- 1Mechanical Engineering Department, Universiti Teknologi PETRONAS, Perak, Malaysia
- 2Faculty of Mechanical and Aeronautical Engineering, University of Engineering and Technology Taxila, Taxila, Pakistan
- 3School of Engineering and Physical Sciences (EPS), Heriot-Watt University, Edinburgh, United Kingdom
- 4Department of Mechanical Engineering, COMSATS University Islamabad (Wah Campus), Wah Cantt, Pakistan
- 5Electrical Engineering Department, Universiti Teknologi PETRONAS, Perak, Malaysia
The integration of evacuated tube receivers with non-imaging compound parabolic concentrators (CPCs) operating in thermosyphon mode provides the opportunity to deliver solar thermal energy in the medium temperature range that is suitable for many industrial applications. However, the performance of single-phase thermosyphon in the medium temperature range has not been comprehensively investigated. This paper presents the design, development, and performance evaluation of a single-phase thermosyphon in an evacuated tube receiver integrated with a modified CPC solar collector. The thermohydraulic performance of the developed system is evaluated in the tropical climate using Therminol-55 oil as heat transfer fluid. The results demonstrate that the maximum outlet temperature reached over 120°C using thermal oil as heat transfer fluid while it remained at 100°C in case of water. The zero-loss thermal efficiency reached up to 70% on a clear sky day. Comparing the thermal performance of the developed CPC collector with an existing model of a non-concentrating collector showed much improvement at elevated temperatures. This indicates that this system can effectively operate in tropical weather conditions to provide sustainable solar thermal energy in the medium temperature range.
Introduction
The major portion of the global energy demand is supplied by hydrocarbon-based resources such as oil, gas, and coal which are non-renewable and limited in amount. The increased consumption of fossil fuels to meet the escalating energy demand is also a main contributor to environmental problems resulting in high CO2 emissions, increased carbon footprint and global warming. In this scenario, the World is looking for a clean and sustainable energy supply while rapidly pursuing development. Tapping the rich resources of renewable energies, especially solar energy, is a promising solution to reduce dependency on fossil fuels. Solar energy is the primary source of renewable energies and possesses desirable characteristics such as freely available, sustainable, inexhaustible and environment friendly. These attributes make solar energy more attractive to be explored.
Solar thermal collectors are used to collect and transform solar energy into heat energy. These are generally categorized as concentrating and non-concentrating types. Non-concentrating collectors such as flat plate and evacuated tube collectors are the most matured solar systems suitable for low-temperature applications (Ramaiah and Shekar, 2018), while concentrating collectors are used to delivering thermal energy in the medium-to-high temperature range (Gorjian et al., 2020). The concentrating collectors are further classified as imaging and non-imaging types (Shneishil, 2017). The conventional concentrating collectors, such as parabolic troughs, parabolic dishes, Linear Fresnel collectors, solar power towers, are all imaging type which essentially need complex and expensive tracking mechanisms to effectively collect and deliver the solar thermal energy (Pranesh et al., 2019). Moreover, large installations of such systems are required for an efficient operation (Xu et al., 2016), making them unsuitable for many small and medium-sized industrial applications operating in the medium temperature range. Industrial sector shares about 35% of the world’s total energy consumption and it is predicted to further increase in the future (Kumar et al., 2019). More than half of the total industrial energy demand is in low to medium temperatures (30–400°C) (Ramaiah and Shekar, 2018). Thermal loads of industrial processes and buildings’ air-conditioning make up major share of the global industrial energy consumption (Sagade et al., 2014). These systems mostly operate at moderate temperatures and their thermal energy demand can be provided by using suitable solar energy technologies. However, solar thermal systems for this temperature range are relatively underdeveloped. This indicates a great potential for further research to develop suitable technologies to meet the energy demands of such applications.
The evacuated tube solar collectors are commonly used for domestic hot water supply. Application of these collectors has seen exponential growth in the recent past, making up to 78% of all solar thermal installations in 2018 (Singh and Samsher, 2020). Integration of CPC with evacuated tube receivers (ETR) can produce solar thermal energy in the medium temperature range (Jiang et al., 2020), which is suitable for many applications such as process heat, boiler feedwater, desalination, wastewater treatment, solar drying, and solar heating/cooling applications. The non-imaging feature of the CPC collectors enables them to operate in stationary installation, thus eliminating complex tracking mechanisms (Tian et al., 2018). In addition, a large portion of diffuse radiations can also be collected by such collectors, thereby making them a preferred choice for tropical weather conditions where the proportion of diffuse radiations mostly remains high throughout the year. CPCs have been employed in many applications such as photovoltaic (PV), thermal collectors and hybrid systems (Tian et al., 2018). The concentration of solar radiation at a smaller absorber area increases the surface temperature, which leads to higher thermal losses in concentrating collectors. However, these thermal losses can be mitigated by employing ETR with the CPC. Conduction losses can be shirked by avoiding direct contact between the absorber and reflectors, while convection losses can be suppressed by creating a vacuum between the absorber and glass envelope. In addition, the application of selective coatings on the absorber surface can reduce radiation losses and increase the absorption of concentrated solar energy.
CPC collectors have many advantages over their counterparts for medium temperature heat supply (Gilani and Hoseinzadeh, 2021). In an experimental investigation (Pei et al., 2012) thermal performance of an evacuated tube solar water heater with and without a mini-CPC was compared. The solar water heater integrated with mini-CPC reflectors performed better than the one without CPC for a medium temperature output. In another study by Brunold et al. (1994), three different solar collectors, evacuated tube collector, a CPC vacuum tube collector, and a flat plate collector using glass capillary as transparent insulation, were tested for process heat applications. The CPC vacuum tube collector was found more lucrative for applications at a temperature higher than 150°C compared to the normal vacuum tube and flat plate collectors. Another study by Suman et al. (2015) concluded that the integration of evacuated tube receivers with CPC yielded more than 50% efficiency in the temperature range of 100–200°C. Li et al. (2014) studied the performance of CPC collectors integrated with the Dewar-type evacuated tube for a solar air-cooled absorption cooling system. However, another investigation (O’Gallagher, 2008) concluded that the thermal contact resistance arising from the direct contact between the absorber tube and inner tube in Dewar-type evacuated tubes substantially reduced the efficiency of the CPC collector. To eliminate this problem, Winston et al. (2014) introduced a CPC system that used a U-pipe with a selectively coated absorber tube. In this configuration, solar radiations concentrate on the absorber tube and are directly absorbed by the working fluid.
