- 1Federal Aviation Administration, Washington, DC, United States
- 2Department of Mechanical Science and Engineering, University of Illinois Urbana-Champaign, Champaign, IL, United States
This study outlines an effort to report on the physicochemical variability of Sustainable Aviation Fuels (SAF), leveraging data from the FAA Alternative Jet Fuels Test Database (AJFTD). The AJFTD, containing fuel sample records of conventional and sustainable aviation fuels to date, was developed by the PIs through the FAA Center of Excellence (ASCENT). With the development of SAF from various feedstocks and processing methods, new approval processes have been developed to accommodate the changing jet fuel landscape. To control for these differences, approval procedures were designed as each new fuel category came through the development pipeline. However, recent studies have suggested that rather than feedstock or processing method, chemical properties and fuel performance can be accurately judged by considering fuel composition characteristics such as carbon chain length, hydrocarbon class, and branching level. To quantify the variability present in recently approved jet fuels, this paper evaluates physicochemical property variability and provides relevant thermophysical property relations for conventional and alternative jet fuels with a discussion of efforts to streamline approval, reducing the time and cost of bringing new SAF to future markets. Findings from this study show that the variability in the composition and properties of SAF as compared to conventional fuels is small enough such that they still satisfy specification requirements outlined by the American Society for Testing and Materials (ASTM) D7566 standards for aviation fuels containing synthesized hydrocarbons.
1 Introduction
The International Civil Aviation Organization (ICAO) defines Alternative Jet Fuels (AJF) as any fuel derived from an unconventional (non-petroleum based) feedstock (CAAFI, 2022). In the past decade, significant strides have been made in developing newer, more optimal production pathways for these fuels. Sustainable Aviation Fuels (SAF) are a subset of AJF that have gained popularity in recent years in response to aggressive global goals in de-carbonizing the aviation industry (CAAFI, 2022). SAF production pathways can be broadly categorized by processing method and include Fischer-Tropsch (FT), Hydroprocessed Esters and Fatty Acids (HEFA) [previously known as Hydrotreated Renewable Jet (HRJ)], Synthesized Iso-Paraffins (SIP), Synthetic Kerosene with Aromatics (SKA), Alcohol to Jet (ATJ), Catalytic Hydrothermolysis (CHJ), and Hydrocarbon Hydroprocessed Esters and Fatty Acids (HC-HEFA). In September 2021, the White House published a new Sustainable Aviation Fuel Grand Challenge, a plan outlining a multi-modal approach to increase annual SAF production to at least 3 billion gallons by 2030 (FACT SHEET, 2021). The push to develop these fuels can be attributed to several key drivers, namely: economic incentives, energy security, and environmental considerations. With high price volatility in the global fuel market, fuel producers and customers want to secure predictable fuel sources independent of external suppliers, additionally supporting domestic skilled job growth. In recent years, jet fuel price volatility has skyrocketed, with fuel prices rising by more than 70% in 2021 alone (Jet Fuel Price Surge is Clouding U.S. Airlines’ Recovery Plans, 2021). The second key driver for SAF development, energy security, is reflected in US government mandates that set clear requirements for various military branches to secure alternative energy sources, with the Navy goal of 50% alternative energy for its fleet by 2020 (GreenFleet, 2010). The national government realizes the advantage in having secure domestic energy resources, not subject to international conflicts, which can cause supply disruptions and further challenges. Finally, some SAF have the potential to provide environmental benefits, namely in the reduction of fossil fuel usage and greenhouse gas/particulate emissions levels. As shown in a recent report, conventional jet fuel blending with HEFA in a 50:50 (by volume) blend reduces particulate emissions from aircraft at cruise conditions by greater than half and up to 70% (Moore et al., 2017). Fuel producers seek to develop “drop-in” SAF that can be blended with conventional fuels and used in current engine architectures. This reduces switching costs of having to update engine systems, fuel lines, storage facilities, and delivery equipment while minimizing risks associated with keeping incompatible fuels and systems separate. Current SAF specifications set maximum blending limits to ensure compatibility in operating systems. Before obtaining blending approval, new SAF must undergo rigorous testing from property evaluation to rig and engine testing, which is cost and time intensive for producers, discouraging greater SAF development. The extensive fuel database maintained by the Air Force Research Laboratory (AFRL) provided much of the needed fuel property data utilized for this study and is available through the Alternative Jet Fuel Test Database (AJFTD) maintained at the University of Illinois at Urbana-Champaign (altjetfuels.illinois.edu). This is in collaboration with the National Jet Fuel Combustion Program and supported by the FAA Center of Excellence for Alternative Jet Fuels and Environment (ASCENT) research program, funded by the FAA, NASA, Department of Defense, Transport Canada, and the EPA. Much of the work in the SAF landscape concerns feedstock and fuel production, life cycle economic and environmental analyses, and end use emissions and noise studies. In safely and efficiently integrating SAF produced from broadly varied feedstocks, understanding the relations between fuel composition and combustion performance and fuel physical/chemical properties is of utmost concern and has lacked significant study. The following sections of this paper will address this area through a statistical analysis of the variability present in jet fuels for key fuel properties preceded by a discussion of the SAF certification process.
2 Methodology
In this manuscript, a statistical analysis of jet fuel variability for certification-relevant properties is conducted through the synthesis of multiple data sources for conventional and alternative jet fuel data. In Section 3, this manuscript outlines current jet fuel specifications, production pathways, and quantification of compositional variation in SAF. Also presented is a detailed description of the University of Illinois’ AJFTD, the main data source for the alternative fuels sampled in Section 3 of this work. Section 4 delivers statistical regression models which outline expected variability in properties of SAF compared to conventional fuel samples. The authors synthesize results from D4054 Fit-For-Purpose properties reports and the 2006 Coordinating Research Council’s (CRC) World Fuel Survey (WFS) report to obtain quantitative values for the deviation of various SAF across select properties. This is done by comparing regressions for speed of sound, viscosity, density, and specific heat via a t-test. Subsequently, a regression demonstrating the range of expected temperature dependent property values for various SAF is provided for select properties. Due to the fact that they are ASTM regulated properties for jet fuel specifications, viscosity at −40°C and −20°C and density at 15°C are selected for regression analysis. A 95% confidence interval is then constructed around the fits to encompass a range of likely expected values for each fuel type and the associated equations are tabulated. Similar relations for specific heat, isentropic bulk modulus, surface tension, speed of sound and thermal conductivity are provided in the Supplementary Materials. Finally, this study provides a discussion of this expected variability for SAF given the largest dataset available and how it may ultimately relate to the certification of such fuels. The final section in this manuscript outlines future routes to streamline the approval pipeline while advancing fuel property prediction techniques to address ongoing challenges for SAF.
All computational work documented in this manuscript was conducted at the University of Illinois Urbana-Champaign Mechanical Engineering Department. No experimental work was conducted by the authors of this manuscript in the development of the datasets for statistical analysis. Rather, the datasets were acquired through sampling fuel property and composition test data from the D4054 Fit-For-Purpose properties reports, the CRC-WFS report and the University of Illinois’ AJFTD. All data sources are available online and can be accessed to replicate procedures undertaken in this study.
3 Sustainable aviation fuels approval process
3.1 Specification development
The current jet fuel approval process for civil aviation in the United States is governed by three key American Society for Testing and Materials (ASTM) standard specifications: D1655, D7566, and D4054. D1655, Standard Specification for Aviation Turbine Fuels, defines aviation fuel types that meet operating requirements for aircraft and engines. This covers the class of kerosene-type jet fuels considered Jet A and Jet A-1, which differ in their freezing point maximum requirements, −40°C and −47°C, respectively, with Jet A being used predominantly in the United States (ASTM Standard D1655, 2018). Excluded from this study, wider cut naphtha-type Jet B is detailed in D6615. For SAF, D7566, Standard Specification for Aviation Turbine Fuel Containing Synthesized Hydrocarbons, details fuel property requirements that must be met before the fuel can be blended with D1655 approved fuels (ASTM Standard D7566, 2018). These blends effectively become D1655 approved drop-in fuels. Finally, D4054, Standard Practice for Qualification and Approval of New Aviation Turbine Fuels and Fuel Additives, guides novel fuel and fuel additive qualification and approval for use in aviation engines (ASTM Standard D4054, 2016). Similar fuel specifications exist for fuels outside of the United States such as the UK Defense Standard DEF STAN 91–91, but are excluded from the scope of this paper. Additional military specifications that govern the approval of fuels for military use will not be discussed in further detail but include MIL-DTL-83133 (JP-8 and F24) and MIL-DTL-5624 (JP-5).
