- 1Division of Chemical Ecology, Department of Plant Protection Biology, Swedish University of Agricultural Sciences, Alnarp, Sweden
- 2INRA, Institute of Ecology and Environmental Sciences iEES-Paris, Versailles, UMR 1392, Versailles, France
- 3Department of Evolutionary Neuroethology, Max Planck Institute for Chemical Ecology, Jena, Germany
- 4Institute of Physiology, University of Hohenheim, Stuttgart, Germany
Pheromone communication relies on highly specific signals sent and received between members of the same species. However, how pheromone preference is determined in moth olfactory circuits remains unknown. Here we describe a potential mechanism that generates preference differences in Ostrinia nubilalis. In Ostrinia nubilalis it was found that a single locus causes strain-specific, diametrically opposed preferences for a 2-component pheromone blend. Previously we found that pheromone preference was correlated with strain and hybrid-specific relative antennal response to both pheromone components. Here we detail the underlying mechanism of this differential response, through chemotopical mapping of the pheromone detection circuit in the antenna. We found that both strains and their hybrids have swapped the neuronal identity of the pheromone-sensitive neurons co-housed within a single sensillum. Furthermore, neurons that mediate behavioral antagonism surprisingly co-express up to five pheromone receptors, mirroring the concordantly broad tuning to heterospecific pheromones. Co-expression appears evolutionarily advantageous as it prevents cross attraction to a range of heterospecific signals, while keeping the pheromone detection system to its simplest tripartite setup.
Introduction
Evolution of signals for species recognition, pheromones, often precedes premating isolation and can lead to gene flow barriers and subsequent speciation (Mayr, 1963; Miller and Svensson, 2014). Although under stabilizing selection (Lofstedt, 1993), moth sex pheromones are diverse, which implies there are strong evolutionary forces acting on species-specific pheromone signals (Cardé and Baker, 1984; McElfresh and Millar, 2001; Groot et al., 2006; Kárpáti et al., 2013). Whereas males “track” these evolving signals (Phelan, 1997), the proximate mechanisms that lead to changes in pheromone preference are poorly understood. Moth sex pheromone signaling thus offers excellent opportunities to concurrently study olfactory coding, evolution of species recognition and speciation (De Bruyne and Baker, 2008).
The moth Ostrinia nubilalis Hübner (Lepidoptera: Crambidae) is a particularly attractive model for studies on the evolutionary dynamics of pheromone signals. A simple binary blend consisting of Z11-14:OAc (Z11) and E11-14:OAc (E11) is used as the sex pheromone (Cardé et al., 1975), whereas Z9-14:OAc (Z9) is a behavioral antagonist (disrupting orientation toward the pheromone). More importantly, the species exhibits a naturally occurring dimorphism (Klun and Robinson, 1971; Klun et al., 1973; Carde et al., 1978; Anglade and Stockel, 1984; Malausa et al., 2005), with two strains that produce and prefer opposite ratios of the binary blend: the Z-strain produces 97:3, and the E-strain 1:99 of Z11:E11 (Anglade and Stockel, 1984). Hybrid moths are intermediate in both pheromone production and preference. The two pheromone components are detected by separate antennal sensory neurons. These sensory neuron types are commonly co-localized in the same sensillum, or more rarely, expressed singly in a separate sensillum type (Hansson et al., 1987; Roelofs et al., 1987; Hansson et al., 1994). The simplicity of the circuitry and robustness of the behavioral response permit dissecting signal preference in this species down to its neural and molecular core.
Recent studies have identified the gene underlying the dimorphism in female pheromone production (Lassance et al., 2010, 2013). The male response preference is mediated through a single sex-linked locus (Dopman et al., 2004). This is mirrored by sex-linked volumetric differences of brain regions targeted by these pheromone-induced signals (Kárpáti et al., 2008, 2010), and the relative sensitivity of the entire antennae to both isomers (Kárpáti et al., 2010). Apparently, a factor at the interface between the antennae and the antennal lobes mediates the shift in pheromone preference in males.
Until now, the mechanism(s) regulating differences in glomerular size in this species has been unknown, and it is not known how a suite of seven identified pheromone receptors (PRs) (Miura et al., 2010; Wanner et al., 2010) together account for the detection of two pheromone compounds and one behavioral antagonist. In this paper, we tested if the volumetric differences between MGC glomeruli is due to differences in number of neurons, or due to differences in the diameter of OSNs that respond to the two pheromone components. To that end we elucidated the expression patterns of all PRs, and resolve discrepancy in literature regarding the morphology of pheromone sensillum types in the antenna (Hansson et al., 1994) and the relative spike amplitude of the olfactory sensory neurons (OSNs) (Hansson et al., 1994; Cosse et al., 1995; Olsson et al., 2010) within this sensillum. In addition, we tested whether the large number of different PRs are all expressed or not, whether they are expressed in both strains, and whether the pattern of expression differed between the strains. We thus links PR expression to the sensory physiology of O. nubilalis OSNs. We propose a model to explain how the pheromone-sensing circuitry in O. nubilalis has kept its simplest tripartite organization, in spite of the diverse heterospecific signaling to which it has been subjected (Gemeno et al., 2006) and how pheromone preference is affected by both peripheral and central neuroanatomical and physiological modifications.
Materials and Methods
Insects
Laboratory colonies of European Corn Borer Z- and E-strains were used for the purpose of this study. The Z-strain colony derived from cornfield-collected adults in Kéty town, county of Tolna, Hungary in 2004. The E-strain colony was established from larvae collected from maize stems collected by Smiljana Tomse from the Agriculture and Forestry Institute, Novo Mesto, Slovenia. The purity of the strains was monitored by gas chromatographic analysis of female pheromone production (protocol as in Kárpáti et al., 2007). F1 hybrids were produced by crossing Z females with E males (ZE hybrid) and E females with Z males (EZ hybrid). Both strains and hybrids were reared on a semi-artificial diet (Mani et al., 1978) until pupation. Adults were fed a 5% honey water solution throughout adult life. All animals were kept at 25°C, relative humidity 70% and under Light: Dark = 18 h: 6 h photoperiod. The day of emergence was considered as day 0.
Tissue Collection, RNA Extraction and cDNA Synthesis
Antennae were dissected from cold-anesthetized animals and were immediately frozen at liquid nitrogen temperature and stored at −80°C till RNA isolation. Total RNAs were isolated from pools of 50 antennae of male or female O. nubilalis (100 and 150 μg respectively) of each strain separately using RNeasy® Mini Kit (Qiagen, Hilden, Germany). First-strand cDNAs were synthesized with SuperScript III kit (Invitrogen, Carlsbad, CA, USA).
Identification of Receptor Sequences
To find genes encoding putative pheromone receptors in O. nubilalis, two antennal subtractive cDNA libraries, one substractive for male and one for female antennae, were screened for sequences of olfactory receptor genes that show a specific or predominant expression in male antennae of O. nubilalis (prepared by Evrogen JSC, Moscow, Russia). An additional male antennal cDNA library in phage lambda UniZAP XR was prepared by Lofstrand Labs Ltd (Gaithersburg, MD, USA).
Screening of the male antennal cDNA library and subcloning of cDNAs from positive clones were performed as described earlier (Krieger et al., 2002) and following the recommendation of the lambda UniZAP XR manual (Stratagene, La Jolla, USA).
Beforehand, partial sequences of different OnubORs were amplified from male antenna by RT-PCR using OR-specific primers. Digoxigenin (DIG)-labeled DNA probes for library screening were generated from plasmids carrying the partial OR-sequences using standard PCR with specific primer pairs and the PCR DIG labeling mix (Roche, Mannheim, Germany). Hybridizations to phage DNA immobilized on Hybond-N+ nylon transfer membranes (Amersham Biosciences, Glattbrugg, Switzerland) were done at 30°C in hybridization solution containing the DIG-labeled OR-probes and 30% formamide. Posthybridization washes were at 50 or 60°C in 0.1X SSC, 0.1% SDS for three times 20 min each. Identified cDNAs were sequenced using vector and cDNA derived primers on an ABI310 system employing the BigDye Terminator Cycle Sequencing Kit (Applied Biosystems, Darmstadt, Germany) or using custom DNA sequence services.
In addition, these OR sequences and cDNAs identified by library screening were used to perform BLAST analysis of other moth olfactory receptor sequences already published at NCBI as well as O. nubilalis OR sequences already published. This led to the identification and comparison of the transcripts we found with already published transcript fragments of O. nubilalis ORs (for naming clarifications see Table S1).
Sequence Analysis
The nucleotide and amino acid sequences were aligned and analyzed using Sequencer 4.7 (Arbor, 2006) and the amino acid sequences using BioEdit (Hall, 1999). Pair wise amino acid comparisons were performed using LALIGN (Huang and Miller, 1991) and the phylogenetic tree was reconstructed through a Neighbor Joining analysis as implemented in Mega5 (Tamura et al., 2011). Support values for MP trees were estimated with 1000 bootstrap replicates.
Quantitative Real-Time PCR
Tissue collection, RNA extraction, cDNA synthesis, and gene expression profiling were performed separately on four sets of male and four sets of female individuals of each strain that corresponded to four different biological replicates per category. Each of these sets contained antennae of 50, day 4 adults and was collected during the second hour of the scotophase. Extreme care was taken in order to pool together antennae coming from adults of exactly the same age and during the exact same time-period (2 h into scotophase).
