- 1Department of Zoology, George S. Wise Faculty of Life Sciences, Tel Aviv University, Tel Aviv, Israel
- 2Department of Evolutionary Biology and Ecology, Université Libre de Bruxelles, Brussels, Belgium
- 3IRBI, UMR 7261, Université Francois Rabelais de Tours, Tours, France
The nesting habits of ants play an important role in structuring ant populations. They vary from monodomy, a colony occupies a single nest, via polydomy, a colony occupies multiple adjacent nests, to supercoloniality, a colony spans over large territories comprising dozen to thousands nests without having any boundaries. The population structure of the desert ant Cataglyphis niger, previously considered to form supercolonies, was studied using genetic, chemical, and behavioral tools in plots of 50 × 50 m at two distinct populations. At the Palmahim site, the plot comprised 15 nests that according to the genetic analysis constituted three colonies. Likewise at the Rishon Leziyyon site 14 nests constituted 5 genetic colonies. In both sites, both chemical analysis and the behavioral (aggression) tests confirmed the colony genetic architecture. The behavioral tests also revealed that aggression between colonies within a population was higher than that exhibited between colonies of different populations, suggesting the occurrence of the “nasty neighbor” phenomenon. In contrast to supercolony structure previously reported in another population of this species, the presently studied populations were composed of polydomous colonies. However, both the genetic and chemical data revealed that the inter-colonial differences between sites were larger than those within site, suggesting some within-site population viscosity. Thus, C. niger exhibits flexible nesting characteristics, from polydomy to supercoloniality, and can be considered at the brink of supercoloniality. We attribute the differences in population structure among sites to the intensity of intraspecific competition.
Introduction
The diversity of spatial organization of ant populations is associated greatly with differences among species in life history, reproductive strategies and dispersion (Bourke and Franks, 1995; Crozier and Pamilo, 1996; Ross, 2001). The classical, possibly ancestral ant colony is composed of a single, monandrous (single mating) queen that is the sole reproducer and her sterile daughters that act as workers and tend to the brood and all other colony tasks. This basic colony kin structure, leading to high levels of relatedness among colony members, is believed to have evolved via kin selection (Hölldobler and Wilson, 1990; Hughes et al., 2008a; Boomsma, 2009). Typically, each colony-family occupies a nest around which it maintains a territory that it defends against intruders, con- and heterospecific alike.
Genetic studies, however, revealed that breeding systems that lower intracolony relatedness have evolved multiple times in ants (Hughes et al., 2008b). For example, both queen number and queen mating frequency may vary greatly between species, and even within species in a population. Colonies may be headed by a single queen (monogynous) or by two or more queens (polygynous) (Bourke and Franks, 1995; Crozier and Pamilo, 1996; Purcell and Chapuisat, 2013). Species may also vary in the number of males with which the queen(s) mate, from strict monandry to high levels of polyandry (Boomsma et al., 2009). This social polymorphism is the result of multiple selective pressures such as ecological constraints on nesting success, predation risks, or increased genetic diversity to better defend against pathogens or to enhance division of labor and colony task efficiency (Bourke and Franks, 1995; Keller, 1995; Crozier and Pamilo, 1996). Colony queen number is usually associated with profound changes in life history strategies and dispersal behavior (Bourke and Franks, 1995; Keller, 1995; Crozier and Pamilo, 1996; Ross, 2001; Chapuisat et al., 2004). Monogyny is generally (but see exceptions in Peeters and Molet, 2010; Cronin et al., 2013) associated with long-range nuptial flights and independent colony foundation. In contrast, under polygyny, mating occurs inside or in the vicinity of the natal nest, and colony reproduction proceeds by budding whereby the mated queen(s) leave their natal nest with a worker force to found a new colony nearby. At the population level, dispersion by budding usually results in a pattern of genetic isolation-by-distance (Sundstrom et al., 2005; Leniaud et al., 2009). In some ant species, the daughter colonies maintain exchanges of workers, brood, and food with the mother nest creating polydomous colonies, each composed of several nest units (Hölldobler and Wilson, 1990; Debout et al., 2007). In its most extreme form, polydomy can lead to the creation of supercolonies composed of several hundreds of nests, with no aggression between workers that belong to different units over a vast geographic scale (Pedersen et al., 2006; Thomas et al., 2006). Such unicoloniality is typical of many invasive ants, and possibly also the key to their success (Holway et al., 2002).
Supercoloniality poses an evolutionary challenge of understanding the selective forces that result in their prosperity, and whether or not kin selection operates despite the multiple genetic lineages that compose such supercolonies (Helantera et al., 2009; Moffett, 2012). Unicoloniality is also typified by the lack of aggression, even between very distant nests e.g., Linepithema humilis (Giraud et al., 2002; Brandt et al., 2009b; Blight et al., 2012), Wasmannia auropunctata (Errard et al., 2005; Vonshak et al., 2009; Foucaud et al., 2010) or Pheidole megacephala (Fournier et al., 2009). This is attributed to the relaxation or complete loss of nestmate recognition in these population, the mechanism of which is postulated to be either “genetic cleansing” following introduction (Giraud et al., 2002) or a “bottleneck” phenomenon (Suarez et al., 2008). Ample evidence indicate that hydrocarbons constitute at least part of the recognition system (reviewed in Hefetz, 2007), and that there is high degree of congruency in the hydrocarbon recognition cues within a supercolony and distinct compositions between supercolonies (Errard et al., 2005; Brandt et al., 2009a). Recently, it was shown in the unicolonial ant Formica yessensis that profile similarity/disparity as well as incomplete chemical discrimination via the hydrocarbon sensilla (Kidokoro-Kobayashi et al., 2012) are important factors in the establishment of unicoloniality.
Cataglyphis ants have evolved a remarkable number of life histories and reproduction strategies (Lenoir et al., 2009). Some species are strictly monogynous (Pearcy et al., 2004a; Timmermans et al., 2008) while others are facultatively or obligatory polygynous (Leniaud et al., 2011; Eyer et al., 2012). Monogynous species may be organized as independent colonies (Leniaud et al., 2011) while others are organized in polydomous colonies, with one central nest holding the queen and the rest of the nests function as queenless satellites (Dahbi et al., 1997). In contrast to the usual association between monogyny and long-range nuptial flights, young queens of the monogyne C. cursor and C. floricola mate close to their natal nest and colony reproduction proceeds by fission (Lenoir et al., 1988; Hardy et al., 2008; Amor et al., 2011; Chéron et al., 2011). Queens of some species are singly mated (Eyer et al., 2012; Leniaud et al., 2012), whereas in other species they are obligatory multiply mated (Timmermans et al., 2008, 2010; Pearcy et al., 2009). Finally, queens of several species use alternative reproductive pathways for the production of the queen and worker castes. New queens are produced asexually by thelytokous parthenogenesis, while workers are produced by normal sexual reproduction (Pearcy et al., 2004a; Leniaud et al., 2012; Eyer et al., 2013).
A recent population genetic study of Cataglyphis niger revealed that it is polygynous, that queens mate multiply and that the population sampled was supercolonial (Leniaud et al., 2011). However, this study encompassed only a single population and a limited sample size of 12 nests in an area of 4 square kilometers. Moreover, neither the chemistry or aggressive behavior of its nest constituents were studied to support the genetic data.
