- 1Division of Sustainable Development, College of Science and Engineering, Hamad Bin Khalifa University, Doha, Qatar
- 2Biological and Environmental Science and Engineering Division, Environmental Science and Engineering Program, King Abdullah University of Science and Technology (KAUST), Thuwal, Saudi Arabia
- 3KAUST Climate and Livability Initiative, King Abdullah University of Science and Technology (KAUST), Thuwal, Saudi Arabia
The healthcare sector is responsible for a significant portion of global carbon dioxide emissions, accounting for approximately 5% of the total. As energy demand in the sector continues to rise, sustainable solutions are urgently needed. Hospitals and healthcare facilities require a range of engineering services, including heat ventilation and air conditioning systems, hot and domestic water supply systems, and backup electricity systems. These energy-intensive services offer an excellent opportunity to integrate renewable energy sources and reduce the carbon footprint of healthcare facilities. This study presents a case study of a hospital located in the Gulf Cooperation Council (GCC) that utilizes a solar-collected water-heated system. The research aims to investigate the impact of adding multi-solar collector and photovoltaic systems to healthcare facilities, analyze the system’s thermodynamic efficiency in terms of energy and exergy, assess its technical and economic viability, and gauge the adoption rate of solar systems by healthcare technical departments. The results demonstrate that the solar thermal system provides around 12% of the total energy needed for the hot water system, while the solar PV system contributes approximately 29.6% of the total load for the HVAC system. This study explores the potential of using solar energy systems in healthcare facilities in the GCC region, analyzing their technical, thermodynamic, and economic viability. It promotes the adoption of solar systems in GCC and Middle East healthcare facilities and identifies research gaps related to solar systems implementation in healthcare facilities in the GCC. The study highlights the potential benefits of solar energy systems in terms of energy efficiency, cost savings, and environmental sustainability, with implications for healthcare facilities in the region and beyond. By utilizing renewable energy in healthcare facilities, the sector can reduce its carbon footprint and contribute to a more sustainable future.
Introduction and background
Hospitals and health facilities are found in every city worldwide and often have similar utilities or engineering, mechanical, and electrical plumbing services. Two main mechanical systems in healthcare facilities are heat, ventilation, air conditioning, and domestic water. These systems supply healthcare facilities with air within specific controlled variables: temperature and humidity. They are subject to strict indoor air quality standards such as The American Society of Heating, Refrigerating, and Air-Conditioning Engineers (ASHRAE). Similarly, hot and cold domestic water is subjected to strict standards and regulations that control variables such as temperature and chemical dosing. In some cases, a unique water application requires further quality control of water that can only be obtained through water deionizers and reverse osmosis systems. Other mechanical systems which are typically found in healthcare facilities are not related to the results of this study and will not be highlighted. On the other hand, electrical systems, such as backup generators and uninterruptable power supply, are two of the key systems that will be highlighted in this study.
The engineering services systems found in health facilities are highly energy-intensive and require a high power demand. Using renewable energy systems to meet the high demand is optimum to make health facilities more environmentally friendly. The strict service requirements, regulations, and standards imposed on health facilities are the main reason for their high energy demand. A simple example is the National Fire Protection Association (NFPA) for health facilities’ backup generators which is frequently followed by most hospitals that dictate that backup generators must be activated within 10 seconds (Code, 2009). As a result of such standards, all generator sets must be equipped with water jacket heaters that pre-heat the engines for faster start-up time. These water jacket heaters run continuously and have high power demand; a solar photovoltaic (PV) system would be suitable to supply a portion of this power demand. Similarly, the collector and photovoltaic solar systems can supply a significant portion of the healthcare engineering services load demand.
Background
Many studies either investigate or design existing thermal solar collectors or photovoltaic systems installed in hospitals. For example, in their research, Tsoutsos et al. (Tsoutsos et al., 2010) examine the solar fraction of a solar cooling system installed in a Greek hospital. They found that the solar fraction cooling and heating for the established solar collectors’ system size can reach as high as 74% and 71%, respectively. Lima et al. (Lima et al., 2015) use simulation to study the technical and financial viability of a water solar collecting system for a hospital laundry in Brazil. Lima found that the solar heating system could result in 6.3% of the initial cost, making the system more green and cost-effective. Meanwhile, in a Cyprus hospital, Kassem’s et al. (Kassem et al., 2021) research objective was to evaluate the potential of photovoltaic solar power. Their research found that annual electricity generated from the proposed photovoltaic system varied between 2.63 GW and 2.85 GW. In addition to the economic and environmental benefits of installing solar energy systems in hospitals, a significant improvement in resilience is added. In their research, Lagrange et al. (Lagrange et al., 2020) attempt to quantify the benefits provided by an improvement of energy resilience after adding renewable energy sources to a hospital. Their results show a possibility to increase resilience in power outages and an overall savings of approximately $440,000 over 20 years of lifetime. According to the International Energy Agency, the healthcare sector contributes approximately 5% (2 billion tons) of global CO2 emissions, while the energy, transportation, industry, and residential/commercial sectors contribute higher percentages, with energy at 70%, transportation at 15%, industry at 10%, and residential/commercial at 8%. In his study, Vourdoubas et al. (Vourdoubas, 2019) demonstrate the high energy consumption of a hospital in Greece to be around 280 kWh m2 per year and the CO2 emissions due to operation to be approximately 168 kg CO2 m2 per year. He also estimated the cost required for different combinations of solar thermal energy, solid biomass, and solar-PV energy to supply the hospital’s energy demand and provide that it would be profitable to replace conventional energy sources. Meanwhile, in Iraq, in their study Ali (Ali, 2021) designed a PV system for a hospital in Mosul city. In a country where power security is poor and routine blackouts occur, power through PV panels would enhance the resilience of the hospital and reduce the dependency on backup generation.
