- Chongqing Key Laboratory of Catalysis and New Environmental Materials, College of Environment and Resources, Chongqing Technology and Business University, Chongqing, China
Lithium-sulfur (Li-S) and lithium-selenium (Li-Se) batteries are both facing the cathode issues of low Coulombic efficiency and unstable cycling stability due to the severe shuttle effect of lithium polysulfides or lithium polyselenides. Simultaneously inhibiting polysulfides/polyselenides dissolution in organic electrolytes and propelling them to conversion via introducing polar, catalytic materials has been proven as an effective strategy to enhance the durability of Li-S and Li-Se batteries. In this mini review, we systematically introduce various metal atom-decorated carbon nanomaterials to determine how to enhance the electrochemical performances of Li-S and Li-Se batteries by inhibiting the polysulfides/polyselenides shuttle phenomenon as well as catalyzing them toward quick redox conversions. We also briefly include the drawbacks and bottlenecks of this kind of material when used in Li-S and Li-Se batteries
Introduction
The expanding electric vehicle market and the popularization of smart grids has triggered the urgent demand for energy-storage devices with long-cycle life and high-energy density (Ma et al., 2020b; Sheng et al., 2020). Li-S and Li-Se batteries have been commonly regarded as appealing choices for high-energy storage technology as they demonstrate high theoretical energy density (2,600 Wh kg−1 and 2,800 Wh L−1 for Li-S battery; 1,160 Wh kg−1 and 2,600 Wh L−1 for Li-Se battery) as well as acceptable low costs (Gu and Lai, 2019; Jin et al., 2020).
However, there are still many technical challenges, from the electrolyte to the anode as well as the cathode, for Li-S/Se batteries that need to be tackled (Chen et al., 2019a; Yan et al., 2019; Liu et al., 2020b). In terms of the cathode, Nazar’s group first employed the CMK-3 as the sulfur host to effectively inhibit polysulfide shuttling (Ji et al., 2009), since then scientists have spent a significant portion of time and energy on how to inhibit the serious shuttle phenomenon of Li polysulfide (LiPS) and Li polyselenide (LiPSe) intermediates during the charge/discharge process. From the very beginning the physical adsorption of porous carbon (Gu et al., 2015b), to the chemical adsorption of heteroatoms doped in a carbon framework (Gu et al., 2015a; Gu et al., 2016b; Gu et al., 2018; Gu et al., 2020b), until now the stronger chemical adsorption by employing various carbon/metal compounds (Gu et al., 2016a; Gu and Lai, 2017; Gu et al., 2020a), the LiPS and LiPSe shuttle phenomenon has been significantly alleviated. Without doubt, a carbon host was the most popular choice to address the problems of Li-S and Li-Se cells during the past decades as it had high conductivity and good adsorption force with LiPS and LiPSe (Zeng et al., 2017; Zheng et al., 2019; Li et al., 2020b; Han et al., 2020).
However, recent research found that if the sluggish redox kinetics, with increased internal resistance cause low S/Se utilization and poor Coulombic efficiency (CE) did not address (Gu and Lai, 2019; He and Manthiram, 2019; Lim et al., 2019; Song et al., 2019; Ruan et al., 2020) the cathode problems of Li-S/Se batteries, these problems would be impossible to solve completely even if the immediate shuttle effect was effectively restrained using various carbon-based hosts. Therefore, researchers around the world are gradually focusing on how to improve redox kinetics and limit the shuttle effects of LiPS/LiPSe simultaneously (Zhang et al., 2019f; Hong et al., 2020).
Interestingly, nano-sized catalysts including single-atom catalysts recently demonstrated excellent catalytic properties compared to conventional catalysts (Liu, 2016; Cao et al., 2018; Cui et al., 2018; O’Connor et al., 2018; Zhang et al., 2019b). However, scientists also found that single-atom catalysts were not easily prepared. An effective strategy is to deposit the ultra-small metal nanoparticles on the carbon surface or dope the metal atoms in the carbon framework to produce a so-called single-atom-like catalyst (Gawande et al., 2020; Ren et al., 2020). As expected, the single metal atom-decorated (deposited/doped) carbon catalysts illustrated excellent catalytic performances on oxygen reduction reaction (Wang et al., 2020b; Ren et al., 2020), oxygen evolution reaction (Hou et al., 2019; Wang et al., 2020b), hydrogen evolution reaction (Zhang et al., 2019d; Ren et al., 2020), CO2 reduction reaction (Wang et al., 2019a; Lu et al., 2019; Yang et al., 2019), and nitrogen electroreduction (Chen et al., 2018b), etc.
As the metal atom-decorated carbon materials not only have an excellent catalytic property that could effectively catalyze the polysulfides conversion during the charge/discharge process, but also provide strong chemical adsorption on polysulfides due to the polar metal atoms/heteroatoms. Therefore, an increasing number of investigations reported the simultaneous use of metal atom-decorated carbon materials as catalysts and shuttle inhibitors for LiPS and LiPSe. And this mini-review has summarized how the performances of Li-S and Li-Se batteries could be improved by these various kinds of metal atom-decorated carbon nanomaterials.
Metal Atom-Decorated Carbon Nanomaterials for Enhancing Li-S Batteries Performances
Reducing particles size to nanometers or even to an atomic scale has emerged as a promising route to extend the reactivity of materials. In this part, nano-metal atoms including single metal atom-decorated carbon materials for enhancing Li-S batteries will be introduced in detail.
Noble Metal Atom-Decorated Carbon Materials
The noble metals, such as Au (Babu et al., 2015; Zhang et al., 2020), Pt (Al Salem et al., 2015; Qu et al., 2018; Liu et al., 2019), and Pd (Ma et al., 2019), etc., when combined with the conductive carbon substrates, commonly demonstrate superior catalytic properties on LiPS redox reactions.
In 2015, Arava’s group first proposed an electrocatalysis concept in non-aqueous polysulfides redox reactions (Babu et al., 2015). They found that coating Pt or Ni on Al foil as the electrocatalytic current collectors could enhance both cycle life and reaction kinetics of the Li-S battery. Before long they reported that dispersed Pt nanoparticles on graphene layers could enhance the specific capacity by 40% over pristine graphene due to the excellent catalytic property of Pt nanoparticles, and such a Pt/graphene can also contribute to sulfur cathode stability cycling over 100 cycles with a Coulombic efficiency of 99.3% at a current rate of 0.2 C (Al Salem et al., 2015). Interestingly, in this work, Arava et al. also found that the Pt/graphene host could improve more performances for Li-S batteries compared to the Ni/graphene as shown in Figures 1B,C. Zhang et al. reported a nanoscale polysulfide reactor achieved by a chemical Au−S interaction as shown in Figure 1D (Fan et al., 2015). The Au NPs with high conductivity can significantly control the deposition of the trapped LiPSs, contributing to the uniform distribution of sulfur species upon charging/discharging. Recently, Liang et al. reported a yolk–shell Au@microporous carbon nanosphere with the synergistic advantages of a hollow nanosphere and functional Au nanoparticles (Zhang et al., 2020), which also contributed to a high specific capacity, and good electrochemical activities and reaction kinetics of Li-S batteries. While Zuo’s group skillfully imbedded palladium nanoparticles in hollow carbon spheres as the sulfur host. The Pd nanoparticles acted not only as electrocatalysts to accelerate the redox reaction kinetics of LiPS but also chemically trapped LiPS via the moderate Pd-S bonding.
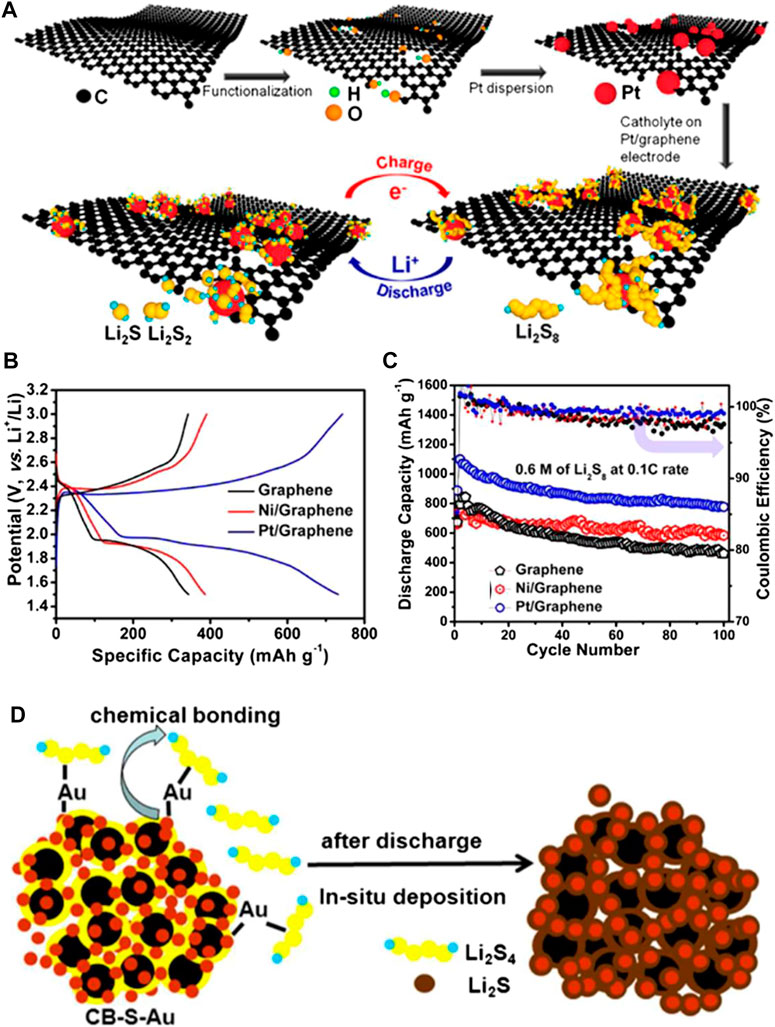
FIGURE 1. (A) Schematic illustration of electrocatalyst-anchored graphene nanocomposite preparation and its interaction with PS during the charge/discharge process of the Li−S battery (B) voltage vs specific capacity profile and (C) galvanostatic charge/discharge behavior and Coulombic efficiency of pristine and electrocatalyst-anchored graphene electrodes vs Li+/Li at 0.1 C rate in the potential range of 1.5–3.0 V. Reproduced with permission from Al Salem et al. (2015). Copyright © 2015 American Chemical Society (D) The function mechanism of the CB-S-Au cathode. Reproduced with permission from Fan et al. (2015). Copyright © 2015 American Chemical Society.
