- 1Institute of Engineering Thermodynamics, German Aerospace Center (DLR), Stuttgart, Germany
- 2Institute of Engineering Thermodynamics, German Aerospace Center (DLR), Cologne, Germany
Molten chloride mixtures such as MgCl2–KCl–NaCl are potential thermal energy storage (TES) materials and heat transfer fluids (HTFs) for next-generation concentrating solar power (CSP) systems due to their high operation temperatures (>700°C) and low costs. This ternary MgCl2–KCl–NaCl salt mixture with a low material cost (<0.35 $/kg) is a promising salt for high-temperature TES/HTF applications, for example, it could enable next-generation CSP plants to have significant power cycle efficiency and lower levelized cost of electricity (LCOE). In this study, the minimum melting temperature composition for the MgCl2–KCl–NaCl mixture was estimated by FactSage simulation, while the binary and ternary phase diagrams of the MgCl2–KCl–NaCl salt system, including their phase transitions, were studied. Five different eutectic compositions of the MgCl2–KCl–NaCl mixture from literature and simulations were selected to determine the minimum melting temperature composition by the differential scanning calorimetry technique. Results show agreement between experiments and simulations of the melting temperature and its composition. Hence, values of the exact and reliable minimum melting temperature and salt composition in mol% and wt% are given. Based on the determined melting temperature, the minimum operating temperature of MgCl2–KCl–NaCl is recommended according to the safety margin for CSP applications.
Introduction
Concentrating solar power (CSP) has been extensively investigated as a key technology for green electricity generation. A dispatchable form of CSP is the possibility to incorporate thermal energy storage (TES) using molten salts. In addition, for large-scale applications, this technology integrated with TES presents an economic advantage regarding manageability which implies lower demand-oriented levelized cost of electricity (LCOE) than volatile photovoltaic technology (Liu et al., 2016). Molten nitrate salts, especially the so-called solar salt (NaNO3-KNO3 60–40 wt%), have been studied extensively for TES and heat transfer applications in the temperature range of 290–565°C (Bonk et al., 2018). However, their thermal stability is limited by the decomposition above 565°C. Thus, the research programs on next-generation CSP, for example, the SunShot Initiative of United States Department of Energy (DOE) focus on higher operation temperature for better power cycle efficiency and major cost reduction using chloride salt mixtures comprising CaCl2, KCl, MgCl2, NaCl, and ZnCl2 among others (Du et al., 2017; Murphy et al., 2019; Vidal and Klammer, 2019).
Low-cost molten chloride salts are some of the most promising high-temperature TES and heat transfer fluid (HTF) materials in next-generation CSP plants and other energy applications (e.g., nuclear molten salt reactor) due to, for example, their high thermal stability (>800°C) and low prices (<0.35 $/kg) (Murphy et al., 2019; Vidal and Klammer, 2019; Ding and Bauer, 2021; Villada et al., 2021). In next-generation CSP plants (see Figure 1), the MgCl2–KCl–NaCl mixture can achieve higher power cycle efficiencies by using, for example, sCO2 Brayton power cycle. In its potential high-temperature TES/HTF applications, this ternary salt presents different challenges which require a study from their initial composition and preparation to the final system integration.
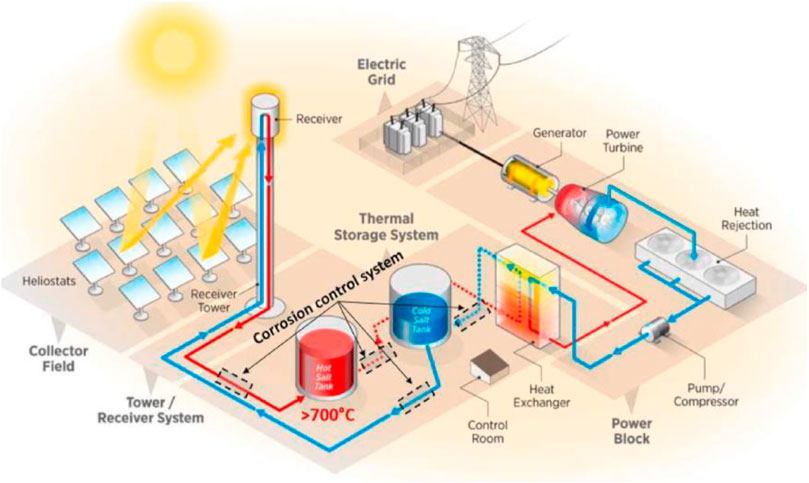
FIGURE 1. Concept of next-generation CSP plant. Adapted from the study by Villada et al., (2021).
The exact and reliable data of the salt properties, such as melting temperature, are vital and essential for the design, construction, and operation of a TES system based on molten salts. However, due to the corrosivity and volatility of molten alkali chlorides at high temperatures and forming of salt hydrates (e.g., MgCl2∙6H2O), the experimental determination of thermodynamic properties, particularly at high temperatures, is complex. Thermodynamic simulation provides a practical way to assist the study on the thermophysical properties of molten chlorides. The salt properties, as well as the corrosion control, of molten chloride salts have been intensively studied in these years for TES/HTF applications. Two recent review articles from our group (Ding and Bauer, 2021; Villada et al., 2021) are suggested for more details on thermophysical properties and corrosion control of MgCl2-containing molten chlorides. The initial studies on chloride salt properties were performed by DOE as molten salt coolants in nuclear reactors using experiments and thermodynamic simulation (Manohar et al., 2010). In the early 1990s, experimental results of binary KCl–MgCl2, KCl–NaCl, and MgCl2–NaCl were reviewed and published by Janz et al. (Rowe et al., 1972; Janz et al., 1975). In the 1920s, the experimental MgCl2–KCl–NaCl ternary diagram has been presented by Scholich with a reported minimum melting temperature below 400°C for the composition 32.5 mol% NaCl, 18.5 mol% KCl, and 49 mol% MgCl2 (Scholich, 1920). However, due to the experimental limitations at that time, the reported old minimum melting temperature and eutectic composition of MgCl2–KCl–NaCl need to be validated. Some research groups have carried out such validation via experiments and simulations, but discrepancies of minimum melting temperature compositions available in the literature (Scholich, 1920; Jänecke, 1950; Podlesnyak et al., 1987; Mohan et al., 2018a; Vidal and Klammer, 2019) are relatively high (about 2 mol%). This may be due to different water contents in the salt mixture used in the experiment, particularly in hygroscopic MgCl2. Currently, our group published a review paper (Villada et al., 2021) to recommend the most relevant salt properties besides minimum melting temperature for the design, construction, and operation of a TES/HTF system based on MgCl2–KCl–NaCl. The properties include vapor pressure, heat capacity, density, thermal conductivity, and dynamic viscosity.
