- 1Department of Agricultural and Resource Economics, University of California, Berkeley, Berkeley, CA, United States
- 2Department of Energy and Industrial Enterprises Management Systems, Ural Federal University, Yekaterinburg, Russia
- 3Department of Trade and Finance, Czech University of Life Sciences Prague, Prague, Czechia
Currently, there is a growing number of studies related to assessing the cost-effectiveness of renewable energy projects around the world. This topic is also very relevant for the Russian energy market that is otherwise dominated by oil and gas. The Russian Ministry of Energy forecasts that local power plants running on the renewable energy sources (RES) may not become economically efficient until 2025 or later. However, a research question arises: Is it possible to effectively implement RES projects in Russia without any state support? In order to answer this question, the authors need to assess the economic feasibility of seven scenarios for the construction of a solar power plant in the Orenburg region of Russia. The methodological basis of this work is the widely used in the energy sector classic method of investment analysis based on the calculation of discounted indicators: net present value (NPV), payback period (DPP) and internal rate of return (IRR) of the project. All our calculations are based on industry-specific initial capital investment estimates, energy storage equipment costs, and related annual operating costs. This led to the development of the scenarios on the basis of an analysis of the features of electricity and capacity generation in the Orenburg region, the existing options for joining the trading system of the energy market, energy storage applications, as well as the availability of the Russian government support and funding for the solar energy projects. Our results demonstrate that the economic feasibility of the development of renewable energy in Russia can become a reality. Out of the seven scenarios, three yielded the positive economic outcome (among them there were the two project scenarios without government support). These two projects featured the sale of electricity in retail markets and the installation of an additional energy storage system, despite an almost twofold increase in capital investments. The projects in question achieved the best economic results according to the three calculated criteria. In particular, the scenario that provided for the work on the retail market directly to the energy consumer receives an NPV of more than 1.5 times higher than other projects, an IRR of 10% and pays off at least 3 years faster. Achieving a positive economic result in the wholesale market was possible only in case of state support for the project. In addition, this scenario of the project numerically reached almost identical indicators, as in the case of selling energy on the retail market in the region where the power facility operates: NPV is almost 127 million rubles, IRR is within 13.9%, and DPP is 15 years. Other scenarios that do not provide for the use of energy storage systems or do not have state support for working in the wholesale market are not self-sustaining even during the 25-year life of energy equipment. These results might have practical significance and will be used in developing an approach to creating a profile of regions in terms of the advancements of renewable energy, as well as in developing strategies for the incentives of this sector in Russia.
Introduction
Russia is one of the world leaders in the volume of reserves and the level of extraction of traditional natural resources such as natural gas (Enerdata, 2020), coal (VivaReit, 2020), oil (Shafranik, 2015; Enerdata, 2019), etc. (Shafranik, 2015; Enerdata, 2019; Enerdata, 2020; VivaReit, 2020). The relatively low cost of their extraction, a long period of depletion, as well as the implementation of large-scale government projects for the transportation of such resources, at first glance, make the development of other modern types of energy impractical. However, the examples of countries like China and the United States prove quite the opposite. These countries also possess a significant number of reserves of traditional resources (Enerdata, 2019; Enerdata, 2020; VivaReit, 2020) but manage to successfully develop alternative renewable energy sources. The reports of a number of leading agencies (REN21, 2020; IRENA, 2020) show that despite the intensifying competition, at the beginning of 2020, these countries still remain not only global leaders in terms of investments in the sector, but also in terms of the amount of installed capacity based on renewable energy sources.
This paper assesses the potential of renewable energy deployment in Russia. Unlike many critics some of whom used to call Russia “a gas station masquerading as a country” (McCain, 2020), the authors perceive the great potential for this country to conduct a transition towards the fossil-free economy and energy generation. In addition, the authors of this study believe that the promotion of the renewable energy projects in Russia is not only desirable but is also economically feasible.
Nowadays, the development of renewable energy source in Russia is almost completely supported by the state. Nevertheless, many researchers and policymakers alike agree that the country has enormous potential when it comes to the wind, solar (eprussia.ru, 2020; in-power.ru, 2020) and small hydro energy (Bushuev et al., 2016; geoenergetics.ru, 2020) (eprussia.ru, 2020; in-power.ru, 2020; Bushuev et al., 2016; geoenergetics.ru, 2020). Among the incentive measures implemented by the Russian government at the federal and regional levels, the following ones can be distinguished (Boute, 2012; Ermolenko et al., 2016; Kozlova and Collan, 2016; Lanshina and Kulakov, 2017; Kozlova et al., 2018; Energy bulletin, 2019; Federal Law “On Power Industry”, 2019; Chebotareva et al., 2020):
• Selection of investment projects of renewable energy according to a set of quantitative and qualitative criteria (Chebotareva et al., 2020)
• Conclusion of contracts for the supply of capacity (CSC) based on the results of selection of investment projects (Boute, 2012; Kozlova and Collan, 2016)
• Compensation of up to 50% of the cost of technical connection of renewable energy facilities through subsidies from the federal budget (Kozlova et al., 2018; Chebotareva et al., 2020)
• Establishment of an indicator of the degree of localization of renewable energy generating facilities to provide price and other benefits for the supply of capacity (Federal Law “On Power Industry”, 2019)
• Application of a currency adjustment factor (for renewable energy projects selected in 2013–2014) (Ermolenko et al., 2016; Chebotareva et al., 2020)
• Mandatory purchase of RES facilities by grid companies (5% of the volume of energy losses in grids) for the retail market (Energy bulletin, 2019)
• Establishment of long-term tariffs for electricity from RES facilities for the payback period of investment projects (Lanshina and Kulakov, 2017; Energy bulletin, 2019)
• Provision of tax incentives at the level of individual regions (Energy bulletin, 2019), etс.
The effects that the introduction of renewable energy projects generates are due to many factors, including: Environmental reasons (saving natural fuel, reducing greenhouse gas emissions into the atmosphere) (Child et al., 2018; Valančius et al., 2018), as well as ensuring energy independence and security of states. Nevertheless, discussions about the possibility of achieving economic feasibility of RES projects (Navid et al., 2020; ZareOskouei et al., 2020) for the subsequent transition of this sector to a competitive market remain rather controversial.
Therefore, the key goal of the research presented in the article is to answer the extremely topical question of under what conditions it is possible to economically develop renewable energy in Russia: With or without using support mechanisms from the state; using energy storage systems; working on various types of energy markets in Russia, etc. The results obtained in the course of this research might be of high practical and scientific significance. In addition, these results can be used for modifying and ameliorating the existing approaches to the selection of renewable energy projects that would be eligible for the state funding as well as for the preparation of the new strategies for the development of renewable energy in Russia at the regional level. Furthermore, they can be used by the investors seeking to support the construction of the new generating facilities for the renewable energy.
Thence, the case study used in this article was the construction projects of solar power plant (SPP) with a capacity of 10 MW located in the Orenburg region (a part of the Ural region of Russia). This region has an enormous potential for the development of solar energy that distinguishes it from the rest of the country (e.g., about 300 sunny days a year) (Kommersant, 2020; Rambler, 2020).
The main tasks solved within the framework of this work are as follows: 1) study of the specifics of electricity generation at solar power plants in the region; 2) development of scenarios for the implementation of SPP projects, depending on the use of state support instruments, the use of energy storage devices, as well as the SPP operation markets; 3) a quantitative assessment of the economic efficiency of SPP projects in the Orenburg region in accordance with the developed scenarios.
Based on the results of a practical assessment of seven scenarios for SPP in the Orenburg region, the possibility of achieving a positive economic effect from RES projects has been proven. A similar result was obtained in three cases. Naturally, it was demonstrated by a project implemented under state support. However, it turns out that the best results are achieved when the solar power plant project is carried out without any incentives and state funds. Based on these premises, the following basic conditions for ensuring the economic feasibility of renewable energy projects in Russia can be formulated:
• Sale of electricity mainly in the retail markets of the region of presence or the territory of the consumer while minimizing such volumes in the wholesale market.
• Ensuring high initial capital investments associated with the construction and commissioning of not only a solar power plant, but also a network storage of generated energy, as well as subsequent costs associated with their maintenance.
Figure 1 shows the block diagram for the methodology highlighting various phases of the work.