Total RNAs were purified from each set using the RNeasy® Micro kit (Qiagen). To eliminate possible contamination by genomic DNA, a DNAse treatment was performed during the extraction procedure. Single-stranded cDNA synthesis was performed for each sample from 1 μg of total RNAs with 200 U of M-MLV reverse transcriptase, using the Advantage® RT-for-PCR kit (Clontech, Mountain View, CA, USA) according to the manufacturer's instructions. The control used was the ribosomal protein-encoding gene, rpL8 (Merlin et al., 2007). Gene-specific primers for O. nubilalis rpL8 and ORs were designed, using Primer 3 software (Rozen and Skaletsky, 2000), to amplify 100-200-bp fragments (Table S2). All qPCR reactions were performed using IQ SYBR Green Supermix 1x (Bio-Rad, Hercules, CA, USA) in a total volume of 12 μL and in the presence of 3 μL of cDNA (or water for the negative control or RNA for controlling for the absence of genomic DNA) and 200 nM of each primer. cDNA amplifications were performed in a Bio-Rad CFX96 System and the following experimental run protocol: denaturation program (95°C for 3 min), amplification and quantification program repeated 40 times (95°C for 10 s, 57°C for 30 s with a single fluorescence measurement, 72°C for 20 s), and a melting curve ramp (65.0–95.0°C: Increment 0.5°C/5 s) to confirm that each reaction did not produce nonspecific amplification. For each gene of interest a standard curve was generated from 10-fold dilution series, from 1/4 to 1/40000, of a cDNA pool, which served evaluating primer efficiency [E = 10(−1/slope)] for relative quantification of the same gene in all other cDNA samples. All experiments included water-template control and a RNA control sample, and all reactions were performed in two technical replicates. After verifying that the amplification efficiencies of both the target and control gene were similar, expression levels were calculated relatively to the expression of the rpL8 control gene and expressed as the ratio = E(ΔCT OnubOR)OnubOR/E(ΔCT rpL8)rpL8 (Pfaffl, 2001). In the analysis of the relative fold change, the male experimental mean value of the pure strain that gave the higher mean expression value was used as the calibrator value. Wilcoxon's test (Wilcoxon, 1945; Mann and Whitney, 1947) was used to statistically test the mean value differences between taxa.
Whole Mount In Situ Hybridization
Two probes, one biotin-labeled and one DIG-labeled antisense riboprobes, for each of the OnubOR1—OnubOR8 were generated using a T3/T7 RNA transcription system (Roche) and linearized recombinant Bluescript plasmids following recommended protocols. Two color double in situ hybridization with two different antisense RNA probes (DIG- or biotin-labeled probes), as well as visualization of hybridization were performed as reported previously (Krieger et al., 2002). DIG-labeled probes were detected by an anti-DIG AP-conjugated antibody in combination with HNPP/Fast Red (Fluorescent detection Set; Roche); for biotin-labeled probes the TSA kit (Perkin Elmer, Boston, MA, USA), including an antibiotin–streptavidin–horseradish peroxidase conjugate and FITC-tyramides as substrate was used.
Antennae of 1- to 2-day-old male O. nubilalis moths were dissected by first cutting off the tips. The remaining antennal stem was further cut into three equally long pieces. This permitted to obtain fragments of approximately 20 segments each that were easily distinguishable as the tip, the base and the middle fragment. All incubations and washes were made in a volume of 0.5 mL tubes with slow rotation on a small table rotor. The protocol used was adapted from the one described in Krieger et al. (2002). Antennal fragments were fixed in 4% paraformaldehyde in 0.1 M NaCO3, pH 9.5 for 24 h at 4°C (PF1) followed by washes at room temperature for 1 min in phosphate-buffered saline (PBS: 0.85 % NaCl, 1.4 mM KH2PO4, 8 mM Na2HPO4, pH 7.1), 10 min in 0.2 M HCl and 2 min in PBS with 1% Triton X-100. In our modified protocol, we performed a subsequent incubation for 30 min in 2 × SSC at 70°C, followed by a wash with H2O at room temperature (RT). A rinsing with proteinase K buffer (prepared according to manufacturer instructions) at RT was followed by 30 min incubation in 1 μg/ml proteinase K at 37°C, which was then washed out two times with H2O. A fixation step in PF1 for 20 min at RT and two washes with 1 × PBS for 2 min followed. The rest of the protocol continued as in Krieger et al. (2002). After hybridization, antennal fragments were analyzed on a Zeiss LSM510 Meta laser-scanning microscope (Zeiss, Oberkochen, Germany). Figures were arranged in Powerpoint (Microsoft) and Adobe Illustrator (Adobe systems, San Jose, CA, USA); images were not altered except to adjust the brightness or contrast for uniform tone within a single figure.
Single Sensillum Recordings
Sexes were separated as pupae and kept in separate plastic boxes to avoid exposing adult males to female sex pheromone. A 0- to 3-days-old male moth was retrained in a plastic micropipette tip, with its head protruding from the aperture. A tungsten wire was inserted into the abdomen as a reference electrode. The head was immobilized with dental wax (Surgident periphery wax, Heraeus Kulzer GmbH, Hanau, Germany) and one antenna was fixed on a microscope glass slide using double-sided sticky tape. An electrolytically sharpened recording electrode was inserted at the base of a sensillum under a light microscope (Olympus BX51WI) at 500X magnification using a micromanipulator (PM-10, Märzhäuser-Wetzlar Gmbh and Co Kg, Wetzlar, Germany).
Solutions of synthetic pheromone compounds were prepared volumetrically with redistilled n-hexane. N-hexane served as control stimulation. Ten μl of solution was applied on a 12.7 mm Ø filter paper disk (Schleicher and Schnell GmbH, Dassel, Germany) and the disk was inserted into a Pasteur pipette. Pipettes were prepared 1 h before experiments and ventilated for 30 s at 5 ml/s to get rid of solvent and excess pheromone. A charcoal-filtered, humidified air stream (2 L.min−1) was directed continuously over the antenna via a glass tube (20 cm length × 6 mm i.d.), positioned approximately 2 cm from the antenna. Stimuli of 0.5 s duration were delivered via a CS-55 Syntech (Kirchzarten, Germany) stimulus controller at 500 mL.min−1 into the continuous airstream. The inter-stimulus interval was at least 20 s and stimuli were presented in random order.
The recording electrode was connected to an AC/DC 10X gain probe (Syntech). When extracellular contact was established, the antenna was stimulated and the activity of the neurons before (2 s), during and after stimulation was recorded. The signal was amplified, digitized (IDAC-4 USB, Syntech) and visualized using a PC with AutoSpike 3.9 software (Syntech). Spikes were counted manually. The response of sensory neurons was expressed as the number of spikes during the stimulation period after stimulus onset minus the number of spikes before stimulus onset (which represents the spontaneous activity of the neuron). The amplitude of the first three spikes following stimulation were used for measuring the spike amplitude following stimulation with Z11, E11, or Z9 and expressed as a percentage of the largest spiking neuron. This was then analyzed using a Kruskall-Wallis test. Differences between means were analyzed with a Tukey's HSD test.
Results
PR Identification
Using homologous hybridization with labeled probes (Krieger et al., 2004; Grosse-Wilde et al., 2007; Forstner et al., 2009), and consensus-degenerate hybrid oligonucleotide primers (CODEHOP primers) (Rose et al., 2003) we identified seven receptors in our Hungarian Z-strain and Slovenian E-strain of O. nubilalis. All identified O. nubilalis candidate pheromone receptors (OnubORs) shared strong homology (≥81%) with those previously reported (Miura et al., 2010; Wanner et al., 2010) (naming follows Yasukochi et al., 2011, see Table S1 for details), with the exception of OnubOR3 (70–82% amino acid identity). OnubORs grouped into two clusters (on the phylogenetic reconstruction Figure S1), with the exception of OnubOR7 that showed a similarity of only 26.4–36.7% with the other Ostrinia receptors (Figure S2).
Interestingly, OnubOR7 was more related to receptors from other moth species such as Amyelois transitella, AtraOR3 (45.8% amino acid identity), Diaphania indica, DiaOR3 (44.9%), Antheraea polyphemus, ApolOR1 and A. pernyi, AperOR1 (43.9 and 43.7% respectively), Heliothis virescens, Hvir13 (42%), and Plutella xylostela, PxylOR1 (39.6%) (Figures S1, S2). Three of those OnubOR7 homologs have been shown to detect Z11-16:Ald (Grosse-Wilde et al., 2007; Mitsuno et al., 2008; Yasukochi et al., 2011; Xu et al., 2012; Vasquez et al., 2013), know as a behavioral antagonist in O. nubilalis (Gemeno et al., 2006; Linn et al., 2007). We identified two duplicate sequences for OnubOR7, called “a” and “b” (Yasukochi et al., 2011). Interestingly, OnubOR7b identified here had an insertion of 384bp to its non-coding 3′ end compared to a previous report (Yasukochi et al., 2011). This difference may hint at two OnubOR7b forms, resulting from alternative splicing or a gap on the BAC clone sequencing (Yasukochi et al., 2011). In our study, the two forms were not further differentiated. Receptor sequences from this study were subsequently used for in situ hybridization probes, and quantative PCR (qPCR) studies.
In Situ Hybridization
Whole antennae of the two O. nubilalis strains were hybridized with antisense RNA probes labeled with biotin and dioxigenin, under high stringency conditions, providing high-resolution spatial maps of each PR across all antennal segments. Comparisons were made between both strains and among all of the OnubORs. Representative confocal microscope scans are shown in Figure 1. Two examples of full 3D scans throughout the antennal segments is available online (https://drive.google.com/folderview?id=0B0w9Z-P0zBXOTzlLZmdQNWJxWjg&usp=sharing). OnubORs were expressed strictly at the base of sensilla trichodea, as expected for PRs.
