- 1Department of Biology, University of Eastern Finland, Joensuu, Finland
- 2Centre for Evolutionary Biology, University of Western Australia, Crawley, WA, Australia
Animals are often individually consistent in their behavior, not only over time, but also across different functional contexts. Recent research has focused on phenotypic and evolutionary mechanisms explaining such personality differences through selection. Parasitism and predation induce important mortality and fitness costs, and are thus main candidates to create and maintain personality differences in the wild. Here, we present data on the behavioral consistency of the Eurasian minnow (Phoxinus phoxinus) from two populations that live in different tributaries of the same river, but whose ecological environment differs fundamentally with regard to predation and parasitism. We experimentally demonstrate that individual minnow in both study populations are consistent in their boldness and activity. However, the two study populations differ notably: in the high predation and parasitism risk population fish show higher mean boldness, but tend to be less active than fish in low predation and parasitism risk population. Parasite (Diplostomum phoxini) load was negatively, but not statistically significantly, associated with fish activity level. Our study suggests that parasitism and predation are likely important agents in the ecology and evolution of animal personalities.
Introduction
Over the last decade there has been considerable interest in animal personalities (e.g., Bell, 2007; Sih et al., 2012; Carere and Maestripieri, 2013; Kortet et al., 2014). Personalities, also known as “temperaments” or “coping styles”, are defined as behaviors that vary predictably among individuals, and are consistent across time and/or contexts within individuals (e.g., Réale et al., 2007; Stamps and Groothuis, 2010). Consistent personalities have been puzzling behavioral ecologists, because they limit behavioral flexibility and occasionally appear to produce seemingly maladaptive behaviors (Dall et al., 2004; Sih et al., 2004). Several mechanisms have been proposed to explain how personality variation is maintained in animal populations over evolutionary time. These mechanisms include, for example, the asset protection principle, i.e., behaviorally mediated trade-offs between current vs. future reproduction (Wolf et al., 2007), related growth-mortality trade-offs (Stamps, 2007; Biro and Stamps, 2008), positive feedback loops and state-dependent behavior (McElreath et al., 2007; Luttbeg and Sih, 2010) and variation in costly cognition (Niemelä et al., 2013). Importantly, these mechanisms are not necessarily mutually exclusive. Rather, they are based on additive and interacting environmental factors such as predation and parasitism that affect the costs and benefits of certain behaviors in various ways.
At the moment, the existence and importance of animal personalities are generally accepted and documented for several taxa, while the relative importance of different ecological factors explaining their evolution and expression are under debate (e.g., Barber and Dingemanse, 2010; Kortet et al., 2010; Niemelä et al., 2012, 2013; Sih et al., 2012). Traditionally, main factors affecting the evolution and ecology of animal personalities have included resource competition (Dingemanse et al., 2004; Cote et al., 2008) and predation pressure (e.g., Bell and Sih, 2007; Dingemanse et al., 2007). Recently, parasitism has also emerged as a potential key driver of the ecology and evolution of animal personalities (Barber and Dingemanse, 2010; Coats et al., 2010; Kortet et al., 2010; Koprivnikar et al., 2012; Poulin, 2013). Parasitism and predation may amplify the effects of each other both additively and in interaction, which emphasizes the importance of understanding their integrative role in inducing selection (Kortet et al., 2010). Unfortunately, most empirical studies so far have ignored the importance of parasitism in the animal personality context.
Within-population and between-population variation in personality traits has been described in a number of taxa including squid (Sinn et al., 2010), spiders (e.g., Hedrick and Riechert, 1989), field crickets (Kortet and Hedrick, 2007; Niemelä et al., 2012), fish (e.g., Bell and Sih, 2007; Dingemanse et al., 2007), crayfish (Vainikka et al., 2011), birds (Atwell et al., 2012) and primates (Weiss and Adams, 2013). In general, it can be predicted that the stronger the environmental selection by parasitism or predation, the more consistently an individual should follow the behavioral trajectory set by its initial and current assets, and the more likely it is that animal personalities will emerge in a population (Kortet et al., 2010). As indirect support for this idea, behavioral syndromes and consistent behavior has been detected especially in high-risk environments. In fishes, both observational and experimental evidence suggests that individuals from high-predation risk environments may behave more predictably than individuals from predator-free populations (Bell and Sih, 2007; Dingemanse et al., 2007; Adriaenssens and Johnsson, 2013). However, according to the best of our knowledge, earlier reports so far have not included risk by parasitism in the interpretation of the between-population differences in personality studies (Barber and Dingemanse, 2010; Kortet et al., 2010). High-parasitism risk environment, often coupled with high-predation risk, may produce interesting patterns in personality traits like boldness and associated phenotypic traits (e.g., Kortet et al., 2007). In general, the theory of parasite-mediated personality evolution suggests that intensified selection by predators and parasites should increase an individual's behavioral consistency over both ecological and evolutionary time scales, by selecting out individuals that do not follow their optimal behavioral trajectories (Kortet et al., 2010).
The aim of the present study was to investigate behavioral consistency in two Eurasian minnow (Cyprinidae: Phoxinus phoxinus) populations that differ fundamentally in their predation and parasitism risks, but originate from the same water-course. One of the populations (Kuusoja River) harbors both parasites and predatory fish while in the other population parasites and piscivorous fish are both non-existent (Kolvananuuro Brook). We experimentally assessed boldness- and activity-indicating behaviors and their repeatability in wild-collected minnows by performing three behavioral trials in standardized laboratory conditions: two trials without a predator cue and one trial with predator odor. We predicted that in the high predation and parasitism risk population, fish would show higher boldness and higher behavioral consistency, because they would need to compensate for the energetics costs of parasitism and try to reduce predation risk by growing fast (Kortet et al., 2010). This result would be also in line with the possible parasite-induced behavioral manipulation. We also tested if parasite load would correlate with an individual's personality (see Kekäläinen et al., 2014a).