The present investigations have proven the efficient catalytic function of LiPS by introducing noble metal atoms into the carbon, however, as shown in Table 1, the enhanced performances of Li-S batteries are not ideal, particularly on long cycling performances with higher sulfur loading.
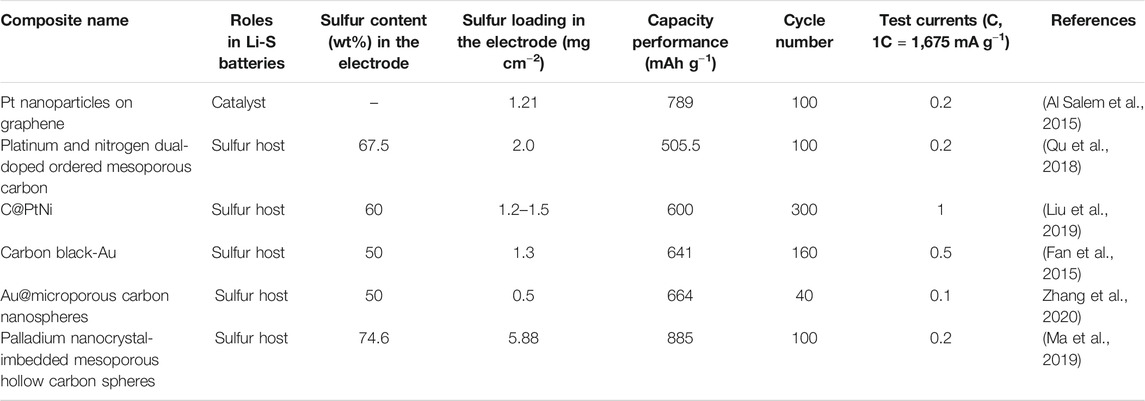
TABLE 1. Various noble metal atom-decorated carbon materials for enhancing the performances of Li-S batteries.
Iron Series Metal Atom (Fe, Co, Ni)-Decorated Carbon Materials
Due to the high prices of noble metals, in recent decades, scientists have been devoted to developing noble-free catalysts, in which the iron series metal-carbon materials are regarded as one of the most promising catalysts. And there are large numbers of literature on this kind of catalyst application in different fields. Taking into account the good conductivity, good physical/chemical adsorption ability, and redox of LiPS, iron series metal atom-decorated carbon materials have begun to pique the interest of Li-S batteries researchers in the last three years (Li et al., 2016; Li et al., 2019f; Zhang et al., 2019g; Jin et al., 2019).
Cobalt-nitrogen doped carbon materials as the sulfur/Li2S host are most frequently reported (He et al., 2016; Zhong et al., 2018; Hu et al., 2019; Li et al., 2019a; Li et al., 2019d; Wu et al., 2019; Yu et al., 2019; Liu et al., 2020a; Shao et al., 2020; Wang et al., 2020a; Wang et al., 2020c; Wang et al., 2020e; Yao et al., 2020). In 2017, Dong et al. reported a honeycomb-like Co@N−C composite that served as the sulfur host as shown in Figure 2A (Li et al., 2017). The cellular flake with a large surface area and honeycomb architecture could encapsulate much more sulfur, leading to high sulfur content (93.6 wt% and 7.5 mg cm−2 in an electrode) and the Co-N-C coordination center served as a bifunctional electrocatalyst to facilitate both the formation and the decomposition of Li2S in the discharge and charge process as shown in Figure 2B. The S/cellular Co−N−C composites exhibited excellent rate performance up to 10 C (3.6 mg cm−2) and great cycling stability as shown in Figure 2C. Huang’s group implanted atomic cobalt within the skeleton of mesoporous carbon via a supramolecular self-templating strategy (Xie et al., 2019). The atomic cobalt sites with high polarity exhibited strong interactions with polysulfides and consequently enhanced the kinetics of the sulfur redox reactions (Xie et al., 2019). More importantly, they systematically evaluated the sulfur redox reaction via CV, EIS, Tafel slope, and a potentiostatic nucleation test, which provided a general evaluation criterion of the metal atom-decorated carbon materials for catalyzing the sulfur redox reaction. While Wu’s group and Shen’s group focused on the function of the Co-N-C bond in Li-S batteries (Du et al., 2019; Xiao et al., 2019). In Wu’s work, they employed a combination of operando X-ray absorption spectroscopy and first-principles calculations to reveal that the Co−N−C coordination center served as a bifunctional electrocatalyst to facilitate both the formation and the decomposition of Li2S in the discharge and charge processes, respectively (Du et al., 2019). The operando XANES experiment (Figures 2D,E) revealed the formation of Li2S at the initial stage of discharge. This early formation of Li2S, together with the relative electrochemical characterizations, demonstrated the improved electrochemical kinetics during the phase change between the soluble LiPSs and insoluble Li2S2/Li2S on the Co-N/G support. And the DFT calculation in Figures 2F–H show that the formation of Li2S from Li2S2 was the rate-limiting step in the whole discharge process as this step had the largest positive Gibbs free energy. The lower Gibbs free energy on Co−N/G (0.71 eV vs. 1.21 eV) for the reduction of Li2S2, indicated that the reduction of S was thermodynamically more favorable on Co−N/G than on the N/G substrate. In Shen’s report (Xiao et al., 2019), they found during the annealing process that the cobalt atoms will coordinate with N atoms to form Co4N. Because the electron transferred from Co to the doped N in the carbon matrix, this caused a larger polarization of Co in Co4N. This synergistic effect between Co and doped N can contribute to increased binding energy between Co4N and polysulfides (Xiao et al., 2019). Moreover, using the Co-decorated carbon materials as an interlayer or to modify the separator has also been widely reported (Chen et al., 2018a; Zhang et al., 2019c; Li et al., 2020d; Jiang et al., 2020; Song et al., 2020).
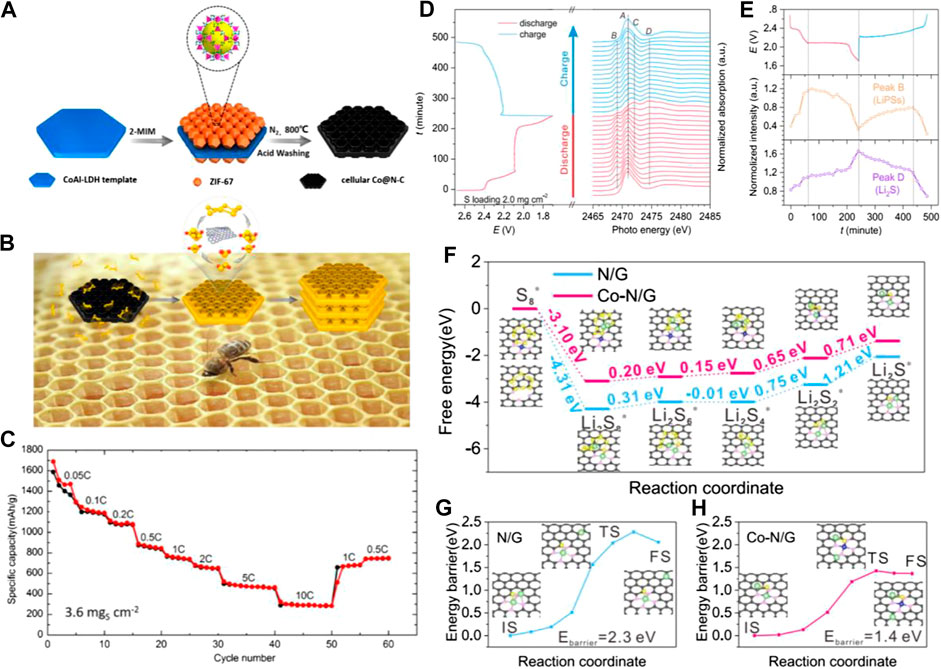
FIGURE 2. (A) Schematic illustration of the step-by-step synthesis strategy for the cellular Co@N−C composite (B) the schematic illustration of the cellular Co@N−C composite which catalyzed the formation and the decomposition of Li2S in the discharge and charge process (C) Rate performances of the 90 S/cellular Co@N−C at different current rates. Reproduced with permission from Li et al. (2017). Copyright © 2017 American Chemical Society (D) Evolution of S K-edge XANS during electrochemical cycling (E) evolution of the intensities of peak B (2,469.0 eV, representing concentration of LiPSs) and peak D (2,474.7 eV, representing Li2S concentration) during electrochemical cycling (F) Energy profiles for the reduction of LiPSs on N/G and Co-N/G substrates, energy profiles of the decomposition of the Li2S cluster on N/G (G) and Co-N/G (H). The black, yellow, green, pink, and dark blue balls represent C, S, Li, N, and Co atoms, respectively. Reproduced with permission from Du et al. (2019). Copyright © 2019 American Chemical Society.
