- Oceanography Center, University of Cyprus, Nicosia, Cyprus
This paper describes the status of the potential of blue energy (BE) in the Mediterranean region, with focus on the region around Cyprus. Previous studies are reviewed, the main findings of the blue energy potential analysis performed in the frame of the MAESTRALE project are presented, and the most promising blue energy sources for the Mediterranean are highlighted. The findings of this report suggest that there is a good exploitability potential of different forms of BE in the Mediterranean. The most highlighted BE form for the Mediterranean region is offshore wind energy. This is also true for Cyprus, where marine biomass follows as the second most promising blue energy form. Marine thermal energy can also be used for heating and cooling. The main physical barrier for the implementation of BE projects is the bathymetry around the island.
Introduction
Energy demand increases year by year, while the current primary energy mix is made up from more than 80% of fossil fuels (IEA, 2015). As a result, the energy sector is responsible for a significant percentage of CO2 emissions globally (IEA, 2015). The European Union (EU) Renewables Directive addresses two of the biggest challenges of our time; energy security, and climate change. In 2004, the European Renewable Energy Council (EREC) set a binding energy target of at least 20% renewable for the EU by 2020 (Zervos et al., 2011). The respective targets of the European commission for 2030 were set to at least 32% (European Commission, 2018). This target is crucial not only for securing the energy supply in the European continent, but also for the mitigation of the climate change consequences and the enhancement of the competitiveness of the economy.
Therefore, the energy sector must turn to new energy sources and more efficient technologies in order to fill the energy demand with clean energy, such as onshore renewable energy sources and ocean energy. While the onshore renewable energy forms, such as photovoltaics, solar thermal, geothermal, wind onshore, biogas, biomass, and other forms, are more developed today, the offshore ocean energy types are less exploited. The ocean is regarded as a vast source of renewable and clean energy that exceeds our present and projected future energy needs many times (Takahashi and Trenka, 1996) and is expected to play a crucial role in the future energy system (Magagna and Uihlein, 2015). It thus has the potential to help reduce CO2 emissions and alleviate the global climate change threat. Nevertheless, it is also critically important that the development of new ocean energy technologies does not harm the marine environment (Pelc and Fujita, 2002).
Blue energy (BE) is not strictly defined in the literature. Initially, this term was used to describe only the energy produced by exploiting salinity differences between fresh and salty water (Ross and Krijgsman, 2004; Kuleszo et al., 2010). More generally, this term describes the energy coming from any form of offshore marine renewable source (Soma and Haggett, 2015; Lillebø et al., 2017). According to Ellabban et al. (2014), ocean energy is the energy coming from waves, tidal currents, ocean currents, salinity gradient, and ocean thermal energy conversion (or temperature gradient energy).
EU is currently at the forefront of blue energy development (Magagna and Uihlein, 2015) but this is still a nascent industry. Even though the highest potential for the development of ocean energy is in the Atlantic seaboard, it is accepted that there exists potential also in the Mediterranean and the Baltic basins. Magagna and Uihlein (2015) presented a critical review of the status of ocean energy technologies. They concluded that tidal and wave energy represent the two most advanced and promising types of ocean energy technologies in converting ocean energy into renewable low-carbon electricity and noted that tidal energy technologies are expected to become commercially viable before wave energy.
In this paper, the energy potential analysis conducted for the purposes of the Maestrale project is presented with emphasis on the region of Cyprus. The Maestrale project, is an Interreg MED 2014-2020 Programme co-financed by the European Regional Development Fund. The University of Siena, (UNISI) coordinates a consortium of 10 partners from Italy, Greece, Malta, Spain, Portugal, Croatia, Slovenia, and Cyprus (Oceanography Centre, University of Cyprus). This project intends to lay the foundation for a Maritime Energy Deployment Strategy in the Mediterranean. The three main objectives of the project are: (i) Knowledge transfer between the partners and professionals who already have experience in the sector outside the MED area; (ii) Creation of regional and transnational networks (Blue Energy Labs) of key stakeholders such as policy makers, public authorities, research institutions, entrepreneurs and citizens, in order to promote and establish BE projects; and (iii) Elaboration of two or more pilot projects in each regional area with the highest feasibility conditions for the region. More information about the MAESTRALE project can be found at https://maestrale.interreg-med.eu/.
Energy Potential in The Mediterreanean
In the framework of the Maestrale project, Blue Energy is considered in a broader sense and includes: (i) wave energy (offshore and onshore), which can be embedded on manmade structures, such as ports and wave-breakers, or on floating buoys; (ii) offshore wind energy by means of floating or fixed-foundation turbines; (iii) marine biomass, which includes sea weed farms or micro-algae absorbing seawater nutrients and CO2; (iv) salinity gradient energy, i.e., energy extracted by exploiting the difference of salt concentration between fresh and salty water; (v) ocean thermal energy, where the temperature difference between air and ocean or between different ocean layers is exploited for cooling or heating buildings; and (vi) marine current energy, using floating, seabed moored, and kite-like turbines.