Chau’s (Chau et al., 2018) case study focuses on the cost and solar efficiency daily operation of a New Jersey hospital’s microgrid containing PV and energy storage systems. Their results encourage investing more in energy storage systems to capitalize on the excess energy generated from the system and store it for later use. However, Chau’s conclusion is only applicable to their case study, particularly hospital location, as the economic viability of the PV and energy storage systems is directly affected by several regional factors such as weather, gross domestic product (GDP), policies, and so on. Examples of support policies for rooftop PV include net metering, feed-in tariffs, tax incentives, and rebates. Alrawi’s results (Alrawi et al., 2022) in Qatar further elaborated on the discrepancy in results. He provides that energy storage systems are not yet economically viable in his region under their current conditions. This revelation opens up new opportunities for research on solar systems case studies depending on their regions. Ghaleb et al. (Ghaleb and Asif, 2022) examine the feasibility of utilizing solar PV systems on commercial building rooftops, identifying physical hurdles and calculating roof utilization factors and PV utilizable area using remote sensing techniques and site visits. The study found that commercial building rooftops have low usability for solar PV due to 16 different types of physical hurdles, leading to roof utilization factors ranging from 0.45 to 0.52, depending on the orientation of the building. The PV utilizable area at the city level was also calculated using regression analysis. At the same time, Soto et al. (Soto et al., 2022) review the challenges and opportunities for implementing solar energy in health centers in developing and underdeveloped countries, particularly in remote or rural areas. The study identifies challenges and opportunities in operational, environmental, and economic impact areas and shares best practices for implementing solar energy in the healthcare sector.
One common theme that most studies on using renewable energy sources in hospitals is increased resilience (Vaziri et al., 2020; Cohen, 2016). For example, Kyriakarakos et al. (Kyriakarakos and Dounis, 2020) discuss the feasibility of renewable energy sources and their capacity to accomplish energy efficiency and on-site power generation. In addition, Liu et al. (Liu et al., 2021) discuss energy storage systems’ role in increasing resilience in healthcare. By calculating economic indicators such as net present cost (NPC) and cost of energy (COE), they could gauge the improvement in a hospital’s microgrid resiliency. Furthermore, Achour et al. (Achour et al., 2014) investigate hospital resilience to natural hazards through the classification and performance of utilities. The six utilities they identified were electricity, gas supply, water supply, landline telecommunication, mobile phone, and Personal Handy-phone System. Achour concluded that hospitals should reduce dependency on these utilities and increase resilience by using alternative sources. Hospitals play a critical role in our societies; they must be robust to run their vital services. Integrating renewable energy systems in hospitals helps increase resiliency and utility security, improve environmental performance, and reduce costs in some cases (Roaf, 2007). In terms of thermo-electrical evaluation of a rooftop PV technology for a residential building, Sohani et al. (Sohani et al., 2023a; Sohani et al., 2023b) study reveals significant annual variations and suggesting the potential for enhanced performance through cooling techniques and concentrating strategies.
There is a noticeable absence of effective solar policies and a consequent lack of widespread solar collectors and PVs implementation in the GCC region. The utilization of renewable energy sources is limited, experimental, and isolated, with only a few government-led projects like solar farms being in operation. The lack of policies has hindered the growth of commercial and residential renewable energy applications. Consequently, there is a scarcity of research in this area, making any studies on this topic highly valuable for the region. A previous study (Alrawi et al., 2022) examines the economic viability of rooftop PV and energy storage systems in Qatar, using three datasets and several economic indicators. The results show that there is a real challenge for PV systems implementation due to the lack of support policies in the region and suggest that Feed in Tariffs and support incentive programs schemes are necessary to encourage the use of PV systems. Still, energy storage systems are unfeasible due to high costs. Cheap electricity prices and energy subsidies also present challenges. Demand-side management techniques and policies that encourage the self-use and selling of surplus power are recommended. In his research, Alghamdi (Alghamdi, 2018) concludes that using PVs to construct a hospital in Dammam is a viable option despite grid power interruptions. The simulated grid-connected PV system provided the most economical solution in all scenarios, with a sellback rate higher than the grid energy price by 5%, yielding the optimum solution. The proposed system reduces all emissions with a minimum percentage of 69.75%, and it results in lower costs compared to the existing system.
Studies that explore the utilization of multiple solar energy sources in conjunction with engineering services in real-world settings, particularly in healthcare facilities in the Gulf Cooperation Council (GCC) region. Most existing research in this field focuses on single-source solar energy and is often limited to simulation-based investigations. The current study aims to bridge this gap by conducting a thermodynamic analysis of a solar photovoltaic system for a GCC hospital that uses two forms of solar energy and is integrated with existing engineering systems. Furthermore, the study employs a unique Solar System evaluation method that draws on the expertise of a technically proficient engineering team. Consequently, this research has the potential to contribute significantly to the understanding of solar energy system design and evaluation in the GCC region and beyond while also providing insights into the technical, economic, and thermodynamic feasibility of integrating such systems in healthcare facilities.
The novelty of the study lies in its investigation of the potential use of solar energy systems in healthcare facilities in the GCC region. This is a unique and previously unexplored area of research, and the study is the first of its kind to examine the impact of multi-solar collector and photovoltaic systems in healthcare facilities in the region. The study also employs a novel Solar System evaluation method involving a realistic and technically proficient engineering team evaluation. Moreover, the thermodynamic analysis and project evaluation conducted in this study are unique and have the potential to benefit all countries in the region that share similar economic and geographical conditions. Therefore, this research has significant implications for healthcare facilities in the GCC region and beyond, as it offers new insights into the potential benefits of solar systems in terms of energy efficiency, cost savings, and environmental sustainability.