Since about 2015, there has been a strong renewed interest in the ternary MgCl2–KCl–NaCl mixture as one of the most promising TES materials in next-generation CSP due to the abundance and low price of the single salts and relatively low melting temperature (Mohan et al., 2018a; Ding et al., 2018). The DLR started research with the MgCl2–KCl–NaCl ternary mixture in 2015 (Maksoud and Bauer, 2015; Ding et al., 2018), and the National Renewable Energy Laboratory (NREL) considered the binary mixture MgCl2–KCl in 2016 (Mehos et al., 2017). Several researchers from Savannah River National Lab (SRNL), Oak Ridge National Laboratory (ORNL), Argonne National Lab, and Australian National University (ANU) have also been exploring the ternary mixture for sensible and latent TES applications (Tian and Zhao, 2013; Mohan et al., 2019). A new molten chloride salt test facility with the high-temperature molten chloride test loop is being designed by the Oak Ridge National Laboratory (ORNL) to demonstrate the viability of molten chlorides for power generation. In their most recent report (Robb et al., 2019), the loop has a designed operating temperature of 725°C with 20 kg of salt mixture with an approximate composition of 21–23 mol% NaCl, 42–43 mol% KCl, and 34–36 mol% of MgCl2. The system considers the alloys Ha C-276/In 600 as the main structural materials, including corrosion monitoring and control. With the most recent advances in the area of molten chlorides, it can be demonstrated that this technology has a lot of application potential in next-generation CSP systems and nuclear molten salt reactors (MSRs) to generate electricity more efficiently and cost-effectively.
Melting temperature is a key parameter in the selection of a sensible TES or heat transfer fluid (HTF) since it determines the minimum operation temperature (partly the storage capacity) and system design to avoid salt freezing (Bonk et al., 2018; Vidal and Klammer, 2019). For this reason, this study covers a relevant topic as it is the selection of the appropriate composition of the mixture which is one of the challenges associated with improving the properties of TES materials. The minimum melting temperature and eutectic composition of the MgCl2–KCl–NaCl ternary mixture were studied via FactSage thermodynamic simulation. Comparison with previous results and experimental validation with a differential scanning calorimeter (DSC) is presented after the study on the binary mixtures and their eutectic compositions as well as comparison with previous eutectic compositions reported in the literature. The current study will provide a reliable eutectic composition and minimum melting temperature of the MgCl2–KCl–NaCl system as an important step of developing it for applications in the CSP industry and other energy industries. This composition can be used for the commercially used salt mixture, and the important properties such as heat capacity, viscosity, and thermal conductivity, can be better determined for engineering the salt for TES/HTF applications.
Materials and Experimental Methods
Molten Salt Preparation
Five kinds of mixed chlorides were prepared by mixing KCl, NaCl, and MgCl2 at different mixed ratios according to the literature and FactSage prediction. The purities of the chloride salts were >99.9% for NaCl purchased from VWR BDH Chemicals, the purities of KCl and anhydrous MgCl2 were both 99%, purchased from Acros Organics and Alfa Aesar, respectively. Since MgCl2 may absorb H2O from the moisture under air, all salt mixtures were prepared inside a glove box under argon atmosphere (argon 5.0, 99.999%, O2 < 0.5 ppm, H2O < 10 ppm). In addition, MgCl2 was held at 150°C for 18 h to remove the most hydrated water and avoid hydration. Subsequently, around 5.0 g of each salt mixture was prepared from stoichiometric weighing of each single chloride salt, followed by mixing and grinding for at least 20 min manually to ensure homogenization. For the DSC analysis, around 10–20 mg sample was transferred into aluminum crucibles, a pierced lid was placed on top of the crucible, and then hermetically sealed with a sealing press from Netzsch. Then, the samples were carefully transported in a desiccator from the glovebox to the DSC apparatus to avoid atmospheric exposure and salt hydration.
Differential Scanning Calorimetry Measurements
Thermal analysis was carried out with a differential scanning calorimeter DSC 404 from Netzsch using aluminum crucibles under argon atmosphere (Argon 5.0), which was temperature-calibrated using Netzsch standard materials (In, Bi, Zn) in a temperature range of 100–480°C. Three heating and cooling runs from room temperature up to 480°C were performed in total to avoid discrepancies in the melting temperature produced by the traces of hydrated MgCl2. The same heating and cooling rates (10°C/min) were applied for all samples to allow qualitative comparison of the melting point between the samples and using an empty pan as a reference. The first cycle was used for the removal of traces of absorbed water followed by pre-melting and homogenization of the mixture. For the phase diagrams, the onset of the melting peak during heating was extracted since it represents the melting temperature of the salt mixture.
Thermodynamic Simulation With FactSage
FactSage is a commercial thermochemical databank system running in a Windows environment with the modules of Information, Database, Calculation, and manipulation (https://www.factsage.com/). This study utilizes version 7.2 of this software. Calculation is the main module of FactSage, which is based on the minimum Gibbs-free energy and CalPhaD (calculation of phase diagrams) theory to predict the equilibrium conditions of a system (Bale et al., 2016). It includes the submodules such as Reaction, Predom, EpH, Equilib, Phase Diagram, and Optisage. FactSage gives access to databases of thermodynamic data for thousands of compounds, including hundreds of liquid and solid metals, liquid and solid oxides, molten and solid salts, and aqueous solutions. In this study, the submodule Phase Diagram was used to simulate the binary and ternary phase diagrams of the MgCl2–KCl–NaCl salt system to support the experimental determination of the minimum melting temperature composition and melting temperature via DSC.