Compared to the cobalt-decorated carbon materials, there have been few reported iron and nickel-decorated carbon materials (Yao et al., 2018; Jiang et al., 2019; Li et al., 2019e; Ye et al., 2019; Zeng et al., 2019). Niu et al. prepared a kind of Ni–N4 structure via doping single nickel atoms on nitrogen-doped graphene (Ni@NG) and then employed them to modify the separators of Li–S batteries (Zhang et al., 2019e). The oxidized Ni sites of the Ni–N4 structure acted as LiPS traps, efficiently accommodating polysulfide ion electrons by forming strong Sx2−⋅⋅⋅Ni–N bonding as well as catalyzing the LiPS redox conversion as shown in Figure 3A. As a result, the Li–S battery based on this Ni@NG modified separator illustrated excellent rate performance and stable cycling life with only 0.06% capacity. Hou et al. created a holey Fe, N co-doped graphene (HFeNG) to promote the cycle stability and rate capacity of Li–S batteries (Wang et al., 2019b). Via the X-ray absorption spectroscopy and density functional theory calculations, they first confirmed that the Fe atoms were anchored by 4 N atoms (Fe–N4 moiety) or 2 N atoms (Fe–N2 moiety) localized on the graphene sheets and edge of holes, respectively. The Fe–N2 moiety at the edges could provide stronger adsorption forces on LiPS (Figures 3B,C) and the holey structure could promote the mass transportation of Li+ as well as prohibit the transportation of LiPS (Figure 3D). Accordingly, the as-obtained S/HFeNG delivered a high rate capacity of 810 mAh g−1 at 5 C and a stable cycling performance with a capacity decay of 0.083% per cycle.
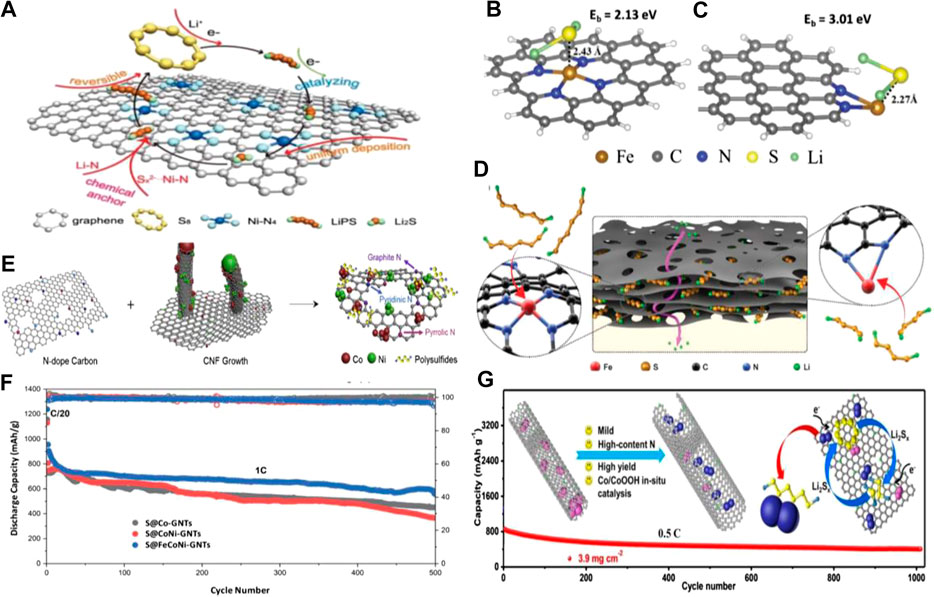
FIGURE 3. (A) The catalytic and chemically trapping mechanism of the LiPS on the surface of Ni@NG in the electrochemical process. Reproduced with permission from Zhang et al. (2019e). Copyright © 2019 WILEY-VCH Verlag GmbH and Co. KGaA, Weinheim. Optimized configurations for the binding of Li2S to (B) Fe–N4 and (C) Fe–N2 moieties on graphene (Li2S binding energies and selected bond distances are indicated in images) (D) Schematic illustration of the confinement of sulfur and polysulfides in the layer structure and the additional ionic diffusion pathways (purple arrow line) through the holey structure (the black sheets). Reproduced with permission from Wang et al. (2019b). Copyright © 2018 WILEY-VCH Verlag GmbH and Co. KGaA, Weinheim (E) Designing strategy of NiCo-CNF@CF interlayer for Li-S batteries. Reproduced with permission from Zhang et al. (2019a) Copyright © 2019 Elsevier Ltd (F) Capacity retention between S@Co-GNTs, S@CoNi-GNTs, and S@FeCoNi-GNTs at 1 C. Reproduced with permission from Ogoke et al. (2019). Copyright © 2019 The Royal Society of Chemistry (G) The schematic of 1D high-content N-doped graphene nanoribbons@Co/CoOOH high-yield and in-situ fabricated as an integrated host for Li-S batteries and the long cycling performance. Reproduced with permission from Tan et al. (2020) Copyright © 2020 The Royal Society of Chemistry.
Except for one kind of metal atom-decorated carbon material, binary or ternary metal atom-decorated carbon materials have also been reported for enhancing the performances of Li-S batteries (Chen et al., 2019b; Jing et al., 2019; Li et al., 2019c; Ogoke et al., 2019; Zhang et al., 2019a). For instance, Chen’s group reported a CoNi-carbon nanofiber@carbon fabric heterostructure (CoNi-CNF-CF, Figure 3E) as the interlayer for Li-S batteries. The weaving carbonaceous scaffold with vertically rooted CNF tentacles facilitated both short- and long-range electrical conduction as well as the efficient exposure of active sites, while the multiple adsorptive and catalytic sites enabled strong sulfur confinement and expedited sulfur conversion. And in this work, they confirmed that the CoNi-CNF@CF showed a stronger current response with a higher deposition capacity as well as a smaller onset potential at around −0.45 V and larger oxidation current compared to Co-CNF@CF. However, why CoNi-CNF@CF could exhibit such superior performances was not clear. While Wu and his co-workers reported a ternary metal atom (Fe, Co, Ni)-decorated graphene nanotube (GNT) material as the sulfur host for Li-S batteries (Ogoke et al., 2019). Compared to Co and CoNi, ternary FeCoNi yielded the largest diameters and the thickest wall of tubes, which increased surface areas and pore volumes with dominant mesopores that benefited from the incorporation of S into the GNT hosts. Moreover, the addition of Fe likely further improved the capacity retention of S@M-GNT cathodes (Figure 3F) through providing more favorable graphitic N and active atomic FeN4 sites, therefore enhancing electrochemical reaction kinetics and chemically/physically encapsulating the sulfur active material.
In addition, a combination of metal atoms and metal compounds to modify the carbon materials is another strategy to construct an effective catalyst and anchor substrate for LiPS (Li et al., 2019b; Li et al., 2020a; Li et al., 2020c; Tan et al., 2020). For example, Yuan et al. reported nitrogen-doped graphene nanoribbons@Co/CoOOH as an integrated sulfur host as shown in Figure 3G (Tan et al., 2020). Due to the exceptional electronic conductivity of nitrogen-doped graphene, the strong chemical adsorption and high catalytic activity of Co/CoOOH, the resulted S/nitrogen-doped graphene@Co/CoOOH cathode illustrated an excellent long-duration cyclic performance even with high areal S loading (∼3.9 mg cm−2).
Finally, our conclusions about these various Fe/Co/Ni-decorated carbon material applications in Li-S batteries are listed in Table 2. As can be seen, these iron series metal atom-doped carbon materials indeed enhance the electrochemical performances of the batteries via strong chemical trapping and excellent catalyzing even at a very high sulfur loading amount.
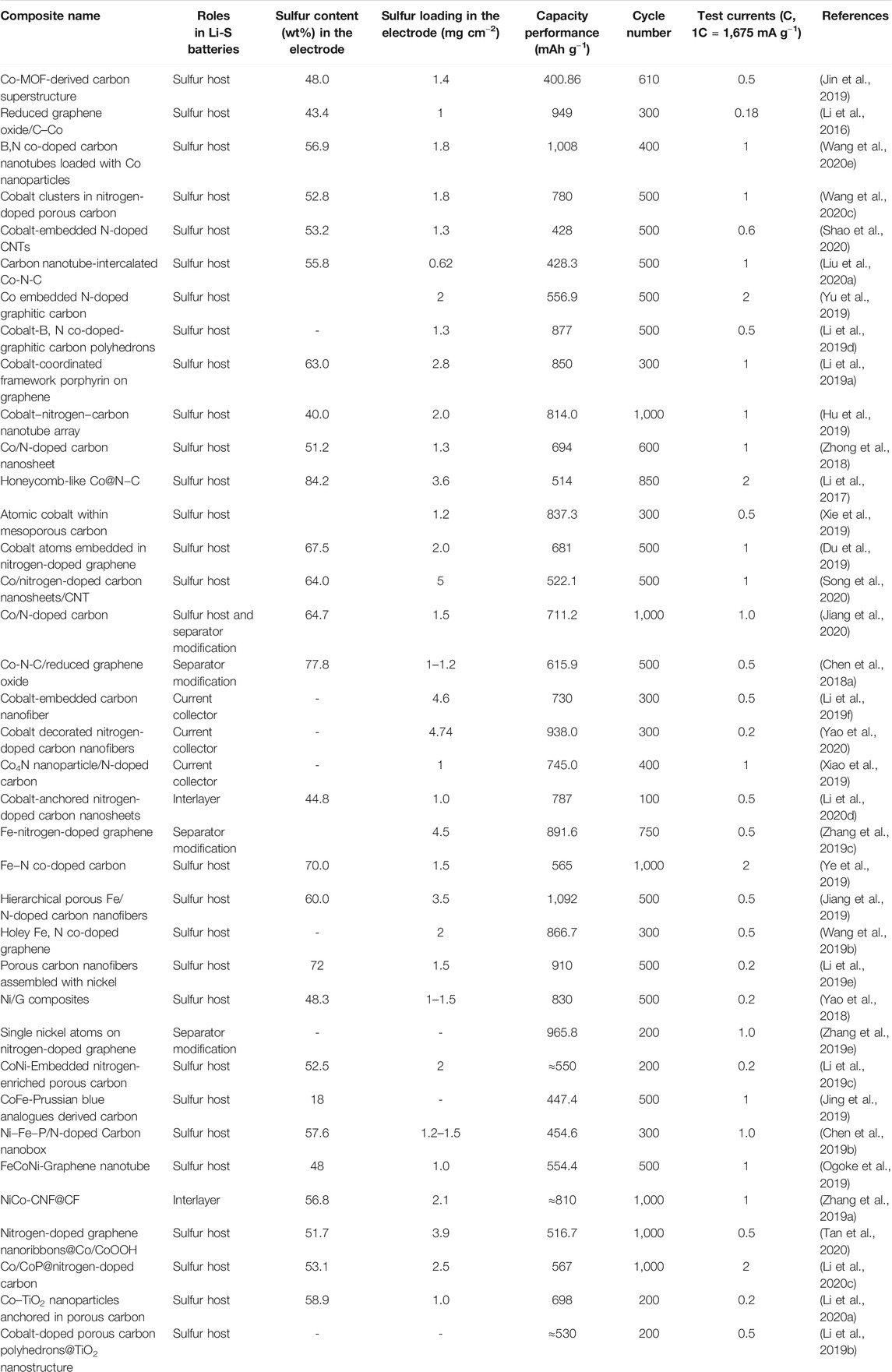
TABLE 2. Various Fe/Co/Ni-decorated carbon materials for enhancing the performances of Li-S batteries.
