- 1Applied Mineral and Petrology Special Task Force for Activating Research (AMP STAR), Department of Geology, Faculty of Science, Chulalongkorn University, Bangkok, Thailand
- 2Basin Analysis and Structural Evolution Research Unit (BASE RU), Department of Geology, Faculty of Science, Chulalongkorn University, Bangkok, Thailand
- 3M.Sc. Program in Petroleum Geoscience, Faculty of Science, Chulalongkorn University, Bangkok, Thailand
- 4Department of Mining and Petroleum Engineering, Faculty of Engineering, Chulalongkorn University, Bangkok, Thailand
The main carbon dioxide (CO2) emissions in Thailand come from the energy sector. Gas-based power plants, including natural gas and biogas, are CO2 point sources, and are mostly located in the Khorat Plateau. Geological CO2 storage is an important element in the effort to reduce CO2 emissions from CO2 point sources. This study is a preliminary assessment of the geological CO2 storage potential of the onshore Khorat Plateau. A potential geological formation is screened and ranked in terms of its suitability as a CO2 storage site (storage optimization, risk minimization and feasibility). The results of this screening and ranking indicate that, among the tested sites in this study, the Khorat Permian carbonate is the most suitable for geological CO2 storage, followed by the Khorat Group sandstone, and Khorat evaporite. However, the Khorat Cenozoic basalts are not suitable for geological CO2 storage in the Khorat Plateau. The results from this study should advance the understanding of petroleum exploration and carbon capture and storage technology in Thailand, especially in the Khorat area. However, it should be noted that more subsurface studies are needed, and more criteria should be included in the future to improve the reliability of the assessment of geological CO2 storage potential in the Khorat Plateau.
1 Introduction
Carbon dioxide (CO2) is primary cause of global warming. Over the last few decades, CO2 levels in the atmosphere have been steadily increasing due to rapid economic growth and increasing energy demand. In fact, global CO2 emissions increased by about 54.5% from 1990 to 2013 (International Energy Agency, 2014). As a party to the Paris Agreement, the Thai government has agreed to reduce CO2 emissions by 20% before 2030 (United Nations ESCAP, 2018; Climate Analytics, 2019). The main CO2 emissions in Thailand come from the energy sector, especially from fossil fuel-based power plants, followed by the transport and industry sectors (Figure 1; Energy Policy and Planning Office (EPPO), 2022). Due to increasing global energy demands, fossil fuels are expected to maintain their dominance in future global energy production. Thus, the reduction of CO2 released into the atmosphere from the emission point sources could help to reduce the effects of climate change. Carbon capture and sequestration (CCS) is one approach that could play an important role in reducing CO2 emissions into the atmosphere, and the technique can be applied in Thailand (e.g., Choomkong et al., 2017; Lau et al., 2021; Lau and Ramakrishna, 2021; Zhang et al., 2022). CO2 sequestration is a process that involves four steps: CO2 capture, separation, transportation, and storage. Geological CO2 storage is a promising technique for mitigating the impact of CO2 emissions, and has been successfully demonstrated in many case studies worldwide (Koide et al., 1993; Gale and Freund, 2001; Koide and Yamazaki, 2001; Bachu, 2003; Kempka et al., 2013). CO2 emissions are captured and stored in a subsurface sedimentary basin where a suitable type of storage rock lies at depths ranging from 800 to 1,000 m in a cold regime (geothermal gradient <30°C/km), and from 1,000 to 1,500 m for a warm regime (geothermal gradient >40°C/km) (Bachu, 2003; Mao et al., 2014). The geological CO2 storage can be done in various ways, including trapping in depleted oil and gas reservoirs, solubility trapping in oil and water reservoirs, adsorption trapping in coal beds, cavern trapping in salt structures, or by mineral immobilization (Blunt et al., 1993; Gunter et al., 1997; Bachu, 2003; Dusseault et al., 2004; Riaz and Cinar, 2014; Lau et al., 2021). It is also necessary for the storage to be covered by a good seal rock that prevents the CO2 from leaking to the surface through overburden rocks (Bachu, 2003).
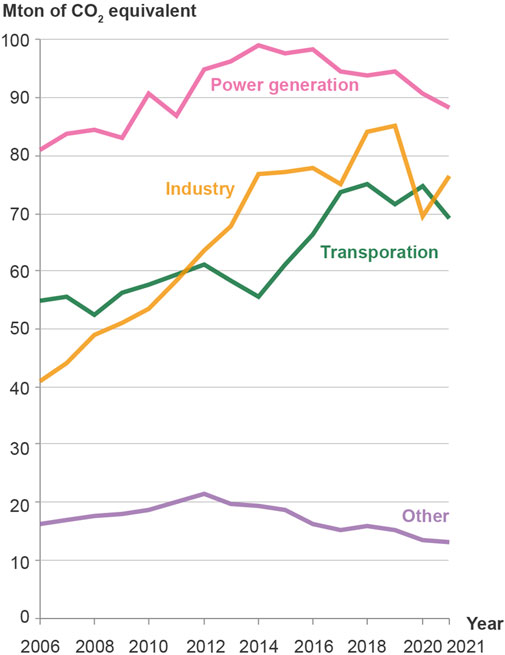
FIGURE 1. Distribution of Thailand’s annual CO2 emissions by sector from the Energy Policy and Planning Office, Ministry of Energy of Thailand (Energy Policy and Planning Office (EPPO), 2022).
Approximately 40% of Thailand’s primary energy consumption is supplied by natural gas, including most of the country’s power generation and growing vehicle fuel usage (Energy Policy and Planning Office (EPPO), 2022). The CO2 emissions from natural gas-based power plants are of significant concern due to the massive discharge of greenhouse gases into the atmosphere, and their subsequent effect on global climate change (Bachu, 2003). The major natural gas-based and biogas-based power plants in Thailand are in northeastern Thailand or the Khorat Plateau (Figure 2), which extends over an area of 200,000 km2 and consists of two sedimentary-filled basins known as Sakon Nakhon basin in the north and Khorat basin in the south (El Tabakh et al., 1999; Sone and Metcalfe, 2008; Tan et al., 2010). These two basins overlay Carboniferous to Triassic sequences, which constitute a main petroleum exploration target in the area (Figure 3). The Upper Paleozoic and Mesozoic sequences in the Khorat Plateau have been explored for petroleum and gas fields, which have been discovered in the Khorat Plateau (Figures 2, 3). To date, two commercial gas fields, Nam Phong and Phu Horm, are producing from Permian carbonate reservoirs (Figure 3; Booth, 1998; Sattayarak, 2005; Chantong et al., 2013). Thus, an existing gas pipeline network, suitable local drilling rigs, and potential active petroleum companies could facilitate CCS development in the future.
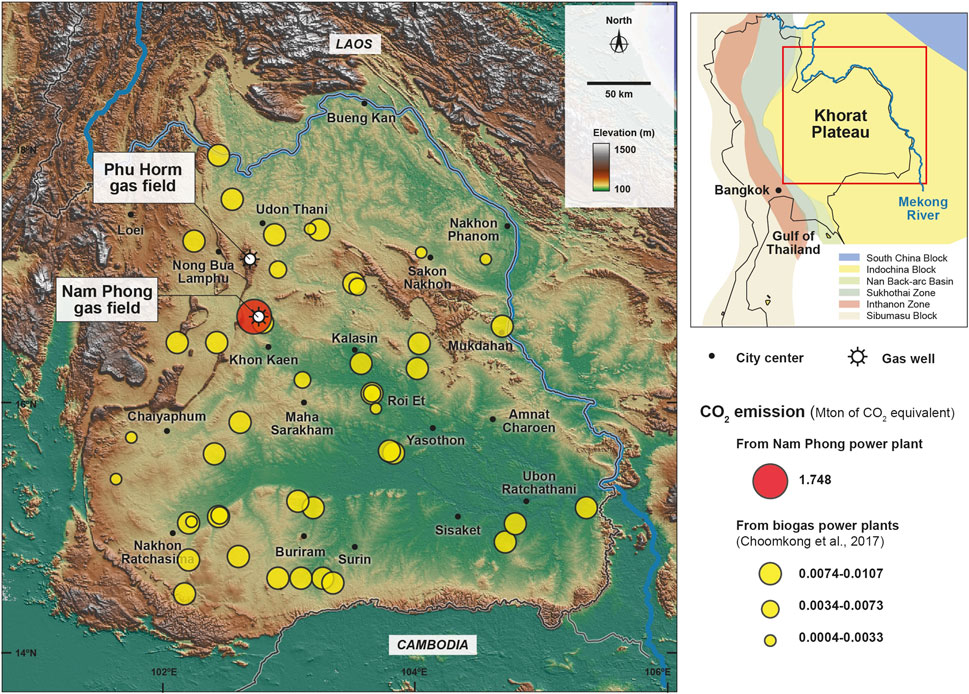
FIGURE 2. Location map of the Khorat Plateau, northeast Thailand, showing the major CO2 emission point sources for geological storage (CO2 emission data sourced from Choomkong et al., 2017).
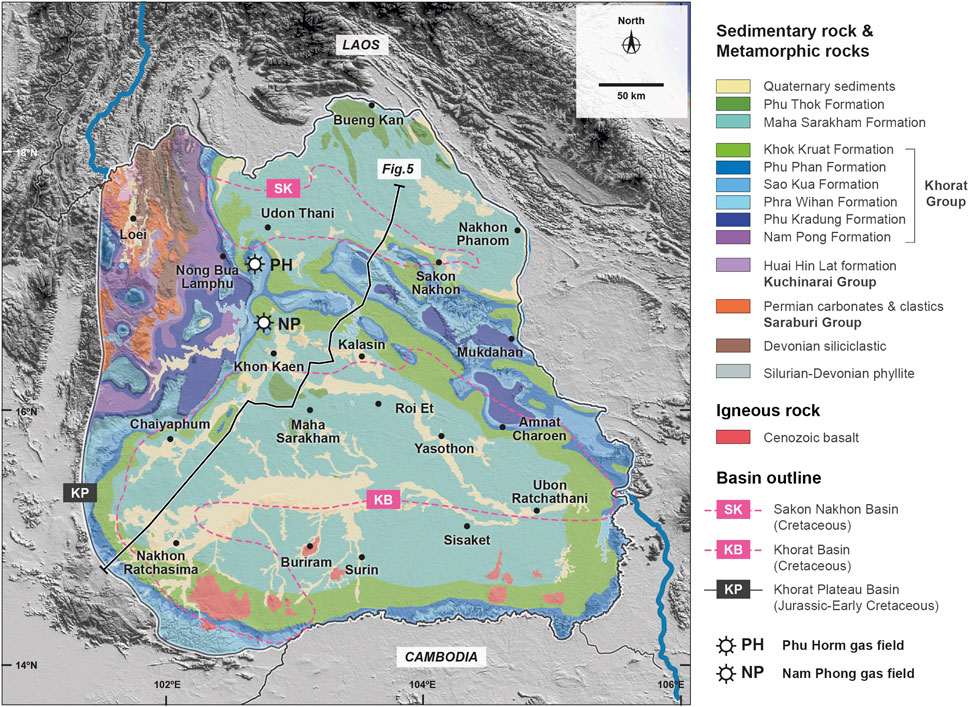
FIGURE 3. Simplified geological map of the Khorat Plateau, with the main producing gas fields (based on Geological Survey Division, 1999).
This study aims to provide a preliminary assessment, from existing subsurface and surface published data from an extensive review of peer-reviewed journals, conference proceedings, and published reports, of the suitability for geological CO2 storage of the geological framework and stratigraphy in the Khorat Plateau. This is the first attempt to focus on the existence of these suitable lithologies (e.g., salt and alkaline igneous rocks) and petroleum reservoirs within the Khorat Plateau for geological CO2 storage.
2 CO2 emission point sources in Khorat Plateau
CO2 emission point sources are considered as either mobile or stationary point sources (Zhang et al., 2022). Mobile CO2 sources (e.g., cars, ships, and planes) are difficult to capture, while stationary CO2 sources (e.g., production factories and processing plants) could be captured by CCS technologies (Zhang et al., 2022). Stationary CO2 sources can come from various sectors, including cement factories, refineries, iron and steel mills, petrochemical plants, gas-based power plants and coal-based power plants. This study focuses on an assessment of subsurface geological storage of CO2 in the Khorat Plateau. Thus, only stationary CO2 sources from the energy and industry sectors are included for evaluation in this study.
Industrial plants and gas-based power plants are potential CO2 emission point sources in the Khorat Plateau , including those powered by natural gas and biogas (Figure 2). In addition, the biggest CO2 emitter in the Khorat Plateau is potentially the natural gas-based power plant in Khon Kaen (2.13 Mtpa), which is the Nam Phong power plant (Figure 2; Zhang et al., 2022). This is presently the largest power plant in the Khorat Plateau and the only power plant that uses natural gas fuel to generate electricity in Thailand. The gas fuel that is used to supply the Nam Phong power plant comes from the gas fields in Khon Kaen, including Nam Phong and Phu Horm fields (Figure 2).
