- 1Departament de Filologia Catalana, Centre de Lingüística Teòrica, Universitat Autònoma de Barcelona, Barcelona, Spain
- 2Departamento de Filología Española, Universidad de Oviedo, Oviedo, Spain
It is a widely shared opinion among specialists that language is an evolutionary innovation, or that it contains some key evolutionary innovations. However, such claims are not based on a correspondingly consensual concept of “evolutionary innovation,” but are rather expressed on atheoretical grounds. This fact has thus far acted as an obstacle for the collaborative effort upon which the task of disentangling the evolution of this human capacity should be built. In this paper, we suggest a formal approach to the issue, based on Wagner's recent theory of homologies and novelties. Within this new framework, we conclude that language is the human instantiation (thus an “homolog”) of a character widely represented in the nervous system of animals, which incorporates a number of interdependent innovative states that allows us conceptualizing it as a “variational modality” of this ancient organ.
Maybe taking this message at heart can alleviate the frustration of those among us that feel that too much arbitrariness is involved in the current evolutionary-psychological debates.
Callebaut (2005, p.21)
Introduction
Language evolution is a lively research topic, regarding which many diverging views coexist that mostly differ in the role they ascribe to natural selection in the evolutionary shaping of the faculty of language (FL). Contrasting to this point of disagreement, a more consensual idea within the field is that the FL is a novelty with no clear correlates in non-human organisms. We however believe that such a stance is almost meaningless, for it has never been seriously grounded on a theory of evolutionary innovations. This paper is aimed at shedding some light on this issue, mostly taking advantage of Wagner's recent theory of homologies and evolutionary innovations (Wagner, 2014).
Building upon prior reconstructions of the FL as a system of natural computation plus an associated array of interfaces with other cognitive systems (Hauser et al., 2002; Balari and Lorenzo, 2013), we argue that language only qualifies as an evolutionary novelty in Wagner's weakest sense: i.e., as a Type II novelty, which means that it does not purport the introduction of a new organ—or Type I novelty. The FL, according to the view put forward here, derives from the evolution of new “states” of computational power and connectivity of a character—the Central Computational Complex (CCC)—whose “identity” largely predates its human instantiation. In any event, language comprises such a characteristic display of states that it may also qualify as a “variational modality” within Wagner's framework: i.e., a developmentally rather removed set of character states relatively to its homologs—comparable, for example, to the case of the tetrapod limb relatively to the teleost fin.
After reviewing some recent proposals as to what kind of innovation language is supposed to be, the paper next explores and justifies the identity of the ancient character of which language is hypothetically deemed a particular homolog. According to Wagner's theory, this task entails deciphering a particular “character identity network,” understood as a system of interactive gene-related resources that interfaces between inductive signals providing positional information, on the one hand, and downstream realizer genes in charge of shaping the different homologs of the character, on the other hand. While it may seem premature to speculate on such a level of analysis regarding the CCC, we nevertheless try to gather and interpret some relevant information supporting our identity claim. We then present some candidate homologs of language within this hypothetical framework and advance some suggestions aimed at substantiating the idea of grouping them into different variational modalities in Wagner's sense. We hope the conclusions of this paper to be illustrative of the important role that language may play in helping to fine-tune a general theory of evo–devo.
The Problem of the Continuity Problem
The idea that there is an evolutionary gap between language and every other aspect of non-human cognition and behavior is almost consensual within the field of expertise currently known as biolinguistics (Boeckx and Grohmann, 2013). The stance entails that language is customarily seen as a distinctly human capacity that raises a “continuity problem” (Chomsky, 1968; Bickerton, 1990) and thus defies a normal evolutionary conceptualization as a modified descendant from other more or less similar cognitive/behavioral traits. In any event, alternative positions exist that are not entirely uniform in this respect, for some representative figures contend that language is “new” from root to branch (Pinker and Jackendoff, 2005), while some others are prepared to admit that there coexist in language shared and non-shared components (Hauser et al., 2002). So a better way of synthesizing the current consensus around this issue is that language incorporates at least some key radical innovations, which most researchers agree to locate in its compositional components—prominently in syntax, which endows the faculty with its open-ended expressive power.
The main concern of this paper has to do with this particular aspect of evolutionary linguistics, for contrarily to, for example, the question of the role of selection in language evolution (Bickerton, 2013), most scholars concur in regarding language—or parts thereof—as new irrespective of any theoretical account of what is and what is not “new” biologically speaking—thus on purely intuitive grounds. A dramatic illustration of this point is provided by the debate in 2005 among Pinker and Jackendoff, on the one side, and Hauser, Chomsky and Fitch (HCF), on the other, most of which revolved around the boundaries between what is old and what is new in language, an assumedly significant point for both parts in order to recruit the best suited evolutionary explanation(s) to their case (Fitch et al., 2005; Jackendoff and Pinker, 2005; Pinker and Jackendoff, 2005). To say the least, the discussion was as acrimonious as unproductive, for contenders were clearly using completely different and non-standard concepts of novelty. Let us elaborate on this a bit more, seeing how the question developed once the dust settled after this squabble.
In what follows we will focus on some spin-offs of the HCF (2002) paper, for alternative proposals developed within a more traditional adaptationist-geneticist logic typical of evolutionary psychology or behavioral ecology (along the lines of Pinker and Bloom, 1990 or Pinker, 2003) appear to have languished (Bolhuis and Wynne, 2009; Bolhuis et al., 2011). As for the alternative view, its current position could be characterized by its emphasis on two main, interconnected themes: (i) a streamlined characterization of the “language phenotype,” and (ii) a radicalization of its uniqueness claims with respect to its allegedly core component (see below), with the net effect of neutralizing any attempt to look for its precursors in other animal species. We will deal with both issues in turn.
Point (i) is important because it represents, at least, a minimal consensus view as to the organic nature of language in contradistinction to other perspectives, where language is attributed the ontological status of an external entity that somehow interacts with the appropriate biological structures. According to the alternative perspective developed in this paper, the language phenotype is a particular functional system internal to the human brain, the specific localization of which is however not consensually agreed upon by specialists. Here we will roughly characterize it as a pallio-stratal-pallial system that interfaces in characteristic ways with other peripheral brain systems, ultimately bringing about different forms of linguistic behavior. In any event, the phenotype of concern must be detached from this outer level of observable behavior, for the latter also relies on historical grammatical traditions, stereotyped patterns of speech acts, etc., which are not particularly relevant for the task of disentangling the biological identity of the former. The generic architectural properties of the language system according to that view are illustrated in Figure 1 (Berwick et al., 2012, 2013; Bolhuis et al., 2014).
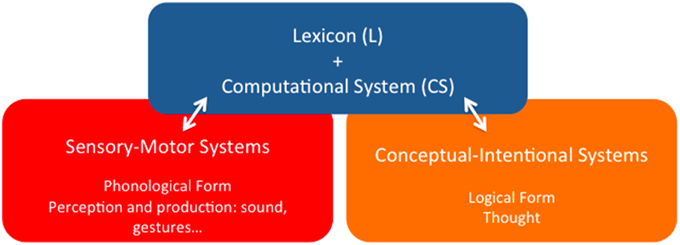
Figure 1. The language phenotype: a minimalist reduction of language to its bare architectural essentials.