In another study, Karwa et al. (2015) studied the thermo-hydraulic performance of a CPC collector incorporating a U-tube absorber with fins. The receiver’s dimensions (tube diameter and fin size) were optimized to maximize the effective efficiency of the CPC system. Kim et al. (2008) demonstrated that the CPC system with a tracking mechanism produced a stable and 15% higher efficiency than a static CPC system. Although active systems achieve better thermal efficiency, the tracking mechanisms need expensive and complicated systems, which render them economically unattractive (Winston et al., 2014). Acuña et al. (2017) compared the performance of the CPC system with a concentric receiver tube and a simple receiver tube and concluded that the concentric tube receiver achieved approximately 10% higher efficiency and 4°C higher outlet temperature compared to simple tube receiver. However, the concentric tube receivers are more expensive to be manufactured and complex to be analysed. Li et al. (2013) tested a single pass evacuated tube collector with asymmetrical CPC at Shanghai Jiao Tong University, China. They reported that daily thermal efficiencies of 48–66% were achieved at the difference of about 59.6 and 3.4 K between the inlet and ambient temperatures, respectively, at a volume flow rate of 0.0077 m3/s. These research studies established the attractive prospect of CPC systems to extract solar energy at medium temperatures. However, all the mentioned studies used forced circulation of working fluids for transporting the collected heat from the collector to the storage.
Thermosyphoning as a method of circulating working fluid has been used in many applications, including passive solar water heating systems (Gálvez et al., 2010). Thermosyphoning operation makes the system self-sustainable, which may quite be helpful for remote areas. In solar thermosyphons, the heat transfer fluid is naturally circulated to the solar collector array without using a mechanical pump through differential pressure created between hot and cold sides induced by the difference in density between the return and advance side of the solar HTF. The schematic of a typical flow through a thermosyphon solar water heating system is shown in Figure 1. It is mainly comprised of evacuated tube receivers connected to a storage tank through riser and downcomer pipes. In the case of a closed-loop system, the storage tank also acts as a heat exchanger transferring heat from the primary circuit (i.e., from the HTF) to the secondary fluid.
During the last few decades, many research studies have been carried out to investigate the performance of thermosyphon-based water heating systems (Budihardjo et al., 2007; Kalogirou, 2009; Liu et al., 2012; Dobson and Ruppersberg, 2017). (Lu et al., 2017) developed numerical models based on heat and momentum balance equations to estimate the performance of a thermosyphon water heating system. The developed models were also validated by experimental testing of solar water heaters operating under natural circulation. In another study (Morrison and Braun, 1985), both simulation and experimental approaches were used to compare the performance of a thermosyphon-based water heating system with horizontal and vertical water tanks. The optimum performance was obtained for vertical tank configuration when the daily draw-of volume of water was equal to the collector volume. Performance of single-phase and two-phase thermosyphon-based evacuated tube solar water heaters was compared in (Chow et al., 2013) for the selected cities in China. The research study concluded that two-phase systems could provide relatively higher temperatures, but the single-phase collectors were better in terms of initial cost and economic payback. Yaman and Küçüka (2019), Yaman (2020) investigated the performance of a thermosyphon-based solar water heater using evacuated heat pipe receivers. The results demonstrated that the annual yield of the ETR coupled with CPC reflectors was more than double the yield of the ETR without CPC reflectors. Nkwetta et al. (2012) also observed 30 and 25.4% enhancement in the differential temperature and daily energy collection, respectively, using low concentration CPC reflectors with single side coated evacuated heat pipes.
Over the years, various designs of CPC collectors with diverse shapes of reflectors and absorbers have been presented and investigated by many researchers (Madala and Boehm, 2017; Pranesh et al., 2019). However, very limited literature is available on the performance investigation of CPC collectors in tropical climate conditions (Canaff and Ratismith, 2015; Ratismith et al., 2017; Ratismith et al., 2020) where the percentage of diffuse radiations mostly remains high and even reaches up to 60% in rainy seasons (Ratismith et al., 2014). Another under-researched area is the solar thermosyphon phenomenon in the ETR coupled with CPCs, which triggers the natural circulation of heat transfer fluid to transport the collected heat from the collector to the storage or directly to the point of application without any mechanical pumping requirement. The past literature survey reveals that thermosyphon has mainly been investigated in non-concentrating solar collectors such as flat plate and evacuated tube collectors. Moreover, the previous studies were limited to low-temperature ranges (<80°C). In addition, single-phase solar thermosyphon using water as the working fluid. The integration of ETR with CPC provides the opportunity to deliver medium-temperature solar thermal energy in sustainable manner which is rarely investigated in the past. Moreover, the natural circulation of HTF by the thermosyphon phenomenon eliminates the need for any moving components leading to simplification in the construction and operation of the system resulting in significant saving in the operational and maintenance costs.
This study presents an investigation of a single-phase thermosyphon in a newly designed CPC solar collector to supply solar thermal energy in a medium temperature range. The system comprises of evacuated tube receivers paired with modified compound parabolic troughs. Therminol-55 oil has been used as heat transfer fluid. The paper is subdivided into sections to describe the problem formulation, methodology, system design, performance evaluation under tropical weather conditions. The results obtained by experimental testing are presented and discussed in section Results and Discussion. Moreover, the experimental thermal efficiency is also compared with a previously developed model for a solar thermosyphon. The last section concludes the findings from this experimental investigation.
Methodology
The proposed medium-temperature solar collector consists of evacuated tube receivers integrated with non-imaging compound parabolic concentrators. The new design of the CPC consists of double parabolic segments, which are developed based on desired acceptance angle and evacuated tube receivers.
Evacuated Tube Receiver
A flow-through type solar vacuum tube (model JJR-T-2140) was used as a receiver. It comprises a metallic absorber tube made of Stainless Steel (SS 304/316) bounded in a high-strength Borosilicate glass envelope, as shown in Figure 2. Antireflective coating on the glass envelope is provided to reduce optical reflection losses and enhance the transmission of sunlight through the tube. The annulus space between the glass envelope and absorber tube is evacuated (3 × 10−4 Pa at 200°C) to avoid convection losses.
Aluminum Nitride selective coating is also applied at the absorber tube to improve absorption of solar energy and minimize infrared losses. In addition, special seals provided at the ends of the tube help maintain vacuum at elevated temperatures while expansion bellows accommodate uneven thermal expansion of metal and glass tubes. The detailed specifications of the ETR are given in Table 1.