The work has been carried out in several stages. Stage 1: Conducting a brief literature review on the existing methods and tools for evaluating the effectiveness of renewable energy projects used in modern industry practice. The review is based on the scientific articles published in leading industry journals. In addition, at the first stage, we studied the prospects and features of setting prices for natural fuels in Russia. Stage 2: The choice of a method for assessing the economic efficiency of building a solar power plant is based on studying the experience of applying widely used approaches in industry practice. It also takes into account specific Russian recommendations for such an assessment of energy projects. Stage 3: At this stage, we studied the limiting capabilities of a solar station with a given installed capacity to generate electricity during the day/year, the features of energy consumption in the region under study, as well as the technical, economic and cost specifics of this project. Stage 4: We developed seven possible scenarios for the implementation of the initial project. The scenario criteria included: 1) The use of state support tools for the SPP project, 2) the installation of an additional energy accumulation and storage system, 3) the peculiarities of calculating the cost of initial capital costs and station maintenance costs, due to the factors listed above, and 4) belonging to markets and price zones for the sale of electricity generated by a solar station. Stage 5: An assessment of the economic feasibility of a solar station project is carried out for each of the seven developed scenarios according to such classic investment criteria as net present value, discounted payback period and internal rate of return. Stage 6: We present the conclusions on the possibility and conditions for the effective implementation of solar power projects in Russia.
This paper is structured as follows: Section Literature Review presents a brief literature review on the methods and tools for evaluating the effectiveness of renewable energy projects used in modern industry practice, as well as the prospects and features of setting prices for natural fuels in Russia. Section Methodology presents a method for assessing economic efficiency is conceptually described, based on the calculation of classical indicators of investment analysis, which was subsequently applied to solar energy projects. Section Features of Electricity Generation at Solar Power Plants presents the specifics of electricity generation based on the case study of the SPP in the Orenburg region which is the basis for the development of initial requirements for ensuring the economic efficiency of such projects. Features of the development of solar energy in the Orenburg region, as well as a description of solar power plant projects are presented in Section Orenburg Region Case Study. Details of the full cost of SPP projects, as well as a practical assessment of their economic feasibility and the discussion of the research results are shown in Sections Estimation of the Cost of Construction and Operation of Solar Power Plants in the Orenburg Region and Discussion of Results, respectively. Finally, Section Conclusion is devoted to the main conclusion.
Literature Review
Literature Review of Methods for Assessing the Effectiveness of Renewable Energy Projects
The economic efficiency of renewable energy projects constitutes a very important field of study that attracts many researchers. Thus, in accordance with the guidelines (Guidelines for Evaluating the Effectiveness and Development of Investment Projects and Business Plans in the Electric Power Industry, 2020) developed for capital-intensive Russian energy projects, such an assessment should fully depend on the technological features of the power facility, be determined by the systemic specifics of the joint work of the industry facilities, take into account the strict dependence on the energy consumption regime, and also be guided by the use of existing and projected tariffs on the wholesale market. Therefore, the assessment of projects in the electric power industry should take place from two positions: system-wide and individual. This takes into account the following types of efficiency: Public, commercial, industrial, social, etc.
In foreign practice, where the experience of implementing renewable energy projects is much higher, the performance indicators are more specific. As a rule, the study of the effectiveness of sector projects involves a comprehensive environmental (Landi et al., 2019), technical and economic (Duman and Güler, 2018; Karmaker et al., 2018; Mehrpooya et al., 2018) assessment. Typically, the technical and economic assessment of the effectiveness of various renewable energy projects is carried out using HOMER (Hybrid Optimization Model for Electric Renewables) software (Diemuodeke et al., 2019; Rad et al., 2020), which is complemented by a set of widely used methods. These include MCDM (Multi Criteria Decision Making), Analytic Hierarchy Process, SAW (Simple Additive Weighting), Artificial Bee Colony, PSO (Particle Swarm Optimization) (Rad et al., 2020), TOPSIS (Technique for Order of Preference by Similarity to Ideal Solution) (Diemuodeke et al., 2019; Rad et al., 2020), DEA (Data Envelopment Analysis) (Camioto et al., 2018; Rentizelas et al., 2019), MILP (Mixed Integer Linear Programming) (Obukhov et al., 2019), etc.
The effectiveness of renewable energy projects can be assessed using a plethora of methods and approaches, however the cost-effective method is by far and large the most popular. It calculates the indicators of output cost of energy, total net present cost (Bezmalinović et al., 2013; Duman and Güler, 2018; Karmaker et al., 2018; Rad et al., 2020), return on investment (Savic et al., 2019), and details other types of costs: total costs (Nieves et al., 2019; Rentizelas et al., 2019; Savic et al., 2019; Adefarati and Obikoya, 2020; Zhu et al., 2020), operating and maintenance costs (Duman and Güler, 2018; Diemuodeke et al., 2019; Ghiasi et al., 2020; Rad et al., 2020), capital costs (Duman and Güler, 2018; Diemuodeke et al., 2019; Adefarati and Obikoya, 2020), decommissioning costs (Duman and Güler, 2018), fuel costs (Kobayashi et al., 2019), financial expenses (Caglayan et al., 2019; Gebrehiwot et al., 2019), energy storage costs (Collins et al., 2018; Schlachtberger et al., 2018). The average cost of individual areas of activity within the framework of RES projects is taken into account in: LCOE (Levelized Cost of Energy) (Bezmalinović et al., 2013; Mehrpooya et al., 2018; Zhu et al., 2020), LCOD (Levelized Cost of Delivery) (Baldinelli et al., 2020), LCOS (Levelized Cost of Storage) (Baldinelli et al., 2020) and LCOA (Levelized Cost of Auxiliary Energy Supply) (Baldinelli et al., 2020). The life cycle cost analysis method (Zhang and Yang, 2019) is similar, which divides all costs into three stages: Cost of construction stage, operation and maintenance stage, as well as retirement stage. In addition to the above, the considered costs may include: The costs of decision-making and project design, depreciation deductions, the cost of a feasibility study, financial costs, etc. Another approach in (Duman and Güler, 2018; Savic et al., 2019) provides for the calculation of not only costs, but also revenues from generating electricity and heat (through economic incentive measures) and preventing greenhouse gas emissions (with environmental incentives).
In works (Karmaker et al., 2018; Dia-Core project, 2019; Diemuodeke et al., 2019; Landi et al., 2019; Ghiasi et al., 2020), when assessing the efficiency of renewable energy projects, such classical investment indicators are used as: Net Present Value, Internal Rate of Return, Pay Back Period, as well as cost of equity, cost of debt and debt/equity-ratio.
Other approaches involve the assessment of other specific criteria, for example: Renewable energy fraction and electricity sold (Rad et al., 2020), the volume of energy produced (Karmaker et al., 2018), total energy consumption (Ho, 2018; Nieves et al., 2019; Rentizelas et al., 2019), total CO2 emissions [34, 38, 39, 46, 50].
The method for assessing energy efficiency in (Camioto et al., 2018) is based on optimization of the criteria: A decrease in energy consumption, the use of labor and capital with an increase in gross domestic product and a decrease in carbon dioxide emissions.
In (Collins et al., 2018; Schlachtberger et al., 2018; Kozarcanin et al., 2019; Zhu et al., 2020), when evaluating efficiency, in addition to costs, uncertainties such as weather data and political constraints are taken into account. This is accompanied by the use of weather-driven modeling for scenario analysis. Reducing dependence on weather conditions is based on forecasting the demand for heat and cooling.
Within the framework described (Landi et al., 2019), the efficiency of a RES project for autonomous users is based on the development of a “user’s energy consumption profile” and, in addition to the traditional economic and investment assessment, provides for modeling energy production based on self-consumption and self-sufficiency indicators.
As a result of the presented literature review, it is obvious that there are no uniform approved methodological bases for the assessment to calculate the efficiency of renewable energy projects. Modern researchers apply a combination of different methods, ranging from classical investment analysis, various cost approaches that take into account the assessment of costs and cash flows for projects, specific private indicators, analysis by life cycle stages, and also study a combination of such uncertainty factors as weather data, the presence of political restrictions, in addition, they use a variety of industry-specific software.
Perspectives for Carbon Pricing in Russia
The development of renewable energy should be based not only on the provision of subsidies or other types of state funding, but also on the increase of the costs of the energy generated by the fossil fuels (Oree et al., 2017; Gielen et al., 2019). Both approaches contribute to the mitigation of the climate changes that include the reduction of the greenhouse gas emissions, as well as to increase of the economic competitiveness of new types of energy.
