- 1School of Agriculture, Western Illinois University, Macomb, IL, United States
- 2Department of Agriculture, Illinois State University, Normal, IL, United States
- 3School of Biological Sciences, Illinois State University, Normal, IL, United States
- 4CoverCress Inc., St. Louis, MO, United States
- 5Commercial Aviation Alternative Fuels Initiative, Cincinnati, OH, United States
Thlaspi arvense L. (Field Pennycress; pennycress) is being converted into a winter-annual oilseed crop that confers cover crop benefits when grown throughout the 12 million-hectares U.S. Midwest. To ensure a fit with downstream market demand, conversion involves not only improvements in yield and maturity through traditional breeding, but also improvements in the composition of the oil and protein through gene editing tools. The conversion process is similar to the path taken to convert rapeseed into Canola. In the case of field pennycress, the converted product that is suitable as a rotational crop is called CoverCress™ as marketed by CoverCress Inc. or golden pennycress if marketed by others. Off-season integration of a CoverCress crop into existing corn and soybean hectares would extend the growing season on established croplands and avoid displacement of food crops or ecosystems while yielding up to 1 billion liters of seed oil annually by 2030, with the potential to grow to 8 billion liters from production in the U.S. Midwest alone. The aviation sector is committed to carbon-neutral growth and reducing emissions of its global market, which in 2019 approached 122 billion liters of consumption in the U.S. and 454 billion liters globally. The oil derived from a CoverCress crop is ideally suited as a new bioenergy feedstock for the production of drop-in Sustainable Aviation Fuel (SAF), renewable diesel, biodiesel and other value-added coproducts. Through a combination of breeding and genomics-enabled mutagenesis approaches, considerable progress has been made in genetically improving yield and other agronomic traits. With USDA-NIFA funding and continued public and private investments, improvements to CoverCress germplasm and agronomic practices suggest that field-scale production can surpass 1,680 kg ha−1 (1,500 lb ac−1) in the near term. At current commodity prices, economic modeling predicts this level of production can be profitable across the entire supply chain. Two-thirds of the grain value is in oil converted to fuels and chemicals, and the other one-third is in the meal used as an animal feed, industrial applications, and potential plant-based protein products. In addition to strengthening rural communities by providing income to producers and agribusinesses, cultivating a CoverCress crop potentially offers a myriad of ecosystem services. The most notable service is water quality protection through reduced nutrient leaching and reduced soil erosion. Biodiversity enhancement by supporting pollinators’ health is also a benefit. While the efforts described herein are focused on the U.S., cultivation of a CoverCress crop will likely have a broader application to regions around the world with similar agronomic and environmental conditions.
Introduction
A reliable, sustainable, and secure biofuels industry in the U.S. requires a diverse portfolio of feedstocks that can be utilized across multiple energy platforms. This includes dedicated industrial crops for production of lipids which can be efficiently converted to drop-in fuels using both proven and emerging technologies. According to the National Oilseed Processing Association (NOPA), companies are investing in oilseed-to-biofuels processing facilities across the U.S. Cargill, Bunge, and ADM are leading the industry in conversion of soy oil, distillers corn oil, and other diverse soft oilseeds for the biodiesel and renewable diesel markets. Additionally, the Commercial Aviation Alternative Fuels Initiative (CAAFI) is monitoring the efforts of multiple companies who have communicated their intent to install over 45.4 billion liters of additional lipid hydro-processing facilities over the next 5 years. However, the limited domestic availability of diverse oilseed feedstocks may hinder such development. To address the demand for lipid feedstocks for sustainable aviation and other biofuels, the authors are working to convert pennycress (Thlaspi arvense L., Field pennycress) into a new oilseed crop to be grown as a winter-annual industrial crop throughout the U.S. Midwest Corn Belt. While the primary near-term goal is to commercialize converted pennycress as a feedstock for renewable diesel, there is a longer-term goal to also commercialize converted pennycress as a feedstock for sustainable aviation fuel. To reach this longer-term goal, there are many steps along the path to commercialization from ‘farm to flight’.
In 2019, the authors were awarded a $10 million grant to fund the IPREFER (Integrated Pennycress Research Enabling Farm and Energy Resilience; www.iprefercap.org/) Project as part of the USDA-NIFA Coordinated Agriculture Projects focused on Sustainable Agricultural Systems. This project has the singular goal of removing production bottlenecks to commercialize converted pennycress by 2022. The IPREFER team consists of academic collaborators from Illinois State University, Ohio State University, University of Minnesota, University of Wisconsin, Southern Illinois University, and Western Illinois University in addition to researchers from the USDA-ARS North Central Soil Conservation Research Lab and the Agricultural Utilization Research Institute in Minnesota. The private partner developing and commercializing a converted form of pennycress is CoverCress Inc. (CCI) in St. Louis, MO.
To ensure a fit with downstream market demand, the conversion of pennycress involves not only improvements in yield and maturity through traditional breeding but also improvements in the composition of the oil and protein through gene editing tools. The conversion process is similar to the path taken to convert rapeseed into Canola. In the case of field pennycress, the converted product that is suitable as a rotational crop is called CoverCress™ as marketed by CCI or golden pennycress if marketed by others. This integrated team of researchers has diverse expertise to deliver advances in pennycress germplasm, gene editing techniques, crop management, harvest efficiency, and post-harvest handling and processing of CoverCress seeds.1
Agronomic studies are conducted on environmentally-diverse test plots located in four Midwestern states (IL, MN, OH, WI). Economic, social, and environmental data generation helps guide research and development directions as well as inform community dissemination through leveraged extension and education components. Results of the agronomic work are integrated into the evaluation of fuel and byproduct production with the goal of economic sustainability for the entire supply chain. These efforts are coupled with techno-economic analyses of the entire energy-crop value chain as well as development of decision-making tools applicable throughout the Midwest region. Outreach is focused on regional producers and agricultural industries along with 4-H, undergraduate and graduate students, and the general public. Through these integrated approaches, the new CoverCress crop is successfully progressing along the path to commercialization as evidenced to date by industry partner CCI who will be delivering CoverCress seed to contracted growers for fall 2022 planting.
Importance to Aviation Industry
The anthropogenic greenhouse gases (GHGs) contributed by the transportation sector are significant. The U.S. Energy Information Administration indicates the commercial aviation market is responsible for up to 13% of such transportation GHG emissions (U.S. Energy Information Administration, 2020b), i.e., generating less than 3% of all manmade CO2 production. However, demand for commercial air travel continues to grow, with expected, sustained average annual traffic growth rates of from 3%–5%, with strong demand coming from developing regions. Depending on traffic growth and new aircraft (with improved efficiency driven by new technology incorporation) assimilation rates, the current global aviation CO2 emissions have the potential to increase to 200%–300% of today’s levels by 2050. The civil aviation industry recognizes the pressure of such emissions growth with respect to societal demands to reduce GHGs and has made multiple voluntary commitments to do so. Aviation is the first industrial sector to make a significant commitment to near-term carbon-neutral growth, from 2020 onward, and making a long-term (2050) commitment on physical net carbon reductions of −50% from 2005 levels. In early 2021, U.S. airline members of Airlines for America updated their goals and committed to 2030 SAF usage levels, and to net-zero carbon by 2050 (Airlines for America, 2020). On 04 October 2021, the International Air Transport Association (IATA) announced an agreement from their member airlines, worldwide, to commit to net zero carbon by 2050 (IATA Net Zero, 2022). The announcement outlines significant targets for SAF production and use as required to meet the goal, as well as calling on the International Civil Aviation Organization (ICAO) to develop by 2022 a long-term goal for SAF than can be promulgated under the Carbon Offsetting and Reduction Scheme (CORSIA). All these goals will rely on the use of sustainable aviation fuels (SAF) that are able to demonstrate significant net lifecycle reductions in CO2 emissions (low Carbon Intensity (CI) scores) versus use of petroleum-based jet fuel.
