- 1Interdisciplinary Center for Energy Planning, University of Campinas (UNICAMP), Campinas, SP, Brazil
- 2School of Agricultural, Earth and Environmental Sciences, University of KwaZulu-Natal, Durban, KZN, South Africa
- 3Dartmouth College, Thayer School of Engineering, Dartmouth, NH, USA
- 4New Partnership for Africa’s Development (NEPAD), Johannesburg, GT, South Africa
- 5Faculty of Agricultural Engineering, University of Campinas (UNICAMP), Campinas, SP, Brazil
For more than half of the Southern African population, human development is limited by a lack of access to electricity and modern energy for cooking. Modern bioenergy merits consideration as one means to address this situation in areas where sufficient arable land is available. While numerous studies have concluded that Africa has significant biomass potential, they do not indicate by how much it can effectively reduce the use of traditional biomass and provide more accessible energy, especially at a country level. Here, we evaluate the potential of sugarcane to replace traditional biomass and fossil fuel and enlarge the access to electricity in Southern Africa. By using its current molasses for ethanol production, Swaziland could increase electricity generation by 40% using bagasse and replace 60% of cooking fuel or 30% of liquid fossil fuel. Sugarcane expansion over 1% of the pasture land in Angola, Mozambique, and Zambia could replace greater than 70% of cooking fuel. Bioelectricity generation from modest sugarcane expansion could be increased by 10% in Malawi, Mozambique, and Zambia and by 20% in Angola. Our results support the potential of sugarcane as a modern energy alternative for Southern Africa.
Introduction
Most of the population of Southern African lacks access to electricity and modern energy for cooking (FAO, 2012c; IEA, 2014a). Their heavy dependence on the traditional biomass for cooking direct affect the living conditions in this region. For example, charcoal and firewood supply more than 95% of the cooking energy consumption in Mozambique and Malawi. By contrast, in South Africa, only 13% of the population relies on the traditional use of biomass (IEA, 2014b). The use of charcoal and firewood has been related to approximately 600,000 premature death per year in Africa (WHO, 2013). The stoves are typically inefficient and placed in poorly ventilated spaces, causing indoor air pollution (IEA, 2014a). Also, the use of these traditional biomass leads to household energy accidents, such as burns, scalds, fires, and poisonings (Kimemia et al., 2014).
Electricity access is lacking for 60% of the Southern African population. In Malawi, less than 10% of the population is supplied with electricity (IEA, 2014b). In some cases, countries are highly dependent on imported electricity. For example, 70% of the electricity in Swaziland is imported. Also, all of the Southern African countries are net importers of gasoline and distillate fuel oil (EIA, 2012) (Table 1), with all except South Africa and Zambia wholly dependent on imports. This scenario reduces their energy security and harms the national trade balance.
Although the Southern Africa economy is changing rapidly, with annual gross domestic product (GDP) growth of 5.7% from 2000 to 2012, attracting investments and opening up new opportunities (IEA, 2014a; Taliotis et al., 2016), the region must expand its population’s access to modern, reliable, and affordable energy and improve the social indicators to maintain and consolidate the economic expansion observed during the last decade.
In regions with sufficient land resources, bioenergy can play this role, promoting energy access and rural development integrated with an improved food security and greater national energy sovereignty (Lynd and Woods, 2011). In terms of physical geography, much of Africa has the capacity to produce bioenergy crops without compromising biodiversity and water use (Lynd and Woods, 2011).
Sugarcane is one of the best feedstocks for bioenergy because of its semiperennial productive cycle, which involves replanting at intervals of 5 years or more (De Cerqueira Leite et al., 2009), and its efficient conversion of solar radiation into chemical energy (Zhu et al., 2010). Sugarcane bioenergy can be cost competitive, promote human development, and comply with strict sustainability indicators, reducing greenhouse gas (GHG) emissions by approximately 80% compared to gasoline (Seabra et al., 2011). Moreover, sugarcane can address the triple challenge of energy insecurity, climate change, and rural poverty in sub-Saharan Africa (Johnson and Seebaluck, 2012).
Approximately 40 million t of sugarcane are produced in Southern Africa, mainly in South Africa, Swaziland, Mauritius, Zimbabwe, Zambia, and Mozambique (FAO, 2012d). Despite the existence of suitable areas for sugarcane cultivation in African countries, the overall potential of the countries is different and depend on particular agricultural and economic issues. The Southeast region has the largest potential for rain-fed sugarcane production, with additional potential to grow this crop using irrigation (Hermann et al., 2014).
However, most of the studies about the potential of the biomass as renewable energy in Africa address only the geographic suitability of renewable energy sources (Watson, 2011; FAO, 2012b) or a general overview about the potential energy supply (IRENA-DBFZ, 2013). There is a lack of studies that quantify how much is the ability to replace the current and future uses of traditional biomass and fossil fuels or even enlarge the electricity access according to the current and projected demand. This intriguing question motivated this study.
By considering two scenarios to produce modern energy from sugarcane in Southern Africa, this study explores the potential of this crop to promote a cleaner and more accessible energy, including the required investment and GHG emissions savings. We assume that sugarcane ethanol will be used as a vehicle fuel and partially replace the traditional use of solid biomass for cooking, thereby contributing to reducing the deforestation associated with burning firewood and charcoal. We also evaluate the potential of cogenerating bioelectricity from bagasse. A probabilistic methodology is applied to nine Southern African countries: Angola, Malawi, Mauritius, Mozambique, South Africa, Swaziland, Tanzania, Zambia, and Zimbabwe. Other countries in Southern Africa (Botswana, Democratic Republic of the Congo, Lesotho, Madagascar, Namibia, and Seychelles) are excluded because of a lack of data or inadequate conditions for sugarcane production.