In the present study, we investigated the association between genetic architecture, chemical signature, and nestmate recognition in two, newly sampled populations of C. niger. First, we determined the structure of populations, using microsatellites markers. Second, we measured intra- and inter-population tolerance on the basis of aggression tests between workers. Finally, we examined the pattern of variation of worker cuticular hydrocarbon profiles in relation to population genetic structure and the spatial distribution of colonies within the population and nests within a colony.
Methods and Materials
Field Collection and Sampling
Ant samples were collected at two study sites, representing two disparate populations (~15 km apart), on the Israeli coastal plain in Rishon Leziyyon (31.98056, 34.742863) and Palmahim (31.935046, 34.705571). All nests at each site (14 and 15 nests for Rishon Leziyyon and Palmahim, respectively) were partially excavated and approximately 50 workers sampled. The spatial distribution and distances between nests and the distances between physical nests in each plot were carefully measured on a defined and marked 50 × 50 m area. A portion of the workers was immediately frozen for further molecular and chemical analysis and the remaining workers were brought back to the laboratory for behavioral bioassays. In the laboratory they were placed in artificial nests composed of a round plastic box 10 cm in diameter and 8.5 cm in height, with a plaster laid floor to obtain sufficient moister. The ants were kept in a rearing room under a controlled temperature of 29 ± 1°C and a photoperiod of 12L:12D, and were provided with an identical diet of sugar water and minced insects three times a week. The colonies from each site were kept in the laboratory for not longer than 1 week, during which the behavioral assays were conducted.
Genetic Analyses
DNA extraction—Individual ants were ground in digestion solution (100 mM NaCl, 50 mM Tris, 1 mM EDTA, 0.5% SDS, and 200 μg/ml proteinase K) and incubated for 12 h at 55°C in 100 μl of solution. Genomic DNA was purified through phenol/chloroform extractions and ethanol precipitation following standard protocols (Sambrook and Russell, 2001). The DNA pellet was resuspended in 100 μl of sterile H2O.
Genomic DNA genotyping—Genotypes of ants were determined at 9 microsatellite loci: four loci previously described for C. cursor (Cc11, Cc51, Cc54, Cc99; Pearcy et al., 2004b), three for C. hispanica (Ch01, Ch11, Ch12; Darras et al., 2014), and two new markers developed for C. niger (Cn04, Cn08). PCR were performed with a MJ Research PTC-200 thermocycler. Annealing temperature and magnesium concentration were optimized for each locus individually (available upon request). PCR products were genotyped on a ABI 3100 automated sequencer (Applied Biosystems). The size of the different alleles was determined using the Peak Scanner version 1.0 analysis software (Applied Biosystems) and used to construct a multilocus genotype for each individual. Control for genotyping errors due to null alleles and allele drop-outs was performed with Micro-Checker (Van Oosterhout et al., 2004). Possible linkage disequilibrium were tested with GENEPOP ON THE WEB (Rousset, 2008).
Genetic analyses were performed on a sample of 168 workers (X ±SE = 11.2 ± 1.6, N = 14 nests) from Rishon Leziyyon, and 168 workers (12 ± 0, N = 15 nests) from Palmahim. The number of alleles, allele frequencies, observed (HO) and expected (HE) heterozygosities were calculated using FSTAT 2.9.3.2 (Goudet, 1995) and averaged across loci. F-statistics were estimated according to the method of Weir and Cockerham (1984) using GENEPOP ON THE WEB (Rousset, 2008). To determine if different nests belonged to the same genetic entity (i.e., polydomy), genotypic frequencies at all collection points were compared using a log-likelihood (G) based test of differentiation, from GENEPOP ON THE WEB (Rousset, 2008). Overall significance was determined using a Fisher's combined probability test; a Bonferroni correction was applied to account for multiple comparisons. Nests were considered as belonging to different colonies if genotypic differentiation was statistically significant (α < 0.0005 and α < 0.0006 after Bonferroni correction for Palmachim and Rishon Leziyyon, respectively). Population structure was investigated by plotting [Fst/(1–Fst)] coefficients between pairs of nests against the ln of the geographical distance (Slatkin, 1993). The correlation coefficients between genetic differentiation [Fst/(1–Fst)], ln of geographical distances and chemical distances were assessed with a Mantel test as implemented in GENEPOP ON THE WEB (Rousset, 2008). Genetic-distance based PCoA was performed with GENALEX v. 6.41. Relatedness coefficients among workers were estimated using the program FSTAT 2.9.3.2 (Goudet, 1995). The mean relatedness was estimated between workers of the same colony relative to the collection site.
Behavioral Bioassays
Encounters were conducted in dyads using randomly selected workers from two randomly selected physical nests either within a site or between sites. Different workers were used for each trial, and the order of encounter types was random. Before each test, each of the workers was gently placed in an open glass tube (3 cm in diameter and 8 cm in height) within the neutral arena (10 cm in diameter and 8.5 cm in height) for 90 s for acclimatization. The test started by lifting the enclosing glass tubes and lasted for 3 min. The behavior of the ants was recorded in real time using the program JWatcher (version 0.9; http://www.jwatcher.ucla.edu), as well as videotaped, as backup, using a Logitech HD webcam pro C910 video camera. Encounters between individuals from the same nests were considered as nestmate control assays; encounters between individuals belonging to different physical nests, whether from the same or different sites, were considered as non-nestmate experimental assays. The arena was paved with a filter paper that was replaced every trial, to avoid residual odors.
Aggression was scored using a graded index from 0–4 as follows: 0, antennation; 1, mandibular threat; 2, short biting with jumping; 3, biting and /or seizing the opponent with mandibles; 4, curling the abdomen and spraying formic acid. The aggression index was calculated as follows:
where Ai and ti are the aggression score and duration of each act, respectively, and T is the total interaction time defined as the sum of times in which the ants were in physical contact (not necessarily equal to assay duration). For each encounter type (that is, involving workers from two specific nests), we performed 4–6 replicate tests and the average of which was defined as the aggression index. A total of 20 (13 involving different physical nests and 6 involving nestmates) and 17 (12 involving different physical nests and 5 involving nestmates) encounter types were done at the Palmahim and Rishon Leziyyon sites, respectively (For details of the physical nests assayed see Table 1). In addition we performed 9 encounters (37 replicates in total) between nests from the two sites.
Chemical Analyses
Previous findings have shown that the cuticular hydrocarbon (CHCs) serve as the recognition cue in C. niger (Lahav et al., 1999) and that there is complete congruency in hydrocarbon composition between the epicuticle and those of the postpharyngeal glands (PPG) (Soroker et al., 1994, 1995). Since the PPG secretion is by far more copious than extractable cuticular hydrocarbons, it enables a much more accurate and fine tuned analysis, and can serve as representative of the CHC composition.
Fifteen workers from each nest were used for the chemical analysis. The PPG of individual workers was cleanly dissected and extracted in 100 μl pentane, containing 625 ng docosane and 6250 ng of tetratriacontane as internal standards. Compound identification was done by combined GC/MS (see also Soroker et al., 1995) and was based on their fragmentation patterns, as compared to authentic standards. Sample quantification was done by gas chromatography, using a VF-5ms capillary column that was temperature-programmed from 150°C (with 1 min initial hold) to 300°C at a rate of 5°C per min, with a final hold of 15 min. Peak integration was performed using the program Galaxie Varian 1.9. Compounds that constituted less than 1% across all samples were omitted from the analyses, because their quantification is unreliable. For assessing hydrocarbon profile-specificity we used multivariate statistics, i.e., discriminant analysis using the stepwise forward mode. This module of the program works initially as a principle component analysis for reducing the number of variables to fit the number of cases, and then perform the discriminant function.