CORSIA was established by ICAO as a framework of standards concerning the assessment and adoption of SAF that demonstrate reduction of CO2 emissions in international aviation. As of September 2021, the United States and 106 other countries have committed to participate in the first voluntary phase of CORSIA from 2021–2026, covering >77% of all international aviation activity. All ICAO member states will participate in the second phase from 2027–2035 representing >90% of all international activity, even with CORSIA allowed exceptions. A CORSIA approved SAF is a renewable or waste-derived fuel that meets the sustainability criteria of CORSIA (ICAO CORSIA, 2022).
The Biden administration, on 9 September 2021 held a Sustainable Aviation Summit where they announced a SAF Grand Challenge entailing government agencies working together to develop a comprehensive strategy for scaling up new technologies to produce SAF on a commercial scale. Goals of the Challenge include a nearer term goal of producing 1.63 billion liters per year by 2030 (or about 10% of U.S. demand) and ultimately supplying sufficient SAF to meet 100% of U.S. aviation fuel demand by 2050 (159 billion liters per year) (The White House, 2021a; Energy, 2021). U.S. airlines simultaneously announced their intent to utilize such fuel volumes. There has also been legislation proposed in the U.S. House and Senate to foster the development of SAF with incentives including the Clean Skies Act and Sustainable Aviation Fuels Act, a primary tenet of which is the implementation of a Blender’s Tax Credit for the production of SAF meeting certain CI scoring. Legislative efforts are also being pursued around the world to either incentivize or mandate the use of SAF, including in the European Union, United Kingdom, and Canada.
Due to U.S. production only occurring at two facilities (World Energy’s Paramount, California, USA, facility, and Gevo’s facility in Silsbee, Texas, USA), SAF has been in regular commercial use since 2016 in limited volumes. In 2020, the Environmental Protection Agency reported U.S. SAF usage (volumes complying with the U.S. Renewable Fuels Standard) of 21 million liters which falls short of the civil aviation demand (U.S. Environmental Protection Agency, 2021d). However, additional SAF production facilities are in development world-wide with the Air Transport Action Group of the International Air Transport Association reporting airline industry SAF offtake commitments of more than 12 billion liters at the end of September 2021 (GreenAir News, 2021), with additional deals being announced on a frequent basis.
Low carbon feedstocks therefore are gaining prominence in the effort to develop SAF and meet industry targets and commitments. Concerns regarding food-versus-fuel conflicts and other unintended consequences of first-generation biofuels have driven bioenergy research towards developing novel feedstocks that minimize competition with food-crop production. Bioenergy feedstocks can be sustainably produced through ‘sustainable intensification’ or, extensification, which is the targeted use of underutilized land or biomass residues or the intensification of conventional crop rotations (Heaton et al., 2013). Among such crop rotations, purpose-grown oilseeds hold promise for meeting the regulatory specifications of SAF and other renewable fuels. Specifically, the use of lipid feedstock as a source of renewable liquid fuels is particularly significant because they can easily be converted to produce drop-in fuels that have been tested successfully in commercial and military operations and are market-ready.
The aviation industry has qualified the use of two thermochemical conversion processes to convert fats, oils, and greases (FOG) to synthetic jet fuel, as well as allowing FOG to be co-processed with petroleum in existing refineries (see ASTM D7566 and D1655 specifications respectively). Additional efforts are underway to: evaluate four additional conversion processes for FOG; expand the possibility for refinery co-processing; and, allow select FOG conversion processes to be used as fully drop-in fuels with no blending requirements.
FOG include waste greases, animal fats, municipal waste and sludge, algae, food processing oils, and purpose grown oil-bearing seeds and nuts. Several industrial oilseed crops fit the criteria of no direct land-use change (Shi et al., 2019) due to being non-food crops and non-land displacing especially since these are suited for winter production in most regions.
Winter oilseeds, like CoverCress seed, are “second generation” feedstocks that are also an example of temporal intensification in which feedstock crops are integrated into the fallow seasons of existing rotations and avoids the direct and indirect land-use change impacts associated with agricultural intensification (Fargione et al., 2008) or displacement of existing crop production (Searchinger et al., 2008), respectively. They also provide a means of achieving the ecosystem service benefits of cover cropping, such as erosion control and reduced nutrient leaching, with a net profit to producers rather than at a significant cost (Plastina et al., 2018). Winter oilseeds are known to be effective in various rotations to break disease and pest cycles, recycle nutrients in the soil, reduce nutrient leaching, and reduce or eliminate weed problems (Seepaul et al., 2016; Shi et al., 2019). Biomass returned to the soil with only the seed being harvested is a major differentiating factor between non-food oilseed crops and other first generation (starches and sugars) or certain second generation (cellulosic and lignocellulosic) crops. This results in maximum sequestration of carbon and return of nutrients to the soil for the following crops (Seepaul et al., 2019).
Why Pennycress?
Pennycress is an oilseed-producing member of the Brassicaceae family, closely related to rapeseed, canola, carinata, and camelina (Best and Mcintyre, 1975; Warwick et al., 2010; Franzke et al., 2011). Containing 30%–32% oil with a fatty acid profile that allows for easy conversion to biofuels meeting the U.S. Renewable Fuels Standard, pennycress can help fill the demand for SAF (Moser et al., 2009; Moser, 2012). Pennycress is unique in that it has a small non-repetitive diploid genome (Dorn et al., 2013; Dorn et al., 2015; McGinn et al., 2018) and can be easily genetically manipulated (Sedbrook et al., 2014; Chopra et al., 2018; McGinn et al., 2018; Chopra et al., 2020), akin to its well-known model relative Arabidopsis thaliana. Extremely winter hardy with high oilseed yields and a short life cycle, pennycress can be integrated into the fallow period of existing cropping systems in the U.S. Midwest as a profitable winter cover crop (Phippen and Phippen, 2012; Fan et al., 2013; Johnson et al., 2015; Jordan et al., 2016; Johnson et al., 2017; Ott et al., 2018). For example, pennycress, as converted to CoverCress, can be planted following corn or soybean in late summer/early fall. Early-maturing pennycress varieties can be harvested in mid-May to June in time to plant full-season summer crops which allows producers to produce two cash crops on the same land in 1 year.
As a protective living cover during the offseason in the Midwest, a pennycress crop, such as CoverCress, provides numerous ecosystem services. Ecosystem benefits include nutrient retention, increased pollinator health, and biodiversity (Malakoff, 1998; Eberle et al., 2015). The deadzone in the Gulf of Mexico, which is the second largest deadzone in the world, has been attributed to nitrogen inputs from agriculture in Minnesota, Iowa, Illinois, Indiana, and Ohio (Malakoff, 1998; Scavia, and Donnelly, 2007; David et al., 2010). Both surface and subsurface drainage modifications bypass traditional mitigation efforts (Baker and Johnson, 1981; Baker et al., 2008). Nutrients losses from agricultural fields can be reduced, in part, with the implementation of reduced fertilizer application rates in combination with optimized spatial and temporal applications (Roth et al., 2018; Wang and Weil, 2018). However, producers are reluctant to reduce nitrogen application due to perceived risk to corn yields. Moreover, tile drainage bypasses traditional best management practices. Cover crops, like pennycress, provide a remedy in that they sequester nutrients before reaching tiles (Lemke et al., 2011; Jarecki et al., 2018).
