- 1Department Management of Regional Energy Systems, Brandenburg University of Technology, Cottbus-Senftenberg, Germany
- 2Institute for Ecological Economy Research, Berlin, Germany
Coal has been one of the main fuels used in Europe. Its decreasing role due to the ongoing transformation of the energy system will create significant socio-economic challenges. The switch into renewable energy systems could be an alternative to maintain jobs and economic activities within the affected regions. Biomass use and bioenergy can play an important role in the energy transition. Instead of energy crops, forest and agricultural residues should be used as biogenic energy sources in the future to avoid impacts on land use and food security. The main objective of this article is to investigate the biomass potential of a coal region and to provide scenarios for the future development of bioenergy production. Due to the changing framework conditions and, as a result, the different biomass focuses, previous bioenergy potential estimates must be reviewed. The methods for determining the potential of biomass for energy production was used for Lusatia (in German: Lausitz), the second largest coal region in Germany. These methods can also be applied in other regions. As a first step, the regional status quo assessment of cultivated areas and yields had decisive relevance for calculating biomass potential ranges. In a second step, the current bioenergy facilities in the region were identified, with a focus on power and heat production. The third step was the estimation of future regional bioenergy use. Therefore, the regional potential was gathered with the generally supra-regional framework conditions. For this purpose, national scenario studies were used, which contain the relevant target values and framework conditions. Two scenarios were developed for future bioenergy estimations: a conservative path based on the current policies and a progressive path, derived from the goal of climate neutrality by 2045. The results show a qualitative comparison among both scenarios and the previously determined potential ranges. Bioenergy can probably contribute to achieving climate neutrality with an increase in wood-fired systems, while agricultural bioenergy potential is likely to decline. In the discussion section, however, the uncertainty of these results is pointed out, as future use of bioenergy will be heavily influenced by the regulatory framework, competition with material use and the influences of climate change.
1 Introduction
The decline of coal mining throughout Europe can be understood as a vital part of the energy transition, as energy systems move away from fossil to renewable energy sources (Fouquet, 2010; Mata Pérez et al., 2019; Cała et al., 2021). Simultaneously, this can result in a loss of revenue with significant socio-economic impacts, specially for the affected regions (Alves et al., 2018; Cha, 2020). Therefore, a so-called Just Transition Mechanism (European Commission, 2020), proposed under the European Green Deal (European Commission, 2019), was established to support structurally weak and specially coal regions. The funding mechanism will be a key tool for supporting coal regions in their structural changes (Gerbelová et al., 2021) while aiming at their climate and environmental objectives (IPCC, 2018). Germany’s decision to phase-out coal mining and power generation by 2038 (BMWK, 2022) can in addition to the national subsidies for coal regions (both established in 2019) accelerate this transition.
The region Lusatia (in German: Lausitz), which is the focus of this case study, is the second largest coal region in Germany. The case study region covers the German part of Lusatia, which in turn extends across two federal states. In the south of the federal state of Brandenburg, the districts of Dahme-Spreewald, Elbe-Elster, Oberspreewald-Lausitz and Spree-Neiße as well as the city of Cottbus belong to Lusatia, as well as in the north-east of the federal state of Saxony the districts of Bautzen and Görlitz. Compared to other German regions, Lusatia has below-average indicators for growth, employment and per capita productivity (Berger et al., 2019; Hirschl et al., 2022). In the light of the existing infrastructure, a differentiated energy industry, various research centers, and potentially suitable land for renewable energy projects, Lusatia can in fact be considered as a region with a strong potential for the regional development (Richwien et al., 2018; Hirschl et al., 2022). The current profile of the bioenergy sector in Lusatia is mainly based on biogas production for heat and electricity generation. The main sources of biomass used in the biogas plants are silage maize and manure. Small-scale heating applications based mostly on waste wood also play an important role in the regional energy matrix.
Renewable energy technologies are a central pillar of the energy transition and can be a significant source of new jobs. In general, bioenergy plays an important role in the substitution of fossil energy necessary to meet global climate targets (IPCC, 2018). Bioenergy makes up 60% of renewable energy in the EU—the majority from solid biomass (IEA, 2022). Biomass is any material of biological origin and can be composed of various, complex components. Natural sources such as crops, trees, and grass are extremely variable in their botanical characteristics and technical applications (Anukam and Berghel, 2020; Tursi and Olivito, 2021). Forest biomass is the most popular and largest contributor to the bioenergy mix globally, accounting for more than 85% of all biomass used for energy purposes (Stolarski et al., 2020; Anca-Couce et al., 2021; IEA, 2022).
Bioenergy includes energy supply for a multitude of energy end-uses including heat, electricity and transport energy carriers (Cornelissen et al., 2012; Guo et al., 2015). Increasing bioenergy supply may cause challenges regarding biomass cultivation and land use impacts (Leemans et al., 1996; Plevin and Kammen, 2013; Slade et al., 2014; Creutzig et al., 2015). The use of modern bioenergy has on average increased by about 3% per year between 2010 and 2022 and is on an upward trend (IEA, 2023). Since biomass demand continues to increase, feedstock trade will have to be broadened to other biomass sources. Crop residues from agriculture or forestry minimizes competition for land, thereby securing a balanced provision of food, material and energy (Matzenberger et al., 2015; IPCC, 2019). Agricultural residues include harvesting and processing residues, while forestry residues include logging, thinning, and wood processing residues (Smith et al., 2014). These bioenergy feedstocks are inexpensive, can generate additional income and have low greenhouse gas (GHG) emissions, if harvested sustainably (Hanssen et al., 2020).
The requirement of climate neutrality from the Paris climate agreement goes hand in hand with the decarbonization of all economic sectors (UN, 2015). For this reason, fossil raw materials must be removed not only from energy use, but also from all material applications. The generic term bioeconomy describes the energy and material use of biomass, but also its priority use for food and animal feed (Fritsche et al., 2020). The European Bioeconomy Strategy paved the way for a more resource-efficient society while ensuring environmental protection (European Commission, 2012). Within the broader concept of bioeconomy, bioenergy will be instrumental to create a future in which not only climate change mitigation is guaranteed, but also where the Sustainable Development Goals (SDG) are met (Junginger et al., 2019; Scarlat and Dallemand, 2019; UN, 2022). In this way, biomass should be seen as a scarce and valuable resource that needs to be used efficiently. It should be first processed and used materially several times, thereby utilizing and recycling by-products; and secondly used as renewable energy while minimizing overall energy use (Muscat et al., 2021). This favors cascading biomass, an integrated agriculture and energy system that co-produces bioenergy streams alongside a number of useful materials and chemicals (Odegard et al., 2012; Jarre et al., 2020). Due to the increasing material requirements, bioenergy potential can only increase if the biomass potential increase in total. This seems to be less likely for most regions, since land competition and land shortages caused by climate change is constantly increasing (EEA, 2017; IPCC, 2022). It follows that previous scenarios on biomass potential for energy use must be reviewed and updated in light of recent findings, developments and needs mentioned above. In addition, national or large-scale potential analyses and scenarios should be broken down for smaller regions to obtain robust information for regional decision-makers, taking into account the specific regional situation.
Against this background, the main objective of this article is to investigate the biomass potential of a coal region regarding the restrictions mentioned and to provide scenarios for the future development of bioenergy production. In this context, a primary focus on electricity and heat production from biomass is given, as these are still the most important biomass outcomes and the data basis is comparatively better. In contrast, the future development of liquid biofuels can hardly be estimated in few valid studies, due to the difficulty in distinguishing biofuels from other bio-based materials production in biorefineries (Prognos et al., 2021).
The practical objective is to calculate the actual biomass potential and to develop new scenarios for the coal region of Lusatia. Currently, only outdated studies regarding biomass production and bioenergy are available for the region (Scheuermann et al., 2012; Zschau et al., 2013). These studies were conducted under different technical and political conditions at the time of publication. By developing a conservative scenario (corresponding to current policies) and a progressive scenario (corresponding more to the requirement level of climate neutrality), regional decision-makers receive information about possible development options and corridors. The third is a methodological objective: to present a transferable approach for a valid estimation in view of the still poor regional data availability on the topic of the energetic use of biomass, as the large variety of biomass and different forms of use makes quantifying regional potentials difficult (Kaltschmitt et al., 2009; Pietsch, 2017; Rai and Ingle, 2019). These methods give a framework for other institutions and organizations for investigating regional biomass potential. The results can deliver a picture of future bioenergy trends. On a broader scope, this research approach can be used by other energy transition regions, which are shifting from fossil-based systems to renewable energy sources.
2 Methods
The methodology used to determine regional biomass and bioenergy potential is presented below. Although energy use is a subset of the available biomass, the development of bioenergy is strongly driven by political framework conditions, which is why a scenario approach was used. The biomass potential represents the total amount available, which in addition to bioenergy use must also cover material use in the future. Due to the currently still very unclear future developments and also a comparatively poor data for material biomass use, the focus here is on the use of biomass for energy. In addition, there is a focus on agricultural and forestry biomass and not on the various biogenic waste fractions such as organic municipal solid waste or sewage sludge. Current scenarios, for example, for the federal state of Brandenburg, assume a decreasing importance of organic waste fractions for bioenergy due to the increase in the circular economy (Hirschl et al., 2023).
For the status quo-analysis a district-related research was carried out in Brandenburg and Saxony on the basis of publicly available statistics, official documents, internet sources and reports. The first step of the analysis was to research which biomass and residues are currently used in Lusatia. Table 1 summarizes the types of biomass and divides them based on the sources of origin and uses. The second step was to estimate their actual availability and to examine competition for use. For a reliable assessment, it was necessary to identify regional actors and find out what potential can be developed, what different exploitation interests exist and what technical challenges are involved.
Methodological approaches for data assessment were developed in order to plausibly depict the status quo of available biomass. In the absence of specific statistical biomass data for Lusatia, calculations and estimates were carried out according to statistical yearbooks for 2018 from the Federal State of Brandenburg (Amt für Statistik Berlin-Brandenburg, 2018) and the Free State of Saxony (Statistisches Landesamt des Freistaates Sachsen, 2018). These data were primarily used to determine the cultivated area and the amount of biomass produced in Lusatia. This calculation offers a first approximation of available quantities. In this way, area data was combined with information about crops and yields in order to calculate how much biomass of which type is produced in Lusatia.
The analysis of bioenergy plants that were operating at the time is based on statistical data from the Brandenburg and Saxony ministries, state offices for agriculture, head foresters, as well as the Climate Protection Atlas of Brandenburg (MWAE, 2023), the Saxon Energy Agency (SAENA, 2023), the Bioeconomy Atlas of the German Biomass Research Center (DBFZ, 2023) and the Biomethan Atlas (DENA, 2023a). The following parameters and aspects were determined: number of plants and installed thermal and electrical power and size class distribution with regard to the number of systems and installed capacity.
There are a large number of studies on the determination of biomass potential on a national or supra-regional scale (Ericsson and Nilsson, 2006; Zeller et al., 2012; Brosowski et al., 2015; Brosowski et al., 2016; Lundmark et al., 2015; Welfle, 2017; FNR, 2018; Hamelin et al., 2019; Klepper and Thrän, 2019). In contrast, only a few studies deal with the determination of potential on a regional level, and most of them only look at specific biomass fractions or influencing factors (Bentsen et al., 2018; Burg et al., 2018; Bao et al., 2020). Therefore, all available regional biomass potential data was used for the case of this article and the missing biomass was mainly derived from supra-regional or national studies (Brosowski et al., 2016; Klepper and Thrän, 2019; Majer et al., 2019).