This could be a turning point in global climate action, following U.S. President Joe Biden’s decision to re-join the Paris Agreement the commitment to achieve carbon neutrality by 2050 (with China making a commitment to do the same by 2060 and Russia promising to reduce its CO2 emissions by 33% by 2030) (Fuhrman et al., 2020; Cho, 2021; Strielkowski et al., 2021). The International Monetary Fund calculates that the average global carbon price is just $2 per ton (Panetta, 2021). A higher price for carbon is not just about reducing emissions, but also about diverting economic activity away from carbon-intensive activities. Carbon pricing is internalized, with emitters emitting and paying the price for their carbon emissions in the form of higher taxes and fees (Snyder, 2020). This will fundamentally shape what can and cannot be achieved in the policy area and will fundamentally influence the so-called distributional effects of carbon pricing on economic activity (Li et al., 2018). The cost of carbon pricing is concentrated in certain sectors, including those that invest significant capital in gas-fired power plants. However, price increases can lead to market share losses for these companies and they will pass some of this on to consumers, depending on the market situation. Advocates claim that carbon pricing is the most efficient climate policy tool, because it allows the market to distribute and take into account emissions as in other sectors, such as energy efficiency and renewable energy (Maestre-Andrés et al., 2019; Tagliapietra et al., 2019; Green, 2021). It is apparent that the carbon tax might stimulate economic growth by using tax revenues for reducing the distorted prices and income taxes (Dissanayake et al., 2020; Hassan et al., 2020).
In order to achieve the goals of the Paris Agreement (i.e., limiting the increase in global average temperature to 2°C above pre-industrial levels and pursuing the commitment to limit the temperature increases to 1.5°C) profound changes should be introduced to the fossil fuel market. The least cost path to achieving this goal would be for coal demand to fall immediately, oil demand to slow by the mid-to-late 2020s, and natural gas to fall to about half its current level by mid-2040. This, in turn, would leave the remaining 2C scenario undeveloped for the next decade or so (Bachmann, 2020). Even where a decision is made on the most cost-effective path to 1.5°C, there must be a clear commitment to a low-carbon energy transition from fossil fuels to renewable energy. Most economists believe that the best way to achieve this goal is to move from fossil fuels to renewable energy by the mid-to-late 2040s (Naylor and Higgins, 2017; Jordan and Moore, 2020).
The benefits of reducing greenhouse gas emissions from carbon pricing far outweigh the costs making it a driving force of the energy transition. Businesses, as the driving force of society, must express their support for sound policies that protect businesses’ ability to create jobs and prosper. One of the most important steps in the right direction is the introduction of own internal carbon price to help community combat climate change. A well-designed price for carbon is crucial to incentivize innovation and investment that will drive the transition to a low-carbon economy. A carbon tax pays for cleaner resources, enabling more investment in clean technologies.
The most flexible and effective mechanisms for such support are recognized almost all over the world by economic (market) instruments that create an actual “carbon price”. For example, carbon taxes allow for the external costs that the economy will incur to cope with the effects of such emissions. As of the end of 2020, according to the World Bank, 64 active or starting carbon pricing initiatives have already been developed. These initiatives cover 46 national and 35 subnational jurisdictions and cover 22.3% of global greenhouse gas emissions (World Bank, 2021).
However, despite the obvious advantages, the issue of introducing a carbon tax in Russia as a measure of economic incentives for renewable energy sources remains controversial until now. Many relevant ministries and other organizations have been discussing the economic feasibility of this instrument in Russia since the summer of 2019, when such an initiative was proposed for the first time. Therefore, Russian Union of Industrialists and Entrepreneurs states that the carbon tax would inevitably lead to an increase in tariffs for housing and communal services, accelerate inflation, and inflate the costs of products of the metallurgical complex and transport services (Gorbunov, 2019; AIF, 2019). In addition, the Russian Ministry of Energy argues that an introduction of a charge for greenhouse gas emissions would create preconditions for an increase of the prices of domestic goods and would lead to an increase in tariffs for heat and electricity for the population (Begiashvili, 2019; Slobodyan, 2019). The current methodological unpreparedness for the introduction of such environmental taxes is due to the insufficient level of elaboration of the following fundamental issues: 1) Taking into account the level of absorption of produced carbon in forests and other ecosystems as compensation for emissions; 2) the possibility of implementing forest climate projects as an alternative to the carbon tax; 3) developing and approval of new methods for assessing carbon emissions, their cost, as well as the balance between the volume of actual emissions and their absorption by the forest; 4) assessment of current and prospective forest reserves (Medvedev, 2021). Along with this, a long-term preparation of the population and business is required for the adoption of new taxes and the accompanying increase in additional obligations.
As a result, according to preliminary estimates of experts, the introduction of a carbon tax in Russia is possible no earlier than 2025, а the value of such a tax can be about $ 15 per 1 ton of CO2 equivalent (Pushkarev, 2021).
Methodology
Based on the results obtained in the course of the above literature review of methods for evaluating the effectiveness of RES projects (Section Literature Review of Methods for Assessing the Effectiveness of Renewable Energy Projects), as well as taking into account the methodological features of evaluating directly Russian energy projects (Guidelines for Evaluating the Effectiveness and Development of Investment Projects and Business Plans in the Electric Power Industry, 2020), the authors used the following approach. To study the economic feasibility of building solar power plants in Russia, a classical investment method for assessing the effectiveness of investment projects was used, based on the calculation of the indicators presented below (Dayananda et al., 2002; Baker and English, 2011; Karmaker et al., 2018; Landi et al., 2019; Ghiasi et al., 2020):
• Net present value estimated based on discounted cash flows (DCF).
• Internal rate of return.
• Discounted payback period.
This method will be used both to assess the effectiveness of a project implemented without state support instruments, and in the case of a project based on the application of the CSC mechanism.
The indicator of the net present value of a solar power plant project is calculated by Eq. 1 (Dayananda et al., 2002; Baker and English, 2011):
where
The discounted cash flow for a separate period t is the present value of the future (expected) cash payment for the SPP project to the current time according to Eq. 2 (Dayananda et al., 2002; Baker and English, 2011):
where
The costs of construction and operation of the SPP project include (Agyekum and Velkin, 2020; Morais et al., 2020; Mouaky and Rachek, 2020):
1 Investments for the purchase of equipment for solar power plants (including solar panels, inverters and automatic inverter control system, cables, complete switchgear equipped with switching equipment, means of relay protection of linear automation, measuring transformers and measurement sensors)
2 Costs for design, construction and commissioning of equipment for solar power plant
3 Annual operating maintenance costs
It is assumed that the investment and commissioning costs of a power plant are incurred at the beginning of the project and operating costs at the beginning of each time period. Funds from the SPP activities are mainly related to the sale of electricity and capacity generated by it. It is assumed that these funds will begin to be generated the next year after the investment is made.
The discount rate for the SPP project within the framework of the presented method is determined by Eq. 3:
where s1 is the key rate of the Bank of Russia, equal to 6.25% (calculations were made before February 10, 2020) (cbr.ru, 2019); s2—inflation rate; s3 is the value of the risk of inaccuracy in assessing the technical effectiveness of measures, equal to 5.00% (PolozheniePAO, 2019; Gitelman et al., 2020). This inaccuracy can be performed by the owner of the SPP which is an energy company or a business enterprise seeking to build the SPP and generate profits from its operation. Hence, this risk is solely carried by the SPP owner.
As part of the calculations, it was assumed that the conditional inflation rate is 0.00%. This is due to the peculiarities of the tariff setting for electricity and capacity. The generated income from the activities of SPP is associated with a natural product in the form of electricity and power, therefore the monetary expression of such income does not depend on inflation.
The internal rate of return of the SPP project is calculated as the minimum acceptable level of profitability of the project according to Eq. 4:
The discounted payback period of the SPP project is numerically equal to the number of the time period when the cumulative total of the discounted cash flow becomes positive in accordance with the conditions Eq. 5:
Features of Electricity Generation at Solar Power Plants
The main feature of electricity and capacity generation at solar power plants is the unevenness of electricity generation both during the day (Figure 2) and throughout the year (Figure 3). The data presented is obtained from the SCADA system.
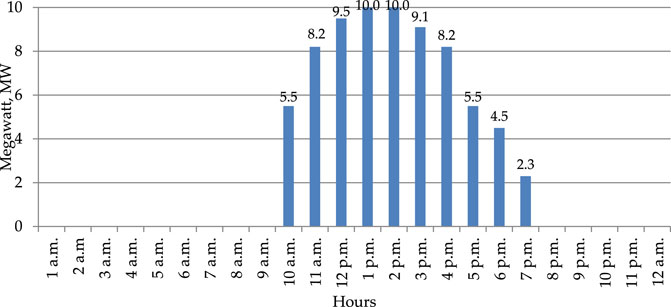
FIGURE 2. Daily irregularity of electricity generation at a 10 MW solar power plant in the Orenburg region (average value, 2019).