Material and Methods
Natural History of the Study Species
The European minnow (or common minnow) is a small cyprinid fish, ubiquitously inhabiting freshwaters in Eurasia, including Britain, Spain, Scandinavia and Siberia. In Finland, the minnow inhabits predominantly cool (summer temperature 12–20°C), brooks streams and well-oxygenated lakes and ponds. It exists also in the brackish water coastal areas of the Baltic Sea. The species adapts well to laboratory conditions and has been used as a model in behavioral studies (e.g., Lai et al., 2013; Kekäläinen et al., 2014a). Many populations of the European minnow harbor harmful trematodean and nematodean parasites that likely impose strong indirect and direct mortality on their hosts. In our previous experimental work, we have demonstrated that minnows infected by the trematodean parasite Diplostomum phoxini show higher repeatability in boldness and activity, and reduced repeatability in exploration compared to non-infected fish (Kekäläinen et al., 2014a).
Study Animals and Characteristics of the Source Populations
A haphazard minnow sample was collected by dip nets from the two study populations in Eastern Finland in June 28 and June 30 2011. In total of 150 fish were sampled from Kolvananuuro Brook (62° 51N, 29° 59E) and Kuusoja River (62° 48N, 30° 1E). Kolvananuuro Brook is a headwater tributary of Kuusoja River that discharges to Pielisjoki River, in the Vuoksi River watershed. Based on our earlier fish samples and their examination for parasites, Kolvananuuro Brook minnow do not host D. phoxini, likely because the lack of a suitable intermediate host in the system. In contrast, in Kuusoja River the prevalence of D. phoxini in minnows is close to 100%, which means that virtually all of the fish larger than 30 mm have been infected with this parasite. The nematodean parasite Philometra ovata has never been found in Kolvananuuro Brook minnows, while in Kuusoja River the prevalence of body cavity dwelling P. ovata in minnows varies from 4.00 to 9.19 percent (Lai et al., 2012). Predation risk by piscivorous fishes in Kolvananuuro Brook is likely non-existent, since we have never encountered any predatory fish in the area (despite numerous fish sampling using traps and landing nets). Potential migration of minnows to areas with predators are not known, but appears unlikely given the abundant physical migration obstacles in the brook. In contrast, based on electrofishing conducted annually during university courses, predatory fish including burbot (Lota lota), perch (Perca fluviatilis) and pike (Esox lucius) are common in Kuusoja River, that also supports small populations of grayling (Thymallus thymallus) and resident brown trout (Salmo trutta) in addition to the common cyprinids roach (Rutilus rutilius) and dace (Leuciscus leuciscus).
Study fish were transported to the laboratory at the Department of Biology (Joensuu campus) of the University of Eastern Finland, where they were housed in two 45 l tanks in 15°C water temperature under a simulated natural photoperiod. After 2 days of acclimatization the water temperature was increased to 17°C. Fish were fed daily with commercial fish food (Biomar®; Aqualife, Aarhus, Denmark). After 4 days, a subsample of 50 fish of about the same size from each population was taken. All of the fish were placed individually in one liter plastic containers, in which they were held during the whole experimental period. To ensure adequate oxygen exchange, two walls of the container had been replaced with plastic nets (mesh size: 1 mm). The plastic containers were distributed into two 600 liter tanks (two replicates/group) and were housed in 17°C water temperature under a simulated natural photoperiod. Fish were fed daily ad libitum with commercial fish food (Biomar®; Aqualife, Aarhus, Denmark) through a small hole in the cap of the individual container. During the experimental period one fish died, so the definitive sample sizes were 49 fish from Kuusoja River and 50 fish from Kolvananuuro Brook.
Behavioral Experiments
We carried out three sequential behavioral aquarium-scale trials for all individuals: (1) 2 weeks after the fish had been sampled from the field, (2) 4 days after the first behavioral trial and (3) 8 days after the first behavioral trial. The third trial included the predator odor effect. All experiments were always conducted on the same day for each group. The predator odor for the third trial came from two live pike (weights 278 and 453 g), that were sampled and brought to the laboratory from the nearby Lake Salmijärvi. The pike were housed in 100 l flow-through tank (water flow 3 l/min), from which the predator odor water was obtained.
In the behavioral trials, individual boldness and activity were studied in a three-part plastic arena (Kekäläinen et al., 2014a) (length 54 × width 35 × height 20 cm, water volume 20 l, Figure 1). Each individual was first placed on the small area (i.e., emergence box) at the other end of the arena, where they were allowed to acclimate to experimental conditions for 2 min. After the acclimation period the gate between the acclimation area and the arena was carefully lifted using a thin fishing line so that fish were not able to see the lifter. Then the fish were allowed to swim freely in the arena for 10 min. The actual arena consisted of two parts which differed in floor color (gray and white). Since the gray-colored area more closely resembles the natural camouflage of the minnows, the white area was assumed to represent a more risky environment for the minnows (e.g., Maximino et al., 2010; Kekäläinen et al., 2014a). In the third trial the minnows were exposed to the pike odor by adding one liter of water from the pike rearing tank to the plastic arena. The pike odor water was added to the main part of arena at the end of the acclimation period. Timing of the pike odor addition was chosen to prevent odor from reaching the acclimation box before opening the gate.
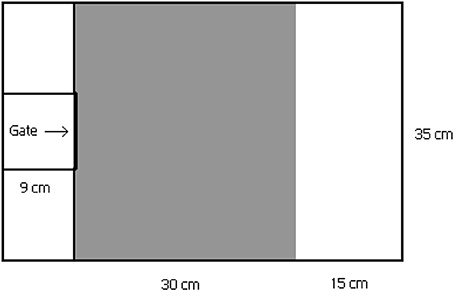
Figure 1. Test arena for the behavioral experiments. Test arena consist three parts: 1. small acclimation area (in the left) with roll-up gate for acclimation period, 2. gray-colored section (in the middle) and 3. white-colored section (in the right). White-colored section is expected to represent more risky environment for the minnows than gray-colored section.