According to the national Renewable Energy Sources (RES) Industry Roadmaps developed in the framework of the REPAP2020 project (Zervos et al., 2011), ocean energy is planned to represent 0.15% of electricity consumption in 2020. Installed capacity is expected to rise from 245 MW in 2010 to 2,543 MW in 2020. The main markets in the Mediterranean in 2020 will be Portugal, France and Spain, while in the rest of Europe it will be Ireland and the United Kingdom. Wind energy is expected to produce 495 TWh and represent over 14% of the total electricity consumption in 2020. Wind power installations will grow from around 85 GW in 2010 to over 213 GW in 2020, with a compound annual growth rate of 9.7%. Offshore wind is expected to play a prominent role, having 43 GW of cumulative capacity in 2020. The projections for Renewable Electricity in 2020 from Wind Offshore Energy and Ocean Energy, taken from the data provided in the Zervos et al. (2011), are tabulated in Tables 1 and 2, respectively.
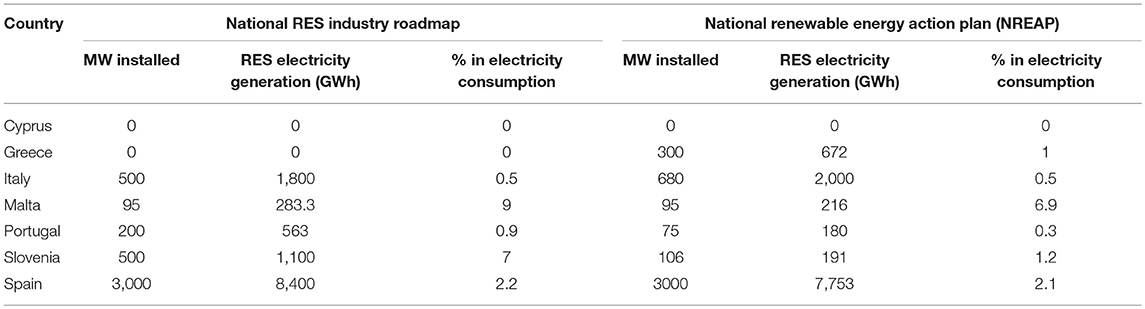
Table 1. Projections for renewable electricity in 2020 from offshore wind energy (Zervos et al., 2011).
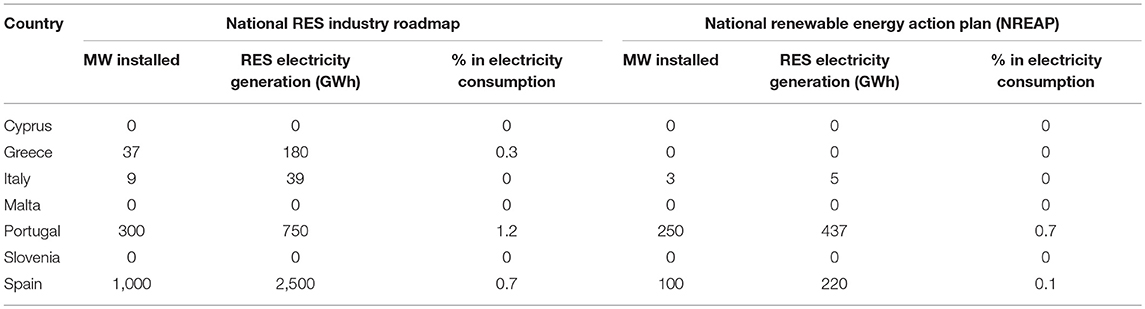
Table 2. Projections for renewable electricity in 2020 from tidal, wave, and ocean energy (Zervos et al., 2011).
To analyse the potential of all BE forms, MAESTRALE partners reviewed and highlighted the most promising sources in their study areas, taking into account physical, legal, technological, economic, and social contexts. The findings of each partner for their region are summarized in Table 3 and in Figure 1. Figure 2 indicates the locations (solid circles) or regions (hollow circles) of the BE forms highligted by each partner.
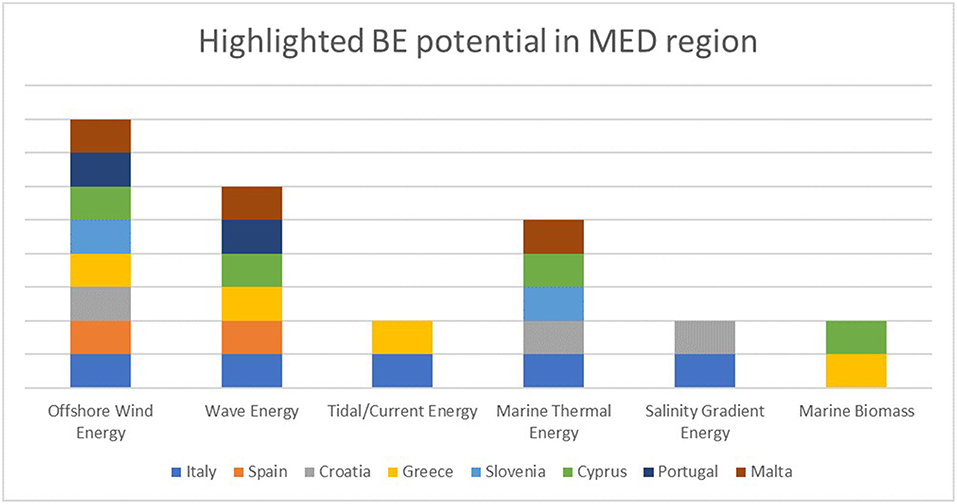
Figure 1. Highlighted BE forms by each country of study. Marine thermal energy is used directly for heating and cooling rather than for electricity production.