As the real potential of biomass in Africa is still not accurate—studies report an enormous range of suitable area for biomass production (IRENA-DBFZ, 2013), we assumed the use of only 1% of the pasture area, which the equivalent area is realistic in terms of suitable land (Watson, 2011; FAO, 2012b; Johnson and Seebaluck, 2012) (Table 2). Pasture lands are usually underutilized, and by using appropriated pasture management integrated with sustainable intensification practices, such as rotational grazing, incorporation of legumes and integrated crop–livestock–forestry systems, is possible to increase agricultural output (Latawiec et al., 2014) without compromising the grazing activity.
Ethanol Initiatives: A Look to Southern Africa
Ethanol is produced by the fermentation of a mash prepared from molasses (residual sugars from sugar production) or sugarcane juice and distillation, resulting in hydrous ethanol (containing approximately 6% water) or anhydrous ethanol. Pure hydrous ethanol can be used in dedicated or flex-fuel engines, which allow the use of any ethanol-gasoline blend, and as a diesel replacement in modified diesel engines (Nylund et al., 2013). Gasoline containing up to 10% anhydrous ethanol can be used in conventional gasoline vehicles without any modification, and higher blending levels (up to 30%) can be used after relatively simple changes (BNDES/CGEE, 2008). Processing sugarcane to produce either ethanol or sugar results in lignocellulosic residue, corresponding to approximately 27% (dry basis) of cane stalks (Rodrigues Filho, 2005). Bagasse is typically burned to produce power and heat for industrial needs and, increasingly, to generate surplus bioelectricity for the grid (Seabra and Macedo, 2011). In Brazil, sugarcane represents a relevant primary electricity source: for example, the power-generation capacity of sugarcane bagasse is 10,500 MW, corresponding to 7.5% of the total installed capacity (ANEEL Fontes de Energia: Biomassa, 2015).
More than 30 countries worldwide have ethanol-blending mandates motivated by various factors, including energy security, rural economic development, and GHG emission reduction (Munyinda et al., 2012; REN21, 2015a). Global ethanol production reached 94 Mm3 in 2014 and was mainly based on sugarcane, corn, and cassava. Biofuels, including biodiesel, represent less than 5% of the global road transport fuel demand on an energy basis (REN21, 2014).
Several initiatives aimed at introducing sugarcane bioenergy in Southern Africa, including various concepts and scales of operation, have been attempted. In 1982, Malawi adopted E10 blending using biofuel locally produced from sugarcane molasses. By 2004, the total production capacity reached 36 million liters per year (Chakaniza, 2013), allowing for E20 blending and the use of pure hydrous ethanol. In 2012, the CleanStar project was launched in Mozambique to promote a transition away from nontraditional biomass and inefficient stoves by disseminating up to 30,000 clean-burning and efficient cooking stoves fueled with locally produced ethanol (UNFCCC, 2013). The project target was to produce 2 million liters per year of ethanol from cassava as cooking fuel, supplied by local small farmers (Novozymes, 2012). However, the progress was impaired by feedstock supply and failed to achieve the required sales to sustain the manufacturing flow, leading to the end of the project in 2014 (REN21, 2015b). Challenges also included overcoming economic and cultural barriers (Dasappa, 2011).
In Angola, the BIOCOM Enterprise was created to diversify the economy by activating the sugarcane agroindustry and generating jobs and income. This project is a joint venture involving Angolan and Brazilian investors (worth US$ 750 million) and relies strongly on technology transferred from the Brazilian sugarcane agroindustry model. The commercial operation of the facility started in June 2015 (Macauhub, 2015). When fully implemented (planned for 2019/2020), 42,000 ha of sugarcane will annually produce 30 million liters of anhydrous ethanol, 235 GWh of surplus bioelectricity, and 260,000 t of sugar (Biocom, 2015), which is sufficient to supply at least 50% of the domestic demand for sugar (FAO, 2013a). Currently, most sugar consumed in Angola is imported (FAO, 2013a).
Materials and Methods
By using energy demand data (Table 1) and applying some assumptions to project a future scenario for 2030 (see Energy Demand Projection), we evaluated the potential of sugarcane to provide cleaner and more accessible energy in Southern Africa by considering two scenarios: Current Molasses (CM) and New Policies (NP). CM represents a short-term framework, in which ethanol is produced exclusively from molasses, considering the existing sugarcane production and the current technology access. NP refers to an enhanced approach likely to be deployed over the medium to long term based on the 2030 scenario proposed by the International Energy Agency (IEA, 2014a); in this case, sugarcane is cultivated over 1% of the pasture land, and ethanol is produced from molasses (existing sugarcane mills) and direct juice (additional sugarcane mills). We assessed the use of ethanol as cooking fuel and a displacer of fossil fuels, including gasoline and diesel. The use of ethanol in diesel engines is supported by the Scania technology, which allows the use of pure ethanol with 5% ignition improver in diesel engines [BioEthanol for Sustainable Transport project (EHA, 2011)]. Ethanol for cooking is likely to replace fuelwood because the latter is the main energy resource used as cooking fuel in Southern Africa (greater than 90%), except in South Africa, where electricity is the primary cooking fuel for approximately 60% of households (Adkins et al., 2012; IEA, 2014a).
Both scenarios correspond to a mill-crushing capacity of one million t of sugarcane and distillery consumption of 30 kWh/t cane (mechanical and electrical energy) (Dias et al., 2011). Further assumptions include the following:
• CM Scenario: Ethanol is produced exclusively from existing molasses, and no additional sugarcane production occurs. We assumed a low efficiency for the cogeneration system, with the ability to generate 60 kWh/t cane (42 bar, 450°C) (BNDES/CGEE, 2008). We consider that all the existing sugarcane industries will be able to deploy such as system, if it does not exist already.
• NP Scenario: This scenario is based on the 2030 scenario proposed by the International Energy Agency (IEA, 2014a). Ethanol is produced from molasses and additional sugarcane (direct juice), which is cultivated over 1% of the current pasture land. For each country, we assessed the availability of suitable areas for sugarcane cultivation. The efficiency for the cogeneration system in this scenario is higher, with a capacity of 110 kWh/t cane (65 bar, 480°C) (BNDES/CGEE, 2008).