In each behavioral trial, we observed fish behavior and recorded nine behavioral variables: total time spent active (1), outside the shelter (2) and in the white-bottomed area (3). In addition we determined the time fish took before showing first activity (4), swimming outside the shelter (5) and visiting the white area (6). Finally, we also counted the number of occasions fish re-activated their swimming behavior after being passive (7), swam from the shelter to the arena (8) and visited in the white-bottomed area (9). Immediately after the experiment, the focal fish were returned to their individual containers. Similar individual aquarium-scale experiments have been demonstrated to predict individual behavior in ecologically relevant contexts in other fish species (Kekäläinen et al., 2014b).
Water temperature in experimental trials was 17°C and water was changed after every trial. To avoid potential disturbance caused by the observer, behavioral observations took place via HD web camera (Logitech, Webcam Pro 9000, Newark, CA, USA), attached above the experimental arena. After the study period all fish were killed with an overdose of tricaine methanesulfonate (MS-222, Sigma®; Sigma Chemical Co., St. Louis, MO, USA) and their length, fresh body mass and sex were determined and the numbers of parasites, including D. phoxini brain parasites and P. ovata nematodes in the body cavity, were counted. The mean size (± SD) of the fish was similar to that in the populations (50.70 ± 6.45 mm and 1.11 ± 0.40 g: Kolvananuuro Brook 50.43 ± 6.97 mm and 1.06 ± 0.45 g: Kuusoja River). Condition factor K was calculated for each fish as: , where m is the body mass in grams, l the total body length in cm, the estimated value of b was 2.85601, and the multiplier a was 100 cmb g−1. Sex was determined by visual inspection. All experiments were performed according to the license of the Finnish Animal Experiment Board (ESLH-2008-03722/Ym-23).
Statistical Analyses
To reduce the number of correlated variables, we conducted principal component analysis on the abovementioned nine behavioral variables (Table 1). All the variables were Ln-transformed prior to PCA. PCA resulted two principal components (eigenvalue > 1), which explained 72.3% of the variation in fish behavior. When PCA was conducted separately on data from each trial and population, the un-rotated component matrix indicated only marginal differences between the PCAs justifying the use of just one PCA on all data. The first component (PC1) was strongly associated with variables describing fish boldness, whereas the second component (PC2) explained variation in fish activity level. Repeatability of the two principal components was studied using the intraclass correlation coefficient (ICC, Lessells and Boag, 1987). 95% confidence intervals of ICC were used to infer between population differences in repeatability.
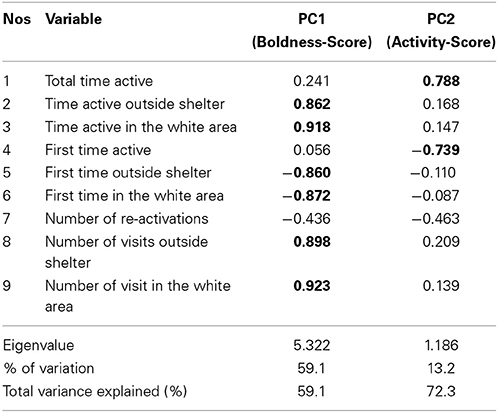
Table 1. Results of the principal component analysis (with Varimax rotation) on the studied behavioral variables.
Behavioral differences (PC1 and PC2, dependent variables) between populations, sexes and measurement periods were modeled using linear mixed effect models using the parsimony principle in model selection (Johnson and Omland, 2004). The models first included fully factorial terms of population, measurement and sex, and covariates (condition factor and fish length). The models were simplified by removing non-significant terms. AIC (Akaike Information Criteria) was used to choose between the two last model candidates with and without the last (lowest p-value) non-significant term. Paired comparison between sexes (male, female and immature) and measurement periods were conducted using equivalent within factor analyses with Bonferroni post-hoc tests.
In the Kuusoja River population, the association between Diplostomum phoxini brain parasite number and PC-scores (fish behavior) was tested using a Spearman correlation analysis. A non-parametric test was selected since the distribution of parasite loads could not be completely normalized. A T-test was used to compare the size and condition of the fish from the two populations. A X2-test was used to test for population differences in the sex ratio of the study fish. Statistical tests were performed with R (The R Foundation for Statistical Computing, Vienna, Austria): Intraclass Correlation Coefficient (ICC) package (version 2.2.) (ICC analyses), and with SPSS version 21.0 (IBM Corp., New York, USA) (all other statistical analyses).
Results
Fish Size and Sex Ratio in the Two Study Populations
Length and fresh body mass of the individuals did not differ between the studied Kuusoja River and Kolvananuuro Brook populations (t-test, df = 97, P ≥ 0.561), but fish from Kolvananuuro Brook had a 5.1% higher condition factor than Kuusoja River fish (t-test, df = 97, P = 0.001). The proportion of males, females and juveniles did not differ between the populations (X = 0.407, df = 2, P = 0.816). Samples from both populations included 12 juveniles. 64% of mature individuals were females.
Repeatability of Behavioral Components and Behavioral Correlations
Both boldness and activity scores were highly repeatable in both populations, both over the first two trials, and across all the three trials, but the repeatability estimates did not differ statistically significantly between the populations (Figure 2).
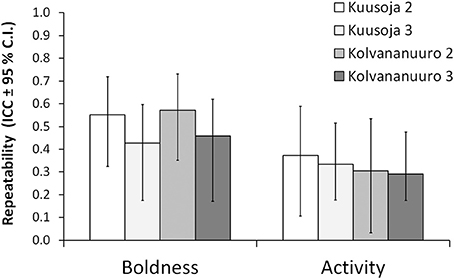
Figure 2. Repeatabilities for boldness and activity in the two study populations (Kuusoja River and Kolvananuuro Brook) over two and all the three trials.