Results
Cartography of the nests revealed the occurrence of 14 and 15 nests, with a mean ±SD distance between nests of 7.52 ± 6.22 m and 12.56 ± 10.83 m in Palmahim and Rishon Leziyyon, respectively. In both sites, nests were not evenly distributed within the plot, but were clustered (Figures 1A,B). The index of aggregation R for the Plamahim site was 0.701 and for Rishon Leziyyon was 0.854. However, if we Exclude nest # 291 who was the only one that belonged to the genetic colony C5, the R value at Rishon Leziyyon was reduced to 0.506. Both indicate that nests were not randomly dispersed, but are aggregated (Clark and Evans, 1954).
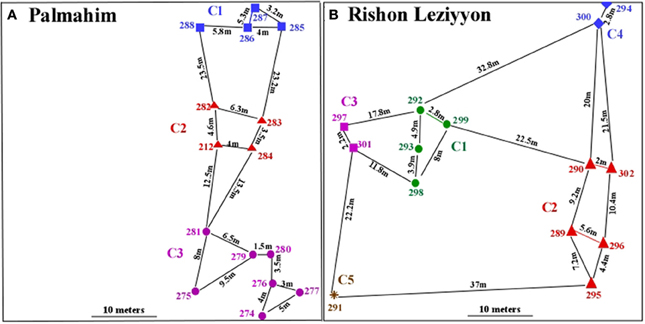
Figure 1. Spatial distribution of nests at the Palmahim (A) and Rishon Leziyyon (B) sites. Each nest is represented by a number (e.g., 285; 286 etc.) and each genetic colony is represented by a different symbol, color and number (e.g., C1; C2 etc.).
Genetic Analyses
None of the 9 microsatellite markers genotyped showed indication of null alleles. However, linkage disequilibrium was found between three marker loci (i.e., Ch01, Ch11, and Cc11), which were therefore removed from our analyses. The number of alleles at the six remaining microsatellite loci ranged from 4 to 15 in Palmahim, and from 5 to 22 in Rishon Leziyyon. Mean heterozygosity was HO = 0.78 (range: 0.59–0.87) and HE = 0.76 (range: 0.54–0.80) in Palmahim and HO = 0.78 (range: 0.40–0.94) and HE = 0.76 (range: 0.69–0.84) in Rishon Leziyyon. The fixation index (FIT) was slightly superior of zero in Palmahim and not different from zero in Rishon Leziyyon, indicative of random mating in both populations sampled (Palmahim: mean ±SEjackknife over loci = 0.055 ± 0.042; 95% CI: 0.017–0.132; permutation test P = 0.001; Rishon Leziyyon: mean ±SEjackknife over loci = 0.018 ± 0.023; 95% CI: 0.025–0.083; permutation test P = 0.23).
In Palmahim, the G-test showed that the 15 nests sampled clustered into 3 genetically distinct colonies, each comprising 4–7 nests that were not differentiated genetically (Figure 1A) (P > 0.00055 after Bonferroni correction; CI: 0.08–0.099): colony 1 (nests 285, 286, 287, 288), colony 2 (nests 212, 282, 283, 284) and colony 3 (nests 274, 275, 276, 277, 279, 280, 281). The Fst estimates between colonies were moderate and significantly different from zero (0.162 ± 0.022; 95% CI: 0.122–0.201; permutation test P = 0.0). The Similarly, in Rishon Leziyyon the G-test (P > 0.0006) indicated that the 14 nests clustered into 5 genetically distinct colonies comprising 1–5 nests (Figure 1B): colony 1 (nests 292, 293, 298, 299), colony 2 (nests 302, 289, 290, 295, 296), colony 3 (nests 297, 301), colony 4 (nests 300, 294) and colony 5 (nest 291). The FST estimate between colonies was moderate and significantly different from zero (0.156 ± 0.012; 95% CI: 0.131–0.174; permutation test P = 0.0).
In Palmahim, the mean within physical nest genetic relatedness among workers (nestmates) was 0.221 ± 0.025 and within-colony (colony-mates) was 0.31 (SEjackknife = 0.03, N = 15). For Rishon Leziyyon it was 0.259 ± 0.023 and 0.30 for nestmates and colony-mates, respectively (SEjackknife = 0.02, N = 14). These values are significantly lower than 0.75 expected under monogyny, monoandry and random mating in haplodiploid organisms (two-tailed t-tests, P < 0.005 for the two populations).
Behavioral Assays
Effect of the workers' origin (nest, colony origin, site) on aggression levels was tested using dyadic encounters. The initial selection of nests for the dyadic encounters was done randomly, without knowledge of their genetic compositions. However, since we hypothesize that nests that are genetically indistinguishable show also high degree of tolerance, the analyses of the aggression tests proceeded according to colony classification using the molecular data as follows: nestmates (i.e., workers from the same physical nest); workers of different nests but within the same genetic colony; workers from nests belonging to different genetic colonies within a site; and workers from nests of the two sites. Figure 2A presents the results according to colony and Figure 2B present the pooled results as well as the result of the encounters between colonies of the different populations. The results of both types of analyses were in accordance with colony assignment using the molecular results. Both at the Palmahim and Rishon Leziyyon sites, level of aggression between workers from different nests belonging to the same genetic colony was low and not significantly different from that between nestmates. In contrast, the aggression between workers from nests belonging to different genetic colonies, within a site, was significantly higher than that between workers of different nests within a genetic colony or nestmates (for particular encounters, Figure 2A: Palmahim site One Way ANOVA, F = 19.2, df = 6, p < 0.001, followed by Tukey post hoc test p < 0.05; Rishon Leziyyon site (One Way ANOVA, F = 20.26, df = 9, p < 0.001, followed by Tukey post hoc test p < 0.05) (For pooled encounters, Figure 2B: One Way ANOVA, F = 54.75, df = 6, p < 0.01, followed by Tukey post hoc test p < 0.05). Interestingly, the aggression between workers belonging to colonies from the different sites was significantly lower than that between colonies of the same site.
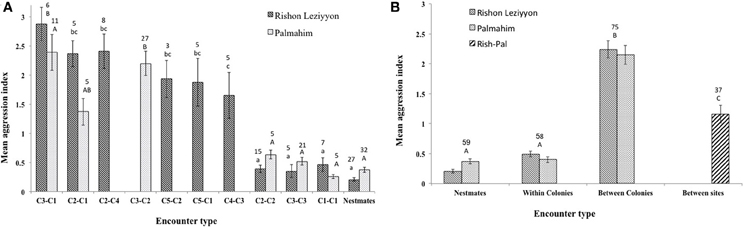
Figure 2. Mean aggression (± standard error) between workers in the different encounter types from either Rishon Leziyyon (hatched bars) or Palmahim (dotted bars) sites. (A) Data of encounters between particular genetic colonies including (1) Nestmates; (2) workers from different nests belonging the same genetic colony (e.g., C2–C2); and (3) of nests belonging to different genetic colonies (e.g., C3-C2). For details of the physical nest assayed see Table 1. (B) Pooled data of the above 3 encounter types and encounters between colonies of the different populations. Statistical significance is denoted by letters above the columns [For (A); capital letters refer to the Palmahim site and small letters to the Rishon Leziyyon site]. The numbers above the columns indicate replications.