Research contribution
This research makes the following contributions to the field.
1. The study analyzes a hospital located in the Gulf Cooperation Council (GCC) region that utilizes a solar-collected water-heated system to investigate the potential impact of adding multi-solar collector and photovoltaic systems to healthcare facilities.
2. The study employs a novel Solar System evaluation method and is the first of its kind to examine the impact of such systems in healthcare facilities in the GCC region.
3. The research findings reveal that the solar thermal system provides approximately 12% of the total energy required for the hot water system, while the solar PV system contributes approximately 29.6% of the total load for the HVAC system.
4. The project is highly recommended by 86% of the reviewing engineers, demonstrating the technical and economic viability of the proposed solar energy systems. Moreover, this study promotes the adoption of solar systems in GCC and Middle East healthcare facilities to achieve energy efficiency, cost savings, and environmental sustainability.
Overall, this study investigates the previously unexplored area of solar energy systems in healthcare facilities in the GCC region, making it the first of its kind to examine the impact of multi-solar collector and photovoltaic systems in this context. The study’s employment of a novel Solar System evaluation method, along with its thermodynamic analysis and project evaluation, provides unique insights that have the potential to benefit not only the GCC region but also other countries with similar economic and geographical conditions. With findings showcasing the significant contributions of solar thermal and PV systems to energy requirements in healthcare facilities, as well as the strong endorsement from reviewing engineers, this research holds significant implications for the adoption of sustainable and cost-effective solar solutions, promoting energy efficiency and environmental sustainability in healthcare facilities in the GCC region and beyond.
Methods and procedures
The research methodology aims to investigate the potential impact of incorporating multi solar collectors and photovoltaic systems into healthcare facilities. The proposed system will be evaluated using thermodynamic analysis to assess energy and exergy efficiencies, as well as its technical and economic viability. Furthermore, the research will measure the adoption rate of solar systems by healthcare technical departments. The primary objective of this research is to determine the feasibility of using solar energy to reduce energy costs, increase energy security, and promote sustainability in healthcare facilities. Any technical or economic barriers to adoption will also be identified. To complete the project evaluation, experts in facilities management and project engineers will be surveyed.
By creating a combined solar collector and PV system, the proposed system aims to generate renewable energy and reduce the healthcare facility’s reliance on grid power. This will lead to a reduction in energy costs, improved energy efficiency, enhanced sustainability, and increased energy security. By utilizing solar energy to provide heating, cooling, and electricity, the healthcare facility can become more energy-efficient, reduce its carbon footprint, and contribute to a cleaner environment.
The proposed system includes renewable energy sources, engineering systems, and services. The primary sources of the proposed system are collected and photovoltaic solar energy. Although water is another input source, any additional additives, such as chemicals used in chemical dosing systems for water treatment, are not considered. The engineering systems that provide the hospitals’ utilities related to the proposed system are the heat ventilation and air conditioning (HVAC), chillers, domestic water supply system, backup generator sets, central battery system (CBS), and uninterruptable power supply (UPS). In addition, the proposed system supplies chilled water used for air cooling, hot water, and freshwater for domestic use, air, and electricity.
System description
The system can be broken down into multiple subsystems, starting with the hot water supply system, which consists of 50, 1.8 m2 solar thermal collectors and supplies four calorifiers with 1,500 L capacity each. The minimum flow temperature of the water leaving the calorifier/water heater should be 60°C. Maintaining the balance of flows to individual pipe branches and draw-off points is necessary to achieve the required circulating temperatures. As for the chilled water, a chiller connected to a cooling tower is used to supply chilled water at 5°C to a heat exchange related to a secondary chilled water cycle that supplies the air handling units. The chiller utilizes R134a, also known as Tetrafluoroethane (CF3CH2F), from the family of HFC refrigerants. With the discovery of the damaging effect of CFCs and HCFCs refrigerants on the ozone layer, the HFC family of refrigerants has been widely used as their replacement. Additionally, the secondary chilled water cycle cools down domestic water. In normal circumstances, temperatures should be delivered below 20°C, but there is growing evidence that supply temperatures may rise above 25°C in the summer months. The cold-water distribution system should be designed to have the same pressure for the hot water service at draw-off points. Figure 1 demonstrates the system components.
The proposed system for the healthcare facility supports crucial subsystems like the hot water supply system and chilled water system, which seamlessly integrate with the overall system to meet specific temperature requirements. The system has maintenance bypasses with controlled valves enabling continuous water supply to ensure uninterrupted operation and corrective maintenance. Additionally, the chilled water and HVAC system features standby motors and ancillary support systems, guaranteeing uninterrupted cooling during system maintenance or failures. Moreover, the integration of a renewable energy source system enhances the facility’s resiliency by reducing reliance on the utility grid and providing an alternative power source. This increased resiliency safeguards critical operations during grid failures, emergencies, or natural disasters. Simultaneously, the renewable energy source contributes to a cleaner environment, reducing carbon emissions and aligning with the facility’s commitment to sustainability.
January is considered one of the coldest months in the region, with temperatures fluctuating around 20°C; the water temperature through the thermal collector fluctuates between 22°C and 77 °C during the day and night cycles. In summer, this difference is expected to decrease and for the waveform to shift up due to increased temperature. Since the hot water supply temperature is 65°C, the electric heaters will compensate for the remaining heating energy demand. The maximum rooftop area available for PV panels is approximately 2,100 m2. The area selected for PV panels is 1800 m2 to accommodate pathways and spacing between PV panels. The selected area is enough to fit 1000 PV Panels. The PV panels chosen are 72 cells, 400 W Pmax, and a 1.8 m2 surface area. Figure 2 shows the rooftop image of the hospital with the current existing solar thermal system and the area selected for the proposed PV system.