A pennycress crop may serve as a nectar and pollen source as early as late April in central Minnesota and earlier in more southern states (Sindelar et al., 2017). No other agronomic crops, aside from winter camelina (a companion oilseed of pennycress), have the ability to flower so early and en masse as a pennycress crop in the U.S. Midwest region, particularly in its converted form as a CoverCress crop. Pennycress fields can flower for 3–4 weeks during which they produce abundant quantities of pollen and nectar (Eberle et al., 2015; Thom et al., 2018). Pennycress flowers are visited frequently by a wide range of insects, including native bees (Eberle et al., 2015; Groeneveld and Klein, 2015; Thomas et al., 2017; Thom et al., 2018). If early, reliable, and extensive floral resources were available in the U.S. Midwest, local honey production would increase, and overall colony health would improve. It is also hypothesized that health and vigor of early-emerging native pollinators would increase with access to fields of spring-flowering pennycress.
Field trials in Illinois and Minnesota have demonstrated that a pennycress crop has a minimal impact on yields of soybean crops following pennycress (Phippen and Phippen, 2012; Johnson et al., 2015; Ott et al., 2018). A pennycress crop, particularly in its converted form as a CoverCress crop, is not invasive, and producers who have grown the crop multiple years in commercial fields attest that it is not a problem weed. Since the same equipment used to manage conventional crops can be used with a pennycress crop (e.g., the same combines used to harvest soybean are used to harvest pennycress), minimal new farm equipment is required. Therefore, a CoverCress crop fits into existing crop rotations resulting in higher total seed yields (two cash crops in 1 year) without requiring new land commitments.
A CoverCress crop is unique among winter cover crops in that it can generate income as an oilseed crop thereby providing incentive for adoption by producers and stakeholders. Off-season integration of a CoverCress crop into existing corn and soybean hectares would extend the growing season on established croplands while avoiding displacement of food crops. At current commodity prices, our economic modeling informed by producers, industry partners, investors, and other stakeholders predicts the modest production increases enabled by the IPREFER project will make a CoverCress crop profitable across the entire supply chain.
How Have Researchers Improved Pennycress for Commercialization as CoverCress?
Researchers have developed varieties with low seed coat fiber content and low erucic acid seed oil that produce seed meal and oil equivalent to that of canola (Chopra et al., 2018; Chopra et al., 2020). After less than a decade of selection and breeding, CoverCress lines yield over 1,680 kg ha−1 (1,500 lb ac−1) of seed with newer varieties approaching 2,241 kg ha−1 (2,000 lb ac−1). Wild pennycress stands have been reported to produce seed yields as high as 2,246 kg ha−1 (Mitich, 1996) which equates to 646 L (170 gal) of oil and 1,460 kg of seed meal per ha (1,302 lb ac−1). Given the success of their breeding, gene editing, and agronomic program, CCI believes 2,465 kg ha−1 (2,200 lb ac−1) can be attained commercially in a CoverCress crop in the near term through marker-assisted breeding in combination with gene editing and improvements to agronomic practices. At 2,200 kg ha−1 (2,000 lb ac−1), a CoverCress crop planted on half of the rotational hectares of the U.S. Midwest Corn Belt would produce 5 billion liters (1.1 billion gal) of oil and 7 million metric tons (MT) of edible seed meal annually.
In addition to rapid advances in breeding, CCI researchers have identified and validated pennycress mutations that confer agronomic trait improvements necessary for commercial launch. These mutations were generated using both classical mutagenesis and Clustered Regularly Interspaced Short Palindromic Repeats (CRISPR)-Cas9 gene editing approaches. Trait improvements include: absence of erucic acid in seed oil (fae1 mutation produces edible oil equivalent to canola oil and well-suited for biodiesel and SAF generation), reduced seed glucosinolate content (e.g., aop2 mutation makes the seed meal highly palatable as an animal feed), reduced seed coat fiber (e.g., ttg1, tt8, or tt2 mutations individually reduce seed coat fiber content thereby improving the nutritional value of the CoverCress meal), increased seed oil from 25%–30% to 30%–34%, and reduced seed pod shatter (ind partial loss-of-function mutations reduce preharvest seed loss) (Chopra et al., 2018; McGinn et al., 2018). As of 2021, researchers have combined these genetic improvements into elite breeding lines and are currently conducting field trials across multiple locations throughout the central U.S. Midwest region as well as commercial seed inventory production for a planned commercial launch of CoverCress in the fall of 2022.
To support our efforts in securing CoverCress as a newly converted pennycress crop, the U.S. Department of Energy Biological and Environmental Research (DOE-BER) program funded in 2020 the $13 million Integrated Pennycress Resilience Project (IPReP; www.pennycressresilience.org/) focused on interrogating natural and induced genetic variation in pennycress to improve abiotic stress tolerance. As a winter annual, pennycress/CoverCress undergoes a plethora of environmental challenges including drought in the fall, freeze-thaw cycles throughout the winter, and water logging and heat waves in the spring. These environmental challenges are becoming more frequent and severe with climate change (IPCC, 2021), hence the need to introduce genetics into future generations of the crop to confer improved resilience. IPReP involves interdisciplinary teams from public universities, non-profit institutions and U.S. DOE labs who are employing eco-evolutionary computational genomics along with traditional mutagenesis and CRISPR genome editing approaches to identify key genetic variants that confer superior abiotic stress resilience.
CoverCress Commercialization Efforts
During the initial years of converting pennycress, it became apparent breeding varieties with consistent seed yields across years would be essential for commercialization. CCI (previously known as Arvegenix) was founded in 2013 and started with a structured plant-breeding program including a broad germplasm base, detailed crossing and testing programs, and the goal to convert pennycress into CoverCress following a path similar to the one taken for converting rapeseed into canola (Bell, 1982) (Figure 1). CoverCress grain will initially be marketed as a feed ingredient in the production of broiler chickens and in its whole grain form at a 2%–4% inclusion rate in the feed ration. As CCI scales hectares and CoverCress grain production increases, CoverCress grain (following completion of product development efforts) will go to crush and the extracted oil will be used in renewable fuel production and the extracted meal as livestock feed, using all carbon components produced.
The transition from whole grain feed to a crush ready product with the extracted oil for fuel will occur when economies of scale are met. This will occur when sufficient volumes of seed reached, product development efforts are completed, and processing facilities are improved to handle diversified feedstocks including CoverCress grain that has been specifically produced under an offtake agreement with Bunge that was announced in the spring of 2022. As part of a recently announced partnership between Bunge and Chevron, Bunge and CCI entered into a product offtake agreement whereby Bunge will purchase and process CoverCress grain. Also as a part of that announced partnership, Bunge will begin modifying and improving the current processing facilities to handle new feedstocks. Bunge’s U.S. soybean processing plants in Destrehan, Louisiana, and Cairo, Illinois, will be a part of the joint venture with Chevron contributing approximately $600 million in cash (Bunge, 2022). Plans include approximately doubling the combined capacity of these facilities from 7,000 MT per day by the end of 2024 (Bunge, 2022). Under the agreements, Bunge will operate the facilities; Chevron will have purchase rights for the oil to use as a renewable feedstock to manufacture transportation fuels with lower lifecycle carbon intensity (Bunge, 2022). The CCI and Bunge offtake agreement is an integral part of the Bunge and Chevron joint venture (Lane, 2022).