As mentioned previously, fuel producers seek to develop drop-in SAF to ensure compatibility with existing engine architecture and mitigate risks of cross-fuel contamination that could pose significant hazards for aircraft engine systems designed to operate with fuels meeting specific operability requirements (Wilson et al., 2013). Drop-in fuels provide the assurance that these fuels will not result in operating hazards or deterioration of aircraft fuel and engine systems. The development of fuel specifications involves stakeholders from various groups in the aviation fuel sector including government, academia, and OEMs. These specifications then determine whether a fuel will be considered as a drop-in fuel for use in blending with conventional fuels. Flight tests over recent decades of alternative fuels blended with conventional jet fuel have affirmed the drop-in viability of these fuels (Blakey et al., 2885). The following flowchart of Figure 1 summarizes the multi-step approval process by which new fuels are approved and annexed in ASTM D7566 that begins with various testing leading to the ASTM research report submitted for review prior to final approval and specification incorporation.
The most recently approved SAF production pathway is IHI Corporation’s HC-HEFA fuel. This pathway was the first to be approved via the D4054 Fast Track approval process. In September 2020, ASTM D4054 was appended to include a fourth Annex A4 which permits reduced testing requirements for fuels falling within standard composition and property ranges similar to conventional fuels (Rumizen, 2021). Any fuel approved through the D4054 Fast Track process is strictly limited to a 10% blend limit with conventional fuel (Fuel Qualification, 2022). Currently, SIP and HC-HEFA are the only two D7566 Annexed fuels limited to a 10% blend ratio. Recent efforts are being made by governmental offices and research organizations across the country to determine what is required to increase the allowable blending limits, such as potentially considering mixing approved blend components with one another in order to achieve a fuel which lies within acceptable composition and property limits, with the eventual goal of transitioning to 100% SAF.
3.2 American society for testing and materials approved production pathways for sustainable blend stocks
ASTM D7566-21, the most recent revision, lists seven approved SAF: FT, HEFA, SIP, SKA, ATJ, CHJ, and HC-HEFA. These fuels differ in feedstock and processing methods shown graphically in Figure 2. The first fuel pathway to be annexed under D7566, FT fuels utilize fossil fuel feedstocks such as coal and natural gas that undergo gasification and reforming processes to produce a synthesis gas (syngas) (Edwards, 2017). Paraffins and olefins are then derived from this syngas via the Fischer-Tropsch process, which are then processed via hydrotreating, hydrocracking, or hydroisomerization and additional refinery processes. Generally, FT fuels contain normal (n-) and branched (iso-) alkanes with little to no heteroatoms or aromatics. The second group, HEFA, has feedstocks which may include renewable lipid sources such as animal tallow or plant oils. These fuels are produced via hydroprocessing of mono-, di-, and triglycerides, fatty acid esters, and free fatty acids to remove oxygen (Gutiérrez-Antonio et al., 2017). Additional processing steps, similar to FT fuels, include hydrocracking, hydroisomerization, isomerization, fractionation, or a combination of these processes and other refinery processes. SIP type fuels cover those fuels produced from hydroprocessing of fermented sugars recovered from renewable sources such as sugar cane through hydrolysis. The SKA category of fuels is also termed SPK/A, FT Synthesized Paraffinic Kerosene plus Aromatics, and is different from the first classification of FT fuels as SKA fuels have aromatics deliberately added to increase the aromatics content, an important factor in ensuring the proper volume swell of elastomer seals utilized in current in fuel systems (Moses, 2015a). This is achieved via alkylation of non-petroleum derived light aromatics, such as benzene, with FT derived olefins. The fifth class of SAF is ATJ, produced by fermenting sugars obtained through hydrolysis to obtain alcohol that is then processed through dehydration, oligomerization, hydrogenation, and fractionation steps (Edwards et al., 2014). Catalytic Hydrothermolysis, referred to as either CH-SK or CHJ, is the sixth fuel class which is derived from fatty acids and triglyceride-based feedstocks like soybean and tung oil. Extracted lipids are subjected to high pressures and temperatures through hydrothermal liquefaction followed by a final fractionation step (Eswaran et al., 2021). The final and most recent pathway, HC-HEFA, is similar to the process for developing HEFA but differs in that the hydrocarbon feedstock is specifically bio-derived from Botryococcus braunii, an oil-rich micro-algae (Goh et al., 2022).
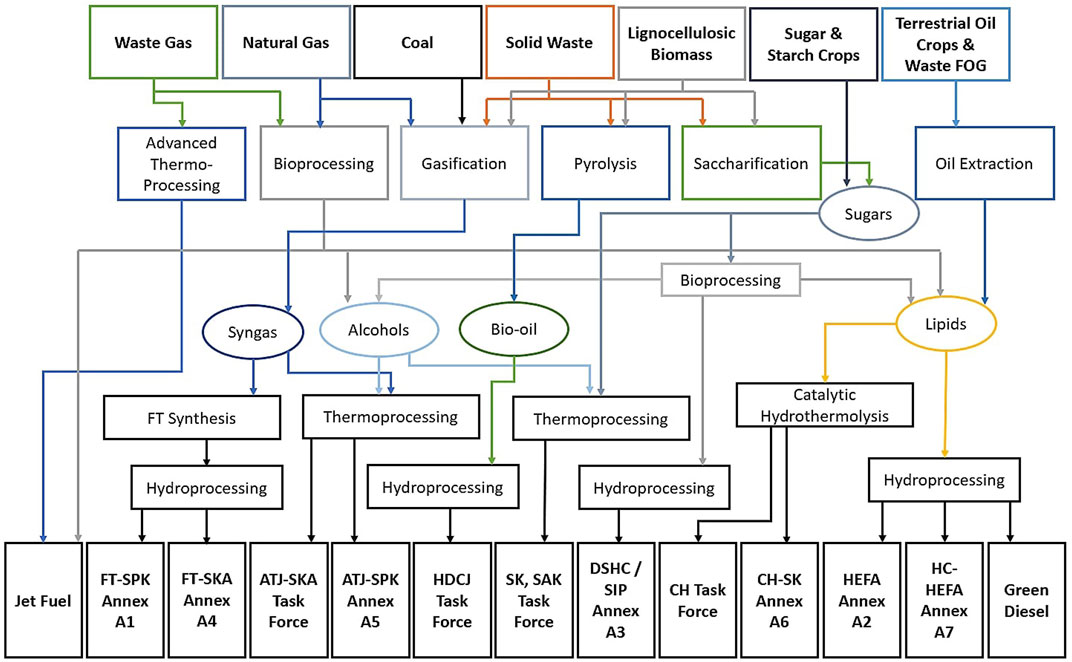
FIGURE 2. SAF pathways [adapted from Brown, Iowa State, 2012 and T. Edwards, USAF/AFRL—ASTM D7566 Task Forces].