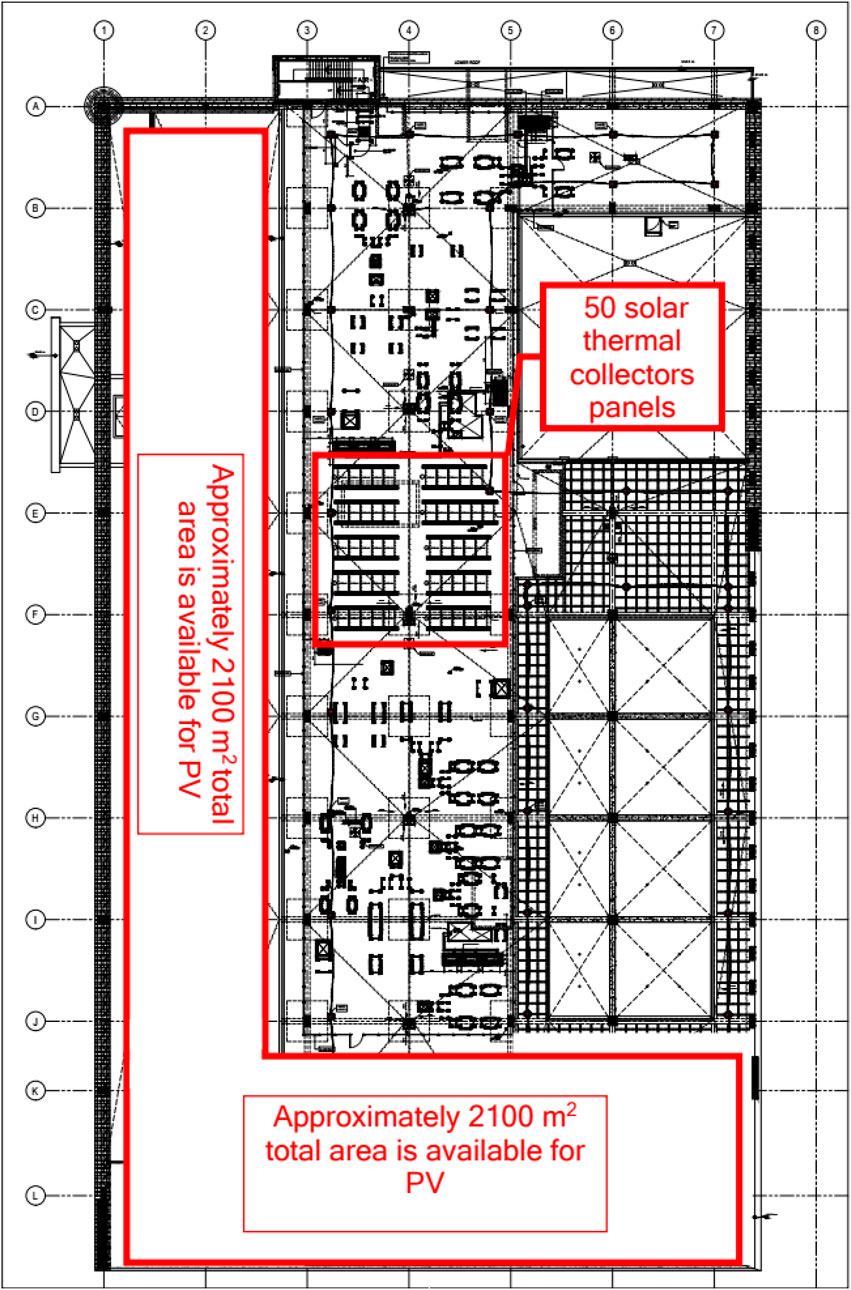
FIGURE 2. Hospital rooftop, the current location of solar thermal system, and proposed solar PV system.
Methodology and analysis
Table 1 demonstrates the operation conditions such as temperature, pressure, mass flow rate, enthalpy, entropy, and exergy of different points in the system, where each point is reflected in Figure 1. The ventilation and water systems have been designed in accordance with international standards such as BSI, ASHRAE, ANSI, ASME, and HTM to supply the hospital with optimum quality services. Therefore, the temperature, mass flow, and pressure parameters are designed to meet these standards. The enthalpy, entropy, and exergy values were calculated using Engineering Equation Solver (EES) software (Software). Table 2 demonstrates the system energy and exergy efficiencies obtained through EES.
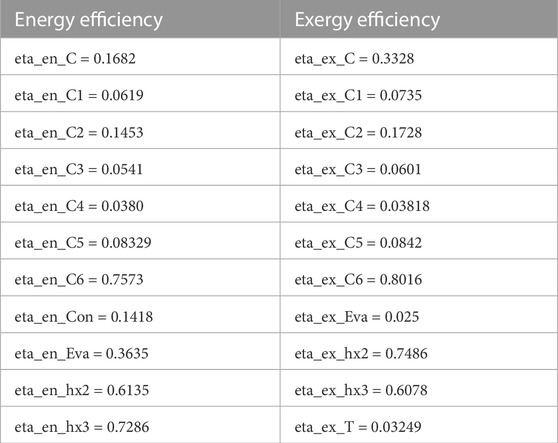
TABLE 2. EES results. The following abbreviations are used C: Compressor, Con: Condenser, Eva: Evaporator, hx: Heat Exchanger and T: solar thermal system.
The temperature and pressure real values are measured in real-time sensors and can be viewed using a building management system. The mass flow values are based on the building design calculations. The surrounding temperature is assumed to have 22°C, and atmospheric pressure is since the majority of the system is inside air-conditioned plant rooms.