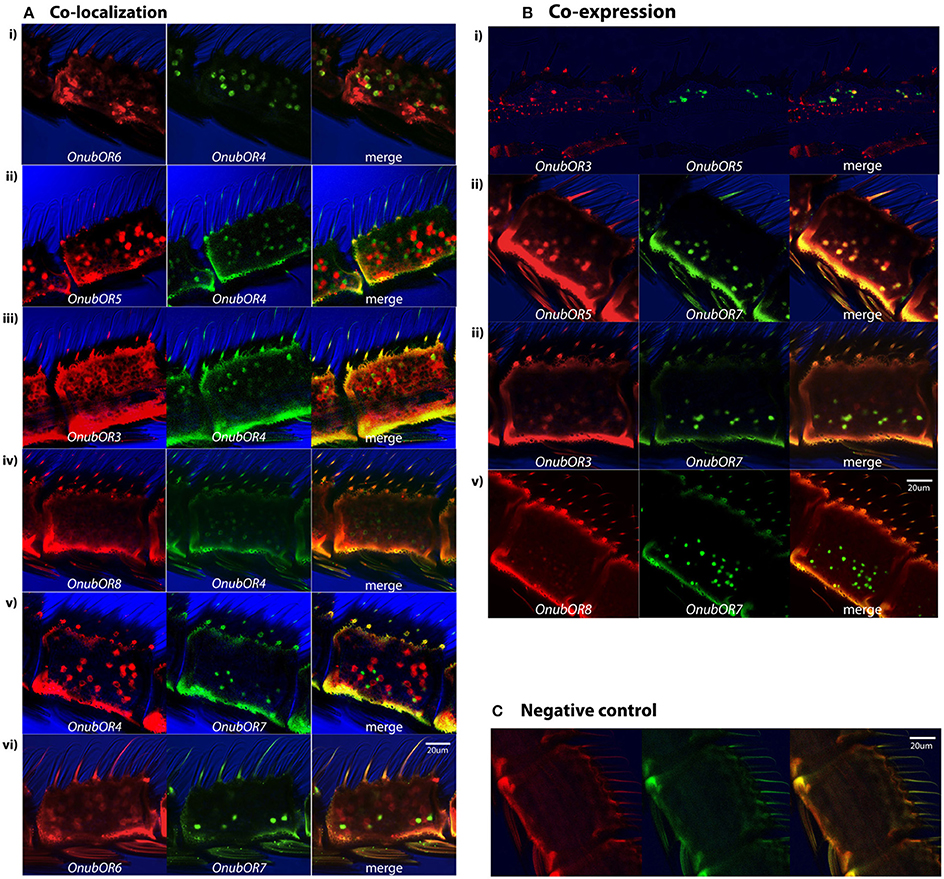
Figure 1. Whole mount expression patterns of 7 O. nubilalis' PRs in male antennae. Two labeled antisense probes were hybridized together in each assay, each containing a different OnubOR probe for comparison. (A) Examples of OnubOR co-localization in the same sensilla. Ai–Aiv full co-localization throughout each segment, and Av,Avi partial co-localization on the lateral parts of the antenna. (B) OnubOR co-expression in the same OSNs (Ai full co-expression and Aii–Aiv partial co-expression, lateral). (C) Negative control showing cuticle autofluorescence. From left to right, red: digoxigenin (DIG) labeled transcripts, green: biotin (BIO) labeled transcripts, merged: red and green labeling (yellow when overlapping). Same scale applies to all images.
Double in situ hybridizations using combinations of the seven OnubORs show that OnubOR6 and OnubOR4 never co-expressed with other OnubORs in the same OSN and that they were always co-localized in the same sensillum (in Figure 1Ai all different color cells are found in couples). OnubOR4 co-localized in the same sensillum with OnubOR5, OnubOR3, and OnubOR8 (examples in Figures 1Aii–Aiv). Therefore, OnubOR6 also co-localized with OnubOR5, OnubOR3, and OnubOR8. Interestingly, OnubOR4 and OnubOR6 co-localized with OnubOR7 only in sensilla located laterally in each antennal segment (close to the scales, Figures 1Av,Avi). Furthermore, OnubOR3 and 5 are co-expressed in each cell (antennal slices, Figure 1Bi) i.e., there is no indication that one of them is expressed anywhere in the antenna in the absence of the other. As OnubOR3 showed a low expression level, it was hard to obtain whole mount in situ hybridization with this probe. Antennal slices were more successful, probably because of better penetration of the probe in the tissue. It was equally difficult to obtain whole antennal mount FISH preparations from OnubOR8. The co-expression of OnubOR3, OnubOR5, and OnubOR8 with OnubOR7 in lateral sensilla of each antennal segment (Figures 1Bii–Biv) and the co-localization with OnubOR4 and OnubOR6 (Figure 1A), led us to conclude that the three receptors (OnubOR3, OnubOR5, and OnubOR8) were co-expressed in sensilla trichodea throughout the whole surface of the antennal segment.
In summary, all PR transcripts (except for OnubOR1) are expressed in a single sensillum trichodeum type that contains three sensory neurons (Figure 2A for a schematic overview): two neurons each expressing only a single PR type, OnubOR6 or OnubOR4, and one co-expressing an array of receptors, OnubOR3, OnubOR5, OnubOR7, and OnubOR8. The latter neuron showed a curious dichotomous OnubOR expression: this neuron co-expressed OnubOR7 in a uniquely lateral distribution. Numerically, tip segments contained approximately 20 PR-expressing sensilla and basal segments roughly 80. As OnubOR7 was only laterally expressed, each segment contained 5–10 (tip), 12–20 (middle), or around 40 (base) cells. No difference was found between the strains concerning the expression pattern and numbers of these six PRs. We only show scans of the Z strain (Figures 1A,B with the negative controls in Figure 1C).
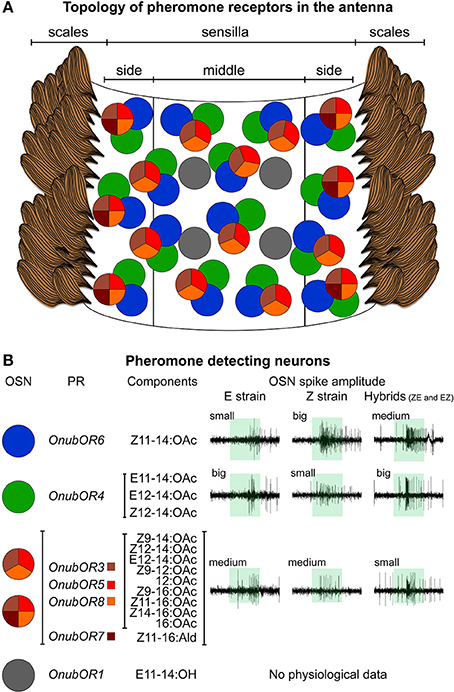
Figure 2. Schematic representation illustrating the in situ hybridization and SSR results. (A) topography of different sensilla types and OnubOR-expressing neurons in a typical O. nubilalis male antenna segment. Three of the OSNs that express OnubORs (green, blue, and red) co-localize in the same type of sensillum, whereas one OSN (OnubOR1, gray) is housed in a separate sensillum type. Two OSN sub-types are found, expressing three (medial sensilla, OnubOR7 missing) or four OnubORs (lateral sensilla, including OnubOR7). (B) Pheromone sensory neuron types, the OnubOR genes they express, compounds stimulating them and sample physiological recording traces.
OnubOR1 was sparsely expressed in separate sensilla trichodea (Figures 2A, 3), which was distributed symmetrically between the medial and the lateral part of each segment in relatively conserved positions. The strains differed in the number of OnubOR1 stained cells per segment: 2 (distal) to 4 (base) in the Z-strain, and 6 (distal) to 8 (base) in the E-strain (Figure 3, lateral views can only show half of the cells at each segment).
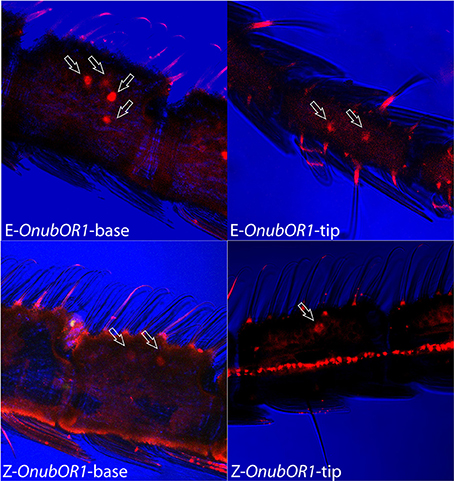
Figure 3. Z and E strain comparison of OnubOR1 transcripts' expression patterns at the tip and base of the antenna. Arrows indicate cells' position.
Quantitative RT-PCR
Antennal expression levels of all seven OnubORs were assessed by qPCR from individuals of the same age and dissected at the same time of the day (see Methods). Nearly all OnubORs were expressed only in male antennae. However, OnubOR7a and b were expressed in antennae of both sexes, albeit at a much lower level in females (Figure S3).
OnubOR6, which is sensitive to Z11 (Wanner et al., 2010), was expressed at significantly higher levels in the Z-strain than in the E-strain, whereas hybrids displayed intermediate expression levels (Figure 4A). In contrast, OnubOR4, sensitive to E11 (Leary et al., 2012), was expressed at higher levels in the E-strain than Z-strain. Hybrid expression levels of OnubOR4 were comparable to the E-strain expression levels (Figure 4A).