Chemical Analyses
The chemical composition and compound identification of the PPG secretion is presented in Figure 3. The secretion is composed of hydrocarbons ranging from tetracosane to tritriacontane comprising linear, monomethyl, diemethyl, and trimethyl branched components. The secretion was dominated by 2 peaks comprising a mixture of 11- + 13- + 15-methylnoacosane and 11,15- + 13,15-dimethylnonacosane, similar to previous analyses (Soroker et al., 1995).
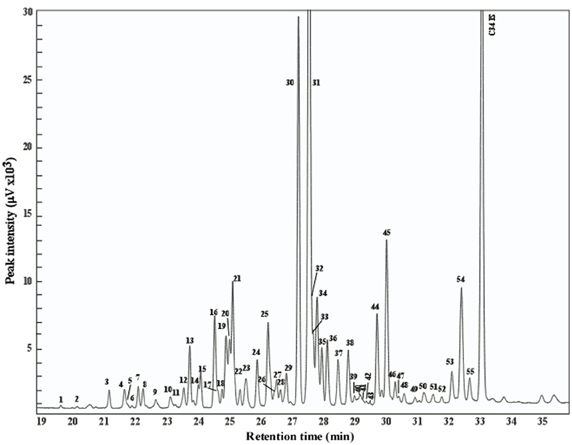
Figure 3. Chromatogram of the PPG secretion of a C. niger worker from Rishon Leziyyon site. Peaks identity is as follows: 1, Tetracosane; 2, 12-methyltetracosane; 3, pentacosane; 4, 11- + 13-methylpentacosane; 5, 7-methylpentacosane; 6, 5-methylpentacosane; 7, 5,17-dimethylpentacosane; 8, 3-methylpentacosane; 9, hexacosane; 10, 10- + 12- + 14-methylhexacosane; 11, 6–methylhexacosane; 12, heptacosene isomer 1; 13, heptacosene isomer 2; 14, heptacosene isomer 3; 15, heptacosane; 16, 11- + 13- methylheptacosane; 17, 7-methylheptacosane; 18, 5-methylheptacosane; 19, 11,15-dimethylheptacosane; 20, 7,11-dimethylheptacosane; 21, 3-methylheptacosane; 22, 5,9-dimethylheptacosane + 5,11-dimethylheptacosane; 23, 7, 11, 15-trimethylheptacosane; 24, 12-methyloctacosane; 25, 8,12-dimethyloctacosane + 12,16-dimethyloctacosane; 26, 6,12-dimethyloctacosane; 27, 4,12-dimethyloctacosane; 28, nonacosene; 29, nonacosane; 30, 11- + 13- + 15 methylnonacosane; 31, 11,15-dimethylnonacosane + 13,15 dimethylnonacosane; 32, 9,13 dimethylnonacosane; 33, 7,13 dimethylnonacosane; 34, 3-methylnonacosane + 5,13 dimethylnonacosane; 35, 7, 11, 15-trimethylnonacosane; 36, 4, 11, 13-trimethylnonacosane; 37, 14-methyltriacontane; 38, 10,14-dimethyltriacontane + 12,14-dimethyltriacontane; 39, 6,14-dimethyltriacontane; 40, 4,14-dimethyltriacontane; 41, 6,12,20-trimethyltriacontane; 42, x,y,z-trimethyltriacontane; 43, 4,12,20-trimethyltriacontane; 44, 13- + 15-methylhentriacontane; 45, 11,15-dimethylhentriacontane; 46, 7,15-dimethylhentriacontane; 47, 5,15-dimethylhentriacontane; 48, 7,11,15-trimethylhentriacontane; 49, 12- + 14-methyldotriacontane; 50, 12, 14-dimethyldotriacontane + 12,16 dimethyldotriacontane; 51, tritriacontane isomer 1; 52, tritriacontane; 53, 13- + 15-methyltritriacontane; 54, 11,15-dimethyltritriacontane; 55, 11,15,19-trimethyltritriacontane.
To examine whether differences in hydrocarbon profiles of workers match the behavioral and molecular data, we performed a disriminant analysis of the genetically defined colonies in Palmahim and Rishon Leziyyon (Figures 4A,B). For that purpose we used all the individuals that belong to a genetic colony irrespective of their nest origin. The analysis performed used the stepwise forwards module, which ranks the variables according to their impact and accordingly reduce their number to fit the appropriate number of cases. All three genetic colonies in Palmahim were completely chemically distinct (Figure 4A; Wilks' Lambda: 0.0090835, F(38, 96) = 23.98068 p < 0.005). Likewise, all five genetic colonies in Rishon Leziyyon were chemically distinct (Figure 4B; Wilks' Lambda: 0.0000376 F(100, 161) = 19.44184 p < 0.0000). Table 2 compares the Mahalanobis distances between nests, measured irrespective of their grouping, within a genetic colony to that between nests belonging to different genetic colonies. For both populations, the cuticular hydrocarbon profiles of nests within a genetic colony were significantly more similar to each other than to those of nests belonging to a different genetic colony (t-test Palmahim: n1 = 30, n2 = 41, t = −7.5779, df = 68, p < 0.0001; t-test Rishon Leziyyon: n1 = 16, n2 = 61, t = −6.8134, df = 35, p < 0.0001).
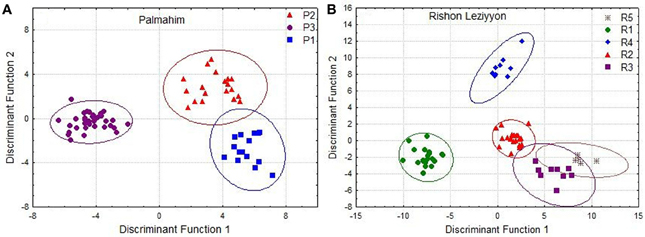
Figure 4. Discriminant analysis based on cuticular hydrocarbons of individuals from Palmahim (A) and Rishon Leziyyon (B) sites. For these analyses we considered all individuals of a specific genetic colony as colony-mates. Clear separation emerges from this analysis and divides the two populations: 3 colonies in Palmahim and 5 colonies in Rishon Leziyyon.
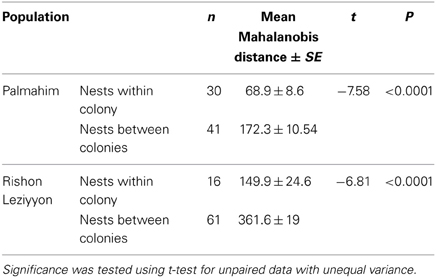
Table 2. Chemical (Mahalanobis) distances, using worker CHC composition, between nests of Cataglyphis niger either within a genetic colony or between genetic colonies.