The Effect of Population, Sex and Measurement Period on Fish Behavior
Variation in boldness score was not explained by fish length or condition factor. Fish from the Kuusoja River were significantly bolder than fish from Kolvananuuro brook (Table 2, Figure 3), and males were significantly bolder than females (Table 2), but no differences were found between males and juveniles (Bonferroni, P = 1.00) or between females and juveniles (P = 0.233). The main effect of measurement period (1–3) was highly significant (Table 2) and paired comparisons revealed that minnows behaved boldest in the first behavioral test (Figure 3; Bonferroni, 1. test vs. 2. test: P = 0.034; 1. test vs. 3. test: P < 0.001) and were bolder in the second test than in the third test (P < 0.001). In other words, on average minnows in each population were less bold in the test where predator odor was present (Figure 3). However, we also found a significant interaction between population and measurement period (Table 2) that resulted from the abrupt decrease in boldness of Kolvananuuro Brook fish when predator odor was present (Figure 3). Such a response was absent in the Kuusoja River population where boldness decreased through all measurements rather linearly (Figure 3).
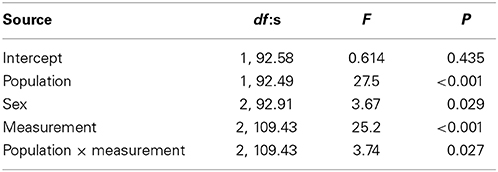
Table 2. Significance of fixed factors in explaining variance in boldness score according to linear mixed effect model.
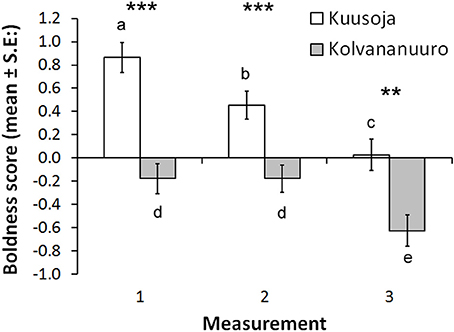
Figure 3. Estimated marginal means for boldness scores in the three behavioral trials in the two study populations (Kuusoja River and Kolvananuuro Brook). Paired differences are indicated with asterisks (***P < 0.001; **P < 0.01), and among measurement differences within populations with different letters (alpha = 0.05).
The activity score was higher in Kolvananuuro Brook population than in the Kuusoja River population (scores 0.202 and −0.208 respectively; Table 3). Activity stayed unchanged through different measurements within and among populations (Figure 4). Source population did not interact with measurement, but the sexes behaved differently at different measurement times (Figure 4). The significant interaction was explained by the strong decrease of activity of juvenile fish in the third trial, and the low activity of females in the first trial (Figure 4). The negative effect of condition factor on activity was not statistically significant, but the inclusion of condition factor improved the AIC of the model (data not shown).
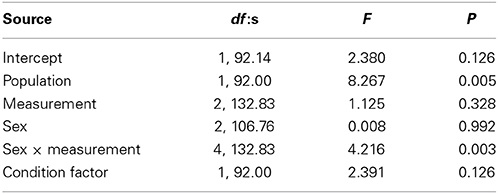
Table 3. Significance of fixed factors in explaining variance in activity score according to linear mixed effect model.
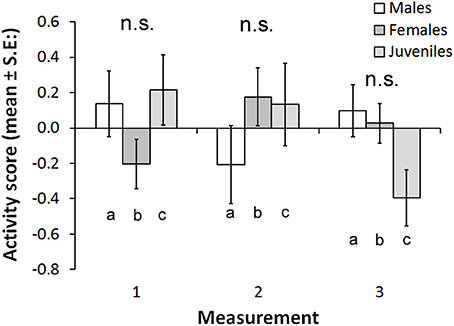
Figure 4. Estimated marginal means for activity scores in the three behavioral trials in the two study populations (Kuusoja River and Kolvananuuro Brook). None of the paired comparisons (both among sexes within measurements and measurements within sexes) were statistically significant.
The Association Between D. Phoxini Parasite Intensity and Personality
All the minnows in the Kuusoja River population were parasitized by D. phoxini with numbers varying from 24 to 922 (mean 171.2 ± 182.7 SD) parasites, while none of the minnows from Kolvananuuro Brook hosted D. phoxini. Prevalence of body cavity dwelling P. ovata was much lower (only 2 fish parasitized with adult worms in Kuusoja River and none in Kolvananuuro Brook), and therefore they were not further analyzed. In the Kuusoja River population the load of D. phoxini was found to be weakly (but statistically insignificantly) negatively associated with fish activity (PC2, rs = −0.232, P = 0.11, n = 49). The correlation was negative in all test-specific analyses (Spearman, 1. test: rs = −0.316, P = 0.027; 2. test: rs = −0.301, P = 0.0363; 3. test: rs = −0.094, P = 0.52, corrected alpha level = 0.025). No association between D. phoxini and boldness (PC1) was found (Spearman, P > 0.8, in all four tests).
Discussion
We found that minnow were individually consistent in boldness and activity in both of the study populations, while the populations did not differ in the repeatability of behavior. In line with our predictions, we found that in the Kuusoja River population (a high predation and high parasitism risk environment), fish showed higher boldness than fish from Kolvananuuro Brook. However, against our predictions, River Kuusoja minnow appeared less active than Brook Kolvananuuro fish. Parasite (Diplostomum phoxini) abundance was negatively, but not statistically significantly, associated with fish activity, which suggests that the infection by these parasites may partly explain the lower activity among parasitized River Kuusoja fish. Since our data come from only two populations, the present results should be interpreted very cautiously.
Recent work in various species has shown that many fishes are fairly consistent in boldness (e.g., Conrad et al., 2011; Kekäläinen et al., 2014b), and that personality components in fish can have heritable components (Kortet et al., 2014). Because fish in both of the study populations were individually consistent in their boldness and activity, the present results are well in line with the previous findings.