Result and Discussion of Binary Systems: KCl–MgCl2, KCl–NaCl, MgCl2–NaCl
Literature Review
There have been numerous studies to investigate the binary KCl–MgCl2, KCl–NaCl, and MgCl2–NaCl mixtures and their compositions as candidate materials for thermal energy storage. The phase diagrams of the KCl–MgCl2 and MgCl2–NaCl binary systems were experimentally studied by Menge (1911) (Menge, 1911) as well as the binary NaCl–KCl by Kurnakow (1907) (Kurnakow and Zhemchuzhnui, 1907). Later, Janz, Scholich, and Jänecke (Scholich, 1920; Jänecke, 1950; Janz et al., 1975; Janz and Tomkins, 1981) confirmed those results and discussed their thermophysical properties.
In the review of the literature published by Rowe et al. (Rowe et al., 1972), three eutectic compositions, namely, E1, E2, and E3 are reported for the KCl–MgCl2 system. The compositions are 58 mol% MgCl2 for E1 with a eutectic temperature of 470°C, 36 mol% MgCl2 for E2, and 30 mol% MgCl2 for E3 with a eutectic temperature of 431 and 430°C, respectively. Polimeni et al. (Polimeni et al., 2018) as well as Xu et al. (Xu et al., 2018a) evaluated the KCl–MgCl2 binary mixture as the HTF in CSP solar tower configuration. In 2016, The National Renewable Energy Laboratory (NREL) considered the eutectic binary mixture MgCl2–KCl (37.5–62.5 wt%, 32.0–68.0 mol%, melting temperature of 426°C) as the TES/HTF in the next-generation CSP system.
For the KCl–NaCl binary mixture, Kurnakow (Kurnakow and Zhemchuzhnui, 1907) found that this system forms a complete solid solution which unmixes at lower temperatures. The minimum temperature of 664°C is located between 50–51 mol% KCl.
The MgCl2-NaCl system was experimentally studied by Menge (Menge, 1911) with a eutectic temperature of 430°C for a composition of 56 mol% NaCl and later, Rowe et al. (Rowe et al., 1972) summarized previous results with a eutectic temperature of 450°C for 62 mol% NaCl.
FactSage Thermodynamic Simulation
The binary phase diagrams of KCl–MgCl2, KCl–NaCl, and MgCl2–NaCl have been estimated using the Phase Diagram module as well as the FTsalt database which contains data of the species Mg, K, and Na chloride, and the temperature range was chosen between 350–850°C. The binary phase diagrams of the KCl–MgCl2, KCl–NaCl, and MgCl2–NaCl estimated with FactSage are shown in Figure 2. The compositions mentioned here are all based on mole fractions.
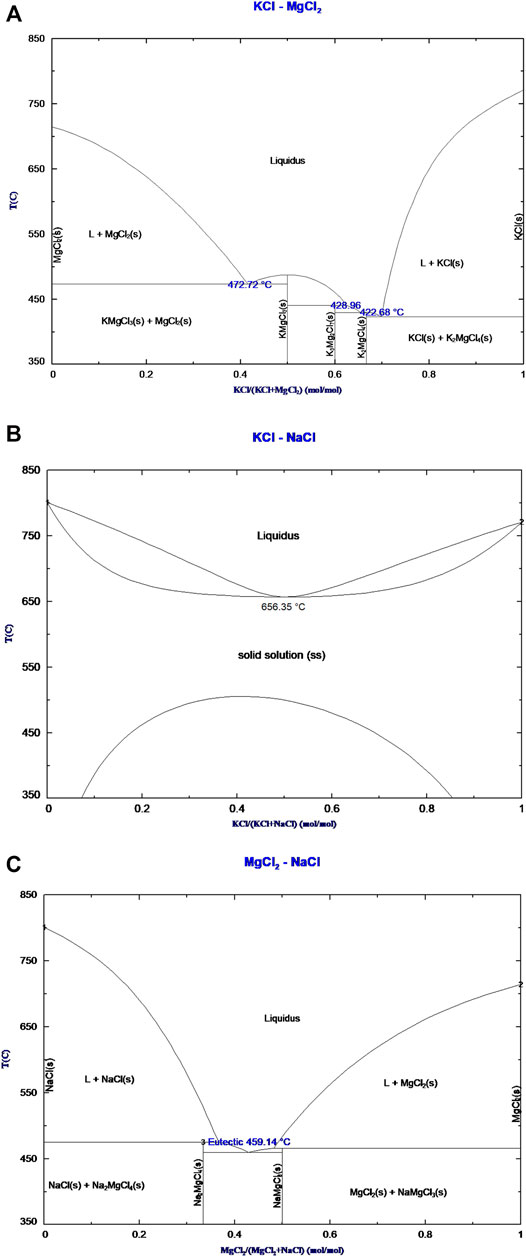
FIGURE 2. Estimated phase diagrams for the binary (A) KCl–MgCl2, (B) KCl–NaCl, and (C) MgCl2–NaCl systems from the FTsalt database using FactSage 7.2 thermodynamic software (FTSalt, 2019).
According to FactSage 7.2, the KCl–MgCl2 system presents three eutectic temperatures at 472.72°C, 428.96 °C, and 422.68°C with the intermetallic KMgCl3, K3Mg2Cl7, and K2MgCl4 respectively, which are in good agreement with the literature data for E1 and E2 (Rowe et al., 1972). Discrepancies in the eutectic temperature for E3 are 8°C lower than the previous reported temperature.
For the KCl–NaCl binary mixture, the formation of the solid solution can be confirmed with a minimum temperature of 656.35°C and a composition of 50.23 mol% NaCl which is good agreement with the previous reported data from Rowe and Kurnakow (Rowe et al., 1972; Kurnakow and Zhemchuzhnui, 1907).
The MgCl2-NaCl system exhibits eutectic temperature at 459.14°C with a composition of 57 mol% NaCl followed by the formation of the compounds NaMgCl3 and Na2MgCl4 that leads to a peritectic transformation at 465 and 474°C, respectively.
Compared to the KCl–NaCl and MgCl2–NaCl phase diagrams, the KCl–MgCl2 has the lowest melting temperature of 422.68°C. These three binary phase diagrams are important as the starting point of the evaluation of the ternary phase diagram.
These results of the binary chloride salt simulation include different eutectic, peritectic, and solid-solution compositions. Those invariant points are summarized in Table 1 at 1 atm in the temperature range 350–850°C.