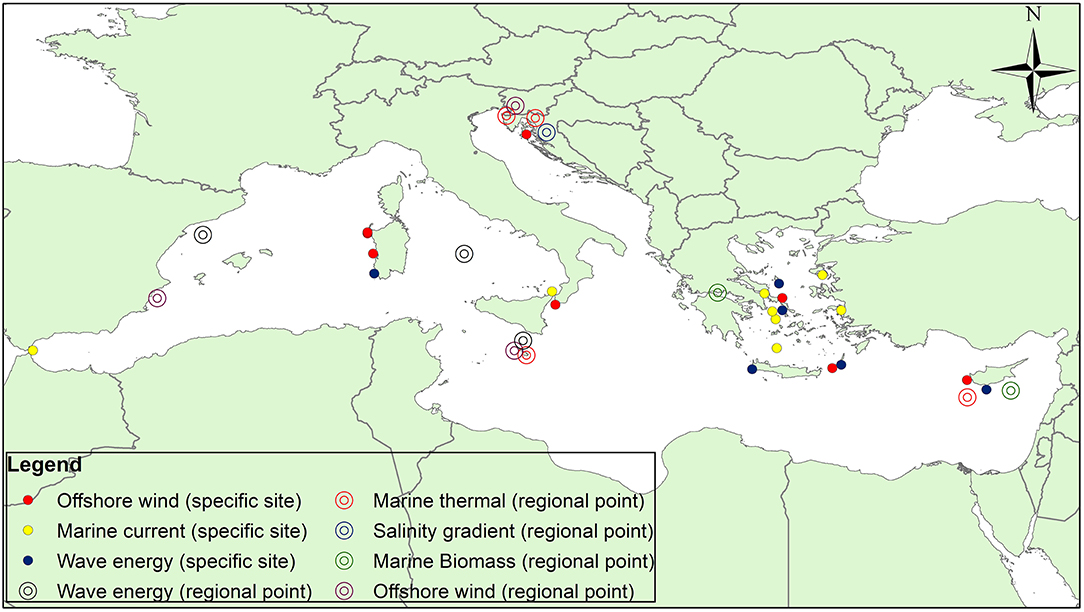
Figure 2. Locations of highlighted BE forms as reported from the partners. Solid circles represent specific locations, while hollow circles represent general regions.
Cyprus
Blue Energy Potential
Exploitation of BE energy resources in Cyprus is of critical importance in order to gradually replace the heavy dependence on fossil fuels with sustainable energy, as required by EU regulations and directives. However, the 20% target set by EU (Zervos et al., 2011) for 2020 proved to be too optimistic. Cyprus achieved only 9.3% by 2016 through the use of wind farms, photovoltaic (PV) systems, solar thermal plants, biomass and biogas utilization plants. Cyprus aims to have 13% of its energy consumption coming from renewables by 2020. The Cypriot National Renewable Energy Action Plan (NREAP) splits the overall 13% renewable energy target for 2020 into 16% renewable electricity, 23.5% renewable heating & cooling and 5% renewable transport (Zervos et al., 2011). However, according to a recent study by the International Renewables Agency (IRENA), Cyprus has the potential to produce 25–40% of its total electricity supply from renewables, mostly solar energy, by 2030; this can be further increased by implementing installations with storage capacity (Cyprus Country Report, 2018). In the Cyprus draft integrated national energy and climate plan for 2021–2030 (Partasides et al., 2019), the renewable energy share targets have been set between 15 and 25% for 2030.
The datasets used to produce mean annual wind speed were produced by SKIRON model of the University of Athens (Kallos et al., 1997). Figure 3 indicates that the mean wind speed, based on data for the period 2010–2017, reaches up to 7 m/s, mainly in the Aegean region and the Karpathian Sea. However, the region around Cyprus does not exhibit such high mean wind values, with the mean wind speed reaching 5.5 m/s at the regions North and South of the island. At the areas East and South of the island the main wind speeds are lower, with mean wind speeds ranging between 3.5–4 m/s. It is visible that the area around Cyprus is not as energetic as the Aegean Sea, but according to Soukissian et al. (2017), an acceptable mean annual wind speed threshold of 4.5 m/s at 10 m height is required for an area to be suitable to accommodate a wind park. As a result, Cyprus is near the lower limit with mean annual wind speeds ranging between 4 and 6 m/s. These wind speeds may allow the creation of a sustainable offshore wind farm but far from the coast. The sustainability of such offshore wind parks might be increased with emerging technologies. Such technologies could be hybrid solutions, harvesting two different energy forms (e.g., wind and wave).