We have demonstrated earlier that experimental D. phoxini infection increases the repeatability of boldness and activity in the Kolvananuuro Brook population (Kekäläinen et al., 2014a), but the effect did not arise through selective mortality of non-consistent individuals. Furthermore, Coats et al. (2010) and Hammond-Tooke et al. (2012) have also found support for parasite-induced personality variation. In contrast to earlier findings, however, we did not found differences in repeatabilities between our two study populations in the present study. This difference between present and earlier studies may be partly related to the methodological differences between studies. For example, Kekäläinen et al. (2014a) studied the repeatability of behavior within a single population (Kolvananuuro Brook) and after experimental infection, whereas the present study is based on correlative data and a between-population comparison. Furthermore, it has been demonstrated that when behavioral trials are conducted at relatively short intervals (e.g., less than 10 days, such as in the present study), the resulting repeatability estimates are generally higher than those when behaviors are recorded over longer intervals (Bell et al., 2009; Kekäläinen et al., 2014a). Behavioral boldness and activity were decreased in our third trial that included pike odor exposure, but unfortunately the experimental set up did not allow separating possible time effects from the predator odor effects.
D. phoxini was present in all the Kuusoja River individuals whereas it was absent in Kolvananuuro Brook fish. Therefore, the differential parasite load of D. phoxini may have an important role in explaining why Kuusoja River fish appeared bolder and less active than Kolvananuuro Brook fish. The negative correlation between activity and parasite load directly supports this conclusion, although the complete lack of this parasite from Kolvananuuro Brook did not allow studying the effect of the parasite on the population means. The lack of correlation between boldness and parasite load does not necessarily mean that the D. phoxini does not affect boldness: we have earlier demonstrated (in experimentally infected Kolvananuuro Brook fish) that the effect of D. phoxini on boldness and activity may be non-linearly dependent on parasite density (see Kekäläinen et al., 2014a). However, as present results do not support this earlier finding more studies are needed to clarify the impact of D. phoxini parasite load on personality traits. We can also argue that the other parasite in the system, i.e., body cavity dwelling P. ovata, is unlikely to explain the differences between the populations in the present data, since we have not detected significant effects of P. ovata on minnow behavior in our other studies (Lai et al., unpublished observations).
One might suggest that the observed behavioral patterns among parasitized fish may be of an adaptive nature. Possibly, minnows in the Kuusoja River population show bold behaviors because they can thus compensate the direct and indirect costs caused by high parasitism and predation risk in the environment. Parasitized fish show often weakened condition and fitness (Seppälä and Jokela, 2008), and display changes in their behavior (e.g., Santos and Santos, 2013). If bold behaviors of minnow are associated with increased foraging and nutrition intake, they also likely positively affect the development and maintenance of efficient immune defense, and may thus further increase individual's fitness. This kind of process has, indeed, already been proposed to explain individual variation in personality traits (Luttbeg and Sih, 2010). However, fish condition factor did not explain personality variation. It might thus be that the overall selection or habituation to the presence of predator cues in the high risk environment in the Kuusoja River population would explain high boldness.
Finally, the impacts of D. phoxini on the host's biology are likely determined by the life history of this parasite. As a trophically transmitted trematode parasite, D. phoxini uses the Eurasian minnow in particular as its second intermediate host (Barber and Crompton, 1997a,b). Sexual reproduction of this brain fluke occurs in the intestines of fish-eating birds that act as definitive hosts for the parasite. The infective stages reach the water through bird feces, and enter the first intermediate host, the freshwater snail Radix peregra. Snails infected with D. phoxini produce freely swimming cercariae larvae, which infect minnows by penetrating through their skin and migrate to the fish brain via the blood circulation system. In the host brain, cercariae will encyst and change into metacercariae, i.e., the maturing and infectious stage of the parasite (Barber and Crompton, 1997a,b). Earlier studies have shown that brain-encysting trematodes have the potential to alter host behavior (e.g., Lafferty and Morris, 1996; Shirakashi and Goater, 2002, 2005; Kekäläinen et al., 2014a). In the brain, D. phoxini metacercariae are known to aggregate mainly in the cerebellum, the optic lobes and the medulla oblongata (Barber and Crompton, 1997a), where they cause pathological damage in the brain tissue (e.g., necrosis and disruption in the integrity of brain tissue, Dezfuli et al., 2007). This may cause manipulative effects as a means of increased boldness and thus lead to increased predation by the definitive avian host. However, unlike the parasite-host system of brain trematode Euhaplorchis californiensis in killifish Fundulus parvipinnis (Lafferty and Morris, 1996; Shaw et al., 2009), the behavioral manipulation of D. phoxini metacercariae in minnows in the other populations has never been tested quantitatively but only described briefly (Dönges, 1969; Kekäläinen et al., 2014a). Possible predation susceptibility increasing behaviors of D. phoxini -infected host minnows nor possible mechanisms of behavioral manipulation by D. phoxini have not been examined in detail. Thus, regarding the lack of necessary knowledge about the interaction between D. phoxini and host minnows, the impact of this brain parasite on the biology of host minnows through personality change has remained unclear until recently (Kekäläinen et al., 2014a). In any case, the European minnow provides an interesting model study system with high potential for further work on animal personalities.
To conclude, the present data provide one of the first observations possibly indicating the roles of environmental predation and parasitism risk in the development of animal personalities. The minnows originating from the high predation and parasitism risk population showed higher boldness but lower activity, which is partially in line with the predictions of parasite-mediated personality evolution (Kortet et al., 2010). Since the present data is based on only two populations, the results should be verified by further experimental work by using parasite naïve, experimentally infected, individuals and a common garden set up in low predation and high predation environments. This kind of approach would help us to understand the magnitude of selection on personality caused by predation (with and without parasitism), and the detailed mechanisms for selection to act in the natural environment.
Conflict of Interest Statement
The authors declare that the research was conducted in the absence of any commercial or financial relationships that could be construed as a potential conflict of interest.
