingruber, H., and Shouse, A. (eds) (2007). Taking science to school: Learning and teaching science in grades K-8. Washington, DC: National Academies Press.
Earle, S. (2014). Formative and summative assessment of science in English primary schools: evidence from the Primary Science Quality Mark. Res. Sci. Technol. Educ. 32, 216–228.
Economic and Social Research Council (2013). What influences participation in science and mathematics?’ A briefing paper from the Targeted Initiative on Science and Mathematics Education (TISME). London: Economic and Social Research Council.
Einarsdóttir, J. (2007). Research with pupils: methodological and ethical challenges. Eur. Early Child. Educ. Res. J. 15, 197–211. doi: 10.1136/bmjopen-2020-044143
Ford, M., and Forman, E. (2006). Refining disciplinary learning in classroom contexts. Rev. Res. Educ. 30, 1–33. doi: 10.1002/bmb.21387
Furman, M., De Angelis, S., Dominguez Prost, E., and Taylor, I. (2018). Tablets as an educational tool for enhancing preschool science. Int. J. Early Years Educ. 27, 6–19.
Furtak, E. M., Seidel, T., Iverson, H., and Briggs, D. C. (2012). Experimental and quasi-experimental studies of inquiry-based science teaching: A meta-analysis. Rev. Educ. Res. 82, 300–329. doi: 10.3102/0034654312457206
García-Carmona, A. (2020). From Inquiry-Based Science Education to the Approach Based on Scientific Practices. Sci. Educ. 29, 443–463. doi: 10.1007/s11191-020-00108-8
Guba, E., and Lincoln, Y. (1994). “Competing paradigms in qualitative research,” in Handbook of qualitative methods, eds N. Denzin and Y. Lincoln (London: Sage).
Hackling, M., and Prain, V. (2008). Impact of Primary Connections on Students’ processes literacies of science and science processes. Canberra: Australian Academy of Science.
Harlen, W. (2010). Principles and Big Ideas of Science Education. Hatfield: Association for Science Education.
Harlen, W. (2021). The Case for Inquiry-based Science Education – IBSE. Trieste: The Inter Academy Partnership (IAP).
Hilton, A. (2018). Engaging primary school students in mathematics: Can iPads make a difference? Int. J. Sci. Math. Educ. 16, 145–165.
Hong, J., Hwang, M., Tai, K., and Tsai, C. (2017). An exploration of students’ science learning interest related to their cognitive anxiety, cognitive load, self-confidence and learning progress using inquiry-based learning with an iPad. Res. Sci. Educ. 47, 193–212.
Justice, C., Rice, J., Roy, D., Hudspith, B., and Jenkins, H. (2009). Inquiry-based learning in higher education: Administrators’ perspectives on integrating inquiry pedagogy into the curriculum. High. Educ. 58, 841–855. doi: 10.1007/s10734-009-9228-7
Kearney, M., Schuck, S., and Burden, K. (2022). Digital pedagogies for future school education: promoting inclusion. Irish Educ. Stud. 41, 117–133.
Kellett, M. (2006). “Pupils as active researchers: Using engagement with research process to enhance creativity and thinking skills in 10-12 year-olds,” in Paper presented at the British Educational Research Association Annual Conference, Warwick.
Keys, C., and Bryan, L. (2001). Co-constructing inquiry-based science with teachers: Essential research for lasting reform. J. Res. Sci. Teach. 38, 631–645.
Kim, M., Hannafin, M., and Bryan, L. (2007). Technology-Enhanced Inquiry Tools in Science Education: An Emerging Pedagogical Framework for Classroom Practice. Sci. Educ. 91, 1010–1030.
Knight, K., and Davies, R. (2016). Using a mobile dichotomous key iPad application as a scaffolding tool in a museum setting. Interact. Learn. Environ. 24, 814–828.
Kvale, S. (1996). Interviews: An Introduction to Qualitative Research Interviewing. Thousand Oaks, CA: Sage Publications.
Lau, W., Lui, V., and Chu, S. (2017). The use of wikis in a science inquiry-based project in a primary school. Educ. Technol. Res. Dev. 65, 533–553.
Lee, A. (2012). Development of a parent’s guide for the Singapore primary science curriculum: Empowering parents as facilitators of their pupils’ science learning outside the formal classrooms. Asia Pac. For. Sci. Learn. Teach. 13, 1–27.