It goes without saying that this is not a neuroanatomical characterization of the linguistic phenotype, but just an identification of its main functional components, which is nonetheless a step forward to overcome one of the main complicating factors in disentangling language evolution, namely that “there is [… ] no consensus regarding the essential nature of the language phenotype” (Bolhuis et al., 2014, p. 1). We share with these authors that in order to circumscribe the “character identity” of language—an obvious prerequisite to progress in explaining its evolution, the task must be undertaken of reducing it to its bare essentials (Balari and Lorenzo, 2013). Such an enterprise purports, according to this perspective, capturing the basic architectural organization that defines language irrespective of its particular socio-cultural instantiations—English, Chinese, Italian, and so on, and also capturing what kind of activity can be assigned to it, now abstracting away from the categories and activity of other language-associated, but not specifically linguistic cognitive systems—motor planning and execution, perceptual categorization, conceptual representation and intention fixing, etc. As we pointed out, a certain consensus exists about the idea that adopting this minimalist position (Chomsky, 1995) boils down to assuming a rather simple architecture that comprises the three basic components displayed in Figure 1:
(i) A finite inventory of primitive sound/meaning pairings—Lexicon (L);
(ii) A combinatorial engine capable of composing an open-ended number of expressions by continuously redeploying lexical units and previous outputs of the engine itself—Computational System (CS); and
(iii) Two interfaces with the sensory-motor and conceptual-intentional systems of the mind/brain, where expressions are respectively interpreted as instructions to the peripheral anatomy associated to different channels of externalization—oral, gestural, and so on, and as vehicles for thought.
Within this restrictive framework, special attention is due to the first (L) and second (CS) components (the blue square in Figure 1), for they are the more persistently pinpointed as supporting claims of uniqueness. For example, Bolhuis et al.(2014, p. 1) promptly claim that language “has no equivalent in any non-human species,” and, a few paragraphs below, add that “before the appearance of merge, there was no faculty of language as such, because this requires merge along with the conceptual atoms of the lexicon” (Bolhuis et al., 2014, p. 2), where “merge” is the term customarily reserved within this tradition to refer to the basic operation in charge of building complex linguistic objects. Thus, in sharp contrast to HCF's original mild speculations suggesting the possibility that all three main components of the linguistic phenotype might have precursors in some both extinct and extant non-human species (Hauser et al., 2002, p. 1578), the uniqueness claim appears to have become an untouchable a priori of sorts, to which views on language must of necessity accommodate (Boeckx, 2013a, p. 8, for a brief history of how the words “unique” and “specific” eventually crept into the minimalist official discourse).
Summing up so far, HCF (2002) has eventually yielded to a mosaic view of language (Boeckx, 2013b) in which a number of different components interact to give rise to the full richness and complexity observed in today's human languages. This view, moreover, while not denying that, at least functionally, language works as a unit, contends that only some of its parts may be shared with other species, while others (notably the CS and plausibly also L) constitute true evolutionary novelties. The emergence of the latter is what would explain the “cognitive gap” between humans and the rest of non-human animals.
The following pages are aimed at shedding some light into a number of complex questions posed by this conception of language, starting with a non-trivial aspect of it: Namely, does language actually qualify as the type of entity to which such considerations legitimately apply? In other words, is it really a (form/function) unit at the morphological level of analysis—i.e., an organ? Only after this question is satisfactorily answered may one proceed to the crucial question whether it is—or in which specific sense it is—an old or a new organic component of the human brain. What is clear from the start is that none of these questions can be confronted in a theoretical vacuum, as they belong to a delicate matter that must be faced respecting all the standards of current biological thought, so let's turn to evolutionary novelties.
What is New about Evolutionary Novelties?
In a sense, every component part of an organism is a novelty, for from the perspective of the present and looking back to its evolutionary past there must be some prior point at which no such component existed. From this general standpoint, it may seem trivial to ask whether this or that organ is biologically innovative. They all unexceptionally are (Wagner, 2011, p. 1). The issue becomes however far from trivial when a more particularized perspective is adopted, so instead of the previous question one may rather ask at which specific point in evolution an organ of concern represented a biological novelty. Note that the issue is thus inextricably linked to the also non-trivial one of establishing homological classes (Müller and Wagner, 2003, p. 221), i.e., collections of organic structures that one may confidently conclude instantiate the same organ in different plants or animals—or in different locations of the same plant or animal, as it seems reasonable to hold the position that evolutionarily speaking such an organ was innovative just once (Wagner, 2014, p. 417, for the conceptualization of apparent counterexamples). So for the best or the worst, the fate of unveiling biological novelties goes clearly hand in hand with the ability of discerning homological relations among series of organic structures. As a matter of fact the link is a definitional one, as nicely captured in the following (definitional) statement by Müller and Wagner:
Definition: A morphological novelty is a structure that is neither homologous to any structure in the ancestral species nor homonomous to any other structure of the same organism. (Müller and Wagner, 1991, p. 243; emphasis in the original)
The good news about all these concerns is that “homology” is a concept relatively easy to grasp intuitively and has served as the grounds of the comparative approach to nature since the late eighteenth and early nineteenth centuries—despite the intermittency due to its neglect by the Darwinian Synthesis (Amundson, 2005, p. 103; Wagner, 2014, pp. 25–26); the bad news is that discerning homologs becomes a much more controversial issue when one tries to operationalize the concept on mechanistic grounds and to apply it to particular instances. Let us briefly review both sides of the matter.
Conceptually speaking, the most agreed upon definition of the homology concept is due to Richard Owen and dates back to 1843: “The same organ in different animals under every variety of form and function” (Owen, 1843, p. 379). So defined, the concept thus refers to a kind of natural identity that is not disturbed by the specializations that the corresponding structures may show in agreement with their common uses or by other formal peculiarities. Take as a suitable example the identity that is supposed to exist between the appendages of the second thoracic segment of flies, beetles, or butterflies, despite the fact that it adopts the form of a wing in the first and third orders and the form of a protective elytron in the second one, and also despite the noticeable differences between insect wings along different axes—shape, color, etc. Note that conversely, the definition above also entails that similarities in terms of form and function do not automatically qualify as evidence for homology. For example, it is not the case that the wings of bats are homologous to insect wings, despite their involvement in the corresponding species' flight and despite certain shared aerodynamic properties. If anything, the wings of bats are putative homologs of insect limbs—together with other vertebrate limbs (Shubin et al., 2009), although this is an open question and one subject to subtle discussions—see below for some comments. For the time being, suffice it to conclude that correspondingly to these quite consensual ideas on the homology concept, an associated concept of “novelty” or “innovation” should also not rely on formal or functional details, but should refer instead to the emergence of character identities, underlying and embracing divergences-to-be in both formal and functional terms. Such a conclusion obviously deserves some elaboration.