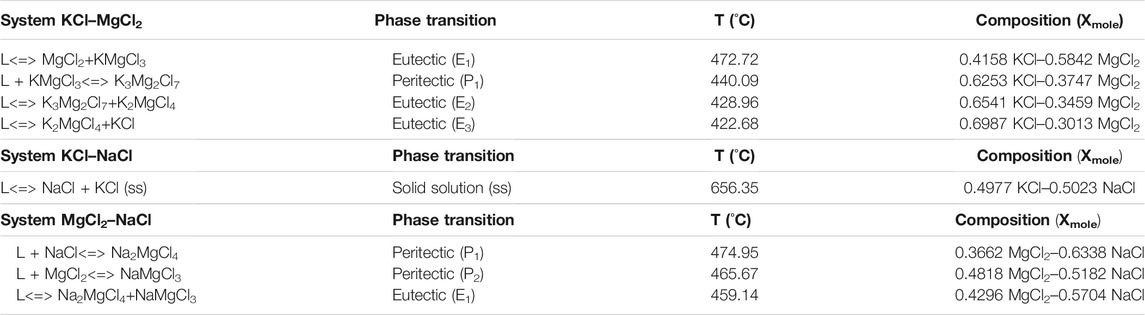
TABLE 1. Invariant reactions of KCl–MgCl2, KCl–NaCl, and MgCl2–NaCl using FactSage thermodynamic simulation (L: Salt liquid).
Result and Discussion of the Ternary System MgCl2–KCl–NaCl
Literature Review
In the first MgCl2–KCl–NaCl ternary diagram presented by Scholich in 1920 (Scholich, 1920), no ternary compounds but incongruently melting binary compounds, such as Na2MgCl4, and the congruently melting binary KMgCl3 were reported. Below 480°C, MgCl2 is insoluble in the (NaCl + KCl) solid solution. This was confirmed by Chartrand and Pelton (Chartrand and Pelton, 2001) who reported evidence of the solid solubility between Na2MgCl4–K2MgCl4 and NaMgCl3–KMgCl3.
Further studies by Bradwell et al. (Bradwell et al., 2012) have used the composition 30 mol% NaCl, 20 mol% KCl, and 50 mol% MgCl2 as the molten electrolyte with a melting temperature of 396°C. In a recent work, Li et al. (Li et al., 2017) investigated a variety of compositions with a minimum temperature between 383–396°C. Xu et al. (Xu et al., 2018b) experimentally studied different compositions of the ternary mixture in the range of 40–50 mol% MgCl2, in which the minimum temperature at 405°C was found for a composition of 27.5 mol% NaCl, 32.5 mol% KCl, and 40 mol% MgCl2. Mohan et al. (Mohan et al., 2018a; Mohan et al., 2018b) experimentally validated the ternary mixture by DSC with a melting temperature of 387°C for the composition reported by Jänecke and Nemecek (Jänecke, 1950; Nemecek et al., 1978) of 45.4 mol% MgCl2, 21.6 mol% KCl, 33 mol% NaCl.
As suggested by Fink et al. (Xu et al., 2018b), the ternary system is incomplete in consequence of missing compounds in the binary systems. Variations of the salt compositions from different literature sources in this mixture lead to slight differences in the minimum melting temperature. For this reason, different liquid–solid transitions have been reported with the solid phases NaMgCl3, KMgCl3, K3Mg2Cl7, Na2MgCl4, and K2MgCl4 which should be considered in order to select the correct minimum temperature and eutectic composition since discrepancies are linked to incomplete identification of those compounds (Baek et al., 2016).
According to Fink and Neil (Fink and Seifert, 1984; Neil et al., 1965), as well as evidenced by the binary MgCl2–NaCl and KCl–MgCl2 systems presented in Figure 2, the presence of incongruently melting compounds leads to peritectic transformations and intermediate compounds. Podlesnyak and Orekhova (Podlesnyak et al., 1987) have experimentally measured the MgCl2–KCl–NaCl ternary system by conductivity techniques in which the ternary compound NaKMgCl4 is reported and associated with a peritectic at 398°C, and Chartrand et al. (Chartrand and Pelton, 2001) measured the KMgCl3–NaCl section in the ternary phase diagram shown in Figure 3. The eutectic KMgCl3/NaCl mixture has the melting temperature of around 400°C and 35–45 mol% NaCl (Chartrand and Pelton, 2001).
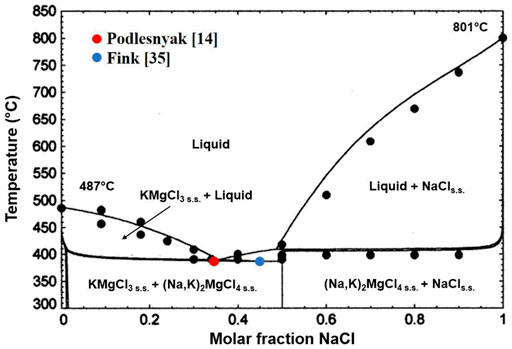
FIGURE 3. KMgCl3/NaCl section in the ternary phase diagram adapted from the study by Chartrand et al. (Chartrand and Pelton, 2001). Two experimental points from the study by Podlesnyak et al. (Podlesnyak et al., 1987) and Fink et al. (Fink and Seifert, 1984) are added to verify this phase diagram.
The salt compositions and minimum melting temperature data from literature are summarized for comparison in Table 2. The discrepancies of minimum melting temperature compositions are relatively high (standard deviation of about 2 mol% for each salt species), while the reported minimum melting temperatures are in the range of 385–400°C.

TABLE 2. Different compositions (in mol%) and minimum melting temperature data following the literature review with phase diagram measurements.
FactSage Thermodynamic Simulation
In this section, a thermodynamic simulation was performed using FactSage 7.2. The ternary phase diagram of MgCl2–KCl–NaCl have been estimated using the Phase Diagram module as well as the FTsalt database which contains data of the species Mg, K, and NaCl, and the temperature range was chosen between 350–850°C. This ternary phase diagram was estimated through the first melting temperature projection and, all compositions are referred on mole fractions.