The equations used throughout the study are listed below. However, losses associated with these systems, such as thermal losses due to insulation inefficiencies and air leaks, are not accounted for. In reality, water lost due to evaporation or leaks is compensated through a make-up water supply.
A. Solar Thermal System
B. Solar PV System contribuation to HVAC system load
C. Compressor
D. Heat Exchanger
E. Economic indicators
Where CF is cash flows, PV is the present value at k% interest for period n, and IC refers to the initial investment cost.
Where N is the lifetime of the system.
Results and Discussion
The results of the EES are depicted in Figure 5. Notable findings are the system contribution of the solar thermal system, which is approximately 12% of the total hot water system energy requirement with 46% efficiency, and the system contribution of the solar PV system, which is around 29.6% of the total HVAC load. The input solar energies of the thermal and PV systems are 180 kW and 400 kW, respectively.
The comparison between the GCC region’s solar thermal and PV systems relies on several factors. The primary factor is the need for the services each system provides; Simultaneously, electricity demand is very high due to the high demand for energy needed for air-conditioning in the region; hot water is a commodity required mainly during winter. However, hot water is necessary for healthcare facilities for multiple applications. The second comparison is related to the system’s complexity. Solar PV requires additional components and storage units; this factor becomes less relevant on a more extensive scale application in healthcare facilities where storage units are already used for power backup systems. The DC voltage supplied by the PV system can be integrated with the existing storage units, reducing cost and maintenance requirements. Both systems enhance the resiliency of the healthcare facility in case of emergencies. In addition, renewable energy further provides environmental and economic benefits.
Sensitivity analysis
Sensitivity analysis can be useful in evaluating the performance of solar thermal collectors and PV systems; the output of these systems will vary according to solar irradiance i. Figure 3 shows a typical solar irradiance curve (Alrawi et al., 2019). During the night, cloudy and rainy days, the missing solar thermal collectors and PV systems outputs are compensated through electric heaters and grid power.
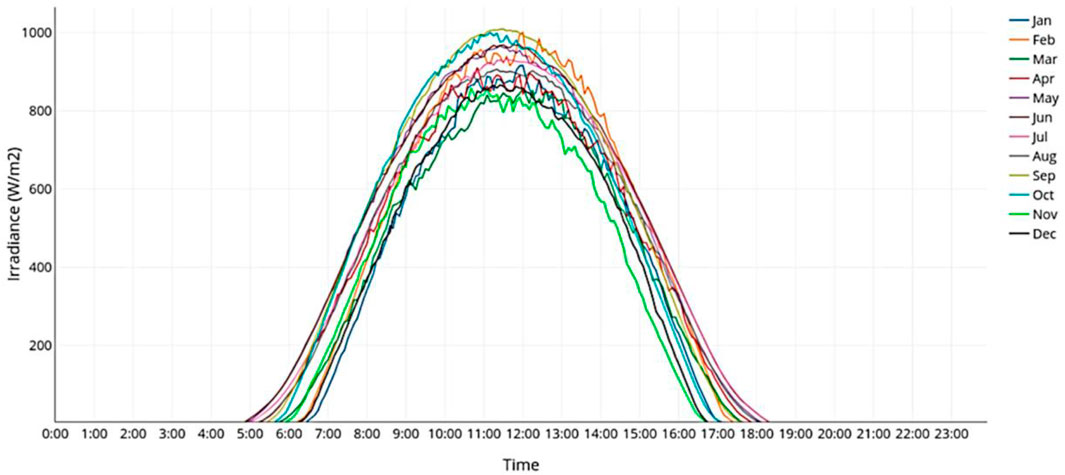
FIGURE 3. Solar irradiance daily curve (Alrawi et al., 2019).
Figure 4: the supply coefficient ratio of the solar thermal system as time changes throughout the day.
The figure shows that the region with a coefficient ratio larger than 1 supplies 100% of the heat demand to supply hot water at the required temperature. The region between 0 and 1 coefficient ratio requires a partial heat supply from the electric heaters. Finally, a region less than 0 requires a full supply from the electric heaters.
Figures 5, 6 represents simulated data using EES showing linear direct proportionality relationship between cooling requirement and external and utility input variables.
Figure 5 shows that the demand for domestic water cooling increases as the utility water supply temperature varies. The utility water supply temperature varies and typically varies with seasonal changes, adding to the region’s overall load demand discrepancy between the summer and winter seasons.
Figure 6 demonstrates the HVAC air conditioning energy demand for outdoor temperature increases. As expected, as the outdoor temperature increases, the cooling demand is determined by subtracting the exergy of supply air from the return air to the air handling units.
Economic analysis
The complete solar thermal system’s total cost is $ 88,000, relatively much smaller than the total cost of the PV systems. It was therefore decided to avoid investigating its economic indicators.