Regarding the wave characteristics, a Cyprus Oceanography Center WAM version was used to produce an analysis for the period 2010–2017. The two main components that characterize wave energy are significant wave height and wave period. The mean significant wave height (SWH) reaches up to 1.2 meters in the EM region (Figure 4). The highest mean values are observed East and West of the island of Crete. The mean SWH around Cyprus reaches up to 0.8 meters at the West side of the island. More specifically, the highest values are observed at the coastline between Akamas peninsula and Akrotiri area. At Pomos region, north-west of the island, the mean SWH values are near 0.6–0.7 meters. At the rest of the coastline the mean SWH values drop significantly near 0.3 meters. As shown in Figure 5, higher wave period (WP) values are in the South part of EM near the African coasts. At those regions the WP values are just below 5 seconds. The highest WP values around Cyprus are observed at the northwest, west and southwest coastlines of the island. The values at these locations are near 4.5 s. At the remaining coastline the WP values are falling to 3 seconds. The combination of WP and SWH analyses shows that the areas with higher wave energy potential are located in the west side of the island. The results for WP and SWH are similar to those reported in the E-WAVE project for the significant wave height and wave period over a decadal period 2001–2010 (Zodiatis et al., 2014).
Using the Copernicus MEDSEA reanalysis data (Fratianni et al., 2015), the mean thermal gradients for the Eastern Mediterranean during 2006-2015 between depths 1.5–7.9 m and 1.5–24.1 m are plotted in Figures 6 and 7, respectively. Clearly, the west side of Cyprus has greater vertical temperature differences and thus the greatest potential at the Cyprus area. It should be noted that marine thermal energy is already used for heating and cooling in a hotel located in Limassol, at the south-west coast of Cyprus.
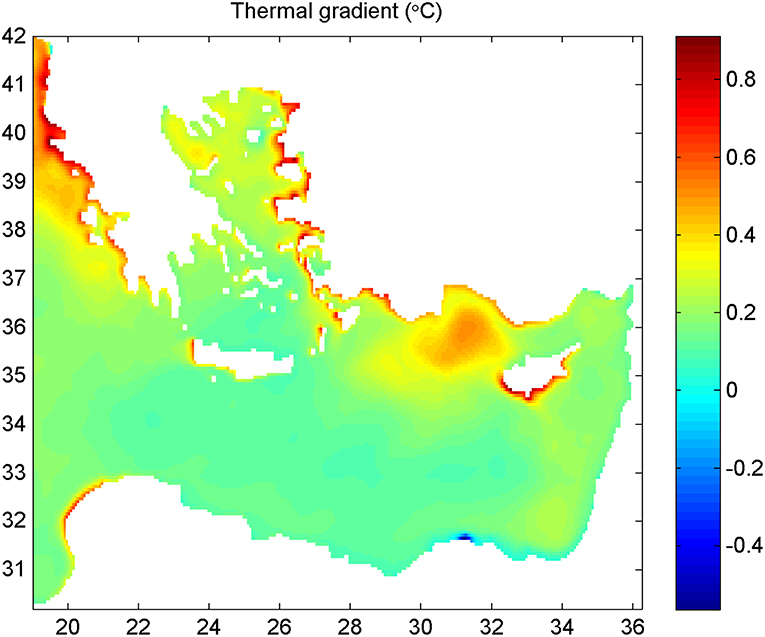
Figure 6. Mean thermal gradient between 1.5 m and 7. 9 m depth for the Eastern Mediterranean during 2006-2015.
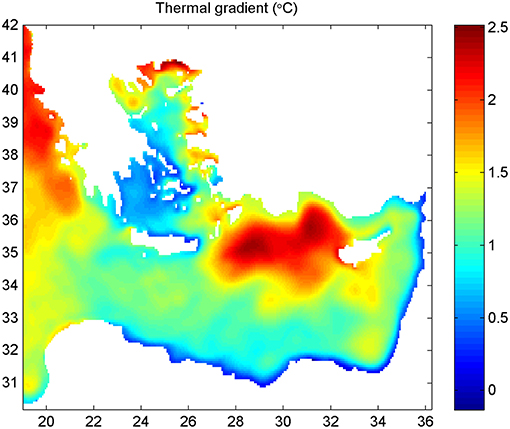
Figure 7. Mean thermal gradient between 1.5 m and 24.1 m depth for the Eastern Mediterranean during 2006-2015.
Using the same reanalysis dataset, the mean surface currents for the Eastern Mediterranean during 2006–2015 were plotted in Figure 8. In the region of EM the surface current speed is low. The peak values are near 0.4 m/s in EM. As a result, marine current energy potential, is considered as very low, since mean current velocities, around Cyprus, are near 0.1 m/s (Figure 8), while the threshold set by Soukissian et al. (2017) requires current speed from 1.5 to 2 m/s.
Finally, regarding marine biomass, in Med-algae, a recently completed research project, it has been shown that the use of micro-algae as a biofuel is a quite promising technology (Omirou et al., 2018). The results of the project indicated that marine biofuel production in the region is highly feasible using local marine algae blooms. The quantity and quality of biofuel produce depends on the aquatic environment and the species of micro algae used.
Geomorphology
A great problem in developing BE initiatives in the Mediterranean region is the deep and steep bathymetry, which characterizes the whole basin. This is visible in Figure 9, which shows that most of the seafloor around Cyprus is very deep. In addition to the deep bathymetry there are not many locations where the seafloor is flat (Figure 10). This fact further restricts the site selection for any offshore BE projects. A solution to the deep bathymetry of the region is the use of floating technologies, which can overcome the high costs of fixed foundation technologies.