The bioenergy potential in the Lusatia region was estimated based on data from Saxony and Brandenburg, from the Agency for Renewable Energies (AEE, 2013b; 2013a), as well as nationwide data from the Federal Environment Agency (Fehrenbach et al., 2017), the Agency for Renewable Resources (Brosowski et al., 2015) and the German Biomass Research Center (Majer et al., 2019).
The calculation of the bioenergy scenarios for Lusatia is based on the on the actual status quo analysis and on the expansion targets in accordance with the German Renewable Energy Act (EEG, 2023). However, these targets are not technology-specific and are not broken down by region. In order to determine plausible regional developments, two scenarios were drawn up that describe a range between a conservative path in line with existing policy instruments and a more progressive path. The scenario Current Policies is based on the federal and regional energy and climate regulations in place at the time of writing and on the study “RESCUE” made by the Federal Environment Agency (Purr et al., 2019). The RESCUE study refers to the target year 2050. The progressive scenario (Climate Neutral 2045) is based on the study “Climateneutral Germany 2045"(Prognos et al., 2021) with the climate neutrality target by 2045. This scenario depicts the regional efforts necessary to adequately support federal climate neutrality targets (Federal Climate Change Act, 2019) in all sectors and breaks down the use of bioenergy in a relatively differentiated manner. As biomethane data was not available in the study of Prognos et al. (2021), actual data from the German Energy Agency was used (DENA, 2023b).
As a last step, the regional bioenergy values determined were compared with the biomass potential and checked for plausibility.
3 Results
3.1 Agriculture and forestry
Lusatia has a total area of around 1.173 million hectares. This is subdivided into agricultural land with approx. 38% (439,600 ha), forest areas with approx. 38% (448,700 ha) and other areas with 24% (284,300 ha) such as urban areas, bodies of water, etc. (Amt für Statistik Berlin-Brandenburg, 2018; Statistisches Landesamt des Freistaates Sachsen, 2018). While Brandenburg has relatively homogeneous physio-geographical conditions for agriculture, Saxony has greater variability in soil slopes, altitude, climate and soil characteristics (Klüter, 2014). The average farm size in Brandenburg is around 245 ha and in Saxony around 139 ha, being far above the German average of around 60 ha (BMEL, 2020). Soil quality in Lusatia varies from sandy soils in southern Brandenburg to silty soils in northern Saxony. In recent years, agricultural yields in Lusatia have stagnated and tend to decrease (Amt für Statistik Berlin-Brandenburg, 2018; Amt für Statistik Berlin-Brandenburg, 2020). Since Lusatia is considered to be a very dry region within Germany, increased periods of drought in particular will pose major challenges for agriculture, forests and forestry in the future.
Considering the absence of specific regional data for shares of biomass use in Lusatia, the national shares were used to estimate the current situation in Lusatia. Around 60% of the agricultural land in Germany is used for the production of animal feed, 22% for the production of food and 14% for energy crops. Only 2% of biomass was destined to industry and 2% for set-aside areas (FNR, 2022a). Hence, it is estimated that approx. 264,000 ha of the total agricultural land in Lusatia are used for animal feed, approx. 97,000 ha for the cultivation of food, approx. 61,000 ha for energy crops, 9,000 ha for industrial crops and about the same amount for set-aside areas.
In 2018, silage maize was grown on 16% of the arable land in Lusatia with a production of approx. 1.2 million tonnes (Amt für Statistik Berlin-Brandenburg, 2018; Statistisches Landesamt des Freistaates Sachsen, 2018). Silage maize is used as animal feed (approx. 67%) and as a substrate for biogas plants (approx. 33%), taking up the largest area of cultivated renewable raw materials in Germany (FNR, 2022a). Since 2018, there is a smooth decrease in the use of silage maize for biogas production and an increase in the animal feed sector (FNR, 2022a).
Cereals were grown on more than half of the total arable land in Lusatia (Figure 1). The total grain production in Lusatia was around 700,000 tonnes. Cereals are primarily used in the animal feed and food industry. The conversion of cereals into liquid biofuels currently plays only a minor role.
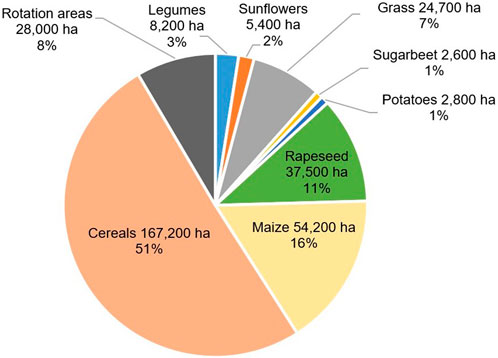
FIGURE 1. Cultivated crops in Lusatia. Own diagram based on the Statistical Yearbooks of 2018 from Brandenburg (Amt für Statistik Berlin-Brandenburg) and Saxony (Statistisches Landesamt des Freistaates Sachsen, 2018).
In 2022, 5.2 million cubic meters of wood (without bark) were felled in Brandenburg’s forests (Statistik Berlin-Brandenburg, 2022) and around 1.5 million cubic meters in Saxony (Staatsbetrieb Sachsenforst, 2022). Hence, it is estimated that approx. Two million m³ of wood can be estimated for Lusatia. Wood stores carbon that forest trees previously filtered out of the atmosphere. Wood is therefore an important climate protector, specially if it comes from regional, sustainable production with short transport routes. Harvesting and processing of wood generate regional value chains, specially in rural areas. Most of the wood is processed in sawmills. Around a third is used for the paper and wood-based material production or for thermal use (Schmitz et al., 2022). While trunk and industrial wood is used for material purposes, energy wood is obtained through thinning and the use of forestry wood residues.
3.2 Residual and waste materials
Biomass residues fall in a variety of places in a wide variety of activities, e.g., in the industry, during care measures of green spaces; in harvesting crops or in sewage treatment plants. The amounts of the individual residues can be small and distributed over a region. Around 215 million tonnes (fresh weight) of agricultural residues are produced in Germany every year (Zeller et al., 2012). Of this, an average of 30 million tonnes is grain straw (Zeller et al., 2012). Straw is the dry stalk and leaves of the threshed grain (Townsend et al., 2018; Harun et al., 2022). The amount of straw in Lusatia was calculated on the basis of the total grain production in Lusatia in 2018 and the German grain-straw ratio of 1 (grain) to 0.9–1.4 (straw) (FNR, 2022b). Since wheat and rye are the types of grain most commonly cultivated in Lusatia, the average of 0.9 was used here as the grain-straw ratio. With a grain harvest of around 700,000 t (Statistisches Landesamt des Freistaates Sachsen, 2018; Amt für Statistik Berlin-Brandenburg, 2019), this corresponds to an estimated value of around 630,000 t straw. Which part of this will actually be accessible depends on soil and weather as well as the management of litter in animal husbandry. According to Zeller et al. (2012), approx. 27%–43% of straw can be removed from the soil without affecting organic matter quantities (humus balance).
Manure refers to liquid and solid excrements from animal husbandry (Rayne and Aula, 2020). The amount of manure in Lusatia was estimated based on animal units and the national average values for manure (FNR, 2022c). In 2018, around 330,000 cattle and cows and around 385,000 pigs were kept on Lusatian farms. Thus, a total manure quantity of approx. 6.2 million m³/a can be estimated based on the national average values for manure (FNR, 2022c). Electricity and heat can be obtained from manure in biogas plants, and the digestate can be used as fertilizer after the process (Cai et al., 2019; Anacleto et al., 2022). A sustainable operation of biogas plants needs to ensure recycling management and avoid over-fertilization (Sommer and Knudsen, 2021).
Wood and forestry residues are leaves, bark, sawmill by-products, wood shavings, landscape maintenance wood and waste wood (KIWUH, 2019; Braghiroli and Passarini, 2020; Thiffault et al., 2023). Waste wood arises from wood that has already been used as a material, e.g., in the construction sector, as packaging material or as old furniture in bulky waste. The recycling of waste wood currently takes place mainly for energy purposes in wood-fired power plants and for the production of chipboards. Smaller proportions of the total production are exported or used for other material purposes (Schmitz et al., 2022). Specific data on the volume of waste wood currently used in Lusatia was not available in the literature.
The most important residual wood in terms of volume are the sawmill by-products. One cubic meter of forest wood generates approx. 0.4 m³ of sawdust, wood chips, trimmings, rinds and splinters (FNR, 2018). Since no current data on sawmill by-products is available for Lusatia, estimates are based on this average data and on the total logging volume in Lusatia of 2 million m³/a of wood (Staatsbetrieb Sachsenforst, 2022; Statistik Berlin-Brandenburg, 2022). According to the German data for sawmills approx. 30% of the total volume of wood felled goes to sawmills and after processing it generates 40% sawmill by-products (FNR, 2022a). Hence, around 600,000 m³/a wood would flow into the Lusatian sawmills, producing around 240,000 m³ of sawmill by-products.
Landscape maintenance wood refers to branches and shrub cuttings (wood) as well as all organic residues that are used in the maintenance of public green spaces such as. B. parks or settlement and traffic areas arise. This also includes clippings from protected areas or from the care of field and bank trees, windbreaks or field hedges (Haak, 2015). There are specific studies regarding landscape conservation material for Brandenburg and Saxony (Stegner et al., 2010; Peters et al., 2014). Based on these data analysis, a potential of approx. 283,000 t/a was estimated for Lusatia.
3.3 Bioenergy status quo
In Lusatia, biomass is mainly used to generate heat and electricity in biogas plants, wood-fired thermal power stations and small combustion plants. Most of the current bioenergy production is based on energy crops and wood residues. In Table 2, the column shares of biomass (%) represents the shares (%) of the biomass resources used in different bioenergy plants in Lusatia. Since the regional shares were not available, we based the calculations on national shares for biomass use for energy generation (FNR 2018; FNR, 2019).