The economic indicators, such as the payback period (PB), must be established before the PV system’s economic viability can be evaluated. In order to do this, the PV payback time is calculated using an economic model. Other financial metrics, such as the Net Present Value (NPV) and Internal Rate of Return (IRR), are calculated in addition to the PV payback time. Numerous factors, including the PV decay rate, must be considered in the computation. The PV module’s efficiency typically degrades by 2%–3% over the first 2 years, at a maximum rate of 0.7% over the following 8 years and at a maximum rate of 0.5% over the final 2 years (IRENA, 2019). The photovoltaic panels’ material, the weather, the location of the installation, and the type of installation are some of the variables that determine the degradation rate. The typical lifetime of a suitable solar cell is at least 25 years. Due to GCC’s harshest, hottest, and most humid weather conditions, this is 5 years less than the average expected lifespan of solar panels worldwide. The figure chosen for this calculation is 0.7% because the range of average decay values is between 0.6% and 1.1%. As there is no actual implementation of FITs for rooftop PV installations in GCC, the tariff power rate for the residential sector is highly subsidized 4.9 c/kWh rate. Although it is impossible to estimate, we may expect that the government would try to promote home PV systems and adopt attractive rates; as a result, it is assumed that the selling price per energy unit will be around 13 c/kWh. The rate of 13 c/kWh is reasonable compared to average global rates, and the nearest country that utilizes FIT at 16 c/kWh is Jordan. In conjunction, the majority of the generated energy is most likely to be self-consumed, and the current governmental sector local electricity tariff is at 9 c/kWh. The current rate is highly subsidies, and previous trends show a steady decline in reducing electricity subsidies; therefore, choosing 13 c/kWh to represent the electricity tariff is more accurate over the project’s lifetime.
The panel cost, which is $280 (NREL) per panel cost in line with worldwide averages, makes up most of the system cost. All Solar System components (including the inverter) are included in the system balance, which is estimated to be worth $520,000. The system’s total cost is estimated to be around $892,000 and is demonstrated in Table 3. Due to the greater cost of water in GCC projected to be used for cleaning, the estimated yearly cost of $150 is a little high in comparison to other regions.
The PV panels and the solar irradiance details are demonstrated in Table 4. The annual energy production of the system is estimated to be approximately 665,973.00 kWh/year, depending on the average solar irradiance, the efficiency of the panels, and the total effective area.
Table 5 demonstrates the annual energy production of the PV system, which slowly degrades due to the decay rate. While the yearly revenue is also shown, which depends on FIT value, the inflation rate is not considered due to its low impact on the calculation. The GCC has, nonetheless, seen substantially lower inflation than most developed and developing nations. The International Monetary Fund estimates that in 2022, inflation will have averaged 3.6%. Additionally, the payback period, NPV, and IRR are demonstrated in Table 5 for different FIT values.
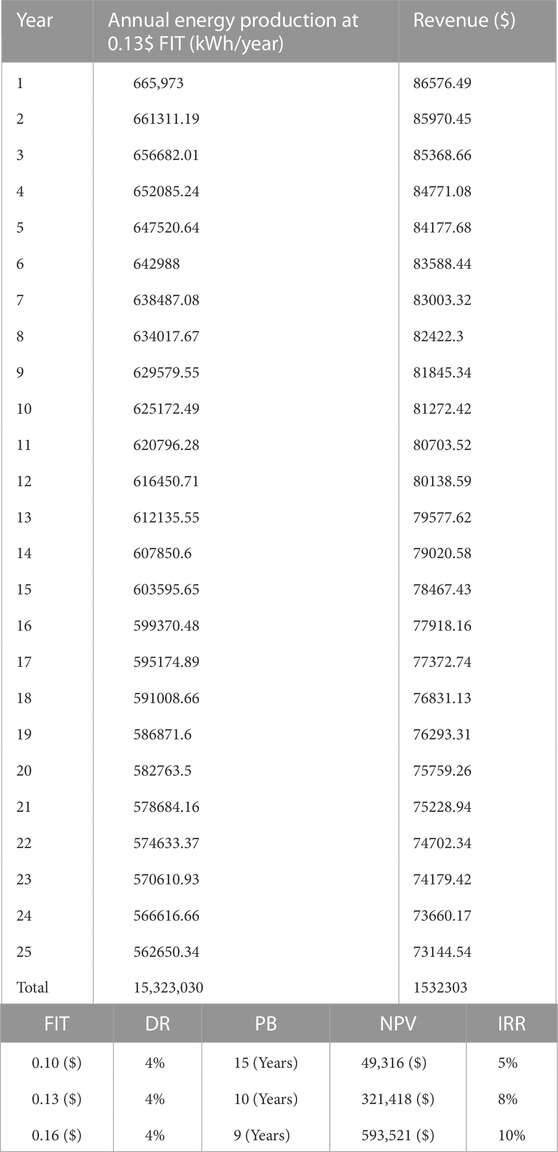
TABLE 5. Economic analysis showing annual PV system revenue, payback period, and financial details of the PV system.
Adoption analysis
A survey was conducted among 22 engineers specializing in project design and management; the engineers are well experienced in implementing engineering services projects in healthcare facilities. The survey aimed to gather experts’ insights and feedback regarding the multi-solar system’s feasibility, practicality, and potential benefits. The engineers were presented with the system design, economic analyses, and results. The engineers were then asked 14 questions through a survey related to their background, experience, and knowledge, in addition to their onion of the system’s design, implementation, and management. The participating engineers’ identities were kept anonymous, and the survey was distributed through emails. Furthermore, they were asked about the design evaluation, recommendations, and the potential challenges or considerations that need to be considered. The survey results provide valuable insights and recommendations for developing and implementing a sustainable and efficient energy supply system for healthcare facilities, which could positively impact the environment and the healthcare industry. The engineers’ background field and years of experience are demonstrated in Figure 7. Figure 8 illustrates the engineers’ strong familiarity with solar energy systems and strong attitudes toward the importance of investing in such systems.