3.3 Alternative Jet Fuels Test Database
With the immense global diversification of SAF in the past decade, a need has risen for a common data sharing platform for storing and reporting information surrounding the composition, properties, and use of these fuels. Such a platform supports a high-level understanding of the current state of airline fuel trends such that obstacles to the development of the SAF industry can be identified, and improvements can be made to optimize widescale SAF adoption. The AJFTD satisfies this need, containing a vast library of testing data for commercial and sustainable aviation fuels. This database was built for Project 33 of ASCENT (https://ascent.aero), a national research coalition committed to addressing sustainability and environmental concerns in the commercial aviation sector. The database’s features a library of over 25,000 fuel samples of local and international origin. Figure 3 outlines the database’s content structure. The database contains representative fuels from the seven Annexes of the ASTM D7566, Standard Specifications for Aviation Turbine Fuels Containing Synthesized Hydrocarbons. Document data categories include aviation emissions, chemical kinetics mechanisms, experimental testing results, and literature publications. Further, a diverse pool of manufacturers producing these Annexed fuels is also represented, from conventional fuel producers like Shell to SAF-exclusive producers like Gevo. All data was retrieved through close collaboration with the National Jet Fuel Combustion Program (NJFCP), Metron Aviation, Naval Air Systems Command (NAVAIR), Air Force Research Laboratory (AFRL), and the European JETSCREEN research program. The JETSCREEN program, short for Jet Fuel SCREENing and Optimization, aimed to developed screening tools for fuel OEMs which could assist them in assessing the potential viability of new fuels while still in the low-volume generation phase of development. This effort was centered around mapping fuel composition to properties and improving property-predictive models which could estimate the likelihood of a fuel falling within the acceptable property range required for certification. Fuel data collected for this project was shared continuously with the AJFTD through an online platform up until project completion in October 2020 (Edwards et al., 2012a).
In addition to searching for fuel-specific composition and property data, users can utilize interactive analysis capabilities such as the compare function for assessing composition or properties of two or more fuels side by side. Figure 4 depicts the viewing scheme for a sample two-dimensional gas chromatograph (GCxGC), available for select fuels. User-interactive functionalities such as these are in continued development on the AJFTD website.
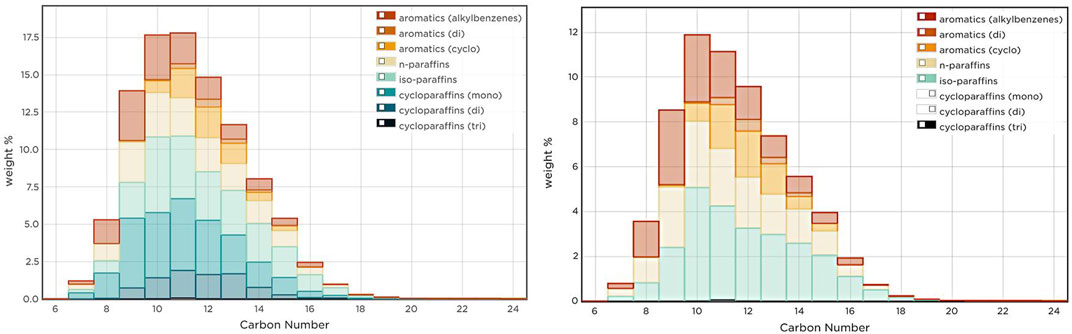
FIGURE 4. Sample fuel data from AJFTD website; view GCxGC data in an interactive categorized bar chart format.
Ongoing data collection and incorporation into the database supports continued development of machine learning data analysis techniques and applications to SAF research. Figure 5 outlines one such example application, a machine learning algorithm built to detect novel fuels in large datasets. Deep autoencoders are utilized in this application to extract hidden features from a data point—such as the GCxGC composition of a new candidate SAF—and compare it to a larger dataset—such as that of conventional aviation fuels—in order to better characterize how different this new fuel is from the pre-existing ones. Other applications include leveraging deep learning algorithms for missing data imputation, which is particularly relevant in the fuels data-scape as fuel property metadata can be inconsistent depending on where it was acquired, leading to missing data or data sparsity. Novel developments in machine learning techniques such as these ones and applications to jet fuel research can provide AJFTD users with more advanced data analysis tools for engaging with the extensive amount of data available to them online. For clarity, the authors would like to note that this manuscript does not attempt to provide a thorough review of such methods, nor does it propose novel machine learning models for assessing physicochemical variability in SAF; however, the authors have chosen to mention the increasing frequency of deployment of such models to underscore the importance big data is beginning to play in the alternative jet fuel research and integration process.
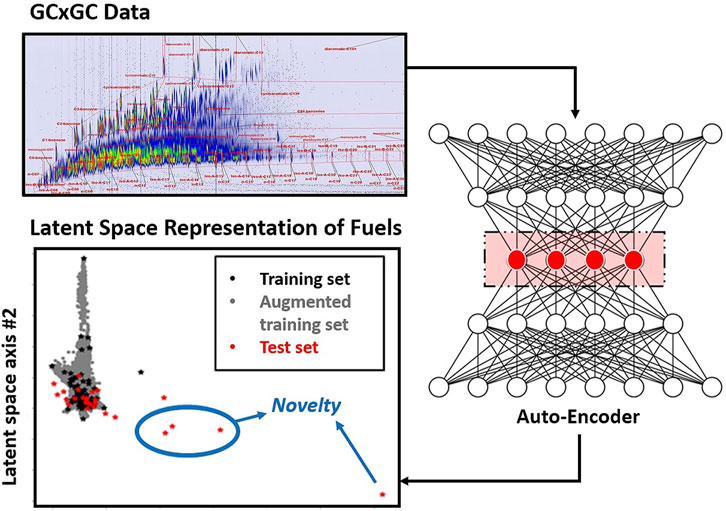
FIGURE 5. Deep autoencoders (lower left) used to detect novelty compositions from GCxGC (upper left); a proposed deep-learning-based framework for screening SAF (right).
The AJFTD outlines a comprehensive overview of archived and recently acquired aviation fuel test data. It is supported by a number of sponsors, including the FAA and NASA. Table 1 contains general summary statistics quantifying the database’s content and relevance across various categories.
Current users represent an international and occupationally diverse group. This includes students, post-doctoral researchers, industry employees, members of environmental organizations, national laboratories and governmental offices. Members employed in more than seven different regions internationally are represented, including the US, Canada, France, United Kingdom, Italy, Japan, and Germany. Even within a single occupational category, such as industry professionals, there is a diverse collection of companies: materials manufacturers, chemical manufacturers, ticketing agencies, renewable energy centers. The heterogeneous nature of the AJFTD website user pool suggests that this database, in practice as well as in theory, provides a relevant source of information for a variety of people related to the alternative jet fuel industry. With future growth of the database in both data categories and dataset size, it is anticipated that the size and diversity of the user pool—and overall relevance of the database—will also grow.
While the current database represents a significant and successful effort to retrieve, synthesize, and organize fuel related data from a variety of sources, ongoing development is required in order to keep up with a quickly evolving arena of aviation fueling, particularly with respect to the rise of novel fuel production pathways. First, improvement of the data retrieval process—primarily the search functionalities and detailed tagging of data within the website—will allow users to locate the most relevant and up to date information for their search. Development of the analytical capabilities of the web portal, such as fuel property comparison functions, will provide additional utility of the database which extend beyond simple data retrieval. Further, the incorporation of state-of-the-art machine learning bulk property predictive models will reflect the current level of analytical capabilities relevant to aviation fuel research, while providing users with the most comprehensive approaches to interact with the data available to them. Beyond this, diversification of the data categories available on the website will ideally reflect not only fuel test data, but also information relevant to the supply chain, environmental impact, and economic impact that specific fuels have on the aviation sector. This will require swift mobilization in establishing data retrieval infrastructure with sources such as airport personnel, airlines, and fuel manufacturers, and will significantly improve high level understanding of aviation fuel industry operations as a whole.
The physicochemical variability among SAF outlined in this paper is a significant motivator for the continued collection and centralization of composition and property information for existing and newly emerging fuels. It will streamline the process for conducting further analysis of SAF properties, composition, and combustion behavior—which will only become more important in the coming years in order to support aggressive global sustainable aviation goals.