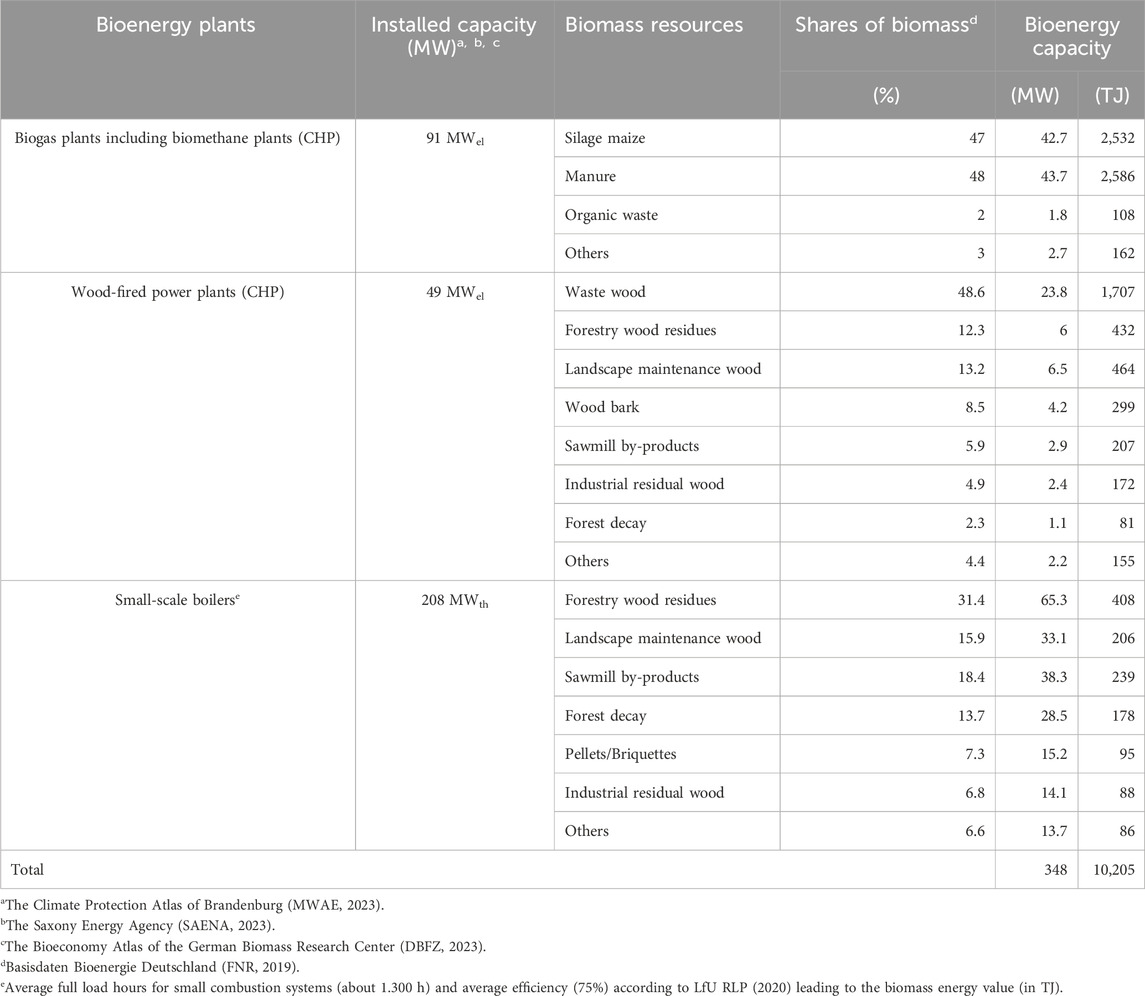
TABLE 2. Share of biomass resources in installed bioenergy plants in Lusatia and its capacity in MW and TJ. Own calculations based on FNR (2018, 2019), MWAE (2023), SAENA (2023), DBFZ (2023) and LfU RLP (2020).
In Germany, the most commonly used substrates for biogas production are silage maize with a share of around 47% and manure with 48% (Table 2). The use of organic waste plays a minor role in biogas production (FNR, 2022a). Maize silage has high biodegradability, energy density and biogas yields. It is the most profitable energy crop for most farmers in Germany. However, the massive cultivation of maize causes issues about food/feed competition and indirect land use change (Garcia et al., 2019; Hamelin et al., 2019). A total of 173 biogas plants are in operation in Lusatia, of which around 10 are small-scale liquid manure plants (Majer et al., 2019) and 14 are biomethane plants (DENA, 2023a; MWAE, 2023; SAENA, 2023).
The total installed capacity of all bioenergy plants in Lusatia in 2018 was 348 MW (see Table 2), of which 91 MWel refers to biogas plants (including biomethane). Assuming that these plants achieve an average number of full-load hours of 6,250 h/a (DBFZ, 2019), this results in around 569,000 MWh.
In Germany, there are currently around 700 wood-fired power plants and most of these plants are located in the Federal State of Bavaria. There is a total of 23 wood-fired power plants in Lusatia with an installed capacity of around 49 MWel (see Table 2). These Combined Heat and Power Plants (CHP) generate electricity and heat, and are usually located near wood-processing plants. Waste wood is the main used solid fuel in larger wood-fired CHP, accounting with approximately the half of the total bioenergy capacity (Table 2). CHP can operate flexibly due to the price development at the power markets while supporting decarbonization in district heating (Koch et al., 2020).
In smaller facilities the mostly used solid fuel is forestry wood residues (Dong et al., 2009). Small-scale boilers based on wood chips, pellets or split logs are widely used in Lusatia. The installed capacity in Lusatia is estimated at around 6,800 boilers, with an installed capacity of approx. 208 MWth (Table 2).
With regard to the increasing share of fluctuating renewable energies in the energy system (wind and solar energy) and the associated increasing demand for providing flexible energy, new requirements and challenges arise. Central questions include which options exist for biogas plants and for which sectors (transport, electricity, heat) is the use of biogas and thus the continued operation economical. Various approaches are being discussed (Daniel-Gromke et al., 2017), such as the direct marketing of (flexible) electricity, heat and outputs (fertilizers) as well as the provision of biomethane as fuel and its injection into the natural gas network. However, there is increasing competition for agricultural raw materials in view of the growing need for substitution in the material sector. Animal feed production is in competition with food security, since intensive livestock farming is heavily dependent on agricultural crops such as maize, soybeans, wheat and other grains. For these reasons, it is assumed that the biogas sector will not continue to grow.
3.4 Bioenergy potential
The total regional potential of energy crops and residues was mainly estimated based on the potential atlas of the federal states from the Renewable Energy Agency in Germany (AEE, 2013b; AEE, 2013a). This atlas shows on which area raw materials are cultivated (e.g., energy crops, forestry) and the amount of biogenic substances that (could) be accumulated (yield) on these areas. According to this study, less than 25% of the identified potentials in Brandenburg and Saxony can be attributed to the Lusatia region. The bioenergy potential reflects the proportion of final energy provided after conversion. For the calculation of the biomass potential, the entire amount of different biomass (in tonnes) like energy crops, straw, manure and wood residues (waste wood, landscape maintenance wood and sawmill by-products) was converted into terajoules (TJ) using specific conversion factors.
The technical biomass potential is derived from the theoretical biomass potential considering technical, physical and socio-ecological factors (Kaltschmitt et al., 2009). The technical biomass potential thus describes the time- and location-dependent amount of biomass that can be used primarily from a technical point of view for material or energetic purposes (Brosowski et al., 2015). Therefore, ranges were given to estimate the technical biomass potential, which are made up of the current biomass status quo in Lusatia, the potential values given in the literature (Stegner et al., 2010; AEE, 2013b; AEE, 2013a; Peters et al., 2014) and based on nationwide data for the development of biomass use (FNR, 2019). The ranges in the technical potential values (see Table 3) mainly reflect the tension between, on the one hand, a reduction of today’s amount of bioenergy and, on the other hand, a more consistent use of biomass. Therefore, ranges for the potentials are given in Table 3, which lie between the current magnitudes for biomass use (see Table 2) and the magnitudes determined for Lusatia from available literature sources.
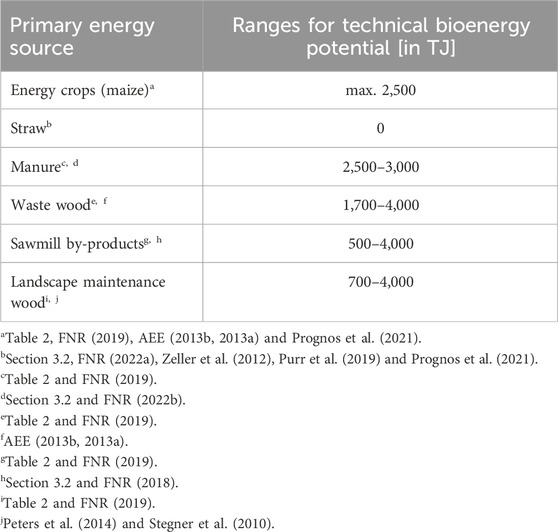
TABLE 3. Ranges for technical bioenergy potential in Lusatia. The potential are estimations based on the values given in the literature, on nationwide data for the development of biomass use and own calculations. The footnotes show the sources for estimations and are directly related to the ranges.
Compared to the neighboring regions in northern Brandenburg and western Saxony, the cultivation of energy crops (mostly maize) in Lusatia is less pronounced, having a potential of 15,000 TJ (AEE, 2013b; AEE, 2013a). According to the current policy framework, energy crop potentials of this magnitude will be no longer available for bioenergy use. In addition to the growing competition for land and increasing land scarcity (e.g., due to increasing demand for food and feed, solar energy, rewetting of moorland but also ongoing climate change), energy crops will be mainly required for material uses (Hirschl et al., 2023). For this reason, there is currently no longer any support for maize cultivation as main substrate for feeding biogas plants in Germany (EEG, 2023). It is therefore to be expected a maximum energy crop potential of around 2,500 TJ for the future in Lusatia (Table 3), which roughly corresponds to the current scope of utilization in this region (see Table 2). Considering this forecast, the cultivation of short rotation coppice (SRC) can become more important in the total mix of energy crops. Prognos et al. (2021) expected a share of more than 20% of SRC for 2030 in Germany. However, it can be assumed that these potentials will, firstly, vary greatly from region to region, secondly, that they will not be available exclusively for bioenergy, but may also be used predominantly for material purposes, and thirdly, that it may not be possible to develop them to the same extent in view of climate change, the resulting scarcity of land and the primacy of food production. Therefore, it is assumed an SRC range of zero to a maximum height according to the study of Prognos et al. (2021) cited above available for bioenergy uses.
The limited regional availability of sustainable biomass resources will increase the global trade of agricultural and forest residues. Increasing competition for material use can also be assumed for residues, which tends to reduce the available share for bioenergy. Based on the currently very low energetic use of straw in Germany, Purr et al. (2019) and Prognos (2021) assumed that there will be no significant increase in straw use for bioenergy as a solid fuel. Even though straw falls in large quantities in Lusatia (see Section 3.2), it should be used entirely as a raw material in other industries. Therefore, it is assumed that straw has no technical bioenergy potential in Lusatia. Here, technological constraints in the conversion of straw into energy, as well as the importance of straw for soil protection were taken into consideration when estimating the technical potential.
The total mass decay of manure in Lusatia is approximately 6.2 million m³/a (see Section 3.2), representing an estimated potential of 3,000 TJ (FNR, 2022c). Since the current use of manure as substrate in biogas and biomethane plants in this region is limited to a potential of approximately 2,500 TJ (see Table 2), its technical potential ranges from 2,500 to 3,000 TJ (Table 3). The quantity that can be utilized in the future depends to a large extent on meat consumption trends, animal welfare and available area.
In the AEE potential atlas, a waste wood potential of around 4,000 TJ is estimated for Lusatia (Table 3). The actually realizable waste wood potential depends on the environmental and energy policy framework. With the expiry of the 20-year EEG funding for the first EEG systems from 2021, the market for waste wood cogeneration systems will be faced with a new challenge. Since the current use of waste wood in CHP plants is limited to approximately 1,700 TJ (see Table 2), its technical potential ranges from 1,700 TJ to 4,000 TJ (Table 3).
The potential of sawmill by-products in Lusatia was estimated in approximately 240,000 m³/a (see Section 3.2), being converted to around 4,000 TJ. Since the current use of sawmill products as solid biofuel is limited to approximately 500 TJ (see Table 2), its technical potential ranges from 500 to 4,000 TJ (Table 3).
The potential of landscape maintenance wood was estimated in approximately 283,000 m³/a (see Section 3.2), being converted to around 4,000 TJ. Since the current use of landscape maintenance wood as solid biofuel is limited to approximately 600 TJ (see Table 2), its technical potential ranges from 700 to 4,000 TJ (Table 3). There are logistical and economic obstacles in particular that stand in the way of tapping into this potential.