Geometric Construction of the Modified CPC
A CPC is a non-imaging concentrating device that consists of two parabolic segments at its ends (Vu and Shin, 2016). The new double parabolic concentrator is made by joining two parabolas. The inner portions of parent parabolas are removed from the common point, making a cusp in the middle, as shown in Figure 3A. The outer portions are then tilted inward by 30° (which also defines the acceptance half-angle of the resulting CPC) to form a combination of two parabolic sections, as shown in Figure 3B.
The receiver tube is placed below the common focus point so that the axes of both parabolic segments are tangent to the inner metallic absorber tube, as illustrated in Figure 4A. A small V-shaped cavity is also formed at the cusp point to lower the receiver’s position further. After reflecting from the CPC reflectors, the incident solar radiations are directed to a region below the common focal point where the receiver is placed.
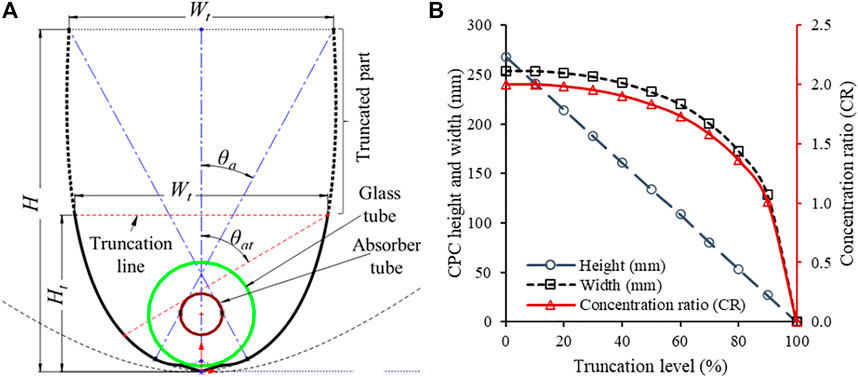
FIGURE 4. Truncation of the modified CPC (A) complete profile of the concentrator paired with ETR, (B) effect of truncation on geometric parameters.
As the upper part of reflectors become almost parallel to the common axis of CPC and thus contributes very little to the concentration of solar rays. This orientational issue provides the opportunity to remove upper portions, thereby saving material and manufacturing costs at the expense of a slight decrease in the collector’s aperture width and concentration ratio, as illustrated in Figure 4B. Furthermore, as a result of truncation, the view field of the receiver is also increased, allowing the direct solar radiations to reach the receiver for more time, even outside the acceptance angle of the CPC. In this way, the overall daily operating time of the collector is also increased. In the present study, truncation level was selected to be 50% which resulted in about 7.5% reduction in the aperture width and the concentration ratio, while reflectors height was reduced to half of the original height.
The detailed specifications of the developed CPC reflector are summarized in Table 2. In addition, details of the ray tracing analysis and optical performance of the designed CPC troughs can be seen in (Akhter et al., 2017).
The Experimental Setup
The 50% truncated CPC, which emerged as the suitable choice, were selected for manufacturing and experimental testing. The CPC collector module comprised a newly designed three double parabolic troughs paired with evacuated tube receivers, which were named East (E), Central (C), and West (W) troughs for comparison of output temperatures when placed in the north-south direction. The optical axes of side troughs were tilted outward by an angle of 15°, as shown in Figure 5, to increase the overall effective time of operation and smooth out the power output by maximizing solar energy collection in the forenoon as well as afternoon. The north-south arrangement of this configuration allows more than one trough to be active during the effective time of operation of the module. The central trough captures maximum radiations around noon. The outer troughs intercept more radiations in the forenoon and afternoon sessions when the incidence angle of solar radiations falls within the acceptance half angles of the outer troughs.
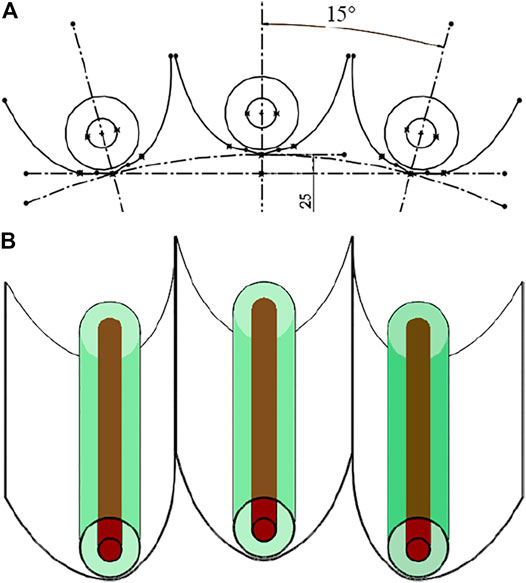
FIGURE 5. Arrangement of CPC troughs in module (A) end view of troughs assembly, (B) isometric view of CAD model.
The concentrating-reflecting surface of the CPC troughs was manufactured using highly reflecting sheets. The ETRs were installed in the focus line of the CPC trough. The experimental test rig consisting of three evacuated tube receivers paired with modified CPCs was installed to investigate the thermal performance of single-phase thermosyphon in the medium temperature range. The header pipe, connecting pipes, and storage tank were carefully insulated to curtail thermal losses. The temperature at the inlet and outlet of all tubes and the header pipe and storage tank were continuously monitored and recorded by installing resistance temperature detectors. A one-way valve was also installed at the inlet side to prevent the possibility of flow reversal during the night-time or low insolation period. The schematics diagram of the experimental test rig is illustrated in Figure 6A, while the CAD model is shown in Figure 6B.
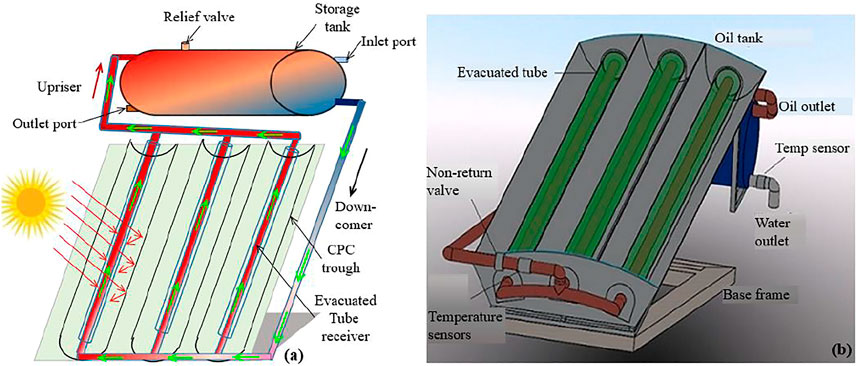
FIGURE 6. CPC troughs with evacuated tube receivers (A) schematic of the experimental setup, (B) CAD model of the complete test rig.