Other Transition Metal Atom-Decorated Carbon Materials
In addition to the above two categories of metal atom-decorated carbon materials, in recent years, some other transition metal atom-decorated carbon materials as the sulfur host have been used to simultaneously accelerate the LiPS redox reaction and chemically trap the LiPS adsorption (Sheng et al., 2019; Fan et al., 2020; Ma et al., 2020).
For example, Cui’s group first used the DFT calculation to calculate the decomposition barrier of various metal atoms (Fe, Mn, Ru, Zn, Co, Cu, V, and Ag) on N-doped graphene materials and discovered that vanadium single atoms on N-doped graphene (SAV@NG) showed the smallest decomposition barrier (1.10 eV) as shown in Figure 4A (Zhou et al., 2019). Reducing the decomposition barrier of Li2S can greatly increase the utilization of active materials, decrease the formation of dead Li2S, and achieve a long cycling life. Additionally, they also calculated the binding energy of Li2S6 on a different substrate. It showed that the SAV@NG also had the strongest binding energy as shown in Figure 4B, indicating the strongest chemical interaction between the SAV@NG and polysulfides, which means the shuttle effect can be effectively inhibited. Based on this guideline, they synthesized large-scale single atom vanadium catalysts deposited on graphene to load as high as 80 wt% sulfur content and the resulted SAV@NG achieved fast kinetics, i.e., a capacity of 645 mAh g−1 at 3 C rate.
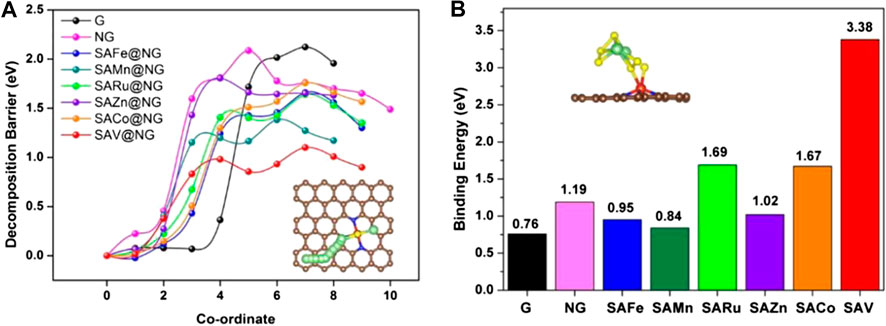
FIGURE 4. (A) Decomposition barriers of Li2S (B) binding energy of Li2S6 on the graphene, NG, SACo@NG, SAV@NG, SAFe@NG, SAMn@NG, SARu@NG, and SAZn@NG, respectively. Reproduced with permission from Zhou et al. (2019). Copyright © 2019 American Chemical Society.
Shen et al. reported a samarium-doped carbon aerogel as a polysulfide anchor for high-performance Li-S batteries (Sheng et al., 2019). Both the anchoring of polysulfides to uniformly doped Sm and the influence of the carbon aerogel structure could effectively prevent polysulfides escaping from the cathode, while also suppressing the shuttle effect and enhancing the utilization of sulfur. As a result, a CA/S/Sm electrode delivered an initial discharge capacity of 1,212 mAh g−1 at 0.5 C and a reversible capacity of 866 mAh g−1 after 300 cycles. Recently, Ding et al. also reported a cadmium-doping carbon aerogel for high-performance Li–S batteries (Ma et al., 2020). And the relative DFT calculation and experiment results have proved that Cd doping played a vital role in effectively entrapping the polysulfides.
Apart from iron series metal atom-decorated carbon materials, other transition metal atom-decorated carbon materials are also good candidates to enhance the long-cycling performances of Li-S batteries with high rate and high sulfur loading as shown in Table 3. They show more promising applications compared to the noble metal atom-decorated carbon materials.
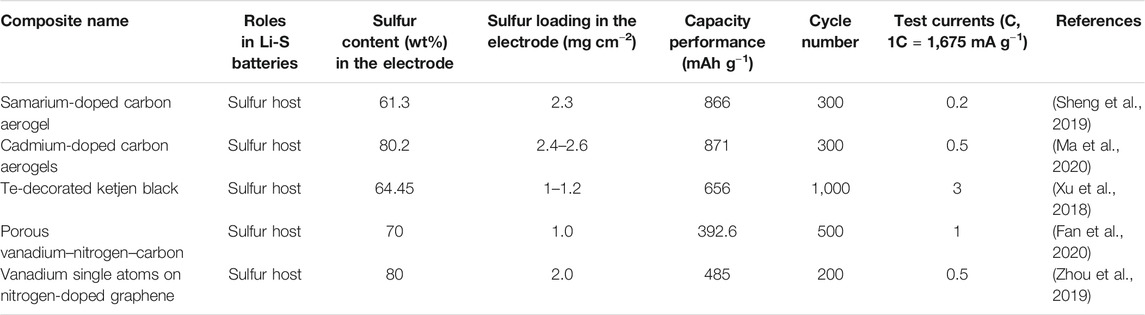
TABLE 3. Other transition metal atom-decorated carbon materials for enhancing the performances of Li-S batteries.
Metal Atom-Decorated Carbon Materials for Enhancing Li-Se Battery Performances
Although a large number of literature have suggested using heteroatom doping carbon materials and metal compounds as selenium carriers for enhancing Li-Se battery performance (Li and Yin, 2015; Yi et al., 2015; Jin et al., 2017; Lv et al., 2017; Choi et al., 2018; Gu et al., 2018; He et al., 2018; Yang et al., 2018; Zhao et al., 2018; Gu et al., 2019; Gu and Lai, 2019; Du et al., 2020), there are few reports about metal atom-doped carbon materials simultaneously catalyzing LiPSe redox reaction and chemically trapping LiPSe.
In 2017, He’s group first reported a 3D porous N-doped graphitic carbon-Co scaffold (C-Co-N) derived from metal-organic frameworks (as shown in Figures 5A,B) as a conductive Lewis base matrix to host selenium for the Li-Se battery (He et al., 2017). They employed the DFT calculation to calculate the adsorption energy of LiPSe (Figure 5C) on this C-Co-N material, which indicated that the C-Co-N matrix had excellent chemical confinement for LiPSe. As a result, the resulted C-Co-N/Se electrode demonstrated excellent cycling stability (capacity fading of only 0.07% per cycle) and rate capabilities (196.9 mAh g−1 at 10 C, 1C = 675 mA g−1) as shown in Figures 5D,E.
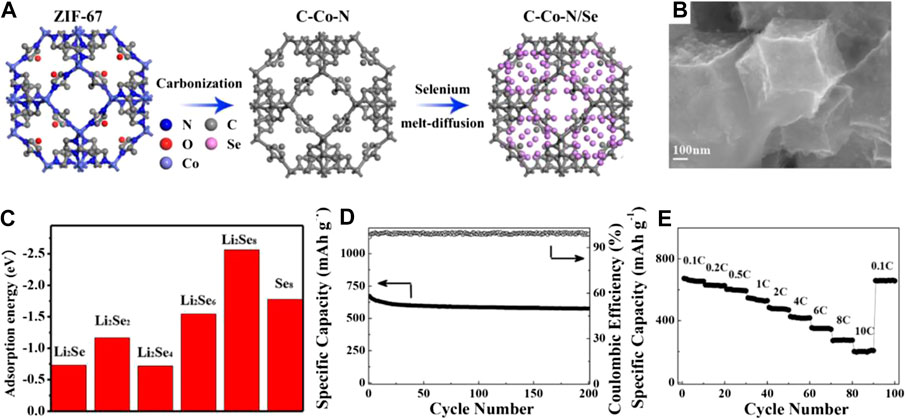
FIGURE 5. (A) Schematic illustration of the synthetic process for C-Co-N/Se (B) SEM images of C-Co-N/Se composites (C) The calculated absorption energy values of lithium polyselenides species in C-Co-N (D) cycling stability of the C-Co-N/Se cathodes at 0.1°C for 200 cycles (E) rate performances at various C-rates of the C-Co-N/Se cathodes. Reproduced with permissions from the ref (He et al., 2017). Copyright © 2017 Elsevier Ltd.
Metal Atom-Decorated Carbon Materials for Enhancing Li-SeSx Batteries Performances
The SexSy cathodes own the advantages of both Se and S, such as low cost and high reversible capacity, etc. But they also face the same issue as pure S and Se cathodes. Thus, in recent years, researchers also utilized the metal atom-decorated carbon materials to address the challenges of Li-SeSx batteries (He et al., 2018; Wang et al., 2020d; Jin et al., 2020).
For example, He et al. used a cobalt- and nitrogen-doped porous carbon (Co-N-C) polyhedron to encapsulate the SeS2 and investigated its electrochemical performances as shown in Figure 6 (He et al., 2018). As can be seen from the SEM, TEM, and corresponding element mapping images, the SeS2 have been encapsulated into the unique hollow of the cobalt- and nitrogen-doped porous carbon, and were distributed homogenously. As a result, the Co-N-C/SeS2 composite with a high loading (66.5 wt%) of SeS2 delivered a reversible capacity of 1,165.1 mAh g−1 and an 84.1% capacity retention of the initial capacity (970.2 mAh g−1) with a nearly 100% Coulombic efficiency after 200 cycles, which were superior to that of the Super P/SeS2 (SP/SeS2) and Super P-Co/SeS2 (SP-Co/SeS2) composite cathodes.