3.5 Political framework for the energetic use of biomass
The type and scope of bioenergy use in Germany is strongly dependent on the political framework which has been changing up to now. The main drivers for power generation and combined power and heat generation from biomass are the German Renewable Energy Sources Act (EEG, 2023), the Biomass Regulation Act (BiomasseV, 2001) and the Combined Heat and Power Act (KWKG, 2015). Electricity generation from solid biomass increased significantly between 2002 and 2006 (FNR, 2022a). After the EEG was amended in July 2004, together with a bonus for energy crops (“NaWaRo bonus”), there was a boom in new installations of biogas plants. After ongoing debates about the excessive space requirements for bioenergy, possible negative effects on food security and indirect land use change, the funding instruments for bioenergy in Germany and Europe shifted and all incentives were massively reduced. From 2017 onwards, new flexibility requirements in combination with a restriction of the expansion of biogas plants with 150 MW (2017–2019) and 200 MW (2020–2022) installed capacity, electricity generation from biomass stagnated.
The use of biomass in the heating sector in Germany plays a more important role than in the electricity sector. In 2022, heat generation from biomass in Germany was around 170 TWh, more than 3 times as high as the amount of electricity generated (around 50 TWh) (UBA, 2023). Specially solid fuels (wood) are responsible for most of the heat produced in small combustion systems such as log wood and pellet stoves (FNR, 2022a). In contrast to the electricity sector, the heating sector developed primarily through subsidy programs such as the Market Incentive Program (MAP), whereas the nationwide Renewable Energy Heat Act (EEWärmeG, 2009) has not led to any significant increase in the renewables share in the heating market to date.
Regarding the current energy crisis, the German Government has once again significantly increased the expansion targets for renewable energies (BMWK, 2022). Within the recently amended Renewable Energy Sources Act, the share of renewables rises from 65% to 80% by 2030 (EEG, 2023). However, the increase is mainly to be achieved through a higher expansion of wind and solar energy. Concerning bioenergy, the government’s forecast is only slightly more optimistic for biomethane but sees no further expansion for other bioenergy uses (BMWK, 2022). The reasons for this are, on the one hand, the high land consumption for biomass energy compared to solar and wind energy as well as the increasing scarcity of land due to climate change and prioritized uses (see above, i.e., Hirschl et al. (2023)). The indirect effects of land use (ILUC), which have been known since the controversial debate on biofuels, must also be taken into account (Plevin and Kammen, 2013; IPCC, 2019) as well as related social-ecological conflicts (Backhouse et al., 2021). In the end, scepticism about the growing use of bioenergy has proven to be stable, even during the crisis, in view of the increasing problems of food security and the need for the substitution of materials based on fossil fuels. Against this background, scenarios modeled in this study refer to the current political framework and are based on relevant (national) studies that regard the limitations regarding the development of bioenergy mentioned above.
3.6 Bioenergy scenarios
The calculation of the scenarios was mainly based on the status quo of energy plants in Lusatia (DENA, 2023a; DBFZ, 2023; MWAE, 2023; SAENA, 2023) and on bioenergy forecasts for Germany (Purr et al., 2019; Prognos et al., 2021; DENA, 2023b), which was proportionally transferred to the case region. The results obtained for the region were checked for plausibility using the determined ranges of regional potential (see Table 3), although direct comparability of the data is only possible to a limited extent.
The Current Policies scenario is based on the “RESCUE” study made by the Federal Environment Agency (Purr et al., 2019), which contains an intersectoral long-term scenario taking into account differentiated bioenergy shares. It aims achieving greenhouse gas neutrality by 2050, following the first German Federal Climate Change Act (Federal Climate Change Act, 2019). The study contains a long-term declining approach to the use of biomass for energy, which reflects the environmental policy priorities in the biomass sector. According to the “RESCUE” study, the installed capacity of wood-based CHP and small-scale boilers will be reduced to approximately 90% due to a massive shift to material uses. This means—proportionally transferred to the region considered here–a projected total installed capacity of around 5 MWel for CHP (Table 4). The quantities of waste wood required for this are covered by the lower value (1,700 TJ) of the estimated waste wood potential ranges (see Table 3). Furthermore, the installed capacity of biogas plants is reduced to 55% and biomethane to 33%, which corresponds to around 40 MWel and 3,900 Nm³/h respectively (Table 4). The potential for energy crops and, in particular, manure estimated in Section 3.4 is therefore not fully utilised (see Table 3). If energy crops are required mainly for material uses in the future, biogas and biomethane production would have to be provided almost entirely by manure-based plants in this scenario.
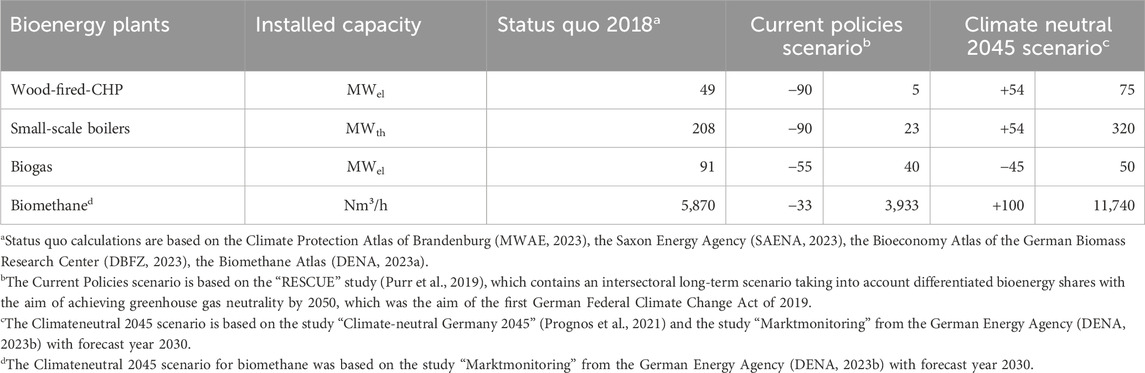
TABLE 4. Bioenergy scenarios in Lusatia (own calculations based on the sources indicated on the footnotes).
The calculation of the Climate Neutral 2045 scenario for Lusatia is mainly based on the study “Climate-neutral Germany 2045”(Prognos et al., 2021), which follows the climate-neutrality target by 2045 in all sectors and breaks down the use of bioenergy in a comparative manner. In this study, bioenergy takes on a slightly more progressive role, as overall a slight increase is seen by 2045. This is largely due to the use of wood for energy (approximately 54% increase). In this scenario, a higher proportion of the wood potential identified above (see Table 3) is utilised. However, as it can be assumed that significant quantities of wood will also be required for material uses, the study by Prognos et al. (2021) also assumes that a significant proportion of SRC will be produced. The SRC production (as well as agroforestry systems) could take place on the vacant maize cultivation areas. The expansion of CHP production can help to stabilize the electricity system, specially if it remains more cost-effective than hydrogen-based alternatives in the longer term.
The expansion of wood-based small combustion plants follows the thesis of a contribution to individual energy supply security, which has become increasingly important since the Russian-Ukraine war. Biogas production - similar to the current policies scenario—falls to 45% (Table 4), in particular due to the phasing out of energy crops. In this scenario, on the one hand, biogas is required in a centralized manner to cover the industrial demand for high-temperature heat, while on the other hand, it is used in a decentralized way for the heat supply of agriculture and for small local heating networks. A further expansion potential is reserved to manure plants, which should be tapped in the medium term up to 2030. This is due to the fact that currently only about 1/3 of manure in Germany is used for energy (Majer et al., 2019). The calculation of biomethane scenario was based on the study “Market Monitoring” from the German Energy Agency (DENA, 2023b), which foresees a doubling increase of biomethane installed capacity in 2030 (Table 4). The assumption of an increase in biomethane production is strengthened by the current natural gas supply crisis in Germany, which was caused by the Russian-Ukraine war. Hence alternatives for gas-based supply must be developed. Combined with the simultaneous limitation of energy crop cultivation, which is politically prescribed and thus included in most studies (Purr et al., 2019; Prognos et al., 2021; DENA, 2023b), a shift from biogas to biomethane production seems plausible.
4 Discussion
The approach used within this paper presents a generic method to derive differentiated biomass and bioenergy data and scenarios for any region. As region-specific data and information are often lacking, valid supra-regional studies must be used for regional projections. However, their developments must be adapted to the regional situation using plausible indicators and assumptions. In addition, the actual and expected (bio)energy and climate policy developments must be considered, as well as the expected effects of climate change on biomass production.
In accordance with the current political framework (of the year 2021), a significant decline in the energetic use of biomass is projected for Lusatia. By contrast, the decline of biogas plants in the Climate Neutral 2045 scenario is smaller, and there is even a significant increase in the biomethane feed-in segment. Two main usage paths for biogas remain foreseeably relevant: the production of electricity and heat as part of the German renewable energy law and the production of fuels via GHG quota regulations, specifically, Section 37a-d of the Federal Emission Control Act (BImSchG, 2023) and subsequent ordinances. Fuel distributors blend fossil fuels with renewable fuels to lower the GHG balance (Naumann et al., 2021). Both paths of use may continue to represent sustainable business models for farmers in the future, provided that the focus in the fuel sector is on waste and residual materials (e.g., manure). More biogas production from manure would reduce emissions from the storage and spreading of animal excrement (methane and nitrous oxide) and from the use of fossil fuels, while the digestate would in turn be available as natural fertilizer.
The extent to which agricultural areas can be used for bioenergy in Lusatia in the future will depend on how the primary uses of these areas develop. Regional aspects like geographical location, land use structure, the specific yield information, population size and the intensity of competing applications influence the biomass potential (Lozano-García et al., 2020; Stolarski et al., 2020; Xu et al., 2022). In addition to the necessary proportions for soil improvement (humus balance) and animal husbandry, straw will increasingly be used for material applications in the future. As a result, the proportion for bioenergy uses is continuously decreasing, which is why most studies (see above) do not assume any further potential for electricity and heat production. The quantities are also unlikely to be sufficient for biorefineries, for example, for the production of biofuels.
Some improvements (e.g., the addition of integrated biomass systems and forest management) may result in increased bioenergy production, while others (e.g., reserving more land for nature protection to reflect higher ambitions concerning conservation) will very likely decrease the amount of bioenergy. In particular, a reduction in meat consumption and the associated decrease in area requirements for grazing land can bring opportunities for other land use forms (Mazac et al., 2022; Parzianello and Carvalho, 2023; Schön and Böhringer, 2023). As an example, the use of agri-photovoltaic, which combines the development of the same land area for solar plants and agriculture, could overcome the shortage of space and land use competition (Chatzipanagi et al., 2023; Klabunde and Engel, 2023).
Strategic considerations in bioeconomy policies emphasize the great potential of residues. The results show that regional potentials, specially of biomass residues, is often too small (Baasch et al., 2022). In practice, many of these leftovers are already being used. The question often arises not whether biomass residues can be used, but rather how they should be used. Due to the seasonal availability of biomass, a combined use of different residues is necessary so that there is enough material available. Enabling energetic use could only be possible by creating collection and storage areas. This affords inter-regional cooperation to clarify not only availability, but also land use competition issues.