3.4 Sustainable aviation fuels compositional variation
As discussed, there are several pathways for SAF production that include various feedstocks and processing methods contributing to physicochemical variability. Conventional JP-8 fuel is similar to commercial Jet A-1 fuel but contains various additives including a static dissipator, corrosion inhibitor, lubricity improver, and a fuel system icing inhibitor as required by MIL-DTL-83133. This fuel primarily contains n-paraffins ranging from C8 to C16, but there is significant variation of JP-8 within the specifications listed, with conventional jet fuel containing hundreds of different hydrocarbons. Other main chemical classes include iso-paraffins, cyclic alkanes, and aromatics. As described in the SPK fuel research report, Petroleum Quality Information Survey (PQIS) data from 2006 reveals variability in aromatic content with 0.06% of jet fuel containing 2.5%–5.0% aromatics by volume versus 5.82% of jet fuel containing 22.5%–25% aromatics by volume (Blakey et al., 2022). From the 2013 PQIS report, the minimum, mean, and maximum reported values for aromatic content in Jet A were 8.4%, 16.25%, and 23.1%, respectively (Defense Logistics Agency Fort Belvoir VA, 2018). These reports indicate that there is significant fuel compositional variability even when solely examining conventional jet fuels in current use. In the following sections, fuel data from the AJFTD is reported for a variety of alternative fuels and fuel blends to show the range of composition and properties present in aviation fuel. The data sampled is not a fully representative dataset of all available fuel types covering all fuel producers, processes, or feedstocks. These selected fuels were chosen for inclusion based on relevance in fuel approval reports and fuel test data availability. The following total ion chromatograms (TIC) provide the chemical class breakdown of conventional JP-8, various FT fuels, and a 50/50 blend of JP-8 and a selected FT fuel. FT 1 is a narrow-cut FT-SPK fuel with a high normal to iso-paraffinic ratio. FT 2 is a natural gas (NG) derived fuel produced utilizing gas to liquid (GTL) processes resulting in a wider cut fuel. FT 3 is coal derived iso-paraffinic kerosene (IPK) as can be seen by the lack of normal paraffins identified as the prominent peaks in the JP-8 TIC. Finally, FT 4 is a second NG derived synthetic fuel produced via a low temperature FT process that results in a fuel with a wide cut and overall composition similar to JP-8. The variable cut of FT fuels can be observed, with FT 1 and FT 3 containing mostly C8-C12 hydrocarbons while FT 2 and FT 4 fuels have a much wider cut spanning C8-C16, similar to conventional JP-8. Some features to note are the lack of normal paraffins in the FT 3 fuel and the greater presence of C9 and C10 normal paraffins in the FT 1 fuel. Figure 6 illustrates the effect of 50/50 blending of FT 3 with JP-8, introducing higher carbon number species (Klein et al., 2006).
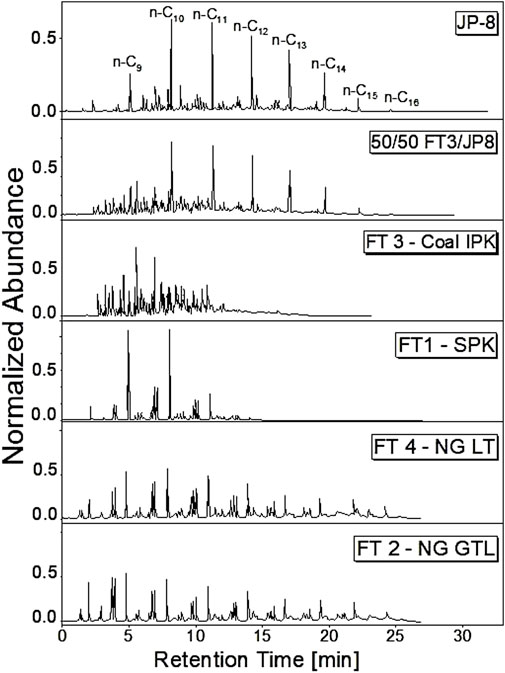
FIGURE 6. Neat JP-8, FT and 50/50 FT3/JP8 blend TICs (Blakey et al., 2022).
In Table 2 the compositional results for selected neat SAF are shown, indicating the relative proportion of the dominant chemical classes in each of the SAF categories. The fuel identification number, POSF, as assigned by AFRL is included to facilitate cross referencing with other studies utilizing these same fuels. The ATJ fuel from Cobalt Technologies is nearly all paraffinic compounds at 98% mass percent with the camelina HEFA fuel from UOP containing the lowest percentage at 85.8% with the remainder of its composition residing in mono-, di-, and tri-cycloparaffins (Edwards et al., 2012b). One feature common to SAF, with the exception of SKA and CHJ fuels, is low to no concentration of aromatics, a result of the various processing methods used (CAAFI, 2020). This feature of SAF provides environmental benefits in usage such as a reduction in soot and particulate matter emissions, but it also reduces the lubricity and proper seal swelling of fuel line system components that are both critical to ensuring drop-in compatibility (Wei and Spikes, 1986). Thus, SAF are only approved for use with blends of conventional jet fuel to ensure that aromatic content meets the minimum requirement of 8%. As outlined by ASTM D7566, CHJ fuels have a relatively high batch property requirement of between 8.4 and 21.2% aromatic content by mass percent (Chong and Ng, 2021). Overall, SAF have a consistent H/C ratio, containing approximately 85% hydrogen with 15% carbon, indicating a low content of other compounds including sulfur or oxygenated components. Data for SIP in Table 2 was obtained from a NAVAIR SYSCOM report from 2014. This report showed that SIP is primarily composed of a single molecule, a C15 iso-paraffin farnesane (Weisser and Turgeon, 2014).
In Table 3, the blends of additional SAF with JP-8 or Jet A are shown, highlighting the changes to the chemical class composition for the blended fuels. Notably, there are significant increases to the proportion of cyclic structures, both in the cycloparaffin and aromatic classes. The fuels all meet the 8% minimum aromatic content requirement once blended with conventional fuels. The blend ratios are also shown, with the Farnesane mixtures included at both 10% and 20% Farnesane blending levels to demonstrate the relative changes in chemical classes as blending ratios vary. As the Farnesane mixtures contain the highest levels of Jet A, the aromatic level in both blends is significantly higher than the other 50/50 blends.
Table 4 details the autoignition characteristics, namely derived cetane number (DCN) and ignition delay (ID) for a selected list of conventional and alternative fuels. The DCN of a fuel indicates its propensity to ignite, with higher DCN corresponding to faster ignition and shorter ID. The fuel ID represents the time it takes a fuel to ignite under a given set of conditions, measured from the end of compression until ignition is observed. It can be seen from the DCNs that the cetane number is not only dependent on fuel type, but it is also fuel structure dependent. Sasol IPK lacks n-paraffins and has a low DCN, while Shell SPK has greater n-paraffin concentration, specifically in the C9-C10 range, and a significantly higher DCN than the Sasol fuel. Weakly branched iso-paraffinic fuels, including Cobalt ATJ-5, Shell SPK, and HEFA, have higher DCN values, while heavily branched iso-paraffinic fuels, such as Sasol IPK and Gevo ATJ, have relatively low cetane values. This is in line with general combustion theory that attributes increased reactivity with straight or weakly branched components and retarded ignition for more heavily branched compounds. Research has also demonstrated a decrease in DCN attributable gem-dimethyl (two methyl groups attached at the same carbon) branch points, whereas the majority ethyl-branch points for Cobalt ATJ-5 may contribute to increased DCN (Lapuerta et al., 2016). DCN is also shown to trend linearly as the 50/50 blends are approximately halfway between the two fuel blendstocks.