3 Geological background
The Khorat Plateau is part of Sundaland, which was originally part of the northern margin of the supercontinent Gondwana, but which drifted to Eurasia during the Late Paleozoic through the Mesozoic (Barber et al., 2011; Metcalfe, 2011; Morley, 2012; Morley et al., 2013). The Khorat Plateau is believed to have formed as a foreland basin in front of the orogenic belts created by the Nan-Uttaradit Suture Zone in central-northern Thailand (Figure 3; Smith et al., 1996; Carter and Bristow, 2003), and has been controlled by the rift, drift, collision, and post-collision history of the Gondwana continental fragments. The present-day geothermal gradient in the Khorat Plateau is low, at about 28 C/km, based on data from the Si That petroleum exploration well.
The Early to Middle Triassic collision between the Shan Thai and the Indochina microplates along the Nan-Uttaradit Suture was followed by Late Triassic tectonic relaxation or extension, which created half-graben basins, where the non-marine sequences were deposited (Drumm et al., 1993). Fluvial and lacustrine facies filled these basins with conglomerates, sandstones, and mudstones that range in age from the latest Triassic to late-Early Cretaceous (Drumm et al., 1993). Provenance sources of the Khorat rocks in Thailand comprise material from erosion of the late-Palaeozoic rocks exposed in the Nan-Uttaradit Suture Zone (Bunopas and Vella, 1978; Hutchison, 1989), and possibly from eastern Laos and central Vietnam (Drumm et al., 1993). In addition, the Qinling Orogenic Belt in China is also believed to be a provenance source for the Khorat Plateau sediments (Carter and Bristow, 2003).
There was a major displacement of the Khorat Plateau along the northwest-trending Red River Fault of about 950 ± 150 km, with a 20–25° clockwise rotation relative to the South China plate during Early Cretaceous through to Early Neogene times (Charusiri et al., 2006). Inversion structure has been observed and is proposed to have occurred during the Mid-Cretaceous in a continental collision to the west between Western Burma and Shan Thai (Metcalfe, 1996). The Khorat sediments were intruded by Cretaceous granites (Campanian and Cenomanian) that had resulted from thermal subsidence of the Khorat Plateau (Smith et al., 1996).
During the Late Cretaceous to Early Paleocene, compression from the northeast due to continental collision of the Indochina microplate with the southeast China plate, and from back-arc compression of the Shan Thai microplate to the west, resulted in uplift and erosion of about 3,000–3,500 m of the Upper Cretaceous sequences, and the formation of the NW-SE-trending Phu Phan anticlinorium in the central part of the Khorat Plateau (Cooper et al., 1989; Bunopas and Vella, 1992; Mouret et al., 1993; El Tabakh et al., 1999). During the Cretaceous, the Indochina microplate is suggested to have been located near 20°N latitude, with arid climatic conditions (Achache et al., 1983). The compression regime that created the inversion structures in this area is thought to have continued until the earliest Paleocene. From the Paleocene time onwards, the transtension regime was dominant in the South China Sea, Thailand Central Plain, and Gulf of Thailand, creating rift basins during the Oligocene-Miocene (Daly et al., 1991). However, there is no evidence of a rift basin or Tertiary sediments, including a lack of major syn-depositional faulting, in the Khorat Plateau (Smith et al., 1996). Finally, the Pliocene–Pleistocene basalts were subsequently extruded, and unconformably overlay the Cretaceous sequences.
3.1 Regional tectonic history
The continental fragments of Indochina and South China that formed the Khorat Plateau were separated from the northern margin of Gondwana in the Late Devonian and drifted over the Palaeotethys Ocean though the Late Permian. The two continental fragments, Indochina and South China, then collided in the Late Permian (Hutchison, 1989; Metcalfe, 1996).
There are five depositional sequences that are separated by unconformities related to major orogenic events (Figures 4, 5; Booth and Sattayarak, 2011). The Indosinian I unconformity is the oldest unconformity related to the Permo-Triassic (P–T) boundary (Figures 4, 5), and resulted from compressional uplift and erosion events of the Nan-Uttaradit Suture between Indochina and Shan Thai that had been fully sutured in the Middle Triassic (Bunopas and Vella, 1983; Cooper et al., 1989; Metcalfe, 1996). In addition, Indosinian I is the most regionally extensive, as recognized from subsurface data, and is followed by the Indosinian II unconformity, which is generally believed to be from the Late Triassic (Figures 4, 5; Sone and Metcalfe, 2008; Metcalfe, 2011; Metcalfe, 2013). However, the Late Jurassic time has been recently been proposed for the closure of the Indosinian II unconformity (Ahrendt et al., 1993). A crustal extension event in the Late Triassic caused the development of localized half-graben structures between the Indosinian I and II unconformities in the Khorat area (Cooper et al., 1989; Sattayarak et al., 1989; Booth and Sattayarak, 2011). These half-grabens were then filled by Triassic sediments, which are preserved as the Kuchinarai Group (Figures 4, 5).
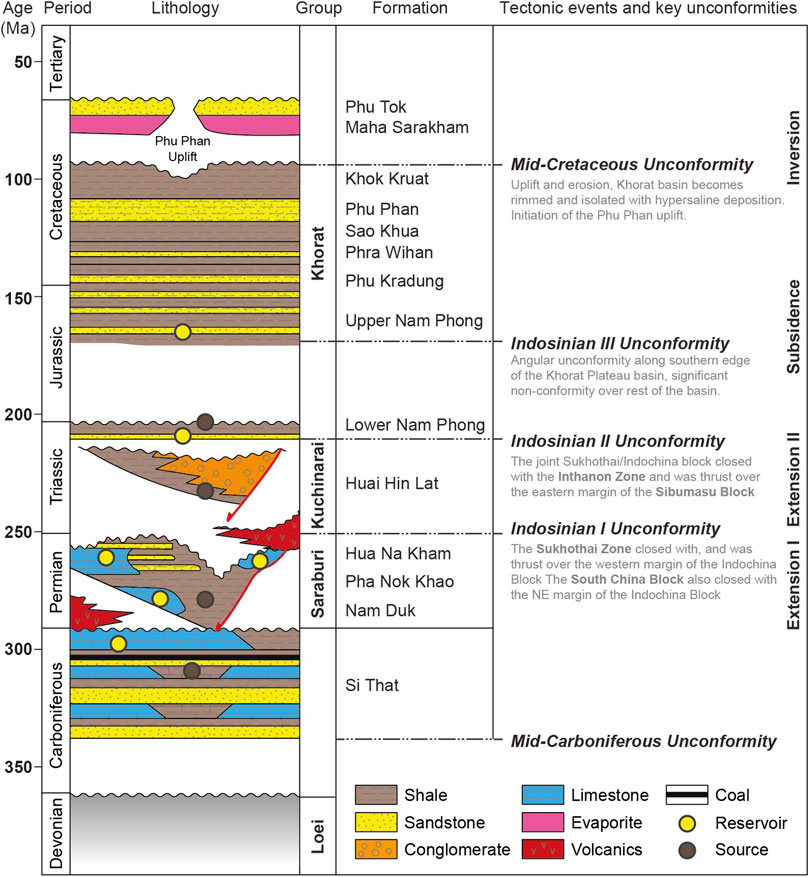
FIGURE 4. Stratigraphic framework of the Khorat Plateau (modified from Sattayarak et al., 1989; Koysamran and Comrie-Smith, 2011; Racey, 2011; Schenk et al., 2017). The potential reservoir and source rocks are indicated as yellow and brown dots, respectively.
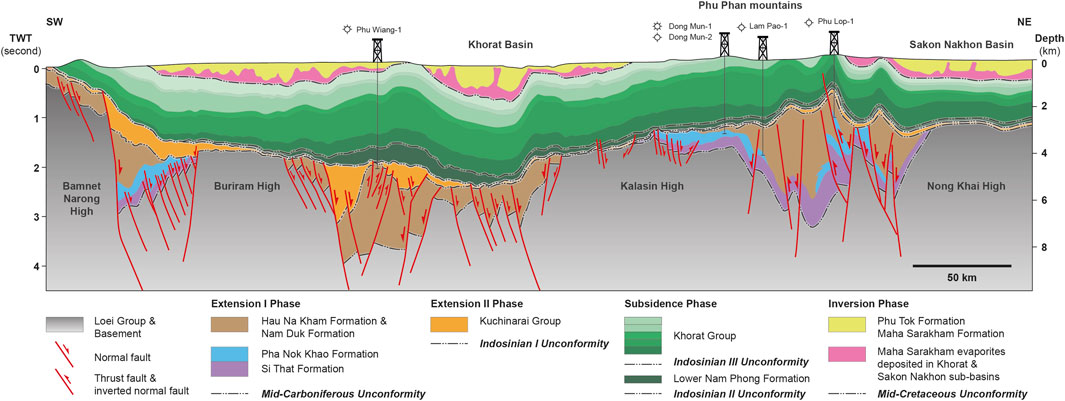
FIGURE 5. Stratigraphic cross sections across the Khorat Plateau, showing the major lithological and tectonic units in the subsurface (modified from Booth and Sattayarak, 2011; Minezaki et al., 2019). The distribution of the Khorat and Sakon Nakhon basins is also shown in this section.
The Khorat Plateau area then became relatively stable and was dominated by fluvial and floodplain deposits in the foreland basin from the Jurassic to Early Cretaceous (Horiuchi et al., 2012), when thick continental sediments (Khorat Group) were slightly deformed as long-wavelength folds and overlaid the Indosinian III unconformity (Figures 4, 5). In the Late Jurassic to Mid-Cretaceous formations, the Khorat Plateau exhibits thermal subsidence with an almost complete absence of syn-depositional faulting. The long-wavelength folding structures of the rock strata in the Khorat Plateau resulted from a combination of Mid-Cretaceous and Tertiary episodes of thick-skinned inversion (Figures 4, 5; Smith and Stokes, 1997) that formed during the suturing of West Burma with Shan Thai at the Early Cretaceous, which would have started a compression regime in the Khorat area (Metcalfe, 1996). As a result of this compression during the Mid-Cretaceous, the Phu Phan range was uplifted, forming two sedimentary-filled basins inside the Plateau: the Sakon Nakhon and Khorat basins (Booth and Sattayarak, 2011). However, several studies argue that the Phu Phan range was actually uplifted during the Tertiary (e.g., Chuaviroj, 1997; Meesook, 2000).
Regional uplift and second inversion events during the continental collision between India and Eurasia (Himalayan orogeny) in the early Paleocene are proposed to have been the most recent uplift events (Mouret et al., 1993; Smith et al., 1996; Chuaviroj, 1997). The deformation events in this period, including the Phu Phan Uplift, strike–slip faults, thrusts, and folds on the southern and eastern margins affected the Khorat rocks (Morley, 2012). The uplift event would have resulted in a height, at the start of the regional erosion period, of around 1,500 m in the Khorat area (Mouret et al., 1993). Today, the maximum thickness of rock strata is about 4,000 m at the Phu Phan Uplift (Mouret et al., 1993; Canham et al., 1996).
3.2 Stratigraphic framework
The Khorat Plateau comprises sedimentary formations from the Carboniferous to Cretaceous ages (Figures 4, 5). The Upper Paleozoic rocks form the basement for the Khorat Plateau and comprise Devonian to Carboniferous mixed siliciclastic-carbonate sediments, the Permian Pha Nok Khao carbonate platform, and the Permian siliciclastic sediments of the Hau Na Kam and Nam Duk Formations. The Pha Nok Khao comprises extensive, distally-steepened ramps composed of varying proportions of carbonates and lesser siliciclastics, and is the main target for petroleum reservoir in the Khorat Plateau. The Pha Nok Khao Formation overlies unconformably on the Devonian-Early Carboniferous sandstone, mudstone, limestone, and coal of the Si That Formation (Figures 4, 5; Burret et al., 2014). The Upper Paleozoic strata are separated from overlying Mesozoic sediments by the Indosinian I unconformity (Figures 4, 5). Moreover, most Paleozoic strata in the Khorat Plateau and adjacent areas were widely deformed during the Indosinian I event (Ridd et al., 2011). The Huai Hin Lat Formation comprises non-marine petroleum source rock distributed along the western margin of the Khorat Plateau (Figures 4, 5; Booth, 1998; Booth and Sattayarak, 2011). The depositional environment of the Huai Hin Lat Formation is broadly interpreted to have been a fluvial and lacustrine setting during the extension and half-graben development (Chonglakmani and Sattayarak, 1978). The age of the Huai Hin Lat Formation is Late Triassic (Norian), based on the Estheria fauna, spore, and pollen found there (Haile, 1973; Kobayashi, 1975; Kon’no and Asama, 1973; Chonglakmani and Sattayarak, 1978).