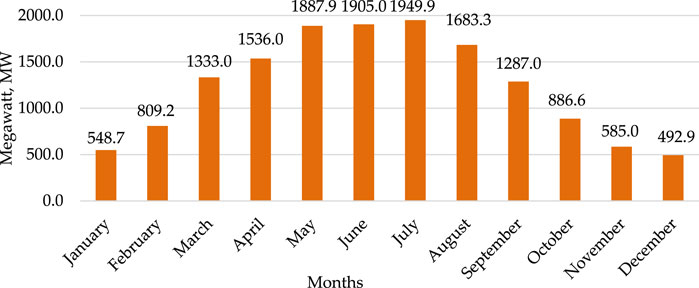
FIGURE 3. Annual irregularity of electricity generation at a 10 MW solar power plant in the Orenburg region (2019).
The distribution of daily and annual electricity generation presented in Figures 2, 3 indicates that the daily hours of maximum generation power of solar stations in the Orenburg region is reached from 12 p.m. up to 3 p.m. mainly in the spring and summer months. For a preliminary assessment of the efficiency of solar power plants, this period should be compared with peak load hours (PLH)—the maximum electricity consumption in a given region. It is during the time period of the PLH that the actual capacity is recorded and paid for in the wholesale electricity and capacity market (WECM). The values of PLH for the Orenburg region are presented in Figure 4 (esplus, 2020).
Hence, during December 2019, PLH in the Orenburg region were in the period from 8 a.m. to 12 p.m. and from 4 to 7 p.m. During the study period, they never coincided with the hours of maximum output of the solar power plant.
As a result, solar power plants operating on the capacity market on a general basis without government support will not be able to receive full payment for capacity on the wholesale market. Thus, the actually affordable income level of solar generation in the capacity market can be no more than 25% of the potential income.
The only available means that will still allow solar power plants to receive payment for capacity in the full potential volume is the use of networked energy storage devices (NES) at SPP. They allow you to accumulate the generated electricity in the hours of maximum generation of solar power plants and deliver it to the grid at the right time, namely during the PLH period. Therefore, in the subsequent practical assessment of the payback of solar power plants, in the absence of government support mechanisms, scenarios involving the installation of solar power plants will be considered. Based on the results of evaluating the distribution of data in Figures 1, 2, it is assumed that the real utilization factor of the installed capacity that can be obtained at solar power plants in the Orenburg region is approximately 17%.
Orenburg Region Case Study
Development of Solar Energy in the Orenburg Region
The Orenburg region is one of the long-term leaders among the regions of Russia, not only in terms of the potential of solar energy (about 300 sunny days a year) (Kommersant, 2020; Rambler, 2020) (Figure 5), but also in terms of the rate of development of this sector in the country.
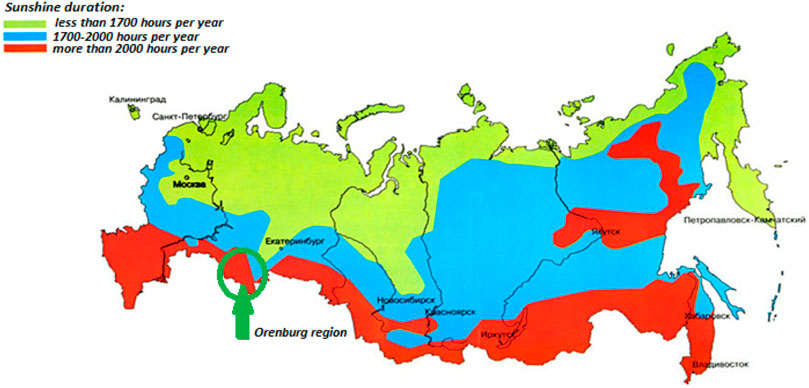
FIGURE 5. Insolation map of Russia (Map of Insolation of Russia, 2019).
At the beginning of 2020, thirteen solar power plants with a total installed capacity of more than 300 MW are already operating in this region (Solar Power Plants in the Orenburg Region, 2019). According to experts’ forecasts (ural56.ru, 2019), by the end of 2020, at least three more SPP with a total installed capacity of about 90 MW should be put into operation. Moreover, some solar stations are hybrid and generate energy together with wind and biogas plants. The main energy companies developing solar energy in the Orenburg region are the Russian Hevel Group, including “Avelar Solar Technology” LLC, and “Renova”, including “T Plus” PJSC, as well as the Finnish company “Fortum” and the French company “Schneider Electric”.
Characteristics of Solar Power Plant Projects in the Orenburg Region
To assess the economic efficiency of the development of solar energy in Russia using the example of the Orenburg region, it is proposed to consider two basic projects for the construction of solar power plants, initially differing depending on the availability of state support.
The first project is a 10 MW solar power plant located in the Orenburg region, the construction and operation of which is planned to be carried out without state support.
The second project is the Orenburg SPP, owned by “Fortum” PJSC, which will be connected to the trading system of the Russian energy market following the selection of renewable energy projects in 2018. It is assumed that power supplies for this project will begin in December 2021. The planned volume of the installed capacity of this station is also 10 MW. As measures of state support for this project, the mechanism of a contract for the supply of capacities is used (geoenergetics.ru, 2020).
It is important to clarify that in order to obtain government support, the selection of investment projects for renewable energy in Russia is carried out on a competitive basis at the level of individual regions (Vygon consulting, 2020). Among the quantitative criteria are:
• The planned volume of electricity production should not exceed 5% of the forecasted losses of grid companies in the region
• Minimization of capital and operating costs that are used in the calculation of electricity tariffs for end users
In turn, the contract for the supply of capacities, which will be applied in the second project, is an economic mechanism of a non-market nature, shifting the costs of modernizing the existing generation and building a new generation based on renewable energy sources to end consumers (Chebotareva et al., 2020).
Estimation of the Cost of Construction and Operation of Solar Power Pants in the Orenburg Region
Initial Cost of the First Project
According to Russian suppliers for solar power plants (altecology.ru, 2019; Solar controller, 2020), the average cost of equipment for solar power plants with an installed capacity of 10 MW is 310 million rubles. The expertly ratedcost of design, delivery, construction, installation of equipment, as well as the purchase of the necessary cable products, wires and a complex electrical switchgear is 100 million rubles (altecology.ru, 2019; Solar controller, 2020).
Thus, the total cost of the first solar power plant project with a capacity of 10 MW, which is planned for implementation without government support, is 410 million rubles.
Initial Cost of the Second Project
Capital costs for the construction and commissioning of the Orenburg SPP which is planned to be connected to the trading system based on the results of the selection of investment projects, are calculated based on the specific value of the planned costs (Competitive selection of renewable energy projects, 2020). The planned value of capital costs per 1 kW of installed capacity of this facility was 0.059 rubles/kW. As a result, when recalculated for the station’s installed capacity of 10 MW, the total capital expenditures amount to 594 million rubles.
Additional costs for design, construction and commissioning of equipment for the SPP are equal to 100 million rubles (altecology.ru, 2019; Solar controller, 2020). This increases the initial cost of the second project to 694 million rubles.
Cost of Network Energy Storage
Based on the results of the analysis of the distribution of peak load hours and maximum power generation in the Orenburg region, it was decided that to increase the efficiency of the SPP, it is possible to use lithium-ion storage network energy storage units with a capacity of 10 MWh. According to ENERZ (ENERZ, 2020), the number of charge-discharge cycles of similar lithium-ion batteries they produce is 5,000 cycles. In the case of operating such batteries with one charge-discharge cycle per day, the service life of such NES is 13–16 years.
The specific cost of lithium-ion batteries per 1 kWh is 0.03 million rubles (ENERZ, 2020). Taking into account the installation and commissioning of the 10 MWh storage battery NES, the project cost may be increased by 300 million rubles.
Operating Costs
The operating costs associated with the maintenance of the solar power plant and the network energy storage device were estimated by experts on the basis of the implementation of projects similar in their characteristics in Russia rubles (altecology.ru, 2019; Solar controller, 2020). As a result, the annual cost of maintenance of a solar power plant with an installed capacity of 10 MW is 0.1 million rubles. The annual cost of maintenance of the lithium-ion battery NES is 0.4 million rubles.
The total construction, commissioning and maintenance costs of the submitted solar energy projects as well as grid storage are detailed in Table 1.