Other Participating Countries
Two partners from Italy have submitted independent potential energy reports, the findings of which are merged here. The highlighted BE forms are offshore wind energy, wave energy and marine currents. For offshore wind energy, two high-potential locations were identified at Alghero and Oristano near Sardinia and in Messina Straits near Sicily with annual mean wind speeds of 4.9, 5.4, and 5.7 m/s, respectively (Soukissian et al., 2017). One important drawback in this case, is bathymetry, which can exceed a depth of 30 m in just a few hundred meters distance off the coast. However, this issue may be addressed with new emerging floating technologies. The mean wave energy identified for the region is 9.4 kW/m at the south-west coast of Sardinia and 4.75 kW/m near Sicily (Soukissian et al., 2017) and at the Tyrrhenian Sea (Luppa et al., 2015). In addition, marine current exploitation is feasible at very specific locations. In general, the marine current velocity is low, apart from Messina Straits where it ranges from 1.8 m/s to 3 m/s during spring tides (Soukissian et al., 2017).
The analysis of Istrian Regional Energy Agency (IRENA) for Croatia showed that the BE forms with the greatest potential are offshore wind, salinity gradient and thermal energy. The most promising areas for offshore wind energy are near Cres and Krk islands and near Senj. According to the feasibility scenarios of Hundleby and Freeman (2017), under certain assumptions, an offshore wind energy park is feasible if the mean wind speeds are 7.5–8 m/s. These scenarios and assumptions lower the feasibility potential of offshore wind energy in Croatia. It has been reported that salinity-gradient energy exploitation is favored by the high vertical differences in salinity observed due to river runoffs in North Adriatic Sea (Russo and Artegiani, 1996). The main drawback for this BE form is the technology, which is still developing and has not reached a commercialization level yet. The BE form with the most highlighted potential is thermal energy. This BE form is used directly for heating and cooling using marine heating pumps. Heat is extracted for heating the buildings and is stored during the cooling phase of buildings. The temperature differences between air temperature and seawater make this BE form the most promising and viable in the region.
In Greece, the BE potential analysis carried out by Aristotle University of Thessaloniki (AUTH) concluded that the most promising BE forms are offshore marine currents, wave energy, wind energy, and marine biomass. Marine current energy is generally low in the MED region. This potential is remarkably high and can be exploited only in certain areas. Such areas in Greece, are located near Evoia, Kea, Samos, Kithnos, and Mytilene (ORECCA Project, 2011), where the minimum spring tide marine current is near 1.75 m/s, a magnitude that allows the exploitation of marine current energy. Wave energy potential ranges between 5 and 10 kW/m near Skyros, Andros and Tinos in the central Aegean Sea, near Karpathos and western Crete, where wave energy harvesting may be feasible. Regarding offshore wind energy, the areas with the highest potential are found in the Aegean Sea at Steno Kafirea, with a mean annual wind speed of 7.5 m/s and with available wind potential energy of 546 W/m2. Another favorable location is Kasos, in the Karpathian Sea, with a mean annual wind speed of 8 m/s and with available wind potential energy of 570 W/m2. Finally, the potential of marine biomass is highlighted, but for its exploitation, further research advances and better understanding of its commercialization impacts are still required.
Two separate potential analyses have been carried out by the two partners (Cluster Maritimo-Marino de Andalucia and Business Innovation Centre of Valencia) in Spain. Offshore wind energy and wave energy are highlighted as the most promising BE forms. The two analyses suggest that the most promising BE form is offshore wind energy. It was also pointed out that the only viable solution for commercialization of offshore wind energy is floating wind turbines due to the deep bathymetry of the MED region. The W2Power floating wind turbine was proposed as a possible solution, since it can operate well at the wind speed ranges encountered in the MED region and has been tested extensively. For wave energy, a hybrid solution of wave extraction technology is suggested, in order to increase the feasibility of wave energy extraction projects due to relatively low wave energy potential at the region. Such technology is the Butterfly converter from Rotary Wave. Finally, tidal energy is also highlighted for the area near the Straits of Gibraltar, where current velocities reach up to 2 m/s. The issue with this BE form is that existing technologies cannot harvest energy at these current speeds and new technologies are not yet mature enough.
The coastline of Slovenia is only 46 km, which limits the possible site allocation of any potential offshore renewable technology. Nevertheless, according to the report of Goriška Local Energy Agency (GOLEA), the most important BE forms are offshore wind and marine thermal energy. The offshore wind energy does not allow very high expectations, since mean annual wind speeds can reach up to 5 m/s. An important feature is micro-siting which can favor higher wind speed at specific locations. The most promising BE form, however, is marine thermal energy. This energy form is not used to produce electricity but is rather used for energy efficiency. In fact, the existing capacity of the region is 1.4 MW with an annual heat extraction of 2,300 MWh. The mean annual sea temperature is 17.6°C ranging from 9 degrees in February to 26 degrees Celsius in July and August. These temperatures are much lower or higher compared to the air temperature, hence they are suitable for heating or cooling.