The engineers were then asked about the factors that they think impact their decision to purchase solar energy systems. The results are depicted in Figure 9. Environmental value and benefit of the system, the local competitive market, and systems cost and affordability are the three most prevalent factors impacting the engineers’ decision to purchase solar energy systems. A project evaluation was conducted as part of the survey to identify a project’s strengths and weaknesses and use this information to improve future projects. By evaluating a project, stakeholders can gain insight into what worked well and what did not and can use this information to make better decisions in the future. Project evaluation can also help identify improvement areas, such as better project management processes, more effective use of resources, or more targeted stakeholder engagement strategies. The results in Figure 10 show that while most engineers approved the project, concerns about maintenance costs, payback period, and high upfront costs must be addressed. Only 27% of the evaluating engineers believe that governmental support policies for PV systems, such as net metering, feed-in tariffs, tax incentives, and rebates, are essential. This result is likely because GCC countries have high GDPs and their belief for cost restraints to have secondary priority. Around 35%–40% of engineers strongly feel the importance of increasing resilience and achieving self-sufficiency. Overall, the results suggest that implementing sustainable and efficient energy supply systems in healthcare facilities can have significant positive impacts on both the environment and the healthcare industry and that it is important to continue exploring and investing in renewable energy solutions. The results can present useful insight to GCC countries’ solar energy policymakers to develop, integrate and enable suitable solar systems supportive policies. Additionally, the results are helpful to all healthcare facilities in the region to consider this case study as a prime reference. Therefore, the results are applicable to all the GCC countries due to their similar economy and geographical conditions. The result is that neighboring countries and countries with similar economic and geographical conditions.
Limitations and assumptions
There were a few limitations and assumptions that were made throughout the study. The main limitation regarding the economic analysis is the feed-in tariff value, the PV panels cost, and BOS cost. Since there is no real application of feed-in tariff in GCC, the value used in the economic analysis was assumed by referring to Jordan, the nearest country where it is applied, and it was proportioned to meet average global values. The PV panels cost was also estimated, as the sales value is expected to be higher for products sold to GCC governmental corporations, a multiplier of 1.5 was assumed. BOS value includes contractor fee, which was assumed based on the local average contractors’ costs. Additionally, a few components were ignored in the thermodynamic analysis. The first and fourth heat exchanges were excluded due to the complexity of the setup where external systems interference deems the system balance equation inapplicable to these components.
Conclusion
In conclusion, the results of this study demonstrate the potential of utilizing combined solar thermal and PV systems in healthcare facilities in the GCC region to reduce energy costs, increase energy security, and promote sustainability. However, the exergy efficiency of the solar thermal system needs to be improved to enhance its overall efficiency.
The thermodynamic analysis results demonstrate that the proposed solar thermal system contributes approximately 12% of the hot water system’s energy requirement with 46% efficiency, while the solar PV system contributes around 29.6% of the total HVAC load, highlighting the potential of both systems to enhance energy efficiency and reduce reliance on conventional energy sources in healthcare facilities.
The economic analysis results of the PV system implementation in the GCC region with FIT values of 0.10 ($), 0.13 ($), and 0.16 ($) reveal that the corresponding payback periods are 15 years, 10 years, and 9 years, respectively. These findings demonstrate that higher FIT values are associated with shorter payback periods, indicating a faster return on investment. This highlights the economic viability and potential profitability of implementing PV systems in the GCC region, with the potential for achieving financial goals within a reasonable timeframe.
Based on the feedback from the survey of engineering staff, it is recommended that healthcare facilities in the GCC region consider adopting solar energy systems to achieve their energy goals. Additionally, it is recommended that the system’s design and operation be optimized to increase efficiency and reduce energy loss.
Future research could investigate the use of energy storage systems to increase the system’s reliability and performance during periods of low solar radiation. Additionally, further studies could explore the potential of integrating solar energy systems with other renewable energy sources, such as wind and geothermal, to create hybrid systems that offer more efficient and reliable energy production. Moreover, studying the economic feasibility of implementing renewable energy technologies in healthcare facilities would be helpful in understanding the potential financial benefits of adopting these systems. Future research should address the social aspects of community acceptance and health benefits, organizational considerations including financial implications and operational readiness, as well as policy aspects such as government incentives and regulatory frameworks, in order to comprehensively explore the challenges and opportunities of integrating renewable energy systems in healthcare facilities. Finally, analyzing the environmental impact of the proposed system in terms of reducing greenhouse gas emissions and promoting sustainability could also be a valuable area of future research.
Data availability statement
The original contributions presented in the study are included in the article/Supplementary material, further inquiries can be directed to the corresponding author.
Ethics statement
The studies involving human participants were reviewed and approved by HBKU—QRBI. The patients/participants provided their written informed consent to participate in this study.
Author contributions
OA-R: Conceptualization, Methodology, Software, Data curation, Writing- Original draft preparation. YB and SA-G: Supervision and Writing- Reviewing and Editing. All authors contributed to the article and approved the submitted version.
Conflict of interest
The authors declare that the research was conducted in the absence of any commercial or financial relationships that could be construed as a potential conflict of interest.
Publisher’s note
All claims expressed in this article are solely those of the authors and do not necessarily represent those of their affiliated organizations, or those of the publisher, the editors and the reviewers. Any product that may be evaluated in this article, or claim that may be made by its manufacturer, is not guaranteed or endorsed by the publisher.