Uncertainty Analysis
The overall uncertainty of ethanol and electricity production, beyond the potential to address cooking and transportation demand, was evaluated by applying a stochastic approach based on the Monte Carlo method. In this method, an appropriate probability distribution is associated with each of the input parameters subjected to uncertainties (Table 3). Values for these parameters are generated randomly and combined with other randomly generated values; we use 10,000 trials. The results are presented as an average value associated with a probability distribution for all possible outputs (ADB, 2002).
Greenhouse Gas Emission
We assessed the GHG emission savings given the replacement of fossil fuels by ethanol and the use of bagasse as bioelectricity source instead of the current electricity mix employed in each country. We considered the emissions throughout the life cycle. The carbon emission was evaluated for the NP scenario to identify the potential carbon savings if the countries invested in improving their energy generation profile for 2030, i.e., using ethanol as vehicle fuel instead of gasoline and diesel and bagasse for bioelectricity generation rather than maintaining the current scenario. We did not estimate the GHG emissions savings for the CM scenario; indeed, suggesting energy replacement in this scenario is not sensible because most of the analyzed countries still lack access to electricity or have a latent demand for fuels. In the NP scenario, fuels are not replaced; instead, the energy sector expansion in the coming years is reevaluated.
The GHG emission factors for electricity production were estimated based on the life cycle emissions of energy systems (Table 3) and the current electricity generation (Table 4). As for ethanol, gasoline, diesel, and electricity from bagasse cogeneration, the GHG emission factors correspond to the life cycle carbon emissions (Table 5).
Investment
We estimate the investment required to implement the additional sugarcane industrial plants for the NP scenario considering an industry crushing capacity of 1 million t per year and an investment of 212 US$/t cane annual crushing capacity (BNDES, 2014), including the agricultural sector, sugarcane mill, and cogeneration system. We assume the investment would occur over 10 years, and thus divided the total investment by 10 and compared the annual investment with the GDP at market price and to the gross fixed capital formation. We do not consider investments in the distribution and transmission systems, assuming that these investments will happen regardless.
Energy Demand Projection
To identify the potential electricity consumption in 2030 for the NP scenario, we applied a 47% rate of increase for South Africa and a 73% rate of increase for the remaining countries relative to 2012 (annual growth rates of 2 and 3%, respectively) (IEA, 2014a) (Table 6). Gasoline and diesel consumption increase by 67 and 50% from 2012 to 2030, corresponding to 2 and 3% p.a., respectively (IEA, 2014b).
Results and Discussion
We evaluated the potential of sugarcane to provide cleaner and more accessible energy in Southern Africa by considering a short-term framework, named CM scenario, and an enhanced approach likely to be deployed over the medium to long term, entitled NP scenario. Results show the potential of energy supply, the GHG emissions savings, and the total investment required to enlarge the sugarcane production, with further discussion on challenges for implementing bioenergy systems in Africa.
Potential Modern Energy Supply and Fossil Fuel Displacement
We found good prospects for implementing modern sugarcane bioenergy in Southern Africa, with large differences among countries (Table 7). By using the existing molasses for ethanol production, Swaziland could meet 57 ± 6% of the household cooking energy demand or displace 30 ± 3% of fossil fuel use. Ethanol is already produced from molasses in this country but is mostly used in the beverage and pharmaceutical industries (IRENA, 2014).
By using 1% of pasture land for sugarcane cultivation, ethanol could meet up to 50% of the cooking fuel demand for most of Southern African countries. A low availability of pasture land reflects, in principle, a lower potential for sugarcane ethanol production and, thus, for displacement of fossil fuels, as found in Mauritius and Tanzania. In countries with low consumption of liquid fuels and large availability of land, such as Mozambique and Zambia, sugarcane expansion plays a high potential to replace fossil fuel. Even though the assumption of 1% of the pasture land may disadvantage countries with low availability of grazing area, it is more realistic than proposing a percentage of sugarcane expansion based on the energy demand, and also 1% is in accordance with the suitable area for sugarcane (Table 2), especially in a scenario of pasture intensification (Latawiec et al., 2014).
In some cases, the need for ethanol for a specific purpose is lower than its production; therefore, the excess could be used as a fuel for both cooking and vehicles (Table 7). In our analysis, we considered a progressive increase in the electricity and transportation fuel consumptions, according to IEA (2014a), which would be related to an economic growth and thus a higher energy consumption. Even assuming that, the ethanol supply would be able to attend a large share of the fuel demand in the future NP scenario (Table 7).
Using ethanol as a cooking fuel could help to substantially reduce or even eliminate the traditional use of biomass for cooking in most Southern African countries (Figure 1). Approximately 85 million t of firewood per year could be saved by implementing the NP scenario in all of the Southern African countries; this would reduce forest exploitation by 145 million ha [estimated assuming the use of 1.5 kg of firewood per capita per day (IEA, 2014b)], 0.9 ha per capita of forest for firewood gathering (IEA, 2014a), and the population projected for 2030 (Table 6). The forest area required for firewood extraction depends on management and biomass stocks, which are not uniform across countries.
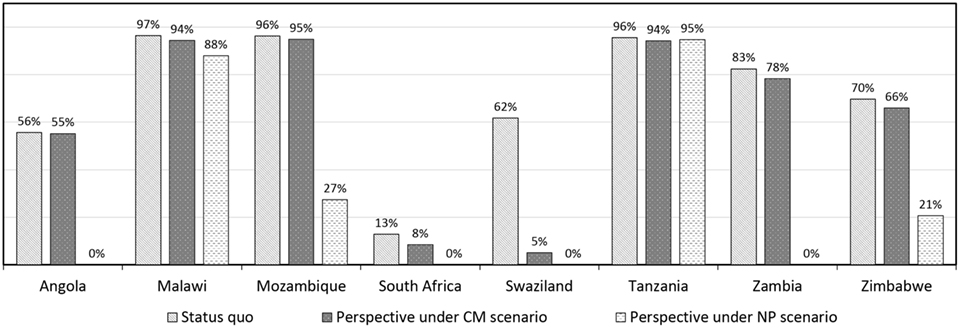
Figure 1. Population relying on traditional use of biomass for cooking. The potential replacement of traditional biomass when ethanol is used exclusively as cooking fuel. Status quo as reported by the International Energy Agency (IEA, 2014a). 0% indicates total replacement of traditional biomass.