Lastly, according to the BE potential analysis report of Malta Intelligent Energy Management Agency (MIEMA), the most promising BE forms for Malta are wave energy, offshore wind energy and marine thermal energy. According to the Italian National Agency for New Technologies, Energy and Sustainable Economic Development (ENEA) the wave potential of the region reaches up to 7 kW/m at 25 km off the coast of Malta and becomes lower closer to the coast. In addition, offshore wind speed reaches 6 to 7 m/s in areas located 25 to 50 meters from the coastline (ORECCA Project, 2011). Once again, floating-turbine technologies are mentioned since the steep bathymetry of the region does not allow the use of fixed-foundation wind turbines. Moreover, like Croatia and Slovenia, the marine thermal energy is proposed for use as energy storage and source when needed. This can be a feasible scenario for energy savings due to the sea/air temperature differences both in the summer and in the winter.
The detailed results of the Blue Energy Potential Analysis for all Mediterranean countries participating in the MAESTRALE project can be found on the MAESTRALE website (https://maestrale.interreg-med.eu/). In addition, a webgis portal (http://maestrale-webgis.unisi.it) has been created, which contains an updated database of Blue Energy potential, best practices projects, stakeholders, as well as physical and environmental constraints in the Mediterranean region.
Discussion
As illustrated in Figure 2, all BE forms were highlighted as promising throughout the MED region. However, the most highlighted BE form in the study area is offshore wind energy, which has been selected by all 8 countries and by 9 out of 10 partners. It is worth noting that in some regions the potential of this BE is characterized as low, since the wind speed is not as high as in other regions outside the MED region. This impacts the feasibility of possible investments in such regions.
The second BE form which was highlighted by most partners is the wave energy. Wave energy has not been considered by countries in the Adriatic Sea. To increase exploitability of wave energy potential, partners made several suggestions. One suggestion is the use of hybrid technologies, which combine wave-energy extraction with photovoltaics technologies. Another suggestion is to use wave energy extraction technologies along with other constructions such as ports or wave breakers to have dual impact.
Marine thermal gradient is highlighted in all central Mediterranean countries and in Cyprus, but not for producing electricity. This BE form is mainly used for heating (winter) and cooling (summer) systems for the benefit of the local businesses and communities. These systems may not produce electricity, but they result in electricity consumption. Partners who highlighted this BE form indicated that it has the greatest feasibility compared to other BE forms. The fact that this BE form has been already tested and established in commercial projects increases its perspectives compared to other BE forms in the MED region.
Less highlighted BE forms are the tidal, the salinity gradient, and marine biofuel energy. Nevertheless, the expectations for tidal-current and salinity gradient energy in the MED region were not high. This is due to the fact that certain physical conditions must be met for those BE forms to have a high potential. On the one hand, the salinity gradient energy potential can be high, only if there is fresh water input on the top layer and high salinity water at the water layer below. The major drawback of salinity gradient energy is that the extraction technologies are still in an experimental phase. On the other hand, tidal-current energy potential is in general low at the MED region with the exception of some isolated regions, identified in Greece (Kea, Kithnos, Mytiline, Evoia) and Italy (Straits of Messina). The least highlighted BE form is marine biomass which has been considered only by Greece and Cyprus.
Other factors that may affect the BE potential in the region have been identified by the partners. A factor, indicated by many partners, is the steep bathymetry, which characterizes most of the MED region and constitutes a major economical barrier. To overlap this barrier, most of the partners recommend floating BE technologies rather than fixed-foundation ones. Floating structures also give the flexibility of avoiding some high interest areas and at the same time remaining at areas of high energy potential.
Additional factors that impact BE projects' development, include bureaucracy of getting the required licenses, lack or insufficient national legislation for offshore renewables-constructions, and public and local acceptance. Social acceptance is often a prerequisite in implementing offshore projects. In Italy for example, the lack of social acceptance for offshore constructions can result in project rejections or costly delays (Pisacane et al., 2018). Another study in Italy revealed that the local society was in favor of onshore renewables, such as photovoltaics and wind farms, but against offshore renewables (Goffetti et al., 2018). In Cyprus, social acceptance for implementing the Orites onshore wind Farm was encouraging but it may not be the same when it comes to offshore constructions near touristic infrastructure (Fokaides et al., 2014). The visual impact of offshore constructions and the lack of Marine Spatial Planning (MSP) is causing conflicts between different stakeholders, which can impact the social acceptance of the project (Soma and Haggett, 2015; Soukissian et al., 2017). However, certain case studies in the UK showed that social acceptance for offshore projects is greater when there is an intensive and early engagement of the public to shape those projects (Soma and Haggett, 2015).
A key component for the introduction of early-stage marine technologies is the rigorous assessment of their environmental effects and impacts. Such assessments must comply with the EU legal obligations and environmental and marine law (Martínez Perez, 2017). Using legislation tools such as the Environmental Impact Assessment and Marine Spatial Planning (MSP), the EU has guided member states toward the introduction of legislation regarding the Blue Energy sector (Martínez Perez, 2017). The Mediterranean countries that participate in the MAESTRALE project have reported that they have incorporated the EU directives referring to energy and renewable energy sources into national legislations. But since the Blue Energy sector in the Mediterranean region is new, there are still many gaps in the existing legislation. The effects of the BE installations have admittedly not been thoroughly identified by the European Commission, and therefore the regulatory framework that should accordingly be followed is still inadequate (Martínez Perez, 2017). This constitutes another constraint to the implementation of BE projects.