Breeding and Gene Editing
CCI’s current CoverCress product offering is a gene edited “golden seed” variety converted from pennycress having low fiber content in the seed meal for improved animal feeding and low erucic acid which produces healthier oil characteristics. Another striking improvement has been in seed germination. Wild pennycress varieties have a characteristically high initial dormancy rate, with first year germination of only 30%–50%. With mutations in the tt8 gene, the seed coat is thinner, has a golden color, and seed exhibits dramatically improved germination rates to greater than 95% for freshly harvested seed. The improved germination has substantially increased yield by allowing for a more reliable and consistent stand establishment in the fall which leads to an earlier harvest in the spring (Figure 2). Reducing seed dormancy also alleviates weediness due to the seeds germinating when planted instead of lingering in the soil. By increasing the crude protein content in the seed meal from 25% to 30%, value is added as a livestock feed. CCI, along with the parallel work at IPREFER institutions, is working together through plant breeding, gene editing, and agronomy programs to continuously improve the current products for higher yields and added positive traits. Traits currently in the development pipeline include reduced glucosinolates and herbicide tolerance.
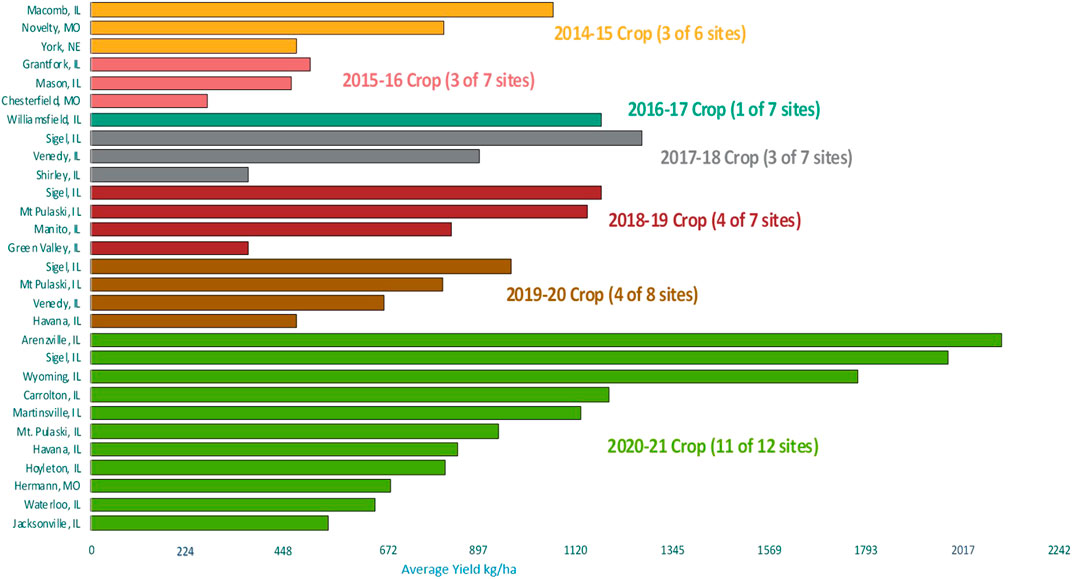
FIGURE 2. Multi-year and multi-location yield data demonstrating significant seed yield increases of CoverCress™ through traditional plant breeding and gene-editing.
Seed Increase and Supply Chain
The first commercial CoverCress product was developed in 2019. This led to foundation-seed-expansion efforts in the 2020–21 season and commercial seed production in the 2021–2022 season. As expansion efforts continue, the details on the immediate supply chain for grain handling becomes paramount. One hectare of commercial seed production can produce the seed for 48 ha (121 acres), assuming a yield of 1,681 kg ha−1 (1,500 lb ac−1) and a seeding rate of 5.6 kg ha−1 (5 lb ac−1).
Ideal foundation-seed-expansion production ground is a relatively flat topography, relatively weed free or free of certain weed types, where no HPPD (4-Hydroxyphenylpyruvate dioxygenase inhibitor) herbicide was applied in the spring/summer before CoverCress seeding. Fields are observed throughout the season for weeds and CoverCress stand establishment. To ensure high quality seed and purity, a very clean combine optimized for small oilseeds is used for harvest.
The main supply chain components are: contract grower production (plant/cultivate/harvest/deliver), transportation to local seed handlers, grain cleaning/drying and storage, and seed delivery to end-product users. After an initial period of expansion (i.e., during the period when CoverCress grain is marketed in its whole grain form for inclusion in broiler feed) and final product development to achieve the low glucosinolate trait, the grain will also go to crush (for oil excrescence and meal production), with the initial customer being Bunge under the aforementioned offtake agreement. The oil and meal will be delivered to subsequent product producers. Harvesting CoverCress grain could use one of three likely models: 1) individual producers harvesting their fields; 2) one producer harvesting for each farm cluster (simplifying out-of-season machinery use); or 3) hired custom harvest. It is likely that a combination of the three models will be employed, especially in the first 5–10 years as hectares increase and the best option is determined.
Grain cleaning efforts show that a single additional sieving process after harvest at the seed handler will provide adequately clean grain when seed is harvested with properly adjusted combines. Forced-air grain drying at ambient temperature works well for CoverCress grain, and a moisture level of ∼10%–12% which is adequate for storage is achieved in a couple of days (when the grain is harvested at ∼18% moisture). While CoverCress grain can be stored in standard bins by grain handlers, customization of the bin flooring is required due to the small seed size. Flat storage with movement of grain via bucket loaders is also a viable option.
Launch Area
The initial launch area considered by CCI is south central Illinois and portions of Missouri (Figure 3). The initial launch is slated for 4,046 ha (10,000 ac) to be planted in fall 2022 with expected expansion to 20,234 ha (50,000 ac) in fall 2023. As producers become more interested about contracting for CoverCress grain production and expansion of hectares increase, CCI will expand its geography to include Iowa, Indiana and Ohio. The commercialization model depicted in Figure 4 mainly considered farmer adoption for increased hectares along with the development of new CoverCress varieties having improved yields as well as earlier maturity (shorter cycle) allowing for expansion of where the crop can be planted.
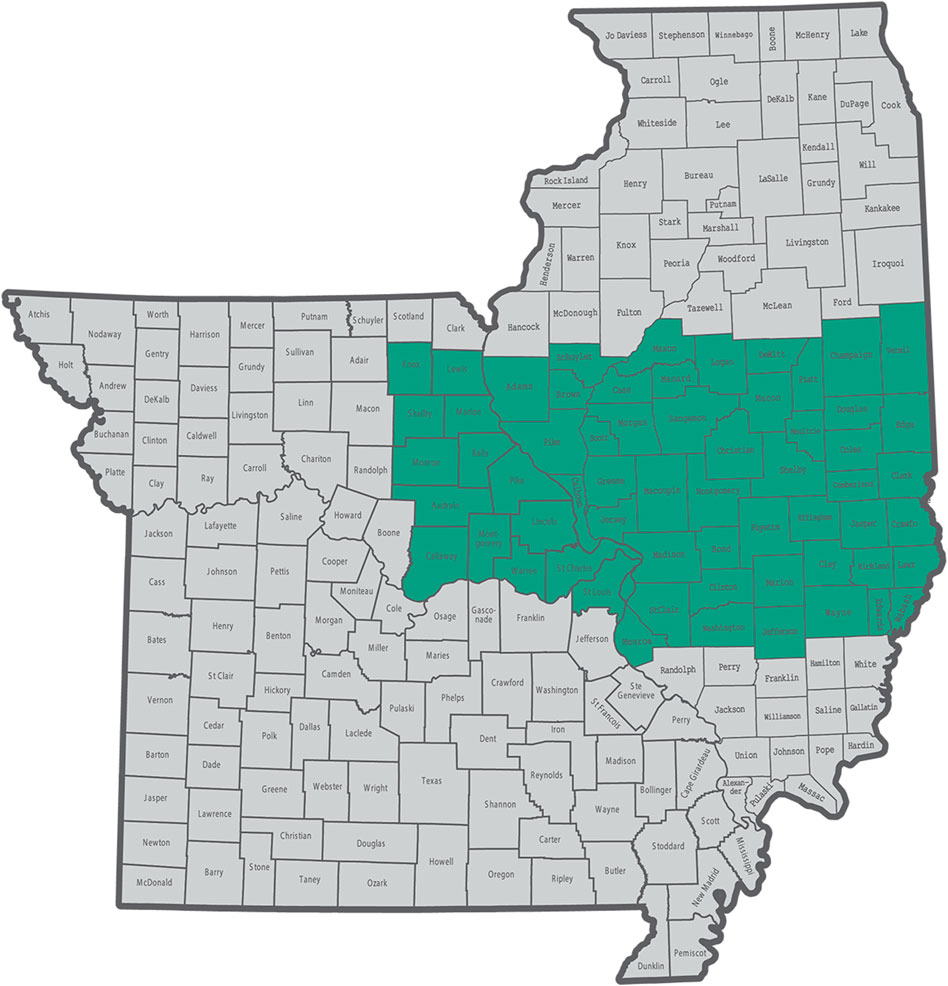
FIGURE 3. Commercial target launch zone for CoverCress™ seed in central Illinois and northeast Missouri, USA.