Acknowledgments
We thank Ann Hedrick and two anonymous referees for comments on the manuscript. We also thank Leena Koponen and Leena Pääkkönen for their help in the maintenance of minnows and Matti Heep for his help in the field. This study was financially supported by Emil Aaltonen Foundation (Yi-Te Lai, grant number 6-15079-13), North Carelia regional fund of Finnish Cultural Foundation (Yi-Te Lai, grant number 77138-15725 and 94513-13416), Kone foundation (Jukka Kekäläinen) and the European Union under a Marie Curie International Outgoing Fellowship for Career Development (PIOF-GA-2013-629472) (Jukka Kekäläinen).
References
Adriaenssens, B., and Johnsson, J. I. (2013). Natural selection, plasticity and the emergence of a behavioural syndrome in the wild. Ecol. Lett. 16, 47–55. doi: 10.1111/ele.12011
Pubmed Abstract | Pubmed Full Text | CrossRef Full Text | Google Scholar
Atwell, J. W., Cardoso, G. C., Whittaker, D. J., Campbell-Nelson, S., Robertson, K. W., and Ketterson, E. D. (2012). Boldness behavior and stress physiology in a novel urban environment suggest rapid correlated evolutionary adaptation. Behav. Ecol. 23, 960–969. doi: 10.1093/beheco/ars059
Pubmed Abstract | Pubmed Full Text | CrossRef Full Text | Google Scholar
Barber, I., and Crompton, D. W. T. (1997a). The distribution of the metacercariae of Diplostomum phoxini in the brain of minnows, Phoxinus phoxinus. Folia Parasit. 44, 9–25.
Barber, I., and Crompton, D. W. T. (1997b). The ecology of Diplostomum phoxini infections in two minnow (Phoxinus phoxinus) populations in Scotland. J. Helminthol. 71, 189–196. doi: 10.1017/S0022149X0001590X
Pubmed Abstract | Pubmed Full Text | CrossRef Full Text | Google Scholar
Barber, I., and Dingemanse, N. J. (2010). Parasitism and the evolutionary ecology of animal personality. Philos. Trans. R. Soc. Lond. B Biol. Sci. 365, 4077–4088. doi: 10.1098/rstb.2010.0182
Pubmed Abstract | Pubmed Full Text | CrossRef Full Text | Google Scholar
Bell, A. M. (2007). Future directions in behavioural syndromes research. Proc. R. Soc. B 274, 755–761. doi: 10.1098/rspb.2006.0199
Pubmed Abstract | Pubmed Full Text | CrossRef Full Text | Google Scholar
Bell, A. M., Hankison, S. J., and Laskowski, K. L. (2009). The repeatability of behaviour: a meta-analysis. Anim. Behav. 77, 771–783. doi: 10.1016/j.anbehav.2008.12.022
Pubmed Abstract | Pubmed Full Text | CrossRef Full Text | Google Scholar
Bell, A. M., and Sih, A. (2007). Exposure to predation generates personality in threespined sticklebacks (Gasterosteus aculeatus). Ecol. Lett. 10, 828–834. doi: 10.1111/j.1461-0248.2007.01081.x
Pubmed Abstract | Pubmed Full Text | CrossRef Full Text | Google Scholar
Biro, P. A., and Stamps, J. A. (2008). Are animal personality traits linked to life-history productivity? Trends Ecol. Evol. 23, 361–368. doi: 10.1016/j.tree.2008.04.003
Pubmed Abstract | Pubmed Full Text | CrossRef Full Text | Google Scholar
Carere, C., and Maestripieri, D. (2013). “Animal personalities: who cares and why?” in Animal Personalities, eds C. Carere and D. Maestripieri (Chigago, IL: University of Chigago Press) 1–9.
Coats, J., Poulin, R., and Nakagawa, S. (2010). The consequences of parasitic infections for host behavioural correlations and repeatability. Behaviour 147, 367–382. doi: 10.1163/000579509X12574307194101
Conrad, J. L., Weinersmith, K. L., Brodin, T., Saltz, J. B., and Sih, A. (2011). Behavioural syndromes in fishes: a review with implications for ecology and fisheries management. J. Fish Biol. 78, 395–435. doi: 10.1111/j.1095-8649.2010.02874.x
Pubmed Abstract | Pubmed Full Text | CrossRef Full Text | Google Scholar
Cote, J., Dreiss, A., and Clobert, J. (2008). Social personality trait and fitness. Proc. R. Soc. B 275, 2851–2858. doi: 10.1098/rspb.2008.0783
Pubmed Abstract | Pubmed Full Text | CrossRef Full Text | Google Scholar
Dall, S. R. X., Houston, A. I., and McNamara, J. M. (2004). The behavioural ecology of personality: consistent individual differences from an adaptive perspective. Ecol. Lett. 7, 734–739. doi: 10.1111/j.1461-0248.2004.00618.x
Dezfuli, B. S., Capuano, S., Simoni, E., Giari, L., and Shinn, A. P. (2007). Histopathological and ultrastructural observations of metacercarial infections of Diplostomum phoxini (Digenea) in the brain of minnows Phoxinus phoxinus. Dis. Aquat. Organ. 75, 51–59. doi: 10.3354/dao075051
Pubmed Abstract | Pubmed Full Text | CrossRef Full Text | Google Scholar
Dingemanse, N. J., Both, C., Drent, P. J., and Tingbergen, J. M. (2004). Fitness consequences of avian personalities in a fluctuating environment. Proc. R. Soc. B 271, 847–852. doi: 10.1098/rspb.2004.2680
Pubmed Abstract | Pubmed Full Text | CrossRef Full Text | Google Scholar
Dingemanse, N. J., Wright, J., Kazem, A. J. N., Thomas, D. K., Hickling, R., and Dawnay, N. (2007). Behavioural syndromes differ predictably between 12 populations of stickleback. J. Anim. Ecol. 76, 1128–1138. doi: 10.1111/j.1365-2656.2007.01284.x