The previous paragraph offers some grounds for choosing among recent proposals concerning what is and what is not new from a biological perspective. In particular, Wagner's (2011) suggestion, following a tradition that may be traced back to Mayr (1960), is clearly a functional one, in that it mostly relies on the benefits that changes may bestow upon their bearers as well as on the opportunities for new extra-benefits that they may open to these bearers' descendants. Andreas Wagner's definition of “innovation” reads as follows:
A new feature that endows its bearer with qualitatively new, often game-changing abilities. These may not only mean the difference between life and death in a given environment (just think of biosynthetic abilities), they may also create broad platforms for future innovations, as did the innovations of photosynthesis and of complex nervous systems (Wagner, 2011, p. 1).
We are not suggesting that Andreas Wagner's position is right away wrong. As a matter of fact, what we actually think is that his observations are aimed at a level of analysis different from the level at which identity statements must be attached in application of the homological method proper. For this reason, it may be useful to keep the term “innovation” to that former level—however unclear it is even in functional terms (Wouters, 2003; Love, 2007), and to specialize the term “novelty” instead—as in Wagner (2014)—to name the kind of basic organic identities that underlie prospective adaptive diversifications in Andreas Wagner's sense. From now on we will respect this terminological distinction (Müller and Wagner, 2003, p. 219; Erwin and Krakauer, 2004; Minelli and Fusco, 2005, p. 521; Brigandt and Love, 2010).
From a formal point of view, while it is true that variation along different axes (shape, color, tissue specialization, patterns of correlations of parts, and so on) can be discerned in less intuitive grounds than variants of function, it is also clear that it may be so without altering the underlying identity of the variants so defined. This is how Mayr introduced the issue some half-century ago:
Most evolutionary changes take place without the origin of new structures. Even when we compare birds or mammals with their strikingly different reptilian ancestors, we are astonished at how few are the truly new structures. Most differences are merely shifts in proportions, fusions, losses, secondary duplications, and similar changes which do not materially affect what the morphologist calls the “plan” of the particular type (Mayr, 1960, p. 358).
The point has been newly raised and in our opinion satisfactorily conceptualized by Wagner, on the grounds of a well-motivated distinction between the concepts of “character identity” and “character state” (Wagner, 2014, pp. 51–54). For example, the fins of teleost fishes consist of four radials attached to the shoulder girdle plus a complex of dermal bones/rays, while the limbs of tetrapods consist of one bone attached to the body and a set of two distal bones plus a number of endochondral long bones/digits. Notwithstanding all these differences, a wide and old consensus exists around the idea that fish fins and tetrapod limbs are but variants of the same organ: Namely, the vertebrate limb (Owen, 1849). So according to Wagner's distinction, the traits above are different states that the character of concern adopts in different organic scenarios. In his own words, “states are still variants of the same thing” (Wagner, 2014, p. 71), where “thing” is a loose manner of referring to a character, the identity of which underlies its manifold actual displays. Turning to our particular concern with evolutionary novelties, a corollary of Wagner's ideas is that in parallel to the “identity/state” distinction a two-level model of novelties can be entertained, for novelties may reasonably purport the emergence of new identities—i.e., brand new organs, or the emergence of new states of pre-existent identities—i.e., innovative variants (Wagner, 2014, pp. 126–127). Wagner refers to the former as “Type I” novelties—e.g., the vertebrate limb (Wagner, 2014, Chap. 10), and as “Type II” novelties to the latter—e.g., the tridactyl limb, as opposed for example to the prototypal pentadactyl variant (Wagner, 2014, Chap. 11). A further related category introduced in Wagner (2014) tries to accommodate the fact that certain Type II traits characteristically appear in clusters, so none of them is as a matter of fact accessible from an alternatively similar cluster—e.g., the bundle of traits that respectively define and jointly oppose fish fins and tetrapod limbs (Wagner, 2014, Chap. 10). Wagner habilitates the “variational modality” concept to the particular aim of referring to these complex arrangements of Type II novelties (Wagner, 2014, p. 63).
Clearly enough, even if the categories and distinctions introduced so far have a direct intuitive appeal, they do not suffice by themselves to ground the most urgent kinds of questions that a homological theory should be ready to answer. For example: Why fish fins and tetrapod limbs are true homologs, instead of similarly organized but different organs—say, analogs? Does this particular homology relation extend to insect limbs? Etc. The more ready a homological theory proves to be to answer these and other similar questions on mechanistic grounds, the better positioned it will be as an accurate account of natural identities. The most promissory theories thus far point to development as the right soil on which such an account must be built (Moczek, 2008). Let us briefly explain why and how.
Wagner contends that the conceptual distinction between “character identity” and “character states”—and thus of “Type I” and “Type II” novelties—has empirical correlates in the mechanisms that govern the development of the different morphologies that compose a body. In a nutshell, Wagner's claim boils down to the idea that components governing the “identity” of a particular morphology, on the one hand, and the “states” that such a morphology specifically displays, on the other hand, can be disentangled at that mechanistic level, for which he devotes the terms “character identity networks” (ChINs) and “realizer genes,” respectively (Wagner, 2014, Chap. 3). According to Wagner's characterization, ChINs are reiterative patterns of interaction between genetic sequences (and products thereof), which interface between the positional information provided by inductive signals, on the one side, and downstream realizers in charge of character states differentiating among collections of homologs, on the other side. Experiments in which certain point mutations lead to the loss of a character identity (e.g., the identity of the hind wing in the case of Ubx mutants of flies, beetles, and butterflies) may be interpreted in a way congenial to Wagner's model, for what shows up instead of the wild condition is an alternative character identity (e.g., the one of the respective forewings) (Deutsch, 2005; Wagner, 2014, p. 127).
From the point of view of the novelty issue, Wagner's elaboration of the previous ideas purports that the origins of new ChINs—i.e., core regulatory machineries, and the origins of realizer genes—i.e., their subordinate genetic sequences and products—must be correspondingly disentangled. Obviously enough, when a particular ChIN originates, it must be already in charge of one or another set of realizer genes, but this is not particularly challenging for the theory. In this respect, a particularly illuminating case is offered by the origins of the tetrapod limb, the prototypal instantiation of which is a pentadactyl limb (Wagner, 2014, Chap. 11). Actually, this variant is the one to which limbs tend to recede in pathological or experimental conditions; but the curious fact is that according to the paleontological record it was not the first to exist, as variants with more than five digits are the most frequent as we move back to the putative origins of the character. These kinds of situations seem to support the autonomy claim between identities and states, as it clearly shows that the former remain constant irrespective of historical changes, which may include the fixation of resilient realizations acting as buffers against natural or artificially induced stress conditions. What we actually observe is thus that a character is ultimately a complexly multifaceted entity, comprising an underlying identity associated to a more or less wide spectrum of common realizations, eventually including cryptic variants to which characters may roll down given unexpected conditions. The fact that claims of origins regarding each of these facets can be sustained independently of the others is a strong piece of support to such ontological claims and reinforces, in our opinion, the combinatorial approach to homology advocated for by Minelli (Minelli, 1998, 2003; Minelli and Fusco, 2005), of which Wagner's approach may be seen as a reformulation—albeit not unproblematically. Figure 2 summarizes Wagner's model.