CoverCress seed is an important part of the broad solution to produce low carbon intensity fuels which are critical to reducing global warming. Because crush facilities for plant-based oil extraction and fuel production from these oils use well-established processes, CoverCress seed oil can be added rapidly to the fuel feedstock markets. As shown by Frank et al. (2021), time is a critical component in the fight against climate change. Well established processes can make the final impact more relevant due to the time it will take to fully implement lower carbon alternatives to our way of life.
How Does CoverCress Fit Into the Low Carbon Bioeconomy?
In the United States, approximately 80% of the total energy consumed is supplied from fossil fuels (U.S. Energy Information Administration, 2020a). The U.S. ranks second globally in GHG emissions, contributing approximately 15% of the total amount released annually (U.S. Environmental Protection Agency, 2021a). The U.S. Energy Independence and Security Act of 2007 mandates an increase in renewable transportation fuels to 136 metric liters by 2022 (Fan et al., 2013; U.S. Environmental Protection Agency, 2021b), but progress in fuel production beyond ethanol and biodiesel has been rather limited versus expectations included in the Act. Recently, President Biden signed an executive order to reduce emissions across federal operations which calls for a 65% reduction in emissions by 2030 and net-zero emissions by 2050 (The White House, 2021b). A few states have imposed their own programs to reduce GHG emissions. One of the most ambitious programs to reduce GHG emissions is the California Low Carbon Fuel Standard (LCFS), which requires oil refineries and distributors to reduce GHG emissions by replacing petroleum-based fuels with renewable fuels used for transportation. The program considers a full life cycle analysis of the fuel, which includes carbon emissions during production and consumption of the fuel. The program is designed to encourage the adoption of low carbon and renewable fuel alternatives which in turn will reduce the carbon intensity of transportation fuels by 20 percent by 2030 (California Air Resource Board, 2021a). An LCFS program also exists in Oregon, modelled closely after the California model, and a Clean Fuel Program was also created in Washington in 2021. This sets the stage for LCFS incentives to draw renewable fuel production and deliveries to west coast markets, irrespective of their area of production. These LCFS programs are designed to provide the greatest reward to those producing biofuels with the lowest CI scores. These programs are designed to provide incentives that are aligned with the desired behavior of producing fuels with increasingly less carbon intensive. This strategy further promotes transitions among alternative fuels.
CI scores measure the amount of carbon dioxide equivalent (C02e), by weight, emitted per unit of energy consumed for a given fuel (e.g. grams (g) of CO2e MJ−1). Fuel derived from biological sources, such as plants which pulled their carbon content from the atmosphere, have much lower CI scores than petroleum-based fuels. Renewable fuels with lower CI scores than petroleum-based fuels will aid with climate and environmental protection and promote the transition from a fossil fuel-based economy towards a sustainable biobased or bioeconomy having sustainable economic growth.
In California, the beginnings of a biobased economy are well underway. Businesses that produce biofuel with CI scores lower than the target value for the year can earn an LCFS credit which can be sold to a company whose fuel production process generates excessive carbon emissions. The credit price of carbon for the week of 15 November 2021 traded at $179.36 MT−1 with a volume of 618,108 MT traded for a total value of $110,862,192 (California Air Resources Board, 2021b). From 2011 to 2018, the LCFS program removed 38 million MT of CO2 emissions and saw a 74% increase in renewable fuel use. In 2018, the total value of credit transactions was approximately $2 billion (California Air Resources Board, 2021b).
Reported CI scores for producing petroleum-based diesel and jet fuel are 91.66 and 89.37 g CO2e MJ−1, respectively (California Air Resource Board, 2021c). By comparison, the CI score for biodiesel and renewable jet fuels has been reported at 35.4 and 36.4 g CO2e MJ−1, respectively (U.S. Environmental Protection Agency, 2021c). CI scores for a fuel can vary based upon inputs (petroleum-based or plant-based oil), production efficiencies, and for plant-based renewable fuels, from how the crop was grown. Growing biofuel crops following best management practices that minimize GHG production will lower CI scores of the fuel produced. Such best management practices include adopting conservation tillage practices, including winter cover crops, and optimizing fertilizer inputs to increase yields while limiting GHG emissions such as CO2 and N2O. Paustian et al. (2019) describes how conservation tillage practices can reduce C losses from soil while improved crop rotations and the inclusion of winter cover crops can add C to soils with the potential of sequestering 4–5 Gt CO2 per year globally for 2–3 decades.
As conservation practices are introduced on cropland, carbon sequestration in soil typically follows a sigmoidal curve, eventually reaching a new equilibrium concentration. It has been estimated that soil may be able to sequester 5%–15% of global fossil fuel emissions (Lal, 2004). Carefully managing fertilizer applications are essential because higher fertilizer rates can stimulate microbial activity that will release more GHG from soil, increasing the crops CI score. A study by Field et al. (2018) found that by optimizing soil cultivation and N fertilizer application on switchgrass used as a feedstock to produce cellulosic ethanol, GHG emissions were reduced by up to 22 g CO2e MJ−1 of energy produced compared to conventional gasoline.
The CoverCress product shows great potential as an oilseed crop because it can produce renewable fuels such as biodiesel, renewable diesel, and SAF and may be suitable to grow on approximately 12 million hectares in the Midwest typically left winter fallow. A preliminary life cycle analysis by CCI has reported a CI score of 30.23 g CO2e MJ−1 for biodiesel production (CoverCress, 2019), although biodiesel produced from fatty acid methyl esters will have a lower carbon intensity than hydrotreated oils used to make jet fuel (Moser, 2012). In addition, CCI conducted an Induced Land Use Changes (ILUC) study through Purdue University using the GTAP-BIO model used by the California Air Resources Board (CARB). This ILUC study examined the effects of a limited stock of 757 million liters (200 million gallons) of biodiesel produced from CoverCress as a second crop with the level of biodiesel produced from pennycress generating an ILUC value of −30.6 g CO2e MJ−1 on average. Fan et al. (2013) conducted a life cycle assessment comparing pennycress to petroleum to synthesize biodiesel and renewable jet fuel. The study evaluated pennycress as a winter annual in the U.S. Midwest, grown between corn harvested in the fall and soybean planted in the spring. The study concluded that using pennycress to produce biodiesel and renewable jet fuel reduced GHG emissions by up to 85% and 63% respectively, compared to producing petroleum-based fuels. It was further concluded that growing pennycress between corn and soybean did not compete with food crops for land, nor did pennycress cause a yield reduction from the subsequent soybean crop (Fan et al., 2013).