Experimental Procedure
The complete module of the CPC collector was fixed on an adjustable base frame and the measuring instruments were installed at designated locations, as demonstrated in Figure 7. All thermocouples and temperature sensors have been calibrated before installation in the test rig. Since the developed CPC collector was aimed to supply solar thermal energy in the medium temperature range, Therminol-55 oil was employed as a heat transfer fluid instead of water to avert phase-change inside the evacuated tube receivers at higher temperatures. The heat transfer oil was filled into the CPC, and the test rig was exposed to solar radiation for experimental testing. Temperatures at selected locations were continuously monitored and recorded at 60 s intervals. The data was stored by a multichannel data logger for postprocessing and analysis of the experimental results.
The fabricated system was installed at the Solar Thermal Advanced Research Center of the Universiti Teknologi PETRONAS, Malaysia (4.386°N, 100.979°E) for experimental testing under outdoor tropical weather conditions. The real-time weather data, including direct and global solar irradiance, and ambient temperature, were recorded during the experimental testing. The direct solar irradiance was measured using a pyrheliometer (EKO MS-56, EKO Instruments Co., Ltd. 1-21-8 Hatagaya, Shibuya-ku. Tokyo, 151-0072 Japan) installed on a dual-axis tracking platform (STR-21G). The global solar irradiance was measured by employing a first-class pyranometer (EKO MS-402, EKO Instruments Co., Ltd., 1-21-8 Hatagaya, Shibuya-ku. Tokyo, Japan) mounted on the mainframe in the plane of the aperture of the CPC collector. Finally, the temperatures at designated places were measured by resistance temperature detectors (PT-100) attached to a multichannel data logger (Graphtec GL-820, Totsuka-ku, Yokohama 244-8503, Japan). Details of the main measured parameters and the specifications of the related measuring instruments are given in Table 3. In addition, the energy absorbed by the CPC collector was determined, and the performance of the CPC collector was evaluated to determine the feasibility of a single-phase thermosyphon in the CPC collector in a tropical climate.
Since the outlet temperature was intended to be in the medium range, Therminol-55 oil was used as the working medium due to its high boiling temperature. The oil was filled in the storage tank of the collector until it reached a level slightly lower than the top of each absorber tube. The Therminol-55 oil level in the closed-loop was ensured by installed a level gauge in the oil storage tank. When the CPC collector was subjected to solar radiation, the heat collected by the metallic absorber tube was transferred to the heat transfer oil inside the absorber tube, causing it to expand. Due to a change in density caused by solar heat absorption, the oil started overflowing from the top of the absorber tubes into the header and onto the storage tank. In the meantime, the colder fluid was pushed down in the return line due to its relative density difference and gravity effect, leading to natural circulation called “solar thermosyphon”. The hot fluid flowing into the storage tank raised the temperature inside the tank. The heated thermal oil, in turn, reached the inlet of the receiver tube, forming a closed-loop system. Thus, the thermal oil temperature was continuously increased unless heat was extracted from the storage tank by circulating water in the secondary loop.
Mathematical Modeling
The overall thermal efficiency of the single-phase closed loop thermosyphon system is defined as the ratio of the heat gained by the primary fluid to the incident solar radiance on the aperture area of the solar collector, given by the following equation (Kalogirou, 2014);
Qu is the useful energy gain by heat transfer fluid, Gt is the total incident solar irradiance on the aperture of CPC, and Ac is the aperture area of the solar collector. The effective or total solar irradiance for a CPC is given by following equation (Kalogirou, 2014).
where Gb is direct or beam irradiance on aperture, Gd is diffuse solar irradiance and C is concentration ratio of the CPC.
In thermosyphon, flow is induced by a pressure difference created by density change which depends on the temperature gradient in the loop. For steady-state conditions, the total driving pressure head (due to buoyancy) is balanced by the frictional pressure head (determined based on Darcy’s equation). Assuming one-dimensional, steady and laminar flow, with linear temperature distribution along the tube, the momentum equation of the loop could be manipulated as under (Recalde et al., 2015):
where, ρ is the density (kg/m3); g is the gravity (m/s2); H is the vertical distance between the heat source and the heat sink (m); f is the coefficient of flow resistance; L total length of thermosyphon loop (m), d is tube diameter (m), A is the cross-sectional area of the tube (m2) and ṁ is the mass flow rate through the tube (kg/s). For small values of ΔT, the change in density between hot and cold fluids can be determined using Eq. 3 (Recalde et al., 2015).
where β is the normalized thermal expansion coefficient of the fluid. By substituting the density difference in the momentum balance, Eq. 3, the equation for mass flow rate can be written as below:
Useful heat gained by the working fluid is determined by Eq. 6.
where Cp is the specific heat capacity; ṁ is the mass flow rate; ΔT is the temperature difference of working fluid between the inlet and the outlet. Substituting the value of ṁ from Eq. 5, the energy equation for one dimensional single-phase flow can be written as below:
where βt is the tilt angle of the collector measured from a horizontal plane and the parameter B is given by the following equation:
Uncertainty Analysis
The accuracy of the experimental results is determined by estimating the uncertainty of the measuring parameters using the B-type method (Moffat, 1988; Chen et al., 2015). In the present experimental investigation, the major errors are related to solar irradiance measurements and temperatures. The errors in the collector area and specific heat capacity of the heat transfer fluid are assumed to be negligible for the determination of total uncertainty. The overall uncertainty of the system is estimated using the following equation.
where uG and uΔT are the possible errors in the measurement of solar irradiance and temperature, respectively.
In the present experimental study, the errors in the measurement of solar irradiance and temperature (together with datalogger) were found to be 1.7 and 1.5%, respectively. Thus, the maximum uncertainty in the experimentally determined thermal efficiency was 5.34%.
Results and Discussion
The experiment was repeated using thermal oil and water as heat transfer fluids. The results obtained by experimental measurements and findings of the investigations are presented and discussed in this section. In addition, measurements’ results obtained using water as heat transfer fluid are also presented and compared with thermal oil results. The last sub-section shows comparison with a previously investigated work on a non-concentrating solar collector.