Enlarging Electricity Access
We identified attractive opportunities to enhance electricity access by burning sugarcane bagasse in a cogeneration system, especially for Swaziland, where bioelectricity production under the CM scenario could increase current generation by 38 ± 4% (Figure 2) and using bagasse under the NP scenario could increase electricity production by 70 ± 8%. The existing bioelectricity produced from sugarcane bagasse in Swaziland is completely consumed by the industry itself. However, the sugarcane mills could provide surplus power to the grid by implementing higher efficient boilers (IRENA, 2014), as in the NP scenario. In Angola, bagasse could contribute highly to power generation under the NP scenario (Figure 2). The lack of electricity for Southern African households would be significantly reduced, or even eliminated, by a slight expansion of sugarcane and the use of bagasse as an energy source (Figure 3).
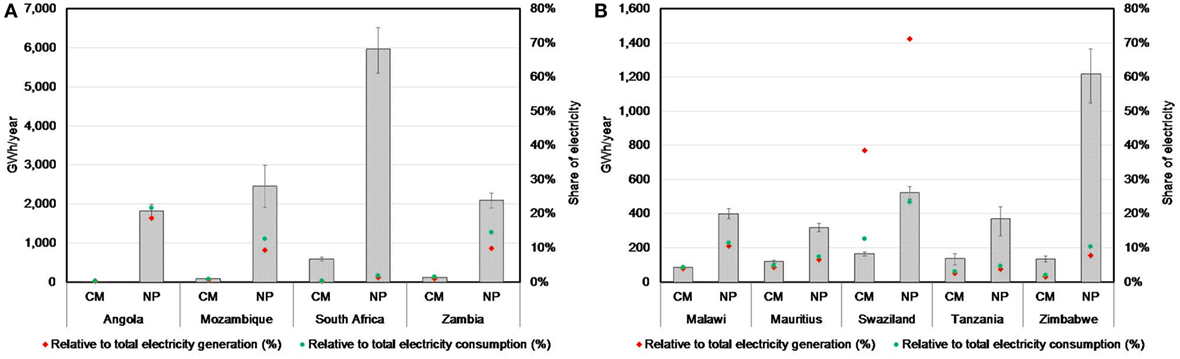
Figure 2. Potential bioelectricity supply. The graphs are split to facilitate visualization: (A) larger producers and (B) smaller producers. The columns show the mean values, and bars represent the 25th and 75th percentiles from the Monte Carlo simulation. The difference between the red and green markers indicates the external dependency on electricity.
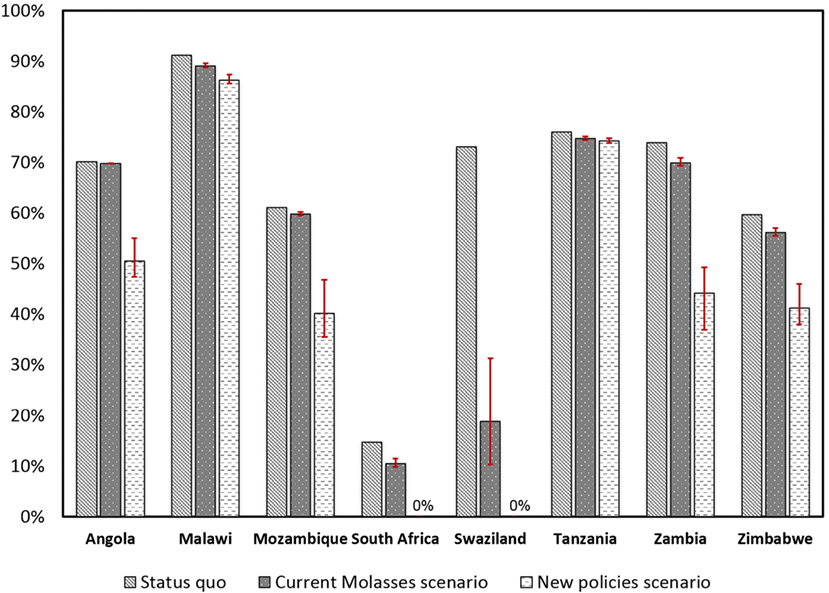
Figure 3. Population without access to electricity. The new perspectives for electricity shortage when implementing the Current Molasses (CM) and New Policies (NP) scenarios. Status quo corresponds to the current situation as reported by the International Energy Agency (IEA, 2014a). Household electricity demands were estimated considering average annual consumptions of 1,120 and 1,430 kWh (Castellano et al., 2015) per household for the CM and NP scenarios, respectively. The columns show the mean values, and the bars represent the 25th and 75th percentiles from the Monte Carlo simulation. Swaziland and South Africa can fully meet the residential demand in the NP scenario.
GHG Implications
Because more than 90% of the electricity generation in South Africa is derived from coal (Table 4) the use of bagasse as an electricity source could eliminate 940-4,800 kt CO2e/year (25th–75th percentiles), in addition to an annual reduction potential of 900-9800 or 550-6,000 kt CO2e by displacing diesel or gasoline, respectively (Figure 4A). Electricity generation in Mozambique and Zambia is based on hydropower. Increasing bagasse use over hydropower would impair the current power profile because of higher life cycle GHG emissions from the biomass (Tables 3 and 5). However, the potential reduction in GHG emissions resulting from displacing fossil fuel compared with 2012 emissions could be as high as 50–70% in these countries. In Swaziland, where 70% of the electricity production is based on coal (Table 4), sugarcane could also contribute to a cleaner energy sector by reducing the annual GHG emissions by more than 60% (Figure 4B).