While in the past, producers have been incurring unrecoverable costs in adopting cover crops, there appears to be progress on clearing this hurdle to CoverCress commercialization. Thompson et al. reviewed the 2017 U.S. Census of Agriculture and reported that cover crops had been adopted on only 11% of farms, and just 4% of cropland acres, in Illinois, Indiana, and Iowa (2021). They further highlighted the reluctance of producers to plant cover crops is evident in that more than half of cover crop adopters planted less than 20 ha (50 ac) of cover crops (Thompson et al., 2021). However, in more recent surveys, producers are expressing an interest in growing a CoverCress crop. A survey conducted by Zhou et al. (2021) evaluated the willingness of producers to grow CoverCress as an energy feedstock. Results showed that approximately 58% of producers surveyed were interested in growing a CoverCress crop if it was profitable; and among those interested, 54.4% would accept the farmgate price of $0.28 kg−1. They further concluded a profit of $179 ha−1 could be achieved from an average harvest of 1,600 kg ha−1 (1,427 lb ac−1) sold at $0.28 kg−1 ($0.62 lb−1). This would generate a gross income of $448 ha−1 ($988 ac−1) and assumed a production cost of $269 ha−1 ($665 ac−1) (Zhou et al., 2021).
Conclusion
In order to substantially reduce GHG emissions of commercial aviation and meet the SAF Grand Challenge, a second-generation of biofuels is needed to develop feedstocks that minimize competition with food-crop production. As an extremely hardy winter cover crop with high oilseed yields and a low CI score, a CoverCress crop helps to fulfill this need. The efforts of IPREFER and its constituent university teams, as well as those of CCI in varietal and product development, supply chain strategies, and rapid growth in commercialization hectares have positioned CoverCress as new rotational crops to become a source of renewable fuels such as renewable diesel, SAF and biodiesel. As commercial farm production of the CoverCress crop begins in the fall 2022, converted pennycress is well on its way from “farm-to-flight” as it becomes a commercial low carbon intensity cash cover crop for sustainable aviation fuel. IPREFER, in close partnership with CCI, will be working a multifaceted research, education, and outreach approach over the next 3 years to remove the remaining hurdles for broad producer and stakeholder adoption.
Author Contributions
WP, RR, JS, CH, and SC contributed to conception and design of the manuscript. WP organized the first draft of the manuscript. WP, RR, JS, CH, and SC wrote sections of the manuscript. All authors contributed to manuscript revision, read, and approved the submitted version.
Funding
In addition to research funding provided by Western Illinois University, Illinois State University, and CoverCress Inc., this material is based upon work that is supported by the National Institute of Food and Agriculture, U.S. Department of Agriculture, under award numbers 2014-67009-22305, 2018-67009-27374, and 2019-67009-29004 and the Agriculture and Food Research Initiative Competitive Grant No. 2019-69012-29851. Portions of this research are also supported by the U.S. Department of Energy, Office of Science, Office of Biological and Environmental Research, Genomic Science Program grant no. DE-SC0021286.
Conflict of Interest
Author CH was employed by the company CoverCress Inc. Author SC was employed by the sponsors of the Commercial Aviation Alternative Fuels Initiative, a public-private-partnership. Illinois State University (JS) has entered licensing agreements with CoverCress, Inc. for use of pennycress mutants. Author JS has stock in CoverCress, Inc.
The remaining authors declare that the research was conducted in the absence of any commercial or financial relationships that could be construed as a potential conflict of interest.
Publisher’s Note
All claims expressed in this article are solely those of the authors and do not necessarily represent those of their affiliated organizations, or those of the publisher, the editors and the reviewers. Any product that may be evaluated in this article, or claim that may be made by its manufacturer, is not guaranteed or endorsed by the publisher.
Footnotes
1Reference to the term CoverCress seed or crop implies inclusion of golden pennycress seed or crop marketed by companies other than CoverCress Inc.
References
Airlines for America (2020). Airlines Fly Green. Available at: https://www.airlines.org/airlines-fly-green/(Accessed Oct 3, 2021).
Baker, J. L., David, M. B., Lemke, A. M., and Jaynes, D. B. (2008). “Understanding Nutrient Fate and Transport, Including the Importance of Hydrology in Determining Field Losses, and Potential Implications for Management Systems to Reduce Those Losses,” in Final Report Gulf Hypoxia and Local Water Quality Concerns Workshop. Upper Mississippi River Sub-basin Hypoxia Nutrient Committee editor (St. Joseph, Michigan, USA: American Society of Agricultural and Biological Engineers), 1–17.
Baker, J. L., and Johnson, H. P. (1981). Nitrate‐Nitrogen in Tile Drainage as Affected by Fertilization. J. Environ. Qual. 10, 519–522. doi:10.2134/jeq1981.00472425001000040020x
Bell, J. M. (1982). From Rapeseed to Canola: A Brief History of Research for Superior Meal and Edible Oil. Poult. Sci. 61, 613–622. doi:10.3382/ps.0610613
Best, K. F., and Mcintyre, G. I. (1975). The Biology of Canadian Weeds: 9. Thlaspi Arvense L. Can. J. Plant Sci. 55, 279–292. doi:10.4141/cjps75-039
Bunge (2022). Bunge and CoverCress Inc. Announce Partnership to Meet Growing Demand for Renewable Fuel Feedstocks. Missouri, USA: Bunge. Available at: https://bunge.com/news/bunge-and-covercress-inc-announce-commercial-partnership-meet-growing-demand-renewable-fuel.
California Air Resource Board (2021c). Low Carbon Fuel Standard. Sacramento, CA, USA. Available at: https://ww2.arb.ca.gov/sites/default/files/2020-05/basics-notes.pdf (Accessed May 23, 2022).
California Air Resources Board (2021a). Low Carbon Fuel Standard. Available at: https://ww2.arb.ca.gov/our-work/programs/low-carbon-fuel-standard (Accessed May 23, 2022).
California Air Resources Board (2021b). Weekly LCFS Credit Transfer Activity Reports. Available at: https://ww3.arb.ca.gov/fuels/lcfs/credit/lrtweeklycreditreports.htm (Accessed May 23, 2022).
Chopra, R., Johnson, E. B., Daniels, E., McGinn, M., Dorn, K. M., Esfahanian, M., et al. (2018). Translational Genomics Using Arabidopsis as a Model Enables the Characterization of Pennycress Genes through Forward and Reverse Genetics. Plant J. 96 (6), 1093–1105. doi:10.1111/tpj.14147
Chopra, R., Johnson, E. B., Emenecker, R., Cahoon, E. B., Lyons, J., Kliebenstein, D. J., et al. (2020). Identification and Stacking of Crucial Traits Required for the Domestication of Pennycress. Nat. Food 1, 84–91. doi:10.1038/s43016-019-0007-z
CoverCress (2019). 1st Cash Cover Crop for the Midwest. St. Louis, MI, USA. Available at: https://caafi.org/resources/pdf/CoverCress_Handel_12_05_2019.pdf (Accessed May 23, 2022).
David, M. B., Drinkwater, L. E., and McIsaac, G. F. (2010). Sources of Nitrate Yields in the Mississippi River Basin. J. Environ. Qual. 39, 1657–1667. doi:10.2134/jeq2010.0115
Dorn, K. M., Fankhauser, J. D., Wyse, D. L., and Marks, M. D. (2015). A Draft Genome of Field Pennycress (Thlaspi Arvense) Provides Tools for the Domestication of a New Winter Biofuel Crop. DNA Res. 22, 121–131. doi:10.1093/dnares/dsu045
Dorn, K. M., Fankhauser, J. D., Wyse, D. L., and Marks, M. D. (2013). De Novo assembly of the Pennycress (Thlaspi Arvense) Transcriptome Provides Tools for the Development of a Winter Cover Crop and Biodiesel Feedstock. Plant J. 75, 1028–1038. doi:10.1111/tpj.12267
Eberle, C. A., Thom, M. D., Nemec, K. T., Forcella, F., Lundgren, J. G., Gesch, R. W., et al. (2015). Using Pennycress, Camelina, and Canola Cash Cover Crops to Provision Pollinators. Industrial Crops Prod. 75, 20–25. doi:10.1016/j.indcrop.2015.06.026
Energy (2021). Sustainable Aviation Fuel Grand Challenge. Available at: https://www.energy.gov/eere/bioenergy/sustainable-aviation-fuel-grand-challenge (Accessed May 23, 2022).