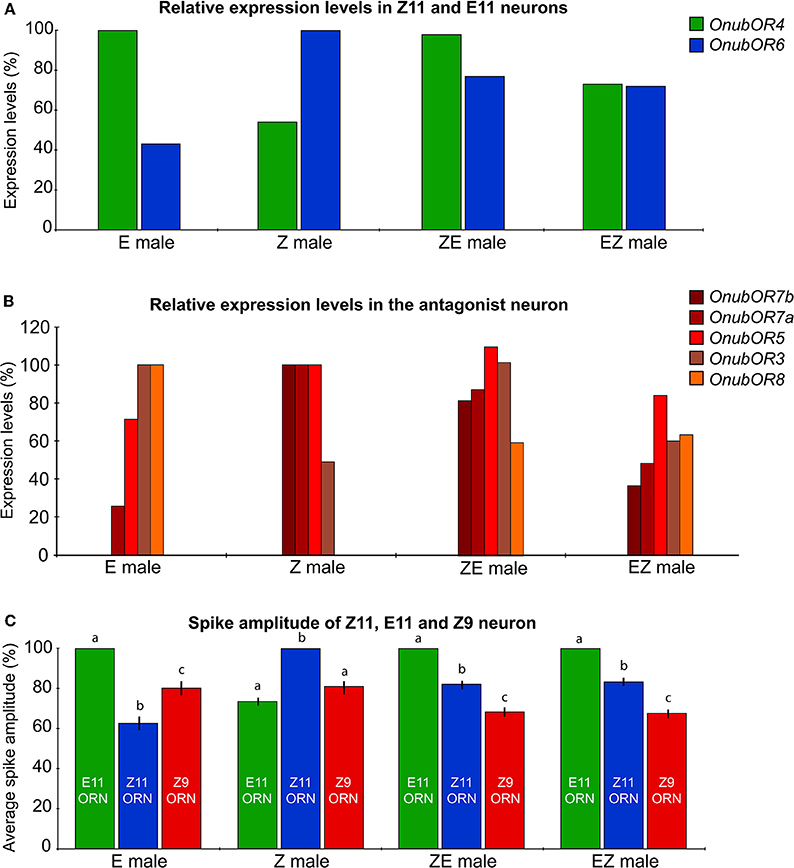
Figure 4. Comparison of pheromone receptor expression level and spike amplitudes in pheromone strains and hybrids. (A) Expression levels (%) of OnubOR4 and OnubOR6, detecting E11, and Z11, respectively, in male antenna (from left to right E-strain, Z-strain and two hybrids, male expression levels). (B) Expression levels, in antennae of male, female, Z-strain, E-strain and hybrids, of the four co-expressed pheromone receptor genes (including two duplicates for OnubOR7a and b). RpL8 was used as internal standard. All mean values within a gene were compared, as a percentage ratio, to the highest mean expression value of the pure strain male. (C) Relative spike amplitude of the three O. nubilalis OSNs responding to Z11, E11, and Z9. The response of the neuron with the largest amplitude cell was set to 100% in each strain and hybrid.
Expression levels of receptors co-expressed in the third neuron (OnubOR3, OnubOR5, OnubOR7a and b, OnubOR8) varied between strains and hybrids (Figure 4B). In general, their expression levels showed no coherent pattern between pure strains and hybrids. OnubOR7b expression was not detected in the E-strain, whereas OnubOR8 was not amplified from Z-strain antennal transcripts (tested using two primer combinations), although the OnubOR8 gene was amplified using genomic DNA. Expression levels of OnubOR1, sensitive to E11-14:OH (Miura et al., 2009), was significantly higher in the E-strain (Figure S3), which is in line with the higher number of OnubOR1-expressing sensilla per antennal segment (see above).
Single Sensillum Recordings (SSR)
In our over 400 recordings from sensilla trichodea, only one type of pheromone-sensitive sensillum was found, irrespective of strain. This sensillum housed three distinct neurons that were sensitive to E11, Z11, or Z9 (Figure 2B). However, spike amplitudes of the three co-housed neurons differed between parental strains and their hybrids (Figures 2B, 4C). Invariably, in parental strains the large spiking neuron responded to the major pheromone component (Z11 in the Z-strain, E11 in the E-strain), the small spiking neuron to the minor pheromone component (E11 in the Z-strain, Z11 in the E-strain), and the medial neuron to the behavioral antagonist (Z9). However, in both hybrids the large spiking neuron responded to E11, the medium-spiking neuron responded to Z11, whereas the smallest neuron responded to Z9 (Figures 2B, 4C). These results mirror our qPCR results (Figure 4A), i.e., the relative expression level of a given OnubOR depends on the amplitude of the neuron in which the receptor is expressed.
We verified the response breadth of the three OSNs using a panel of 13 different pheromone-type compounds. The Z11 neuron was highly specific to Z11 (Wanner et al., 2010). The E11 neuron, although highly tuned to E11 (Wanner et al., 2010; Leary et al., 2012) at low doses (Kárpáti et al., 2013), also responded, to a lesser extent, to closely related compounds (E12-14:OAc, Z12-14:OAc) (Mitsuno et al., 2008; Leary et al., 2012) at higher doses. In contrast, the neuron tuned to the behavioral antagonist Z9 was broadly tuned, an unexpected finding for a pheromone-sensitive OSN (Figure 5). Ten of thirteen compounds elicited a response from this OSN (specific spike amplitudes): Z9, Z12-14:OAc, E12-14:OAc, Z9-12:OAc, 12:OAc, Z9-16:OAc, Z11-16:OAc, Z14-16:OAc, 16:OAc and Z11-16:Ald (Figure 5). A response to these compounds was though not present in all antagonist neurons, even within the same individual (see Table S3 in electronic supplementary data). In particular, the Z11-16:Ald responses were exclusive to lateral sensilla, which co-express OnubOR7a and b (Figure 5 and Table S3). In the E-strain, which expresses low levels of OnubOR7a and no OnubOR7b, a response to Z11-16:Ald was observed only once.
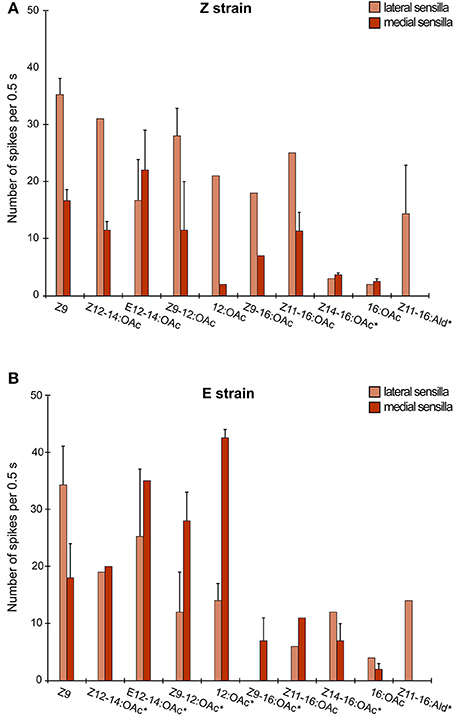
Figure 5. The response of the behavioral antagonist neuron to 10 pheromone compounds, in the Z-strain (A) and the E-strain (B). Medial (dark brown) and lateral (light brown) sensilla differed in their response. Also, the response of this neuron was mosaic: not all neurons uniformly responded to all compounds in the test panel. A percentage on each bar demarcates the fraction of the tested neurons that responded to the compound. Compounds were tested at 1 μg dose on filter paper. A * in the x-axis labels indicates that this compound was tested at 10 μg.
Discussion
Our understanding of insect olfaction has made important strides forward particularly through the identification of olfactory receptors and their ligand affinities (Sakurai et al., 2004; Nakagawa et al., 2005; Grosse-Wilde et al., 2007; Mitsuno et al., 2008). Although this has accelerated our understanding of the neurophysiology and interconnectivity of the olfactory circuit, coding features that determine behavioral preferences still remain to be deciphered to a large extent. In this study we describe the layout of the peripheral pheromone circuitry of O. nubilalis, a species with two strains exhibiting diametrically opposite pheromone preferences. Here we demonstrate that the differences in glomerular volume are not due to differences in the numerical representation of Z11 and E11-responding neurons, but instead correlate with differences in the size of sensory neurons. We furthermore demonstrate that some insect olfactory neurons can express up to 5 ORs, contrary to the current canonical precept of one OR for every OSN type. Multi-OR neurons, such as in O. nubilalis, may be at the basis of heterospecific chemical communication in moths.
First, we demonstrate using in situ hybridization and physiological recordings, that there is only one type of sensillum involved at the detection of pheromone components. This sensillum-type contains three OSNs, responding to either E11, Z11, or Z9. In contrast, previous sensory physiological studies on O. nubilalis described three different types of sensilla (e.g., Hansson et al., 1994 and references therein). Technical issues may have caused this discrepancy. The cut-tip technique used in previous studies may have affected responses of the underlying OSNs or the sensitivity of underlying neurons. In addition previous studies typically used several orders of magnitudes higher concentrations than more recent studies (Kárpáti et al., 2013), which, given the cross-sensitivity of PRs at high pheromone concentration, has likely obscured differences in spike amplitude between Z11 and E11 neurons in hybrids (Hansson et al., 1994).
As both strains and hybrids only have one pheromone sensillum type, pheromone detection in the two strains and hybrids differs only in neuronal specification within this sensillum (i.e., which of the neurons is sensitive to Z11, E11, or Z9, see Figures 2B, 4C). Previous electroantennogram (EAG) recordings made on the two strains, their hybrids and backcrosses demonstrated that the relative response of the antenna to Z11 and E11 followed a sex-linked pattern (Kárpáti et al., 2010). As we found here that only one sensillum type underlies the responses to pheromone, it follows that the difference in spike amplitude is reflected in differences of the releative EAG response. This is conceivable, as relative spike amplitude reflects differences in cell body size and axonal diameter in insect pheromone OSN, including O. nubilalis (Meng et al., 1989; Hansson et al., 1994; Kumar and Keil, 1996), and thereby the strength of the EAG response.