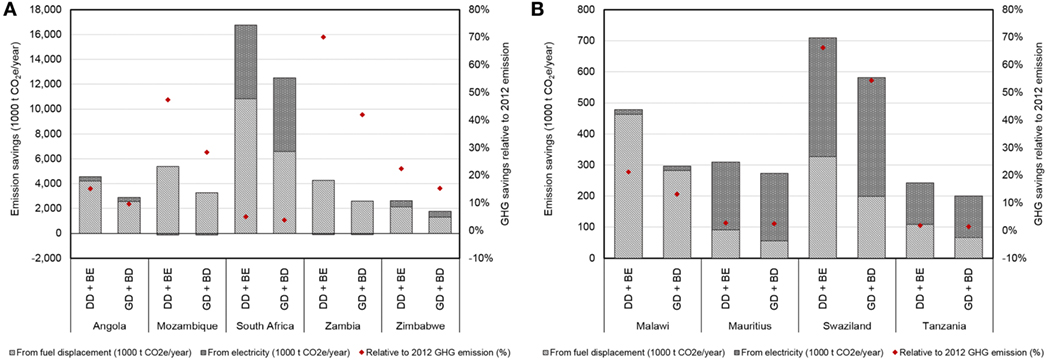
Figure 4. Greenhouse gas (GHG) emission savings by introducing sugarcane as energy source (2030 scenario). The primary axis represents the emissions savings from promoting bagasse as an electricity source (BE), rather than keeping the current electrical system, and displacing diesel (DD) or gasoline (GD) by implementing ethanol use. The secondary axis corresponds to the relative GHG emissions savings compared with 2012 fossil fuel emissions attributed to goods and services discounted from cement manufacturing emissions; data (UNFCCC, 2014; Boden et al., 2015; British Petroleum, 2015) were retrieved from the Global Carbon Atlas (2012). The graphs are split to facilitate visualization: (A) larger scale emission and (B) smaller scale emission. The columns show the mean values from the Monte Carlo simulation.
Investments and Land Use
The investment needed to expand sugarcane production and processing over 1% of the pasture land varies according to the available area of each country. The required annual investment for all of the countries is insignificant compared to the GDP at the market price. However, in Mozambique, it represents more than 30% of the fixed capital formation and, thus, may be prohibitively high (Table 8). In South Africa, it would be infeasible to invest in 55 new sugarcane industries.
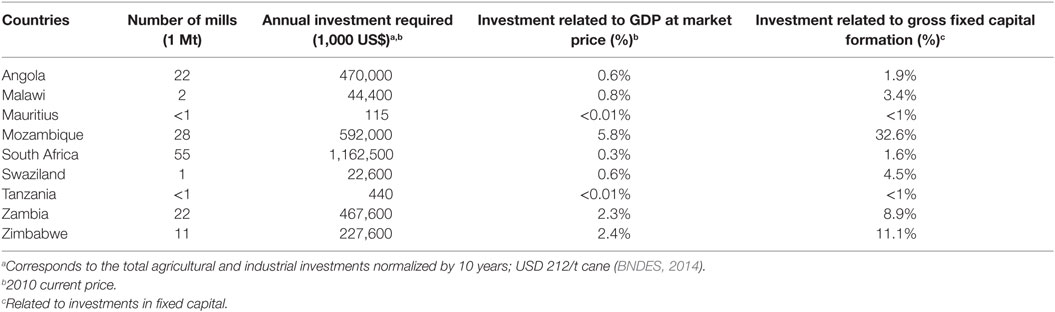
Table 8. Number of new 1 million t sugarcane mills and total investment for the new policies scenario.
With regard to the sugarcane expansion in the NP scenario, the additional area at a national level is quite small compared to the current sugarcane production in the Southern Africa countries, except for Angola in which the required area would correspond to over 40% of the existing crop area and thus would place a barrier for the NP scenario (Table 2). However, a deeper analysis at local level is essential to identify the need for irrigation and the soil and climate conditions.
Challenges in Implementing Sugarcane Energy in Southern Africa
Despite the potential of energy production using sugarcane, Southern Africa countries face a number of economic, social, and environmental challenges that can hamper the development of bioenergy systems. Among them are the need of adequate logistics infrastructure and the limited number of trained professionals, which reduces productivity. The situation is further aggravated by land acquisition schemes, in which the legal procedures make the investment processes quite difficult, by corruption, abuse of human rights and lack of governance transparency, which compromises the assurance of energy programs (Mwakasonda and Farioli, 2012), and by the access to affordable loans and sound business models (Rutz and Janssen, 2012).
Hence, policies must take the people’s welfare and the sustainable supply as first priorities through implementing and integrating effective plans for land use, energy, agriculture, and rural development focused on employment opportunities, education, and energy security. However, policies must be aligned with strategies to limit negative impacts of biofuels by promoting and applying sustainability standards and criteria based on aspects such as biodiversity, GHG emissions, land use, and water use. Furthermore, Africa is predicted to experience designed to undergo the adverse effects of the climate change on the agriculture systems, such as land degradation, water stress, increased occurrence of pests, droughts and floods, and loss of yield, which require adequate strategies to address such impacts, besides developing more accurate researches in this region to ensure the real effects of climate change (Malaviya and Ravindranath, 2012). Cooperation among African countries could boost the development of regional strategies and technologies suitable for their reality.
Under planning and monitoring actions, bioenergy can enhance agricultural and technological progress, boost social growth, and contribute to the development of the food sector and well-being of Africans at the local level. These results require efficient enterprises, financial mechanisms, and government support for research, education, and agriculture, such as extension services to guide farmers. International agreements, such as carbon emission reduction policies, can play an important role in promoting modern energy in developing countries.