Discussion of Results
Calculations for assessing the efficiency of the SPP were carried out for three types of market:
• In the first price zone on the wholesale electricity and capacity market, which includes the Orenburg region (Annual report on the activities of the organization, 2019; Federal Law “On Power Industry”, 2019)
• In the retail electricity market when placing a solar power plant in the Orenburg region
• In the retail market with the placement of a solar power plant near or on the territory of the consumer to exclude payment for services for the transmission of electricity and capacity of the power grid company
Table 2 contains the results of assessing the annual income of SPP from the sale of electricity and capacity, depending on the markets for providing services.

TABLE 2. Annual income of 10 MW SPP from the sale of electricity and capacity on the energy market, million rubles.
Thus, the following scenarios for connecting solar power plants to the electricity and capacity trading system are possible, which are shown in Table 3.
Also, when assessing the economic efficiency of projects, the following assumptions were made:
• 2020 was adopted as the first time period for investment
• For the first SPP project, implemented without state support, the number of income-generating years is set at 25 years
• For the project of the Orenburg SPP, implemented through the CSC, the state guarantees a payback within 15 years (Vygon consulting, 2020)
• Discount rate is 11.25%
• Numerical values of electricity and capacity sales volumes are determined on the basis of official published data on prices and tariffs for electricity, capacity and services for the transmission of electricity and capacity through electric grids
The results of assessing the main indicators of the economic efficiency of SPP projects in the Orenburg region according to the scenarios are presented in Table 4.
The calculations presented above showed that in three of the seven cases presented, a positive economic effect is achieved on solar energy projects in the Orenburg region. These include the third, fifth and seventh scenarios. For each of them, the main estimated net present value is positive, the internal rate of return is the highest, and the discounted payback period is the lowest among the scenarios presented.
As a result, high economic efficiency is achieved when working in retail markets, both directly in the region of presence (S3), and with the placement of SPP on the consumer’s territory (S5), provided that a network energy storage device is installed and used. The Orenburg SPP which is being implemented with the support of relevant government measures (S7), is naturally among the economically efficient projects. However, the indicators of its economic efficiency practically coincide with the results of the third scenario, but significantly inferior to the fifth. So, when comparing the fifth and seventh scenarios, NPV of the first project is 73.6 million rubles higher, IRR—by 1.4%, and the payback period is reduced by 3 years.
As the main reason for the results obtained, it is important to note the peculiarities of tariff and pricing in the wholesale (first price zone) and retail electricity markets. Even without the installation of NES, the income of the SPP in question in the retail markets significantly exceeds the income in the wholesale market. Installing an energy storage device almost doubles the initial cost of a project, and quadruples its annual maintenance costs. Nevertheless, such investments are reasonable, since they allow achieving high economic efficiency of the operation of such a solar power plant.
Conclusion
All in all, this paper demonstrates that renewable energy has some future in Russia, with solar plants being the most obvious choice given the conditions in this country. We elaborate on a case study of a solar power plant in a southeast Orenburg region of Russia to prove that the economic return on investment of renewable energy projects in this country is not only possible but also economically feasible. The findings show that this outcome is highly possible despite the high cost of equipment for power facilities, both with the application of government support measures and without their use.
Our findings demonstrate that the payback of solar power plants in Russia without government support at the current energy prices can be achieved when they operate at the retail electricity markets. In this case, the payback period does not exceed 15 years. The recoupment of such Russian SPP can be accelerated up to 12 years when it is located in the immediate vicinity of its end consumers and the transmission range is short. In this case, a reduction in the payback period of the SPP is achieved by eliminating the payment for services for the transmission of electricity and power through the electric network and the compromise distribution of the obtained economic effect between the SPP and the consumer (50% by 50%).
To ensure the state-guaranteed 15-year payback period for the Orenburg SPP operating under the CSC, the annual payment for capacity alone should amount to 75 million rubles. If we take into account that on the WECM such a power plant without CSC and without NES would receive about 6.5 million rubles per year for capacity, the state will pay almost 70 million rubles a year to the investors who have built this SPP. The resulting difference by non-market methods will be included in the tariffs for paying for electricity for the population, business and small enterprises.
For investors, it is undoubtedly more profitable to build plants under the terms of a CSC, since in this case state guarantees are provided that the station will pay off within a certain period of time, and the number of risks is significantly reduced at the same capital costs. However, these conditions are categorically disadvantageous for end users of electricity who pay increased electricity tariffs.
Thus, with the rejection of state support for renewable energy and the transition to a competitive market environment, it is possible to achieve a positive economic effect from the implementation of RES projects in Russia. However, this requires the following important conditions to be met:
• Work mainly in the retail electricity and capacity markets, since it is market prices that provide the required level of income for renewable energy facilities
• Minimization of the volume of trade in electricity and capacity in the wholesale markets, since the payback of SPP facilities at the current level of equipment prices is not ensured
• Ensuring the supply of electricity and capacity of SPP under bilateral agreements with consumers of the retail market, but not with a supplier of last resort. The guaranteeing supplier of the retail market purchases electricity and capacity at prices that do not exceed the price of the WECM which do not provide a payback for the SPP
• The need for additional capital investments associated with the acquisition, installation, and subsequently maintenance of network energy storage devices. This is due to the peculiarities of energy generation at SPP: The discrepancy between the hours of maximum generation and peak electricity consumption. For SPP with an installed capacity of 10 MW, costs will increase by almost 2 and 4 times, respectively
• Installation of storage battery NESs at SPPs should ensure the combination of the maximum output of power, equal to the rated power of the generating installation, with the hour of peak consumption, during which the fulfillment of obligations to supply power to the market is recorded
These conditions can be used when developing new and improving existing approaches to the procedure for selecting renewable energy projects for state support. Moreover, these results might be used as the basis for the creation of the regional profiles from the standpoint of energy production and consumption based on the renewable energy sources for the subsequent development of a new strategy for the development of renewable energy not only in Russia but also in other gas- and oil-rich economies. Additionally, the findings might also be used by the investors in the design and comprehensive assessment of the new generating facilities in Russia focusing on the alternative energy sources. From the point of view of subsequent scientific research, a rather promising direction is the assessment of the economic efficiency of the implementation of hybrid renewable energy projects in Russia, i.e., those combining several types of renewable energy sources with traditional energy facilities.
In the context of future research opportunities, it might be interesting to extend the scope of this research to other regions in Russia to assess the feasibility and the economic profitability of the solar power plants in other locations. Furthermore, comparative studies for the results of SPP projects in Russia with those of countries could prove of some value.
Data Availability Statement
The raw data supporting the conclusion of this article will be made available by the authors, without undue reservation.
Author Contributions
Author Contributions: Conceptualization, GR, GC, WS and LS; methodology, GR, GC and JS; software, WS and LS; validation, GR, GC, LS, WS and JS; formal analysis, JS and LS; investigation, GR and WS; resources, GC, LS and JS; data curation, WS and GC; writing—original draft preparation, GR, GC, LS, WS and JS; writing—review and editing, GR, GC, LS, WS and JS; visualization, GC and WS; supervision, GR and WS; project administration, GR, LS and WS; funding acquisition, GC. All authors have read and agreed to the published version of the manuscript.
Conflict of Interest
The authors declare that the research was conducted in the absence of any commercial or financial relationships that could be construed as a potential conflict of interest.
Publisher’s Note
All claims expressed in this article are solely those of the authors and do not necessarily represent those of their affiliated organizations, or those of the publisher, the editors and the reviewers. Any product that may be evaluated in this article, or claim that may be made by its manufacturer, is not guaranteed or endorsed by the publisher.
Abbreviations
CSC, Capacity Supply Contracts; DPP, Discounted Payback Period; IRR, Internal Rate of Return; kW, Kilowatt; kWh, Kilowatt hours; MW, Megawatt; MWh, Megawatt hours; NES, Networked Energy Storage Devices; NPV, Net Present Value; PLH, Peak Load Hours; RES, Renewable Energy Sources; SPP, Solar Power Plant; WECM, Wholesale electricity and capacity market.
References
Adefarati, T., and Obikoya, G. D. (2020). Assessment of Renewable Energy Technologies in a Standalone Microgrid System. Jera 46, 146–167. doi:10.4028/www.scientific.net/jera.46.146
Agyekum, E. B., and Velkin, V. I. (2020). Optimization and Techno-Economic Assessment of Concentrated Solar Power (CSP) in South-Western Africa: A Case Study on Ghana. Sustainable Energ. Tech. Assessments 40, 100763. doi:10.1016/j.seta.2020.100763
Annual report on the activities of the organization (2019). Annual Report on the Activities of the Organization "NP Sovet Rynka" for 2019 [Godovoj Otchet O Deyatel'nosti Organizacii "NP Sovet Rynka" Za 2019 God]. Available online: https://www.np-sr.ru/sites/default/files/1_godovoy_otchet_0.pdf (accessed on 01 June 2020).