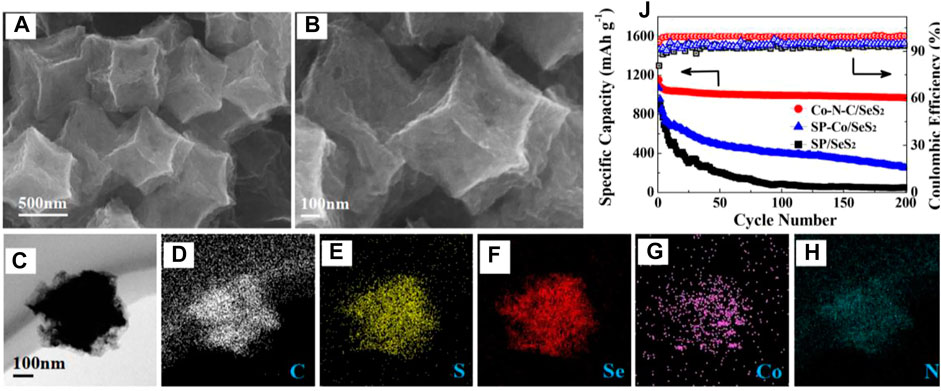
FIGURE 6. SEM images of (A) Co-N-C and (B) Co-N-C/SeS2 composites. The TEM image of a single Co-N-C/SeS2 polyhedron (C) and the corresponding elemental mapping images of (D) C (E) S (F) Se (G) Co, and (H) N (J) Cyclic stability of the SP/SeS2, SP-Co/SeS2, and Co-N-C/SeS2 cathodes at 0.2 C for 200 cycles. Reproduced with permissions from the ref (He et al., 2018). Copyright © 2018 the Royal Society of Chemistry.
Conclusion
In terms of the cathode problems of Li-S and Li-Se batteries, the sluggish redox reaction kinetics as well as the easy solubility of intermediates are major causes for the shuttle effect of LiPS/LiPSe. Therefore, it is indispensable to incorporate catalytic materials with strong adsorption and catalysis toward LiPS/LiPSe.
Metal atom-decorated carbon materials exhibited multifunctional roles, i.e., enhancing the electrode conductivity, accommodating high loading and volume expansion, adsorbing the LiPS/LiPSe, and overwhelmingly accelerating the reaction rate, which are beneficial to promote battery performance, and has shown great potential as the advanced materials for state-of-the-art energy storage devices.
Current investigations have demonstrated that metal atom-decorated carbon materials, particularly the single metal atom doping carbon, exhibited high activity on the adsorption-diffusion-conversion of LiPSs. According to previous reports, the noble metal atom-decorated carbon materials did not improve the electrochemical performances of Li-S batteries compared to the iron series metal atom-decorated carbon materials, even though they have been verified to have excellent catalytic properties in the fields of HER, OER, and CO2 reduction, etc. In contrast, other transition metal atom-decorated carbon materials, such as vanadium and samarium-decorated carbon materials, demonstrated great potential on improving the electrochemical performances of Li-S batteries. However, we should pay attention to the fact that the roles of metal atom-decorated carbon materials on LiPSe still need investigating. Future investigations could pay more attention to constructing suitable transition metal atom-decorated carbon materials for Li-Se batteries.
What is more, an in-depth understanding of the chemical scission of S-S or Se-Se bonds induced by these catalysts is still out of reach. In order to clearly understand the conversion process of sulfur/selenium redox, the in-situ characterizations, i.e., in-situ Raman/X-ray absorption spectroscopy/TEM, are suggested to observe and trace the full chemical reaction.
Another problem that cannot be ignored is the safety of the lithium anode, as it is well known that the lithium anode suffers from severe lithium dendrite problems during reduction (Ju et al., 2020). Recently, quite a few studies have reported that building lithium alloys could effectively inhibit lithium dendrite growth (Yan et al., 2016; Xue et al., 2018; Zhu et al., 2018; Wan et al., 2020). Thus, using active metal atoms in decorated carbon materials to react with lithium, form lithium alloys, and then incorporate them into the carbon framework would be a promising strategy.
Finally, for commercial application, the cost should be taken into consideration too. Low-cost and large-scale production of metal atom-decorated carbon materials are highly recommended. Presently, it is undeniable that this kind of material, especially single metal atom-doped carbon materials still cannot realize large-scale production, and the cost is still high due to the complex synthesis process. Therefore, the design structure of cost-effective metal atom-decorated carbon materials needs to be carefully considered. Anyhow, metal atom-decorated carbon materials are still promising and worth looking forward to.
Author Contributions
XG and LD conceived the idea and co-wrote the manuscript. XR and XG discussed the results and commented on the manuscript.
Funding
This work received financial support from the National Natural Science Foundation of China (No. 51902036), the Natural Science Foundation of Chongqing Science and Technology Commission (No. cstc2019jcyj-msxm1407), the Natural Science Foundation of Chongqing Technology and Business University (No. 1952009), the Venture and Innovation Support Program for Chongqing Overseas Returnees (Grant No. CX2018129), the Science and Technology Research Program of Chongqing Municipal Education Commission (Grant Nos. KJQN201900826 and KJQN201800808), the Innovation Group of New Technologies for Industrial Pollution Control of Chongqing Education Commission (Grant No. CXQT19023), and the Key Disciplines of Chemical Engineering and Technology in Chongqing Colleges and Universities during the 13th Five Year Plan.
Conflict of Interest
The authors declare that the research was conducted in the absence of any commercial or financial relationships that could be construed as a potential conflict of interest.
References
Al Salem, H., Babu, G., Rao, C. V., and Arava, L. M. (2015). Electrocatalytic polysulfide traps for controlling redox shuttle process of Li-S batteries. J. Am. Chem. Soc. 137, 11542–11545. doi:10.1021/jacs.5b04472
Babu, G., Ababtain, K., Ng, K. Y., and Arava, L. M. (2015). Electrocatalysis of lithium polysulfides: current collectors as electrodes in Li/S battery configuration. Sci. Rep. 5, 8763. doi:10.1038/srep08763
Cao, L., Luo, Q., Liu, W., Lin, Y., Liu, X., Cao, Y., et al. (2018). Identification of single-atom active sites in carbon-based cobalt catalysts during electrocatalytic hydrogen evolution. Nat. Catal. 2, 134–141. doi:10.1038/s41929-018-0203-5
Chen, G., Song, X., Wang, S., Wang, Y., Gao, T., Ding, L.-X., et al. (2018a). A multifunctional separator modified with cobalt and nitrogen co-doped porous carbon nanofibers for Li-S batteries. J. Membr. Sci. 548, 247–253. doi:10.1016/j.memsci.2017.11.026
Chen, Z., Zhao, J., Cabrera, C. R., and Chen, Z. (2018b). Computational screening of efficient single‐atom catalysts based on graphitic carbon nitride (g‐C 3 N 4 ) for nitrogen electroreduction. Small Methods 3, 1800368. doi:10.1002/smtd.201800368
Chen, M., Zheng, J., Sheng, O., Jin, C., Yuan, H., Liu, T., et al. (2019a). Sulfur-nitrogen co-doped porous carbon nanosheets to control lithium growth for a stable lithium metal anode. J. Mater. Chem. A. 7, 18267–18274. doi:10.1039/C9TA05684J
Chen, X., Zeng, S., Muheiyati, H., Zhai, Y., Li, C., Ding, X., et al. (2019b). Double-shelled Ni-Fe-P/N-doped carbon nanobox derived from a prussian blue analogue as an electrode material for K-ion batteries and Li-S batteries. ACS Energ. Lett. 4, 1496–1504. doi:10.1021/acsenergylett.9b00573
Choi, D. S., Yeom, M. S., Kim, Y. T., Kim, H., and Jung, Y. (2018). Polyselenide anchoring using transition-metal disulfides for enhanced lithium-selenium batteries. Inorg. Chem. 57, 2149–2156. doi:10.1021/acs.inorgchem.7b03001
Cui, X., Li, W., Ryabchuk, P., Junge, K., and Beller, M. (2018). Bridging homogeneous and heterogeneous catalysis by heterogeneous single-metal-site catalysts. Nat. Catal. 1, 385–397. doi:10.1038/s41929-018-0090-9
Du, H., Feng, S., Luo, W., Zhou, L., and Mai, L. (2020). Advanced Li-Se S battery system: electrodes and electrolytes. J. Mater. Sci. Tech. 55, 1. doi:10.1016/j.jmst.2020.01.001
Du, Z., Chen, X., Hu, W., Chuang, C., Xie, S., Hu, A., et al. (2019). Cobalt in nitrogen-doped graphene as single-atom catalyst for high-sulfur content lithium-sulfur batteries. J. Am. Chem. Soc. 141, 3977–3985. doi:10.1021/jacs.8b12973
Fan, C. Y., Xiao, P., Li, H. H., Wang, H. F., Zhang, L. L., Sun, H. Z., et al. (2015). Nanoscale polysulfides reactors achieved by chemical Au-S interaction: improving the performance of Li-S batteries on the electrode level. ACS Appl. Mater. Inter. 7, 27959–27967. doi:10.1021/acsami.5b10300
Fan, Y., Ma, F., Liang, J., Chen, X., Miao, Z., Duan, S., et al. (2020). Accelerated polysulfide conversion on hierarchical porous vanadium-nitrogen-carbon for advanced lithium-sulfur batteries. Nanoscale 12, 584–590. doi:10.1039/c9nr09037a