Sugarcane can serve as a core bioenergy source for the growing energy demands of African countries and help to reduce GHG emissions and fossil fuel imports by changing their current energy generation profiles. However, displacing the use of fossil fuels and nonsustainable bioenergy requires new perspectives and solutions throughout the energy life cycle to ensure modern energy access. Additionally, implementing the scenarios proposed in this study are associated with improvements in biomass production, conversion technology, rural infrastructure, and societal integration (Lynd and Woods, 2011), especially for the NP scenario, which relies on more efficient technologies.
Conclusion
This study confirms the great potential for sugarcane ethanol production, the good prospects for using this biofuel in cooking stoves and transport sector in Southern Africa, and the opportunity for this sector to contribute to enhancing electricity access in the long term. As consequence of promoting the sugarcane sector for energy proposal, there are benefits regarding the reduction in fossil fuel consumption and the external dependency in fossil fuels imports, as well as reducing the GHG emission, in line with the voluntary national pledges assumed in COP21.
The development of sugarcane bioenergy is aligned with the goals proposed by the United Nations at the Sustainability Energy for All Program (SE4ALL): universal energy access, renewable energy, and energy efficiency. We believe that this study can help decision-makers and stakeholders on planning energy strategies in Southern Africa based on sugarcane as alternative to promote the modern energy supply. However, challenges such as financing the agroindustry, transport infrastructure, education and personnel training, and regulatory adjustment must be properly evaluated and solved, and a clear and effective government commitment is essential to make the sustainable sugarcane bioenergy a reality in Southern Africa.
Author Contributions
SS was the primary author of the manuscript, performed the research, collected the data, analyzed the results, applied the uncertainty analysis, and elaborated the tables and charts. LN was the leader of the paper and an active coauthor, contributed in elaborating the scope, charts, and tables, and also played an important role in analyzing and discussing the results. HW contributed to the land use data and provided a deeper analysis and perspective of the sugarcane development in Southern Africa. LL played an important role in editing the manuscript and defining the scope of the study and also performed a deep analysis of the results. ME provided perspectives on bioenergy deployment in Southern Africa, especially the challenges for implementing sugarcane sector in this region. LC is the leader of the research project and was involved in the discussion of scope and results.
Conflict of Interest Statement
The authors declare that the research was conducted in the absence of any commercial or financial relationships that could be construed as a potential conflict of interest.
Acknowledgments
Special thanks to professor Marcelo Cunha for his contribution to the discussion of the economic aspects and the uncertainty analysis.
Funding
This research was funded by São Paulo Research Foundation (FAPESP) under Grants 2012/00282-3 and 2015/02270-0.
Supplementary Material
The Supplementary Material for this article can be found online at http://journal.frontiersin.org/article/10.3389/fenrg.2016.00039/full#supplementary-material.
References
ADB. (2002). Handbook for Integrating Risk Analysis in the Economic Analysis of Projects. Manila: Asian Development Bank.
Adkins, E., Oppelstrup, K., and Modi, V. (2012). Rural household energy consumption in the millennium villages in Sub-Saharan Africa. Energy Sustain. Dev. 16, 249–259. doi:10.1016/j.esd.2012.04.003
ANEEL Fontes de Energia: Biomassa. (2015). Banco de Informações de Geração. Available at: http://www2.aneel.gov.br/aplicacoes/capacidadebrasil/CombustivelPorClasse_fn1.cfm
Biocom. (2015). The Company: Biocom in Numbers. Available at: http://www.biocom-angola.com/en/company/biocom-numbers
BNDES. (2014). Estudo de Viabilidade de Produção de Biocombustíveis na UEMOA (União Econômica e Monetária do Oeste Africano). Rio de Janeiro, RJ: Banco Nacional de Desenvolvimento Econômico e Social.
BNDES/CGEE. (2008). “Ethanol as vehicle fuel,” in Sugarcane-Based Bioethanol: Energy for Sustainable Development (Rio de Janeiro, RJ: Banco Nacional de Desenvolvimento Econômico e Social, Centro de Gestão e Estudos Estratégicos), 304. Available at: http://sugarcane.org/resource-library/studies/BNDES%20-%20Sugarcane%20Based%20Bioethanol.pdf
Boden, T., Marland, G., and Andres, R. (2015). Global, Regional, and National Fossil-Fuel CO2 Emissions. Oak Ridge, TN, USA: Carbon Dioxide Information Analysis Center, Oak Ridge National Laboratory, U.S. Department of Energy.
British Petroleum. (2015). BP Statistical Review of World Energy. 48. Available at: http://scholar.google.com/scholar?hl=en&btnG=Search&q=intitle:BP+Statistical+Review+of+World+Energy#0
Castellano, A., Kendall, A., Nikomarov, M., and Swemmer, T. (2015). Brighter Africa: The Growth Potential of the Sub-Saharan Electricity Sector. New York, NY. Available at: http://www.mckinsey.com/~/media/mckinsey/dotcom/insights/energyresourcesmaterials/poweringafrica/brighter_africa_the_growth_potential_of_the_sub-saharan_electricity_sector.ashx
Chakaniza, L. (2013). Production of Ethanol for National Consumption in Malawi. Maputo, Mozambique: Workshop on Sustainable Biomass Production in Southern Africa. Available at: https://www.b2match.eu/system/biomass-workshop-2013-maputo/files/CHAKANIZA.pdf?1364980612
Dasappa, S. (2011). Potential of biomass energy for electricity generation in sub-Saharan Africa. Energy Sustain. Dev. 15, 203–213. doi:10.1016/j.esd.2011.07.006
De Cerqueira Leite, R. C., Verde Leal, M. R. L., Barbosa Cortez, L. A., Griffin, W. M., and Gaya Scandiffio, M. I. (2009). Can Brazil replace 5% of the 2025 gasoline world demand with ethanol? Energy 34, 655–661. doi:10.1016/j.energy.2008.11.001
Dias, M. O. S., Cunha, M. P., Jesus, C. D. F., Rocha, G. J. M., Pradella, J. G. C., Rossell, C. E. V., et al. (2011). Second generation ethanol in Brazil: can it compete with electricity production? Bioresour. Technol. 102, 8964–8971. doi:10.1016/j.biortech.2011.06.098
Dunkelberg, E., Finkbeiner, M., and Hirschl, B. (2014). Sugarcane ethanol production in Malawi: measures to optimize the carbon footprint and to avoid indirect emissions. Biomass Bioenergy 71, 37–45. doi:10.1016/j.biombioe.2013.10.006
EHA. (2011). BioEthanol for Sustainable Transport – Results and Recommendations from the European Best Project, eds P. Fenton, and H. Carlsson (Stockholm, Sweden: Environment and Health Administration (EHA)).