AIF (2019). Ministry of Economic Development Abandons Chubais' Proposed Carbon Tax. Available at: https://aif.ru/money/economy/minekonomrazvitiya_otkazalos_ot_predlozhennogo_chubaysom_uglerodnogo_naloga (accessed on May 30, 2021).
altecology.ru (2019). Grid-based Solar Power Plant [Setevaya Solnechnaya Elektrostanciya]. Available online: http://www.altecology.ru/projects/setevaya_solnechnaya_elektrostantsiya/(accessed on December 20, 2019).
Bachmann, T. M. (2020). Considering Environmental Costs of Greenhouse Gas Emissions for Setting a CO2 Tax: A Review. Sci. Total Environ. 720, 137524. doi:10.1016/j.scitotenv.2020.137524
Baker, H. K., and English, P. (2011). Capital Budgeting Valuation: Financial Analysis for Today’s Investment Projects. 1st ed. New Jersey, USA: John Wiley & Sons, 57–196.
Baldinelli, A., Barelli, L., Bidini, G., and Discepoli, G. (2020). Economics of Innovative High Capacity-To-Power Energy Storage Technologies Pointing at 100% Renewable Micro-grids. J. Energ. Storage 28, 101198. doi:10.1016/j.est.2020.101198
Begiashvili, A. (2019). In Russia, They Are Discussing a "carbon Tax". Does it Really Threaten to Increase Utility Tariffs? Available at: https://tjournal.ru/analysis/121814-v-rossii-obsuzhdayut-uglerodnyy-nalog-deystvitelno-li-eto-grozit-povysheniem-tarifov-zhkh ((accessed on May 29, 2021).
Bezmalinović, D., Barbir, F., and Tolj, I. (2013). Techno-economic Analysis of PEM Fuel Cells Role in Photovoltaic-Based Systems for the Remote Base Stations. Int. J. Hydrogen Energ. 38, 417–425. doi:10.1016/j.ijhydene.2012.09.123
Boute, A. (2012). Promoting Renewable Energy through Capacity Markets: An Analysis of the Russian Support Scheme. Energy Policy 46, 68–77. doi:10.1016/j.enpol.2012.03.026
Bushuev, V. V., Gromov, A. I., Belogorev, A. M., and Mastepanov, A. M. (2016). Energy of Russia: A post-strategic View of 50 Years Ahead [Energetika Rossii: Poststrategicheskij Vzglyad Na 50 Let Vpered]. 1st ed. Moscow, Russia: PAC Energiay, 20–32.
Caglayan, D. G., Ryberg, D. S., Heinrichs, H., Linßen, J., Stolten, D., and Robinius, M. (2019). The Techno-Economic Potential of Offshore Wind Energy with Optimized Future Turbine Designs in Europe. Appl. Energ. 255, 113794. doi:10.1016/j.apenergy.2019.113794
Camioto, F. d. C., Mariano, E. B., Santana, N. B., Yamashita, B. D., and Rebelatto, D. A. d. N. (2018). Renewable and Sustainable Energy Efficiency: An Analysis of Latin American Countries. Environ. Prog. Sustain. Energ. 37 (6), 2116–2123. doi:10.1002/ep.12877
cbr.ru (2019). Key Rate of the Bank of Russia [Klyuchevaya Stavka Banka Rossii]. Available online: https://cbr.ru/hd_base/keyrate/(accessed on December 10, 2019).
Chebotareva, G., Strielkowski, W., and Streimikiene, D. (2020). Risk Assessment in Renewable Energy Projects: A Case of Russia. J. Clean. Prod. 269, 122110. doi:10.1016/j.jclepro.2020.122110
Child, M., Koskinen, O., Linnanen, L., and Breyer, C. (2018). Sustainability Guardrails for Energy Scenarios of the Global Energy Transition. Renew. Sustain. Energ. Rev. 91, 321–334. doi:10.1016/j.rser.2018.03.079
Cho, R. (2021). The U.S. Is Back in the Paris Agreement. Now what? Available online: https://news.climate.columbia.edu/2021/02/04/u-s-rejoins-paris-agreement/(accessed June 01, 2021).
Collins, S., Deane, P., Ó Gallachóir, B., Pfenninger, S., and Staffell, I. (2018). Impacts of Inter-annual Wind and Solar Variations on the European Power System. Joule 2 (10), 2076–2090. doi:10.1016/j.joule.2018.06.020
Competitive selection of renewable energy projects (2020). Project Selection Results [Konkursnyj Otbor Proektov VIE. Rezul'taty Otbora Proektov]. Available online: https://www.atsenergo.ru/vie/proresults (accessed on January 20, 2020).
Dayananda, D., Irons, R., Harrison, S., Herbohn, J., Rowland, P., and Rowland, P. (2002). Capital Budgeting: Financial Appraisal of Investment Projects. 1st ed. Cambridge, United Kingdom: Cambridge University Press, 12–83.
Dia-Core project, (2019). The Impact of Risks in Renewable Energy Investment and the Role of Smart Policies. Available online: http://diacore.eu/results/item/enhancing-res-investments-final-report (accessed November 17, 2019).
Diemuodeke, E. O., Addo, A., Oko, C. O. C., Mulugetta, Y., and Ojapah, M. M. (2019). Optimal Mapping of Hybrid Renewable Energy Systems for Locations Using Multi-Criteria Decision-Making Algorithm. Renew. Energ. 134, 461–477. doi:10.1016/j.renene.2018.11.055
Dissanayake, S., Mahadevan, R., and Asafu-Adjaye, J. (2020). Evaluating the Efficiency of Carbon Emissions Policies in a Large Emitting Developing Country. Energy Policy 136, 111080. doi:10.1016/j.enpol.2019.111080
Duman, A. C., and Güler, Ö. (2018). Techno-Economic Analysis of Off-Grid PV/Wind/Fuel Cell Hybrid System Combinations with a Comparison of Regularly and Seasonally Occupied Households. Sustain. Cities Soc. 42, 107–126. doi:10.1016/j.scs.2018.06.029
Enerdata (2020). Statistical Review of World Energy 2020. Available online: https://www.bp.com/en/global/corporate/news-and-insights/press-releases/bp-statistical-review-of-world-energy-2020-published.html (accessed June 23, 2020).
Enerdata (2019). Statistical Yearbook of World Energy 2019 [Statisticheskij Ezhegodnik Mirovoj Energetiki 2019]. Available online: https://yearbook.enerdata.ru/total-energy/world-consumption-statistics.html (accessed April 15, 2020).
Energy bulletin (2019). Promotion of Renewable Energy [Energeticheskij Byulleten'. Stimulirovanie Vozobnovlyaemoj Energetiki]. Available online: http://ac.gov.ru/files/publication/a/3822.pdf (accessed December 20, 2019).
ENERZ (2020). Product [Produkciya]. Available online: http://enerz.ru/produkcija/(accessed on January 15, 2020).
eprussia.ru (2020). Joint Use of Solar and Wind Energy for Small-Scale Power Generation [Sovmestnoe Ispol'zovanie Solnechnoj I Vetrovoj Energii Dlya Energetiki Malyh Moshchnostej]. Available online: https://www.eprussia.ru/epr/216/14774.htm (accessed April 15, 2020).
Ermolenko, G. V., Tolmacheva, I. S., Ryapin, I. Y., Fetisova, Y. A., Matshura, A. A., and Reutiva, A. B. (2016). Handbook on Renewable Energy the European Union [Spravochnik Po Vozobnovlayemoy Energetike Evropeiskogo Souza]. 1st ed. Moscow, Russia: Kanon, 18–25.
esplus (2020). Monthly Report on Peak Hours [Ezhemesyachnyj Otchet Po Chasam Pikovoj Nagruzki]. Available online: https://oren.esplus.ru/(accessed on January 10, 2020).
Federal Law “On Power Industry” (2019). [Federalnyi Zakon “Ob Elektroenergetike”]. Available online: http://www.consultant.ru/document/cons_doc_LAW_41502/(accessed December 12, 2019).
Fuhrman, J., Clarens, A. F., McJeon, H., Patel, P., Doney, S. C., Shobe, W. M., et al. (2020). China’s 2060 Carbon Neutrality Goal Will Require up to 2.5 GtCO2/year of Negative Emissions Technology Deployment. arXiv preprint. arXiv:2010.06723.