Gawande, M. B., Fornasiero, P., and Zbořil, R. (2020). Carbon-based single-atom catalysts for advanced applications. ACS Catal. 10, 2231–2259. doi:10.1021/acscatal.9b04217
Gu, X., Kang, H., Shao, C., Ren, X., and Liu, X. (2020a). A Typha angustifolia-like MoS2/carbon nanofiber composite for high performance Li-S batteries. Front. Chem. 8, 149. doi:10.3389/fchem.2020.00149
Gu, X., Tong, C. J., Rehman, S., Liu, L. M., Hou, Y., and Zhang, S. (2016b). Multifunctional nitrogen-doped loofah sponge carbon blocking layer for high-performance rechargeable lithium batteries. ACS Appl. Mater. Inter. 8, 15991–16001. doi:10.1021/acsami.6b02378
Gu, X., and Lai, C. (2019). One dimensional nanostructures contribute better Li-S and Li-Se batteries: progress, challenges and perspectives. Energ. Storage Mater. 23, 190–224. doi:10.1016/j.ensm.2019.05.013
Gu, X., and Lai, C. (2017). Recent development of metal compound applications in lithium-sulphur batteries. J. Mater. Res. 33, 16–31. doi:10.1557/jmr.2017.282
Gu, X., Li, H., Wen, H., Zhou, Y., Kang, H., Liao, H., et al. (2020b). From agaric hydrogel to nitrogen-doped 3D porous carbon for high-performance Li-S batteries. J. Mater. Sci. 55, 1136–1147. doi:10.1007/s10853-019-03999-3
Gu, X., Tang, T., Liu, X., and Hou, Y. (2019). Rechargeable metal batteries based on selenium cathodes: progress, challenges and perspectives. J. Mater. Chem. A. 7, 11566–11583. doi:10.1039/c8ta12537f
Gu, X., Tong, C.-J., Lai, C., Qiu, J., Huang, X., Yang, W., et al. (2015a). A porous nitrogen and phosphorous dual doped graphene blocking layer for high performance Li-S batteries. J. Mater. Chem. A. 3, 16670–16678. doi:10.1039/C5TA04255K
Gu, X., Tong, C.-J., Wen, B., Liu, L.-M., Lai, C., and Zhang, S. (2016a). Ball-milling synthesis of ZnO@sulphur/carbon nanotubes and Ni(OH)2@sulphur/carbon nanotubes composites for high-performance lithium-sulphur batteries. Electrochimica Acta 196, 369–376. doi:10.1016/j.electacta.2016.03.018
Gu, X., Wang, Y., Lai, C., Qiu, J., Li, S., Hou, Y., et al. (2015b). Microporous bamboo biochar for lithium-sulfur batteries. Nano Res. 8, 129–139. doi:10.1007/s12274-014-0601-1
Gu, X., Xin, L., Li, Y., Dong, F., Fu, M., and Hou, Y. (2018). Highly reversible Li-Se batteries with ultra-lightweight N,S-codoped graphene blocking layer. Nano-micro Lett. 10, 59–68. doi:10.1007/s40820-018-0213-5
Han, X.-R., Guo, X.-T., Xu, M.-J., Pang, H., and Ma, Y.-W. (2020). Clean utilization of palm kernel shell: sustainable and naturally heteroatom-doped porous activated carbon for lithium-sulfur batteries. Rare Met. 39, 1099–1106. doi:10.1007/s12598-020-01439-9
He, J., Chen, Y., Lv, W., Wen, K., Xu, C., Zhang, W., et al. (2016). From metal-organic framework to Li2S@C-Co-N nanoporous architecture: a high-capacity cathode for lithium-sulfur batteries. ACS Nano 10, 10981–10987. doi:10.1021/acsnano.6b05696
He, J., Lv, W., Chen, Y., Xiong, J., Wen, K., Xu, C., et al. (2018). Direct impregnation of SeS2 into a MOF-derived 3D nanoporous Co-N-C architecture towards superior rechargeable lithium batteries. J. Mater. Chem. A. 6, 10466–10473. doi:10.1039/c8ta02434k
He, J., Lv, W., Chen, Y., Xiong, J., Wen, K., Xu, C., et al. (2017). Three-dimensional hierarchical C-Co-N/Se derived from metal-organic framework as superior cathode for Li-Se batteries. J. Power Sourc. 363, 103–109. doi:10.1016/j.jpowsour.2017.07.065
He, J., and Manthiram, A. (2019). A review on the status and challenges of electrocatalysts in lithium-sulfur batteries. Energ. Storage Mater. 20, 55–70. doi:10.1016/j.ensm.2019.04.038
Hong, X., Wang, R., Liu, Y., Fu, J., Liang, J., and Dou, S. (2020). Recent advances in chemical adsorption and catalytic conversion materials for Li-S batteries. J. Energ. Chem. 42, 144–168. doi:10.1016/j.jechem.2019.07.001
Hou, Y., Qiu, M., Kim, M. G., Liu, P., Nam, G., Zhang, T., et al. (2019). Atomically dispersed nickel-nitrogen-sulfur species anchored on porous carbon nanosheets for efficient water oxidation. Nat. Commun. 10, 1392. doi:10.1038/s41467-019-09394-5
Hu, C., Yang, C., Yang, J., Han, N., Yuan, R., Chen, Y., et al. (2019). An entangled cobalt-nitrogen-carbon nanotube Array electrode with synergetic confinement and electrocatalysis of polysulfides for stable Li-S batteries. ACS Appl. Energ. Mater. 2, 2904–2912. doi:10.1021/acsaem.9b00243
Ji, X., Lee, K. T., and Nazar, L. F. (2009). A highly ordered nanostructured carbon-sulphur cathode for lithium-sulphur batteries. Nat. Mater. 8, 500–506. doi:10.1038/nmat2460
Jiang, M., Wang, R., Wang, K., Gao, S., Han, J., Yan, J., et al. (2019). Hierarchical porous Fe/N doped carbon nanofibers as host materials for high sulfur loading Li-S batteries. Nanoscale 11, 15156–15165. doi:10.1039/c9nr04408f
Jiang, S., Huang, S., Yao, M., Zhu, J., Liu, L., and Niu, Z. (2020). Bimetal-organic frameworks derived Co/N-doped carbons for lithium-sulfur batteries. Chin. Chem. Lett. 31, 2347. doi:10.1016/j.cclet.2020.04.014
Jin, J., Tian, X., Srikanth, N., Kong, L. B., and Zhou, K. (2017). Advances and challenges of nanostructured electrodes for Li-Se batteries. J. Mater. Chem. A. 5, 10110–10126. doi:10.1039/c7ta01384a
Jin, W.-W., Zou, J.-Z., Zeng, S.-Z., Inguva, S., Xu, G.-Z., Li, X.-H., et al. (2019). Tailoring the structure of clew-like carbon skeleton with 2D Co-MOF for advanced Li-S cells. Appl. Surf. Sci. 469, 404–413. doi:10.1016/j.apsusc.2018.11.052
Jin, Y., Liu, K., Lang, J., Jiang, X., Zheng, Z., Su, Q., et al. (2020). High-energy-density solid-electrolyte-based liquid Li-S and Li-Se batteries. Joule 4, 262–274. doi:10.1016/j.joule.2019.09.003
Jing, S., Ding, P., Zhang, Y., Liang, H., Yin, S., and Tsiakaras, P. (2019). Lithium-sulfur battery cathodes made of porous biochar support CoFe@NC metal nanoparticles derived from prussian blue analogues. Ionics 25, 5297–5304. doi:10.1007/s11581-019-03065-7
Ju, Z., Nai, J., Wang, Y., Liu, T., Zheng, J., Yuan, H., et al. (2020). Biomacromolecules enabled dendrite-free lithium metal battery and its origin revealed by cryo-electron microscopy. Nat. Commun. 11, 488. doi:10.1038/s41467-020-14358-1
Li, Z., Li, C., Ge, X., Ma, J., Zhang, Z., Li, Q., et al. (2016). Reduced graphene oxide wrapped MOFs-derived cobalt-doped porous carbon polyhedrons as sulfur immobilizers as cathodes for high performance lithium sulfur batteries. Nano Energy 23, 15–26. doi:10.1016/j.nanoen.2016.02.049
Li, Y., Fan, J., Zhang, J., Yang, J., Yuan, R., Chang, J., et al. (2017). A honeycomb-like Co@N-C composite for ultrahigh sulfur loading Li-S batteries. ACS Nano 11, 11417–11424. doi:10.1021/acsnano.7b06061
Li, B. Q., Kong, L., Zhao, C. X., Jin, Q., Chen, X., Peng, H. J., et al. (2019a). Expediting redox kinetics of sulfur species by atomic‐scale electrocatalysts in lithium-sulfur batteries. InfoMat 1, 533–541. doi:10.1002/inf2.12056
Li, M., Feng, W., Su, W., Song, C., and Chen, L. (2019b). Titanium dioxide wrapped MOFs-derived cobalt-doped porous carbon polyhedrons as sulfur hosts for advanced lithium-sulfur batteries. Integrated Ferroelectrics 200, 82–89. doi:10.1080/10584587.2019.1592623
Li, M., Feng, W., Su, W., and Wang, X. (2019c). CoNi-embedded nitrogen-enriched porous carbon framework for long-life lithium-sulfur batteries. J. Solid State. Electrochem. 23, 2317–2324. doi:10.1007/s10008-019-04346-x
Li, N., Chen, K., Chen, S., Wang, F., Wang, D., Gan, F., et al. (2019d). Manipulating the redox kinetics of Li S chemistry by porous hollow cobalt-B, N codoped-graphitic carbon polyhedrons for high performance lithium-sulfur batteries. Carbon 149, 564–571. doi:10.1016/j.carbon.2019.04.022
Li, Q., Guo, J., Zhao, J., Wang, C., and Yan, F. (2019e). Porous nitrogen-doped carbon nanofibers assembled with nickel nanoparticles for lithium-sulfur batteries. Nanoscale. 11, 647–655. doi:10.1039/c8nr07220e
Li, S., Chen, X., Hu, F., Zeng, R., Huang, Y., Yuan, L., et al. (2019f). Cobalt-embedded carbon nanofiber as electrocatalyst for polysulfide redox reaction in lithium sulfur batteries. Electrochimica Acta 304, 11–19. doi:10.1016/j.electacta.2019.02.087