Cretaceous rocks are widespread in the Khorat Plateau, and all are clastic non-marine sediments (Figures 4, 5). They are called the Khorat Group and are composed of (from the oldest to youngest) the Phu Kradung, Phra Wihan, Sao Khua, Phu Phan, Khok Kruat, Maha Sarakham, and Phu Tok Formations (Figure 4).
The Nam Phong Formation varies in thickness from 2,500 m to several meters (Nulay and Arjwech, 2021). The area of maximum thickness is located in the central portion of the Khorat Plateau, and gradually thins or disappears along the flanks of the plateau. The Nam Phong Formation is divided into the Upper and Lower Nam Phong Formations, which are separated by an unconformity (Figure 4). The Lower Nam Phong Formation dates to the Late Triassic (Rhaetian), based on palynology, while the Upper Nam Phong Formation is considered to be no older than Early Jurassic (Pliensbachian), based on petroleum-well correlation (Racey and Goodall, 2009). The Formation consists of reddish-brown micaceous sandstone, conglomerate, siltstone, and mudstone. The depositional environment is considered to be fluviatile, with deposit activity commencing after the end of the deformation event (Indosinian II) of the half-graben basin of the Huai Hin Lat Formation (Nulay and Arjwech, 2021).
The Phu Kradung Formation lies conformably on the upper Nam Phong, separated by a hiatus, and it varies in thickness from 1,200 m in the basin center to around 500 m on the basin flanks (Racey, 2009; Nulay and Arjwech, 2021). The hiatus at the base of the Phu Kradung Formation is generally considered to be the base of the Khorat Group (e.g., Racey, 2009; Booth and Sattayarak, 2011). However, some authors place the base of the Khorat Group at the base of the Lower Nam Phong Formation (e.g., Meesook and Saengsrichan, 2011). The Phu Kradung Formation is dominated by maroon siltstone and claystone, while sandstone and conglomerate are subordinate in the Phu Kradung Formation (Meesook and Saengsrichan, 2011). Coarsening-upward sequences have been observed throughout the Phu Kradung Formation from subsurface study (Booth and Sattayarak, 2011). Thus, the environmental deposition is considered to be alluvial at the lower part and grade into fluvial in the upper part, under what was once a meandering river system in a semi-arid paleoclimate (Racey et al., 1996; Booth and Sattayarak, 2011; Meesook and Saengsrichan, 2011). The age of the Phu Kradung Formation is Late Jurassic to Early Cretaceous, based on palynology (Racey and Goodall, 2009).
The Phra Wihan Formation lies conformably on the Phu Kradung Formation and varies in thickness from around 50–300 m (Nulay and Arjwech, 2021). The Phra Wihan Formation is generally composed of white, coarse-grained, well-sorted arkosic to orthoquartzitic sandstone, but siltstone, mudstone, and conglomerate are also found (Nulay and Arjwech, 2021). Because of its dominant sandstone beds, the Phra Wihan Formation is believed to have been deposited by a high energy braided river, with a subordinate meandering river in a subtropical to tropical palaeoclimate during the Early Cretaceous (Barriasian to Barremian), based on palynology (Racey et al., 1996; Meesook, 2000; Racey and Goodall, 2009; Horiuchi et al., 2012).
The Sao Khua Formation lies gradationally and conformably on the Phra Wihan Formation, and varies widely in thickness from around 100–700 m (Nulay and Arjwech, 2021). The Sao Khua Formation is generally composed of reddish-brown conglomeratic sandstone with calcrete and silcrete layers at the base of the Formation (Meesook, 2000). Siltstone and mudstone are also found within this formation, which is believed to have been deposited by a low-energy meandering river and extensive flooding under a warm and cool semi-arid paleoclimate. The age of the Sao Khua Formation is the Early Cretaceous (Hauterivian to Late Barremian), based on palynology, and vertebrate and bivalve fossils (Racey and Goodall, 2009; Nulay and Arjwech, 2021).
The Phu Phan Formation lies conformably on the Sao Khua Formation and varies in thickness between 50 and 100 m (Nulay and Arjwech, 2021). The Formation is dominated by light-buff to brown, medium-to coarse-grained, well-sorted sandstone, while siltstone and mudstone are subordinated. Pebbly conglomerate with well-rounded quartz pebbles and minor mudstone intraclasts are found in some places (Racey et al., 1996). The depositional environment is interpreted to be from a high energy braided river for the sandstone, and floodplain deposits for finer-grained sediments (Racey et al., 1996). The age of the Phu Phan Formation is the Early Cretaceous based on palynology (Racey et al., 1996).
The Khok Kruat Formation lies conformably on the Phu Phan Formation and exhibits variations in thickness from 200 m in the northwestern part of the Khorat Plateau to 850 m in the in the southeastern part of the Khorat Plateau, possibly due to erosion events (Sattayarak and Polachan, 1990; Smith et al., 1996; Racey, 2009). The Khok Kruat Formation consists mainly of light grey to light brown, thin-to thick-bedded, fine-to medium-grained micaceous or arkosic sandstone with clay rip-up clasts in several places. Siltstone, mudstone, and conglomerate beds are also found in this formation (Meesook, 2000). The Khok Kruat Formation is believed to have been deposited by meandering rivers on a floodplain. The age of the Khok Kruat Formation is the late Early to Middle Cretaceous (Aptian), based on freshwater bivalves in sandstone (Racey and Goodall, 2009).
The Maha Sarakam Formation lies unconformably on the Khok Kruat Formation, showing an angular unconformity, which is well-imaged from seismic data, between the Khok Kruat and the Maha Sarakam Formations. The unconformity extends throughout the region and indicates non-deposition or extensive erosion during the Mid-Cretaceous (Smith et al., 1996; Charusiri et al., 2006). The unconformity was formed by an inversion during Mid-Cretaceous, leading to a deposition of red-bed clastic rocks and evaporite rocks in a hypersaline land-locked basin under an arid paleoclimate (Figures 4, 5; Racey et al., 1996; Meesook, 2000). Consequently, the Maha Sarakham Formation consists of red-bed clastic rocks and evaporites, including halite, sylvite, carnallite, tachyhydrite, anhydrite, and borate minerals (Sattayarak et al., 1991; Hansen et al., 2002, 2016). The age of the Maha Sarakam Formations is the Mid-Cretaceous (Cenomanian), based on palynology (Sattayarak et al., 1991). Subsequently, the Khorat Plateau was covered by the Phu Tok Formation in the Late Cretaceous under an arid desert environment (Meesook, 2000; Hasegawa et al., 2010; Hasegawa et al., 2012).
4 Khorat evaporites
Evaporite caverns have been studied for CO2 storage worldwide because of their low permeability and self-healing ability, which can prevent the leakage of CO2 (e.g., Pajonpai et at., 2019). In the Khorat Plateau, thick evaporites are preserved within the Maha Sarakham Formation. Hence, Khorat evaporite is one of the possible geological CO2 storage sites in the Khorat Plateau.
The Maha Sarakham evaporites in the Khorat Plateau are present in the Khorat and the Sakon Nakhon basins within an area of 50,000 km2 (Suwanich, 2007). The resources of the potash deposit could be as much 400 billion tons of carnallite and 7 billion tons of sylvite, making it one of the largest potash deposits in the world (Hite and Japakasetr, 1979; Yumuang et al., 1986; El Tabakh et al., 1999; Shen and Siritongkham, 2020). These two basins are separated by the northwest-southeast striking Phu Phan anticline. The deposit of the evaporites can be correlated to the extensive Late Cretaceous potash deposits in the Lanping-Simao Basin in southwestern China, due to the worldwide high sea level in the Late Cretaceous (Haq et al., 1987; Qin et al., 2020; Rattana et al., 2022). The evaporite deposit in the Khorat Plateau is proposed to primarily be of marine origin, based on the geochemical data, sedimentary features, mineral sequences, and stratigraphic ages (e.g., Qin et al., 2020; Rattana et al., 2022); however, hydrothermal and continental fluids could have affected the evaporite basins (El Tabakh et al., 1999; Zhang et al., 2015; Li et al., 2018; Rattana et al., 2022).
The evaporites in the Khorat Plateau have a thickness of about 250 m to 1,100 m in some areas, and have been deformed by complex tectonics leading to different salt features in the subsurface, such as domes, anticlines, ridges, and mini-basins (Figure 5; El Tabakh et al., 1999; Suwanich, 2007). Salt deformations can be found at the shallow subsurface, approximately 50 m below the surface, due to salt movement caused by thick overburden sediments (Figure 5; El Tabakh et al., 1999). The evaporites are thickest in the depocenter of the evaporite basins and they gradually become thinner at the margins of the basins. The evaporites can be divided into three depositional members (El Tabakh et al., 1999; Shen et al., 2021):
1) The Lower Salt Member is well preserved throughout both evaporite basins and consists of massive halite, potash minerals (carnallite and sylvite), and banded colored salt (El Tabakh et al., 1999; Hansen et al., 2016; Rattana et al., 2022). This salt member is highly deformed, resulting in significant local differences in thickness from place to place (Figure 5).
2) The Middle Salt Member is mainly composed of two halite units that are separated by an anhydrite marker bed, with little to no potassic salt in this member (El Tabakh et al., 1999).
3) The Upper Salt Member is mainly composed of halite with minor anhydrite. In most cases, the Upper Salt Member is absent in the Khorat Plateau because of its shallow burial depth and wet climate. It seems that at least half of the salt of this member has been leached and dissolved (Shen and Siritongkham, 2020). Therefore, this member is either completely absent or very thin, with a thickness ranging from 3 to 17 m at the present day (Shen et al., 2021).
5 Khorat basaltic rocks
Large igneous provinces around the globe have been documented for CO2 storage due to their internal fractures formed during the cooling of lava and their chemical reaction between mineral compositions and CO2 (Raza et al., 2022). The suitable igneous rocks for effective carbon mineralization are mafic or ultramafic compositions, due to their high content of divalent cations (Ca2+, Mg2+, Fe2+), such as the pilot project in Iceland (McGrail et al., 2017; Raza et al., 2022). Thus, basaltic provinces in the Khorat Plateau could be an advantage for geological CO2 storage sites.
Basaltic rocks are widely distributed in Thailand, including in the Khorat Plateau, especially those dating to the Cenozoic Era. The Cenozoic basalts in Thailand can be divided into three main groups: 1) Basanitoid basalt, 2) Hawaiiitic basalt, and 3) Tholeiitic basalt. Cenozoic basalts on the Khorat Plateau are distributed among several provinces, including Nakhon Ratchasima, Ubon Ratchathani, Buriram, Surin and Sisaket, and are mostly located on the southernmost edge of the Khorat Plateau (Figure 3; Thanachit et al., 2006; Atayos et al., 2021; Singtuen and Phajan, 2021; Singtuen et al., 2021). The basaltic rocks in the Khorat Plateau usually show vesicular texture formed during the cooling of lava, which enhance the porosity and permeability of the rock matrix (Figure 6).
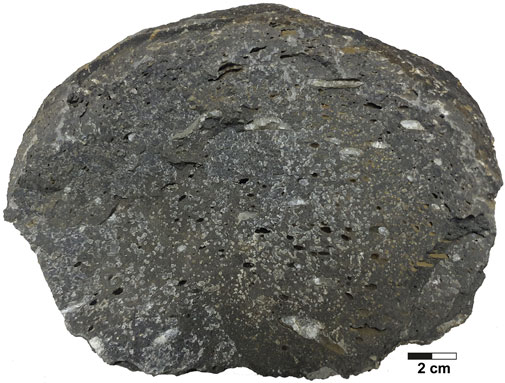
FIGURE 6. An example of the vesicular basalt from the Buriram-Nakhon Ratchasima area in the Khorat Plateau.
Nakhon Ratchasima basalts are classified as sub-alkali basalt, and are composed mostly of olivine, clinopyroxene and plagioclase phenocrysts (Barr and James, 1990; Atayos et al., 2021). Groundmass is composed of lath-plagioclase intergranular by clinopyroxene and opaque mineral. Alteration product found in the sample is normally iddingsite and sericite (Atayos et al., 2021), while Buriram basalts are classified as basaltic trachyandesite and alkali basalt (Barr and James, 1990), and show porphyritic texture. Phenocrysts are composed mostly of olivine, clinopyroxene, and plagioclase (An40-50: andesine), with minor amounts of iddingsite. Groundmass is composed of trachytic textured plagioclase intergranular, with clinopyroxene and opaque mineral (magnetite?) (Atayos et al., 2021).
The Surin basalts demonstrate trachytic texture with olivine phenocryst and groundmass of plagioclase intergranular, with clinopyroxene (Atayos et al., 2021). Glass is intersertal in the groundmass (Atayos et al., 2021). Ubon Ratchathani basalts are composed of olivine and clinopyroxene phenocrysts embedded in plagioclase, showing as intergranular/intersertal with olivine, clinopyroxene, opaque mineral, and glass (Atayos et al., 2021).