The central shifts in bioenergy policy include increased sustainability demands, the primacy of food security, the requirement for a coupled, cascading use of material and energy use and, as a result, the focus on the primary use of residues and waste materials for bioenergy use. Which role biomass residues will play in the future depends heavily on the development of energy prices, technologies, economic production and value chains as well as the political framework (Choi et al., 2019; Laibach et al., 2019). State governments in Brandenburg and Saxony have established independent strategies and programs to manage structural change in Lusatia. Examples of successful initiatives are various clusters in Saxony and Brandenburg (BMBF, 2021). However, administrative and jurisdictional boundaries pose a particular challenge when it comes to developing a spatial planning strategy that goes beyond existing formal state-specific (regional) plans.
The growing availability of arable land, as a consequence of the decline of coal mining and the decline in energy crop cultivation (e.g., maize), provides ample opportunities for performing successful feedstock production on unmanaged areas in several regions (Pietrzykowski, 2019; Nurcholis et al., 2021; Paredes-Sánchez et al., 2022). Hybrid land uses that are as coupled as possible must be established, including those that enable biomass production in addition to a GHG sink function. Compared to energy crops, SRC have advantages such as higher carbon sequestration in the soil, lower fertilizer requirements, simpler and more flexible storage and transport of the solid biomass (Falkenberg et al., 2021) and they contribute to the expansion of domestic supply (Prognos et al., 2021). In addition, for the decarbonization of the economy as a whole, there is a need of biogenic material uses on a larger scale. The production of fuel in biorefineries and the production of biomethane, which can be used flexibly in all sectors as a substitute for natural gas, are promising technologies (Fava et al., 2015; Duan et al., 2022; Saratale et al., 2022; Carvalho et al., 2023; Sulewski et al., 2023; Thrän et al., 2023). However, in this case study, the expansion of biomethane plants is foreseen (see the Climate Neutral 2045 scenario above) due to the widespread biomethane technology and its comparatively low demand for raw materials, whose regional availability will remain limited.
The results presenting a possible bioenergy development in a specific region by estimating biomass potentials are plausible. However, it must be emphasized that a complete investigation of all biomass applications has not been carried out in this analysis, as material applications are becoming increasingly important and will develop strong competition with energy use (Rupp et al., 2020; González-Castaño et al., 2021; Wydra et al., 2021; Zander et al., 2022). Ideally, scenarios for estimating the future development of bioenergy should therefore also take into account the material requirements and a matching of regional biomass with all possible energy and material applications. Due to the current lack of momentum and the small number of players and plants in the new fields of the bioeconomy, there is little valid data available for material applications. In addition, the regional system boundary is also a simplification of reality, as biomass can be exchanged across regions (to a certain extent), which is why there is no 1:1 relationship between regionally available biomass and processing technologies (Szarka et al., 2021; Richter et al., 2022). However, there are also important ecological reasons in favour of using as much of the regionally available residual and waste materials as possible locally in the future.
Ultimately, both material and energy use will continue to be strongly determined by the political framework. The approach presented here is a secondary data-based analysis, which combines differentiated regional baseline and potential data with current supra-regional studies, to be a valid, simplified method for estimating regional bioenergy developments that can be transferred to any other region. However, it is also clear that the collection of concrete regional primary data is always preferable if possible.
5 Conclusion
Regional biomass and bioenergy use can become an important economic pillar for any region, specially for structurally weak and former coal regions. However, the production of biomass for energy purposes is subject to restrictions and increasing technological and land competition, which is why the development of bioenergy is currently subject to substantial change. The exact development varies for many regions and depends significantly on the available biomass, climatic conditions, economic structures and the political framework, which has changed considerably in recent years. Therefore, regional analyses need to be updated in many cases, as older studies are based on assumptions that are no longer valid. Against the backdrop of the manifold possible uses of biomass in different sectors as well as the generally inadequate regional data situation, there is usually no good regional database for biomass and bioenergy available, and the effort required for a primary data-based analysis is often not affordable. For this reason, this analysis presents a simplified, secondary data-based method for creating regional bioenergy scenarios.
The case study region is Lusatia (Lausitz), the second largest coal-mining region in Germany. By intersecting regional data and developments with supraregional data and scenarios, two regional bioenergy scenarios were developed for Lusatia: one that updates the current policy framework and one that is mainly derived from national studies that map the goal of climate neutrality by 2045. In the Climate Neutral 2045 scenario, climatic projections and technological developments were considered in addition to the political priorities. For Lusatia, the picture that emerges is that, on the one hand, there is a shift from the direct use of biogas to increased processing and feeding in as biomethane, as this can be used more widely in different sectors. On the other hand, it is assumed that decentralized use in heating boilers will stabilize, and possibly even expand slightly, which takes into account the increased sensitivity to energy supply security issues. As a result of the increased demand for solid biomass, the energy crop areas should decrease. These areas could be used to meet the demand for solid biomass through agroforestry systems, hedge or short rotation plantations, but also for other renewable technologies like (agri-)photovoltaics.
The demand for biomass is shifting due to the increasingly profitable energy generation from wind and solar technologies as well as the future increase in demand for material uses. In general, the use of biomass for energy can only stabilize or even increase if the shortage of land is overcome, available biomass fractions are tapped in a targeted manner and conversion efficiency is increased. The amount of agricultural land available can be reduced through multiple uses and a reduction in meat production, and cascades of material applications should be established, with energy use at the end.
The methodological approach presented here represents a simplified option for determining regional development options for bioenergy on the basis of secondary data, if and as long as there is no valid regional data on the future development of the regional bioeconomy.
Data availability statement
The raw data supporting the conclusion of this article will be made available by the authors, without undue reservation.
Author contributions
BL: Conceptualization, Data curation, Formal Analysis, Investigation, Methodology, Project administration, Software, Validation, Visualization, Writing–original draft, Writing–review and editing. BH: Data curation, Formal Analysis, Funding acquisition, Project administration, Resources, Software, Supervision, Validation, Visualization, Writing–review and editing.
Funding
The author(s) declare financial support was received for the research, authorship, and/or publication of this article. This article and the case study within were developed as part of the project “Mobilising endogenous potentials for structural change—decarbonisation in a lignite mining region—the case of Lusatia” (DecarbLau), funded between 2019 and 2022 by the Federal Ministry of Education and Research (BMBF) within the framework of the funding priority Economics of Climate Change (phase II).
Acknowledgments
Many thanks to the very helpful and constructive comments and advice from the reviewers. Special thanks also to our colleague Annika Bode for her help throughout the entire process.
Conflict of interest
The authors declare that the research was conducted in the absence of any commercial or financial relationships that could be construed as a potential conflict of interest.
Publisher’s note
All claims expressed in this article are solely those of the authors and do not necessarily represent those of their affiliated organizations, or those of the publisher, the editors and the reviewers. Any product that may be evaluated in this article, or claim that may be made by its manufacturer, is not guaranteed or endorsed by the publisher.
References
AEE (2013a). Potenzialatlas. Bioenergie in den Bundesländern. Teilkapitel Brandenburg (Potenzialatlas). Agentur für Erneuerbare Energien. Available at: https://www.unendlich-viel-energie.de/media/file/237.AEE_Potenzialatlas_Bioenergie_Brandenburg_jan13.pdf.
AEE (2013b). Potenzialatlas. Bioenergie in den Bundesländern. Teilkapitel Sachsen. Agentur für Erneuerbare Energien. Available at: https://www.unendlich-viel-energie.de/media/file/249.AEE_Potenzialatlas_Bioenergie_Sachsen_jan13.pdf.
Alves, D., Kanellopoulos, K., Medarac, H., Kapetaki, Z., Miranda Barbosa, E., Shortall, R., et al. (2018). EU coal regions: opportunities and challenges ahead (EUR 29292 EN). Publications Office of the European Union. Available at: https://publications.jrc.ec.europa.eu/repository/handle/JRC112593.
Amt für Statistik Berlin-Brandenburg (2018). Statistisches jahrbuch 2018. Available at: https://www.statistik-berlin-brandenburg.de/produkte/Jahrbuch/jb2018/JB_2018_BB.pdf.
Amt für Statistik Berlin-Brandenburg (2019). Ernteberichterstattung über Feldfrüchte und Grünland im Land Brandenburg 2018 C II 2 – j/18. statistik Berlin Brandenburg Available at: https://www.statistik-berlin-brandenburg.de/publikationen/stat_berichte/2019/SB_C02-02-00_2018j01_BB.pdf.
Amt für Statistik Berlin-Brandenburg (2020). Statistische jahrbuch 2020. Available at: https://download.statistik-berlin-brandenburg.de/c0aa18dc1cbbcf4c/e6ed45c747d0/AfS_Jahrbuch_2020_BB.pdf.
Anacleto, T. M., Oliveira, H. R., Diniz, V. L., de Oliveira, V. P., Abreu, F., and Enrich-Prast, A. (2022). Boosting manure biogas production with the application of pretreatments: a meta-analysis. J. Clean. Prod. 362, 132292. doi:10.1016/j.jclepro.2022.132292
Anca-Couce, A., Hochenauer, C., and Scharler, R. (2021). Bioenergy technologies, uses, market and future trends with Austria as a case study. Renew. Sustain. Energy Rev. 135, 110237. doi:10.1016/j.rser.2020.110237
Anukam, A., and Berghel, J. (2020). Biomass pretreatment and characterization: a review. Biotechnological Applications of Biomass. (1. Aufl.). IntechOpen. doi:10.5772/intechopen.93607
Baasch, S., Bauriedl, S., Lenz, C., and Wiechers, H. (2022). Bioenergie aus Rest-und Abfallstoffen – chancen für die regionale Wärmewende Klimaresiliente Stadt [Projekt, Klimaresiliente Stadt-Umland Kooperation. Regionale Innovationen energetischer Biomassenutzung und Governance (KlimaInnoGovernance)] Available at: https://klimainnogovernance.de/wp-content/uploads/2021/11/KlimaInnoGovernance_Ergebnisse_web.pdf.
Backhouse, M., Lehmann, R., Lorenzen, K., Lühmann, M., Puder, J., Rodríguez, F., et al. (2021). Bioeconomy and global inequalities: socio-ecological perspectives on biomass sourcing and production. Cham: Springer Nature.
Bao, K., Padsala, R., Coors, V., Thrän, D., and Schröter, B. (2020). A method for assessing regional bioenergy potentials based on GIS data and a dynamic yield simulation model. Energies 13 (24), 6488. doi:10.3390/en13246488
Bentsen, N. S., Nilsson, D., and Larsen, S. (2018). Agricultural residues for energy—a case study on the influence of resource availability, economy and policy on the use of straw for energy in Denmark and Sweden. Biomass Bioenergy 108, 278–288. doi:10.1016/j.biombioe.2017.11.015
Berger, W., Lademann, S., Schnellenbach, J., Weidner, S., and Zundel, S. (2019). Standortpotentiale Lausitz—studie im Auftrag der Zukunftswerkstatt Lausitz. Available at: https://zw-lausitz.de/fileadmin/user_upload/01-content/03-zukunftswerkstatt/02-downloads/studie-standortpotenziale-lausitz.pdf.
BImSchG (2023). Gesetz zum Schutz vor schädlichen Umwelteinwirkungen durch. Available at: https://www.gesetze-im-internet.de/bimschg/BImSchG.pdf.
BiomasseV (2001). BGBl. I S. 123 Verordnung über die Erzeugung von Strom aus Biomasse. Available at: https://www.gesetze-im-internet.de/biomassev/BiomasseV.pdf.