Gebrehiwot, K., Mondal, M. A. H., Ringler, C., and Gebremeskel, A. G. (2019). Optimization and Cost-Benefit Assessment of Hybrid Power Systems for Off-Grid Rural Electrification in Ethiopia. Energy 177, 234–246. doi:10.1016/j.energy.2019.04.095
geoenergetics.ru (2020). Development of Renewable Energy in Russia [Razvitie VIE Energetiki V Rossii]. Available online: http://geoenergetics.ru/2019/09/27/razvitie-vie-energetiki-v-rossii/(accessed April 09, 2020).
Ghiasi, M., Esmaeilnamazi, S., Ghiasi, R., and Fathi, M. (2020). Role of Renewable Energy Sources in Evaluating Technical and Economic Efficiency of Power Quality. Technol. Econ. Smart Grids Sustain. Energ. 5 (1), 1. doi:10.1007/s40866-019-0073-1
Gielen, D., Boshell, F., Saygin, D., Bazilian, M. D., Wagner, N., and Gorini, R. (2019). The Role of Renewable Energy in the Global Energy Transformation. Energ. Strategy Rev. 24, 38–50. doi:10.1016/j.esr.2019.01.006
Gitelman, L. D., Kozhevnikov, M. V., Chebotareva, G. S., and Kaimanova, O. A. (2020). Assets Management of Energy Company Based on Risk-Oriented Strategy. WIT Trans. Ecol. Environ. 256, 125–135. (in press). doi:10.2495/EPM200121
Gorbunov, A. (2019). The Introduction of a Carbon Tax Will lead to an Increase in Utility Tariffs. Available at: https://aif.ru/money/economy/vvedenie_uglerodnogo_naloga_privedet_k_rostu_tarifov_zhkh_izvestiya (accessed on May 29, 2021).
Green, J. F. (2021). Does Carbon Pricing Reduce Emissions? A Review of Ex-post Analyses. Environ. Res. Lett. 16 (4), 043004. doi:10.1088/1748-9326/abdae9
Guidelines for Evaluating the Effectiveness and Development of Investment Projects and Business Plans in the Electric Power Industry (2020). [Metodicheskie Rekomendacii Po Ocenke Effektivnosti I Razrabotke Investicionnyh Proektov I Biznes-Planov V Elektroenergetike]. Available online: https://library-full.nadzor-info.ru/doc/51123 (accessed February 10, 2020).
Hassan, M., Oueslati, W., and Rousselière, D. (2020). Environmental Taxes, Reforms and Economic Growth: An Empirical Analysis of Panel Data. Econ. Syst. 44 (3), 100806. doi:10.1016/j.ecosys.2020.100806
Ho, H.-X. T. (2018). “Forecasting of CO2 Emissions, Renewable Energy Consumption and Economic Growth in Vietnam Using Grey Models,” in Proceedings 2018 4th International Conference on Green Technology and Sustainable Development, 452–455. 8595679. doi:10.1109/GTSD.2018.8595679
in-power.ru (2020). Russia Has a Huge Potential for Developing Offshore Wind Energy [Rossiya Obladaet Ogromnym Potencialom Razvitiya Offshornoj Vetroenergetiki]. Available online: https://in-power.ru/news/alternativnayaenergetika/30623-rossija-obladaet-ogromnym-potencialom-razvitija-offshornoi-vetroenerg.html (accessed April 11, 2020).
IRENA (2020). Renewable Capacity Statistics 2020. Available online: https://www.irena.org/publications/2020/Mar/Renewable-Capacity-Statistics-2020 (accessed April 11, 2020).
Jordan, A. J., and Moore, B. (2020). Durable by Design? Policy Feedback in a Changing Climate. Cambridge, United Kingdom: Cambridge University Press.
Karmaker, A. K., Ahmed, M. R., Hossain, M. A., and Sikder, M. M. (2018). Feasibility Assessment & Design of Hybrid Renewable Energy Based Electric Vehicle Charging Station in Bangladesh. Sustain. Cities Soc. 39, 189–202. doi:10.1016/j.scs.2018.02.035
Kobayashi, D., Ohtake, H., Takemoto, K., Hoang, V. N., and Masuta, T. (2019). Reliability and Economic Efficiency in Power System with PV Considering Import Fuel Price Transition. IEEE PES GTD Grand Int. Conf. Exposition Asia 8715868, 752–756. doi:10.1109/GTDAsia.2019.8715868
Kommersant (2020). Turn to the Sun [Povorot K Solncu]. Available online: https://www.kommersant.ru/doc/3585196 (accessed January 10, 2020).
Kozarcanin, S., Liu, H., and Andresen, G. B. (2019). 21st Century Climate Change Impacts on Key Properties of a Large-Scale Renewable-Based Electricity System. Joule 3, 992–1005. doi:10.1016/j.joule.2019.02.001
Kozlova, M., Collan, M., and Luukka, P. (2018). “Russian Mechanism to Support Renewable Energy Investments: Before and after Analysis,” in Computational Methods and Models for Transport. Editors P. Diez, P. Neittaanmäki, J. Periaux, T.T. Tuovinen, and O. Bräysy. 1st ed. (Springer, Cham: Springer International Publishing), 243–252. doi:10.1007/978-3-319-54490-8_15
Kozlova, M., and Collan, M. (2016). Modeling the Effects of the New Russian Capacity Mechanism on Renewable Energy Investments. Energy Policy 95, 350–360. doi:10.1016/j.enpol.2016.05.014
Landi, D., Castorani, V., and Germani, M. (2019). Interactive Energetic, Environmental and Economic Analysis of Renewable Hybrid Energy System. Int. J. Interact. Des. Manuf. 13 (3), 885–899. doi:10.1007/s12008-019-00554-x
Lanshina, T. A., and Kulakov, A. V. (2017). Development of Renewable Energy in China: Studying the Experience and Making Recommendations for Russia. Therm. Eng. 64 (7), 526–533. doi:10.1134/s0040601517070035
Li, M., Zhang, D., Li, C.-T., Mulvaney, K. M., Selin, N. E., and Karplus, V. J. (2018). Air Quality Co-benefits of Carbon Pricing in China. Nat. Clim Change 8 (5), 398–403. doi:10.1038/s41558-018-0139-4
Maestre-Andrés, S., Drews, S., and van den Bergh, J. (2019). Perceived Fairness and Public Acceptability of Carbon Pricing: a Review of the Literature. Clim. Pol. 19 (9), 1186–1204. doi:10.1080/14693062.2019.1639490
Map of Insolation of Russia (2019). [Karta Insolyacii Rossii]. Available online: http://www.mos-invertor.ru/spr5.html ((accessed on June 15, 2019).
McCain, J. (2020). Russia Is a 'gas Station'. Available online: https://www.politico.com/story/2014/03/john-mccain-russia-gas-station-105061 (accessed April 12, 2020).
Medvedev, Y. (2021). What Is the Threat to Russia of the New EU Carbon Tax on Imported Products? Available at: https://rg.ru/2021/01/26/chem-grozit-rossii-novyj-uglerodnyj-nalog-es-na-importnuiu-produkciiu.html (accessed on 05 25, 2021).
Mehrpooya, M., Mohammadi, M., and Ahmadi, E. (2018). Techno-economic-environmental Study of Hybrid Power Supply System: A Case Study in Iran. Sustain. Energ. Tech. Assessments 25, 1–10. doi:10.1016/j.seta.2017.10.007
Morais, P. H. d. S., Lodi, A., Aoki, A. C., and Modesto, M. (2020). Energy, Exergetic and Economic Analyses of a Combined Solar-Biomass-ORC Cooling Cogeneration Systems for a Brazilian Small Plant. Renew. Energ. 157, 1131–1147. doi:10.1016/j.renene.2020.04.147
Mouaky, A., and Rachek, A. (2020). Energetic, Exergetic and Exergeoeconomic Assessment of a Hybrid Solar/biomass Poylgeneration System: A Case Study of a Rural Community in a Semi-arid Climate. Renew. Energ. 158, 280–296. doi:10.1016/j.renene.2020.05.135
Navid, T., Lone, A. H., and Siddiqui, A. S. (2020). “Techno-Economic Feasibility Analysis of Hybrid System,” in Smart Cities—Opportunities and Challenges. Lecture Notes in Civil Engineering. Editors S. Ahmed, S. Abbas, and H. Zia. 1st ed. (Singapore: Springer), 58, 341–352. doi:10.1007/978-981-15-2545-2_29
Naylor, R. L., and Higgins, M. M. (2017). The Political Economy of Biodiesel in an Era of Low Oil Prices. Renew. Sustain. Energ. Rev. 77, 695–705. doi:10.1016/j.rser.2017.04.026
Nieves, J. A., Aristizábal, A. J., Dyner, I., Báez, O., and Ospina, D. H. (2019). Energy Demand and Greenhouse Gas Emissions Analysis in Colombia: A LEAP Model Application. Energy 169, 380–397. doi:10.1016/j.energy.2018.12.051
Obukhov, S., Ibrahim, A., Tolba, M. A., and El-Rifaie, A. M. (2019). Power Balance Management of an Autonomous Hybrid Energy System Based on the Dual-Energy Storage. Energies 12 (24), 4690. doi:10.3390/en12244690
Oree, V., Sayed Hassen, S. Z., and Fleming, P. J. (2017). Generation Expansion Planning Optimisation with Renewable Energy Integration: A Review. Renew. Sustain. Energ. Rev. 69, 790–803. doi:10.1016/j.rser.2016.11.120
Panetta, F. (2021). A Global accord for Sustainable Finance. Available at: https://www.ecb.europa.eu/press/blog/date/2021/html/ecb.blog210511∼7810445372.en.html (accessed May 22, 2021).