Association between Variation of the Cuticular Profiles, Genetic Composition, and Spatial Distribution
Table 3 presents the results of the Mantel tests of the correlation between the genetic composition, cuticular hydrocarbon composition and the geographical distances between nests. In both populations, genetic differentiation between pairs of nests was significantly correlated with geographical distance, indicating a pattern of isolation-by-distance (rgen, geo = 0.68 and 0.57 in Rishon Leziyyon and Palmahim, respectively; both p < 0.0001). Likewise there was a significant correlation between the genetic differentiation and variation in cuticular hydrocarbon composition (rgen, chem = 0.53 and 0.66, for Rishon Leziyyon and Palmahim respectively; both p < 0.0001). The correlation between chemical composition and geographic distance was less pronounced albeit it was also significant (rgeo, chem = 0.48, p < 0.0001 and rgeo, chem = 0.24, p < 0.02, for Rishon Leziyyon and Palmahim respectively).
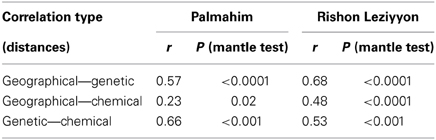
Table 3. Correlation between geographical (between-nest distances), genetic (microsatellite variation) and chemical (cuticular hydrocarbon composition) distances of workers of Cataglyphis niger.
Inspection of the CHC composition of nests from the two populations showed some more profound differences between populations. We therefore performed a discriminant analysis comprising nests from both populations. This revealed that colonies within a population were more similar to each other than between populations (Figure 5A; Wilks' Lambda: 0.0000014 F(174, 585) = 29.74149 p < 0.000). Consistent with these results, a PCoA based on the genetic distances (Eigen values) between nests belonging to the two populations showed that the two populations are mostly distinct genetically, with only some overlap involving colony 3 of Palmahim and colony 3 of Rishon Leziyyon (Figure 5B).
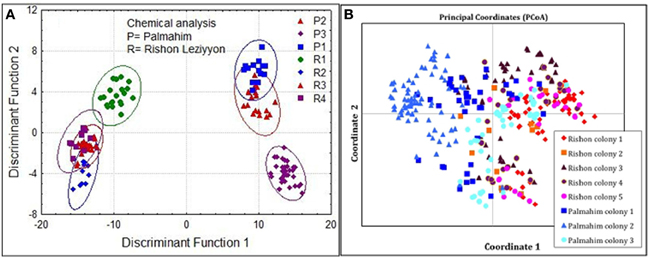
Figure 5. Chemical and genetic comparisons between colonies comprising the two populations studied, Palmahim and Rishon Leziyyon. Discriminant analysis of cuticular hydrocarbons (A) and Principal Coordinates Analysis (PCoA) based on the microsatellite analyses (B). R1–R4 denote the 4 colonies in Rishon Leziyyon population (colony 5 comprised only one nest and therefore excluded), and P1-P3 denote the 3 colonies of the Palmahim population.
Discussion
Polydomy is a nesting habit whereby a colony is composed of several nests that extend over a large territory and is characterized by constant worker movement between nests. It enables the colony a larger foraging area, the extent of which (i.e., the number of nests per colony and their spatial distribution) is limited by competition with other colonies in the population (reviewed by Hölldobler and Wilson, 1990; Debout et al., 2007). Highly extended polydomy can comprise many nests and when it extends over very large geographic areas the species is considered supercolonial. Supercolonial population-structure typifies many invasive ant populations (Holway et al., 2002), but it may also occurs in native populations (Higashi and Yamauchi, 1979; Holzer et al., 2006; Buczkowski and Bennett, 2008; Buczkowski, 2010). Polydomy and supercoloniality can be detected genetically as well as behaviorally and chemically. Members of a supercolony are tolerant to each other even if they originate from different nests at the time of their testing, and exhibit chemical uniformity of cuticular hydrocarbon compositions (Errard et al., 2005; Brandt et al., 2009b; Vonshak et al., 2009; Blight et al., 2012; Kidokoro-Kobayashi et al., 2012). Therefore, assessing the population structure of an ant species is best done by encompassing all three parameters, genetic, behavior and recognition chemistry. Recently we have reported a genetic analysis of colonies of C. niger from a population (Ashqelon), located in the southern coastal plain of Israel. We found a genetic uniformity of 12 nests that span over 4 km2, suggesting that the species forms a supercolony (Leniaud et al., 2011). However, this study was based on a single population with limited sample size.
In contrast to our previous finding with the Ashqelon population (Leniaud et al., 2011), our present study combining genetic, behavioral assays and chemical analyses shows that both the Palmahim and Rishon Leziyyon populations are polydomous rather than supercolonial. Our genetic analysis revealed that average relatedness values among workers from the same colony are very similar in both Palmahim and Rishon Leziyyon (rw–w ≈ 0.30). Quite surprisingly, these values are 10 folds of that registered for the Ashqelon population (0.02). Despite the fact that both sites in the present study were somewhat smaller (50 × 50 m each) than that in Ashqelon we could detect 3 genetically different colonies in Palmahim, comprising 4,−7 nests, and 5 colonies in Rishon Leziyyon, comprising 1–5 nests. Since we limited our analyses to a 50 × 50 m plots it is hard to generalize on a mean number of nests per colony. Yet, together these results do not support supercoloniality in the two presently studied populations of C. niger. The marked difference in within-colony genetic relatedness between this work and the population of Ashqelon remains enigmatic. A research constraint we had in the current study was our inability to completely excavate the nests. As a consequence, no queen could be sampled; hence, the number of queens per nest/colony and their mating frequency, two primary factors on relatedness values within colonies, remain unknown. Therefore, our genetic data are limited to the analyses of workers only. Nevertheless comparing the within and between colony genetic similarity of the two populations indicate some genetic viscosity within each, facilitating high polydomy and perhaps bringing the population to the verge of supercoloniality. Thus the differences in population structure between Ashqelon and Palmahim and Rishon Leziyyon lend credence to the hypothesis that C. niger shows large plasticity in social structure, and what we may observe is a transition state from high polydomy to supercoloniality. Similar plasticity was also recorded for Formica truncorum where in certain sites the population is largely polydomus while in other sites it is unicolonial (Rosengren et al., 1985; Elias et al., 2005).
There is a good congruency between the genetic data and both the behavior and the chemistry. Workers from different nests but from the same genetic colony were as tolerant to each other as are nestmates. This was true for both populations and is depicted more pronouncedly when we compared nestmate vs colony-mate vs. between colonies, irrespective of colony identity (Figure 2B). Interestingly, aggression between colonies of the two populations was significantly lower than that between colonies within population. We consider this phenomenon as “nasty neighbor” (as opposed to the “dear enemy” phenomenon). Nasty neighbor phenomenon is common in social insects, in particular ants where the threat from neighboring nests is far greater than a wondering individual from a colony that is further away (Knaden and Wehner, 2003; Sanada-Morimura et al., 2003; Boulay et al., 2007; Thomas et al., 2007; Wilgenburg, 2007; Newey et al., 2010; Roux et al., 2013). Competition between colonies for space in polydomous species is predicted to be even higher compared to monodomous species due to their ability to create new satellite nests as strongholds in the new territory and by that facilitating the expansion of their foraging area.