Srisaket basalts are classified as olivine basalt and nephelinite, showing brownish-red weathered surfaces with a high alteration rate. Olivine basalts are characterized by aphanitic, porphyritic, and vesicular textures with holocrystalline, while nephelinites show porphyritic and vesicular textures with holocrystalline. The mineral compositions in the olivine basalts are mainly plagioclase with high anorthite content (66–85), ranging from labradorite to bytownite, associated with minor forsterite, ilmenite, and clinopyroxene. The nephelinites are composed mainly of plagioclase, with very high anorthite content (81–94), ranging from bytownite to anorthite and nepheline associated with forsterite, diopside, augite, pigeonite, iddingsite, and ilmenite. These basaltic rocks in Srisaket show 5.62–11.07 wt% MgO and 7.63–10.17 wt% CaO. Thus, the Srisaket basalts are highly alkaline and alkaline volcanic rocks (Singtuen et al., 2021; Singtuen and Phajan, 2021).
6 Petroleum plays in the khorat plateau
Another option for geological CO2 storage is to inject the CO2 into petroleum reservoirs at active or abandoned petroleum fields (e.g., Godec et al., 2011; Zhou et al., 2020). Petroleum exploration and production have been operated in the Khorat Plateau for a long time. Hence, petroleum plays and elements are reviewed in this section to assess the carbon storage sites related to petroleum fields in the Khorat Plateau.
6.1 Source rocks
There are three possible hydrocarbon source rocks in the Khorat Plateau: 1) Lower Cretaceous siliciclastic rocks of the Khorat Group, which contain a few centimeters of woody organic materials in the lateral red-bed sequence, producing minimal gas by locally mature stage; 2) Triassic Huai Hin Lat organic-rich shale (Kuchinarai Group); and 3) Upper Carboniferous - Upper Permian of the Saraburi Group (Figure 4; Sattayarak et al., 1989; Racey, 2011). It should be noted that the Huai Hin Lat Formation is a significant source rock for petroleum gas fields in the Khorat Plateau. Upper Carboniferous - Upper Permian of the Saraburi Group is also identified as a major source rock in the Khorat Plateau and is expected to be regionally extensive throughout the Permian carbonate platforms. The Carboniferous-Triassic source-rock intervals experienced several phases of deformation, uplift and erosion that may have affected petroleum preservation in some areas, comprising tight sandstones, siltstones, and shale reservoirs. These source rocks are believed to have a hydrocarbon peak generation in the Late Triassic (Kozar et al., 1992).
6.2 Reservoir rocks
There are three petroleum potential reservoirs in the Khorat Plateau: 1) Permian carbonate of the Pha Nok Khao Formation of the Saraburi Group; 2) fluvial sandstones of the Khorat Group; and 3) basement reservoirs (Racey, 2011). The porosity and permeability of the potential reservoir rocks in the Khorat Plateau are listed in Table 1. Permian fractured carbonates of the Saraburi Group are the main proven reservoir to have been encountered to date (Racey, 2011). The reservoir in the two gas fields is comprised of carbonate rocks from the Pha Nok Khao Formation, providing dry gas with almost no CO2 in the reservoirs (Booth, 1998). These carbonate rocks are generally of low porosity, with a field wide average effective porosity of only 2–2.5% (Booth, 2005). The interplay of the petroleum system elements gives various petroleum plays in the Khorat Plateau, as presented in Figure 7. Potential carbonate reservoirs in the Khorat Plateau include fore reef slope and reefal build-up (Figure 7). However, there is clearly an extensive fracture network within the gas fields, which have been drained from various structural trap styles (Figure 7; Booth, 2005). The fracture network has been shown to enhance the reservoir quality, but the effect varies from place to place (Figure 7; Smith and Stokes, 1997). The proven carbonate reservoirs in Nam Phong area are mostly related to surface exposure and freshwater-related diagenesis during deposition and early burial (Kozar et al., 1992). The occurrence of dolomitization and fracture that enhance the reservoir quality of the Permian carbonates can be observed in several outcrops (see examples in Figure 8). Hence, Permian palaeo-highs are most likely to have a good-quality reservoir and are the main reservoir targets in the Khorat Plateau. However, several petroleum exploration wells have failed due to a complex deformation, such as inverted Permo-Triassic grabens (Figure 9; Assavaritiprom et al., 1995; Smith and Stokes, 1997). The complex deformation also causes porosity- or fracture-occluding calcite cement, which makes it difficult to predict the structure and composition of the subsurface.
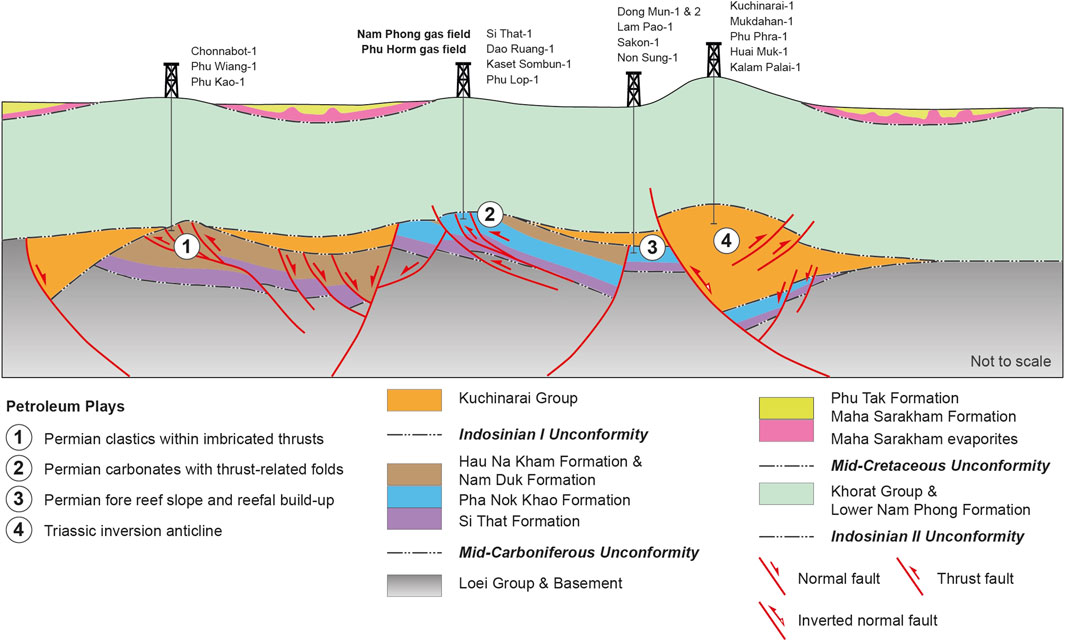
FIGURE 7. A schematic cross-section illustrating petroleum play concepts in the Khorat Plateau (modified from Booth, 1998; Booth and Sattayarak, 2011).
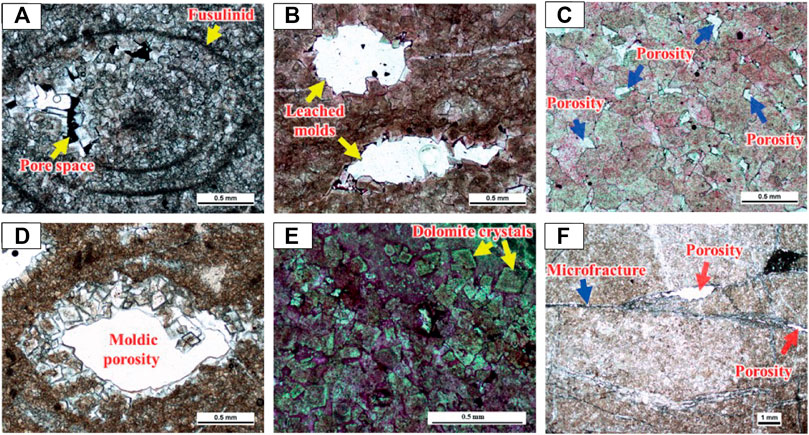
FIGURE 8. Photomicrographs of the dolomitized limestone from the Permian carbonate outcrops (modified from Kananusak, 2020). (A) a partially dolomitized fusulinid fossil with porosity, (B) dissolution pores with partially filled by dolomite, (C) intercrystalline pores (blue arrows) at crystal junctions, (D) crystal growths in moldic pore with partially filled by dolomite, (E) planar dolomite porphyrites in dolomized limestone, and (F) pores related to fractures (red arrows).
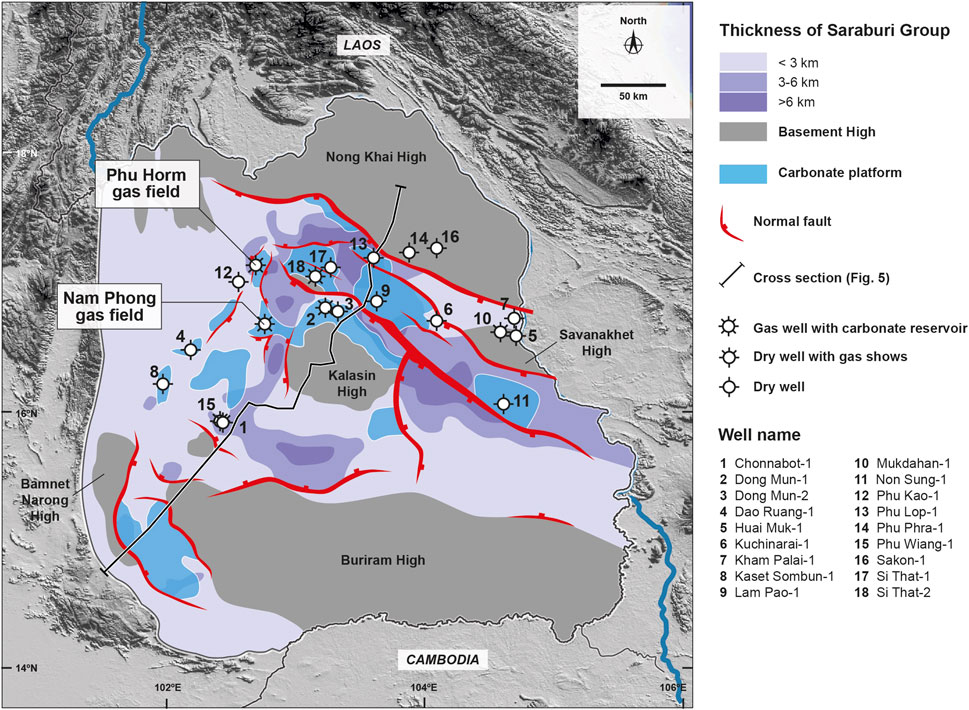
FIGURE 9. Map showing locations of selected petroleum exploration wells, gas fields, basement faulting, and the thickness of the Saraburi Group (modified from Booth and Sattayarak, 2011; Minezaki et al., 2019). The main petroleum targets are located on the Permian carbonate platform.
The reservoir quality of the Khorat Group sandstones decreases with increasing depth of burial due to the diagenesis process (Canham et al., 1996). Maximum values of around 20% porosity and 18 mD permeability have been observed from outcrop studies, and the porosity of the sandstones likely decreases to 3–5% at greater depths (Figure 10; Smith and Stokes, 1997; Suteerapongpan, 2018). Thus, the Khorat Group sandstones should provide fair- to good-quality gas reservoirs. Moreover, conglomerates, sandstones, or claystones at the base of the Khorat Group are associated with Indosinian erosion surface, and overlie Permian limestones and dolomites. Gas was detected from these rocks in the Phu Horm-1 well at rates of 4 MM SCF gas per day (Assavaritiprom et al., 1995). These rock strata can provide a proven seal in some areas within the Khorat Plateau (Smith and Stokes, 1997), although the basement rocks in the Khorat Plateau are Lower Carboniferous granite and are unproven with respect to gas reservoirs (Sattayarak et al., 1989; Smith and Stokes, 1997). Nevertheless, there have been some positive results from fractured basement reservoirs in Tertiary basins in Thailand (e.g., Madon et al., 2020).