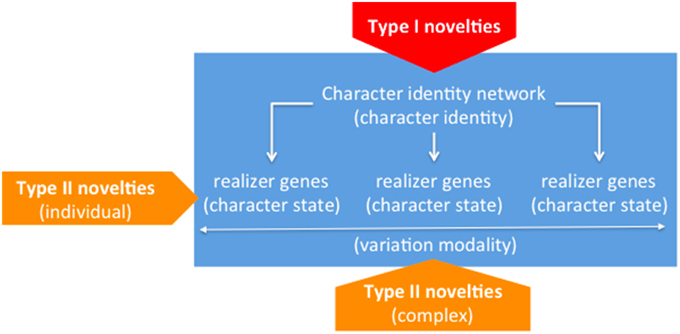
Figure 2. Constitutive dimensions of characters (“identity” vs. “states/modalities”) and underlying generative mechanisms (“ChINs” vs. “realizers”). Within pentagons, novelty types associated to the corresponding loci (“Type I” vs. “Type II”).
Let us turn now to the kinds of specific challenges that the theory of homology/novelty thus far introduced should be ready to answer. According to it, the traditional tenet that fish fins and tetrapod limbs are true homologs, for example, should be grounded on shared patterns of interactive activity between genetic sequences and products. Actually, the prediction seems to be borne out that a character identity network is conserved in the development of shark and skate pectoral fins, teleost medial and paired ray-fins, and tetrapod limbs, despite differences in the respective skeletal elements. Crucial to the development of all three structures is the early configuration of a structure known as fin/limb bud, which derives from a self-sustaining loop of Fgf8 expression between the epidermis and the mesenchyme, induced by other fibroblast growth factors (Fgfs)—mostly Fgfs10—and Wnt molecules acting as signals. From that point on, a spatial pattern of development is in place, controlled by signals that determine the three main axes of the fin/limb bud (proximodistal, dorsal-ventral, and anterior-posterior) in a rather integrative manner. For example, the proximal-distal axis depends on signals from the apical epidermal ridge (AER) of the bud, while the anterior-posterior crucially depends on Sonic hedgehog (Shh) molecules present in a zone of polarizing activity (ZPA) located on the posterior ridge of the bud. Besides, Shh expression in the ZPA is maintained by Fgf signaling from the AER, which in turn is dependent on Shh to maintain Fgf4 activity in AER. As for the third axis, two differentiated compartments of activity are observed: The dorsal one, where Wnt7a expression signals the underlying mesenchyme by activating the Limx1 transcription factor, and the ventral one, where the transcription factor En1 prevents Wnt7a expression, thus creating the corresponding axis asymmetry (Figure 3). These are, in a nutshell, the core components of a conserved chain of genetic activity that determines the underlying identity of fin/limbs across vertebrate species—for more detailed accounts, see Wagner (2014, pp. 341–347) and particularly Gilbert (2014, pp. 489–518). According to the theory, the organ resulting from the evolutionary emergence of this particular ChIN is a Type I novelty, irrespective of the particular states that it originally displayed and the subsequent specializations that it acquired in different classes and parts of animals.
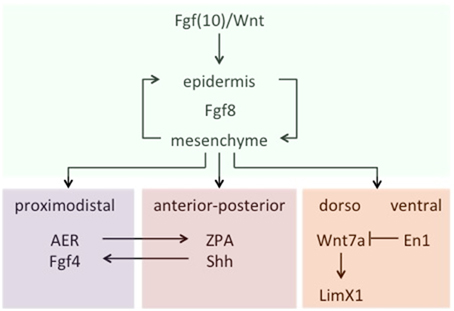
Figure 3. A schematic (partial) representation of the fin/limb ChIN. Data from Wagner (2014, 341ff).
Is this fin/limb ChIN also associated to insect limbs? Apparently not, which obviously runs against the idea of an across-the-board (or deep) homologization of the corresponding structures, along the lines of Shubin et al. (1997, 2009)—or historically, of Étienne Geoffroy Saint-Hilaire. It is a well-attested observation that common genetic sequences and patterning mechanisms express in all these cases—for example, distal activity of Fgf signaling or posterior expression of Shh. Nevertheless, it is important to take into consideration that the recruitment and reuse of the same or similar developmental resources is more the norm than the exception in very different organisms and structures, implying that said resources are essentially unspecific regarding the constructive roles that they ultimately acquire in different organic contexts. Müller and Newman's (2005) “initiating conditions” may be helpful in this regard, for it purports that very unspecific and general causal agents have as a norm to pave the way to developmental innovations leading to bona fide novelties. According to them, such “remote” or “ultimate” causes bring into existence primordial units corresponding to what they refer to as “origination” processes, however devoid of the identity of characters proper—an example that comes to mind of such “leading up” event is “protruding,” in the sense of Shubin et al. (2009), for it is equally involved in the origins of different kinds of appendages. But assuming this, and contrarily to Shubin et al.'s preferred interpretation, the resulting structures are not necessarily homologs, even if sharing a deep background of developmental resources. In the case that specifically concerns us here, what still seem to be lacking are comparable patterns of interactive activity between the component pieces of a whole array of such developmental means, crossing the invertebrate/vertebrate divide. So if anything, similarities between the specific participants in limb development across the corresponding classes should more realistically be submitted to a different chapter of molecular convergence (Minelli, 1998; Moczek, 2008, pp. 437–438).
The conclusion is thereby reached that all vertebrate appendages (fins and limbs) are homologs—“serially,” in the same organism, and “specially,” in different ones, and that they individuate by means of regulatory genes specific to their position in different organismal contexts (Wagner, 2014, p. 345), maybe as to also cover the paramorphic status that Minelli (2000, 2003) ascribes to appendages as putative axial homologs of the main body axis. This claim entails that positional information provided by different kinds of signals also seems to be crucial for an organ to display a particular set of character states, and eventually a deeply interconnected (or “variational”) set thereof: i.e., one where the instantiation of a particular state strongly compromises the fate of development in the direction of some other state or some set of interconnected states—e.g., autopod formation, which acts as a suppressor of distal branching, thus directing development toward a digit-styled instead of a ray-styled pattern of termination.
Thus, the individuality of a given organ, both in terms of its identity and in terms of the states that it manifests across different organisms or within the same one, is according to the model deeply rooted in the genetic make up of its bearers, parts of which differentially react to the signals of concern leading the way to the corresponding cascades of developmental events. Novelties are therefore to be explored and decided at the corresponding levels of analysis, establishing how they ultimately deserve to be ranked in terms of type (i.e., “I/II”) or complexity (i.e., “individual states/variational modalities”). As for the case of language, the application of the model thus far presented depends on the preliminary question of deciding whether an organic individuality can be legitimately assigned to it, obviously enough not a trivial matter. Granted that it can—as we conclude in the next section, the door will be open to further evaluate whether it is a novelty and if so, in which particular sense it is. As we show in the following sections, the fact that verbal behavior is exclusively attested in the human species may be deceiving regarding such controversial issues.