EIA. (2012). International Energy Statistics. Available at: http://www.eia.gov/cfapps/ipdbproject/IEDIndex3.cfm?tid=5&pid=5&aid=2
FAO. (2012a). Inputs, Land. FAOSTAT. Available at: http://faostat3.fao.org/download/R/RL/E
FAO. (2012b). Natural Resource Assessment for Crop and Land Suitability: An Application for selected bioenergy crops in Southern Africa region. Rome, Italy: Integrated.
FAO. (2012c). Population, Annual Population. FAOSTAT. Available at: http://faostat3.fao.org/download/O/OA/E
FAO. (2012d). Production, Crops. FAOStat. Available at: http://faostat3.fao.org/download/Q/QC/E
FAO. (2013a). Food Balance Sheets. FAOStat. Available at: http://faostat3.fao.org/download/FB/FBS/E
FAO. (2013b). Trade. FAOStat. Available at: http://faostat3.fao.org/download/T/*/E
Global Carbon Atlas. (2012). Territorial Emission. Available at: http://www.globalcarbonatlas.org/?q=en/emissions
Hermann, S., Miketa, A., and Fichaux, N. (2014). Estimating the Renewable Energy Potential in Africa. Abu Dhabi: IRENA-KTH working paper.
ICF International. (2012). United States Agency for International Development. Mean Number of Household Members. STAT Compil. – Demogr. Heal. Surv. Progr. Available at: http://www.statcompiler.com/en/index.html
IEA. (2012). International Energy Agency: Statistics. Electr. Heat. Available at: http://www.iea.org/statistics/statisticssearch/
IEA. (2014a). Africa Energy Outlook: A Focus on Energy Prospects in Sub-Saharan Africa, ed. R. Priddle (Paris, France: Organisation for Economic Co-operation and Development (OECD), International Energy Agency (IEA)).
IRENA. (2014). Swaziland: Renewables Readiness Assessment. Abu Dhabi, United Arab Emirates: International Renewable Energy Agency (IRENA).
IRENA-DBFZ. (2013). Biomass Potential in Africa, eds K. Stecher, A. Brosowski, and D. Thrän (Abu Dhabi, United Arab Emirates: International Renewable Energy Agency (IRENA)). Available at: http://www.irena.org/DocumentDownloads/Publications/IRENA-DBFZ_Biomass%20Potential%20in%20Africa.pdf
Johnson, F. X., and Seebaluck, V. (2012). Bioenergy for Sustainable Development and International Competitiveness: The Role of Sugar Cane in Africa. London and. New York: Routledge Taylor & Francis Group.
Kimemia, D., Vermaak, C., Pachauri, S., and Rhodes, B. (2014). Burns, scalds and poisonings from household energy use in South Africa: are the energy poor at greater risk? Energy Sustain. Dev. 18, 1–8. doi:10.1016/j.esd.2013.11.011
Latawiec, A. E., Strassburg, B. B. N., Valentim, J. F., Ramos, F., and Alves-Pinto, H. N. (2014). Intensification of cattle ranching production systems : socioeconomic and environmental synergies and risks in Brazil. Animal 8, 1255–1263. doi:10.1017/S1751731114001566
Lynd, L. R., and Woods, J. (2011). Perspective: a new hope for Africa. Nature 474, S20–S21. doi:10.1038/474S020a
Macauhub. (2015). Start of Ethanol and Sugar Production Increases Diversification of Angola’s Economy. Features. Available at: http://www.macauhub.com.mo/en/2015/06/01/start-of-ethanol-and-sugar-production-increases-diversification-of-angolas-economy/
Malaviya, S., and Ravindranath, N. H. (2012). “Implications of climate change on sustainable biofuel production in Africa,” in Bioenergy for Sustainable Development in Africa, eds R. Janssen, and D. Rutz (Munich: Springer), 281–298.
Moomaw, W., Burgherr, P., Heath, G., Lenzen, M., Nyboer, J., and Verbruggen, A. (2012). “Annex II: methodology,” in Renewable Energy Sources and Climate Change Mitigation: Special Report of the Intergovernmental Panel on Climate Change, eds O. Edenhofer, R. Pichs-Madruga, Y. Sokona, K. Seyboth, P. Matschoss, S. Kadner, et al. (New York, NY: Intergovernmental Panel on Climate Change), 1076. Available at: http://www.ipcc.ch/pdf/special-reports/srren/Annex%20II%20Methodology.pdf
Munyinda, K., Yamba, F. D., and Walimwipi, H. (2012). “Bioethanol potential and production in Africa: sweet Sorghum as a complementary feedstock,” in Bioenergy for Sustainable Development in Africa, eds R. Janssen, and D. Rutz (Munich: Springer), 81–91. doi:10.1007/978-94-007-2181-4_8
Mwakasonda, S., and Farioli, F. (2012). “Social impacts of biofuel production in Africa,” in Bioenergy for Sustainable Development in Africa, eds R. Janssen, and D. Rutz (Munich: Springer), 323–334.