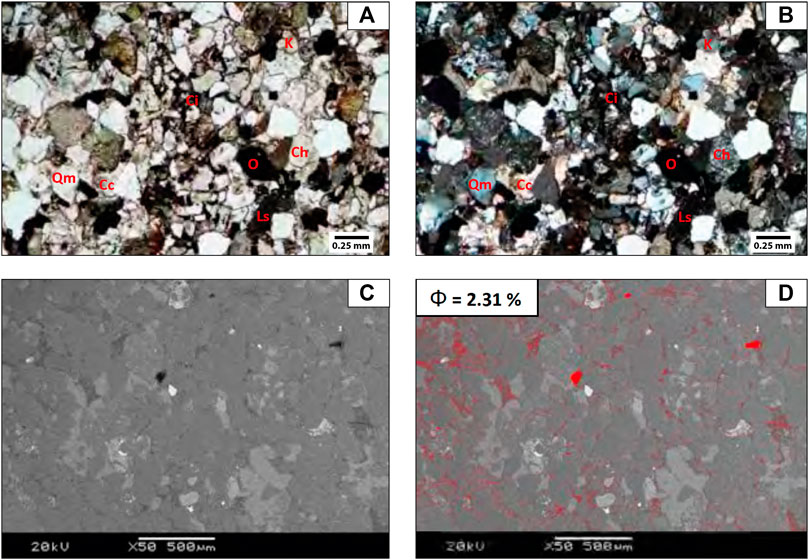
FIGURE 10. Photomicrographs of outcrop study from the Nam Phong sandstones (the base of the Khorat Group) in plane-polarized light (A) and in cross-polar (B). Note: K is potassium feldspar, Ci is iron oxide cement, Ch is chert, O is opaque mineral, Ls is sedimentary lithic fragments, Qm is monocrystalline quartz, and Cc is calcite. The backscattered electron image of the Nam Phong sandstones shows thresholding to binary image of pore space (C). Red marks cover pore space during thresholding operation for porosity analysis (D). These images are modified from Suteerapongpan (2018).
6.3 Petroleum traps
A complex tectonic history may have affected the development and preservation of viable petroleum trap styles and petroleum plays, such as Permian carbonate fault-reactivated anticline, shallow carbonate anticline, thick Permian carbonate syncline, Permian reverse fault related fold, Triassic inversion-anticline, Triassic truncation, and Triassic pinch out (Figure 7). Due to the limitations of the published subsurface data, only the main petroleum traps are described in this study; and are presented in Figure 11. Although most trap formations are reactivated by Tertiary deformation after the main period of hydrocarbon generation, the structural traps in this area have been proven to contain gas (e.g., Nam Phong gas field).
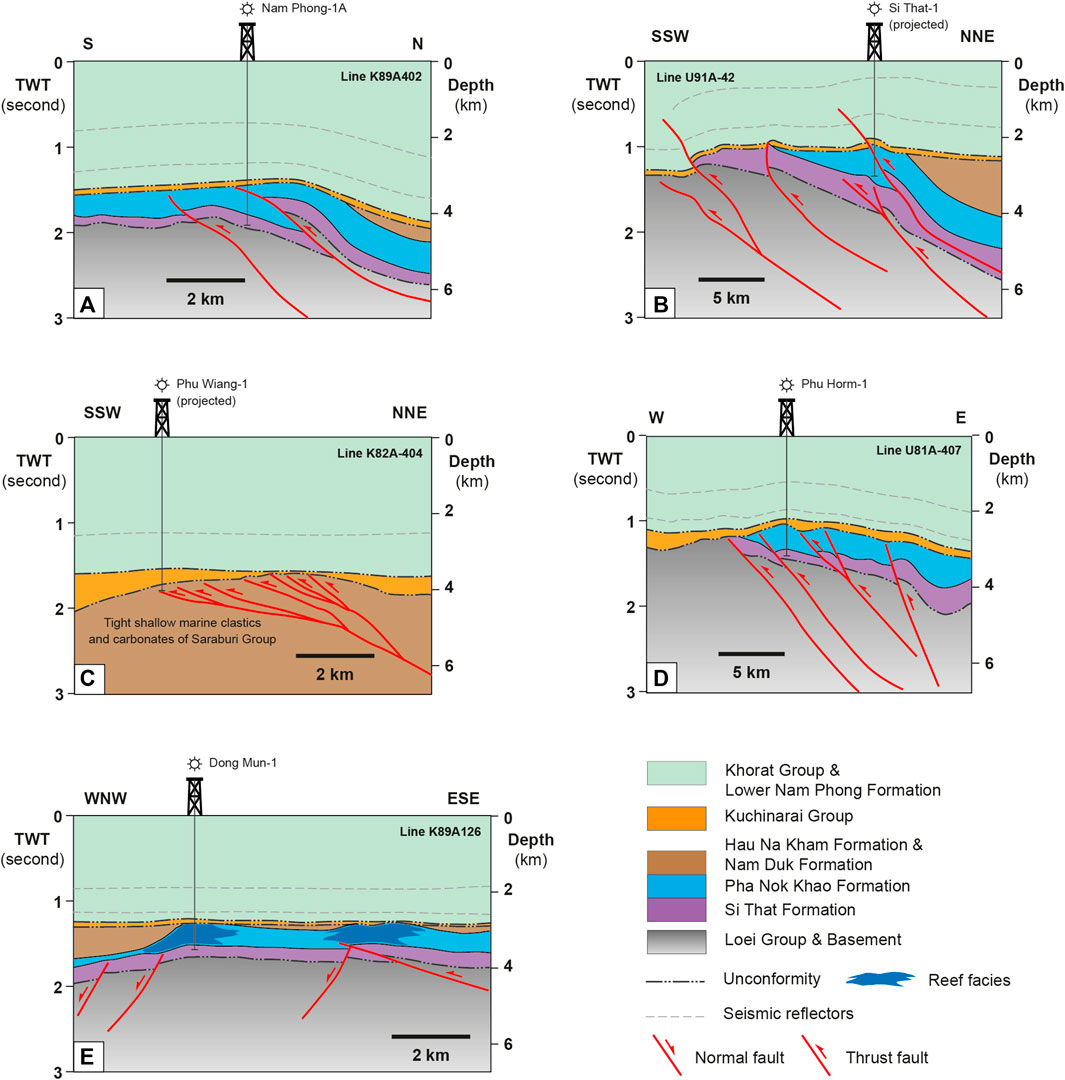
FIGURE 11. Simplified geological cross-section of the structural traps related to producing and abandoned petroleum fields in the Khorat Plateau. (A) Nam Phong structure, (B) Si That structure, (C) Phu Wiang structure, (D) Phu Horm structure, and (E) Dong Mun Structure (modified from Booth, 1998; Booth and Sattayarak, 2011).
The Nam Phong structure consists of a north-south trending, fault-bounded, inversion anticline overlying a reactivated and eroded, tilted Permian fault block (Smith and Stokes, 1997). Carbonate reservoirs are associated with the inversion anticline structure and a regional lower Khorat Group top-seal. Additionally, the down-flank closure of the anticline structure is overlaid by the Triassic Group and Upper Permian clastics (Figure 11A).
The Si That structure is also an anticline with SSW-NNE trend, resulting from inversion and reactivated faulting (Figure 11B). The Permian carbonate reservoirs are located beneath the Khorat Group and the Huai Hin Lat Formation. The anticline was formed before the deposition of the Huai Hin Lat Formation, based on seismic interpretation. The Si That structure is interpreted as being a paleo-high of Permian carbonate that could provide a good-quality reservoir, similar to Nam Phong gas field; however, without fracture networks, the Permian carbonate in this field would be a very dense and poor reservoir rock.
The SSW-NNE Phuwiang structure is a larger Permian platform than is the Nam Phong structure, but it has a similar structural history and trapping style (Figure 11C; Smith and Stokes, 1997). A petroleum exploration well was encountered at the top of a porous Permian carbonate formation, with the presence of a gas “kick” (Kozar et al., 1992).
The Phu Horm gas field is now the largest gas field in the Khorat Plateau. The Phu Horm structure exhibits an anticline with a northwest-southeast trend and lies at the eastern part of the Khorat Plateau (Figure 11D). This structure is also similar to the Nam Phong structure overlying a thin Huai Hin Lat Formation. The carbonate reservoirs are associated with faulting and folding, and are laid on the clastic Si That Formation. There are minor faults within the Permian carbonates that could enhance porosity and permeability in the reservoirs.
A potential stratigraphic trap of the Permian carbonate reservoirs is observed in the Dong Mun area, with a platform-margin carbonate build-up (Figure 11E; Kozaret al., 1992). The Kalasin structure is a northwest-southeast trending Permian carbonate that lies beneath the Khorat Group. The Kalasin structure is an extension of the Dong Mun Structure in the northwest. Similar to the Dong Mun Structure, the reefal structure is well recognized from seismic facies data.
6.4 Petroleum seal
The regional top-seal in the gas fields (Nam Phong, Dong Mun and Phu Horm) is the lower part of the Khorat Group, consisting of conglomerates, claystones, siltstones, and tight sandstones that are geometrically conformable with the inversion traps (Kozar et al., 1992; Mouret et al., 1993; Canham et al., 1996; Racey et al., 1996). Additional potential seals would be found in the Permian clastic Formation or the Triassic rocks over the crests of the Permian carbonate traps, or as down-flank wedges, such as in the Nam Phong gas field (Smith and Stokes, 1997).
7 Assessment and ranking of potential lithological formations
This section discusses the screening criteria that help to address a suitable CO2 storage site in the Khorat Plateau. The selection of criteria and indicators (e.g., storage optimization, risk minimization, and feasibility) is basically used for assessment and evaluation to develop a safe and effective geological CO2 storage site. Thus, a set of 13 criteria for the assessment and ranking of geological formations in the Khorat Plateau in terms of their suitability for CO2 storage sites is presented in Table 2. These criteria have been selected to facilitate the determination of the ease and feasibility of deploying CO2 storage sites in the Khorat Plateau. The criteria were adapted from Bachu (2003), and the weight and score of each criterion were determined based on its relative importance with respect to evaluation of the Khorat Plateau as a CO2 storage site. In addition, the Khorat Plateau has been designated as low- to non-seismic hazard region (Harnpattanapanich and Luddakul, 2011). Being located in a non-seismic hazard zone is important for safe CO2 storage and protection against potential leakage or catastrophic escape, which could potentially cause environmental problems. Although there has been low to moderate ground shaking from a large earthquake outside the Khorat Plateau, subsurface geological storage for CO2 in the plateau is considered to be safe from natural hazards that might jeopardize the storage security.
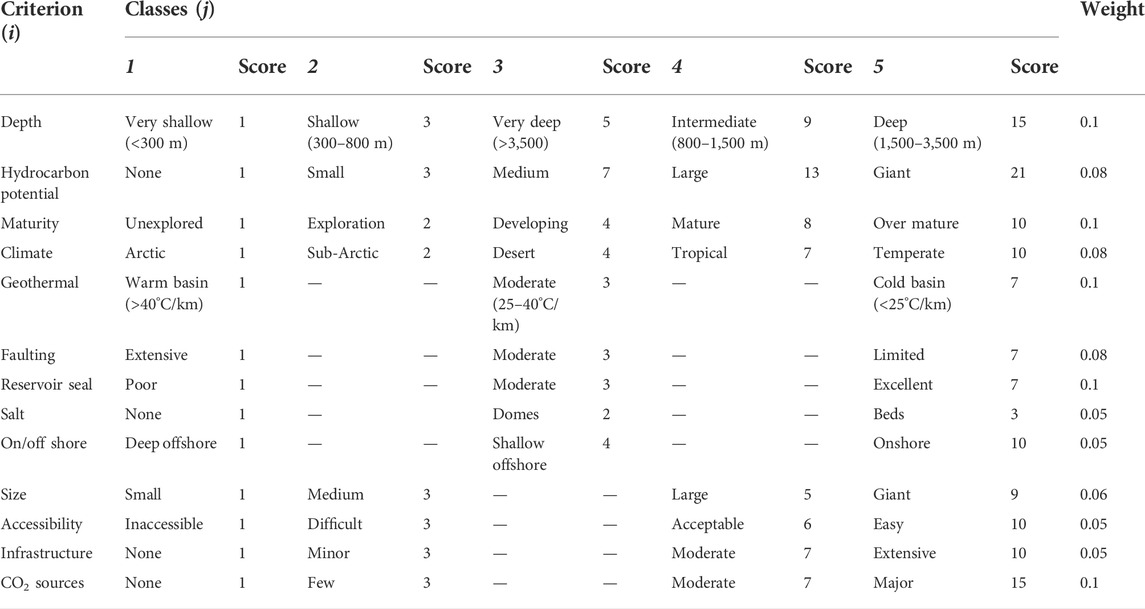
TABLE 2. Evaluation criteria for preliminary assessment of geological CO2 storage in the Khorat Plateau (adapted from Bachu, 2003).
The assessment of the potential lithological formations is based on a screening analysis to identify sites that should be suitable for CO2 utilization and storage in the future. One key criterion is CO2 point sources within a 100-km radius, such as the Nam Phong power plant (Figure 2). Based on this criterion, the potential lithological formations are the Khorat Group sandstones, Khorat Permian carbonates, Khorat evaporites, and Khorat Cenozoic basalts. Because these potential lithological formations are all in the Khorat Plateau, several screening conditions are assumed to be the same, including tectonic setting, geothermal gradient, and climate (Table 3).