Li, H., Li, C., Liu, N., Wang, Y., and Zhang, Y. (2020a). Co-TiO2 nanoparticles anchored in porous carbon matrix as an efficient sulfur host for lithium/sulfur batteries. J. Alloys Comp. 818, 152868. doi:10.1016/j.jallcom.2019.152868
Li, Y., Guo, X.-T., Zhang, S.-T., and Pang, H. (2020b). Promoting performance of lithium-sulfur battery via in situ sulfur reduced graphite oxide coating. Rare Met. 40, 417. doi:10.1007/s12598-020-01498-y
Li, Y., Xu, P., Chen, G., Mou, J., Xue, S., Li, K., et al. (2020d). Enhancing Li-S redox kinetics by fabrication of a three dimensional Co/CoP@nitrogen-doped carbon electrocatalyst. Chem. Eng. J. 380, 122595. doi:10.1016/j.cej.2019.122595
Li, Y., Zhou, P., Li, H., Gao, T., Zhou, L., Zhang, Y., et al. (2020c). A freestanding flexible single‐atom cobalt‐based multifunctional interlayer toward reversible and durable lithium‐sulfur batteries. Small Methods 4, 1900701. doi:10.1002/smtd.201900701
Li, Z., and Yin, L. (2015). MOF-derived, N-doped, hierarchically porous carbon sponges as immobilizers to confine selenium as cathodes for Li-Se batteries with superior storage capacity and perfect cycling stability. Nanoscale 7, 9597–9606. doi:10.1039/c5nr00903k
Lim, W. G., Kim, S., Jo, C., and Lee, J. (2019). A comprehensive review of materials with catalytic effects in Li-S batteries: enhanced redox kinetics. Angew. Chem. Int. Ed. Engl. 58, 18746–18757. doi:10.1002/anie.201902413
Liu, B., Fan, K., Li, J., Liu, G., Liu, Y., Yang, C., et al. (2020a). Carbon nanotubes-intercalated Co-N-C as a robust sulfur host for lithium-sulfur batteries. Mater. Res. Bull. 121, 110625. doi:10.1016/j.materresbull.2019.110625
Liu, J. (2016). Catalysis by supported single metal atoms. ACS Catal. 7, 34–59. doi:10.1021/acscatal.6b01534
Liu, T., Hu, H., Ding, X., Yuan, H., Jin, C., Nai, J., et al. (2020b). 12 years roadmap of the sulfur cathode for lithium sulfur batteries (2009-2020). Energ. Storage Mater. 30, 346–366. doi:10.1016/j.ensm.2020.05.023
Liu, Y., Kou, W., Li, X., Huang, C., Shui, R., and He, G. (2019). Constructing patch‐Ni‐shelled Pt@Ni nanoparticles within confined nanoreactors for catalytic oxidation of insoluble polysulfides in Li‐S batteries. Small 15, 1902431. doi:10.1002/smll.201902431
Lu, P., Yang, Y., Yao, J., Wang, M., Dipazir, S., Yuan, M., et al. (2019). Facile synthesis of single-nickel-atomic dispersed N-doped carbon framework for efficient electrochemical CO2 reduction. Appl. Catal. B: Environ. 241, 113–119. doi:10.1016/j.apcatb.2018.09.025
Lv, H., Chen, R., Wang, X., Hu, Y., Wang, Y., Chen, T., et al. (2017). High-performance Li-Se batteries enabled by selenium storage in bottom-up synthesized nitrogen-doped carbon scaffolds. ACS Appl. Mater. Inter. 9, 25232–25238. doi:10.1021/acsami.7b04321
Ma, L., Li, X., Gao, W., Zhang, X., Xu, P., Shu, Y., et al. (2020). The immobilizing polysulfide mechanism of cadmium-doping carbon aerogels via a microtemplate for high performance Li-S batteries. New J. Chem. 44, 1001–1008. doi:10.1039/c9nj05405g
Ma, Q., Chen, Z., Zhong, S., Meng, J., Lai, F., Li, Z., et al. (2021b). Na-substitution induced oxygen vacancy achieving high transition metal capacity in commercial Li-rich cathode. Nano Energy 81, 105622. doi:10.1016/j.nanoen.2020.105622
Ma, S., Wang, L., Wang, Y., Zuo, P., He, M., Zhang, H., et al. (2019). Palladium nanocrystals-imbedded mesoporous hollow carbon spheres with enhanced electrochemical kinetics for high performance lithium sulfur batteries. Carbon. 143, 878–889. doi:10.1016/j.carbon.2018.11.086
Ogoke, O., Hwang, S., Hultman, B., Chen, M., Karakalos, S., He, Y., et al. (2019). Large-diameter and heteroatom-doped graphene nanotubes decorated with transition metals as carbon hosts for lithium-sulfur batteries. J. Mater. Chem. A. 7, 13389–13399. doi:10.1039/c9ta02889g
O’connor, N. J., Jonayat, A. S. M., Janik, M. J., and Senftle, T. P. (2018). Interaction trends between single metal atoms and oxide supports identified with density functional theory and statistical learning. Nat. Catal. 1, 531–539. doi:10.1038/s41929-018-0094-5
Qu, D., Li, R., Zhu, X., Chen, K., Shen, Y., Liu, D., et al. (2018). Performances of platinum and nitrogen dual-doped ordered mesoporous carbon as sulfur host for Li-S battery. Int. J. Electrochem. Sci. 13(11), 11294–11322. doi:10.20964/2018.11.31
Ren, S., Yu, Q., Yu, X., Rong, P., Jiang, L., and Jiang, J. (2020). Graphene-supported metal single-atom catalysts: a concise review. Sci. China Mater. 63, 903–920. doi:10.1007/s40843-019-1286-1
Ruan, S., Huang, Z., Cai, W., Ma, C., Liu, X., Wang, J., et al. (2020). Enabling rapid polysulfide conversion kinetics by using functionalized carbon nanosheets as metal-free electrocatalysts in durable lithium-sulfur batteries. Chem. Eng. J. 385, 123840. doi:10.1016/j.cej.2019.123840
Shao, A.-H., Zhang, Z., Xiong, D.-G., Yu, J., Cai, J.-X., and Yang, Z.-Y. (2020). Facile synthesis of a “Two-in-One” sulfur host featuring metallic-cobalt-embedded N-doped carbon nanotubes for efficient lithium-sulfur batteries. ACS Appl. Mater. Inter. 12, 5968–5978. doi:10.1021/acsami.9b20943
Sheng, H., Li, X., Huang, B., Wang, J., Li, X., and Hua, Y. (2019). A samarium-doped carbon aerogel cathode with anchored polysulfides for lithium-sulfur batteries with high electrochemical performance: a metal-organic framework template method. Chempluschem 84, 838–844. doi:10.1002/cplu.201900216
Sheng, O., Zheng, J., Ju, Z., Jin, C., Wang, Y., Chen, M., et al. (2020). In situ construction of a LiF-enriched interface for stable all-solid-state batteries and its origin revealed by cryo-TEM. Adv. Mater. 32, e2000223. doi:10.1002/adma.202000223
Song, C.-L., Li, G.-H., Yang, Y., Hong, X.-J., Huang, S., Zheng, Q.-F., et al. (2020). 3D catalytic MOF-based nanocomposite as separator coatings for high-performance Li-S battery. Chem. Eng. J. 381, 122701. doi:10.1016/j.cej.2019.122701
Song, Y., Cai, W., Kong, L., Cai, J., Zhang, Q., and Sun, J. (2019). Rationalizing electrocatalysis of Li-S chemistry by mediator design: progress and prospects. Adv. Energ. Mater. 10, 1901075. doi:10.1002/aenm.201901075
Tan, K., Liu, Y., Tan, Z., Zhang, J., Hou, L., and Yuan, C. (2020). High-yield and in situ fabrication of high-content nitrogen-doped graphene nanoribbons@Co/CoOOH as an integrated sulfur host towards Li-S batteries. J. Mater. Chem. A. 8, 3048–3059. doi:10.1039/c9ta13414j
Wan, M., Kang, S., Wang, L., Lee, H. W., Zheng, G. W., Cui, Y., et al. (2020). Mechanical rolling formation of interpenetrated lithium metal/lithium tin alloy foil for ultrahigh-rate battery anode. Nat. Commun. 11, 829. doi:10.1038/s41467-020-14550-3
Wang, T., Zhao, Q., Fu, Y., Lei, C., Yang, B., Li, Z., et al. (2019a). Carbon‐Rich nonprecious metal single atom electrocatalysts for CO 2 reduction and hydrogen evolution. Small Methods 3, 1900210. doi:10.1002/smtd.201900210
Wang, Y., Adekoya, D., Sun, J., Tang, T., Qiu, H., Xu, L., et al. (2019b). Manipulation of edge‐site Fe-N 2 moiety on holey Fe, N codoped graphene to promote the cycle stability and rate capacity of Li-S batteries. Adv. Funct. Mater. 29, 1807485. doi:10.1002/adfm.201807485
Wang, J., Jia, L., Duan, S., Liu, H., Xiao, Q., Li, T., et al. (2020a). Single atomic cobalt catalyst significantly accelerates lithium ion diffusion in high mass loading Li2S cathode. Energ. Storage Mater. 28, 375–382. doi:10.1016/j.ensm.2020.03.023
Wang, P., Ren, Y., Wang, R., Zhang, P., Ding, M., Li, C., et al. (2020b). Atomically dispersed cobalt catalyst anchored on nitrogen-doped carbon nanosheets for lithium-oxygen batteries. Nat. Commun. 11, 1576. doi:10.1038/s41467-020-15416-4
Wang, R., Yang, J., Chen, X., Zhao, Y., Zhao, W., Qian, G., et al. (2020c). Highly dispersed cobalt clusters in nitrogen‐doped porous carbon enable multiple effects for high‐performance Li-S battery. Adv. Energ. Mater. 10, 1903550. doi:10.1002/aenm.201903550