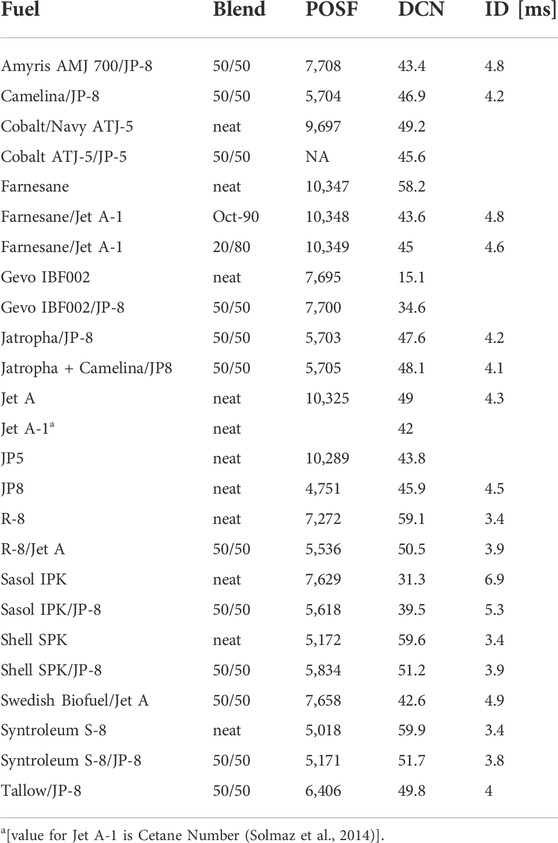
TABLE 4. Autoignition characteristics for selected fuels (Hutzler et al., 2014).
4 Sustainable aviation fuels specification properties evaluation
4.1 World fuel survey and sustainable aviation fuels comparison
To ensure SAF compatibility with current aerospace systems, property specifications detail the fuel characteristics required to meet conventional fuel standards. Table 5 lists fuel identifiers used for data reported in subsequent graphs detailing relevant ASTM D7566 fuel specification requirements for both conventional and alternative jet fuels. Results are shown for fuels from the 2006 CRC WFS supplemented with data from the AJFTD with the number of samples for the WFS fuels indicated in parentheses on the figures (Hadaller and Johnson, 2006; Moses, 2008; Yost et al., 2014). Fuel selection was prioritized based on data availability, inclusion in ASTM approval reports, and any instances reported in relevant literature. Further information on a selection of the listed fuels is provided in the Supplementary Material along with results for additional specification properties including acid number, distillation residue, electrical conductivity, and olefin content. The properties described below including viscosity, flash point, density, freezing point, lubricity, and heat content are significant when considering combustion performance, low temperature fluidity, fuel volatility, and aircraft range.
Aromatics increase fuel density and are of importance to ensure satisfactory swelling of elastomer seals such as those used in O-ring seals. Fuels lacking aromatics have been shown to cause fuel system leaks due to insufficient seal swelling (Corporan et al., 2011). As seen in Figure 7A, SAF blend stocks lack aromatics and could pose a hazard if not appropriately blended. To ensure proper swelling, the SAF are required to be blended with conventional fuels which contain aromatics and the resulting blends can be seen to contain appreciable aromatic content (Bessee, 2012). Density is another crucial fuel characteristic as it impacts the resulting fuel load for the plane and is linked to additional fuel properties such as dielectric constant (Kinder, 2010). Figure 7B indicates that SAF blends fall within the specified range for density. Some neat SAF blend stocks (a few FT and ATJ samples) fall below the specification minimum and thus must be blended to meet the density requirements. Fuel heat content indicates the energy contained within the fuel and therefore is an important fuel property when considering aircraft performance and flying range. Fuels that do not meet the minimum value would require a greater amount of fuel to provide the same energy as a higher heat content fuel. For planes with limited fuel payloads, having a lower heat content fuel would result in a shorter flying range, impacting planned flying routes and posing serious safety hazards if it is unaccounted for. Figure 7C indicates that all the fuels shown, including SAF, meet the net heat of combustion requirement.
Another fuel safety property is freezing point, important when considering cold operating conditions at high altitudes. Fuels falling above specification maximum could pose fuel line hazards as the fuel may solidify and cause lines to seize up. The freezing point is one of the low-temperature fuel properties that is controlled in the ASTM specifications. This specification ensures that systems can operate reliably in expected flight conditions. The freezing point is determined as the temperature at which visible solid fuel wax particles disappear on warming. As shown in Figure 8A, all fuels meet the specification maximum of −40°C for Jet A and −47°C for JP8, with the exception of CHJ falling just above the JP8 minimum. The hydrogen content of fuels is used to establish the net heat of combustion, determined by subtracting the hydrogen content of the fuel from the gross heat of combustion. As shown in Figure 8B, this value is set with a specification minimum value of 13.4% for JP8, and all fuels shown meet this specification value. Fuel lubricity is an important property when considering pumping systems as fuel lubricates system components including pumps, engine controls, and servo valves and protects against corrosion. Fuels with high wear scar diameters are considered hard fuels with poor lubricity that results in high friction and metal-to-metal contact, causing high wear rates. As shown in Figure 8C, FT1 and HC-HEFA fuel samples are considered hard fuels with ATJ falling close to the specification maximum. The poor lubricity of these fuels is a result of hydrocracking and other severe refining conditions, which remove natural fuel lubricants. Blended with JP8, FT fuels fall below the specification maximum and thus meet lubricity requirements (Sympson, 2014; Yost and Brandt, 2012).
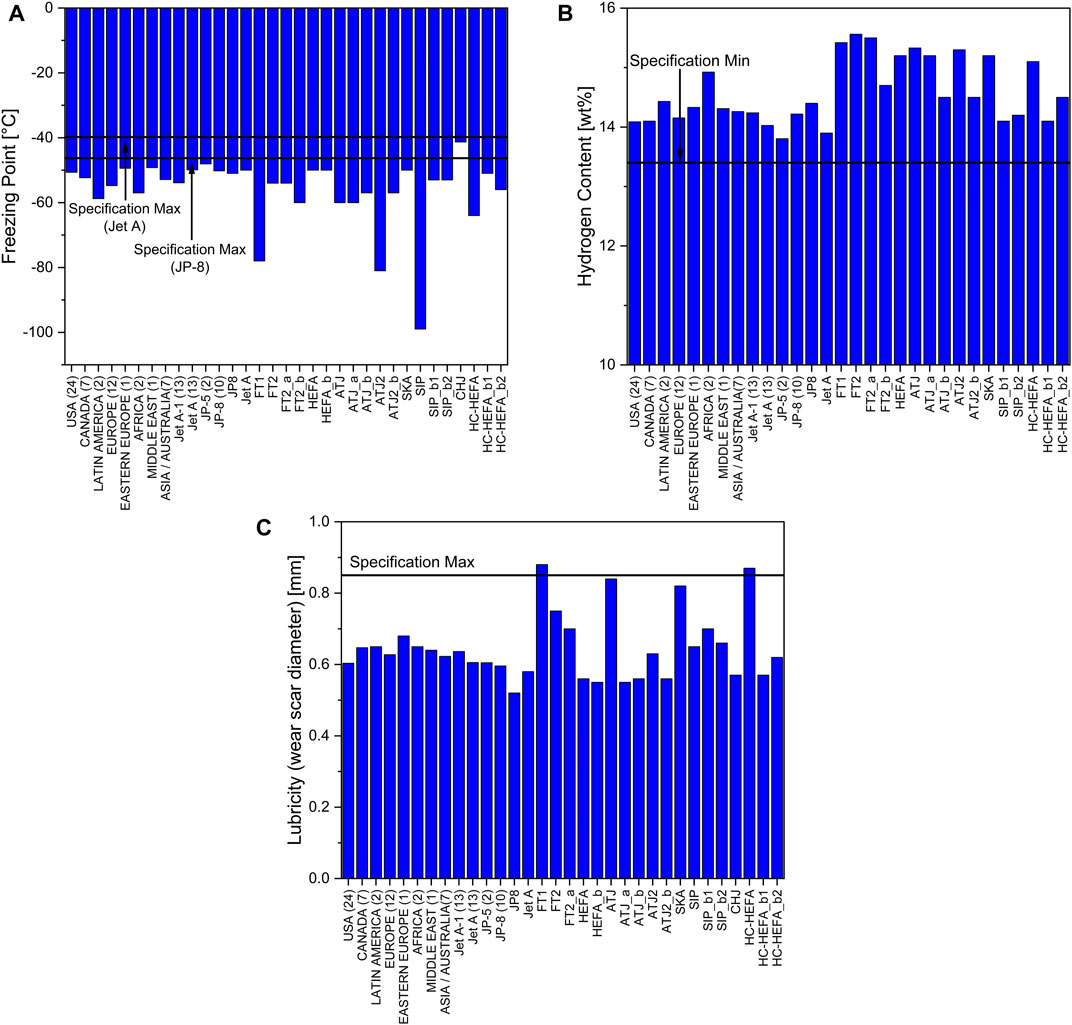
FIGURE 8. Results for WFS and SAF (A) freezing point (Jet A, JP 8), (B) hydrogen content, (C) lubricity.