According to FactSage simulation shown in Figure 4, the zone in which the minimum temperature is located corresponds to a composition of 30.2 mol% NaCl, 22.7 mol% KCl, and 47.1 mol% of MgCl2 with a minimum temperature of 385.4°C compared to the minimum temperature of 383°C for 32.96 mol% NaCl, 21.62 mol% KCl, and 45.42 mol% MgCl2 previously reported by Mohan et al. (Mohan et al., 2018a; Villada et al., 2019), as well as the average and standard deviation of the minimum melting temperature salt composition from Table 2. In addition, the eutectic composition is formed by the compounds KMgCl3 + NaMgCl3 (36–64 mol%) according to our thermodynamic analysis. This implies that the theoretical exact eutectic composition of MgCl2–KCl–NaCl is 50–18–32 mol%. In the KMgCl3/NaCl section from the ternary system, it has also been reported previously that the eutectic point at about 400°C (Fink and Seifert, 1984; Chartrand and Pelton, 2001).
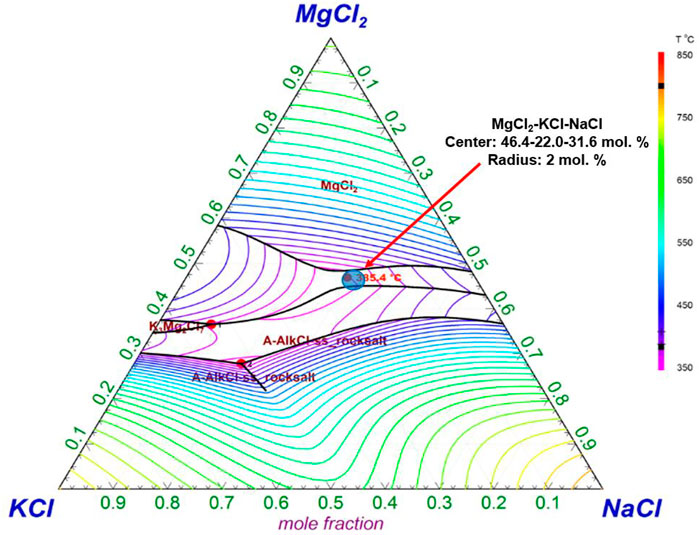
FIGURE 4. Ternary phase diagram of the MgCl2–KCl–NaCl obtained from FactSage 7.2 thermodynamic database in this work (lines: isothermal liquidus projections). The blue circle, which represents the average and standard deviation of the minimum melting temperature salt composition from Table 2, is also presented for comparison.
Differential Scanning Calorimetry Measurements
Since there are limited experimental measurements for the MgCl2–KCl–NaCl ternary system, more detailed discussion is useful in understanding the minimum melting temperature and composition of this mixture. The minimum melting temperature and related eutectic composition of the MgCl2–KCl–NaCl ternary system were validated by DSC experiments on the salt mixtures (Samples A–D) from the literature listed in Table 2 and the predicted salt mixture (Sample E) 30.2 mol% NaCl, 22.7 mol% KCl, and 47.1 mol% of MgCl2 in this work. The DSC heating and solidification curves of the five compositions are given in Figure 5.
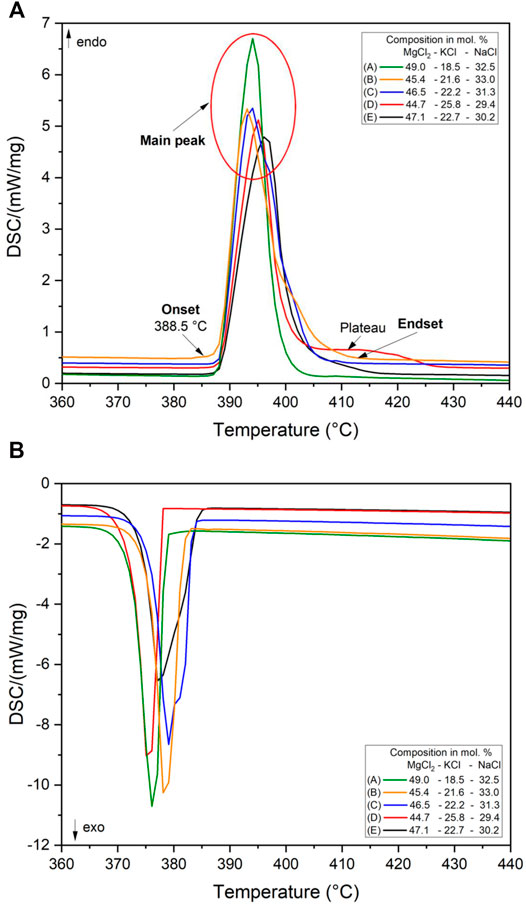
FIGURE 5. DSC heating (A) and solidification (B) curves for the ternary MgCl2–KCl–NaCl mixtures with the minimum melting temperature composition reported and listed above.
Figure 5 (a) shows the melting temperature which is taken from the onset of the main endothermic peak at the heating cycle from the DSC curve, excluding the water losses in the first cycle. There is only one main melting peak at approximately 388.5°C in all curves, as would be expected for a congruent melting compound. Moreover, an overlapping melting peak (Sample B, C, and E) or a plateau (Sample D) can be observed which implies that the salt mixture is not completely eutectic. A similar phenomenon can be observed in the solidification curves. The onset temperature of the main endothermic peak in the heating curve is about 3°C higher than the predicted temperature value by FactSage. The end temperatures of phase transition during heating were between ∼405 and 425°C. The melting temperatures for the five salt compositions measured via DSC in this work are slightly higher than the melting temperatures reported in Table 2. The experimental results show that among these five salt compositions, the composition of Sample A (MgCl2–KCl–NaCl 49.0–18.5–32.5 mol%) is shown to be very close to the exact eutectic composition, as almost no secondary peak can be found in the heating and solidification curve. This also agrees with the fact that the composition of Sample A is closer to the theoretical exact eutectic composition.