Fan, J., Shonnard, D. R., Kalnes, T. N., Johnsen, P. B., and Rao, S. (2013). A Life Cycle Assessment of Pennycress (Thlaspi Arvense L.) -derived Jet Fuel and Diesel. Biomass Bioenergy 55, 87–100. doi:10.1016/j.biombioe.2012.12.040
Fargione, J., Hill, J., Tilman, D., Polasky, S., and Hawthorne, P. (2008). Land Clearing and the Biofuel Carbon Debt. Science 319 (5867), 1235–1238. doi:10.1126/science.1152747
Field, J. L., Evans, S. G., Marx, E., Easter, M., Adler, P. R., Dinh, T., et al. (2018). High-resolution Techno-Ecological Modelling of a Bioenergy Landscape to Identify Climate Mitigation Opportunities in Cellulosic Ethanol Production. Nat. Energy 3, 211–219. doi:10.1038/s41560-018-0088-1
Frank, J., Brown, T., Haverly, M., Slade, D., and Malmsheimer, R. (2021). Quantifying the Comparative Value of Carbon Abatement Scenarios over Different Investment Timing Scenarios. Fuel Commun. 8, 100017. doi:10.1016/j.jfueco.2021.100017
Franzke, A., Lysak, M. A., Al-Shehbaz, I. A., Koch, M. A., and Mummenhoff, K. (2011). Cabbage Family Affairs: The Evolutionary History of Brassicaceae. Trends Plant Sci. 16, 108–116. doi:10.1016/j.tplants.2010.11.005
GreenAir News (2021). Aviation Industry Looks to ICAO for Leadership on Delivering a Global Long-Term Goal to Reduce Emissions. Available at: https://www.greenairnews.com/?p=1769 (Accessed May 23, 2022).
Groeneveld, J. H., and Klein, A. M. (2015). Pennycress-corn Double-Cropping Increases Ground Beetle Diversity. Biomass Bioenergy 77, 16–25. doi:10.1016/j.biombioe.2015.03.018
Heaton, E. A., Schulte, L. A., Berti, M., Langeveld, H., Zegada-Lizarazu, W., Parrish, D., et al. (2013). Managing a Second-Generation Crop Portfolio through Sustainable Intensification: Examples from the USA and the EU. Biofuels, Bioprod. Bioref 7 (6), 702–714. doi:10.1002/bbb.1429
IATA Net Zero (2022). IATA Net-Zero Carbon Emissions by 2050. Available at: https://www.iata.org/en/pressroom/2021-releases/2021-10-04-03/(Accessed May 23, 2022).
ICAO CORSIA (2022). ICAO CORSIA Eligible Fuels. Available at: https://www.icao.int/environmental-protection/CORSIA/Pages/CORSIA-Eligible-Fuels.aspx (Accessed May 23, 2022).
IPCC (2021). Sixth Assessment Report of the United Nations Intergovernmental Panel on Climate Change. Geneva: IPCC. Available at: https://www.ipcc.ch/(Accessed May 23, 2022).
Jarecki, M., Grant, B., Smith, W., Deen, B., Drury, C., VanderZaag, A., et al. (2018). Long-term Trends in Corn Yields and Soil Carbon under Diversified Crop Rotations. J. Environ. Qual. 47, 635–643. doi:10.2134/jeq2017.08.0317
Johnson, G. A., Kantar, M. B., Betts, K. J., and Wyse, D. L. (2015). Field Pennycress Production and Weed Control in a Double Crop System with Soybean in Minnesota. Agron. J. 107, 532–540. doi:10.2134/agronj14.0292
Johnson, G. A., Wells, M. S., Anderson, K., Gesch, R. W., Forcella, F., and Wyse, D. L. (2017). Yield Tradeoffs and Nitrogen between Pennycress, Camelina, and Soybean in Relay‐ and Double‐Crop Systems. Agron. J. 109, 2128–2135. doi:10.2134/agronj2017.02.0065
Jordan, N. R., Dorn, K., Runck, B., Ewing, P., Williams, A., Anderson, K. A., et al. (2016). Sustainable Commercialization of New Crops for the Agricultural Bioeconomy. Elem. Sci. Anthropocene 4, 000081. doi:10.12952/journal.elementa.000081
Lal, R. (2004). Soil Carbon Sequestration Impacts on Global Climate Change and Food Security. Science 304, 1623–1627. doi:10.1126/science.1097396
Lane, J. (2022). Bunge, CoverCress, Chevron Taking Us into the Future and into the Past. Florida, USA: The Digest. Available at: https://www.biofuelsdigest.com/bdigest/2022/05/09/bunge-covercress-chevron-taking-us-into-the-future-and-into-the-past/.
Lemke, A. M., Kirkham, K. G., Lindenbaum, T. T., Herbert, M. E., Tear, T. H., Perry, W. L., et al. (2011). Evaluating Agricultural Best Management Practices in Tile-Drained Subwatersheds of the Mackinaw River, Illinois. J. Environ. Qual. 40, 1215–1228. doi:10.2134/jeq2010.0119
Malakoff, D. (1998). Death by Suffocation in the Gulf of Mexico. Science 281, 190–192. doi:10.1126/science.281.5374.190
McGinn, M., Phippen, W. B., Chopra, R., Bansal, S., Jarvis, B. A., Phippen, M. E., et al. (2018). Molecular Tools Enabling Pennycress (Thlaspi Arvense) as a Model Plant and Oilseed Cash Cover Crop. Plant Biotechnol. J. 17 (4), 776–788. doi:10.1111/pbi.13014
Mitich, L. W. (1996). Field Pennycress (Thlaspi Arvense L.) -The Stinkweed. Weed Technol. 10, 675–678. doi:10.1017/S0890037X00040604
Moser, B. R. (2012). Biodiesel from Alternative Oilseed Feedstocks: Camelina and Field Pennycress. Biofuels 3, 193–209. doi:10.4155/bfs.12.6
Moser, B. R., Knothe, G., Vaughn, S. F., and Isbell, T. A. (2009). Production and Evaluation of Biodiesel from Field Pennycress (Thlaspi Arvense L.) Oil. Energy fuels. 23, 4149–4155. doi:10.1021/ef900337g
Ott, M. A., Eberle, C. A., Thom, M. D., Archer, D. W., Forcella, F., Gesch, R. W., et al. (2019). Economics and Agronomics of Relay‐Cropping Pennycress and Camelina with Soybean in Minnesota. Agron. J. 111, 1281–1292. doi:10.2134/agronj2018.04.0277
Paustian, K., Larson, E., Kent, J., Marx, E., and Swan, A. (2019). Soil C Sequestration as a Biological Negative Emission Strategy. Front. Clim. 1, 8. doi:10.3389/fclim.2019.00008
Phippen, W. B., and Phippen, M. E. (2012). Soybean Seed Yield and Quality as a Response to Field Pennycress Residue. Crop Sci. 52, 2767–2773. doi:10.2135/cropsci2012.03.0192
Plastina, A., Liu, F., Sawadgo, W., Miguez, F. E., Carlson, S., and Marcillo, G. (2018). Annual Net Returns to Cover Crops in Iowa. J. Appl. Farm Econ. 2 (2), 19–36. doi:10.7771/2331-9151.1030
Roth, R. T., Ruffatti, M. D., O'Rourke, P. D., and Armstrong, S. D. (2018). A Cost Analysis Approach to Valuing Cover Crop Environmental and Nitrogen Cycling Benefits: A Central Illinois on Farm Case Study. Agric. Syst. 159, 69–77. doi:10.1016/j.agsy.2017.10.007
Scavia, D., and Donnelly, K. A. (2007). Reassessing Hypoxia Forecasts for the Gulf of Mexico. Environ. Sci. Technol. 41 (23), 8111–8117. doi:10.1021/es0714235