Nestmate recognition in C. niger, and in all likelihood in other ant species, is mediated by cuticular hydrocarbons (Lahav et al., 1999). We therefore estimated their chemical similarity/disparity according to the genetic colonies. For both sites there was a clear segregation of profiles according to colony identity, with almost no overlap. Comparing the chemical (Mahalanobis) distances confirmed that the distance between workers of a colony, irrespective of their nest origin, was significantly lower than that between workers of different colonies. Given the moderate genetic viscosity within site, we were interested in comparing the sites also with respect to their CHC profiles. It is clear from Figure 5A that the two populations are markedly different, much more than that based on genetics. This suggests that both genetics and the environment, i.e., difference in locality, may affect the composition of the CHCs.
In summary, all three parameters investigated, genetics, behavior and chemistry point to the fact that colonies of C. niger in both Palmahim and Rishon Leziyyon are polydomous in nature. This is in contrast to a previous study in which the population of Ashqelon was indicated by genetics to be unicolonial. We tend to exclude the possibility that in Ashqelon the population is also polydomous rather than unicolonial. The total area that the 12 colonies occupied was 4 km2 and the mean distance between physical nests was over 50 m, in line with the phenomenon of unicoloniality. The present site span over a smaller area of 2.5 km2, and the mean distance between nests ranged between 7 and 12 m, in line with polydomy. These differences may be attributed to several factors, among which we consider intraspecific competition to be a major one. Adephic and climatic conditions among the 3 sites do not differ by much, but the populations in Palmahim and Rishon Leziyyon are situated sites that are highly visited by human, compared to the site in Ashqelon, which seemingly generate greater resources. If the existence and survival of a colony is a balance between foraging and fighting efforts, these conditions may be supportive for the establishment of new colonies and thus mutually limiting the expansion of each. If, on the other hand resources are more limited the establishment of new colonies become more difficult and may be prohibited by the already existing colonies. Since all population show isolation by distance, indicating reproduction by colony splitting, supercolonies may be formed in uncompetitive areas, but limited to polydomy in highly competitive area. The ant Cataglyphis niger thus merits the statement of “being at the brink of supercoloniality.”
Authors and Contributors
Maya Saar conducted the field studies, including the behavioral assays and the chemical analyses. Laurianne Leniaud conducted the molecular population genetic studies. Abraham Hefetz and Serge Aron conceived the idea and supervised the work. All authors contributed to the writing of the manuscript.
Conflict of Interest Statement
The authors declare that the research was conducted in the absence of any commercial or financial relationships that could be construed as a potential conflict of interest.
Acknowledgments
We thank Tovit Simon (TAU) and L. Grumiau (ULB) for technical assistance. This work was supported by grants from Tel Aviv University internal grant (#2191) (Abraham Hefetz), the Belgian FRS-FNRS (Laurianne Leniaud and Serge Aron) and Action de Recherché Concertée Grant N°2010-2015#5 (Serge Aron)
References
Amor, F., Ortega, P., Jowers, M. J., Cerdá, X., Billen, J., Lenoir, A., et al. (2011). The evolution of worker–queen polymorphism in Cataglyphis ants: interplay between individual- and colony-level selections. Behav. Ecol. Sociobiol. 65, 1473–1482. doi: 10.1007/s00265-011-1157-7
Blight, O., Berville, L., Vogel, V., Hefetz, A., Renucci, M., Orgeas, J., et al. (2012). Variation in the level of aggression, chemical, and genetic distance among three supercolonies of the Argentine ant in Europe. Mol. Ecol. 21, 4106–4121. doi: 10.1111/j.1365-294X.2012.05668.x
Boomsma, J. J. (2009). Lifetime monogamy and the evolution of eusociality. Philos. Trans. R. Soc. Lond. B Biol. Sci. 364, 3191–3207. doi: 10.1098/rstb.2009.0101
Boomsma, J. J., Kronauer, D. J. C., and Pedersen, J. S. (2009). “The evolution of social insect mating systems,” in Organization of Insect Societies: From Genome to Sociocomplexity, eds J. Gadau and J. Fewell (Cambridge, MA: Harvard University Press), 3–25.
Boulay, R., Cerdá, X., Simon, T., Roldan, M., and Hefetz, A. (2007). Intraspecific competition in the ant Camponotus cruentatus: should we expect the “dear enemy” effect? Anim. Behav. 74, 985–993. doi: 10.1016/j.anbehav.2007.02.013
Bourke, A. F. G., and Franks, N. R. (eds.). (1995). Social Evolution in Ants. Princeton, NJ: Princeton University Press.
Brandt, M., Van Wilgenburg, E., Sulc, R., Shea, K. J., and Tsutsui, N. D. (2009a). The scent of supercolonies: the discovery, synthesis and behavioural verification of ant colony recognition cues. BMC Biol. 7:71. doi: 10.1186/1741-7007-7-71
Brandt, M., Van Wilgenburg, E., and Tsutsui, N. D. (2009b). Global-scale analyses of chemical ecology and population genetics in the invasive Argentine ant. Mol. Ecol. 18, 997–1005. doi: 10.1111/j.1365-294X.2008.04056.x
Buczkowski, G. (2010). Extreme life history plasticity and the evolution of invasive characteristics in a native ant. Biol. Invasions 12, 3343–3349. doi: 10.1007/s10530-010-9727-6
Buczkowski, G., and Bennett, G. (2008). Seasonal polydomy in a polygynous supercolony of the odorous house ant, Tapinoma sessile. Ecol. Entomol. 33, 780–788. doi: 10.1111/j.1365-2311.2008.01034.x
Chapuisat, M., Bocherens, S., and Rosset, H. (2004). Variable queen number in ant colonies: no impact on queen turnover, inbreeding, and population genetic differentiation in the ant Formica selysi. Evolution 58, 1064–1072. doi: 10.1111/j.0014-3820.2004.tb00440.x
Chéron, B., Cronin, A. L., Doums, C., Fédérici, P., Haussy, C., Tirard, C., et al. (2011). Unequal resource allocation among colonies produced by fission in the ant Cataglyphis cursor. Ecology 92, 1448–1458. doi: 10.1890/10-2347.1
Clark, J. P., and Evans, F. C. (1954). Distance to nearest neighbor as a measure of spatial relationships in populations. Ecology, 445–453. doi: 10.2307/1931034
Cronin, A. L., Molet, M., Doums, C., Monnin, T., and Peeters, C. (2013). Recurrent evolution of dependent colony foundation across eusocial insects. Annu. Rev. Entomol. 58, 37–55. doi: 10.1146/annurev-ento-120811-153643
Crozier, R. H., and Pamilo, P. (1996). Evolution of Social Insect Colonies: Sex Allocation and Kin Selection. Oxford: Oxford University Press.