Pubmed Abstract | Pubmed Full Text | CrossRef Full Text | Google Scholar
Dönges, J. (1969). Entwicklungs- und Lebensdauer von Metacercariaen. Z. Parasitenk. 31, 340–366. doi: 10.1007/BF00259732
Hammond-Tooke, C. A., Nakagawa, S., and Poulin, R. (2012). Parasitism and behavioural syndromes in the fish Gobiomorphus cotidianus. Behaviour 149, 601–622. doi: 10.1163/156853912X648903
Hedrick, A. V., and Riechert, S. F. (1989). Genetically-based variation between two spider populations in foraging behavior. Oecologia 80, 533–539. doi: 10.1007/BF00380078
Johnson, J. B., and Omland, K. S. (2004). Model selection in ecology and evolution. Trends Ecol. Evol. 19, 101–108. doi: 10.1016/j.tree.2003.10.013
Pubmed Abstract | Pubmed Full Text | CrossRef Full Text | Google Scholar
Kekäläinen, J., Lai, Y.-T., Vainikka, A., Sirkka, I., and Kortet, R. (2014a). Do brain parasites alter host personality? - Experimental study in minnows. Behav. Ecol. Sociobiol. 68, 197–204. doi: 10.1007/s00265-013-1634-2
Kekäläinen, J., Podgorniak, T., Puolakka, T., Hyvärinen, P., and Vainikka, A. (2014b). Individually assessed boldness predicts European perch's Perca fluviatilis behaviour in shoals, but is not associated with the capture order or angling method. J. Fish. Biol. 85, 1603–1616. doi: 10.1111/jfb.12516
Pubmed Abstract | Pubmed Full Text | CrossRef Full Text | Google Scholar
Koprivnikar, J., Gibson, C. H., and Redfern, J. C. (2012). Infectious personalities: behavioural syndromes and disease risk in larval amphibians. Proc. R. Soc. B 279, 1544–1550. doi: 10.1098/rspb.2011.2156k
Pubmed Abstract | Pubmed Full Text | CrossRef Full Text | Google Scholar
Kortet, R., and Hedrick, A. (2007). A behavioural syndrome in the field cricket Gryllus integer: intrasexual aggression is correlated with activity in a novel environment. Biol. J. Linn. Soc. 91, 475–482. doi: 10.1111/j.1095-8312.2007.00812.x
Kortet, R., Hedrick, A. V., and Vainikka, A. (2010). Parasitism, predation and the evolution of animal personalities. Ecol. Lett. 13, 1449–1458. doi: 10.1111/j.1461-0248.2010.01536.x
Pubmed Abstract | Pubmed Full Text | CrossRef Full Text | Google Scholar
Kortet, R., Rantala, M. J., and Hedrick, A. (2007). Boldness in anti-predation behavior and immune defence in field crickets. Evol. Ecol. Res. 9, 185–197.
Kortet, R., Vainikka, A., Janhunen, M., Piironen, J., and Hyvärinen, P. (2014). Behavioral variation shows heritability in juvenile brown trout Salmo trutta. Behav. Ecol. Sociobiol. 6, 927–934. doi: 10.1007/s00265-014-1705-z
Lafferty, K. D., and Morris, A. K. (1996). Altered behavior of parasitized killifish increases susceptibility to predation by bird final hosts. Ecology 77, 1390–1397. doi: 10.2307/2265536
Lai, Y.-T., Kekäläinen, J., and Kortet, R. (2013). Male ornamentation in the European minnow (Phoxinus phoxinus) signals swimming performance. Ethology 119, 1077–1085. doi: 10.1111/eth.12167
Lai, Y.-T., Kekäläinen, J., Taskinen, J., and Kortet, R. (2012). Non-invasive diagnosis for Philometra ovata (Nematoda) infection in the common minnow Phoxinus phoxinus. Parasit. Res. 111, 2411–2418. doi: 10.1007/s00436-012-3099-z
Pubmed Abstract | Pubmed Full Text | CrossRef Full Text | Google Scholar
Lessells, C. M., and Boag, P. T. (1987). Unrepeatable repeatabilities: a common mistake. Auk 104, 116–121. doi: 10.2307/4087240
Luttbeg, B., and Sih, A. (2010). Risk, resources and state-dependent adaptive behavioral syndromes. Philos. Trans. R. Soc. Lond. B Biol. Sci. 365, 3977–3990. doi: 10.1098/rstb.2010.0207
Pubmed Abstract | Pubmed Full Text | CrossRef Full Text | Google Scholar
Maximino, C., de Brito, T. M., de Mattos Dias, C. A. G., Gouveia, A. Jr., and Morato, S. (2010). Scototaxis as anxiety-like behavior in fish. Nat. Protoc. 5, 221–228. doi: 10.1038/nprot.2009.225
Pubmed Abstract | Pubmed Full Text | CrossRef Full Text | Google Scholar
McElreath, R., Luttbeg, B., Fogarty, S., Brodin, T., and Sih, A. (2007). Evolution of animal personalities. Nature 450, E5–E6. doi: 10.1038/nature06326
Pubmed Abstract | Pubmed Full Text | CrossRef Full Text | Google Scholar
Niemelä, P. T., Vainikka, A., Forsman, J. T., Loukola, O. J., and Kortet, R. (2013). How does variation in the environment and individual cognition explain the existence of consistent behavioural differences? Ecol. Evol. 3, 457–464. doi: 10.1002/ece3.451
Pubmed Abstract | Pubmed Full Text | CrossRef Full Text | Google Scholar
Niemelä, P. T., Vainikka, A., Hedrick, A. V., and Kortet, R. (2012). Integrating behaviour with life history: boldness of the field cricket, Gryllus integer, during ontogeny. Funct. Ecol. 26, 450–456. doi: 10.1111/j.1365-2435.2011.01939.x
Poulin, R. (2013). Parasite manipulation of host personality and behavioural syndromes. J. Exp. Biol. 216, 18–26. doi: 10.1242/jeb.073353
Pubmed Abstract | Pubmed Full Text | CrossRef Full Text | Google Scholar