One Character in Search of an Identity
The theoretical model of homology/novelty thus far presented purports that circumscribing the identity of a particular character entails reducing it, so to speak, to its bare essentials, which ultimately means: (i) to connect it with a level of developmental organization at which an organic core can be abstracted away from a periphery of divergent properties; and (ii) to unveil the genetic circuitry (ChIN) that correlates with the expression of such an elementary structure. The identification of ChINs and accordingly of homologs/novelties at the phenotypic level is thus the target at which the operationalization of the theory is obviously aimed. The model looks pretty straightforward in the case of a priori undisputed characters—e.g., fins, limbs, and so on, the proper location, limits, typical and deviant forms of which, etc., are not subject to relevant contestation.
Unfortunately, this is not the case of language. To be sure, as pointed out in Section The Problem of the Continuity Problem, some important steps in the right direction have already been taken, with the identification of the basic components of the linguistic phenotype. Recall, however, that this characterization implies just a break down of language into its elementary functional components, while, crucially, it is as yet far from being an accurate specification of the character in organic terms. Accordingly, the granularity problem, as formulated by Poeppel and Embick (2005) is still an issue of concern, in spite of the fact that the gap between linguistics and neuroscience has become much narrower. Besides, as also noted in Section The Problem of the Continuity Problem, consensus as to what the basic functional components of the linguistic phenotype are has not come together with consensus with respect to the evolutionary status of these components, although opinion appears to have stabilized around the idea that most, if not all, of these components are true novelties, with the possible exception of the vocal-auditory externalization system, which might contain both homologous and analogous elements with that of birds (Bolhuis et al., 2010; Berwick et al., 2011, 2012). In this section we would like, firstly, to examine the arguments proposed so far for considering language (both as a whole or only partially) a true evolutionary novelty in order to show that they are largely unwarranted; secondly, we would like to offer an alternative, more nuanced view, arguing that language is an evolutionary novelty, but only of the Type II kind in Wagner's (2014) sense, and accordingly one for which an analysis in terms of homology is entirely suitable and appropriate.
The Quest for “Humaniqueness”
The uniqueness of language is generally presented as an a priori assumption, which is nonetheless typically complemented by a number of a posteriori arguments. These arguments are concocted by incorporating different types of evidence coming from such disparate areas of research as paleontology and archeology, molecular biology, neuroscience, and comparative behavioral science, but these pieces of evidence are generally put forward to buttress what may be considered the central element of the argumentation and what we will call here the “complexity argument.” The argument is based on a fairly transparent inference:
(i) The formal analysis of natural language expressions reveals that they present a high degree of structural complexity only computable by a device with the appropriate level of computational power;
(ii) No animal behavior, especially of the communicative kind, seems to possess a structural complexity comparable to that of natural language expressions;
(iii) Therefore, the computational system subserving the acquisition and use of language is a unique character with no homologs in the natural world.
In other words, the rationale underlying this position is that expressions constructed by means of this computational engine (CS of Figure 1) conform to patterns of internal organization that attain a level of formal complexity beyond anything known in other sequentially organized non-human observable behaviors, thus favoring the thesis of “human uniqueness” or “humaniqueness” (Hauser, 2009). In this respect, the most commonly used tool to assess structural complexity is formal language theory (FLT), through which specific patterns may be identified and classified according to the Chomsky Hierarchy of languages. In the technical sense of the term reserved to FLT, a “language” is just a set of strings of symbols arranged following a pattern describable by a collection of rules (a “grammar”) obeying a characteristic number of constraints that determine the types of structural patterns that may be present in the language. Complementary to the notion of “grammar,” the notion of “automaton” refers to an abstract computational device capable of generating a language by recursive application of the rules making up the grammar for that language. Since grammars and automata are equivalent devices, we have a more or less direct translation of the constraints imposed over the grammar rules to the memory resources an automaton needs to compute a string in a given language. Informally, the Chomsky Hierarchy may then be seen as containing the three following linguistically relevant levels of complexity:
(a) Regular Systems: Only linear inter-symbol dependencies are allowed in strings. No external memory is required to compute a string.
(b) Context-Free Systems: Long-distance inter-symbol dependences are also allowed, so a further level of complexity is added relatively to (a). An external memory stack is required to compute a string.
(c) Mildly Context-Sensitive Systems: Dependences crossing discontinuous substrings are additionally allowed, increasing the complexity of strings relatively to (b). An external complex system of structured memory is required to perform the computation.
Languages may be further sub-classified within each type attending to their relative complexity (Rogers and Pullum, 2011), but the resulting (quantitative) sub-hierarchies are not particularly relevant to our concerns here. Note, however, that we use the term “Mildly context-sensitive system” already to indicate that no natural language pattern appears to require the full power of context-sensitive systems.
Patterns of the first type are typically observed in the phonology of natural languages, as in the arrangement of consonants and vowels in syllables or of syllables (and morphemes) within a word, which always obey strictly linear constraints. Before we move to the next levels of complexity, it is relevant to point out that not only are regular patterns typical of phonology, but they also seem to be exclusive of this level (Heinz and Idsardi, 2011, 2013), while higher levels of complexity are only observed in syntax. This observation will be of some importance later in this section.
The accessibility of language to context-free complexity is illustrated by syntactic embedding—as in (1); its accessibility to context-sensitivity is illustrated by such common syntactic phenomena as agreement or co-reference, for example—as in (2):
(1) [Mary said that [John will never change] very acidly]
(2) [Maryi said that [heri father will never change] very acidly]
Note that in (1) a dependency exists between the verb said and the adverb very acidly that is interrupted by the dependency existing between the verb and the intervening clause in square brackets, giving rise to the nested pattern typical of context-free systems. In (2), however, in addition to the verb-adverb dependency we have another one between the proper noun Mary and the pronoun her, yielding a crossed pattern, since the verb-adverb dependency is only resolved after the antecedent-pronoun dependency.
Now, these observations conform to a robust enough collection of facts about the structural complexity of human languages that define a frame of reference against which the complexity of other cognitive abilities, human and non-human, may be assessed (Fitch and Friederici, 2012; see however Boeckx, 2013a; Benítez-Burraco and Boeckx, 2014, for some skeptical remarks). To be sure, it is from comparative evidence of this kind that most uniqueness claims come, inasmuch as when FLT is applied to assess, for example, the communicative behavior of birds or monkeys, nothing beyond linear regular patterns is actually observed (Berwick et al., 2011, 2012; Filippi, 2014; Hauser et al., 2014). Thus, on the one hand, when we look at birdsong we see that songs are complex arrangements of motifs or tunes combined following some easily deducible rules, which nonetheless never transcend the structural complexity of a regular system; moreover, nothing in the songs of birds suggests that motifs are conveyors of semantic content or, to put it differently, that they behave as human words, with the ability, for example, of combining and forming hierarchical syntactic patters. On the other hand, a quick look at the vocalizations of monkeys and apes indicates that, while there may be some hints of semantics in them, this does not come together with the ability to combine them into complex vocalizations to produce new expressions. This evidence is customarily interpreted in the sense that what both birds and primates are lacking is a CS implementing a basic combinatory computational operation (typically referred to as merge) that is supposed to account for the full power of human language syntax and that makes it a unique character in the natural world (Berwick et al., 2012, 2013; Bolhuis et al., 2014; Watumull et al., 2014).