Lee, O., Quinn, H., and Valdés, G. (2013). Science and language for English language learners in relation to next generation Science standards and with implications for common core state standards for English language arts and mathematics. Educ. Res. 42, 223–233.
Leonard, J., Boakes, N., and Moore, C. (2009). Conducting Science Inquiry in Primary Classrooms: Case Studies of Two Preservice Teachers’. Inquiry Based Pract. J. Element. Sci. Educ. 21, 27–50.
Linn, M., Davis, E., and Bell, E. (2004). Internet environments for science education. London: Lawrence Erlbaum.
Looi, C., Zhang, B., Chen, W., Seow, P., Chia, G., Norris, C., et al. (2011). 1:1 mobile inquiry learning experience for primary science students: a study of learning effectiveness. J. Comput. Assist. Learn. 27, 269–287. doi: 10.1111/j.1365-2729.2010.00390.x
Lynch, J., and Redpath, T. (2014). ‘Smart’ technologies in early years literacy education: A meta-narrative of paradigmatic tensions in iPad use in an Australian preparatory classroom. J. Early Child. Liter. 14, 147–174. doi: 10.1177/1468798412453150
Mellander, E., and Svärdh, T. (2018). Inquiry-based learning put to the test: medium-term effects of a science and technology for children programme. Rev. Educ. 6, 103–141. doi: 10.1002/rev3.3109
Miller, B., Krockover, G., and Doughty, T. (2013). Using iPads to teach inquiry science to students with a moderate to severe intellectual disability: A pilot study. J. Res. Sci. Teach. 50, 887–911. doi: 10.1002/tea.21091
Minner, D., Levy, A., and Century, J. (2010). Inquiry-based science instruction-what is it and does it matter? Results from a research synthesis years 1984 to 2002. J. Res. Sci. Teach. 47, 474–496. doi: 10.1002/tea.20347
Moje, E. (2008). Foregrounding the disciplines in secondary literacy teaching and learning: A call for change. J. Adolesc. Adult Liter. 52, 96–107.
Monem, R., Bennett, K., and Barbetta, P. (2018). The Effects of Low-Tech and High-Tech Active Student Responding Strategies during History Instruction for Students with SLD. Learn. Disabil. 16, 87–106.
Moore, J. (2012). A personal insight into researcher positionality. Nurse Res. 19, 11–14. doi: 10.7748/nr2012.07.19.4.11.c9218
National Curriculum Board of Australia (2009). Shape of the Australian Curriculum: Science. Canberra: National Curriculum Board of Australia.
National Research Council (1996). Science education standards, Washington, DC: The National Academies Press.
National Research Council (2012). A Framework for K-12 Science Education: Practices, Crosscutting Concepts, and Core Ideas. Washington, DC: The National Academies Press.
OFSTED (2011). Successful science: an evaluation of science education in England 2007–2010. England: OFSTED.
Pedaste, M., Mäeots, M., Siiman, L., de Jong, T., van Riesen, S., Kamp, E., et al. (2015). Phases of inquiry-based learning: Definitions and the inquiry cycle. Educ. Res. Rev. 14, 47–61. doi: 10.1016/j.edurev.2015.02.003
Prain, V., and Waldrip, B. (2010). Representing Science Literacies: An Introduction. Res. Sci. Educ. 40, 1–3. doi: 10.1007/s11165-009-9153-x
Rönnebeck, S., Bernholt, S., and Ropohl, M. (2016). Searching for a common ground – A literature review of empirical research on scientific inquiry activities. Stud. Sci. Educ. 52, 161–197.
Rønningsbakk, L. (2020). “Digital Natives and Educational Traditions. What Changes When Exchanging Textbook Content with Internet Search?,” in Innovative Technologies and Learning. ICITL 2020. Lecture Notes in Computer Science, eds T. Huang, T. Wu, J. Barroso, F. E. Sandnes, P. Martins, and Y. Huang (Cham: Springer).
Sandoval, W. (2005). Understanding students’ practical epistemologies and their influence on learning through inquiry. Sci. Educ. 89, 634–656. doi: 10.1002/sce.20065
Schwartz, R., Lederman, N., and Crawford, B. (2004). Developing views of nature of science in an authentic context: An explicit approach to bridging the gap between nature of science and scientific inquiry. Sci. Teach. Educ. 88, 610–645. doi: 10.1002/sce.10128
Science Community Representing Education [SCORE] (2013). Resourcing Practical Science in Primary Schools. London: SCORE.