The same study (Kárpáti et al., 2010) further demonstrated that the volume of the glomeruli sensitive to Z11 and E11, located in the macro-glomerular complex (MGC) in the antennal lobe, correlated with the EAG response and was indeed also sex-linked. Differences in volume cannot have been caused by differences in number of sensory neurons, as we demonstrate here that the total number of Z11 and E11-type sensory neurons is identical between the strains and hybrids. The volume differences thus likely stem from the difference in size of peripheral neurons: large neurons have more synapses and thereby cause their target glomeruli to be larger (Desantis and Limwongse, 1983; Gulyas et al., 1999). It is noteworthy that in moth species examined that have two or more pheromone components and co-localized pheromone-sensitive sensory neurons, the large-spiking OSN is invariably tuned to the major pheromone component (Hansson et al., 1987, 1989; Priesner, 1988; Todd et al., 1992). Similarly, in moths in which pheromones are detected across more than one sensillum type, invariably the sensilla containing the OSNs sensitive to the major pheromone component are the most abundant (Baker et al., 2012). In the ordinary olfactory circuitry of Drosophila spp. numerical representation and preference appears to be also positively correlated (Dekker et al., 2006; Ibba et al., 2010). Finally, without exception, the largest glomerulus in the antennal lobe is tuned to the major pheromone component (see Kárpáti et al., 2010 and references therein). How these characteristics of the OSNs link to behavioral preference, and through which mechanism(s), requires further research. This connection can only be answered after identification and characterization of those neurons in higher brain centers that receive projections from the antennal lobe projection neurons and gate signals to motor output centers in the thorax.
In addition to the significance that OSN specification may have for pheromone preference, the factor(s) that causes neuronal specification to change between the strains and hybrids deserves further investigation. Our physiological data demonstrate that in the pure pheromone strains, only the large and small neurons swap identity, leaving the antagonist neuron unaffected. However, in hybrids the antagonist neuron also changes specificity. Yet, in spite of the change in neuronal specificity, the antagonist neuron retains its posterior arborization in the MGC (Kárpáti et al., 2010) (Figure 6). This, therefore, necessitates a regulatory factor that in hybrids rewires the OSNs to the correct target area in the antennal lobes. We infer that the factor that causes the shift in neuronal specificity is also involved in neuronal targeting during development. Such a hypothesis for the exchange of OSN specification has been inferred before in Bombyx mori (Fujii et al., 2011). Mutant silkworm moths with disrupted transcription factor acj6 function display OSNs, normally sensitive to bombykol in wildtype moths, that have shifted their specificity to bombykal (Fujii et al., 2011). Further research should aim to identify the sex-linked factor that regulates the specificity and targeting of pheromone-sensitive OSNs in O. nubilalis.
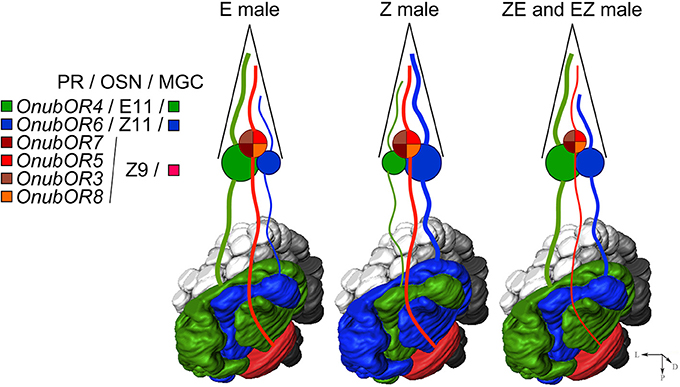
Figure 6. Hypothetical scheme of O. nubilalis pure strains and hybrids sex-pheromone circuit between the antenna and the MGC in the antennal lobe.
We also confirm the presence of seven PRs in O. nubilalis. How these PRs act together to mediate pheromone orientation and heterospecific behavioral antagonism is only partially known. OnubOR6 appeared to mediate the response to Z11 (Wanner et al., 2010), whereas OnubOR4 is sensitive to the E11 component (Leary et al., 2012). This fits with our in situ hybridization observations that both receptors are expressed singly in OSNs. OnubOR1 is sensitive to an “ancestral” pheromone compound, E11-14:OH (Miura et al., 2009), and although it may act as an antagonist receptor, its low expression may also indicate that it could also be a relict of past pheromone or antagonist communication. Of interest is the higher number of OnubOR1 expressing sensilla in the E strain. How this correlates with the evolutionary history of the E and Z-strains is unclear.
Since OnubOR4 and OnubOR6 code for pheromone receptors, this leaves the third neuron, which co-expresses a range of different receptors, to be tuned to the behavioral antagonist Z9-14:OAc. Two receptors emerge as candidates in Z9 detection i.e., OnubOR5 and OnubOR3. OnubOR8, found in the E-strain only, and OnubOR7, only in the lateral sensilla, are excluded as candidates. OnubOR5 responded mildly to Z9 when expressed in Xenopus eggs (Miura et al., 2010; Wanner et al., 2010). OnubOR5 and OnubOR8, are highly related (Miura et al., 2010) and phylogenetically cluster with OnubOR4 (E11) and OnubOR6 (Z11) receptors. Perhaps detection of 14 carbon acetates in the genus evolved through this group of receptors. The other candidate for the response to Z9 is OnubOR3, a rather divergent PR in Ostrinia. OnubOR3 is more closely related to OnubOR1, the E11-14:OH sensitive receptor (Miura et al., 2009), and this receptor responded only slightly and non-specifically to all Ostrinia pheromones (Miura et al., 2010; Wanner et al., 2010). The alternative is that all or a subset of, the receptors expressed in the antagonist OSN together convey Z9 sensitivity.
This study is the first report on co-expression of multiple PRs in moths. At least five distinct PRs (OnubOR3, 5, 7a, 7b, 8) could be demonstrated in a single neuron type, that is the highest number of ORs co-expressed in an OSN reported in an insect. Although the expression of multiple chemoreceptors is common for taste receptors in insects and mammals (Goldman et al., 2005; Behrens et al., 2007; Weiss et al., 2011), the co-expression of odorant receptors is rare. The co-expression of two different Ors has been described in D. melanogaster (Dobritsa et al., 2003; Hallem et al., 2004; Couto et al., 2005) and hypothesized in studies on H. virescens and H. subflexa for PRs in antagonist neurons (Baker et al., 2006). Functional co-expression has been reported once in D. melanogaster (Dobritsa et al., 2003; Hallem et al., 2004; Couto et al., 2005). In O. nubilalis, it seems functionally significant, as the antagonist neuron appeared to be unusually broadly tuned, compared to what has been previously reported for pheromone-sensitive OSNs. Ten out of thirteen compounds, some of which being known as behavioral antagonists (Gemeno et al., 2006; Linn et al., 2007), elicited a response. However, neurons responded generally to a subset of compounds, giving a mosaic response pattern across antagonist neurons. Such a mosaic pattern has been previously reported in bitter taste responding neurons in vertebrates that express multiple receptors, which was inferred to be caused by a competition of the various GRs (Behrens et al., 2007). How the various PRs in Ostrinia together contribute to the overall response, either through addition, through a mosaic of competitive exclusion, or possibly through heteromeric dimerization contribute to each neuron's response spectrum requires further experimentation.
Of the receptors that were co-expressed, OnubOR7 may be coding for Z11-16:Ald. We infer this from the expression pattern of OnubOR7 exclusively restricted to lateral sensilla, a pattern that coincided with that of the electrophysiological responses to Z11-16:Ald, observed uniquely in lateral sensilla. Also, OnubOR7 was the least related to any other pheromone receptor in Ostrinia spp. and grouped with AtraOR3, Hvir13 and PxylOR1 receptors, which are known to detect Z11-16Ald (Grosse-Wilde et al., 2007; Mitsuno et al., 2008; Xu et al., 2012; Vasquez et al., 2013) in phylogenetically distantly related species. This implies that the amino acids of this receptor, and seemingly its function, have been conserved over tens of millions of years, down from basal Ditrysia moth clades. The pattern of OnubOR7 expression was highly unusual. In insects, OSNs are characterized by the OR(s) they express (Chess et al., 1994; Clyne et al., 1999; Vosshall et al., 2000; Elmore and Smith, 2001), and as a rule OSNs express the same ORs across sensilla of the same type (Couto et al., 2005). O. nubilalis violates this rule by expressing OnubOR7 uniquely in OSNs of lateral sensilla of each antennal segment. The split in two functional OSNs and sensillum subtypes may reflect an ongoing evolution of this sensillum type, with the current state representing an intermediate evolutionary stage between one and two sensillum types. One would expect sensillar splits to cause glomerular splits. Accordingly, indications of a split in the antagonist glomerulus of O. nubilalis were observed (Kárpáti et al., 2010).
Olfactory receptors that are not under selection rapidly pseudogenize (Niimura and Nei, 2005; Sanchez-Gracia et al., 2009; Carraher et al., 2012). In the genus Ostrinia, however, all receptors expressed in the antagonist neuron have remained intact throughout the Ostrinia genus (Miura et al., 2010) and even within Ditrysia (OnubOr7, this study). The co-expression may reflect current pressures experienced in pheromone communication (Gemeno et al., 2006). As E11-14:OAc and Z11-14:OAc are commonly found components in pheromone blends of other species (see e.g., pherobase.org), Ostrinia spp. may rely on broad behavioral antagonism to protect itself against heterospecific mating. Be it as it may, the accumulation of multiple receptors in a single neuron confers broad antagonism and effectively enables the moth to increase its specificity, while keeping pheromone coding down to its simplest tripartite system. This may be an important evolutionary factor that overrides the otherwise canonical one-receptor-one-neuron rule. Whether antagonist neurons in moths more often “promiscuously” accept receptors needs further study.
In summary this paper identifies antennal factors that are linked to pheromone preference in moths. Since pheromone preference is genetically determined and shows little phenotypic plasticity, these factors are of prime significance in species recognition and thus evolution of pheromone blends and the speciation process.