Discussion
The melting temperature is a key parameter in the development of next-generation molten chloride salts, and it is represented by the onset of the DSC melting peak for a minimum melting temperature or eutectic mixture. From previous experience in our group with molten nitrate salts, the melting temperature of minimum melting temperature compositions can be successfully determined by differential scanning calorimetry—DSC (e.g., ASTM E794 (Bonk et al., 2018)). For mixtures with a melting range, the liquids temperature is relevant. A simple endset or peak maximum measurement as reported by some authors is misleading due to the influence of heating rates, sample mass, and composition (Yu-ting et al., 2017). Overall, the minimum operating temperature of the MgCl2–KCl–NaCl ternary system for sensible TES applications should not exceed 420°C assuming a melting temperature of about 390°C and the recommended safety margin of 30°C for CSP applications (Bonk et al., 2018). It can also be seen from this work that the composition values for the minimum melting temperature are usually referred to compositions of 44.7–49 mol% MgCl2, 18.5–25.8 mol% KCl, and 29.4–33 mol% NaCl.
The melting temperatures of the ternary MgCl2–KCl–NaCl system with different compositions, including this work, are listed in Table 3. All onset values are mostly around 388.40 ± 0.55°C. The variations of the DSC liquidus temperature for the five different ternary mixtures at 10 K/min are shown in Figure 6, in which the influence of each single chloride from the ternary mixture is notable. Both NaCl and MgCl2 contribute to a decrease in the liquidus temperature, whereas the KCl content increases the liquidus temperature. The results confirm the main conclusion in the last subsection that the composition of Sample A (MgCl2–KCl–NaCl 49.0–18.5–32.5 mol%), which has the highest MgCl2 content and lowest KCl content, is closer to the exact eutectic composition.

TABLE 3. Comparison of salt compositions and melting temperatures of the studied five MgCl2–KCl–NaCl ternary salt mixtures experimentally obtained by DSC at 10°C/min heating rate. The error of the measured temperatures is the standard deviation of three different measurements.
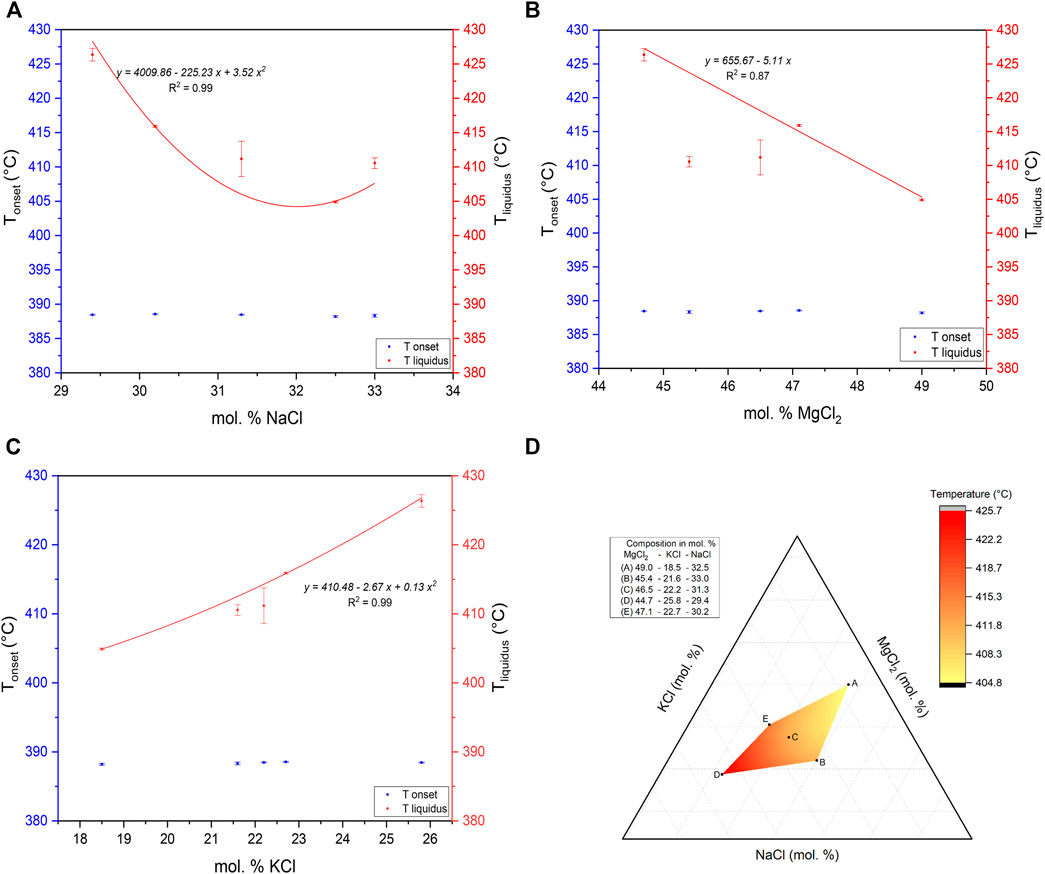
FIGURE 6. Determined onset and liquidus at 10 K/min depending on (A) NaCl, (B) MgCl2, and (C) KCl concentration of DSC measurements for five different ternary mixtures. (D) Detailed amplified triangle section.
Summary and Conclusions
This study investigated the binary and ternary phase diagrams of the MgCl2–KCl–NaCl system using literature data and FactSage simulation and own DSC measurements in order to determine the minimum melting temperature of the MgCl2–KCl–NaCl ternary salt. This mixture is relevant for high temperature TES for next-generation CSP systems.
In this study, five different compositions of the ternary MgCl2–KCl–NaCl salt mixture from literature and simulation of this study have been synthesized in an inert atmosphere (i.e., water impurity in the salt mixtures is neglectable) and tested according to the previous reported compositions, and their experimental melting temperatures were determined by the DSC technique.
After detailed analysis of literature, FactSage simulation and own DSC measurements, a minimum melting composition of MgCl2–KCl–NaCl 49.0–18.5–32.5 mol% or 58.7–17.4–23.9 wt% with the experimental melting temperature of 388.5°C is recommended. In addition, the phase transition that describes this eutectic composition is given by the composition KMgCl3 + NaMgCl3 (36–64 mol%). This implies that the theoretical exact eutectic composition of MgCl2–KCl–NaCl is 50–18–32 mol%, which agrees very well with the recommended composition. Based on these results, the minimum recommended operating temperature for high-temperature TES is 420°C (more than 30°C higher than the melting temperature). However, material cost aspects and larger safety margins may lead to other salt compositions (e.g., less MgCl2 and more NaCl in salt mixture) and operating temperatures higher than 420°C regarding the overall TES costs. Moreover, as the salt composition and melting temperature can be changed (i.e., shift of salt composition) by the by-reactions of the salt with impurities in salt or gas atmosphere during the use, monitoring of salt health is suggested in order to avoid inconveniences such as salt freezing.