In our opinion, this interpretation is premature, for a number of reasons. Take, first, the definition of merge, which is assumed to be an operation with the power of building structure. For example, in a recent paper by Noam Chomsky we find the following definition (Chomsky, 2013, p. 40):
Merge (X, Y) = {X, Y}, where X and Y are syntactic objects.
Now, as already pointed out by Boeckx (2013c), merge as defined above cannot really be a structure building operation, but just an operation putting two things together to form a set. Accordingly, the product of merging two objects is a new object where no ordering or prominence is assigned to any of the objects. Merge combines objects, but it doesn't build structure around them. How exactly the latter is achieved is not something that should concern us here; let us just point out that there are two logical possibilities:
(i) Either merge is complemented with other operations; or
(ii) Structure building follows from some special property of the objects to which the operation is applied.
(i) Appears to be the option most favored by Chomsky (2013), who appeals to an operation of labeling to account for the inherent asymmetry of syntactic objects, i.e., the fact that the object {blue, book} has nominal properties, not adjectival ones. Option (ii) assumes that the asymmetry has something to do with a property of words that is preserved and carried over in further applications of merge (Boeckx, 2013c). In our opinion, at first blush (ii) appears to be the most parsimonious option, insofar as it favors a really minimal characterization of the CS (i.e., it performs just one type of operation). Thus, and leaving aside the question of what this specific property of words might be, we will make the preliminary assumption that the CS just implements a simple combinatory operation, which we will refer to as merge, but only as a generic term ranging over whatever operation of this kind may eventually be identified as the most appropriate to capture the actual performance of the CS (Hagoort, 2005, 2013, for some suggestions). Let us turn then to birdsong.
As noted above, birds' songs show combinatorial properties, although, as far as human observers can tell, their structure is not asymmetric and, therefore, also not hierarchical. Crucially, however, from this it does not follow that a bird's song cannot be the product of a simple combinatory operation like merge. From the fact that birdsong's structures just show linear, regular patterns it would only follow that either bird-merge is operating with a shortage of memory resources (unlike human-merge) or that the objects that bird-merge combines are fundamentally different from human words; or both. But even in this case, we could not rule out the possibility that the structural simplicity observed in birdsong is just an artifact of constraints imposed by the mode of production/perception, i.e., of the vocal-auditory channel. Recall that human phonetics/phonology is structurally as simple as birdsong and that only through the application of sophisticated tools of linguistic analysis it is possible to unveil the complexity of human syntax. Note that we are not claiming here that birds possess complex conceptual systems and that their productions may turn out to be as rich as human expressions; we are just claiming that by comparing the simplicity of birdsong with the complexity of human syntax one cannot infer the absence of a CS in birds comparable to that of humans, because the simplicity of birdsong might be an artifact just as the simplicity of human phonetics/phonology appears to be an artifact of the externalization channel.
Thus, in our opinion, the widely shared idea within modern generative linguistics that the border separating regular systems from context-free systems behaves as a Rubicon of sorts and that context-freeness acts as the evolutionary hallmark of language and the ultimate basis of its organic uniqueness is unwarranted. The point that we specifically want to raise here is that we are convinced that by embracing such a stance it is dubious that linguists will make much progress in clarifying the question that seems to ultimately motivate their evolutionary musings: Namely, has language really introduced a new “identity” into the organic world? It is our contention that connecting language with a particular degree of computational complexity is only tangential to the issue. For if anything, a certain complexity level is a possible character “state” among others of a putative organic trait: Namely, a CS with one or another level of complexity. So it is our conclusion that the question of how language connects—or eventually not—with the composition of other non-human organisms, a mandatory step in order to disentangle its evolution, is not satisfactorily answered by just locating it in a particular position within a scale of complexity, as exceptional as this position may happen to be.
An alternative better-grounded program for evolutionarily clarifying language should be built, according to our view, out of the following questions: What character identity can be accorded to language? Is it a new or a shared kind of identity? In other words, is language a Type I or a Type II novelty—eventually, a combination thereof?
Uniqueness Demoted
Let us reiterate again that once a minimalist reduction of language has been made, we certainly are in the position of starting to formulate serious hypotheses to answer such questions. For example, one may entertain the idea, given the obvious fact that sequentially organized forms of behavior apart from language are ubiquitous, that an organic component of brains exists—perhaps once a certain degree of organization is attained (Balari and Lorenzo, 2013, Chap. 8)—subject to interspecific variation along the following two main axes:
(i) A specific pattern of interface connections to other cognitive modules; and
(ii) A specific degree of computational complexity.
From now on, we will refer to this component as CCC—for Central Computational Complex, as in Balari and Lorenzo (2013), taking into consideration that CS just happens to be its core subcomponent, but not its only defining trait: Interfaces also matter (Hauser, 2009). So let us explore in some detail the idea in the remainder of this subsection, yet only to conclude that it is a well-motivated one. Note that if the idea is on the right track, as we believe, it is CCC—and not language—that should ultimately be deemed as a Type I novelty—with language just being a Type II elaboration, arguably a variational modality thereof. For the time being, let us concentrate on arguing that the idea that an organ of computation (CCC) exists is a well-supported one, using its human instantiation as a model system.
In this respect, the perspective provided by FLT proves to be crucial, for it establishes the bare requisites that an actual CS should fulfill in order to deal with language-related tasks. Before we go on, however, we would like to open a brief parenthesis in order to clarify the role of FLT in the evolutionary study of cognition, which is often controversial. Criticisms of the applicability of FLT are usually articulated along two different axes (Benítez-Burraco and Boeckx, 2014, for an overview):
(i) The relative failure of the Artificial Grammar Learning (AGL) paradigm, in particular with non-human subjects; and
(ii) The impossibility to characterize human language as occupying some well-defined region of the Chomsky Hierarchy.
As for AGL, it is an experimental paradigm aimed at assessing the learning abilities typically of non-human subjects by presenting them stimuli in some formal language incorporating structural patterns observed in natural languages, like, for example, nested dependencies (Fitch et al., 2012, and the papers that make up the special issue to which the cited article is an introduction). The very idea of AGL is, therefore, that if a subject is able to learn an artificial language presenting some specific structural pattern, then its CS may be at least capable of computing stimuli with that degree of complexity. The problem here lies in the difficulty of determining what it means exactly for a subject to have learned the grammar of the language, or, in other words, what the subject has learned exactly; this problem is particularly hard when non-human animal learners are involved (Rogers and Hauser, 2010; Heinz, 2014). Note that there is a crucial difference between the application of FLT in the AGL paradigm and the use we are making of it here: while AGL focuses on the possibility/impossibility of learning certain structural patters, we focus on the complexity of patterns already observable in a sequentially organized form of behavior. So, to the extent that the patterns are observed in the behavior in question, it is obvious that they had been learned (or developed) by the subject previously. Accordingly, whatever criticisms that may be raised against AGL are not applicable here, because learnability is not an issue.