Author Contributions
Fotini A. Koutroumpa: Conceived the project. Provided most experimental data and wrote the paper. Zsolt Kárpáti: Provided experimental data, analyzed data and commented on the manuscript. Christelle Monsempes: Helped and advised Fotini A. Koutroumpa on the qPCR experiments and commented the manuscript. Sharon R. Hill: Helped and advised on experiments. Provided comments on the manuscript. Bill S. Hansson: Provided comments on the manuscript. Emmanuelle Jacquin-Joly: Provided reagents, helped and advised Fotini A. Koutroumpa on the qPCR experiments and provided comments on the manuscript. Jürgen Krieger: Provided advice on experimental procedures, provided experimental data and comments on the manuscript. Teun Dekker: Conceived the project. Advised with experimental procedures. Carried out experiments. Wrote and commented on the manuscript.
Accession Numbers
KJ830833–KJ830840.
Conflict of Interest Statement
The authors declare that the research was conducted in the absence of any commercial or financial relationships that could be construed as a potential conflict of interest.
Acknowledgments
We thank David Heckel and Astrid Groot for valuable discussion and comments on the manuscript. We also thank Maike Forstner for her advice throughout the in situ hybridization experiments and Anneli Nordén for her participation in the first steps of the O. nubilalis OR identification. This work was funded through the Linneaus grant Insect Chemical Ecology, Ethology and Evolution IC-E3 (www.ice3.se) (Fotini A. Koutroumpa, Zsolt Kárpáti, Bill S. Hansson, Teun Dekker), FORMAS grant 2007-1491 (#220-2007-1491) to Teun Dekker, Marie Curie IEF-255193 CIG (0303E3980P), Hungarian Scientific Research Fund (OTKA PD 1041310) and János Bolyai Research Scholarship of the Hungarian Academy of Sciences to Zsolt Kárpáti. The funders had no role in study design, data collection and analysis, decision to publish, or preparation of the manuscript.
Supplementary Material
The Supplementary Material for this article can be found online at: http://www.frontiersin.org/journal/10.3389/fevo.2014.00065/abstract
Abbreviations
OSNs, Olfactory sensory neurons; PR, Pheromone receptor; OnubOR, Ostrinia nubilalis odorant receptor; SSR, Single sensillum recordings; EAG, Electroantennogram; qPCR, Quantitative PCR; DIG, Digoxigenin; Z11, Z11-14:OAc; E11, E11-14:OAc; Z9, Z9-14:OAc.
References
Arbor, A. (2006). Sequencher* version 4.7 Sequence Analysis Software. Ann Arbor, MI: Gene Codes Corporation. Available online at: http://www.genecodes.com
Anglade, P., and Stockel, J. (1984). Intraspecific sex pheromone variability in the European corn borer, Ostrinia nubilalis Hbn. (Lepidoptea, Pyralidae). Agronomie 4, 183–187. doi: 10.1051/agro:19840209
Baker, T. C., Domingue, M. J., and Myrick, A. J. (2012). Working range of stimulus flux transduction determines dendrite size and relative number of pheromone component receptor neurons in moths. Chem. Senses 37, 299–313. doi: 10.1093/chemse/bjr122
Pubmed Abstract | Pubmed Full Text | CrossRef Full Text | Google Scholar
Baker, T. C., Quero, C., Ochieng, S. A., and Vickers, N. J. (2006). Inheritance of olfactory preferences II. Olfactory receptor neuron responses from Heliothis subflexa x Hetliothis virescens hybrid male moths. Brain Behav. Evol. 68, 75–89. doi: 10.1159/000093375
Behrens, M., Foerster, S., Staehler, F., Raguse, J.-D., and Meyerhof, W. (2007). Gustatory expression pattern of the human TAS2R bitter receptor gene family reveals a heterogenous population of bitter responsive taste receptor cells. J. Neurosci. 27, 12630–12640. doi: 10.1523/JNEUROSCI.1168-07.2007
Pubmed Abstract | Pubmed Full Text | CrossRef Full Text | Google Scholar
Cardé, R. T., and Baker, T. C. (1984). “Sexual communication with pheromones,” in The Chemical Ecology of Insects, eds W. J. Bell and R. T. Cardé (London: Chapman & Hall), 355–383.
Cardé, R. T., Kochansky, J., Stimmel, J. F., Wheeler, A. G., and Roelofs, W. L. (1975). Sex-pheromone of European corn-borer (Ostrinia-nubilalis) - cis-responding and trans-responding males in Pennsylvania. Environ. Entomol. 4, 413–414.
Carde, R. T., Roelofs, W. L., Harrison, R. G., Vawter, A. T., Brussard, P. F., Mutuura, A., et al. (1978). European corn borer: Pheromone polymorphism or sibling species? Science 199, 555–556. doi: 10.1126/science.199.4328.555
Pubmed Abstract | Pubmed Full Text | CrossRef Full Text | Google Scholar
Carraher, C., Authier, A., Steinwender, B., and Newcomb, R. D. (2012). Sequence comparisons of odorant receptors among tortricid moths reveal different rates of molecular evolution among family members. PLoS ONE 7:e38391. doi: 10.1371/journal.pone.0038391
Pubmed Abstract | Pubmed Full Text | CrossRef Full Text | Google Scholar
Chess, A., Simon, I., Cedar, H., and Axel, R. (1994). Allelic inactivation regulates olfactory receptor gene expression. Cell 78, 823–834. doi: 10.1016/S0092-8674(94)90562-2
Pubmed Abstract | Pubmed Full Text | CrossRef Full Text | Google Scholar
Clyne, P. J., Warr, C. G., Freeman, M. R., Lessing, D., Kim, J., and Carlson, J. R. (1999). A novel family of divergent seven-transmembrane proteins: candidate odorant receptors in Drosophila. Neuron 22, 327–338. doi: 10.1016/S0896-6273(00)81093-4
Pubmed Abstract | Pubmed Full Text | CrossRef Full Text | Google Scholar
Cosse, A. A., Campbell, M. G., Glover, T. J., Linn, C. E., Todd, J. L., Baker, T. C., et al. (1995). Pheromone behavioral-responses in unusual male European corn-borer hybrid progeny not correlated to electrophysiological phenotypes of their pheromone-specific antennal neurons. Experientia 51, 809–816. doi: 10.1007/BF01922435
Couto, A., Alenius, M., and Dickson, B. J. (2005). Molecular, anatomical, and functional organization of the Drosophila olfactory system. Curr. Biol. 15, 1535–1547. doi: 10.1016/j.cub.2005.07.034
Pubmed Abstract | Pubmed Full Text | CrossRef Full Text | Google Scholar
De Bruyne, M., and Baker, T. C. (2008). Odor detection in insects: volatile codes. J. Chem. Ecol. 34, 882–897. doi: 10.1007/s10886-008-9485-4
Pubmed Abstract | Pubmed Full Text | CrossRef Full Text | Google Scholar
Dekker, T., Ibba, I., Siju, K.-P., Stensmyr, M. C., and Hansson, B. S. (2006). Olfactory shifts parallel superspecialism for toxic fruit in Drosophila melanogaster sibling, D. sechellia. Curr. Biol. 16, 101–109. doi: 10.1016/j.cub.2005.11.075
Pubmed Abstract | Pubmed Full Text | CrossRef Full Text | Google Scholar
Desantis, M., and Limwongse, V. (1983). Relationships between size, number and density of axosomatic boutons and motor neuronal size. J. Theor. Neurobiol. 2, 213–236.
Dobritsa, A. A., van Der Goes van Naters, W., Warr, C. G., Steinbrecht, R. A., and Carlson, J. R. (2003). Integrating the molecular and cellular basis of odor coding in the Drosophila antenna. Neuron 37, 827–841. doi: 10.1016/S0896-6273(03)00094-1
Pubmed Abstract | Pubmed Full Text | CrossRef Full Text | Google Scholar
Dopman, E. B., Bogdanowicz, S. M., and Harrison, R. G. (2004). Genetic mapping of sexual isolation between E and Z pheromone strains of the European corn borer (Ostrinia nubilalis). Genetics 167, 301–309. doi: 10.1534/genetics.167.1.301
Pubmed Abstract | Pubmed Full Text | CrossRef Full Text | Google Scholar
Elmore, T., and Smith, D. P. (2001). Putative Drosophila odor receptor OR43b localizes to dendrites of olfactory neurons. Insect Biochem. Mol. Biol. 31, 791–798. doi: 10.1016/S0965-1748(00)00184-3
Pubmed Abstract | Pubmed Full Text | CrossRef Full Text | Google Scholar
Forstner, M., Breer, H., and Krieger, J. (2009). A receptor and binding protein interplay in the detection of a distinct pheromone component in the silkmoth Antheraea polyphemus. Int. J. Biol. Sci. 5, 745–757. doi: 10.7150/ijbs.5.745
Pubmed Abstract | Pubmed Full Text | CrossRef Full Text | Google Scholar
Fujii, T., Fujii, T., Namiki, S., Abe, H., Sakurai, T., Ohnuma, A., et al. (2011). Sex-linked transcription factor involved in a shift of sex-pheromone preference in the silkmoth Bombyx mori. Proc. Natl. Acad. Sci. U.S.A. 108, 18038–18043. doi: 10.1073/pnas.1107282108
Pubmed Abstract | Pubmed Full Text | CrossRef Full Text | Google Scholar
Gemeno, C., Sans, A., Lopez, C., Albajes, R., and Eizaguirre, M. (2006). Pheromone antagonism in the european corn borer moth Ostrinia nubilalis. J. Chem. Ecol. 32, 1071–1084. doi: 10.1007/s10886-006-9046-7
Pubmed Abstract | Pubmed Full Text | CrossRef Full Text | Google Scholar
Goldman, A. L., Van Naters, W. V., Lessing, D., Warr, C. G., and Carlson, J. R. (2005). Coexpression of two functional odor receptors in one neuron. Neuron 45, 661–666. doi: 10.1016/j.neuron.2005.01.025
Pubmed Abstract | Pubmed Full Text | CrossRef Full Text | Google Scholar
Groot, A. T., Horovitz, J. L., Hamilton, J., Santangelo, R. G., Schal, C., and Gould, F. (2006). Experimental evidence for interspecific directional selection on moth pheromone communication. Proc. Natl. Acad. Sci. U.S.A. 103, 5858–5863. doi: 10.1073/pnas.0508609103
Pubmed Abstract | Pubmed Full Text | CrossRef Full Text | Google Scholar
Grosse-Wilde, E., Gohl, T., Bouche, E., Breer, H., and Krieger, J. (2007). Candidate pheromone receptors provide the basis for the response of distinct antennal neurons to pheromonal compounds. Eur. J. Neurosci. 25, 2364–2373. doi: 10.1111/j.1460-9568.2007.05512.x
Pubmed Abstract | Pubmed Full Text | CrossRef Full Text | Google Scholar
Gulyas, A. I., Megias, M., Emri, Z., and Freund, T. F. (1999). Total number and ratio of excitatory and inhibitory synapses converging onto single interneurons of different types in the CA1 area of the rat hippocampus. J. Neurosci. 19, 10082–10097.