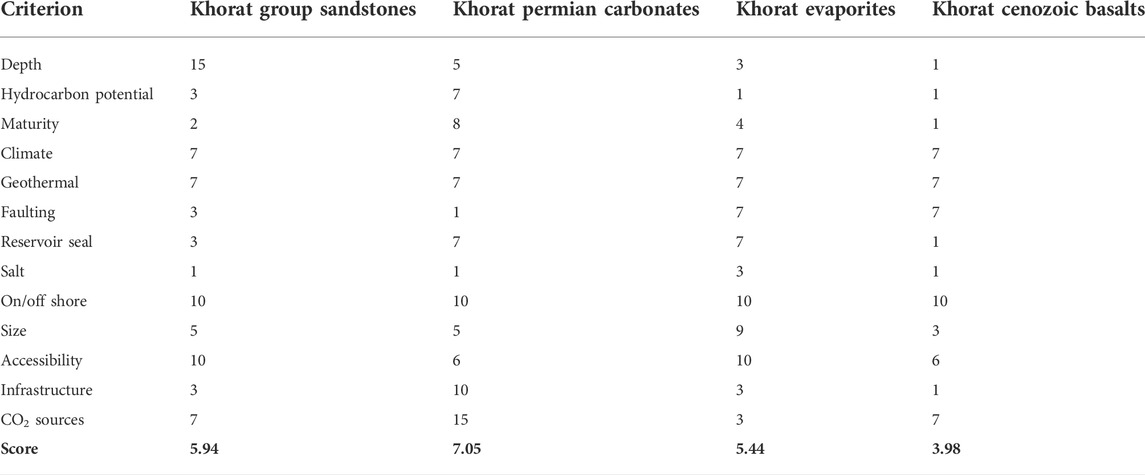
TABLE 3. Criterion scores and ranks of the potential lithological formations in the Khorat Plateau for geological CO2 storage.
By compiling data on the criteria in this study, different lithological units can be compared, contrasted, and ranked for their suitability for geological storage of CO2 (Table 3). In this study, the selected potential sites are evaluated based on their lithologies and available published data; however, more criteria and detailed geological information should be included in the assessment of potential CO2 storage sites in the future as more criteria are developed and more data are made available for the assessment.
All of the potential geological formations evaluated in the Khorat Plateau are located in the same geological setting, giving them identical scores of 10 for the criterion of onshore/offshore, as they are all located in an onshore stable area (Tables 2 and 3). Similarly, each is in a tropical area on the Khorat Plateau, meaning that each receives an identical score of 7 for the climate criterion (Tables 2 and 3). Although geothermal heat flow may be different in the subsurface due to different lithologies, all of the evaluated lithologies and sites for geological CO2 storage are assumed to be in moderate geothermal regime with 25–40 C, which means that each one scored 7 for the geothermal criterion (Tables 2 and 3). Other criteria are assigned for different potential geological formations, suggesting that the Khorat Permian carbonate is the most suitable for geological CO2 storage in the Khorat Plateau (Table 3).
The storage capacity of the potential lithological formations cannot be determined in this study due to lack of critical information, such as size, thickness, net-to-gross ratio, effective porosity, and CO2 storage efficiency factor. Thus, we cannot compare the storage capacity between the potential lithological formations. In this case, existing information was primarily derived from outcrop observations, published data, seismic sections, and petroleum exploration wells, which are mostly located within the gas field in the Khorat Plateau. The criterion scores and ranks of the potential lithological formations are summarized in Table 3 and discussed below.
7.1 Khorat Permian carbonates
The Permian carbonate reservoirs demonstrate good storage capability with 2–2.5% effective porosity, and are the highest ranked of the potential locations, based on criterion scores in Table 3 (Booth, 2005). CO2 can be stored as a gas, liquid, or supercritical fluid. The advantage of storing CO2 as a supercritical fluid is that a larger amount can be stored due to the increase in density as temperature and pressure rise with depth. The supercritical fluid state of CO2 requires a critical temperature of 31.1°C and a critical pressure of 7.39 MPa (Bachu, 2003). The depth of the Permian carbonate reservoirs is greater than 2,000 m, which is suitable for the supercritical phase of CO2 (Figure 11). However, it should be noted that CO2 sequestration and subsequent storage in sites at depths exceeding 2,500 m are relatively much more costly. Thus, the depths of the Permian carbonate reservoirs should be taken into account in assessing the geological CO2 storage suitability of the Khorat Plateau. Therefore, the different geological structures that affect the depths and distributions of the Permian carbonate reservoirs should be carefully investigated. Although the Permian carbonate reservoirs are located at great depths that increase costs associated with CO2 sequestration, the Permian carbonate reservoirs have been subjected to hydrocarbon exploration, with gas fields being produced that may have post-depletion CO2 storage potential. In addition, the main two gas fields are also located close to the main CO2 point source, the Nam Phong power plant, with existing infrastructure in place between the power plant and the gas fields. Abandoned exploration fields are interesting for geological CO2 storage, due to the existing infrastructure and potential storage capacity. Thus, the criterion scores are higher than those of the other three potential lithological formations in the Khorat Plateau. Furthermore, the Permian carbonate reservoirs from the gas fields Phu Horm, Dong Mun, and Nam Phong, in the Khorat Plateau have been evaluated to have average CO2 storage capacity at 0.1 Gt (Minezaki et al., 2019). Because the best potential storage reservoir in Khorat is Permian carbonate, the analysis of the reservoir quality and caprock integrity must be performed carefully before CO2 sequestration due to more chemically reactive between CO2 and carbonate rocks when compared to sandstone reservoirs (e.g., Siqueira et al., 2017).
7.2 Khorat Group sandstones
The Khorat Group sandstones are the second ranked option, based on criterion scores shown in Table 3. The Khorat Group sandstones are likely to provide effective geological CO2 storage in the Khorat Plateau. The sandstones are considered to offer a good-quality reservoir, especially given that the Upper Triassic–Upper Jurassic sandstones have a gross thickness of 300 m and an average porosity of 15% (Canham et al., 1996; El Tabakh et al., 1999). However, the reservoir quality of these sandstones is decreased at deep burial (Smith and Stokes, 1997). A potential good seal is, however, problematic because there is no proven good seal due to well-stacked fluvial systems in the Khorat Group sandstones. In fact, the Indosinian III unconformity within the Nam Phong Formation is the only potential seal for these sandstones; thus, vertical and lateral migrations of CO2 need to be of concern with regard to CO2 sequestration. Although there are some trap structures that can be developed for geological CO2 storage sites in the Khorat Group sandstones, studies of the suitability of the Khorat Group sandstone as a petroleum reservoir are limited. Moreover, the Khorat Group sandstones have not been developed for petroleum fields; thus, several criterion scores are lower than those of the Khorat Permian carbonate reservoirs.
7.3 Khorat evaporites
The Khorat evaporites are widely distributed in the Khorat Plateau, with mixed clastic sediments and evaporites of the Maha Sarakham Formation layering at the depocenter of the Plateau; which achieves the third rank, based on criterion scores shown in Table 3. The Maha Sarakham Formation consists of three members: Upper, Middle, and Lower. The thickness and depth of the massive layers of rock salt present in the Lower Member make them suitable for the excavation of salt caverns and long-term storage of CO2. In the Khorat Plateau, at a depth of more than 800 m, the salt layers of the Maha Sarakham Formation fulfill the criteria for storage of CO2 as a supercritical fluid. Although the Khorat evaporites are at shallow depths, the salt cavern stability modeling indicates that safe storage can be relied upon for a time span of at least 500 years (Pajonpai et at., 2019).
7.4 Khorat Cenozoic basalts
The Khorat Cenozoic basalts are locally exposed in the western margin of the Khorat Plateau. Although the Khorat Cenozoic basalts have an alkaline composition, these basalts either comprise outcrops or have no clear seal to prevent leakage of CO2 and cannot be considered for geological CO2 storage in the Khorat Plateau. The outcrops are usually at the surface and possibly extend to shallow depths, which are unsuitable for the storage of CO2. In addition, porosities in the Cenozoic alkaline basalts typically comprise less than 1% in unfractured outcrops. Little to no subsurface data in the western margin of the Khorat Plateau makes these alkaline basalts insufficient for the storage of CO2 as a supercritical fluid. Thus, the Khorat Cenozoic basalts are placed in the last rank (Table 3).
8 Conclusion
This study provides the first assessment of the potential for geological CO2 storage in the Khorat Plateau, Thailand. There are four potential lithological formations that can be developed as CO2 storage sites. The potential lithological formations are the Khorat Group sandstones, Khorat Permian carbonates, Khorat evaporites, and Khorat Cenozoic basalts. A set of criteria is applied for the assessment and ranking of the potential lithological formations in terms of the suitability of each as a possible CO2 storage site, based on storage optimization, risk minimization, and feasibility. The results suggest that the Khorat Permian carbonates are the most suitable for geological CO2 storage in the Khorat Plateau. The Khorat Group sandstones and Khorat evaporites are assessed as being in the second and third ranks, respectively. The Khorat Cenozoic basalts are not suitable for geological CO2 storage. The criteria scores that identify the Khorat Permian carbonate as the best choice are the maturation of petroleum exploration, the existing infrastructure, and a location close to a CO2 point source. However, chemical reaction between CO2 and carbonate rocks could be problematic. Thus, more subsurface studies should be carried out and more detailed criteria should be considered before CO2 sequestration is undertaken in the Khorat Plateau.
Author contributions
All authors listed have made a substantial, direct, and intellectual contribution to the work and approved it for publication.
Acknowledgments
The authors gratefully acknowledge the financial support from the creation of a teaching-led research platform, the Ratchadaphisek Somphot Endowment Fund, Chulalongkorn University. Reviewers are thanked for their useful and constructive comments.
Conflict of interest
The authors declare that the research was conducted in the absence of any commercial or financial relationships that could be construed as a potential conflict of interest.
Publisher’s note
All claims expressed in this article are solely those of the authors and do not necessarily represent those of their affiliated organizations, or those of the publisher, the editors and the reviewers. Any product that may be evaluated in this article, or claim that may be made by its manufacturer, is not guaranteed or endorsed by the publisher.
References
Achache, J., Courtillot, V., and Besse, J. (1983). Paleomagnetic constraints on the late cretaceous and cenozoic tectonics of southeastern asia. Earth Planet. Sci. Lett. 63 (1), 123–136. doi:10.1016/0012-821x(83)90028-6
Ahrendt, H., Chonglakmani, C., Hansen, B. T., and Helmcke, D. (1993). Geochronological cross section through northern Thailand. J. Southeast Asian Earth Sci. 8 (1-4), 207–217. doi:10.1016/0743-9547(93)90022-h
Assavaritiprom, V., Chaisilboon, B., and Polachan, S. (1995). “Review on petroleum exploration in northeast Thailand,” in Proceedings of the international conference on geology, geotechnology and mineral resources of indochina, Khon Kaen, Thailand, November 22-25, 1995.
Atayos, P., Srichan, W., and Limtrakul, P. (2021). “Petrography and occurrence of cenozoic volcanic rocks in southern margin of Khorat plateau,” in Proceedings of the 10th international science, social science, engineering and energy conference, Sakon Nakhon, Thailand, 20-23 November 2019 (Rajamangala University of Technology Isan Sakon Nakhon Campus), 1–8.
Bachu, S. (2003). Screening and ranking of sedimentary basins for sequestration of CO2 in geological media in response to climate change. Environ. Geol. 44 (3), 277–289. doi:10.1007/s00254-003-0762-9
Barber, A. J., Ridd, M. F., and Crow, M. J. (2011). The origin, movement and assembly of the pre-tertiary tectonic units of Thailand. London: Geological Society of London.
Barr, S. M., and James, D. E. (1990). Trace element characteristics of upper cenozoic basaltic rocks of Thailand, kampuchea and Vietnam. J. Southeast Asian Earth Sci. 4 (3), 233–242. doi:10.1016/s0743-9547(05)80016-9
Blunt, M., Fayers, F. J., and Orr, F. M. (1993). Carbon dioxide in enhanced oil recovery. Energy Convers. Manag. 34 (9-11), 1197–1204. doi:10.1016/0196-8904(93)90069-m
Booth, J. E. (1998). “The Khorat Plateau of NE Thailand-exploration history and hydrocarbon potential,” in Proceedings of the 1998 SEAPEX exploration conference, Singapore, December 2-3, 1998, 169–202.
Booth, J. (2005). “Khorat plateau basin, NE Thailand, discoveries and future exploration potential,” in Proceedings of the 2005 south east asia petroleum exploration society (SEAPEX) conference, Singapore.
Booth, J., and Sattayarak, N. (2011). “Subsurface carboniferous-cretaceous geology of northeast Thailand,” in The geology of Thailand. Editors M. F. Ridd, A. J. Barber, and M. J. Crow (London: Geological Society of London), 185–222.