Shanahan, T., and Shanahan, C. (2008). Teaching Disciplinary Literacy to Adolescents: Rethinking Content-Area Literacy. Harvard Educ. Rev. 78, 40–61.
Siry, C., Ziegler, G., and Max, C. (2012). “Doing science” through discourse-in-interaction: Young children’s science investigations at the early childhood level. Sci. Educ. 96, 311–326. doi: 10.3310/hta25220
Song, Y., and Wen, Y. (2018). Integrating Various Apps on BYOD (Bring Your Own Device) into Seamless Inquiry-Based Learning to Enhance Primary Students’ Science Learning. J. Sci. Educ. Technol. 27, 165–176. doi: 10.1007/s10956-017-9715-z
Strat, T. T. S., and Jegstad, K. M. (2022). Norwegian teacher educators’ reflections on inquiry-based teaching and learning in science teacher education. J. Sci. Teacher Educ. doi: 10.1080/1046560X.2022.2125623
Turner, J., Keogh, B., Naylor, S., and Lawrence, L. (2011). It’s Not Fair - Or is It? A Guide to Developing Children’s Ideas Through Primary Science Enquiry. Richmond: Millgate House.
United Nations Education Scientific and Cultural Organisation [UNESCO] (2017). Science and technology centres: reducing the gap between knowledge and action. Paris: UNESCO.
van der Graaf, J. (2020). Inquiry-based learning and conceptual change in balance beam understanding. Front Psychol. 11:1621. doi: 10.3389/fpsyg.2020.01621
van Deursen, A., Ben Allouch, S., and Ruijter, L. (2016). Tablet use in primary education: Adoption hurdles and attitude determinants. Educ. Inf. Technol. 21:971. doi: 10.1007/s10639-014-9363-3
van Uum, M., Verhoeff, R., and Peeters, M. (2016). Inquiry based science education: towards a pedagogical framework for primary school teachers. Int. J. Sci. Educ. 38, 450–469.
Wang, J., Tigelaar, D., Zhou, T., and Admiraal, W. (2022). The effects of mobile technology usage on cognitive, affective, and behavioural learning outcomes in primary and secondary education: A systematic review with meta-analysis. J. Comput. Assist. Learn. 39:12759. doi: 10.1111/jcal.12759
Wellcome Trust (2013). The Deployment of Science and Maths Leaders in Primary Schools. London: Wellcome Trust.
Wellcome Trust (2014). Primary Science: Is It Missing Out? Recommendations for reviving primary science. London: Wellcome Trust.
Wilson, D., Joseph, A., Taylor, J., Kowalski, S., and Carlson, J. (2009). The relative effects and equity of inquiry-based and commonplace science teaching on students’ knowledge, reasoning, and argumentation. J. Res. Sci. Teach. 47, 276–301. doi: 10.1002/tea.20329
Worth, K. (2010). Science in Early Childhood Classrooms: Content and Process. STEM in Early Education and Development Conference. Cedar Falls: University of Northern Iowa.
Yacoubian, H., and BouJaoude, S. (2010). The Effect of Reflective Discussions Following Inquiry-Based Laboratory Activities on Students’ Views of Nature of Science. J. Res. Sci. Teach. 47, 1229–1252. doi: 10.1002/tea.20380
Keywords: Inquiry Based Science Education, primary education, iPads, app, scientific literacy, multimedia
Citation: Blackmore K and Rønningsbakk L (2023) Let us explain everything: pupils’ perspectives of the affordances of mobile technology during primary science inquiry. Front. Educ. 8:1168459. doi: 10.3389/feduc.2023.1168459
Received: 17 February 2023; Accepted: 05 May 2023;
Published: 19 May 2023.
Edited by:
Subramaniam Ramanathan, Nanyang Technological University, SingaporeReviewed by:
Watcharee Ketpichainarong, Mahidol University, ThailandJosé Cravino, University of Trás-os-Montes and Alto Douro, Portugal
Copyright © 2023 Blackmore and Rønningsbakk. This is an open-access article distributed under the terms of the Creative Commons Attribution License (CC BY). The use, distribution or reproduction in other forums is permitted, provided the original author(s) and the copyright owner(s) are credited and that the original publication in this journal is cited, in accordance with accepted academic practice. No use, distribution or reproduction is permitted which does not comply with these terms.
*Correspondence: Lisbet Rønningsbakk, lisbet.ronningsbakk@uit.no