Oil-Based Solar Thermosyphon
The variation in the temperatures at selected collector locations is plotted in Figures 8, 9 for two different testing days, day 1 and day 2. The effect of outward tilting of side troughs can be seen in the rising temperature trends for a south-facing collector. The truncation of top portions of the reflectors further increases the overall operating time by enabling the receivers to intercept direct radiations beyond the acceptance angles. The incidence angle of solar radiation affects the energy absorbed by the collector, as the oil temperature in the eastern tube started rising earlier in the morning.
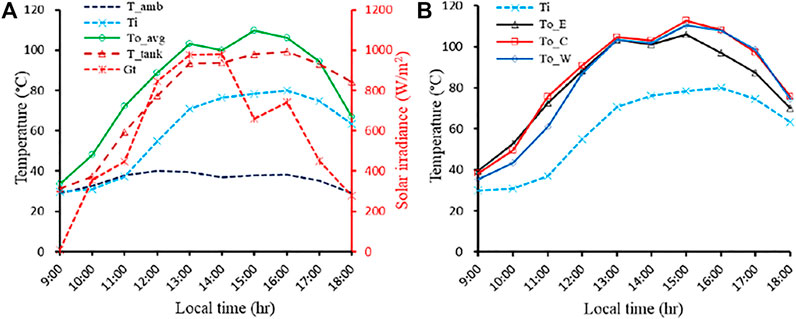
FIGURE 8. Variation of hourly averaged temperatures using thermal oil under actual weather conditions on day-1 (A) average temperatures and solar irradiance, (B) inlet and outlet temperatures of individual receiver tubes.
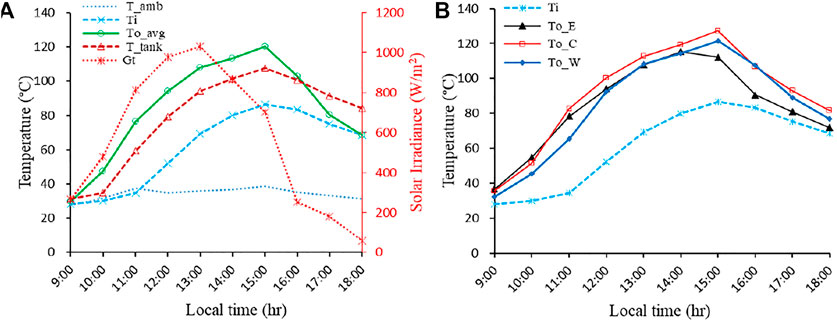
FIGURE 9. Variation of hourly averaged temperatures using thermal oil under actual weather conditions on day-2 (A) average temperatures and solar irradiance, (B) inlet and outlet temperatures of individual receiver tubes.
On both measurement days, the temperature in the central tube increased and reached the highest value near noon. Following this trend, the oil temperature in the western tube should have taken the lead in the afternoon. However, it remained slightly lower than the central tube due to a large decrease in solar radiation in the afternoon, typical in tropical regions. The average outlet temperature also dropped in the afternoon due to a large reduction in solar radiation. As a result, it became less than tank temperature, which could cause reverse flow in the evacuated tube receivers. However, flow reversal was avoided by employing a non-return valve in the return line. The maximum temperature achieved by the collector at the outlet was in the range of 115–120°C.
The maximum value of average and storage temperature is shifted from the middle of the day towards the afternoon due to the working fluid’s heat capacity and thermal inertia. As the thermal oil in the ETR reached up to a temperature of 90°C even on a day with low solar radiation, the data collected supported the hypothesis that the CPC collector can function effectively and deliver sustainable solar thermal energy at a medium temperature range under the tropical climate conditions.
The model presented by Kalogirou et al. (2013) for thermosyphon in a non-concentrating solar collector was modified to take into account the effect of concentration of solar radiation by including the aperture area of the developed CPC collector. The characteristic curve for thermal efficiency was determined using the experimental data collected during the selected days. Figure 10 shows the overall efficiency of the developed CPC solar collector operating in thermosyphon mode plotted against reduced mean fluid temperature (Tm*) given by following equation:
It can be observed that the zero-loss thermal efficiency of the developed collector, which represents the system optical efficiency, reached up to 70% during the test period. On the other hand, the thermal efficiency of the developed CPC solar collector slumped at higher inlet temperatures and low solar irradiance. The systems operating in thermosyphon mode are typical behaviour as the natural circulation of working fluid is quite low in these systems compared to the active mode (forced circulation).
Water-Based Single-phase Solar Thermosyphon
The developed CPC solar collector was also tested using water as a working fluid. Figure 11 shows the experimental results of weather parameters and temperatures at designated locations of the collector recorded on day 3. The weather was mostly sunny on the selected measurement days with an average solar irradiance of about 800 ± 20 W/m2 except for sometimes intermittent clouds, as illustrated in Figure 11A. The average outlet temperature of the water as working fluid reached the saturation temperature of water at atmospheric pressure at about noon. The maximum temperature in the tank reached 90°C, as an average of all testing days. The effect of tilting the side troughs is also evident in the case of water as a working fluid, as shown in Figure 11B. The outlet temperature of the eastern tube started rising earlier than the central tube, followed by the western receiver tube. Some fluctuations in outlet temperature of the western tube were observed after reaching the saturation limit of water which might be attributed to some reverse flow or boiling of the water.
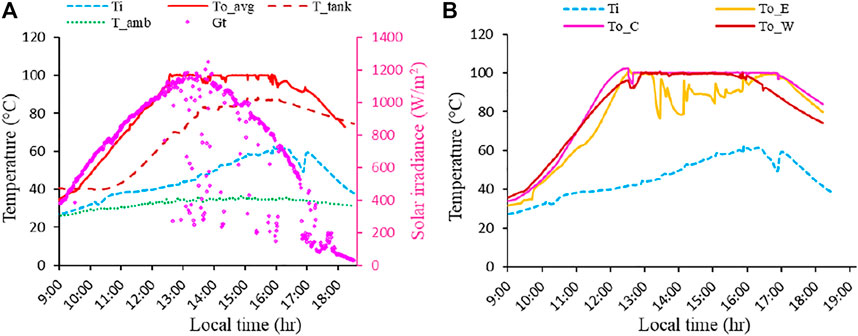
FIGURE 11. Variation of temperatures using water under actual weather conditions on day-3 (A) temperatures and solar irradiance, (B) inlet and outlet temperatures of individual receiver tubes.