Viscosity is a key fuel property when considering operations at low-temperature conditions found in the high-altitude environments where jet fuels are utilized. Fuel viscosity relates to fuel pumpability over the operational temperature range and fuel atomization in the nozzle spray. The specification max of 8.0 mm2/s is met by all fuels excluding farnesane (SIP) as shown in Figure 9A. Due to its high viscosity, SIP fuels are limited to a max 10% blending level as annexed under D7566 Annex A3, but this blending level can be even lower in cases of viscosity exceeding permissible values due to the specific SIP that is blended (Rolland and Garcia, 2014). The flash point of fuels is another important characteristic when dealing with fuel safety and is the most widely used property when evaluating the flammability hazard of combustible liquids. Fuels that fall below this value are considered hazardous due to their possibility of autoignition in air. As seen in Figure 9B, all SAF meet the specification minimum of 38°C for fuel flash point. The highest flash point of the fuel samples is seen in neat SIP, potentially making it a suitable agent for increasing the flash point of conventional fuels through blending.
4.2 Sustainable aviation fuels variability statistical analysis
Table 6 provides a statistical analysis of the variability of thermophysical property relations for data taken from the Fit-for-Purpose D4054 Review report for selected SAF categories, where FSJF represents Fully Synthetic Jet Fuel (Moses, 2015b). The conclusion of this report stated that overall trends for thermophysical property relations were qualitatively similar, suggesting that fuels behave independent of processing method. In this study, we sought to provide a quantitative analysis of the observed trends and found that significant variance occurs in all examined fuel properties excluding surface tension, which is therefore not included in Table 6. Categories with significant variance (p < 0.05) from conventional values, where WFS results are the hypothesized population mean, are indicated in bold font. Additional slope results for hydrocarbon groupings (HC1, HC2) as well as CRC handbook values are included in the Supplementary Materials, but these categories were excluded from the analyses of slope deviation from WFS fuels as they do not represent SAF categories (Biddle, 2013).
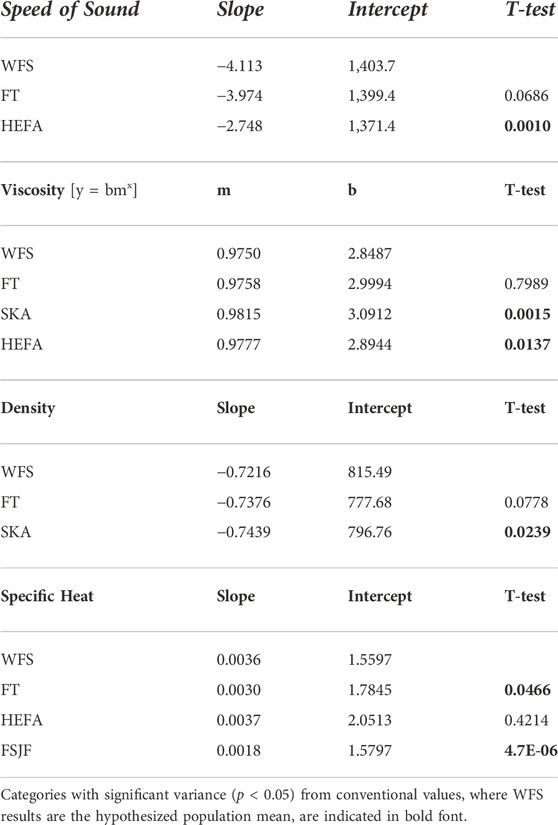
TABLE 6. Statistical analysis results for slope variability for thermophysical property relations for SAF.
Table 7 presents the calculated thermophysical relations for WFS conventional fuels SAF as presented in the 2013 CRC WFS report for conventional fuels. These relations were used to compute 95% confidence interval expected property value ranges for properties listed in the specification requirements, detailed in Table 8. Not all properties contained values for the SAF categories examined, so results are shown where relevant data was available. Additional thermophysical property relations for isentropic bulk modulus, specific heat, surface tension, speed of sound and thermal conductivity are provided in the Supplementary Material. These equations can inform readers as to the expected values for these properties over a selected range of temperatures when experimental data is not available.
Table 8 details the expected ranges for viscosity and density given the variability observed from fuel type data as listed in approval reports. Of the thermophysical properties reported in Table 6, only viscosity and density are currently regulated by ASTM specifications for SAF. The tables indicate the given fuel types meet specification requirements excluding FT and SKA exceeding density requirements. As these fuels only slightly exceed the listed fuel specifications, it can be safely assumed that for actual fuel production, fuel producers are able to meet the required values with slight modifications to the processing procedures. From these results, we conclude that although the previous thermophysical relations indicated significant variation from conventional fuels, the expected property ranges still fall within specification requirements. Additional ranges for neat HC and CRC handbook values are provided in the Supplementary Material.
5 Evolution of sustainable aviation fuels certification
The investigation of fuel composition and property variability outlined in this manuscript precedes a broader discussion of fuel characterization and certification. Recent discussions have focused on an effort to further streamline the multi-year SAF approval process by limiting approval to fuel composition of final blend components. The ASTM Generic Annex had been proposed as a route to introduce sustainable aviation fuels into the pipeline by establishing conservative blending limits that could ensure fuel properties are appropriately controlled. The blend level for sustainable fuels was proposed to be capped at 10% by volume, much lower than the 50% blending limit allowed for sustainable fuels approved through the various annexes to ASTM D7566. One justification for this low blending level was to control fuel property variability, as sustainable fuels introduced at such reduced ratios would have little discernable impact on observable fuel properties. As a result, these sustainable fuels could forgo extensive rig testing, reducing both the time and cost for bringing such fuels into the marketplace. This would have significant advantages for fuel producers and their investors who seek to mitigate risks involved with developing new sustainable fuels, opening them up to increased markets even at low blending levels. While safety always remains the top priority, another upcoming industry objective as seen by the research community is providing a scientific directive on the feasibility of 100% SAF operation. With high profile flight tests running engines on 100% SAF making news with increasing frequency, researchers in the area are looking at ways to address the main composition-property discrepancies between sustainable and conventional fuels. For example, such considerations include employing a “blend of blends” approach in which a variety of SAF blend stocks are blended with one another to address deficits in composition or property specifications, such as aromatic content. Additionally, new fuels are now entering the certification process at unprecedented rates, which, if certified, provide a more diverse selection of blend stocks to incorporate in such a SAF-centered approach to blending.