As for point (ii) above, the problem mostly regards the fact that many logically possible patterns within the same degree of complexity have never been observed in any human language (Heinz and Idsardi, 2011, 2013); in other words, structural variation appears to be finite and FLT is not a tool precise enough to explain why certain possible patterns never become actual. Note that this problem is in many ways not too different from similar questions about variation faced by evolutionary developmental biology such as whether there is a pentadactyl ground plan among tetrapods (Wagner, 2014) and, we submit, it is likely that both deserve similar kinds of answers in terms of the canalization or fixation of certain developmental pathways but not others. Clearly, FLT is of limited use here, but this is not in our opinion a sufficient reason to consider it unworthy of serious consideration. Moreover, in a naturalistic interpretation of the Chomsky Hierarchy in terms of a “morphospace” of possible computational phenotypes (Balari and Lorenzo, 2008, 2013, Chap. 6), where real phenotypes tend to occupy the theoretical space in a patchy way, the alleged weakness rather seems to be a prediction, and one that happens to be borne out. In any event, the main point is that we think that FLT serves as a bridge toward explanations of the evolution of language taken as a bona fide organic object to the extent that FLT does provide the tools to establish the basic specifications of the generic computational operations that can plausibly be implemented in a neuronal circuit—a desideratum on which many neuroscientists, especially Poeppel, have recurrently insisted (Poeppel and Embick, 2005; Poeppel, 2011, 2012, 2014; Carandini, 2012; Embick and Poeppel, 2015). We can, in a nutshell, establish the minimal specification of the computational architecture of the CS as an organic character.
To be precise, such a device should be organized attending to a dual division of labor model, with a first component in charge of sequencing proper—“sequencer,” and a second one in charge of maintaining sequences in active memory for going-over concerns—“working memory.” It is one of our main claims that human brains contain one such articulated system. Well-established anatomical data can be alleged in support of the idea:
(i) Basal ganglia have been previously described as a “sequencing engine” (Lieberman, 2006, p. 169), with parts showing language-related specializations—namely, the dorsolateral prefrontal circuit (Cummings, 1993; Lieberman, 2006; Bonelli and Cummings, 2007);
(ii) Cortical circuits serving as working memory loops are also well-known (Aboitiz et al., 2006), with Broca's region playing a crucial role in their linguistic specializations (Embick et al., 2000; Musso et al., 2003; Grodzinsky and Amunts, 2006); and
(iii) Reentering tracts of prefrontal nervous fibers connecting (i) and (ii) are also known (Lieberman, 2006, 163ff, and references therein), adding credit to the idea that they conjointly act as an integrated system in the sense hypothesized above.
Moreover, information about the developmental basis of this system has accumulated in the last years. A still tentative, but very promising hypothesis is that these data support the idea that a network of genetic activity exists underlying the system, with the properties of a ChIN. What follows is a list of relevant conclusions in this area of research that clearly point to that position:
(i) From the early years of the twenty-first century, correlations between forms of specific language impairment and disruptions of FOXP2 have been solidly established (Lai et al., 2001). This is an extremely suggestive finding for, on the one hand, FOXP2 is a gene encoding a forkhead-domain transcription factor that plays an important role in the regulation of development of several organic systems—guts, lungs, brains, etc.; and on the other hand, because its regulatory role has been specifically attested in the cortical plate and the basal ganglia, among other brain structures, by means of in vitro transcription and in situ hybridization techniques (Lai et al., 2003). This result is moreover congenial with the study of brain abnormalities using fMRI in individuals affected by the relevant forms of the linguistic impairment, which mostly locate in Broca's region and in the putamen (Liégeois et al., 2003). Concurrently, Reimers-Kipping et al. (2011) report that by “humanizing” mice Foxp2—i.e., by inserting the relevant substitutions at the precise loci—a new pattern of expression obtains that characteristically targets the same brain structures.
(ii) The discovery has been added more recently that FOXP2 promotes neuronal differentiation in these specific subpopulations of neurons in the brain by interacting with the retinoic acid signaling pathway (Devanna et al., 2014), thus regulating neuronal migration, by reducing it, and neurite outgrowth, by increasing it (Vernes et al., 2011), two key processes for neural circuit formation.
(iii) Finally, an important number of genes have been identified as targets of FOXP2 in the development of the human brain (Spiteri et al., 2007; Vernes et al., 2007; Konopka et al., 2009). According to one estimate, FOXP2 targets 285 genes in fetal brain development (Spiteri et al., 2007). Interestingly, the same source pinpoints 34 transcriptional targets that show overlapping patterns of activity in the basal ganglia and in the inferior frontal cortex. Many of these genes remain inactive in the development of other structures, like the lungs. While it is obviously premature to establish whether each of these genes cooperates with FOXP2 in fixing the essential character identity of a system of computation as defined in this section, or it rather acts as a realizer gene modeling specific states of its human variant, the resulting picture invites thinking that such a task may become a promising avenue of comparative research in the near future.
Taking all these observations into consideration, we believe that the idea that a ChIN exists, in exactly the terms set in Wagner (2014), in relation to the bare computational essentials of language seems to be well grounded. So the door is now open to formulate the main questions that this paper is aimed at clarifying:
(i) Is language—qua CCC instantiation, a radically new character—i.e., a Type I novelty?; and if not
(ii) What are its homologs and how did it evolve its human-specific states—i.e., its Type II associated novelties?
One Identity in Search of Homologs
The study of the brain of songbirds and other vocal-learning avian species has proved thus far the most promising source of information regarding the questions above. Partial as it already is, results overwhelmingly point to the conclusion that postulating the presence of CCC homologs within their brain architectures seems to be on the right track, thus supporting our prior conclusion that no in principle objection exists for postulating the same kind of computational activity (e.g., merge) as underlying birdsong composition. In this final section of our paper we first try to ground as solidly as possible our homological claim and then devote some comments to characterizing the most relevant evolutionary and developmental parameters of the human instantiation of CCC.
The CCC Identity Network
The most extensive study thus far regarding the bird/human connection at brain level (Pfenning et al., 2014), offers wide empirical plausibility to the following set of claims:
(i) A massively shared molecular background speaks of the homologous character of certain structures of the pallium/cortex and the striatum of song-learning birds and humans—the striatum is the substructure of the basal ganglia system comprising the caudate nucleus and the putamen, among other components, that serves as the primary intermediary channel between the pallium/cortex and other deeper ganglial structures;
(ii) Within the striatal component, the best-aligned correlates are the avian Area X and the human putamen;
(iii) Within the pallial/cortical component, a significant correlation exists between birds' RA and zones of the human central sulcus—particularly around the laryngeal motor cortex (LMC), next to Broca's area in the inferior motor cortex; and
(iv) Relevant molecular correlations decrease when out-groups are considered, like vocal-non-learning avian species—e.g., doves or hummingbirds, and non-human primates.