The experimental results, using water as working fluid, demonstrate that phase change can occur in an evacuated tube receiver paired with CPC if used in thermosyphon mode at standard atmospheric pressure. This indicates that the loop should be pressurized if the water is used as the working fluid in a single-phase or some other heat transfer fluid with a higher boiling point (such as thermal oil) is suitable for a closed loop thermosyphon system operating in the medium temperature range.
Comparative Analysis of the Results
The experimental thermal efficiency of the ETR paired with modified CPC is also compared with the thermal efficiency estimated by using the model proposed by (Kalogirou et al., 2013) following EN12975 standard for testing solar collectors. Figure 12 presents the comparison of both efficiencies under tropical climate conditions. It is observed that the results are closely matching at lower inlet temperatures and higher solar irradiance. However, significant improvement in the experimentally determined thermal efficiency is achieved at higher inlet temperatures and low solar irradiance. This improvement in thermal efficiency is attributed to relatively higher differential temperatures between the inlet and outlet of the receiver, which is achieved due to the concentration of solar radiation by CPC reflectors.
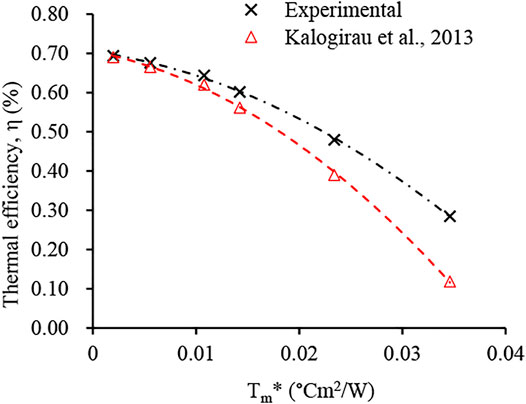
FIGURE 12. Comparison of the experimental thermal efficiency with a previously developed model efficiency.
Conclusion
This paper presents design, development, and experimental investigation of a single-phase thermosyphon in an evacuated tube receiver integrated with a modified CPC solar collector. A non-imaging CPC with 30° acceptance half-angle is designed with flow-through type evacuated tube receiver. An experimental test rig comprising of three troughs each having 50% truncated CPC reflectors with effective concentration ratio of 1.85 and ETR is fabricated. Thermohydraulic performance of the developed system is evaluated under tropical climate conditions. Therminol-55 oil and water is used as heat transfer fluid and the feasibility of single-phase thermosyphon in the medium temperature range is investigated. The experimental results show that the maximum outlet temperature reached over 120°C using thermal oil as HTF while it remained at 100°C in case of water. The zero-loss thermal efficiency reached 70% during the selected operating time on a clear sky day. Comparing the experimental efficiency of the developed CPC collector with an existing standard testing model shows good agreement at lower temperatures while significant improvement is achieved at higher temperatures. The results demonstrate that the proposed CPC collector can effectively function in tropical weather conditions. Moreover, single-phase thermosyphon is practicable to deliver solar thermal energy in the medium temperature range by employing CPC collectors. The feasibility of this system for a small/medium sized industrial application, operating in the medium temperature range, can be assessed by developing and testing a prototype comprising of scale-up model of the proposed system.
Data Availability Statement
The raw data supporting the conclusion of this article will be made available by the authors, without undue reservation.
Author Contributions
This paper presents design, development and experimental investigation of a single-phase thermosyphon using a CPC collector for medium temperature heat supply. JA drafted the manuscript along with experimentation, data collection and analysis. SG supervised and HA-K facilitated the experimental work. MA and MM reviewed the manuscript to improve the language and presentation of results. Dr. Barkaullah and FM helped in fabrication of test rig and experimental data collection while MAA performed data analysis and revision.
Conflict of Interest
The authors declare that the research was conducted in the absence of any commercial or financial relationships that could be construed as a potential conflict of interest.
Publisher’s Note
All claims expressed in this article are solely those of the authors and do not necessarily represent those of their affiliated organizations, or those of the publisher, the editors and the reviewers. Any product that may be evaluated in this article, or claim that may be made by its manufacturer, is not guaranteed or endorsed by the publisher.
Acknowledgments
The authors are grateful to the Universiti Teknologi PETRONAS, Malaysia, for supporting this research work through Universiti Internal Research Fund (URIF), cost center 015LB0-050.
References
Acuña, A., Velázquez, N., Sauceda, D., and Aguilar, A. (2017). Modeling, Construction, and Experimentation of a Compound Parabolic Concentrator with a Concentric Tube as the Absorber. J. Energ. Eng. 143 (3), 04016059. doi:10.1061/(ASCE)EY.1943-7897.0000416
Akhter, J., Gilani, S. I., Ali, M., and Gilani, S. Z. A. (2017). Design and Optical Modeling of a Low-Profile Stationary Concentrating Solar Collector for Medium Temperature Heat Supply. J. Telec., Electron. Comput. Eng. 10 (12), 65–71.
Azad Gilani, H., and Hoseinzadeh, S. (2021). Techno-economic Study of Compound Parabolic Collector in Solar Water Heating System in the Northern Hemisphere. Appl. Therm. Eng. 190, 116756. doi:10.1016/j.applthermaleng.2021.116756
Brunold, S., Frey, R., and Frei, U. (1994). “Comparison of Three Different Collectors for Process Heat Applications,” in Proc. SPIE 2255. Optic. Mater. Technol. Energ. Efficiency Sol. Energ. Convers. XIII, Freiburg, Germany, September 9, 1994 (Freiburg, Germany. doi:10.1117/12.185361
Budihardjo, I., Morrison, G. L., and Behnia, M. (2007). Natural Circulation Flow through Water-In-Glass Evacuated Tube Solar Collectors. Solar Energy 81, 1460–1472. doi:10.1016/j.solener.2007.03.002
Canaff, M., and Ratismith, W. (2015). “High Productivity Non-tracking Solar Collectors for Process Heat Applications,” in IEEE 15th Int. Conf. Environ. Elect. Eng. (EEEIC), Rome, Italy, June 10–13, 2015, 343–346. doi:10.1109/eeeic.2015.7165184
Chen, F., Li, M., Zhang, P., and Luo, X. (2015). Thermal Performance of a Novel Linear Cavity Absorber for Parabolic Trough Solar Concentrator. Energ. Convers. Manage. 90, 292–299. doi:10.1016/j.enconman.2014.11.034
Chow, T.-T., Bai, Y., Dong, Z., and Fong, K.-F. (2013). Selection between Single-phase and Two-phase Evacuated-Tube Solar Water Heaters in Different Climate Zones of China. Solar Energy 98, 265–274. doi:10.1016/j.solener.2013.10.011
Dobson, R. T., and Ruppersberg, J. C. (2017). Flow and Heat Transfer in a Closed Loop Thermosyphon. Part I - Theoretical Simulation. J. Energy South. Afr. 18 (4), 32–40. doi:10.17159/2413-3051/2007/v18i4a3389
Gálvez, J. B., Rodríguez, S. M., Delyannis, E., Belessiotis, V. G., Bhattacharya, S. C., and Kumar, S. (2010). Solar Energy Conversion and Photoenergy Systems: Thermal Systems and Desalination Plants-Volume I. Paris, France: EOLSS Publications.