Dahbi, A., Cerdá, X., Hefetz, A., and Lenoir, A. (1997). Adult transport in the ant Cataglyphis iberica: a means to maintain a uniform colonial odour in a species with multiple nests. Physiol. Entomol. 22, 13–19. doi: 10.1111/j.1365-3032.1997.tb01135.x
Darras, H., Leniaud, L., and Aron, S. (2014). Large-scale distribution of hybridogenetic lineages in a Spanish desert ant. Proc. Biol. Sci. 281:20132396. doi: 10.1098/rspb.2013.2396
Debout, G., Schatz, B., Elias, M., and Mckey, D. (2007). Polydomy in ants: what we know, what we think we know, and what remains to be done. Biol. J. Linn. Soc. 90, 319–348. doi: 10.1111/j.1095-8312.2007.00728.x
Elias, M., Rosengren, R., and Sundström, L. (2005). Seasonal polydomy and unicoloniality in a polygynous population of the red wood ant Formica truncorum. Behav. Ecol. Sociobiol. 57, 339–349. doi: 10.1007/s00265-004-0864-8
Errard, C., Delabie, J., Jourdan, H., and Hefetz, A. (2005). Intercontinental chemical variation in the invasive ant Wasmannia Auropunctata (Roger) (Hymenoptera Formicidae): a key to the invasive success of a tramp species. Naturwissenschaften 92, 319–323. doi: 10.1007/s00114-005-0628-y
Eyer, P. A., Freyer, J., and Aron, S. (2012). Genetic polyethism in the polyandrous desert ant Cataglyphis cursor. Behav. Ecol. 24, 144–151. doi: 10.1093/beheco/ars146
Eyer, P. A., Leniaud, L., Darras, H., and Aron, S. (2013). Hybridogenesis through thelytokous parthenogenesis in two Cataglyphis desert ants. Mol. Ecol. 22, 947–955. doi: 10.1111/mec.12141
Foucaud, J., Orivel, J., Loiseau, A., Delabie, J. H. C., Jourdan, H., Konghouleux, D., et al. (2010). Worldwide invasion by the little fire ant: routes of introduction and eco-evolutionary pathways. Evol. Appl. 3, 363–374. doi: 10.1111/j.1752-4571.2010.00119.x
Fournier, D., Biseau, J. C., and Aron, S. (2009). Genetics, behaviour and chemical recognition of the invading ant Pheidole megacephala. Mol. Ecol. 18, 186–199. doi: 10.1111/j.1365-294X.2008.04018.x
Giraud, T., Pedersen, J. S., and Keller, L. (2002). Evolution of supercolonies: the Argentine ants of Southern Europe. Proc. Natl. Acad. Sci. U.S.A. 99, 6075–6079. doi: 10.1073/pnas.092694199
Goudet, J. (1995). FSTAT (Version 1.2): a computer program to calculate F-statistics. J. Hered. 86, 485–486.
Hardy, O. J., Pearcy, M., and Aron, S. (2008). Small-scale spatial genetic structure in an ant species with sex-biased dispersal. Biol. J. Linn. Soc. 93, 465–473. doi: 10.1111/j.1095-8312.2007.00898.x
Hefetz, A. (2007). The evolution of hydrocarbon pheromone parsimony in ants (Hymenoptera: Formicidae) – interplay of colony odor uniformity and odor idiosyncrasy. A review. Myrmecol. News 10, 59–68.
Helantera, H., Strassmann, J. E., Carrillo, J., and Queller, D. C. (2009). Unicolonial ants: where do they come from, what are they and where are they going? Trends Ecol. Evol. 24, 341–349. doi: 10.1016/j.tree.2009.01.013
Higashi, S., and Yamauchi, K. (1979). Influence of a supercolonial ant Formica (Formica) yessensis Forel on the distribution of other ants in Ishikari coast. Jap. J. Ecol. 29, 257–264.
Hölldobler, B., and Wilson, E. (1990). The Ants. Cambridge, MA: Harvard University Press. doi: 10.1007/978-3-662-10306-7
Holway, D. A., Lach, L., Suarez, A. V., Tsutsui, N. D., and Case, T. J. (2002). The causes and consequences of ant invasions. Annu. Rev. Ecol. Syst. 33, 181–233. doi: 10.1146/annurev.ecolsys.33.010802.150444
Holzer, B., Chapuisat, M., Kremer, N., Finet, C., and Keller, L. (2006). Unicoloniality, recognition and genetic differentiation in a native Formica ant. J. Evol. Biol. 19, 2031–2039. doi: 10.1111/j.1420-9101.2006.01133.x
Hughes, W. O. H., Oldroyd, B. P., Beekman, M., and Ratnieks, F. L. W. (2008a). Ancestral monogamy shows kin selection is key to the evolution of eusociality. Science 320, 1213–1216. doi: 10.1126/science.1156108
Hughes, W. O. H., Ratnieks, F. L. W., and Oldroyd, B. P. (2008b). Multiple paternity or multiple queens: two routes to greater intracolonial genetic diversity in the eusocial Hymenoptera. J. Evol. Biol. 21, 1090–1095. doi: 10.1111/j.1420-9101.2008.01532.x
Keller, L. (1995). Social life: the paradox of multiple-queen colonies. Trends Ecol. Evol. 10, 355–360. doi: 10.1016/s0169-5347(00)89133-8.
Kidokoro-Kobayashi, M., Iwakura, M., Fujiwara-Tsujii, N., Fujiwara, S., Sakura, M., Sakamoto, H., et al. (2012). Chemical discrimination and aggressiveness via cuticular hydrocarbons in a supercolony-forming ant, Formica yessensis. PLoS ONE 7:e46840. doi: 10.1371/journal.pone.0046840
Knaden, M., and Wehner, R. (2003). Nest defense and conspecific enemy recognition in the desert ant Cataglyphis Fortis. J. Insect Behav. 16, 717–730. doi: 10.1023/B:JOIR.0000007706.38674.73
Lahav, S., Soroker, V., Hefetz, A., and Vander Meer, R. K. (1999). Direct behavioral evidence for hydrocarbons as ant recognition discriminators. Naturwissenschaften 86, 246–249. doi: 10.1007/s001140050609
Leniaud, L., Darras, H., Boulay, R., and Aron, S. (2012). Social hybridogenesis in the clonal ant Cataglyphis hispanica. Curr. Biol. 22, 1188–1193. doi: 10.1016/j.cub.2012.04.060
Leniaud, L., Hefetz, A., Grumiau, L., and Aron, S. (2011). Multiple mating and supercoloniality in Cataglyphis desert ants Biol. J. Linn. Soc. 104, 866–876. doi: 10.1111/j.1095-8312.2011.01772.x
Leniaud, L., Pichon, A., Uva, P., and Bagnères, A.-G. (2009). Unicoloniality in Reticulitermes urbis: a novel feature in a potentially invasive termite species. Bull. Entomol. Res. 99, 1–10. doi: 10.1017/S0007485308006032
Lenoir, A., Aron, S., Cerdá, X., and Hefetz, A. (2009). Cataglyphis desert ants: a good model for evolutionary biology in Darwin's anniversary year—A review. Isr. J. Entomol. 39, 1–32.