Hall, T. A. (1999). BioEdit: a user-friendly biological sequence alignment editor and analysis program for Windows 95/98/NT. Nucleic Acids Symp. 41, 95–98.
Hallem, E. A., Ho, M. G., and Carlson, J. R. (2004). The molecular basis of odor coding in the Drosophila antenna. Cell 117, 965–979. doi: 10.1016/j.cell.2004.05.012
Pubmed Abstract | Pubmed Full Text | CrossRef Full Text | Google Scholar
Hansson, B. S., Hallberg, E., Lofstedt, C., and Steinbrecht, R. A. (1994). Correlation between dendrite diameter and action potential amplitude in sex pheromone specific receptor neurons in male Ostrinia nubilalis (Lepidoptera: Pyralidae). Tissue Cell 26, 503–512. doi: 10.1016/0040-8166(94)90003-5
Pubmed Abstract | Pubmed Full Text | CrossRef Full Text | Google Scholar
Hansson, B. S., Lofstedt, C., and Foster, S. P. (1989). Z-linked inheritance of male olfactory response to sex pheromone components in two species of tortricid moths, Ctenopseustis obliquana and Ctenopseustis sp. Entomol. Exp. Appl. 53, 137–145. doi: 10.1111/j.1570-7458.1989.tb01298.x
Hansson, B. S., Löfstedt, C., and Roelofs, W. L. (1987). Inheritance of olfactory response to sex pheromone components in Ostrinia nubilalis. Naturwissenschaften 74, 497–499. doi: 10.1007/BF00447935
Ibba, I., Angioy, A. M., Hansson, B. S., and Dekker, T. (2010). Macroglomeruli for fruit odors change blend preference in Drosophila. Naturwissenschaften 97, 1059–1066. doi: 10.1007/s00114-010-0727-2
Pubmed Abstract | Pubmed Full Text | CrossRef Full Text | Google Scholar
Kárpáti, Z., Dekker, T., and Hansson, B. S. (2008). Reversed functional topology in the antennal lobe of the male European corn borer. J. Exp. Biol. 211, 2841–2848. doi: 10.1242/jeb.017319
Pubmed Abstract | Pubmed Full Text | CrossRef Full Text | Google Scholar
Kárpáti, Z., Molnar, B., and Szoecs, G. (2007). Pheromone titer and mating frequency of E- and Z-strains of the European corn borer, Ostrinia nubilalis: Fluctuation during scotophase and age dependence. Acta Phytopathol. Hun. 42, 331–341. doi: 10.1556/APhyt.42.2007.2.15
Kárpáti, Z., Olsson, S., Hansson, B. S., and Dekker, T. (2010). Inheritance of central neuroanatomy and physiology related to pheromone preference in the male European corn borer. BMC Evol. Biol. 10:286. doi: 10.1186/1471-2148-10-286
Pubmed Abstract | Pubmed Full Text | CrossRef Full Text | Google Scholar
Kárpáti, Z., Tasin, M., Cardé, R. T., and Dekker, T. (2013). Early quality assessment lessens pheromone specificity in a moth. Proc. Natl. Acad. Sci. U.S.A. 110, 7377–7382. doi: 10.1073/pnas.1216145110
Pubmed Abstract | Pubmed Full Text | CrossRef Full Text | Google Scholar
Klun, J. A., Chapman, O. L., Mattes, K. C., Wojtkowski, P. W., Beroza, M., and Sonnet, P. E. (1973). Insect sex pheromones: minor amount of opposite geometrical isomer critical to attraction. Science 181, 661–663. doi: 10.1126/science.181.4100.661
Pubmed Abstract | Pubmed Full Text | CrossRef Full Text | Google Scholar
Klun, J. A., and Robinson, J. F. (1971). European corn borer moth - sex attractant and sex attraction inhibitors. Ann. Entomol. Soc. Am. 64, 1083–1086.
Krieger, J., Grosse-Wilde, E., Gohl, T., Dewer, Y. M., Raming, K., and Breer, H. (2004). Genes encoding candidate pheromone receptors in a moth (Heliothis virescens). Proc. Natl. Acad. Sci. U.S.A. 101, 11845–11850. doi: 10.1073/pnas.0403052101
Pubmed Abstract | Pubmed Full Text | CrossRef Full Text | Google Scholar
Krieger, J., Raming, K., Dewer, Y. M., Bette, S., Conzelmann, S., and Breer, H. (2002). A divergent gene family encoding candidate olfactory receptors of the moth Heliothis virescens. Eur. J. Neurosci. 16, 619–628. doi: 10.1046/j.1460-9568.2002.02109.x
Pubmed Abstract | Pubmed Full Text | CrossRef Full Text | Google Scholar
Kumar, G. L., and Keil, T. A. (1996). Pheromone stimulation induces cytoskeletal changes in olfactory dendrites of male silkmoths (Lepidoptera, Saturniidae, Bombycidae). Naturwissenschaften 83, 476–478. doi: 10.1007/BF01144018
Lassance, J. M., Groot, A. T., Lienard, M. A., Antony, B., Borgwardt, C., Andersson, F., et al. (2010). Allelic variation in a fatty-acyl reductase gene causes divergence in moth sex pheromones. Nature 466, 486–489. doi: 10.1038/nature09058
Pubmed Abstract | Pubmed Full Text | CrossRef Full Text | Google Scholar
Lassance, J. M., Lienard, M. A., Antony, B., Qian, S., Fujii, T., Tabata, J., et al. (2013). Functional consequences of sequence variation in the pheromone biosynthetic gene pgFAR for Ostrinia moths. Proc. Natl. Acad. Sci. U.S.A. 110, 3967–3972. doi: 10.1073/pnas.1208706110
Pubmed Abstract | Pubmed Full Text | CrossRef Full Text | Google Scholar
Leary, G. P., Allen, J. E., Bunger, P. L., Luginbill, J. B., Linn, C. E. Jr., Macallister, I. E., et al. (2012). Single mutation to a sex pheromone receptor provides adaptive specificity between closely related moth species. Proc. Natl. Acad. Sci. U.S.A. 109, 14081–14086. doi: 10.1073/pnas.1204661109
Pubmed Abstract | Pubmed Full Text | CrossRef Full Text | Google Scholar
Linn, C. E. Jr., Domingue, M. J., Musto, C. J., Baker, T. C., and Roelofs, W. L. (2007). Support for (Z)-11-hexadecanal as a pheromone antagonist in Ostrinia nubilalis: Flight tunnel and single sensillum studies with a New York population. J. Chem. Ecol. 33, 909–921. doi: 10.1007/s10886-007-9268-3
Pubmed Abstract | Pubmed Full Text | CrossRef Full Text | Google Scholar
Lofstedt, C. (1993). Moth pheromone genetics and evolution. Philos. Trans. R. Soc. Lond. B Biol. Sci. 340, 167–177. doi: 10.1098/rstb.1993.0055
Malausa, T., Bethenod, M. T., Bontemps, A., Bourguet, D., Cornuet, J. M., and Ponsard, S. (2005). Assortative mating in sympatric host races of the European corn borer. Science 308, 258–260. doi: 10.1126/science.1107577
Pubmed Abstract | Pubmed Full Text | CrossRef Full Text | Google Scholar
Mani, E., Riggenbach, W., and Mendik, M. (1978). Rearing of the codling moth Laspeyresia pomonella on artificial diet 1968-1978. Mitteilungen der Schweizerischen Entomologischen Gesellschaft 51, 315–326.