Gorjian, S., Ebadi, H., Calise, F., Shukla, A., and Ingrao, C. (2020). A Review on Recent Advancements in Performance Enhancement Techniques for Low-Temperature Solar Collectors. Energ. Convers. Manage. 222, 113246. doi:10.1016/j.enconman.2020.113246
Jiang, C., Yu, L., Yang, S., Li, K., Wang, J., Lund, P. D., et al. (2020). A Review of the Compound Parabolic Concentrator (CPC) with a Tubular Absorber. Energies 13 (3), 695. doi:10.3390/en13030695
Kalogirou, S. A., Panayiotou, G. P., Florides, G. A., Roditis, G., Katsellis, N., Constantinou, A., et al. (2013). “Investigation of the Parameters Affecting the Thermosiphonic Phenomenon in Solar Water Heaters,” in World Renew. Energ. Congress 2013, Int. Conf. Renew. Energ. Sustain. Develop. Decarbon (Perth, Western Australia: Murdoch University).
Kalogirou, S. (2014). Solar Energy Engineering: Processes and Systems. The Boulevard, Langford Lane, Kidlington, Oxford OX5 1GB, UK, 225 Wyman Street, Waltham, MA 02451, USA: Academic Press.
Kalogirou, S. (2009). Thermal Performance, Economic and Environmental Life Cycle Analysis of Thermosiphon Solar Water Heaters. Solar Energy 83 (1), 39–48. doi:10.1016/j.solener.2008.06.005
Karwa, N., Jiang, L., Winston, R., and Rosengarten, G. (2015). Receiver Shape Optimization for Maximizing Medium Temperature CPC Collector Efficiency. Solar Energy 122, 529–546. doi:10.1016/j.solener.2015.08.039
Kim, Y., Han, G., and Seo, T. (2008). An Evaluation on thermal Performance of CPC Solar Collector. Int. Commun. Heat Mass Transfer 35 (4), 446–457. doi:10.1016/j.icheatmasstransfer.2007.09.007
Kumar, L., Hasanuzzaman, M., and Rahim, N. A. (2019). Global Advancement of Solar thermal Energy Technologies for Industrial Process Heat and its Future Prospects: A Review. Energ. Convers. Manage. 195, 885–908. doi:10.1016/j.enconman.2019.05.081
Kumar Singh, A., and Samsher, (2020). Analytical Study of Evacuated Annulus Tube Collector Assisted Solar Desaltification System: A Review. Solar Energy 207, 1404–1426. doi:10.1016/j.solener.2020.07.097
Li, X., Dai, Y. J., Li, Y., and Wang, R. Z. (2013). Performance Investigation on a Novel Single-Pass Evacuated Tube with a Symmetrical Compound Parabolic Concentrator. Solar Energy 98, 275–289. doi:10.1016/j.solener.2013.10.015
Li, Z., Ye, X., and Liu, J. (2014). Performance Analysis of Solar Air Cooled Double Effect LiBr/H2O Absorption Cooling System in Subtropical City. Energ. Convers. Manage. 85, 302–312. doi:10.1016/j.enconman.2014.05.095
Liu, Y.-M., Chung, K.-M., Chang, K.-C., and Lee, T.-S. (2012). Performance of Thermosyphon Solar Water Heaters in Series. Energies 5 (12), 3266–3278. doi:10.3390/en5093266
Lu, L., Wang, X., Wang, S., and Liu, X. (2017). Optimal Structure Design of a Thermosyphon Solar Water Heating System with Thermal and Dynamic Models. Heat Transfer Eng. 39 (16), 1470–1481. doi:10.1080/01457632.2017.1379347
Madala, S., and Boehm, R. F. (2017). A Review of Nonimaging Solar Concentrators for Stationary and Passive Tracking Applications. Renew. Sustain. Energ. Rev. 71, 309–322. doi:10.1016/j.rser.2016.12.058
Moffat, R. J. (1988). Describing the Uncertainties in Experimental Results. Exp. Therm. Fluid Sci. 1 (1), 3–17. doi:10.1016/0894-1777(88)90043-X
Morrison, G. L., and Braun, J. E. (1985). System Modeling and Operation Characteristics of Thermosyphon Solar Water Heaters. Solar Energy 34 (4/5), 389–405. doi:10.1016/0038-092x(85)90051-9
Nkwetta, D. N., Smyth, M., Zacharopoulos, A., and Hyde, T. (2012). In-door Experimental Analysis of Concentrated and Non-concentrated Evacuated Tube Heat Pipe Collectors for Medium Temperature Applications. Energy and Buildings 47, 674–681. doi:10.1016/j.enbuild.2012.01.010
O’Gallagher, J. J. (2008). Nonimaging Optics in Solar Energy. San Rafael, CA: Synthesis Lectures on Energy and the Environment, Technology, 2, 1-120, Morgan & Claypool Publishers. doi:10.2200/S00120ED1V01Y200807EGY002
Pei, G., Li, G., Zhou, X., Ji, J., and Su, Y. (2012). Comparative Experimental Analysis of the Thermal Performance of Evacuated Tube Solar Water Heater Systems with and without a Mini-Compound Parabolic Concentrating (CPC) Reflector(C<1). Energies 5 (12), 911–924. doi:10.3390/en5040911
Pranesh, V., Velraj, R., Christopher, S., and Kumaresan, V. (2019). A 50 Year Review of Basic and Applied Research in Compound Parabolic Concentrating Solar thermal Collector for Domestic and Industrial Applications. Solar Energy 187, 293–340. doi:10.1016/j.solener.2019.04.056
Ramaiah, R., and Shashi Shekar, K. S. (2018). Solar Thermal Energy Utilization for Medium Temperature Industrial Process Heat Applications - A Review. IOP Conf. Ser. Mater. Sci. Eng. 376, 012035. doi:10.1088/1757-899x/376/1/012035
Ratismith, W., Ali, D., and Briggs, J. S. (2020). A Non‐tracking Semi‐circular Trough Solar Concentrator. Int. J. Energ. Res 45, 3185–3199. doi:10.1002/er.6013
Ratismith, W., Favre, Y., Canaff, M., and Briggs, J. (2017). A Non-tracking Concentrating Collector for Solar thermal Applications. Appl. Energ. 200, 39–46. doi:10.1016/j.apenergy.2017.05.044
Ratismith, W., Inthongkhum, A., and Briggs, J. (2014). Two Non-tracking Solar Collectors: Design Criteria and Performance Analysis. Appl. Energ. 131, 201–210. doi:10.1016/j.apenergy.2014.06.030
Recalde, C., Cisneros, C., Avila, C., Logroño, W., and Recalde, M. (2015). Single Phase Natural Circulation Flow through Solar Evacuated Tubes Collectors on the Equatorial Zone. Energ. Proced. 75, 467–472. doi:10.1016/j.egypro.2015.07.424
Sagade, M. A. A., Shinde, N. N., and Patil, P. S. (2014). Effect of Receiver Temperature on Performance Evaluation of Silver Coated Selective Surface Compound Parabolic Reflector with Top Glass Cover. Energ. Proced. 48, 212–222. doi:10.1016/j.egypro.2014.02.026
Shneishil, A. H. (2017). Optical Design of Two Dimension Compound Parabolic Solar thermal. Int. J. Sci. Eng. Technol. 6 (1), 29–34.