Réale, D., Reader, S. M., Sol, D., McDougall, P. T., and Dingemanse, N. J. (2007). Integrating animal temperament within ecology and evolution. Biol. Rev. 82, 291–318. doi: 10.1111/j.1469-185X.2007.00010.x
Pubmed Abstract | Pubmed Full Text | CrossRef Full Text | Google Scholar
Santos, E. G. N., and Santos, C. P. (2013). Parasite-induced and parasite development-dependent alteration of the swimming behavior of fish hosts. Acta Tropica 127, 56–62. doi: 10.1016/j.actatropica.2013.03.008
Pubmed Abstract | Pubmed Full Text | CrossRef Full Text | Google Scholar
Seppälä, O., and Jokela, J. (2008). Host manipulation as a parasite transmission strategy when manipulation is exploited by non-host predators. Biol. Lett. 4, 663–666. doi: 10.1098/rsbl.2008.0335
Pubmed Abstract | Pubmed Full Text | CrossRef Full Text | Google Scholar
Shaw, J. C., Korzan, W. J., Carpenter, R. E., Kuris, A. M., Lafferty, K. D., Summers, C. H., et al. (2009). Parasite manipulation of brain monoamines in California killifish (Fundulus parvipinnis) by the trematode Euhaplorchis californiensis. Proc. R. Soc. B 276, 1137–1146. doi: 10.1098/rspb.2008
Pubmed Abstract | Pubmed Full Text | CrossRef Full Text | Google Scholar
Shirakashi, S., and Goater, C. P. (2002). Intensity-dependent alteration of minnow (Pimephales promelas) behavior by a brain-encysting trematode. J. Parasit. 88, 1071–1074. doi: 10.1645/0022-3395(2002)088[1071:IDAOMP]2.0.CO;2
Pubmed Abstract | Pubmed Full Text | CrossRef Full Text | Google Scholar
Shirakashi, S., and Goater, C. P. (2005). Chronology of parasite-induced alteration of fish behaviour: effects of parasite maturation and host experience. Parasitology 130, 177–183. doi: 10.1017/S0031182004006432
Pubmed Abstract | Pubmed Full Text | CrossRef Full Text | Google Scholar
Sih, A., Bell, A. M., Johnson, J. C., and Ziemba, R. E. (2004). Behavioral syndromes: an integrative overview. Q. Rev. Biol. 79, 241–277. doi: 10.1086/422893
Pubmed Abstract | Pubmed Full Text | CrossRef Full Text | Google Scholar
Sih, A., Cote, J., Evans, M., Fogarty, S., and Pruitt, J. (2012). Ecological implications of behavioural syndromes. Ecol. Lett. 15, 278–289. doi: 10.1111/j.1461-0248.2011.01731.x
Pubmed Abstract | Pubmed Full Text | CrossRef Full Text | Google Scholar
Sinn, D. L., Moltschaniwskyj, N. A., Wapstra, E., and Dall, S. R. X. (2010). Are behavioral syndromes invariant? Spatiotemporal variation in shy/bold behaviour in squid. Behav. Ecol. Sociobiol. 64, 693–702. doi: 10.1007/s00265-009-0887-2
Stamps, J. A. (2007). Growth-mortality tradeoffs and personality traits in animals. Ecol. Lett. 10, 355–363. doi: 10.1111/j.1461-0248.2007.01034.x
Pubmed Abstract | Pubmed Full Text | CrossRef Full Text | Google Scholar
Stamps, J., and Groothuis, T. G. (2010). The development of animal personality: relevance, concepts and perspectives. Biol. Rev. 85, 301–325. doi: 10.1111/j.1469-185X.2009.00103.x
Pubmed Abstract | Pubmed Full Text | CrossRef Full Text | Google Scholar
Vainikka, A., Rantala, M. J., Niemelä, P., Hirvonen, H., and Kortet, R. (2011). Boldness as a consistent personality trait in the noble crayfish, Astacus astacus. Acta Ethol. 14, 17–25. doi: 10.1007/s10211-010-0086-1
Weiss, A., and Adams, M. J. (2013). “Differential behavioral ecology. the structure, life history and evolution of primate life-history,” in Animal Personalities, eds C. Carere and D. Maestripieri (Chigago, IL: University of Chigago Press), 96–123.
Wolf, M., van Doorn, G. S., Leimar, O., and Weissing, F. J. (2007). Life-history trade-offs favour the evolution of animal personalities. Nature 447, 581–585. doi: 10.1038/nature05835
Pubmed Abstract | Pubmed Full Text | CrossRef Full Text | Google Scholar
Keywords: activity, boldness, exploration, parasitism, predation, personality, Diplostomum phoxini
Citation: Kortet R, Sirkka I, Lai Y-T, Vainikka A and Kekäläinen J (2015) Personality differences in two minnow populations that differ in their parasitism and predation risk. Front. Ecol. Evol. 3:9. doi: 10.3389/fevo.2015.00009
Received: 12 November 2014; Accepted: 17 January 2015;
Published online: 09 February 2015.
Edited by:
Ann Valerie Hedrick, University of California, Davis, USAReviewed by:
Pim Edelaar, University Pablo de Olavide, SpainJudy Stamps, University of California, Davis, USA
Copyright © 2015 Kortet, Sirkka, Lai, Vainikka and Kekäläinen. This is an open-access article distributed under the terms of the Creative Commons Attribution License (CC BY). The use, distribution or reproduction in other forums is permitted, provided the original author(s) or licensor are credited and that the original publication in this journal is cited, in accordance with accepted academic practice. No use, distribution or reproduction is permitted which does not comply with these terms.
*Correspondence: Raine Kortet, Department of Biology, University of Eastern Finland, Yliopistokatu 7, PO Box 111, FI-80101 Joensuu, Finland e-mail:cmFpbmUua29ydGV0QHVlZi5maQ==