Data Availability Statement
The simulation details have been added to the article. Further inquiries can be directed to the corresponding author.
Author Contributions
CV: conceptualization, investigation, writing–original draft, preparation, and visualization. WD: conceptualization, investigation, writing–original draft, preparation, visualization, review and editing, and revision. AB: Writing–review and editing. TB: Writing–review and editing and funding acquisition.
Funding
This research has been performed within the DLR-DAAD fellowship program (Grant number 91716495), which is funded by German Academic Exchange Service (DAAD) and German Aerospace Center (DLR).
Conflict of Interest
The authors declare that the research was conducted in the absence of any commercial or financial relationships that could be construed as a potential conflict of interest.
Publisher’s Note
All claims expressed in this article are solely those of the authors and do not necessarily represent those of their affiliated organizations, or those of the publisher, the editors, and the reviewers. Any product that may be evaluated in this article, or claim that may be made by its manufacturer, is not guaranteed or endorsed by the publisher.
References
Baek, U.-H., Lee, B.-D., Lee, K.-W., Yoon, J.-Y., Han, G.-S., and Han, J.-W. (2016). Removal of Ca from Magnesium Melt by Flux Refining. Mater. Trans. 57, 1156–1164. doi:10.2320/matertrans.m2015426
Bale, C. W., Bélisle, E., Chartrand, P., Decterov, S. A., Eriksson, G., Gheribi, A. E., et al. (2016). FactSage Thermochemical Software and Databases, 2010-2016. Calphad 54, 35–53. doi:10.1016/j.calphad.2016.05.002
Bonk, A., Sau, S., Uranga, N., Hernaiz, M., and Bauer, T. (2018). Advanced Heat Transfer Fluids for Direct Molten Salt Line-Focusing CSP Plants. Prog. Energ. Combustion Sci. 67, 69–87. doi:10.1016/j.pecs.2018.02.002
Bradwell, D. J., Kim, H., Sirk, A. H. C., and Sadoway, D. R. (2012). Magnesium-antimony Liquid Metal Battery for Stationary Energy Storage. J. Am. Chem. Soc. 134, 1895–1897. doi:10.1021/ja209759s
Chartrand, P., and Pelton, A. D. (2001). Thermodynamic Evaluation and Optimization of the LiCl-NaCl-KCl-RbCl-CsCl-MgCl2-CaCl2 System Using the Modified Quasi-Chemical Model. Metallurgical Mater. Trans. A 32, 1361–1383. doi:10.1007/s11661-001-0227-2
Ding, W., and Bauer, T. (2021). Progress in Research and Development of Molten Chloride Salt Technology for Next Generation Concentrated Solar Power Plants. Engineering 7, 334–347. doi:10.1016/j.eng.2020.06.027
Ding, W., Bonk, A., and Bauer, T. (2018). Corrosion Behavior of Metallic Alloys in Molten Chloride Salts for thermal Energy Storage in Concentrated Solar Power Plants: A Review. Front. Chem. Sci. Eng. 12, 564–576. doi:10.1007/s11705-018-1720-0
Du, L., Ding, J., Tian, H., Wang, W., Wei, X., and Song, M. (2017). Thermal Properties and thermal Stability of the Ternary Eutectic Salt NaCl-CaCl2-MgCl2 Used in High-Temperature thermal Energy Storage Process. Appl. Energ. 204, 1225–1230. doi:10.1016/j.apenergy.2017.03.096
Fink, H., and Seifert, H. J. (1984). Quaternary Compounds in the System KCl/NaCl/MgCl2? Thermochim. Acta 72, 195–200. doi:10.1016/0040-6031(84)85073-x
Jänecke, E. (1950). Über das Schmelz‐und Erstarrungsbild des doppelt‐ternären Systemes (K2· Na2· Mg)(Cl2· SO4). Z. für anorganische Chem. 261, 213–225. doi:10.1002/zaac.19502610311
Janz, G. J., Tomkins, R. P. T., Allen, C. B., Downey, J. R., Garner, G. L., Krebs, U., et al. (1975). Molten Salts: Volume 4, Part 2, Chlorides and Mixtures-Electrical Conductance, Density, Viscosity, and Surface Tension Data. J. Phys. Chem. Reference Data 4, 871–1178. doi:10.1063/1.555527
Janz, G. J., and Tomkins, R. P. T. (1981). Physical Properties Data Compilations Relevant to Energy Storage. IV. Molten Salts: Data on Additional Single and Multi-Component Salt Systems. Troy, NY: National Bureau of Standards.
Kurnakow, N. S., and Zhemchuzhnui, S. F. (1907). Isomorphism of Potassium and Sodium Compounds. Z. Anorg. Chem. 52, 186–201. doi:10.1002/zaac.19070520118
Li, Y., Xu, X., Wang, X., Li, P., Hao, Q., and Xiao, B. (2017). Survey and Evaluation of Equations for Thermophysical Properties of Binary/ternary Eutectic Salts from NaCl, KCl, MgCl2, CaCl2, ZnCl2 for Heat Transfer and thermal Storage Fluids in CSP. Solar Energy 152, 57–79. doi:10.1016/j.solener.2017.03.019
Liu, M., Steven Tay, N. H., Bell, S., Belusko, M., Jacob, R., Will, G., et al. (2016). Review on Concentrating Solar Power Plants and New Developments in High Temperature thermal Energy Storage Technologies. Renew. Sust. Energ. Rev. 53, 1411–1432. doi:10.1016/j.rser.2015.09.026
Maksoud, L., and Bauer, T. (2015). Experimental Investigation of Chloride Molten Salts for Thermal Energy Storage Applications, 10th International Conference on Molten Salt Chemistry and Technology. China: Shenyang.
Manohar, S. S., Matthias, A. E., Piyush, S., and Phil, S. (2010). Engineering Database of Liquid Salt Thermophysical and Thermochemical Properties. United States.
Mehos, T. C. M., Vidal, J., Wagner, M., Ma, Z., Ho, C., Kolb, W., et al. (2017). Concentrating Solar Power Gen3 Demonstration Roadmap. Golden, CO: National Renewable Energy Laboratory.