Mann, H. B., and Whitney, D. R. (1947). On a test of whether one of two random variables is stochastically larger than the other. Ann. Math. Statist. 18, 50–60. doi: 10.1214/aoms/1177730491
McElfresh, J. S., and Millar, J. G. (2001). Geographic variation in the pheromone system of the saturniid moth Hemileuca eglanterina. Ecology 82, 3505–3518. doi: 10.1890/0012-9658(2001)082[3505:GVITPS]2.0.CO;2
Meng, L. Z., Wu, C. H., Wicklein, M., Kaissling, K. E., and Bestmann, H. J. (1989). Number and sensitivity of three types of pheromone receptor-cells in Antheraea pernyi and Antheraea polyphemus. J. Comp. Physiol. A 165, 139–146. doi: 10.1007/BF00619188
Merlin, C., Lucas, P., Rochat, D., Francois, M.-C., Maibeche-Coisne, M., and Jacquin-Joly, E. (2007). An antennal circadian clock and circadian rhythms in peripheral pheromone reception in the moth Spodoptera littoralis. J. Biol. Rhythms 22, 502–514. doi: 10.1177/0748730407307737
Pubmed Abstract | Pubmed Full Text | CrossRef Full Text | Google Scholar
Miller, C. W., and Svensson, E. I. (2014). Sexual selection in complex environments. Annu. Rev. Entomol. 59, 427–445. doi: 10.1146/annurev-ento-011613-162044
Pubmed Abstract | Pubmed Full Text | CrossRef Full Text | Google Scholar
Mitsuno, H., Sakurai, T., Murai, M., Yasuda, T., Kugimiya, S., Ozawa, R., et al. (2008). Identification of receptors of main sex-pheromone components of three Lepidopteran species. Eur. J. Neurosci. 28, 893–902. doi: 10.1111/j.1460-9568.2008.06429.x
Pubmed Abstract | Pubmed Full Text | CrossRef Full Text | Google Scholar
Miura, N., Nakagawa, T., Tatsuki, S., Touhara, K., and Ishikawa, Y. (2009). A male-specific odorant receptor conserved through the evolution of sex pheromones in Ostrinia moth species. Int. J. Biol. Sci. 5, 319–330. doi: 10.7150/ijbs.5.319
Pubmed Abstract | Pubmed Full Text | CrossRef Full Text | Google Scholar
Miura, N., Nakagawa, T., Touhara, K., and Ishikawa, Y. (2010). Broadly and narrowly tuned odorant receptors are involved in female sex pheromone reception in Ostrinia moths. Insect Biochem. Mol. Biol. 40, 64–73. doi: 10.1016/j.ibmb.2009.12.011
Pubmed Abstract | Pubmed Full Text | CrossRef Full Text | Google Scholar
Nakagawa, T., Sakurai, T., Nishioka, T., and Touhara, K. (2005). Insect sex-pheromone signals mediated by specific combinations of olfactory receptors. Science 307, 1638–1642. doi: 10.1126/science.1106267
Pubmed Abstract | Pubmed Full Text | CrossRef Full Text | Google Scholar
Niimura, Y., and Nei, M. (2005). Evolutionary dynamics of olfactory receptor genes in fishes and tetrapods. Proc. Natl. Acad. Sci. U.S.A. 102, 6039–6044. doi: 10.1073/pnas.0501922102
Pubmed Abstract | Pubmed Full Text | CrossRef Full Text | Google Scholar
Olsson, S. B., Kesevan, S., Groot, A. T., Dekker, T., Heckel, D. G., and Hansson, B. S. (2010). Ostrinia revisited: Evidence for sex linkage in European Corn Borer Ostrinia nubilalis (Hubner) pheromone reception. BMC Evol. Biol. 10:285. doi: 10.1186/1471-2148-10-285
Pubmed Abstract | Pubmed Full Text | CrossRef Full Text | Google Scholar
Pfaffl, M. W. (2001). A new mathematical model for relative quantification in real-time RT-PCR. Nucleic Acids Res. 29:e45. doi: 10.1093/nar/29.9.e45
Pubmed Abstract | Pubmed Full Text | CrossRef Full Text | Google Scholar
Phelan, P. L. (1997). “Genetics and phylogenetics in the evolution of sex pheromones,” in Insect Pheromone Research: New Directions, eds R. T. Carde and A. K. Minks (New York, NY: Chapman & Hall), 563–579.
Priesner, E. (1988). (Z)-3- and (Z)-5-decenyl acetates, sex attractant components for male Eustrotiu uncuku Cl. (Lepidoptera: Noctuidae). Z. Naturforsch. C 43, 961–962.
Roelofs, W. L., Glover, T., Tang, X. H., Robbins, P., Eckenrode, C. J., Löfstedt, C., et al. (1987). Sex pheromone production and perception in European corn borer moths is determined by both autosomal and sex-linked genes. PNAS 84, 7585–7589.
Rose, T. M., Henikoff, J. G., and Henikoff, S. (2003). CODEHOP (COnsensus-DEgenerate hybrid oligonucleotide primer) PCR primer design. Nucleic Acids Res. 31, 3763–3766. doi: 10.1093/nar/gkg524
Pubmed Abstract | Pubmed Full Text | CrossRef Full Text | Google Scholar
Rozen, S., and Skaletsky, H. J. (2000). “Primer3 on the WWW for general users and for biologist programmers,” in Bioinformatics Methods and Protocols: Methods in Molecular Biology, eds S. Krawetz and S. Misener (Totowa, NJ: Humana Press), 365–386.
Sakurai, T., Nakagawa, T., Mitsuno, H., Mori, H., Endo, Y., Tanoue, S., et al. (2004). Identification and functional characterization of a sex pheromone receptor in the silkmoth Bombyx mori. Proc. Natl. Acad. Sci. U.S. A. 101, 16653–16658. doi: 10.1073/pnas.0407596101
Pubmed Abstract | Pubmed Full Text | CrossRef Full Text | Google Scholar
Sanchez-Gracia, A., Vieira, F. G., and Rozas, J. (2009). Molecular evolution of the major chemosensory gene families in insects. Heredity 103, 208–216. doi: 10.1038/hdy.2009.55
Pubmed Abstract | Pubmed Full Text | CrossRef Full Text | Google Scholar
Tamura, K., Peterson, D., Peterson, N., Stecher, G., Nei, M., and Kumar, S. (2011). MEGA5: Molecular evolutionary genetics analysis using maximum likelihood, evolutionary distance, and maximum parsimony methods. Mol. Biol. Evol. 28, 2731–2739. doi: 10.1093/molbev/msr121
Pubmed Abstract | Pubmed Full Text | CrossRef Full Text | Google Scholar
Todd, J. L., Haynes, K. F., and Baker, T. C. (1992). Antennal neurones specific for redundant pheromone components in normal and mutant Trichoplusia ni males. Physiol. Entomol. 17, 183–192. doi: 10.1111/j.1365-3032.1992.tb01198.x
Vasquez, G. M., Syed, Z., Estes, P. A., Leal, W. S., and Gould, F. (2013). Specificity of the receptor for the major sex pheromone component in Heliothis virescens. J. Insect Sci. 13:160. doi: 10.1673/031.013.16001
Pubmed Abstract | Pubmed Full Text | CrossRef Full Text | Google Scholar
Vosshall, L. B., Wong, A. M., and Axel, R. (2000). An olfactory sensory map in the fly brain. Cell 102, 147–159. doi: 10.1016/S0092-8674(00)00021-0
Pubmed Abstract | Pubmed Full Text | CrossRef Full Text | Google Scholar
Wanner, K. W., Nichols, A. S., Allen, J. E., Bunger, P. L., Garczynski, S. F., Linn, C. E. Jr., et al. (2010). Sex pheromone receptor specificity in the European corn borer moth, Ostrinia nubilalis. PLoS ONE 5:e8685. doi: 10.1371/journal.pone.0008685
Pubmed Abstract | Pubmed Full Text | CrossRef Full Text | Google Scholar
Weiss, L. A., Dahanukar, A., Kwon, J. Y., Banerjee, D., and Carlson, J. R. (2011). The molecular and cellular basis of bitter taste in Drosophila. Neuron 69, 258–272. doi: 10.1016/j.neuron.2011.01.001
Pubmed Abstract | Pubmed Full Text | CrossRef Full Text | Google Scholar
Wilcoxon, F. (1945). Individual comparisons by ranking methods. Biometrics Bull. 1, 80–83. doi: 10.2307/3001968
Xu, P., Garczynski, S. F., Atungulu, E., Syed, Z., Choo, Y.-M., Vidal, D. M., et al. (2012). Moth sex pheromone receptors and deceitful parapheromones. PLoS ONE 7:e41653. doi: 10.1371/journal.pone.0041653
Pubmed Abstract | Pubmed Full Text | CrossRef Full Text | Google Scholar
Yasukochi, Y., Miura, N., Nakano, R., Sahara, K., and Ishikawa, Y. (2011). Sex-Linked pheromone receptor genes of the European Corn Borer, Ostrinia nubilalis, are in Tandem Arrays. PLoS ONE 6:e18843. doi: 10.1371/journal.pone.0018843
Pubmed Abstract | Pubmed Full Text | CrossRef Full Text | Google Scholar
Keywords: Ostrinia nubilalis, olfactory evolution, pheromone receptors, in situ hybridization, single sensillum recordings, qPCR
Citation: Koutroumpa FA, Kárpáti Z, Monsempes C, Hill SR, Hansson BS, Jacquin-Joly E, Krieger J and Dekker T (2014) Shifts in sensory neuron identity parallel differences in pheromone preference in the European corn borer. Front. Ecol. Evol. 2:65. doi: 10.3389/fevo.2014.00065
Received: 14 July 2014; Accepted: 25 September 2014;
Published online: 29 October 2014.
Edited by:
Matthieu Keller, Centre National de la Recherche Scientifique, FranceCopyright © 2014 Koutroumpa, Kárpáti, Monsempes, Hill, Hansson, Jacquin-Joly, Krieger and Dekker. This is an open-access article distributed under the terms of the Creative Commons Attribution License (CC BY). The use, distribution or reproduction in other forums is permitted, provided the original author(s) or licensor are credited and that the original publication in this journal is cited, in accordance with accepted academic practice. No use, distribution or reproduction is permitted which does not comply with these terms.
*Correspondence: Teun Dekker, Division of Chemical Ecology, Department of Plant Protection Biology, Swedish University of Agricultural Sciences, PO Box 102, 230 53 Alnarp, Sweden e-mail:dGV1bi5kZWtrZXJAc2x1LnNl
†Present address: Fotini A. Koutroumpa, INRA, UMR iEES-Paris, Versailles Cedex, France;
Zsolt Kárpáti, Department of Zoology, Centre for Agricultural Research, Plant Protection Institute, Hungarian Academy of Sciences, Budapest, Hungary