BMBF (2021). Nationale Bioökonomiestrategie: mit der Nationalen Bioökonomiestrategie legt die Bundesregierung die Leitlinien und Ziele ihrer Bioökonomie-Politik fest und benennt Maßnahmen für deren Umsetzung. bioökonomie.de Available at: https://biooekonomie.de/themen/politikstrategie-deutschland.
BMEL (2020). “Land-, Forst-und Ernährungswirtschaft mit Fischerei und Wein-und Gartenbau (S. 44) [Daten und Fakten],” in Bundesministerium für Ernährung und Landwirtschaft. Available at: https://www.bmel.de/SharedDocs/Downloads/DE/Broschueren/daten-fakten-2022.pdf?__blob=publicationFile&v=5.
BMWK (2022). “Germany’s current climate action status,” in Bundesministerium für Wirtschaft und Klimaschutz. Available at: https://www.bmwk.de/Redaktion/EN/Downloads/E/germany-s-current-climate-action-status.pdf?__blob=publicationFile&v=11.
Braghiroli, F. L., and Passarini, L. (2020). Valorization of biomass residues from forest operations and wood manufacturing presents a wide range of sustainable and innovative possibilities. Curr. For. Rep. 6 (2), 172–183. doi:10.1007/s40725-020-00112-9
Brosowski, A., Adler, P., Erdmann, G., Stinner, W., Thrän, D., Mantau, U., et al. (2015). Biomassepotenziale von Rest-und Abfallstoffen Status quo in Deutschland. Fachagentur Nachwachsende Rohstoffe e. V. 36 Available at: https://mediathek.fnr.de/broschuren/bioenergie/band-36-biomassepotenziale-von-rest-und-abfallstoffen-status-quo-in-deutschland.html.
Brosowski, A., Thrän, D., Mantau, U., Mahro, M., Erdmann, G., Adler, P., et al. (2016). A review of biomass potential and current utilisation – status quo for 93 biogenic wastes and residues in Germany. Biomass Bioenergy 95, 257–272. doi:10.1016/j.biombioe.2016.10.017
Burg, V., Bowman, G., Erni, M., Lemm, R., and Thees, O. (2018). Analyzing the potential of domestic biomass resources for the energy transition in Switzerland. Biomass Bioenergy 111, 60–69. doi:10.1016/j.biombioe.2018.02.007
Cai, A., Xu, M., Wang, B., Zhang, W., Liang, G., Hou, E., et al. (2019). Manure acts as a better fertilizer for increasing crop yields than synthetic fertilizer does by improving soil fertility. Soil Tillage Res. 189, 168–175. doi:10.1016/j.still.2018.12.022
Cała, M., Szewczyk-Świątek, A., and Ostręga, A. (2021). Challenges of coal mining regions and municipalities in the face of energy transition. Energies 14 (20), 6674. doi:10.3390/en14206674
Carvalho, F. S., Reis, L. C., Lacava, P. T., Araújo, F. H., and Carvalho, J. A. (2023). Substitution of natural gas by biomethane: operational aspects in industrial equipment. Energies 16 (2), 839. doi:10.3390/en16020839
Cha, J. M. (2020). A just transition for whom? Politics, contestation, and social identity in the disruption of coal in the Powder River Basin. Energy Res. Soc. Sci. 69, 101657. doi:10.1016/j.erss.2020.101657
Chatzipanagi, A., Taylor, N., and Jaeger-Waldau, A. (2023). Overview of the potential and challenges for agri-photovoltaics in the European union. (JRC132879). Publications Office of the European Union. Available at: https://www.cde.ual.es/wp-content/uploads/2023/05/overview-of-the-potential-and-challenges-for-agri-photovoltaics-KJNA31482ENN.pdf.
Choi, H. S., Grethe, H., Entemann, S. K., Wiesmeth, M., Blesl, M., and Wagner, M. (2019). Potential trade-offs of employing perennial biomass crops for the bioeconomy in the EU by 2050: impacts on agricultural markets in the EU and the world. GCB Bioenergy 11 (3), 483–504. doi:10.1111/gcbb.12596
Cornelissen, S., Koper, M., and Deng, Y. (2012). The role of bioenergy in a fully sustainable global energy system. Biomass Bioenergy 41, 21–33. doi:10.1016/j.biombioe.2011.12.049
Creutzig, F., Ravindranath, N. H., Berndes, G., Bolwig, S., Bright, R., Cherubini, F., et al. (2015). Bioenergy and climate change mitigation: an assessment. Gcb Bioenergy 7 (5), 916–944. doi:10.1111/gcbb.12205
Daniel-Gromke, J., Rensberg, N., Denysenko, V., Trommler, M., Reinholz, T., Völler, K., et al. (2017). Anlagenbestand Biogas und Biomethan – biogaserzeugung und -nutzung in Deutschland. (Broschüre Report 30; Schriftenreihe, Biogas, Biomethan, Anlagenbestand, Monitoring). DBFZ. Available at: https://www.dbfz.de/pressemediathek/publikationsreihen-des-dbfz/dbfz-reports/dbfz-report-nr-30/.
DBFZ (2019). Leitfaden flexibilisierung der Strombereitstellung von Biogasanlagen (LF flex). Available at: https://www.dbfz.de/fileadmin/user_upload/Referenzen/Studien/20191108_LeitfadenFlex_Abschlussbericht.pdf.
DBFZ (2023). Bioökonomie Atlas für das Mitteldeutschland und Lausitzer Revier [Map]. DBFZ. Available at: https://www.dbfz.de/biooekonomieatlas/start.
DENA (2023a). Einspeiseatlas. Available at: https://www.biogaspartner.de/einspeiseatlas/.
DENA (2023b). Marktmonitoring Bioenergie. Available at: https://www.dena.de/fileadmin/dena/Publikationen/PDFs/2023/ANALYSE_Marktmonitoring_Bioenergie_2023.pdf.
Dong, L., Liu, H., and Riffat, S. (2009). Development of small-scale and micro-scale biomass-fuelled CHP systems – a literature review. Appl. Therm. Eng. 29 (11), 2119–2126. doi:10.1016/j.applthermaleng.2008.12.004
Duan, Y., Tarafdar, A., Kumar, V., Ganeshan, P., Rajendran, K., Shekhar Giri, B., et al. (2022). Sustainable biorefinery approaches towards circular economy for conversion of biowaste to value added materials and future perspectives. Fuel 325, 124846. doi:10.1016/j.fuel.2022.124846
EEA (2017). EEA Report: climate change, impacts and vulnerability in Europe 2016. An indicator-based report. Available at: https://www.eea.europa.eu/publications/climate-change-impacts-and-vulnerability-2016.
EEG (2023). EEG 2023-Gesetz für den Ausbau erneuerbarer Energien. Available at: https://www.gesetze-im-internet.de/eeg_2014/EEG_2023.pdf.
EEWärmeG (2009). Erneuerbare-energien. Available at: https://www.erneuerbare-energien.de/EE/Navigation/DE/Recht-Politik/Das_EEWaermeG/das_eewaermeg.html.
Ericsson, K., and Nilsson, L. J. (2006). Assessment of the potential biomass supply in Europe using a resource-focused approach. Biomass Bioenergy 30, 1–15. doi:10.1016/j.biombioe.2005.09.001
European Commission (2012). Innovating for sustainable growth. A bioeconomy for Europe (Directorate-General for research and innovation directorate biotechnologies, agriculture, food). 10.2777/6462 Available at: https://op.europa.eu/en/publication-detail/-/publication/1f0d8515-8dc0-4435-ba53-9570e47dbd51/language-en/format-PDF/source-281389251.
European Commission (2019). European commission, COM (2019) 640 final. Available at: https://eur-lex.europa.eu/legal-content/EN/TXT/?uri=COM:2019:640:FIN.
European Commission (2020). COM(2020) 22 final, 2020/0006(COD). Available at: https://eur-lex.europa.eu/legal-content/EN/TXT/?uri=CELEX%3A52020PC0022.
Falkenberg, H., Hobohm, J., Lübbers, S., Malik, F., Mellahn, S., and Srikandam, R. (2021). Gutachten zur Energiestrategie Brandenburg 2040—aktualisierung und Weiterentwicklung der Energiestrategie 2030 des Landes Brandenburg [Endbericht]. Im Auftrag des Ministeriums für Wirtschaft, Arbeit und Energie des Landes Brandenburg. Available at: https://mwae.brandenburg.de/media/bb1.a.3814.de/Prognos-Gutachten_Fortschreibung_Energiestratgie%20Bbg_2040.pdf.
Fava, F., Totaro, G., Diels, L., Reis, M., Duarte, J., Carioca, O. B., et al. (2015). Biowaste biorefinery in Europe: opportunities and research & development needs. New Biotechnol. 32 (1), 100–108. doi:10.1016/j.nbt.2013.11.003
Federal Climate Change Act (2019). Federal climate change Act, BGBl. I S. 2513. Available at: https://www.gesetze-im-internet.de/englisch_ksg/englisch_ksg.pdf.
Fehrenbach, H., Köppen, S., Kauertz, B., Detzel, A., and Wellenreuther, F. (2017). Biomassekaskaden. Mehr Ressourceneffizienz durch Kaskadennutzung von Biomasse – von der Theorie zur Praxis. [Abschlussbericht]. Im Auftrag des Umweltbundesamtes Available at: https://www.umweltbundesamt.de/sites/default/files/medien/1410/publikationen/2017-06-13_texte_53-2017_biokaskaden_abschlussbericht.pdf.
FNR (2018). Rohstoffmonitoring Holz [Broschüre]. Fachagentur Nachwachsende Rohstoffe e. V. Available at: https://www.fnr.de/fileadmin/allgemein/pdf/broschueren/Handout_Rohstoffmonitoring_Holz_Web_neu.pdf.
FNR (2019). Basisdaten Bioenergie Deutschland 2019. Fachagentur Nachwachsende Rohstoffe e. V. Available at: https://www.fnr.de/fileadmin/allgemein/pdf/broschueren/basisdaten_bioenergie_2019_web.pdf.
FNR (2022a). Basisdaten Bioenergie Deutschland. Available at: https://www.fnr.de/fileadmin/Projekte/2022/Mediathek/broschuere_basisdaten_bioenergie_2022_06_web.pdf.
FNR (2022b). Energiepflanzen-getreide. Available at: https://pflanzen.fnr.de/energiepflanzen/pflanzen/getreide/.
FNR (2022c). Faustzahlen biogas. Available at: https://biogas.fnr.de/daten-und-fakten/faustzahlen.
Fouquet, R. (2010). The slow search for solutions: lessons from historical energy transitions by sector and service. Energy Policy 38 (11), 6586–6596. doi:10.1016/j.enpol.2010.06.029
Fritsche, U., Brunori, G., Chiaramonti, D., Galanakis, C., Hellweg, S., Matthews, R., et al. (2020). Future transitions for the bioeconomy towards sustainable development and a climate-neutral economy (knowledge synthesis final report JRC121212). Publications Office of the European Union. Available at: https://publications.jrc.ec.europa.eu/repository/bitstream/JRC121212/fritsche_et_al_%282020%29_d2_synthesis_report_final_1.pdf.