Some countries, such as Croatia, Spain and Slovenia, have specific laws that regulate renewable energy projects in the marine environment (MAESTRALE Project, 2018). Other countries have issues on permitting procedures and regulations regarding coastal zones and marine spatial planning (MAESTRALE Project, 2018). In Greece, the procedures are very complicated and time consuming due to the large number of public services having jurisdiction in the sea (MAESTRALE Project, 2018). Goffetti et al. (2018) have characterized the current permitting procedures and existing gaps in the Italian law as a weakness and threat. In addition, according to European Marine Spatial Planning (https://www.msp-platform.eu/), none of the Mediterranean countries that are involved in the MAESTRALE project have a legally binding MSP. Cyprus has only recently established an MSP for the district of Limassol. The deadline for establishing MSP has been set for 2021 (European Commission, 2015). A recently drafted new law along with its regulations aiming at improving the permitting procedures have been submitted to the parliament for approval.
Conclusions
Offshore wind energy is the most promising BE source in Cyprus and in the entire Mediterranean region. The proven economic viability in other parts of the Mediterranean Sea along with the fast-technological progression in this sector, the current wind energy market and the stable annual energy availability, make it the most promising candidate BE source for Cyprus. In contrast, wave and current/tidal energy are not firmly commercially established and depend on the prevailing atmospheric conditions. Moreover, they have limited potential to be considered for exploitation, at least with currently available technology.
Most BE forms can be applied throughout the MED region. The differences between countries are expected, given the different locations and geomorphological characteristics. It is evident that BE can be used in the MED region to produce electricity power or to reduce electricity consumption. A challenge in implementing BE projects is the steep and deep bathymetry in the MED region, which, however, may be tackled with floating technologies. In addition to the energy potential and bathymetry, any future BE endeavor in the MED region must also consider socio-economic factors, such as national legislation and impact on the local society, which may affect the feasibility of the project.
Overall, the Mediterranean region should play a prominent role with a clear long-term commitment to blue renewable energy. Although the task remains challenging today, it is the only true sustainable alternative to the current European energy system, in environmental, social and economic terms, as well as targets beyond 2020.
Author Contributions
GN, AK, AN, and MN worked on the Blue Energy Potential Analysis and contributed to the literature review and the discussion of the results. AM obtained the results for the Cyprus region and contributed to the literature review and the discussion of the results. GG supervised the Blue Energy Potential Analysis and the results around Cyprus and contributed to the literature review and the discussion of the results.
Funding
This work was carried out in the framework of the Maestrale project, funded by the Interreg MED 2014-2020 Programme, and co-financed by the European Regional Development Fund.
Conflict of Interest Statement
The authors declare that the research was conducted in the absence of any commercial or financial relationships that could be construed as a potential conflict of interest.
References
Cyprus Country Report (2018). Available online at: https://www.cyprusprofile.com/en/sectors/energy-and-environment/
Ellabban, O., Abu-Rub, H., and Blaabjerg, F. (2014). Renewable energy resources: current status, future prospects and their enabling technology. Renew. Sustain. Energy Rev. 39, 748–764. doi: 10.1016/J.RSER.2014.07.113
European Commission (2015). Energy Sectors and the Implementation of the Maritime Spatial Planning Directive. doi: 10.2771/32703
European Commission (2018). Communication From the Commission to the European Parliament, the European Council, the Council, the European Economic and Social Committee, The Committee of the Regions and the European Investment Bank. European Commission. Available online at: https://ec.europa.eu/clima/sites/clima/files/docs/pages/com_2018_733_en.pdf (accessed May 10, 2019).
Fokaides, P., Miltiadous, I. C., Neophytou, M., and Spyridou, L. P. (2014). Promotion of wind energy in isolated energy systems: the case of the orites wind farm. Clean Technol. Environ. Policy 16, 477–488. doi: 10.1007/s10098-013-0642-2
Fratianni, C., Simoncelli, S., Pinardi, N., Cherchi, A., Grandi, A., and Dobricic, S. (2015). Mediterranean RR 1955-2015 (Version 1), E.U. Copernicus Marine Service Information.
Goffetti, G., Montini, M., Volpe, F., Gigliotti, M., Pulselli, F. M., Sannino, G., et al. (2018). Disaggregating the SWOT analysis of marine renewable energies. Front. Energy Res. 6, 1–11. doi: 10.3389/fenrg.2018.00138
Hundleby, G., and Freeman, K. (2017). Unleashing Europe's Offshore Wind Potential: A New Resource Assessment. Brussels: WindEurope. Available online at: https://windeurope.org/wp-content/uploads/files/about-wind/reports/Unleashing-Europes-offshore-wind-potential.pdf (accessed May 10, 2019).
IEA (2015). Energy and Climate Change - World Energy Outlook Special Report. Paris: International Energy Agency. Available online at: https://www.iea.org/publications/freepublications/publication/WEO2015SpecialReportonEnergyandClimateChange.pdf (accessed May 10, 2019).