Wang, Y., Liang, P., Yang, H., Li, W., Wang, Z., Liu, Z., et al. (2020d). Hollow CoP nanoparticles embedded in Two-Dimensional N-doped carbon arrays enabling advanced Li-SeS2 batteries with rapid kinetics. Mater. Today Energ. 17, 100423. doi:10.1016/j.mtener.2020.100423
Wang, Z., Shen, J., Ji, S., Xu, X., Zuo, S., Liu, Z., et al. (2020e). B,N codoped graphitic nanotubes loaded with Co nanoparticles as superior sulfur host for advanced Li-S batteries. Small 16, 1906634. doi:10.1002/smll.201906634
Wu, Q., Zhou, X., Xu, J., Cao, F., and Li, C. (2019). Carbon-based derivatives from metal-organic frameworks as cathode hosts for Li-S batteries. J. Energ. Chem. 38, 94–113. doi:10.1016/j.jechem.2019.01.005
Xiao, K., Wang, J., Chen, Z., Qian, Y., Liu, Z., Zhang, L., et al. (2019). Improving polysulfides adsorption and redox kinetics by the Co 4 N nanoparticle/N‐doped carbon composites for lithium‐sulfur batteries. Small 15, 1901454. doi:10.1002/smll.201901454
Xie, J., Li, B. Q., Peng, H. J., Song, Y. W., Zhao, M., Chen, X., et al. (2019). Implanting atomic cobalt within mesoporous carbon toward highly stable lithium-sulfur batteries. Adv. Mater. 31, 1903813. doi:10.1002/adma.201903813
Xu, K., Liu, X., Liang, J., Cai, J., Zhang, K., Lu, Y., et al. (2018). Manipulating the redox kinetics of Li-S chemistry by tellurium doping for improved Li-S batteries. ACS Energ. Lett. 3, 420–427. doi:10.1021/acsenergylett.7b01249
Xue, P., Liu, S., Shi, X., Sun, C., Lai, C., Zhou, Y., et al. (2018). A hierarchical silver-nanowire-graphene host enabling ultrahigh rates and superior long-term cycling of lithium-metal composite anodes. Adv. Mater. 30, e1804165–1804174. doi:10.1002/adma.201804165
Yan, K., Lu, Z., Lee, H.-W., Xiong, F., Hsu, P.-C., Li, Y., et al. (2016). Selective deposition and stable encapsulation of lithium through heterogeneous seeded growth. Nat. Energ. 1, 1–8. doi:10.1038/nenergy.2016.10
Yan, M., Wang, W.-P., Yin, Y.-X., Wan, L.-J., and Guo, Y.-G. (2019). Interfacial design for lithium-sulfur batteries: from liquid to solid. EnergyChem. 1, 100002. doi:10.1016/j.enchem.2019.100002
Yang, J., Gao, H., Ma, D., Zou, J., Lin, Z., Kang, X., et al. (2018). High-performance Li-Se battery cathode based on CoSe 2 -porous carbon composites. Electrochimica Acta 264, 341–349. doi:10.1016/j.electacta.2018.01.105
Yang, Q., Yang, C. C., Lin, C. H., and Jiang, H. L. (2019). Metal-organic-framework-derived hollow N-doped porous carbon with ultrahigh concentrations of single Zn atoms for efficient carbon dioxide conversion. Angew. Chem. Int. Ed. Engl. 58, 3511–3515. doi:10.1002/anie.201813494
Yao, S., Guo, R., Xie, F., Wu, Z., Gao, K., Zhang, C., et al. (2020). Electrospun three-dimensional cobalt decorated nitrogen doped carbon nanofibers network as freestanding electrode for lithium/sulfur batteries. Electrochimica Acta 337, 135765. doi:10.1016/j.electacta.2020.135765
Yao, X., Xu, J., Hong, Z., Li, G., Wang, X., Lu, F., et al. (2018). Metal/graphene composites with strong metal-S bondings for sulfur immobilization in Li-S batteries. J. Phys. Chem. C. 122, 3263–3272. doi:10.1021/acs.jpcc.7b12063
Ye, H., Sun, J., Zhang, S., Lin, H., Zhang, T., Yao, Q., et al. (2019). Stepwise electrocatalysis as a strategy against polysulfide shuttling in Li-S batteries. ACS Nano 13, 14208–14216. doi:10.1021/acsnano.9b07121
Yi, Z., Yuan, L., Sun, D., Li, Z., Wu, C., Yang, W., et al. (2015). High-performance lithium-selenium batteries promoted by heteroatom-doped microporous carbon. J. Mater. Chem. A. 3, 3059–3065. doi:10.1039/c4ta06141a
Yu, M., Zhou, S., Wang, Z., Wang, Y., Zhang, N., Wang, S., et al. (2019). Accelerating polysulfide redox conversion on bifunctional electrocatalytic electrode for stable Li-S batteries. Energ. Storage Mater. 20, 98–107. doi:10.1016/j.ensm.2018.11.028
Zeng, L.-C., Li, W.-H., Jiang, Y., and Yu, Y. (2017). Recent progress in Li-S and Li-Se batteries. Rare Met. 36, 339–364. doi:10.1007/s12598-017-0891-z
Zeng, Q.-W., Hu, R.-M., Chen, Z.-B., and Shang, J.-X. (2019). Single-atom Fe and N co-doped graphene for lithium-sulfur batteries: a density functional theory study. Mater. Res. Express 6, 095620. doi:10.1088/2053-1591/ab33ad
Zhang, J., Li, G., Zhang, Y., Zhang, W., Wang, X., Zhao, Y., et al. (2019a). Vertically rooting multifunctional tentacles on carbon scaffold as efficient polysulfide barrier toward superior lithium-sulfur batteries. Nano Energy 64, 103905. doi:10.1016/j.nanoen.2019.103905
Zhang, J., Liu, C., and Zhang, B. (2019b). Insights into single‐atom metal-support interactions in electrocatalytic water splitting. Small Methods 3, 1800481. doi:10.1002/smtd.201800481
Zhang, K., Chen, Z., Ning, R., Xi, S., Tang, W., Du, Y., et al. (2019c). Single-atom coated separator for robust lithium-sulfur batteries. ACS Appl. Mater. Inter. 11, 25147–25154. doi:10.1021/acsami.9b05628
Zhang, L., Jia, Y., Liu, H., Zhuang, L., Yan, X., Lang, C., et al. (2019d). Charge polarization from atomic metals on adjacent graphitic layers for enhancing the hydrogen evolution reaction. Angew. Chem. Int. Ed. Engl. 58, 9404–9408. doi:10.1002/anie.201902107
Zhang, L., Liu, D., Muhammad, Z., Wan, F., Xie, W., Wang, Y., et al. (2019e). Single nickel atoms on nitrogen‐doped graphene enabling enhanced kinetics of lithium-sulfur batteries. Adv. Mater. 31, 1903955. doi:10.1002/adma.201903955
Zhang, M., Chen, W., Xue, L., Jiao, Y., Lei, T., Chu, J., et al. (2019f). Adsorption‐catalysis design in the lithium‐sulfur battery. Adv. Energ. Mater. 10, 1903008. doi:10.1002/aenm.201903008
Zhang, W., Yang, C., Ding, B., Peng, J., Xu, F., Zheng, M., et al. (2020). A self-crosslinking procedure to construct yolk-shell Au@microporous carbon nanospheres for lithium-sulfur batteries. Chem. Commun. (Camb) 56, 1215–1218. doi:10.1039/c9cc07625e
Zhang, W., Zhang, J., Zhao, Y., and Wang, X. (2019g). Multi-functional carbon cloth infused with N-doped and Co-coated carbon nanofibers as a current collector for ultra-stable lithium-sulfur batteries. Mater. Lett. 255, 126595. doi:10.1016/j.matlet.2019.126595
Zhao, X., Yin, L., Zhang, T., Zhang, M., Fang, Z., Wang, C., et al. (2018). Heteroatoms dual-doped hierarchical porous carbon-selenium composite for durable Li-Se and Na-Se batteries. Nano Energy 49, 137–146. doi:10.1016/j.nanoen.2018.04.045
Zheng, M., Chi, Y., Hu, Q., Tang, H., Jiang, X., Zhang, L., et al. (2019). Carbon nanotube-based materials for lithium-sulfur batteries. J. Mater. Chem. A. 7, 17204–17241. doi:10.1039/c9ta05347f
Zhong, M.-E., Guan, J., Feng, Q., Wu, X., Xiao, Z., Zhang, W., et al. (2018). Accelerated polysulfide redox kinetics revealed by ternary sandwich-type S@Co/N-doped carbon nanosheet for high-performance lithium-sulfur batteries. Carbon 128, 86–96. doi:10.1016/j.carbon.2017.11.084
Zhou, G., Zhao, S., Wang, T., Yang, S.-Z., Johannessen, B., Chen, H., et al. (2019). Theoretical calculation guided design of single-atom catalysts toward fast kinetic and long-life Li-S batteries. Nano Lett. 20, 1252–1261. doi:10.1021/acs.nanolett.9b04719
Keywords: metal atoms, decorated, carbon nanomaterials, Li-S batteries, Li-Se batteries
Citation: Gu X, Deng L and Ren X (2021) Metal Atom-Decorated Carbon Nanomaterials for Enhancing Li-S/Se Batteries Performances: A Mini Review. Front. Energy Res. 9:626596. doi: 10.3389/fenrg.2021.626596
Received: 06 November 2020; Accepted: 21 January 2021;
Published: 31 March 2021.
Edited by:
Zhan Lin, Zhejiang University, ChinaReviewed by:
Tiefeng Liu, Zhejiang University of Technology, ChinaHuan Pang, Yangzhou University, China
Copyright © 2021 Gu, Deng and Ren This is an open-access article distributed under the terms of the Creative Commons Attribution License (CC BY). The use, distribution or reproduction in other forums is permitted, provided the original author(s) and the copyright owner(s) are credited and that the original publication in this journal is cited, in accordance with accepted academic practice. No use, distribution or reproduction is permitted which does not comply with these terms.
*Correspondence: Xingxing Gu, eC5ndUBjdGJ1LmVkdS5jbg==
†ORCID: Xingxing Gu orcid.org/0000-0002-5145-7751