Searchinger, T., Heimlich, R., Houghton, R. A., Dong, F., Elobeid, A., Fabiosa, J., et al. (2008). Use of U.S. Croplands for Biofuels Increases Greenhouse Gases through Emissions from Land-Use Change. Science 319 (5867), 1238–1240. doi:10.1126/science.1151861
Sedbrook, J. C., Phippen, W. B., and Marks, M. D. (2014). New Approaches to Facilitate Rapid Domestication of a Wild Plant to an Oilseed Crop: Example Pennycress (Thlaspi Arvense L.). Plant Sci. 227, 122–132. doi:10.1016/j.plantsci.2014.07.008
Seepaul, R., George, S., and Wright, D. L. (2016). Comparative Response of Brassica Carinata and B. Napus Vegetative Growth, Development and Photosynthesis to Nitrogen Nutrition. Industrial Crops Prod. 94, 872–883. doi:10.1016/j.indcrop.2016.09.054
Seepaul, R., Marois, J., Small, I. M., George, S., and Wright, D. L. (2019). Carinata Dry Matter Accumulation and Nutrient Uptake Responses to Nitrogen Fertilization. Agron. J. 111 (4), 2038–2046. doi:10.2134/agronj2018.10.0678
Shi, R., Archer, D. W., Pokharel, K., Pearlson, M. N., Lewis, K. C., Ukaew, S., et al. (2019). Analysis of Renewable Jet from Oilseed Feedstocks Replacing Fallow in the U.S. Northern Great Plains. ACS Sustain. Chem. Eng. 7, 18753–18764. doi:10.1021/acssuschemeng.9b02150
Sindelar, A. J., Schmer, M. R., Gesch, R. W., Forcella, F., Eberle, C. A., Thom, M. D., et al. (2017). Winter Oilseed Production for Biofuel in the US Corn Belt: Opportunities and Limitations. GCB Bioenergy 9, 508–524. doi:10.1111/gcbb.12297
The White House (2021b). Executive Order on Catalyzing Clean Energy Industries and Jobs through Federal Sustainability. Available at: https://www.whitehouse.gov/briefing-room/presidential-actions/2021/12/08/executive-order-on-catalyzing-clean-energy-industries-and-jobs-through-federal-sustainability/(Accessed May 23, 2022).
The White House (2021a). FACT SHEET: President Biden’s Leaders Summit on Climate. Available at: https://www.whitehouse.gov/briefing-room/statements-releases/2021/04/23/fact-sheet-president-bidens-leaders-summit-on-climate/(Accessed May 23, 2022).
Thom, M. D., Eberle, C. A., Forcella, F., Gesch, R., and Weyers, S. (2018). Specialty Oilseed Crops Provide an Abundant Source of Pollen for Pollinators and Beneficial Insects. J. Appl. Entomol. 142, 211–222. doi:10.1111/jen.12401
Thomas, J. B., Hampton, M. E., Dorn, K. M., Marks, D. M., and Carter, C. J. (2017). The Pennycress (Thlaspi Arvense L.) Nectary: Structural and Transcriptomic Characterization. BMC Plant Biol. 17, 201. doi:10.1186/s12870-017-1146-8
Thompson, N. M., Reeling, C. J., Fleckenstein, M. R., Prokopy, L. S., and Armstrong, S. D. (2021). Examining Intensity of Conservation Practice Adoption: Evidence from Cover Crop Use on U.S. Midwest Farms. Food Policy 101, 102054. doi:10.1016/j.foodpol.2021.102054
U.S. Energy Information Administration (2020b). July 2020, Monthly Energy Review. Washington, DC, USA. Available at: https://www.eia.gov/totalenergy/data/monthly/pdf/mer.pdf (Accessed May 23, 2022).
U.S. Energy Information Administration (2020a). Primary Energy Overview. Washington, DC, USA. Available at: https://www.eia.gov/totalenergy/data/monthly/pdf/sec1_3.pdf (Accessed May 23, 2022).
U.S. Environmental Protection Agency (2021a). Global Greenhouse Gas Emissions Data. Available at: https://www.epa.gov/ghgemissions/global-greenhouse-gas-emissions-data#Country (Accessed May 23, 2022).
U.S. Environmental Protection Agency (2021c). Lifecycle Greenhouse Gas Results. Available at: https://www.epa.gov/fuels-registration-reporting-and-compliance-help/lifecycle-greenhouse-gas-results (Accessed May 23, 2022).
U.S. Environmental Protection Agency (2021b). Renewable Fuel Standard Program: Regulations and Volume Standards for Renewable Fuel Standards. Available at: https://www.epa.gov/renewable-fuel-standard-program/regulations-and-volume-standards-renewable-fuel-standards (Accessed May 23, 2022).
U.S. Environmental Protection Agency (2021d). RINs Generated Transactions. Available at: https://www.epa.gov/fuels-registration-reporting-and-compliance-help/rins-generated-transactions (Accessed May 23, 2022).
Wang, F., and Weil, R. R. (2018). The Form and Vertical Distribution of Soil Nitrogen as Affected by Forage Radish Cover Crop and Residual Side-Dressed N Fertilizer. Soil Sci. 183, 22–33. doi:10.1097/SS.0000000000000224
Warwick, S. I., Mummenhoff, K., Sauder, C. A., Koch, M. A., and Al-Shehbaz, I. A. (2010). Closing the Gaps: Phylogenetic Relationships in the Brassicaceae Based on DNA Sequence Data of Nuclear Ribosomal ITS Region. Plant Syst. Evol. 285, 209–232. doi:10.1007/s00606-010-0271-8
Keywords: bioenergy, Thlaspi arvense, cover crop, low-carbon fuel, public-private partnership, carbon intensity, sustainable aviation fuel
Citation: Phippen WB, Rhykerd R, Sedbrook JC, Handel C and Csonka S (2022) From Farm to Flight: CoverCress as a Low Carbon Intensity Cash Cover Crop for Sustainable Aviation Fuel Production. A Review of Progress Towards Commercialization. Front. Energy Res. 10:793776. doi: 10.3389/fenrg.2022.793776
Received: 12 October 2021; Accepted: 02 June 2022;
Published: 24 June 2022.
Edited by:
Season Hoard, Washington State University, United StatesReviewed by:
May M. Wu, Argonne National Laboratory (DOE), United StatesJohnathan Holladay, LanzaTech, United States
Jonathan Lloyd Male, Pacific Northwest National Laboratory (DOE), United States
Copyright © 2022 Phippen, Rhykerd, Sedbrook, Handel and Csonka. This is an open-access article distributed under the terms of the Creative Commons Attribution License (CC BY). The use, distribution or reproduction in other forums is permitted, provided the original author(s) and the copyright owner(s) are credited and that the original publication in this journal is cited, in accordance with accepted academic practice. No use, distribution or reproduction is permitted which does not comply with these terms.
*Correspondence: Winthrop B. Phippen, d2ItcGhpcHBlbkB3aXUuZWR1