References
Achour, N., Miyajima, M., Pascale, F., and Price, A. D. F. (2014). Hospital resilience to natural hazards: Classification and performance of utilities. Disaster Prev. Manag. Int. J. 23, 40–52. doi:10.1108/dpm-03-2013-0057
Alghamdi, A. H. S. (2018). Technical and economic analysis of solar energy application for a hospital building in Dammam, Saudi arabia author: Abdulrahim hamed S Alghamdi supervisor: Ramon zamora A thesis submitted to auckland university of technology in fulfilment of the requir. Available at: http://openrepository.aut.ac.nz/handle/10292/12314
Ali (2021). Fawaz design and sizing of solar plant for qayarah general hospital and simulation with the PV-sol program. NTU J. Eng. Technol. NTU J. Eng. Technol. E 1, 67–71. doi:10.56286/ntujet.v1i1.89
Alrawi, O., Bayram, I. S., Al-Ghamdi, S. G., and Koç, M. (2019). High-resolution household load profiling and evaluation of rooftop PV systems in selected houses in Qatar. Energies 12, 3876. doi:10.3390/en12203876
Alrawi, O., Bayram, I. S., Koc, M., and Al-Ghamdi, S. G. (2022). Economic viability of rooftop photovoltaic systems and energy storage systems in Qatar. Energies 15, 3040. doi:10.3390/en15093040
Chau, K., Mahani, K., Jafari, M. A., and Haghani, S. (2018). Solar-powered microgrid capacity planning for a general hospital. 2018 IEEE Green Energy Smart Syst. Conf. IGESSC 2018, 1–6. doi:10.1109/IGESC.2018.8745520
Code, L. S. (2009). Nfpa 101® 2. The NFPA report is found at: https://www.nfpa.org/codes-and-standards/all-codes-and-standards/list-of-codes-and-standards/detail?code=101
Ghaleb, B., and Asif, M. (2022). Application of solar PV in commercial buildings: Utilizability of rooftops. Energy Build. 257, 111774. doi:10.1016/j.enbuild.2021.111774
Kassem, Y., Gökçekuş, H., and Güvensoy, A. (2021). Techno-economic feasibility of grid-connected solar pv system at near East university hospital, northern Cyprus. Energies 14, 7627. doi:10.3390/en14227627
Kyriakarakos, G., and Dounis, A. (2020). Intelligent management of distributed energy resources for increased resilience and environmental sustainability of hospitals. Sustain. 12, 7379–7413. doi:10.3390/su12187379
Lagrange, A., de Simón-Martín, M., González-Martínez, A., Bracco, S., and Rosales-Asensio, E. (2020). Sustainable microgrids with energy storage as a means to increase power resilience in critical facilities: An application to a hospital. Int. J. Electr. Power Energy Syst. 119, 105865,. doi:10.1016/j.ijepes.2020.105865
Lima, T. P., Dutra, J. C. C., Primo, A. R. M., Rohatgi, J., and Ochoa, A. A. V. (2015). Solar water heating for a hospital laundry: A case study. Energy 122, 737–748. doi:10.1016/j.solener.2015.10.006
Liu, J., Jian, L., Wang, W., Qiu, Z., Zhang, J., and Dastbaz, P. (2021). The role of energy storage systems in resilience enhancement of health care centers with critical loads. J. Energy Storage 33, 102086. doi:10.1016/j.est.2020.102086
NREL NREL solar market research and analysis. Available at: https://www.nrel.gov/solar/market-research-analysis/solar-decision-support.html.
Roaf, S. (2007). Resilient hospital design: The zero carbon cooling challenge. AIVC Conf. Build. Low. Energy Cool 2, 2004–2007.
Software, F.-C. Energy equation solver. Available at: https://fchartsoftware.com/ees/.
Sohani, A., Cornaro, C., Shahverdian, M. H., Hoseinzadeh, S., Moser, D., Nastasi, B., et al. (2023b). Thermography and machine learning combination for comprehensive analysis of transient response of a photovoltaic module to water cooling. Energy 210, 451–461. doi:10.1016/j.renene.2023.04.073
Sohani, A., Sayyaadi, H., Moradi, M. H., Zabihigivi, M., Arıcı, M., Shahverdian, M. H., et al. (2023a). An in-depth thermo-electrical evaluation of a rooftop PV technology for a residential building using advanced infrared thermography. Eng. Anal. Bound. Elem. 152, 243–258. doi:10.1016/j.enganabound.2023.04.010
Soto, E. A., Hernandez-Guzman, A., Vizcarrondo-Ortega, A., McNealey, A., and Bosman, L. B. (2022). Solar energy implementation for health-care facilities in developing and underdeveloped countries: Overview, opportunities, and challenges. Energies 15, 8602. doi:10.3390/en15228602
Tsoutsos, T., Aloumpi, E., Gkouskos, Z., and Karagiorgas, M. (2010). Design of a solar absorption cooling system in a Greek hospital. Energy Build. 42, 265–272. doi:10.1016/j.enbuild.2009.09.002
Vaziri, S. M., Rezaee, B., and Monirian, M. A. (2020). Utilizing renewable energy sources efficiently in hospitals using demand dispatch. Energy 151, 551–562. doi:10.1016/j.renene.2019.11.053
Keywords: integrated system, renewable energy, solar adoption, solar energy, healthcare
Citation: Al-Rawi OF, Bicer Y and Al-Ghamdi SG (2023) Sustainable solutions for healthcare facilities: examining the viability of solar energy systems. Front. Energy Res. 11:1220293. doi: 10.3389/fenrg.2023.1220293
Received: 10 May 2023; Accepted: 13 July 2023;
Published: 28 July 2023.
Edited by:
Siamak Hoseinzadeh, Sapienza University of Rome, ItalyReviewed by:
Hassan Bazazzadeh, Poznań University of Technology, PolandSunil K. Sansaniwal, Malaviya National Institute of Technology, India
Grigorios L. Kyriakopoulos, National Technical University of Athens, Greece
Copyright © 2023 Al-Rawi, Bicer and Al-Ghamdi. This is an open-access article distributed under the terms of the Creative Commons Attribution License (CC BY). The use, distribution or reproduction in other forums is permitted, provided the original author(s) and the copyright owner(s) are credited and that the original publication in this journal is cited, in accordance with accepted academic practice. No use, distribution or reproduction is permitted which does not comply with these terms.
*Correspondence: Sami G. Al-Ghamdi, c2FtaS5hbGdoYW1kaUBrYXVzdC5lZHUuc2E=