Lenoir, A., Querard, L., Pondicq, N., and Berton, F. (1988). Reproduction and dispersal in the Ant Cataglyphis Cursor (Hymenoptera, Formicidae). Psyche 95, 21–44. doi: 10.1155/1988/54685
Moffett, M. W. (2012). Supercolonies of billions in an invasive ant: what is a society? Behav. Ecol. 23, 925–933. doi: 10.1093/beheco/ars043
Newey, P. S., Robson, S. K. A., and Crozier, R. H. (2010). Weaver ants Oecophylla smaragdina encounter nasty neighbors rather than dear enemies. Ecology 91, 2366–2372. doi: 10.1890/09-0561.1
Pearcy, M., Aron, S., Doums, C., and Keller, L. (2004a). Conditional use of sex and parthenogenesis for worker and queen production in ants. Science 306, 1780–1783. doi: 10.1126/science.1105453
Pearcy, M., Clemencet, J., Chameron, S., Aron, S., and Doums, C. (2004b). Characterization of nuclear DNA microsatellite markers in the ant Cataglyphis cursor. Mol. Ecol. Notes 4, 642–644. doi: 10.1111/j.1471-8286.2004.00759.x
Pearcy, M., Timmermans, I., Allard, D., and Aron, S. (2009). Multiple mating in the ant Cataglyphis cursor: testing the sperm limitation and the diploid male load hypotheses. Insectes Soc. 56, 94–102. doi: 10.1007/s00040-008-1043-0
Pedersen, J. S., Krieger, M. J. B., Vogel, V., Giraud, T., and Keller, L. (2006). Native supercolonies of unrelated individuals in the invasive Argentine ant. Evolution 60, 782–791. doi: 10.1554/05-365.1
Peeters, C., and Molet, M. (2010). “Colonial reproduction and life histories,” in Ant Ecology, eds L. Lach, C. Parr, and K. Abbott. (Oxford: Oxford University Press), 402.
Purcell, J., and Chapuisat, M. (2013). Bidirectional shifts in colony queen number in a socially polymorphic ant population. Evolution 67, 1169–1180. doi: 10.1111/evo.12010
Rosengren, R., Cherix, D., and Pamilo, P. (1985). Insular ecology of the red wood ant Formica truncorum Fabr.. I. Polydomous nesting, population size and foraging. Bull. Soc. Entomol. Suisse 58, 147–175.
Ross, K. G. (2001). Molecular ecology of social behaviour: analyses of breeding systems and genetic structure. Mol. Ecol. 10, 265–284. doi: 10.1046/j.1365-294x.2001.01191.x
Rousset, F. (2008). genepop,'007: a complete re-implementation of the genepop software for Windows and Linux. Mol. Ecol. Resour. 8, 103–106. doi: 10.1111/j.1471-8286.2007.01931.x
Roux, O., Rossi, V., Céréghino, R., Compin, A., Martin, J.-M., and Dejean, A. (2013). How to coexist with fire ants: the roles of behaviour and cuticular compounds. Behav. Processes 98, 51–57. doi: 10.1016/j.beproc.2013.04.014
Sambrook, J., and Russell, D. (2001). Molecular Cloning: a Laboratory Manual. New York, NY: Cold Spring Harbor Laboratory Press.
Sanada-Morimura, S., Minai, M., Yokoyama, M., Hirota, T., Satoh, T., and Obara, Y. (2003). Encounter-induced hostility to neighbors in the ant Pristomyrmex Pungens. Behav. Ecol. 14, 713–718. doi: 10.1093/beheco/arg057
Slatkin, M. (1993). Isolation by distance in equilibrium and nonequilibrium populations. Evolution 47, 264–279. doi: 10.2307/2410134
Soroker, V., Hefetz, A., Cojocaru, M., Billen, J., Franke, S., and Francke, W. (1995). Structural and chemical ontogeny of the postpharyngeal gland in the desert ant Cataglyphis niger. Physiol. Entomol. 20, 323–329. doi: 10.1111/j.1365-3032.1995.tb00822.x
Soroker, V., Vienne, C., Hefetz, A., and Nowbahari, E. (1994). The postpharyngeal gland as a “gestalt” organ for nestmate recognition in the ant Cataglyphis niger. Naturwissenschaften 81, 510–513.
Suarez, A. V., Holway, D. A., and Tsutsui, N. D. (2008). Genetics and behavior of a colonizing species: the invasive Argentine ant. Am. Nat. 172, S72–S84. doi: 10.1086/588638
Sundstrom, L., Seppa, P., and Pamilo, P. (2005). Genetic population structure and dispersal patterns in Formica ants - a review. Ann. Zool. Fenn. 42, 163–177.
Thomas, M. L., Payne-Makrisa, C. M., Suarez, A. V., Tsutsui, N. D., and Holway, D. A. (2006). When supercolonies collide: territorial aggression in an invasive and unicolonial social insect. Mol. Ecol. 15, 4303–4315. doi: 10.1111/j.1365-294X.2006.03038.x
Thomas, M. L., Payne-Makrisâ, C. M., Suarez, A. V., Tsutsui, N. D., and Holway, D. A. (2007). Contact between supercolonies elevates aggression in Argentine ants. Insectes Soc. 54, 225–233. doi: 10.1007/s00040-007-0935-8
Timmermans, I., Grumiau, L., Hefetz, A., and Aron, S. (2010). Mating system and population structure in the desert ant Cataglyphis livida. Insectes Soc. 57, 39–46. doi: 10.1007/s00040-009-0048-7
Timmermans, I., Hefetz, A., Fournier, D., and Aron, S. (2008). Population genetic structure, worker reproduction and thelytokous parthenogenesis in the desert ant Cataglyphis sabulosa. Heredity 101, 490–498. doi: 10.1038/hdy.2008.72
Van Oosterhout, C., Hutchinson, W., Wills, D., and Shipley, P. (2004). MICRO-CHECKER: software for identifying and correcting genotyping errors in microsatellite data. Mol. Ecol. Notes 4, 535–538. doi: 10.1111/j.1471-8286.2004.00684.x
Vonshak, M., Dayan, T., Foucaud, J., Estoup, A., and Hefetz, A. (2009). The interplay between genetic and environmental effects on colony insularity in the clonal invasive little fire ant Wasmannia auropunctata. Behav. Ecol. Sociobiol. 63, 1667–1677. doi: 10.1007/s00265-009-0775-9
Weir, B. S., and Cockerham, C. C. (1984). Estimating f-statistics for the analysis of population-structure. Evolution 38, 1358–1370. doi: 10.2307/2408641
Keywords: Cataglyphis, polydomy, supercoloniality, nestmate recognition, population genetic structure, cuticular hydrocarbons, “nasty neighbor effect”
Citation: Saar M, Leniaud L, Aron S and Hefetz A (2014) At the brink of supercoloniality: genetic, behavioral, and chemical assessments of population structure of the desert ant Cataglyphis niger. Front. Ecol. Evol. 2:13. doi: 10.3389/fevo.2014.00013
Received: 01 February 2014; Accepted: 09 April 2014;
Published online: 01 May 2014.
Edited by:
Patrizia D'Ettorre, University Paris 13 - Sorbonne Paris Cité, FranceReviewed by:
Jelle Stijn Van Zweden, KU Leuven, BelgiumClaudie Doums, Ecole Pratique des Hautes Etudes, France
Copyright © 2014 Saar, Leniaud, Aron and Hefetz. This is an open-access article distributed under the terms of the Creative Commons Attribution License (CC BY). The use, distribution or reproduction in other forums is permitted, provided the original author(s) or licensor are credited and that the original publication in this journal is cited, in accordance with accepted academic practice. No use, distribution or reproduction is permitted which does not comply with these terms.
*Correspondence: Abraham Hefetz, Department of Zoology, George S. Wise Faculty of Life Sciences, Tel Aviv University, Tel Aviv 69978, Israel e-mail:aGVmZXR6QHBvc3QudGF1LmFjLmls
† These authors have contributed equally to this work.