Menge, O. (1911). Die binren Systeme von MgCl2 und CaCl2 mit den Chloriden der Metalle K, Na, Ag, Pb, Cu?, Zn, Sn? und Cd. Z. Anorg. Chem. 72, 162–218. doi:10.1002/zaac.19110720113
Mohan, G., Venkataraman, M. B., and Coventry, J. (2019). Sensible Energy Storage Options for Concentrating Solar Power Plants Operating above 600 °C. Renew. Sust. Energ. Rev. 107, 319–337. doi:10.1016/j.rser.2019.01.062
Mohan, G., Venkataraman, M., Gomez-Vidal, J., and Coventry, J. (2018). Assessment of a Novel Ternary Eutectic Chloride Salt for Next Generation High-Temperature Sensible Heat Storage. Energ. Convers. Manag. 167, 156–164. doi:10.1016/j.enconman.2018.04.100
Mohan, G., Venkataraman, M., Gomez-Vidal, J., and Coventry, J. (2018). Thermo-economic Analysis of High-Temperature Sensible thermal Storage with Different Ternary Eutectic Alkali and Alkaline Earth Metal Chlorides. Solar Energy 176, 350–357. doi:10.1016/j.solener.2018.10.008
Murphy, C., Sun, Y., Cole, W. J., Maclaurin, G. J., Mehos, M. S., and Turchi, C. S. (2019). The Potential Role of Concentrating Solar Power within the Context of DOE's 2030 Solar Cost Targets. United States.
Neil, D. E., Clark, H. M., and Wiswall, R. H. (1965). Thermodynamic Properties of Molten Solutions of MgCl2-KCl, MgCl2-NaCl, and MgCl2-KCl-NaCl. J. Chem. Eng. Data 10, 21–24. doi:10.1021/je60024a009
Nemecek, J. J., Simmons, D. E., and Chubb, T. A. (1978). Demand Sensitive Energy Storage in Molten Salts. Solar Energy 20, 213–217. doi:10.1016/0038-092x(78)90099-3
Podlesnyak, N. P., Orekhova, A. I., and Dieva, E. N., Izv. Vyssh. Uchebn. Zaved. Tsvet. Metall (1987) 105–106.
Polimeni, S., Binotti, M., Moretti, L., and Manzolini, G. (2018). Comparison of Sodium and KCl-MgCl2 as Heat Transfer Fluids in CSP Solar tower with sCO2 Power Cycles. Solar Energy 162, 510–524. doi:10.1016/j.solener.2018.01.046
Robb, K. R., Mulligan, P. L., Yoder, G. L., Smith, K., and Massengale, J. (2019). “Facility to Alleviate Salt Technology Risks (FASTR): Preliminary Design Report with Failure Modes and Effects Analysis,” in Oak Ridge National Lab. (ORNL) (Oak Ridge, TN (United States): Size), 63. doi:10.2172/1615802
Rowe, J. J., Morey, G. W., and Zen, C. S. (1972). in The Quinary Reciprocal Salt System Na,K,Mg,Ca/Cl,SO4 - A Review of the Literature with New Data. Editor U. S. D. o. t. Interior (Washington: United States Government Printing Offide).
Tian, Y., and Zhao, C. Y. (2013). A Review of Solar Collectors and thermal Energy Storage in Solar thermal Applications. Appl. Energ. 104, 538–553. doi:10.1016/j.apenergy.2012.11.051
Vidal, J. C., and Klammer, N. (2019). Molten Chloride Technology Pathway to Meet the U.S. DOE Sunshot Initiative with Gen3 CSP. AIP Conf. Proc. 2126, 080006. doi:10.1063/1.5117601
Villada, C., Ding, W., Bonk, A., and Bauer, T. (2021). Engineering Molten MgCl2-KCl-NaCl Salt for High-Temperature thermal Energy Storage: Review on Salt Properties and Corrosion Control Strategies. Solar Energ. Mater. Solar Cell 232, 111344. doi:10.1016/j.solmat.2021.111344
Villada, C., Ding, W., Bonk, A., and Bauer, T. (2019). Thermodynamic Simulation of Molten Chlorides for Next-Generation Concentrating Solar Power Applications, Eurotherm Seminar 112 Advances in Thermal Energy Storage. Spain: Lleida.
Xu, X., Dehghani, G., Ning, J., and Li, P. (2018). Basic Properties of Eutectic Chloride Salts NaCl-KCl-ZnCl2 and NaCl-KCl-MgCl2 as HTFs and thermal Storage media Measured Using Simultaneous DSC-TGA. Solar Energy 162, 431–441. doi:10.1016/j.solener.2018.01.067
Xu, X., Wang, X., Li, P., Li, Y., Hao, Q., Xiao, B., et al. (2018). Experimental Test of Properties of KCl–MgCl2 Eutectic Molten Salt for Heat Transfer and Thermal Storage Fluid in Concentrated Solar Power Systems. J. Solar Energ. Eng. 140, 40065. doi:10.1115/1.4040065
Keywords: concentrating solar power, eutectic composition, heat transfer fluid, thermal energy storage, phase diagram
Citation: Villada C, Ding W, Bonk A and Bauer T (2022) Simulation-Assisted Determination of the Minimum Melting Temperature Composition of MgCl2–KCl–NaCl Salt Mixture for Next-Generation Molten Salt Thermal Energy Storage. Front. Energy Res. 10:809663. doi: 10.3389/fenrg.2022.809663
Received: 05 November 2021; Accepted: 07 February 2022;
Published: 04 March 2022.
Edited by:
Chuan Li, Beijing University of Technology, ChinaReviewed by:
Terry David Humphries, Curtin University, AustraliaHaochun Zhang, Harbin Institute of Technology, China
Copyright © 2022 Villada, Ding, Bonk and Bauer. This is an open-access article distributed under the terms of the Creative Commons Attribution License (CC BY). The use, distribution or reproduction in other forums is permitted, provided the original author(s) and the copyright owner(s) are credited and that the original publication in this journal is cited, in accordance with accepted academic practice. No use, distribution or reproduction is permitted which does not comply with these terms.
*Correspondence: Wenjin Ding, wenjin.ding@dlr.de