Bunopas, S., and Vella, P. (1992). “Geotectonics and geologic evolution of Thailand,” in Proceedings of the national conference on geologic resources of Thailand: potential for future development, Bangkok, Thailand, November 17 - 24, 1992, 209–228.
Bunopas, S., and Vella, P. (1978). “Late palaeozoic and mesozoic structural evolution of northern Thailand: a plate tectonic model,” in Proceedings of the third regional conference on geology and mineral resources of Southeast Asia, Bangkok, November 14 - 18, 1978, 122–140.
Bunopas, S., and Vella, P. (1983). “Tectonic and geologic evolution of Thailand,” in Proceedings of a workshop on stratigraphic correlation of Thailand and Malaysia, Thailand, September 8-10, 1983, 1, 307–322.
Burrett, C., Zaw, K., Meffre, S., Lai, C. K., Khositanont, S., Chaodumrong, P., et al. (2014). The configuration of greater gondwana—evidence from LA ICPMS, U–Pb geochronology of detrital zircons from the paleozoic and mesozoic of southeast asia and China. Gondwana Res. 26 (1), 31–51. doi:10.1016/j.gr.2013.05.020
Canham, A. C., Love, M. A., Racey, A., and Polachan, S. (1996). Stratigraphy and reservoir potential of the mesozoic Khorat group, NE Thailand: Part 2: diagenesis and reservoir quality. J. Pet. Geol. 19 (3), 321–338. doi:10.1111/j.1747-5457.1996.tb00437.x
Carter, A., and Bristow, C. S. (2003). Linking hinterland evolution and continental basin sedimentation by using detrital zircon thermochronology: a study of the Khorat plateau basin, eastern Thailand. Basin Res. 15 (2), 271–285. doi:10.1046/j.1365-2117.2003.00201.x
Chantong, W., Srisuwon, P., Kaewkor, C., Praipipan, C., and Ponsri, S. (2013). “Distributions of the permo-carboniferous rocks in the Khorat plateau basin,” in Proceedings of the 2nd Lao-Thai technical conference on geology and mineral resources, Thailand, 17–18 January 2013, 73–80.
Charusiri, P., Imsamut, S., Zhuang, Z., Ampaiwan, T., and Xu, X. (2006). Paleomagnetism of the earliest cretaceous to early late cretaceous sandstones, Khorat group, northeast Thailand: implications for tectonic plate movement of the Indochina block. Gondwana Res. 9 (3), 310–325. doi:10.1016/j.gr.2005.11.006
Chonglakmani, C., and Sattayarak, N. (1978). “Stratigraphy of the Huai Hin Lat Formation (upper triassic) in northeastern Thailand,” in Proceedings of the regional conference on geology and mineral resources of southeast asia, Thailand, November 14-18, 1978, 739–762.
Choomkong, A., Sirikunpitak, S., Darnsawasdi, R., and Yordkayhun, S. (2017). A study of CO2 emission sources and sinks in Thailand. Energy Procedia 138, 452–457. doi:10.1016/j.egypro.2017.10.198
Chuaviroj, S. (1997). “Deformations in Khorat plateau, Thailand,” in Proceedings of the international conference on stratigraphy and tectonic evolution of Southeast Asia and South Pacific, Thailand, 30 January-2 February, 1989, 321–325.
Climate Analytics (2019). Country profile Thailand: Decarbonising south and southeast asia. Available at: https://climateanalytics.org/media/decarbonisingasia2019-profile-thailand-climateanalytics.pdf (Accessed December 20, 2021).
Cooper, M. A., Herbert, R., and Hill, G. S. (1989). “The structural evolution of triassic intermontane basins in northeastern Thailand,” in Proceedings of the international symposium on intermontane basins: geology and resources, Thailand, 30 January-2 February 1989, 231–242.
Daly, M. C., Cooper, M. A., Wilson, I. B. G. D., Smith, D. T., and Hooper, B. G. D. (1991). Cenozoic plate tectonics and basin evolution in Indonesia. Mar. Pet. Geol. 8 (1), 2–21. doi:10.1016/0264-8172(91)90041-x
Drumm, A., Heggemann, H., and Helmcke, D. (1993). “Contribution of sedimentology and sediment petrology of the non-marine mesozoic sediments in northern Thailand (phrae and nan provinces),” in Proceedings of the international symposium on biostratigraphy of mainland southeast asia: facies & paleontology, Chiang Mai, Thailand, 31 January - 5 February 1993, 2, 299–318.
Dusseault, M. B., Bachu, S., and Rothenburg, L. (2004). “Sequestration of CO2 in salt caverns,” in Proceedings of the canadian international petroleum conference, Calgary, Alberta, June 2002. PETSOC-2002-237. doi:10.2118/2002-237
El Tabakh, M., Utha-Aroon, C., and Schreiber, B. C. (1999). Sedimentology of the cretaceous maha sarakham evaporites in the khorat plateau of northeastern Thailand. Sediment. Geol. 123 (1-2), 31–62. doi:10.1016/s0037-0738(98)00083-9
Energy Policy and Planning Office (EPPO) (2022). CO2 emission. Available at: http://www.eppo.go.th/index.php/en/en-energystatistics/co2-statistic (Accessed December 20, 2021).
Gale, J., and Freund, P. (2001). Coal-bed methane enhancement with CO2 sequestration worldwide potential. Environ. Geosci. 8 (3), 210–217. doi:10.1046/j.1526-0984.2001.008003210.x
Geological Survey Division (1999). Geological map of Thailand in scale of 1:1,000,000, geological Survey division, department of mineral resources; digital captured by geological information section, geological Survey division, department of mineral resources (2002). Available at: http://www.dmr.go.th/subsite3.php?nid=110351 (Accessed December 20, 2021).
Godec, M., Kuuskraa, V., Van Leeuwen, T., Melzer, L. S., and Wildgust, N. (2011). CO2 storage in depleted oil fields: the worldwide potential for carbon dioxide enhanced oil recovery. Energy Procedia 4, 2162–2169. doi:10.1016/j.egypro.2011.02.102
Gunter, W. D., Gentzis, T., Rottenfusser, B. A., and Richardson, R. J. H. (1997). Deep coalbed methane in alberta, Canada: a fuel resource with the potential of zero greenhouse gas emissions. Energy Convers. Manag. 38, S217–S222. doi:10.1016/s0196-8904(96)00272-5
Haile, N. S. (1973). Note on triassic fossil pollen from nam pha formation, chulabhon (Nam phrom) dam, Thailand, geological society of Thailand. Newsletter 6 (1), 15–16.
Hansen, B. T., Wemmer, K., Eckhardt, M., Putthapiban, P., and Assavapatchara, S. (2016). Isotope dating of the potash and rock salt deposit at bamnet narong, NE-Thailand. Open J. Geol. 6 (08), 875–894. doi:10.4236/ojg.2016.68067
Hansen, B. T., Wemmer, K., Pawlig, S., Klaus, J., Assavapatchara, S., Nontaso, M., et al. (2002). “Isotopic evidence for a late cretaceous age of the potash and rock salt deposit at bamnet narong, NE Thailand,” in Proceedings of the symposium on geology of Thailand, Bangkok, Thailand, August 26-31, 2002 (Department of Mineral Resources), 26–31.
Haq, B. U., Hardenbol, J. A. N., and Vail, P. R. (1987). Chronology of fluctuating sea levels since the Triassic. Science 235 (4793), 1156–1167. doi:10.1126/science.235.4793.1156
Harnpattanapanich, T., and Luddakul, A. (2011). “Seismic hazard of the Khorat plateau: preliminary review,” in Proceeding of the international conference on geology, geotechnology and mineral resources of indochina (GEOINDO 2011), Thailand, December 1-3, 2011.
Hasegawa, H., Imsamut, S., Charusiri, P., Tada, R., Horiuchi, Y., and Hisada, K. (2010). Thailand was a desert” during the mid-cretaceous: equatorward shift of the subtropical high-pressure belt indicated by eolian deposits (phu thok formation) in the khorat basin, northeastern Thailand. Isl. Arc 19, 605–621. doi:10.1111/j.1440-1738.2010.00728.x
Hasegawa, H., Tada, R., Jiang, X., Suganuma, Y., Imsamut, S., Charusiri, P., et al. (2012). Drastic shrinking of the hadley circulation during the mid-cretaceous supergreenhouse. Clim. Past. 8, 1323–1337. doi:10.5194/cp-8-1323-2012
Hite, R. J., and Japakasetr, T. (1979). Potash deposits of the Khorat plateau, Thailand and Laos. Econ. Geol. 74 (2), 448–458. doi:10.2113/gsecongeo.74.2.448
Horiuchi, Y., Charusiri, P., and Hisada, K. I. (2012). Identification of an anastomosing river system in the early cretaceous Khorat basin, northeastern Thailand, using stratigraphy and paleosols. J. Asian Earth Sci. 61, 62–77. doi:10.1016/j.jseaes.2012.08.022
International Energy Agency (2014). Energy technology perspective 2014 – harnessing electricity’s potential. Available at: https://www.iea.org/reports/energy-technology-perspectives-2014 (Accessed December 20, 2021).
Kananusak, D. (2020). Dolomitization related to fracture porosity evolution: a case study of carbonate outcrops in Nam maholan formation. Bull. Earth Sci. Thail. 12 (2), 57–67.
Kempka, T., Klein, E., De Lucia, M., Tillner, E., and Kühn, M. (2013). Assessment of long-term CO2 trapping mechanisms at the ketzin pilot site (Germany) by coupled numerical modelling. Energy Procedia 37, 5419–5426. doi:10.1016/j.egypro.2013.06.460
Kobayashi, T. (1975). “Upper triassic estheriids in Thailand and the conchostracan development in asia in the mesozoic era,” in Geology and palaeontology of southeast asia. Editors T. Kobayashi, and R. Toriyama (Tokyo, Japan: University of Tokyo Press), 16, 57–90.
Koide, H., Tazaki, Y., Noguchi, Y., Iijima, M., Ito, K., and Shindo, Y. (1993). Underground storage of carbon dioxide in depleted natural gas reservoirs and in useless aquifers. Eng. Geol. 34 (3-4), 175–179. doi:10.1016/0013-7952(93)90086-r
Koide, H., and Yamazaki, K. (2001). Subsurface CO2 disposal with enhanced gas recovery and biogeochemical carbon recycling. Environ. Geosci. 8 (3), 218–224. doi:10.1046/j.1526-0984.2001.008003218.x
Kon'no, E., and Asama, K. (1973). “Mesozoic plants from khorat, Thailand,” in Geology and palaeontology of southeast asia. Editors T. Kobayashi, and R. Toriyama (Tokyo, Japan: University of Tokyo Press), 12, 149–172.
Koysamran, S., and Comrie-Smith, N. (2011). “Basin modeling of block L26/50, eastern Khorat plateau, northeast Thailand,” in Proceeding of the 4th petroleum forum, Thailand, 26-27 May 2011, 5–10.
Kozar, M. G., Crandall, G. F., and Hall, S. E. (1992). “Integrated structural and stratigraphic study of the khorat basin, rat buri limestone (permian), Thailand,” in Proceedings of a national conference on geologic resources of Thailand: potential for future development, Bangkok, 17–24 November 1992, 692–736.
Lau, H. C., and Ramakrishna, S. (2021). “Roadmap for decarbonization of Singapore and its implications for ASEAN—opportunities for 4IR technologies and sustainable development,” in Asia pacific tech monitor (Bangkok, Thailand, New Delhi, India: Asian and Pacific Centre for Transfer of Technology United Nations Economic and Social Commission for Asia and the Pacific), 29–39.
Lau, H. C., Ramakrishna, S., Zhang, K., and Radhamani, A. V. (2021). The role of carbon capture and storage in the energy transition. Energy Fuels 35 (9), 7364–7386. doi:10.1021/acs.energyfuels.1c00032
Li, M., Yan, M., Fang, X., Zhang, Z., Wang, Z., Sun, S., et al. (2018). Origins of the mid-cretaceous evaporite deposits of the sakhon Nakhon basin in Laos: evidence from the stable isotopes of halite. J. Geochem. Explor. 184, 209–222. doi:10.1016/j.gexplo.2017.11.001
Madon, M., Jong, J., Kessler, F. L., Damanhuri, M. H., and Amin, M. K. A. (2020). Pre-tertiary basement subcrops beneath the Malay and penyu basins, offshore peninsular Malaysia: their recognition and hydrocarbon potential. Bull. Geol. Soc. Malays. 70, 163–193. doi:10.7186/bgsm70202014
McGrail, B. P., Schaef, H. T., Spane, F. A., Cliff, J. B., Qafoku, O., Horner, J. A., et al. (2017). Field validation of supercritical CO2 reactivity with basalts. Environ. Sci. Technol. Lett. 4 (1), 6–10. doi:10.1021/acs.estlett.6b00387
Mao, C., Yamada, Y., and Matsuoka, T. (2014). A preliminary assessment of geological CO2 storage in Cambodia. Int. J. Greenh. Gas Control. 30, 19–33. doi:10.1016/j.ijggc.2014.08.016
Meesook, A. (2000). “Cretaceous environments of northeastern Thailand,” in Developments in palaeontology and stratigraphy. Editors O. Hakuyu, and J. M. Nlall (Amsterdam: Elsevier), 207–223.