Suman, S., Khan, M. K., and Pathak, M. (2015). Performance Enhancement of Solar Collectors-A Review. Renew. Sustain. Energ. Rev. 49, 192–210. doi:10.1016/j.rser.2015.04.087
Tian, M., Su, Y., Zheng, H., Pei, G., Li, G., and Riffat, S. (2018). A Review on the Recent Research Progress in the Compound Parabolic Concentrator (CPC) for Solar Energy Applications. Renew. Sustain. Energ. Rev. 82, 1272–1296. doi:10.1016/j.rser.2017.09.050
Vu, N., and Shin, S. (2016). A Concentrator Photovoltaic System Based on a Combination of Prism-Compound Parabolic Concentrators. Energies 9 (8), 645. doi:10.3390/en9080645
Winston, R., Jiang, L., and Widyolar, B. (2014). Performance of a 23kW Solar thermal Cooling System Employing a Double Effect Absorption Chiller and Thermodynamically Efficient Non-tracking Concentrators. Energ. Proced. 48, 1036–1046. doi:10.1016/j.egypro.2014.02.118
Xu, X., Vignarooban, K., Xu, B., Hsu, K., and Kannan, A. M. (2016). Prospects and Problems of Concentrating Solar Power Technologies for Power Generation in the Desert Regions. Renew. Sustain. Energ. Rev. 53, 1106–1131. doi:10.1016/j.rser.2015.09.015
Yaman, P. (2020). Design and Performance Evaluation of a Compound Parabolic Collector. Istanbul, Turkey: Yildiz Technical University. Master of Science in Mechanical Engineering.
Yaman, P., and Küçüka, S. (2019). “Annual Optical Performance Comparison of Compound Parabolic Concentrator and Traditional Vacuum Tube,” in 8th Solar Energy Systems Symposium and Exhibitio (Tukey: Mersin).
Glossary
AlN Aluminium Nitride
CPC Compound parabolic concentrator
CR Concentration ratio
ETR Evacuated tube receiver
HTF Heat transfer fluid
N-S North-south
RTD Resistance temperature detector
Symbols
A Area (m2)
C Central/concentration ratio
d Diameter of absorber tube (m)
D Diameter of glass tube (m)
E East
G Solar irradiance (W/m2)
f coefficient of flow resistance
g acceleration due to gravity (m/s2)
H Height of collector (m)
L Length of collector/receiver (m)
ṁ Mass flow rate (kg/s)
Q Heat gain (kJ)
T Temperature (°C)
Tm* Reduced fluid mean temperature (°C)
W Width (m)/West
Greek symbols
α Absorptivity
β Coefficient of fluid expansion/tilt angle
∆ Increment/difference
η Efficiency (%)
θ Acceptance half-angle (°)
ρ Reflectivity/density (kg/m3)
τ Transmissivity
Subscripts
a Acceptance
abs Absorber
apr Aperture
amb ambient
b Beam
c Collector/cover
d Diffuse
f Fluid
g Geometric
i inlet/incident
o outlet/optical
t Tilt/truncated
u Useful
Keywords: evacuated tube receiver, compound parabolic concentrator, concentration ratio, concentrated solar collector, single-phase thermosyphon
Citation: Akhter J, Gilani SI, Al-Kayiem HH, Mehmood M, Ali M, Ullah B, Alam MA and Masood F (2021) Experimental Investigation of a Medium Temperature Single-Phase Thermosyphon in an Evacuated Tube Receiver Coupled With Compound Parabolic Concentrator. Front. Energy Res. 9:754546. doi: 10.3389/fenrg.2021.754546
Received: 06 August 2021; Accepted: 22 October 2021;
Published: 19 November 2021.
Edited by:
Ibrahim Halil Yilmaz, Adana Alparslan Turkes Science and Technology University, TurkeyReviewed by:
Aniruddha Pandit, Institute of Chemical Technology, IndiaMurat Kunelbayev, MES Republic of Kazakhstan, Kazakhstan
Copyright © 2021 Akhter, Gilani, Al-Kayiem, Mehmood, Ali, Ullah, Alam and Masood. This is an open-access article distributed under the terms of the Creative Commons Attribution License (CC BY). The use, distribution or reproduction in other forums is permitted, provided the original author(s) and the copyright owner(s) are credited and that the original publication in this journal is cited, in accordance with accepted academic practice. No use, distribution or reproduction is permitted which does not comply with these terms.
*Correspondence: Javed Akhter, amF2ZWRha2h0ZXIudWV0QGdtYWlsLmNvbQ==