Polozhenie PAO (2019). The Position of JSC Rosseti on the Unified Technical Policy in Electric Grid Complex» [Polozhenie PAO «Rosseti» «O Edinoj Tekhnicheskoj Politike V Elektrosetevom Komplekse»]. Available online: https://www.lenenergo.ru/upload/_about/tehnicheskay_politika/polozheniya_pao_rosseti_o_edinoy_tehnicheskoy_politike_v_elektrosetevom_komplekse_utverzhdennuyu_sovetom_direktorov_pao_lenenergo_protokol_ot_20.12.2019___26.pdf (accessed on December 01, 2019).
Pushkarev, G. (2021). Carbon Tax: Innovation May lead to an Increase in Utility Tariffs. Available at: https://www.kp.ru/putevoditel/nauka/uglerodnyj-nalog/(accessed on June 07, 2021).
Rad, M. A. V., Ghasempour, R., Rahdan, P., Mousavi, S., and Arastounia, M. (2020). Techno-economic Analysis of a Hybrid Power System Based on the Cost-Effective Hydrogen Production Method for Rural Electrification, a Case Study in Iran. Energy 190, 116421. doi:10.1016/j.energy.2019.116421
Rambler (2020). Orenburg region - leader of alternative energy [Solnechnaya sistema. Orenburzh'e – lider al'ternativnoj energetiki]. Available online: https://news.rambler.ru/other/42475751-solnechnaya-sistema-orenburzhe-lider-alternativnoy-energetiki/?article_index=1 (accessed March 09, 2020).
REN21 (2020). Renewables 2020 Global Status Report. Available online: https://www.ren21.net/wp-content/uploads/2019/05/gsr_2020_full_report_en.pdf (accessed May 11, 2020).
Rentizelas, A., Melo, I. C., Alves Junior, P. N., Campoli, J. S., and Aparecida do Nascimento Rebelatto, D. (2019). Multi-criteria Efficiency Assessment of International Biomass Supply Chain Pathways Using Data Envelopment Analysis. J. Clean. Prod. 237, 117690. doi:10.1016/j.jclepro.2019.117690
Savic, N., Katic, V., Dumnic, B., Milicevic, D., Corba, Z., and Katic, N. (2019). The Investment Justification Estimate and Techno-Economic and Ecological Aspects Analysis of the University Campus Microgrid. Els 23 (1), 26–38. doi:10.7251/ELS1923026S
Schlachtberger, D. P., Brown, T., Schäfer, M., Schramm, S., and Greiner, M. (2018). Cost Optimal Scenarios of a Future Highly Renewable European Electricity System: Exploring the Influence of Weather Data, Cost Parameters and Policy Constraints. Energy 163, 100–114. doi:10.1016/j.energy.2018.08.070
Shafranik, J. K. (2015). Global Energy and Geopolitics (Russia and the World) [Global'naya Energetika I Geopolitika (Rossiya I Mir)]. 1st ed. Moscow, Russia: PH Energiya, 50–55.
Slobodyan, E. (2019). What Is a Carbon Tax? Available at: https://aif.ru/money/economy/chto_takoe_uglerodnyy_nalog (accessed on May 29, 2021).
Solar Power Plants in the Orenburg Region (2019). Solar Power Plants in the Orenburg Region [Solnechnye Elektrostancii V Orenburgskoj Oblasti]. Available online: https://56stroyka.ru/solnechnyie-elektrostantsii-v-orenburgskoy-oblasti/(accessed on June 15, 2019).
Snyder, B. F. (2020). Beyond the Social Cost of Carbon: Negative Emission Technologies as a Means for Biophysically Setting the price of Carbon. Ambio 49 (9), 1567–1580. doi:10.1007/s13280-019-01301-y
Solar controller (2020). Solar Controller [Solnechnyj Kontroller]. Available online: http://www.invertor.ru/zzz/item/eco_mppt_pro_200_100 (accessed on January 20, 2020).
Strielkowski, W., Sherstobitova, A., Rovny, P., and Evteeva, T. (2021). Increasing Energy Efficiency and Modernization of Energy Systems in Russia: A Review. Energies 14 (11), 3164. doi:10.3390/en14113164
Tagliapietra, S., Zachmann, G., Edenhofer, O., Glachant, J.-M., Linares, P., and Loeschel, A. (2019). The European union Energy Transition: Key Priorities for the Next Five Years. Energy Policy 132, 950–954. doi:10.1016/j.enpol.2019.06.060
ural56.ru (2019). Independent Information and Analytical Website [Nezavisimyj Informacionno-Analiticheskij Sajt]. Available online: https://www.ural56.ru (accessed on December 15, 2019).
Valančius, K., Vilutienė, T., and Rogoža, A. (2018). Analysis of the Payback of Primary Energy and CO2 Emissions in Relation to the Increase of thermal Resistance of a Building. Energy and Buildings 179, 39–48. doi:10.1016/j.enbuild.2018.08.037
VivaReit (2020). Leading Countries in Coal Reserves [Strany-Lidery Po Zapasam Uglya]. Available online: https://vivareit.ru/strany-lidery-po-zapasam-uglya/(accessed June 15, 2020).
Vygon consulting (2020). Support for Renewable Energy on Retail Markets [Podderzhka VIE Na Roznichnyh Rynkah]. Available online: https://vygon.consulting/upload/iblock/411/vygon_consulting_res_retail.pdf (accessed on January 15, 2020).
World Bank (2021). Carbon Pricing Dashboard. Available at: https://carbonpricingdashboard.worldbank.org (accessed May 27, 2021).
Zare Oskouei, M., and Mohammadi-Ivatloo, B. (2020). “Introduction to Techno-Economic Assessment of Renewable Energy Sources,” in Integration of Renewable Energy Sources into the Power Grid through Power Factory. Editors M. Z. Oskouei, and B. Mohammadi-Ivatloo. 1st ed. (Cham: Springer), 1–19. doi:10.1007/978-3-030-44376-4_1
Zhang, C., and Yang, J. (2019). Economic Benefits Assessments of "Coal-To-Electricity" Project in Rural Residents Heating Based on Life Cycle Cost. J. Clean. Prod. 213, 217–224. doi:10.1016/j.jclepro.2018.12.077
Keywords: renewable energy, solar energy, solar power plants, economic efficiency, energy storage, government support, Russia
Citation: Rausser G, Chebotareva G, Smutka L, Strielkowski W and Shiryaeva J (2022) Future Development of Renewable Energy in Russia: A Case of Solar Power. Front. Energy Res. 10:862201. doi: 10.3389/fenrg.2022.862201
Received: 25 January 2022; Accepted: 28 February 2022;
Published: 24 March 2022.
Edited by:
K. Sudhakar, Universiti Malaysia Pahang, MalaysiaReviewed by:
Muhammad Amjad, University of Engineering and Technology, Lahore, PakistanJay Zarnikau, University of Texas at Austin, United States
Copyright © 2022 Rausser, Chebotareva, Smutka, Strielkowski and Shiryaeva. This is an open-access article distributed under the terms of the Creative Commons Attribution License (CC BY). The use, distribution or reproduction in other forums is permitted, provided the original author(s) and the copyright owner(s) are credited and that the original publication in this journal is cited, in accordance with accepted academic practice. No use, distribution or reproduction is permitted which does not comply with these terms.
*Correspondence: Wadim Strielkowski, c3RyaWVsa293c2tpQHBlZi5jenUuY3o=