Meesook, A., and Saengsrichan, W. (2011). “Jurassic,” in The geology of Thailand. Editors M. F. Ridd, A. J. Barber, and M. J. Crow (London: Geological Society of London), 151–168.
Metcalfe, I. (2013). Gondwana dispersion and asian accretion: tectonic and palaeogeographic evolution of eastern tethys. J. Asian Earth Sci. 66, 1–33. doi:10.1016/j.jseaes.2012.12.020
Metcalfe, I. (2011). Palaeozoic–mesozoic history of SE asia. Geol. Soc. Lond. Spec. Publ. 355 (1), 7–35. doi:10.1144/sp355.2
Metcalfe, I. (1996). “Pre-Cretaceous evolution of SE Asian terranes,” in Tectonic evolution of southeast asia. Editors R. Hall, and D. Blundell (London: The Geological Society of London), 106, 97–122. Special Publication.
Minezaki, T., Hisada, K. I., Hara, H., and Kamata, Y. (2019). Tectono-stratigraphy of Late Carboniferous to Triassic successions of the Khorat Plateau Basin, Indochina Block, northeastern Thailand: initiation of the Indosinian Orogeny by collision of the Indochina and South China blocks. J. Asian Earth Sci. 170, 208–224. doi:10.1016/j.jseaes.2018.10.020
Morley, C. K., Ampaiwan, P., Thanudamrong, S., Kuenphan, N., and Warren, J. (2013). Development of the Khao khwang fold and thrust belt: Implications for the geodynamic setting of Thailand and Cambodia during the indosinian orogeny. J. Asian Earth Sci. 62, 705–719. doi:10.1016/j.jseaes.2012.11.021
Morley, C. K. (2012). Late cretaceous–early palaeogene tectonic development of SE asia. Earth. Sci. Rev. 115 (1-2), 37–75. doi:10.1016/j.earscirev.2012.08.002
Mouret, C., Heggemann, H., Gouadain, J., and Krisadasima, S. (1993). “Geological history of the siliciclastic mesozoic strata of the khorat group in the Phu phan range area, northeastern Thailand,” in Proceedings of the international symposium on biostratigraphy of main-land Southeast Asia: facies and paleontology, Thailand, 31 January - 5 February, 1993, 23–49.
Nulay, P., and Arjwech, R. (2021). A review of the lithostratigraphy of the early cretaceous Sao Khua Formation, Khorat group in northeastern Thailand. Open J. Geol. 11 (9), 381–395. doi:10.4236/ojg.2021.119021
Pajonpai, N., Bissen, R., Pumjan, S., and Henk, A. (2019). Geomechanical Modeling of Salt Cavern Stability for Carbon Dioxide Storage in Northeast Thailand. Bull. Earth Sci. Thailand 11 (1), 1–12.
Qin, Z., Li, Q., Zhang, X., Fan, Q., Wang, J., Du, Y., et al. (2020). Origin and recharge model of the late cretaceous evaporites in the Khorat plateau. Ore Geol. Rev. 116, 103226. doi:10.1016/j.oregeorev.2019.103226
Racey, A., and Goodall, J. G. S. (2009). “Palynology and stratigraphy of the mesozoic Khorat group red bed sequences from Thailand,” in Late palaeozoic and mesozoic ecosystems in SE asia. Editors E. Buffetaut, G. Cuny, J. Leloeuff, and V. Suteethorn (London: The Geological Society of London), 69–83.
Racey, A., Love, M., Canham, A., Goodall, J., Polachan, S., and Jones, P. (1996). Stratigraphy and reservoir potential of the mesozoic Khorat group, NE Thailand: Part 1: stratigraphy and sedimentary evolution. J. Pet. Geol. 19, 5–39. doi:10.1111/j.1747-5457.1996.tb00511.x
Racey, A. (2009). “Mesozoic red bed sequences from SE asia and the significance of the khorat group of NE Thailand,” in Late palaeozoic and mesozoic ecosystems in SE asia. Editors E. Buffetaut, G. Cuny, J. Leloeuff, and V. Suteethorn (London: The Geological Society of London), 41–67.
Racey, A. (2011). “Petroleum geology,” in The geology of Thailand. Editors M. F. Ridd, A. J. Barber, and M. J. Crow (London: Geological Society of London), 351–392.
Rattana, P., Choowong, M., He, M. Y., Tan, L., Lan, J., Bissen, R., et al. (2022). Geochemistry of evaporitic deposits from the cenomanian (upper cretaceous) maha sarakham formation in the khorat basin, northeastern Thailand. Cretac. Res. 130, 104986. doi:10.1016/j.cretres.2021.104986
Raza, A., Glatz, G., Gholami, R., Mahmoud, M., and Alafnan, S. (2022). Carbon mineralization and geological storage of CO2 in basalt: mechanisms and technical challenges. Earth. Sci. Rev. 229, 104036. doi:10.1016/j.earscirev.2022.104036
Riaz, A., and Cinar, Y. (2014). Carbon dioxide sequestration in saline formations: Part I—review of the modeling of solubility trapping. J. Pet. Sci. Eng. 124, 367–380. doi:10.1016/j.petrol.2014.07.024
Ridd, M. F., Barber, A. J., and Crow, M. J. (2011). The geology of Thailand. London: Geological Society of London.
Sattayarak, N. (2005). “Petroleum potential of northeast, Thailand,” in Proceedings of international conference on geology, geotechnology and mineral resources of indochina (GEOINDO 2005), Khon Kaen, Thailand, 28–30 November 2005, 21–30.
Sattayarak, N., Polachan, S., and Charusirisawad, R. (1991). “Cretaceous rock salt in the northeastern part of Thailand,” in Proceedings of the 7th regional conference geology and mineral resources Southeast Asia (GEOSEA VII), Thailand, November 5 - 8, 1991.
Sattayarak, N., and Polachan, S. (1990). “Rocksalt underneath the Khorat plateau,” in Proceedings of the department of mineral resources technical conference, Bangkok, 2-6 July 1990, 1–14.
Sattayarak, N., Srilulwong, S., and Pum-Im, S. (1989). “Petroleum potential of the triassic pre-khorat intermontane basin in northeastern Thailand,” in Proceeding of the international symposium on intermontane basins: geology and resources, Thailand, 30 January-2 February, 1989, 43–58.
Schenk, C. J., Klett, T. R., Mercier, T. J., Finn, T. M., Tennyson, M. E., Gaswirth, S. B., et al. (2017). Assessment of continuous gas resources in the Khorat plateau province, Thailand and Laos, 2016 (No. 2017-3023). US Geological Survey.
Shen, L. J., and Siritongkham, N. (2020). The characteristics, formation and exploration progress of the potash deposits on the khorat plateau, Thailand and Laos, southeast asia. China Geol. 3 (1), 67–82. doi:10.31035/cg2020009
Shen, L., Siritongkham, N., Wang, L., Liu, C., Nontaso, A., Khadsri, W., et al. (2021). The brine depth of the khorat basin in Thailand as indicated by high-resolution Br profile. Sci. Rep. 11 (1), 8673–8678. doi:10.1038/s41598-021-88037-6
Singtuen, V., Phajan, S., Anumart, A., Phajuy, B., Srijanta, K., and Promkotra, S. (2021). Alteration of high alkaline and alkaline basaltic rocks: parent rocks in the lava durian orchard, Sisaket province, NE Thailand. Heliyon 7, e08619. doi:10.1016/j.heliyon.2021.e08619
Singtuen, V., and Phajan, S. (2021). Petrographic and geochemical data of high alkaline basalts, Sisaket terrain, NE Thailand. Data Brief. 39, 107540. doi:10.1016/j.dib.2021.107540
Siqueira, T. A., Iglesias, R. S., and Ketzer, J. M. (2017). Carbon dioxide injection in carbonate reservoirs–a review of CO2-water-rock interaction studies. Greenh. Gas. Sci. Technol. 7 (5), 802–816. doi:10.1002/ghg.1693
Smith, P. F. L., Stokes, R. B., Bristow, C., and Carter, A. (1996). “Mid-cretaceous inversion in the northern Khorat plateau of Lao PDR and Thailand,” in Tectonic evolution of southeast asia. Editors R. Hall, and D. Blundell (London: The Geological Society of London), 106, 233–247. Special Publication.
Smith, P. L., and Stokes, R. B. (1997). Geology and petroleum potential of the khorat plateau basin in the vientiane area of Lao PDR. J. Petroleum Geol. 20 (1), 27–49. doi:10.1111/j.1747-5457.1997.tb00754.x
Sone, M., and Metcalfe, I. (2008). Parallel tethyan sutures in mainland southeast asia: new insights for palaeo-tethys closure and implications for the indosinian orogeny. Comptes Rendus Geosci. 340 (2-3), 166–179. doi:10.1016/j.crte.2007.09.008
Suteerapongpan, N. (2018). Petrography of some sandstones from nam phong formation along the highway no.12, lom sak district, petchabun province: assessment of porosity for petroleum reservoir potentiality. Thailand: Chulalongkorn University, 80.
Suwanich, P. (2007). “Potash-evaporite deposits in Thailand,” in Proceedings of the international conference on geology of thailand, Thailand, November 21 - 22, 2007, 252–262.
Tan, H., Ma, H., Li, B., Zhang, X., and Xiao, Y. (2010). Strontium and boron isotopic constraint on the marine origin of the khammuane potash deposits in southeastern Laos. Chin. Sci. Bull. 55 (27), 3181–3188. doi:10.1007/s11434-010-4010-x
Thanachit, S., Suddhiprakarn, A., Kheoruenromne, I., and Gilkes, R. J. (2006). The geochemistry of soils on a catena on basalt at Khon buri, northeast Thailand. Geoderma 135, 81–96. doi:10.1016/j.geoderma.2005.10.010
United Nations ESCAP (2018). The energy transition pathways for the 2030 agenda in asia and the pacific: regional trends report on energy for sustainable development 2018. Available at: https://www.unescap.org/publications/energy-transition-pathways-2030-agenda-asia-and-pacific-regional-trends-report-energy (Accessed November 15, 2021).
Warren, J., Morley, C. K., Charoentitirat, T., Cartwright, I., Ampaiwan, P., Khositchaisri, P., et al. (2014). Structural and fluid evolution of Saraburi group sedimentary carbonates, central Thailand: a tectonically driven fluid system. Mar. Pet. Geol. 55, 100–121. doi:10.1016/j.marpetgeo.2013.12.019
Yumuang, S., Khantaprab, C., and Taiyagupt, M. (1986). The evaporite deposits in bamnet narong area, northeastern Thailand. Bull. Geol. Soc. Malays. 20, 249-267.
Zhang, H., Liu, C., Zhao, Y., Mischke, S., Fang, X., and Ding, T. (2015). Quantitative temperature records of Mid cretaceous hothouse: evidence from halite fluid inclusions. Palaeogeogr. Palaeoclimatol. Palaeoecol. 437, 33–41. doi:10.1016/j.palaeo.2015.07.022
Zhang, K., Bokka, H. K., and Lau, H. C. (2022). Decarbonizing the energy and industry sectors in Thailand by carbon capture and storage. J. Pet. Sci. Eng. 209, 109979. doi:10.1016/j.petrol.2021.109979
Keywords: carbon storage, Khorat Plateau, petroleum reservoir, evaporite, alkaline basalt
Citation: Chenrai P, Jitmahantakul S, Bissen R and Assawincharoenkij T (2022) A preliminary assessment of geological CO2 storage in the Khorat Plateau, Thailand. Front. Energy Res. 10:909898. doi: 10.3389/fenrg.2022.909898
Received: 31 March 2022; Accepted: 06 July 2022;
Published: 05 August 2022.
Edited by:
Yihuai Zhang, Imperial College London, United KingdomCopyright © 2022 Chenrai, Jitmahantakul, Bissen and Assawincharoenkij. This is an open-access article distributed under the terms of the Creative Commons Attribution License (CC BY). The use, distribution or reproduction in other forums is permitted, provided the original author(s) and the copyright owner(s) are credited and that the original publication in this journal is cited, in accordance with accepted academic practice. No use, distribution or reproduction is permitted which does not comply with these terms.
*Correspondence: Piyaphong Chenrai, UGl5YXBob25nLkNAY2h1bGEuYWMudGg=