Garcia, N. H., Mattioli, A., Gil, A., Frison, N., Battista, F., and Bolzonella, D. (2019). Evaluation of the methane potential of different agricultural and food processing substrates for improved biogas production in rural areas. Renew. Sustain. Energy Rev. 112, 1–10. doi:10.1016/j.rser.2019.05.040
Gerbelová, H., Spisto, A., and Giaccaria, S. (2021). Regional energy transition: an analytical approach applied to the Slovakian coal region. Energies 14 (1), 110. doi:10.3390/en14010110
González-Castaño, M., Kour, M. H., González-Arias, J., Baena-Moreno, F. M., and Arellano-Garcia, H. (2021). Promoting bioeconomy routes: from food waste to green biomethane. A profitability analysis based on a real case study in eastern Germany. J. Environ. Manag. 300, 113788. doi:10.1016/j.jenvman.2021.113788
Guo, M., Song, W., and Buhain, J. (2015). Bioenergy and biofuels: history, status, and perspective. Renew. Sustain. energy Rev. 42, 712–725. doi:10.1016/j.rser.2014.10.013
Haak, F. (2015). Effizientes Erschließen von Landschaftspflegematerial in Bioenergieregionen – das Beispiel Gehölze und Heckenmanagement. DBFZ. Available at: https://www.dbfz.de/fileadmin/user_upload/Referenzen/Berichte/LPM_Bioenergie_Regionen.pdf.
Hamelin, L., Borzęcka, M., Kozak, M., and Pudełko, R. (2019). A spatial approach to bioeconomy: quantifying the residual biomass potential in the EU-27. Renew. Sustain. Energy Rev. 100, 127–142. doi:10.1016/j.rser.2018.10.017
Hanssen, S. V., Daioglou, V., Steinmann, Z. J. N., Frank, S., Popp, A., Brunelle, T., et al. (2020). Biomass residues as twenty-first century bioenergy feedstock—a comparison of eight integrated assessment models. Clim. Change 163 (3), 1569–1586. doi:10.1007/s10584-019-02539-x
Harun, S. N., Hanafiah, M. M., and Noor, N. M. (2022). Rice straw utilisation for bioenergy production: a brief overview. Energies 15 (15), 5542. doi:10.3390/en15155542
Hirschl, B., Heinbach, K., Salecki, S., Bode, A., Leuner, B., Bergmann, J., et al. (2022). Energiewende in der Lausitz – regionalökonomische effekte relevanter technologien. Schriftenr. Des. IÖW 223/22, 268.
Hirschl, B., Torliene, L., Schwarz, U., Dunkelberg, E., Weiß, J., Lenk, C., et al. (2023). “Gutachten für den Klimaplan Brandenburg—erarbeitung einer Klimaschutzstrategie für das Land Brandenburg,” in Endbericht. Studie im Auftrag des Landes Brandenburg, vertreten durch das Ministerium für Landwirtschaft, Umwelt und Klimaschutz. Available at: https://mluk.brandenburg.de/sixcms/media.php/9/ZwBericht-Gutachten-KlimaplanBB_finale%20Fassung.pdf.
IEA (2022). Renewables 2022. Analysis and forecast to 2027. Available at: https://iea.blob.core.windows.net/assets/ada7af90-e280-46c4-a577-df2e4fb44254/Renewables2022.pdf.
IEA (2023). Tracking bioenergy. Available at: https://www.iea.org/energy-system/renewables/bioenergy.
IPCC (2018). Global warming of 1.5°C. Available at: https://www.ipcc.ch/site/assets/uploads/sites/2/2022/06/SPM_version_report_LR.pdf.
IPCC (2019). IPCC special report on climate change, desertification, land degradation, sustainable land management, food security, and greenhouse gas fluxes in terrestrial ecosystems [summary for policymakers, approved draft 07]. Available at: https://www.ipcc.ch/site/assets/uploads/2019/08/Edited-SPM_Approved_Microsite_FINAL.pdf.
IPCC (2022). IPCC sixth assessment report: climate change 2022: impacts, adaptation and vulnerability. Available at: https://www.ipcc.ch/report/ar6/wg2/downloads/report/IPCC_AR6_WGII_Chapter05.pdf.
Jarre, M., Petit-Boix, A., Priefer, C., Meyer, R., and Leipold, S. (2020). Transforming the bio-based sector towards a circular economy-What can we learn from wood cascading? For. Policy Econ. 110, 101872. doi:10.1016/j.forpol.2019.01.017
Junginger, H. M., Mai-Moulin, T., Daioglou, V., Fritsche, U., Guisson, R., Hennig, C., et al. (2019). The future of biomass and bioenergy deployment and trade: a synthesis of 15 years IEA Bioenergy Task 40 on sustainable bioenergy trade. Biofuels, Bioprod. Bioref. 13, 247–266. doi:10.1002/bbb.1993
Kaltschmitt, M., Hartmann, H., and Hofbauer, H. (2009). Energie aus Biomasse—grundlagen, Techniken und Verfahren (2. Aufl.). Cham: Springer Berlin Heidelberg.
KIWUH (2019). Wald und Holz in Deutschland [Broschüre]. Fachagentur Nachwachsende Rohstoffe e. V. Available at: https://www.fnr.de/fileadmin/kiwuh/broschueren/Brosch_Wald_Holz_KIWUH_Auflage2_web.pdf.
Klabunde, F., and Engel, B. (2023). Rooftop photovoltaic systems in German agriculture – an analysis of the status quo and potential for the future. ETG Congr. 2023, 1–6.
Klepper, G., and Thrän, D., (2019). Biomasse im Spannungsfeld zwischen Energie-und Klimapolitik. Potenziale – technologien – Zielkonflikte. Schriftenreihe Energiesysteme der Zukunft. Available at: https://www.acatech.de/publikation/biomasse-im-spannungsfeld-zwischen-energie-und-klimapolitik-potenziale-technologien-zielkonflikte/.
Klüter, H. (2014). Die Landwirtschaft in Sachsen im Vergleich mit anderen Bundesländern. [Endbericht im Auftrag der Fraktion BÜNDNIS 90/DIE GRÜNEN] Available at: https://www.gruene-fraktion-sachsen.de/fileadmin/user_upload/Studien/Landwirtschaftsstudie_web_2015-01.pdf.
Koch, K., Höfner, P., and Gaderer, M. (2020). Techno-economic system comparison of a wood gas and a natural gas CHP plant in flexible district heating with a dynamic simulation model. Energy 202, 117710. doi:10.1016/j.energy.2020.117710
KWKG (2015). Gesetz für die Erhaltung, die Modernisierung und den Ausbau der. Available at: https://www.gesetze-im-internet.de/kwkg_2016/KWKG_2023.pdf.
Laibach, N., Börner, J., and Bröring, S. (2019). Exploring the future of the bioeconomy: an expert-based scoping study examining key enabling technology fields with potential to foster the transition toward a bio-based economy. Technol. Soc. 58, 101118. doi:10.1016/j.techsoc.2019.03.001
Leemans, R., van Amstel, A., Battjes, C., Kreileman, E., and Toet, S. (1996). The land cover and carbon cycle consequences of large-scale utilizations of biomass as an energy source. Integr. Scenarios Glob. Chage Results IMAGE 2 Model 6 (4), 335–357. doi:10.1016/S0959-3780(96)00028-3
Lozano-García, D. F., Santibañez-Aguilar, J. E., Lozano, F. J., and Flores-Tlacuahuac, A. (2020). GIS-based modeling of residual biomass availability for energy and production in Mexico. Renew. Sustain. Energy Rev. 120, 109610. doi:10.1016/j.rser.2019.109610
Lundmark, R., Athanassiadis, D., and Wetterlund, E. (2015). Supply assessment of forest biomass. A bottom-up approach for Sweden. Biomass Bioenergy 75, 213–226. doi:10.1016/j.biombioe.2015.02.022
Majer, S., Kornatz, P., Daniel-Gromke, J., Rensberg, N., Brosowski, A., Oehmichen, K., et al. (2019). Stand und Perspektiven der Biogaserzeugung aus Gülle [Broschüre]. DBFZ Dtsch. Biomasseforschungszentrum. 978-3-946629-48-1.
Mata Pérez, M. de la E., Scholten, D., and Smith Stegen, K. (2019). The multi-speed energy transition in Europe: opportunities and challenges for EU energy security. Energy Strategy Rev. 26, 100415. doi:10.1016/j.esr.2019.100415
Matzenberger, J., Kranzl, L., Tromborg, E., Junginger, M., Daioglou, V., Goh, C. S., et al. (2015). Future perspectives of international bioenergy trade. Renew. Sustain. Energy Rev. 43, 926–941. doi:10.1016/j.rser.2014.10.106
Mazac, R., Meinilä, J., Korkalo, L., Järviö, N., Jalava, M., and Tuomisto, H. L. (2022). Incorporation of novel foods in European diets can reduce global warming potential, water use and land use by over 80%. Nat. Food 3 (4), 286–293. doi:10.1038/s43016-022-00489-9
Muscat, A., de Olde, E. M., Ripoll-Bosch, R., Van Zanten, H. H. E., Metze, T. A. P., Termeer, C. J. A. M., et al. (2021). Principles, drivers and opportunities of a circular bioeconomy. Nat. Food 2 (8), 561–566. doi:10.1038/s43016-021-00340-7
MWAE (2023). Energieinfrastruktur: Bioenergie. Energie-und Klimaschutzatlas Brandenburg (EKS). Available at: https://eks.brandenburg.de/.
Naumann, K., Müller-Langer, F., Meisel, K., Majer, S., Schröder, J., and Schmieder, U. (2021). Further development of the German greenhouse gas reduction quota. Background paper. DBFZ Available at: https://www.dbfz.de/fileadmin/user_upload/Referenzen/Statements/Background_Paper_GHG_Quota.pdf.
Nurcholis, M., Ahlasunnah, W., Utami, A., Krismawan, H., and Wibawa, T. (2021). Management of degraded land for developing biomass energy industry. AIP Conf. Proc. 2363 (1), 050005. doi:10.1063/5.0061179
Odegard, I., Croezen, H., and Bergsma, G. (2012). Cascading of biomass. 13 solutions for a sustainable bio-based economy. Making better choices for use of biomass residues. By-products and Wastes. CE Delft. Available at: http://www.cedelft.eu/?go=home.downloadPub&id=1277&file=CE_Delft_2665_Cascading_of_Biomass_def.pdf.
Paredes-Sánchez, B. M., Paredes-Sánchez, J. P., and García-Nieto, P. J. (2022). Evaluation of implementation of biomass and solar resources by energy systems in the coal-mining areas of Spain. Energies 15 (1), 232. doi:10.3390/en15010232
Parzianello, L., and Carvalho, T. S. (2023). What if Brazilians reduce their beef consumption? Available at SSRN 4457482.
Peters, W., Schicketanz, S., and Kinast, P. (2014). Landschaftspflegematerial im Land Brandenburg: potenzialermittlung und Möglichkeiten der energetischen Verwertung [Endbericht]. Ministeriums für Umwelt, Gesundheit und Verbraucherschutz des Landes Brandenburg. Available at: https://mluk.brandenburg.de/sixcms/media.php/9/Landschaftspflegematerial-im-Land-Brandenburg.pdf.