After further discussions with original equipment manufacturers (OEMs) and other stakeholders involved in the jet fuel marketplace, it was decided that the Generic Annex would be replaced with a less aggressive Fast Track proposal. This route would be an option that would allow emerging SAF that resemble conventional jet fuel in their chemical composition to receive reduced fuel testing as part of the D4054 qualification process. Having a controllable method by which to input blend stock properties and predict final blend properties would support the goals of more readily introducing SAF into the blended aviation fuel market. FCAST, a fuel chemometric software developed by the Naval Research Laboratory, shows promise in achieving this aim. FCAST predicts global performance parameters of an arbitrary fuel blend based on gas chromatography mass spectrometry (GC-MS) and physical properties. The results have been validated on an extensive property library from the Defense Logistics Agency. Adapting the current format of the FCAST software to include datasets from SAF will be a crucial step in validating its applicability for future ASTM SAF approval routes. The aim to streamline fuel certification through controllable fuel blend property prediction can help guide the advancement of the software and stimulate further SAF development. The advancement of fuel analysis techniques and their incorporation into fuel screening procedure and specification requirements will be another avenue to further streamline the SAF approval process. Utilizing two-dimensional gas chromatography (GCxGC), can be especially beneficial for alternative fuels as it is uniquely able to capture fuel compositional features such as cycloparaffinic and heavily branched paraffinic content (Striebich et al., 2014). Efforts to standardize GCxGC testing methods will be crucial to its potential inclusion as a standardized specification under ASTM D7566.
As mentioned in Section 3.3, in recent years there has been a particular emphasis on developing and deploying low-volume prescreening methods for modeling and predicting certification-relevant properties for new fuels entering the certification process. These models may be purely data driven (i.e., fuel agnostic) or may incorporate domain knowledge on fuel chemical compositions and properties to further improve model accuracy, including but not limited to features such as isomer compositions and distributions within a sample (Isaacman et al., 2012; Feldhausen et al., 2022; Heyne et al., 2022). Further, experimental methods such as vacuum ultraviolet (VUV) spectroscopy are being explored for improving resolution of compositional data from processes like GCxGC by resolving specific isomers whose individual chemical properties are known, which shows potential for reducing uncertainty in predictive models (Heyne et al., 2022). A combination of higher fidelity, higher resolution low-volume test methods and the incorporation of larger, more inclusive composition-property datasets across the broadest range of fuels possible will provide the best outlook for optimizing fuel prescreening procedures and the certification process as a whole.
6 Conclusion
For reasons including energy security, price certainty, and environmental benefits, research continues to grow in evaluating alternative transportation fuels that meet combustion performance requirements in existing engine architecture. This study aims to deliver an overview of relevant chemical characteristics and property variability in SAF annexed under ASTM D7566. Although significant variance for thermophysical properties exists for SAF, the variability is not so great as to result in expected property values outside of specification ranges. Additionally, most SAF blend stocks meet ASTM D7566 specification requirements, and all SAF blends satisfy property specifications. This work provides valuable data to support future certification efforts aimed at reducing prohibitive costs of the approval process for experimental SAF. Previous proposals, such as the ASTM Generic Annex, sought to control fuel properties through conservative fuel blend limits to open the door for fuel producers to bring new fuels online. Beyond these conservative limits, fuel producers will want to evaluate how higher alternative blend stock ratios impact overall blend properties, and this will be a key area of future research. The knowledge that fuels can be classified, and their properties predicted by carbon number, hydrocarbon class, chain length, and branching level are crucial factors to improve SAF certification to remove the necessity for evaluating new fuels based on feedstock or processing method. As aptly stated, “hydrocarbons do not know where they come from,” therefore it is more important to consider the fuel chemical makeup (Moses, 2015b). This knowledge can enhance fuel analysis tools that utilize chemometric analysis, such as NRL’s FCAST software, to provide property predictions for fuel blends. As new fuels are integrated into the aviation fuel pipeline, there will likely be a re-examination of specification requirements to more accurately control properties based on actual fuel performance metrics and not simply existing legacy concerns. Tracking the latest trends and changes in physicochemical properties of newly emerging fuels on the AJFTD will become increasingly critical to streamlining this process as diversification in the sustainable fuel industry continues to accelerate. Further, implementing new analysis methods such as two-dimensional gas chromatography, GCxGC, could also become an avenue for future specification modification. As fuel research continues down many pathways, it will become ever more important for certification procedures to stay abreast of the latest analysis methods to ensure an efficient and effective approval process is in place to accommodate the ongoing development of SAF.
Data availability statement
The original contributions presented in the study are included in the article/Supplementary Material, further inquiries can be directed to the corresponding author.
Author contributions
AO: AJFTD establishment, manuscript ideation and writing, statistical analyses. AS: AJFTD development, documentation, manuscript writing and analysis. TL: Principal Investigator.
Funding
This work was funded by the US Federal Aviation Administration (FAA) Office of Environment and Energy as a part of ASCENT Project 33 under FAA Award Number: 13-C-AJFE-UI Amendment 33. Any opinions, findings, and conclusions or recommendations expressed in this material are those of the authors and do not necessarily reflect the views of the FAA or other ASCENT sponsors. This research was performed while Anna Oldani held a National Science Foundation Graduate Research Fellowship.
Acknowledgments
The work presented in this paper has been cleared for public release by the Air Force Research Laboratory.
Conflict of interest
The authors declare that the research was conducted in the absence of any commercial or financial relationships that could be construed as a potential conflict of interest.
Publisher’s note
All claims expressed in this article are solely those of the authors and do not necessarily represent those of their affiliated organizations, or those of the publisher, the editors and the reviewers. Any product that may be evaluated in this article, or claim that may be made by its manufacturer, is not guaranteed or endorsed by the publisher.
Supplementary material
The Supplementary Material for this article can be found online at: https://www.frontiersin.org/articles/10.3389/fenrg.2022.1052267/full#supplementary-material
References
Astm Standard D1655 (2018). Specification for Aviation Turbine Fuels. West Conshohocken, PA: ASTM International.
Astm Standard D4054 (2016). Practice for qualification and approval of new aviation turbine fuels and fuel additives. West Conshohocken, PA: ASTM International.
Astm Standard D7566 (2018). Specification for aviation turbine fuel containing synthesized hydrocarbons. West Conshohocken, PA.
Defense Logistics Agency Fort Belvoir VA (2018). PQIS annual report petroleum quality information system,” Belvoir, VA.
Bessee, G. R. (2012). Propulsion and power rapid response research and development (R&D) support delivery order 0011: Advanced propulsion fuels R&D subtask : Advanced propulsion fuels research and development support to AFRL/RQTF. Fort Belvoir: Defense Technical Information Center.
Biddle, T. (2013). Handbook of aviation fuel properties. Fort Belvoir: Defense Technical Information Center. Fourth Edition.
Blakey, S., Rauch, B., Oldani, A., and Lee, T. (2022). Advanced fuel property data platform: Overview and potential applications. Front. Energy Res. 10. 1325. doi:10.3389/fenrg.2022.771325
Blakey, S., Rye, L., and Wilson, C. W. (28852011). Aviation gas turbine alternative fuels: A review. Proc. Combust. Inst. 33 (2), 2863–2885. doi:10.1016/j.proci.2010.09.011
Caafi (2022). Alternative jet fuels (AJF) and sustainale aviation fuel (SAF). Available at: https://www.caafi.org/resources/faq.html#Alternative (Accessed Jan 30, 2022).
CAAFI (2020). Aviation industry approval of catalytic hydrothermolysis jet ful (CHJ) brings number of SAF approved pathways to six. CAAFI. Available at: https://caafi.org/news/NewsItem.aspx?id=10492.
Chong, C. T., and Ng, J.-H. (2021). Bio-jet fuel in aviation applications.” in Property specifications of alternative jet fuels. Elsevier, 143.
Corporan, E., Edwards, T., Shafer, L., DeWitt, M. J., Klingshirn, C., Zabarnick, S., et al. (2011). Chemical, thermal stability, seal swell, and emissions studies of alternative jet fuels. Energy fuels. 25 (3), 955–966. doi:10.1021/ef101520v
Edwards, J. T., Shafer, L. M., and Klein, J. K. (2012). U.S. Air Force hydroprocessed renewable jet. Fort Belvoir: Defense Technical Information Center.
Edwards, J. T., Shafer, L. M., and Klein, J. K. (2012). U.S. Air Force synthetic paraffinic kerosene (SPK) fuel research. Fort Belvoir: Defense Technical Information Center.
Edwards, T., Fleming, S., Johnston, G., McCall, M., Rumizen, M., and Wright, M. E. (2014). Evaluation of alcohol to jet synthetic paraffinic kerosenes. ATJ-SPKs.