Kallos, G., Nickovic, S., Papadopoulos, A., Jovic, D., Kakaliagou, O., Misirlis, N., et al. (1997). “The regional weather forecasting system Skiron: an overview,” in Proceedings of the Symposium on Regional Weather Prediction on Parallel Computer Environments (Athens), 109-122.
Kuleszo, J., Kroeze, C., Post, J., and Fekete, B. M. (2010). The potential of blue energy for reducing emissions of CO2 and Non-CO2 greenhouse gases. J. Integ. Environ. Sci. 7, 89–96. doi: 10.1080/19438151003680850
Lillebø, A. I., Pita, C., Rodrigues, J. G., Ramos, S., and Villasante, S. (2017). How can marine ecosystem services support the blue growth agenda? Mar. Policy 81, 132–142. doi: 10.1016/J.MARPOL.2017.03.008
Luppa, C., Contestabile, P., Cavallaro, L., Foti, E., Andersen, T. L., and Vicinanza, D. (2015). “Experimental investigation of rubble mound breakwaters for wave energy conversion,” in Proceedings of the 11th European Wave and Tidal Energy Conference (Nantes), ID 2298629666.
MAESTRALE Project (2018). BE Potential in the MED Area Report. Available online at: https://maestrale.interreg-med.eu/fileadmin/user_upload/Sites/Blue_Growth/Projects/MAESTRALE/D3.3.2_BE_Potental_analysis_MED_04_2018.pdf (accessed May 10, 2019).
Magagna, D., and Uihlein, A. (2015). Ocean energy development in Europe: current status and future perspectives. Int. J. Mar. Energy 11, 84–104. doi: 10.1016/j.ijome.2015.05.001
Martínez Perez, E. J. (2017). “The environmental legal framework for the development of Blue Energy in Europe,” in The Future of the Law of the Sea, ed G. Andreone (Cham: Springer International Publishing AG).
Omirou, M., Tzovenis, I., Charalampous, P., Tsaousis, P., Polycarpou, P., Chantzistrountsiou, X., et al. (2018). Development of marine multi-algae cultures for biodiesel production. Algal. Res. 33, 462–469. doi: 10.1016/j.algal.2018.06.025
Partasides, G., Mesimeris, T., Kythreotou, N., Piripitsi, K., Karapitta-Zachariadou, N., Hadjinikolaou, C., et al. (2019). Draft Integrated National Energy and Climate Plan for the Period 2021-2030. Available online at: https://ec.europa.eu/energy/sites/ener/files/documents/cyprus_draftnecp.pdf (accessed May 10, 2019).
Pelc, R., and Fujita, R. M. (2002). Renewable energy from the ocean. Mar. Policy 26, 471–479. doi: 10.1016/S0308-597X(02)00045-3
Pisacane, G., Sannino, G., Carillo, A., Struglia, M. V., and Bastianoni, S. (2018). Marine energy exploitation in the Mediterranean region: steps forward and challenges. Front. Energy Res. 6, 1–17. doi: 10.3389/fenrg.2018.00109
Ross, R., and Krijgsman, J. (2004). “New ionomer membranes for blue energy - making water trees useful,” in Proceedings of the 2004 IEEE International Conference on Solid Dielectrics, 2004 (ICSD 2004), Vol. 2, 780–783.
Soma, K., and Haggett, C. (2015). Enhancing social acceptance in marine governance in Europe. Ocean Coast. Manag. 117, 61–69. doi: 10.1016/J.OCECOAMAN.2015.11.001
Soukissian, T. H., Denaxa, D., Karathanasi, F., Prospathopoulos, A., Sarantakos, K., Iona, A., et al. (2017). Marine renewable energy in the Mediterranean Sea: status and perspectives. Energies 10:1512. doi: 10.3390/en10101512
Zervos, A., Lins, C., Tesnière, L., and Smith, E. (2011). EU Roadmap, Mapping Renewable Energy Pathways towards 2020. Belgium: European Renewable Energy Council, Brussels.
Keywords: renewable energy, offshore marine renewables, ocean energy, blue energy, Maestrale project
Citation: Nikolaidis G, Karaolia A, Matsikaris A, Nikolaidis A, Nicolaides M and Georgiou GC (2019) Blue Energy Potential Analysis in the Mediterranean. Front. Energy Res. 7:62. doi: 10.3389/fenrg.2019.00062
Received: 30 July 2018; Accepted: 07 June 2019;
Published: 25 June 2019.
Edited by:
Uwe Schröder, Technische Universitat Braunschweig, GermanyReviewed by:
Konstantina Vasiliki Iakovou, Aristotle University of Thessaloniki, GreeceSimone Bastianoni, University of Siena, Italy
Copyright © 2019 Nikolaidis, Karaolia, Matsikaris, Nikolaidis, Nicolaides and Georgiou. This is an open-access article distributed under the terms of the Creative Commons Attribution License (CC BY). The use, distribution or reproduction in other forums is permitted, provided the original author(s) and the copyright owner(s) are credited and that the original publication in this journal is cited, in accordance with accepted academic practice. No use, distribution or reproduction is permitted which does not comply with these terms.
*Correspondence: Georgios C. Georgiou, Z2Vvcmdpb3NAdWN5LmFjLmN5