Novozymes. (2012). CleanStar Mozambique Launches World’s First Sustainable Cooking Fuel Facility. Bioenergy, Corp. News. Available at: http://novozymes.com/en/news/news-archive/Pages/CleanStar-Mozambique-launches-world’s-first-sustainable-cooking-fuel-facility.aspx
Nylund, N.-O., Laurikko, J., Laine, P., Suominen, J., and Anttonen, M. P. A. (2013). Benchmarking heavy-duty ethanol vehicles against diesel and CNG vehicles. Biomass Convers. Biorefinery 3, 45–54. doi:10.1007/s13399-012-0049-z
REN21. (2014). Renewables 2014 Global Status Report. Paris: Renewable Energy Policy Network for the 21st Century. Available at: http://www.ren21.net/Portals/0/documents/Resources/GSR/2014/GSR2014_full report_low res.pdf
REN21. (2015a). Renewables 2015 – Global Status Report. France: Renewable Energy Policy Network for the 21st Century.
REN21. (2015b). Status Report: SADC Renewable Energy and Energy Efficieny. France: Renewable Energy Policy Network for the 21th Century.
Rodrigues Filho, J. P. (2005). “Trash recovery cost,” in Biomass power generation: Sugar cane bagasse and trash, eds S. J. Hassuani, M. R. L. V. Leal, and I. de Carvalho Macedo (Piracicaba: PNUD – Programa das Nações Unidas para o Desenvolvimento, CTC – Centro de Tecnologia Canavieira), 74–85. Available at: http://www.ctcanavieira.com.br/site/images/downloads/biomassa.pdf
Rutz, D., and Janssen, R. (2012). “Opportunities and risks of bioenergy in Africa,” in Bioenergy for Sustainable Development in Africa, eds R. Janssen, and D. Rutz (Munich: Springer), 391–400.
Schulze, R. E., Maharaj, M., Lynch, S. D., Howe, B. J., and Melvil-Thompson, B. (1997). South African Atlas of Agrohydrology and Climatology. Pretoria, South Africa: WRC Report No. TT 82/96.
Seabra, J. E. A., and Macedo, I. C. (2011). Comparative analysis for power generation and ethanol production from sugarcane residual biomass in Brazil. Energy Policy 39, 421–428. doi:10.1016/j.enpol.2010.10.019
Seabra, J. E. A., Macedo, I. C., Chum, H. L., Faroni, C. E., and Sarto, C. A. (2011). Life cycle assessment of Brazilian sugarcane products: GHG emissions and energy use. Biofuels Bioprod. Biorefining 5, 519–532. doi:10.1002/bbb.289
Souza, S. P., De Ávila, M. T., and Pacca, S. (2012). Life cycle assessment of sugarcane ethanol and palm oil biodiesel joint production. Biomass Bioenergy 44, 70–79. doi:10.1016/j.biombioe.2012.04.018
Taliotis, C., Shivakumar, A., Ramos, E., Howells, M., Mentis, D., Sridharan, V., et al. (2016). An indicative analysis of investment opportunities in the African electricity supply sector – using TEMBA (the electricity model base for Africa). Energy Sustain. Dev. 31, 50–66. doi:10.1016/j.esd.2015.12.001
UNFCCC. (2013). CDM Project: Cleanstar Mozambique – Maputo Ethanol Cookstove and Cooking Fuel Project 1. Mozambique: CleanStar Mozambique Community Bioinnovation Ltd; Merrill Lynch Commodities (Europe) Ltd.
UNFCCC. (2014). National Inventory Submissions. Available at: http://unfccc.int/national_reports/annex_i_ghg_inventories/national_inventories_submissions/items/8108.php
United Nations. (2006). Costos y Precios para Etanol Combustible en América Central, ed. L. A. H. Nogueira (México: Comisión Económica para América Latina y El Caribe (CEPAL)).
United Nations. (2007). Feasibility Study for the Use of Ethanol as a Household Cooking Fuel in Malawi – Draft Final Report, ed. Ethio Resource Group Pvt. Ltd., Growing Sustainable Business for Poverty Reduction Program in Malawi (Lilongwe, Malawi: The United Nations Development Program (UNDP))
Wang, M., Sabbisetti, R., Elgowainy, A., Dieffenthaler, D., Anjum, A., Sokolov, V., et al. (2014). GREET Model: The Greenhouse Gases, Regulated Emissions, and Energy Use in Transportation Model. Chicago, USA: Argonne National Laboratory.
Watson, H. K. (2011). Potential to expand sustainable bioenergy from sugarcane in southern Africa. Energy Policy 39, 5746–5750. doi:10.1016/j.enpol.2010.07.035
WHO. (2013). Database on use of solid fuels. World Heal. Organ. Available at: http://apps.who.int/gho/data/view.main.37000
Keywords: sustainability, bioenergy, bioelectricity, sugarcane ethanol, traditional biomass
Citation: Souza SP, Horta Nogueira LA, Watson HK, Lynd LR, Elmissiry M and Cortez LAB (2016) Potential of Sugarcane in Modern Energy Development in Southern Africa. Front. Energy Res. 4:39. doi: 10.3389/fenrg.2016.00039
Received: 20 May 2016; Accepted: 09 December 2016;
Published: 26 December 2016
Edited by:
Fu Zhao, Purdue University, USAReviewed by:
Subbu Kumarappan, Ohio State University, IndiaKarl Haapala, Oregon State University, USA
Copyright: © 2016 Souza, Horta Nogueira, Watson, Lynd, Elmissiry and Cortez. This is an open-access article distributed under the terms of the Creative Commons Attribution License (CC BY). The use, distribution or reproduction in other forums is permitted, provided the original author(s) or licensor are credited and that the original publication in this journal is cited, in accordance with accepted academic practice. No use, distribution or reproduction is permitted which does not comply with these terms.
*Correspondence: Simone P. Souza, c3Auc291emFAeWFob28uY29tLmJy