Edwards, T. (2017). Reference jet fuels for combustion testing. Reston: American Institute of Aeronautics and Astronautics ARC.
Eswaran, S., Subramaniam, S., Scott, G., Brandt, K., Wolcott, M., and Zhang, X. (2021). Techno-economic analysis of catalytic hydrothermolysis pathway for jet fuel production. Renew. Sustain. Energy Rev. 151, 111516. Available:. doi:10.1016/j.rser.2021.111516
Fact Sheet (2021). Biden administration advances the future of sustainable aviation fuels in American aviation. Available at: https://www.whitehouse.gov/briefing-room/statements releases/2021/09/09/fact-sheet-biden-administration-advances-the-future-of-sustainable-fuels-in-american aviation/(Accessed: Jan 30, 2022).
Feldhausen, J., Bell, D. C., Yang, Z., Faulhaber, C., Boehm, R., and Heyne, J. (2022). Synthetic aromatic kerosene property prediction improvements with isomer specific characterization via GCxGC and vacuum ultraviolet spectroscopy. Elsevier Fuel.
Fuel Qualification (2022). Caafi. Available at: https://www.caafi.org/focus_areas/fuel_qualification.html.
Goh, B. H. H., Chong, C. T., Ong, H. C., Seljak, T., Katrašnik, T., Józsa, V., et al. (2022). Recent advancements in catalytic conversion pathways for synthetic jet fuel produced from bioresources. Energy Convers. Manag. 251, 114974. doi:10.1016/j.enconman.2021.114974
GreenFleet (2010). Department of the navy’s energy program for security and independence. Available at: http://greenfleet.dodlive.mil/files/2010/04/Naval_Energy_Strategic_Roadmap_100710.pdf.
Gutiérrez-Antonio, C., Gómez-Castro, F. I., de Lira-Flores, J. A., and Hernández, S. (2017). A review on the production processes of renewable jet fuel. Renew. Sustain. Energy Rev. 79, 709–729. doi:10.1016/j.rser.2017.05.108
Heyne, J., Bell, D., Feldhausen, J., Yang, Z., and Boehm, R. (2022). Towards fuel composition and properties from Two-dimensional gas chromatography with flame ionization and vacuum ultraviolet spectroscopy. Amsterdam: Elsevier Fuel.
Hutzler, S. A., Jeyashekar, N., and Petersen, K. M. (2014). Rapid response research and development (R&D) for the aerospace systems directorate delivery order 0021: Engineering research and technical analyses of advanced airbreathing propulsion fuels. Fort Belvoir: Defense Technical Information Center.
Isaacman, G., Wilson, K. R., Chan, A. W. H., Worton, D. R., Kimmel, J. R., Nah, T., et al. (2012). Improved resolution of hydrocarbon structures and constitutional isomers in complex mixtures using gas chromatography-vacuum ultraviolet mass spectrometry. Anal. Chem. 84, 2335–2342. doi:10.1021/ac2030464
Jet Fuel Price Surge is Clouding U.S. Airlines’ Recovery Plans (2021). Bloomberg. Available at: https://www.bloomberg.com/news/articles/2021-10-19/jet-fuel-price-surge-is-clouding-u-s-airlines-recovery-plans.
Kinder, J. D. (2010). Evaluation of bio-derived synthetic paraffinic kerosenes. Miami: Ascension Publishing.
Klein, J. K., Puterbaugh, M., and Morris, R. (2006). Propulsion and power rapid response research and development support delivery order 0042: Demonstration and evaluation of fischer-tropsch research fuels for the DoD assured fuels programFort Belvoir: Defense Technical Information Center.
Lapuerta, M., Hernández, J. J., and Sarathy, S. M. (2016). Effects of methyl substitution on the auto-ignition of C16 alkanes. Combust. Flame 164, 259–269. doi:10.1016/j.combustflame.2015.11.024
Moore, R. H., Thornhill, K. L., Weinzierl, B., Sauer, D., D’Ascoli, E., Kim, J., et al. (2017). Biofuel blending reduces particle emissions from aircraft engines at cruise conditions. Nature 543 (7645), 411–415. doi:10.1038/nature21420
Moses, C. A. (2008). Comparative evaluation of semi-synthetic jet fuels addendum: Further analysis of hydrocarbons and trace materials to support DXXXX. Washington, DC: National Center for Food and Agricultural Policy.
Moses, C. A. (2015). Evaluation of synthesized aromatics Co produced with iso-paraffinic kerosene for the production of semi-synthetic jet fuel. Fort Belvoir: Defense Technical Information Center. no. Technical Report CAM-TR-2015-1.
Moses, C. A. (2015). Review of fit-for-purpose properties of synthesized hydrocarbon kerosenes and blends. Fort Belvoir: Defense Technical Information Center. Technical Report CAM-TR-2015-2.
Rolland, O., and Garcia, F. (2014). Evaluation of synthesized iso-paraffins produced from hydroprocessed fermented sugars. Fort Belvoir: Defense Technical Information Center.
Rumizen, M. A. (2021). Qualification of alternative jet fuels. Front. Energy Res. 2. Available at: https://www.frontiersin.org/articles/10.3389/fenrg.2021.760713/full.
Solmaz, H., Yamık, H., Çingür, Y., and Calam, A. (2014). Investigation of the effects of civil aviation fuel Jet A1 blends on diesel engine performance and emission characteristics. Indian J. Eng. Mat. Sci. 21, 200
Striebich, R. C., Shafer, L. M., Adams, R. K., West, Z. J., DeWitt, M. J., and Zabarnick, S. (2014). Hydrocarbon group type Analysis of petroleum-derived and synthetic fuels using GCxGC. Washington, DC: Energy and Fuels ACS Publications.
Sympson, J. (2014). Rapid response research and development (R&D) for the aerospace systems directorate delivery order 0021: Engineering research and technical analyses of advanced airbreathing propulsion fuels subtask: T700 biofuel low lubricity endurance.
Weisser, K. L., and Turgeon, R. T. (2014). The work was published in the Naval fuels and lubricants cross functional team research report.” in Synthesized iso-paraffin specification and fit-for-purpose test results.
Wilson, G. R., Edwards, T., Corporan, E., and Freerks, R. L. (2013). Certification of alternative aviation fuels and blend components.
Yost, D. M., Brandt, A. C., and Hansen, G. A. T. (2014). Rapid response research and development (R&D) for the aerospace systems directorate delivery order 0021: Engineering research and technical analyses of advanced airbreathing propulsion fuels subtask: Engine and pump studies utilizing JP-8 and alcohol-to-J.
Keywords: sustainable aviation fuel, alternative jet fuel test database, AJFTD, certification, fuel properties, fuel variability, physicochemical, jet fuel
Citation: Oldani AL, Solecki AE and Lee T (2022) Evaluation of physicochemical variability of sustainable aviation fuels. Front. Energy Res. 10:1052267. doi: 10.3389/fenrg.2022.1052267
Received: 23 September 2022; Accepted: 03 November 2022;
Published: 24 November 2022.
Edited by:
IMR Fattah, University of Technology Sydney, AustraliaReviewed by:
Md Abul Kalam, University of Technology Sydney, AustraliaJo-Han Ng, University of Southampton Malaysia, Malaysia
Claudia Gutiérrez-Antonio, Universidad Autónoma de Querétaro, Mexico
S. M. Ashrafur Rahman, Queensland University of Technology, Australia
Copyright © 2022 Oldani, Solecki and Lee. This is an open-access article distributed under the terms of the Creative Commons Attribution License (CC BY). The use, distribution or reproduction in other forums is permitted, provided the original author(s) and the copyright owner(s) are credited and that the original publication in this journal is cited, in accordance with accepted academic practice. No use, distribution or reproduction is permitted which does not comply with these terms.
*Correspondence: Alexandra E. Solecki, c29sZWNraTRAaWxsaW5vaXMuZWR1