Pietrzykowski, M. (2019). Tree species selection and reaction to mine soil reconstructed at reforested post-mine sites: central and eastern European experiences. Articles initially Publ. Ecol. Eng. X issues 1-4 142, 100012. doi:10.1016/j.ecoena.2019.100012
Plevin, R., and Kammen, D. (2013). “Indirect land use and greenhouse gas impacts of biofuels,” in Encyclopedia of biodiversity. 2 (Academic Press). (S. 293–297). doi:10.1016/B978-0-12-384719-5.00364-6
Prognos, Öko-Institut, & Wuppertal-Institut (2021). Klimaneutrales Deutschland 2045. Wie Deutschland seine Klimaziele schon vor 2050 erreichen kann [Langfassung im Auftrag von Stiftung Klimaneutralität, Agora Energiewende und Agora Verkehrswende]. Available at: https://static.agora-energiewende.de/fileadmin/Projekte/2021/2021_01_DE_KNDE2045/KNDE2045_Langfassung.pdf.
Purr, K., Günther, J., Lehmann, H., and Nuss, P. (2019). Wege in eine ressourcenschonende Treibhausgasneutralität. RESCUE - studie (36/2019; CLIMATE CHANGE). Umweltbundesamt. Available at: https://www.umweltbundesamt.de/sites/default/files/medien/1410/publikationen/rescue_studie_cc_36-2019_wege_in_eine_ressourcenschonende_treibhausgasneutralitaet_auflage2_juni-2021.pdf.
Rai, M., and Ingle, A. P. (2019). Sustainable bioenergy. Advances and impacts. (1. Aufl.). Elsevier.
Rayne, N., and Aula, L. (2020). Livestock manure and the impacts on soil health: a review. Soil Syst. 4 (4), 64. doi:10.3390/soilsystems4040064
Richter, S., Szarka, N., Bezama, A., and Thrän, D. (2022). What drives a future German bioeconomy? A narrative and steeple analysis for explorative characterisation of scenario drivers. Sustainability 14 (5), 3045. doi:10.3390/su14053045
Richwien, M., Baums, B., Rehfeldt, K., Simmering, J., Remmers, K.-H., Krömke, F., et al. (2018). Erneuerbare Energie-Vorhaben in den Tagebauregionen [Bericht im Auftrag des Bundesministeriums für Wirtschaft und Energie (BMWi)]. Available at: https://www.ioew.de/projekt/erneuerbare_energien_vorhaben_in_den_tagebauregionen/.
Rupp, J., Bluhm, H., Hirschl, B., Grundmann, P., Meyer-Aurich, A., Huwe, V., et al. (2020). “Nachhaltige Bioökonomie in Brandenburg Biobasierte Wertschöpfung – regional und innovativ,” in [Broschüre]. Ministerium für Landwirtschaft, Umwelt und Klimaschutz des Landes Brandenburg. Available at: https://mluk.brandenburg.de/sixcms/media.php/9/Nachhaltige-Biooekonomie.pdf.
SAENA (2023). Energieportal sachsen. Available at: https://www.energieportal-sachsen.de/.
Saratale, R. G., Cho, S.-K., Bharagava, R. N., Patel, A. K., Varjani, S., Mulla, S. I., et al. (2022). A critical review on biomass-based sustainable biorefineries using nanobiocatalysts: opportunities, challenges, and future perspectives. Bioresour. Technol. 363, 127926. doi:10.1016/j.biortech.2022.127926
Scarlat, N., and Dallemand, J.-F. (2019). “Future role of bioenergy,” in The role of bioenergy in the bioeconomy (S. 435–547) (Academic Press). doi:10.1016/B978-0-12-813056-8.00010-8
Scheuermann, A., Erfurt, I., Eggemann, J., Reichmuth, M., Schiffler, A., Voigtländer, C., et al. (2012). Regionales Energie-und Klimaschutzkonzept für die Planungsregion Oberlausitz-Niederschlesien—teil B Landkreis Görlitz.
Schmitz, N., Iost, S., Polley, H., and Weimar, H. (2022). Charta für Holz 2.0 Kennzahlenbericht 2021 Forst & Holz. Fachagentur Nachwachsende Rohstoffe e. V. (FNR). Available at: https://www.charta-fuer-holz.de/fileadmin/charta-fuer-holz/dateien/service/mediathek/Web_Kennzahlenbericht_2021.pdf.
Schön, A.-M., and Böhringer, M. (2023). Land consumption for current diets compared with that for the planetary health diet—how many people can our land feed? Sustainability 15 (11), 8675. doi:10.3390/su15118675
Slade, R., Bauen, A., and Gross, R. (2014). Global bioenergy resources. Nat. Clim. Change 4 (2), 99–105. doi:10.1038/nclimate2097
Smith, P., Bustamante, M., and Ahammad, H. (2014). “Agriculture, forestry and other land use (AFOLU),” in (IPCC fifth assessment report: climate change 2014: mitigation of climate change.) (IPCC). Available at: https://www.ipcc.ch/site/assets/uploads/2018/02/ipcc_wg3_ar5_chapter11.pdf.
Sommer, S. G., and Knudsen, L. (2021). Impact of Danish livestock and manure management regulations on nitrogen pollution, crop production, and economy. Front. Sustain. 2. doi:10.3389/frsus.2021.658231
Staatsbetrieb Sachsenforst (2022). Sachsenforst 2021—jahresbericht. Sachsenforst ist eine nachgeordnete Behörde des Sächsischen Staatsministeriums für Energie, Klimaschutz, Umwelt und Landwirtschaft. Available at: file:///C:/Users/leune/Downloads/Jahresbericht_Sachsenforst_2021_bf.pdf.
Statistik Berlin-Brandenburg (2022). Holzeinschlag in Brandenburg. Available at: https://www.statistik-berlin-brandenburg.de/c-v-1-j.
Statistisches Landesamt des Freistaates Sachsen (2018). Statistisches jahrbuch sachsen. Available at: https://www.statistischebibliothek.de/mir/servlets/MCRFileNodeServlet/SNHeft_derivate_00008510/StatistischesJahrbuch_2018_a1b.pdf.
Stegner, J., Kleinknecht, U., Lakner, S., Fleischer, K., and Chamsa, C. (2010). Rahmenkonzept zur energetischen Verwertung von Biomasse aus der Landschaftspflege im Freistaat Sachsen [Abschlussbericht]. Sächsisches Staatsministerium für Umwelt und Landwirtschaft. Available at: https://www.natur.sachsen.de/download/Energetische_Verwertung_von_Biomasse_aus_Landschaftspflege_A.pdf.
Stolarski, M. J., Warminski, K., Krzyżaniak, M., Olba–Zięty, E., and Akincza, M. (2020). Bioenergy technologies and biomass potential vary in Northern European countries. Renew. Sustain. Energy Rev. 133, 110238. doi:10.1016/j.rser.2020.110238
Sulewski, P., Ignaciuk, W., Szymańska, M., and Wąs, A. (2023). Development of the biomethane market in Europe. Energies 16 (4), 2001. doi:10.3390/en16042001
Szarka, N., Haufe, H., Lange, N., Schier, F., Weimar, H., Banse, M., et al. (2021). Biomass flow in bioeconomy: overview for Germany. Renew. Sustain. Energy Rev. 150, 111449. doi:10.1016/j.rser.2021.111449
Thiffault, E., Gianvenuti, A., Zuzhang, X., and Walter, S. (2023). The role of wood residues in the transition to sustainable bioenergy − Analysis of good practices and recommendations for the deployment of wood residues for energy. FAO. doi:10.4060/cc3826en
Thrän, D., Deprie, K., Dotzauer, M., Kornatz, P., Nelles, M., Radtke, K. S., et al. (2023). The potential contribution of biogas to the security of gas supply in Germany. Energy, Sustain. Soc. 13 (1), 12. doi:10.1186/s13705-023-00389-1
Townsend, T. J., Sparkes, D. L., Ramsden, S. J., Glithero, N. J., and Wilson, P. (2018). Wheat straw availability for bioenergy in England. Energy Policy 122, 349–357. doi:10.1016/j.enpol.2018.07.053
Tursi, A., and Olivito, F. (2021). “Biomass conversion: general information, chemistry, and processes,” in Advances in bioenergy and microfluidic applications (S. 3–39) (Elsevier). doi:10.1016/B978-0-12-821601-9.00002-9
UBA (2023). Erneuerbare Energien in Zahlen: Wärme aus erneuerbaren Energien. Available at: https://www.umweltbundesamt.de/themen/klima-energie/erneuerbare-energien/erneuerbare-energien-in-zahlen#uberblick.
UN (2015). The Paris Agreement [UN climate change conference (COP21)]. Available at: https://unfccc.int/sites/default/files/resource/docs/2015/cop21/eng/10.pdf?download.
UN (2022). The sustainable development goals report 2022. United Nations Department of Economic and Social Affairs Sustainable Development. Available at: https://unstats.un.org/sdgs/report/2022/The-Sustainable-Development-Goals-Report-2022.pdf.
Welfle, A. (2017). Balancing growing global bioenergy resource demands—Brazil’s biomass potential and the availability of resource for trade. Biomass Bioenergy 105, 83–95. doi:10.1016/j.biombioe.2017.06.011
Wydra, S., Hüsing, B., Köhler, J., Schwarz, A., Schirrmeister, E., and Voglhuber-Slavinsky, A. (2021). Transition to the bioeconomy – analysis and scenarios for selected niches. J. Clean. Prod. 294, 126092. doi:10.1016/j.jclepro.2021.126092
Xu, X., Liu, G., and Mola-Yudego, B. (2022). Barriers and opportunities for bioenergy expansion in Chinese rural areas. Energy Sustain. Dev. 70, 181–193. doi:10.1016/j.esd.2022.06.012
Zander, K., Will, S., Göpel, J., Jung, C., and Schaldach, R. (2022). Societal evaluation of bioeconomy scenarios for Germany. Resources 11 (5), 44. doi:10.3390/resources11050044
Zeller, V., Thrän, D., Zeymer, M., Bürzle, B., Adler, P., Ponitka, J., et al. (2012). Basisinformationen für eine nachhaltige Nutzung von landwirtschaftlichen Reststoffen zur Bioenergiebereitstellung. (13; DBFZ Reports). DBFZ Deutsches Biomasseforschungszentrum. Available at: https://www.dbfz.de/fileadmin/user_upload/Referenzen/DBFZ_Reports/DBFZ_Report_13.pdf.
Zschau, B., Beblek, A., Gutzler, C., Mechler, M., Mixdorf, U., Ludwig, A., et al. (2013). Regionales Energiekonzept für die Region Lausitz-Spreewald [Endbericht]. Available at: https://www.region-lausitz-spreewald.de/de/projekte/regionales-energiemanagement/regionales-energiekonzept/artikel-regionales-energiekonzept-lausitz-spreewald-2013.html.
Keywords: regional biomass potential, bioenergy scenarios, coal region, regional energy transition, Lusatia
Citation: Leuner B and Hirschl B (2024) Biomass and bioenergy perspectives of a coal region: status quo, potential and scenarios in Lusatia. Front. Energy Res. 11:1275733. doi: 10.3389/fenrg.2023.1275733
Received: 10 August 2023; Accepted: 29 December 2023;
Published: 15 January 2024.
Edited by:
Philip Owende, Technological University Dublin, IrelandReviewed by:
Anthony Lau, University of British Columbia, CanadaNiamh Power, Munster Technological University, Ireland
Copyright © 2024 Leuner and Hirschl. This is an open-access article distributed under the terms of the Creative Commons Attribution License (CC BY). The use, distribution or reproduction in other forums is permitted, provided the original author(s) and the copyright owner(s) are credited and that the original publication in this journal is cited, in accordance with accepted academic practice. No use, distribution or reproduction is permitted which does not comply with these terms.
*Correspondence: Bruna Leuner, bGV1bmVyQGItdHUuZGU=