From an anatomical point of view, these observations offer strong support to the idea that a pallio-striato-pallial system exists in the central nervous system with the properties of an organ, the identity of which traverses species boundaries. Moreover, the well-established fact that, in parallel to the linguistic specializations of the basal ganglia and lower/pre-frontal cortical areas in the case of humans, the corresponding avian areas are crucial components of the song learning/production system of birds (Doupe and Kuhl, 1999) gives in our opinion extra support to our thesis that a bare computational functionality must be attributed to this organ—see Figure 4.
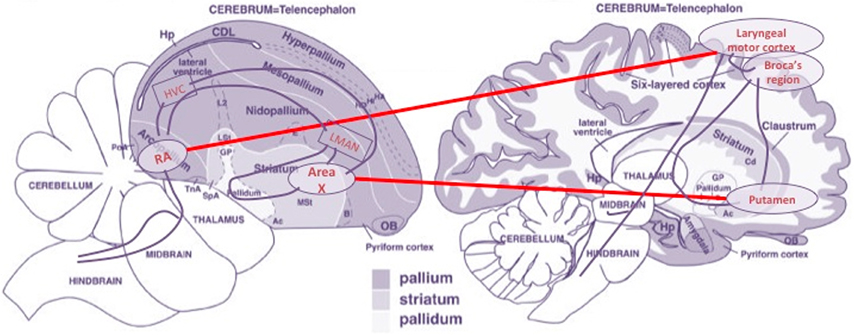
Figure 4. Main morphological alignments of brain components of the birdsong and language circuits. Based on an image from Reiner et al. (2004). (Figure 1), reproduced with permission of John Wiley and Sons, Inc.
In Pfenning's et al. (2014) interpretation of the observed correlations, these are repeatedly referred to as cases of molecular convergence between analogous brain regions—as the last common ancestor can only be traced back as far as some 310 millions years and with obvious intermittencies, which if anything speaks of a deeply shared supply of developmental resources and a limited array of potential evolutionary arrangements among them. However, it is also plausible that an anatomical “language-like” system already existed in the most recent common ancestor of birds and humans as part of a larger neuroanatomical system, but which only individuated from that larger system in later evolution. The question cannot be decided with the evidence at hand, but we need to keep that possibility in mind. For the time being, we will adopt the more conservative position that an underlying ChIN is not only accessible from this developmental background, but actually attested in different specific contexts, creating bona fide homologs despite evolutionary distance—a possibility actually envisaged by Wagner (2014) in the following terms:
If the idea holds up that homology (i.e., continuity of character identity) is tied to the continuity of ChINs, then the notion of homology can be generalized to situations where historical continuity of the phenotypic character does not apply. There can be dormant ChINs and, thus, there can be continuity of potential character identity despite discontinuity of characters' expression. (Wagner, 2014, p. 417).
What follows is an enumeration of relevant observations that in our opinion gives strong support to this specific interpretation:
(i) The role of the FOXP2 transcription factor in learning and producing birdsong motifs was firmly established right after the announcement of its role in language (Haesler et al., 2004; Teramitsu et al., 2004). Moreover, Area X was also early pinpointed as critically targeted by the regulatory role of the bird version of the gene—FoxP2 (Haesler et al., 2007).
(ii) The role of retinoic acid as a key inductive signal in the development of different nuclei of the bird song system was already known even earlier (Denisenko-Nehrbass et al., 2000; Denisenko-Nehrbass and Mello, 2001). The original papers in this field of research emphasized HVC—a further pallial center within the song system—as an area where the effect was clearly observed due to the expression of a retinoic acid synthesis enzyme—RalDH. More recently, the observation has also been extended to lMAN and RA within the same pallial component of the system, and particularly to the striatal Area X, where the profile of retinoic receptor expression is markedly strong (Roeske et al., 2014). HVC and lMAN are routinely described as the bird correlates of Wernicke's and Broca's area—the classical language areas, respectively. According to Roeske's et al., (2014) extensive investigation, the role of retinoic acid signaling in neurogenesis control of the referred areas is a well-established fact.
(iii) Pfenning et al. (2014) also offer quantitative data regarding shared specialized expression of genes in the critical areas thus far commented. According to their estimates, scores of genes contribute to delineate songbird Area X and human putamen (78 genes) and songbird RA and the surroundings of the human central sulcus (40 genes). The study also identifies tens of genes specifically contributing to the anatomical specialization of RA and the laryngeal motor cortex (LMC), but mostly non-overlapping (only three out of 55 actually overlap). Special consideration in this paper is given to the axon guidance ligand SLIT1, for it is a target of the FOXP2 transcription factor previously identified in Konopka et al. (2009). For concreteness, SLIT1 is a ligand of ROBO1, which causes repulsion of axonal processes from cell bodies. Down-regulation of SLIT1 via FOXP2—as well as of other putative genes—in RA and LMC thus behaves as a permissive mechanism leading to specialized patterns of connectivity. Other findings reported in Pfenning et al. (2014) also point to the shared expression of other genes, but obeying to different patterns of down-regulation, as in the case of NEUROD6, probably active in defining boundaries within RA and the dorsal component of LMC. Considering the whole picture, what we observe is a pattern of partially shared and partially divergent mechanisms, seemingly correlating both with how the basic identity of the character obtains and with how shared and deviant states also obtain from such a background.
Note that we have devoted this section to the specific case of songbirds simply for the extensive and detailed literature that it has generated in the last few years. In any event, other organisms have also inspired research projects in the wake of the putative FOXP2/computation connection—see Shu et al. (2007) for ultrasonic vocalizations in mice, Li et al. (2007) for echolocation in bats, Kiya et al. (2008) for waggle dance in bees, and Lawton et al. (2014) for pulse-song in Drosophila. All these studies offer obvious candidates for extending and validating our proposal. But in our opinion, data reviewed in this section suffice to give clear support to the idea that a supra-specific character exists corresponding to what we denominate CCC, the identity of which relies on a shared core of developmental resources chained in a distinctively interactive pattern, which paves the way to a minimal form/function unit however open to a wide range of formal and functional diversification. The next subsection is devoted to briefly reflect on the specifics of the human variant of this character, once properly located within its homological framework.
The Human CCC: States and Beyond
According to the perspective put forward in Section Uniqueness Demoted, CCCs vary along the following two dimensions: (i) their connective specificities in different cognitive contexts, and (ii) the specific degree of computational complexity that they attain in these contexts. Up to this point we have expounded the main reasons why we believe that this idea is on the right track, so we think that the claim is well motivated that the human instantiation of this cognitive organ differs from its non-human homologs according to the following two Type II novelties:
(i) The interface connecting CS with the systems in charge of conceptual categorization, intention fixing, reasoning, and so on, to which we will refer as the “syntax-semantics interface”—i.e., the orange square in Figure 1; and
(ii) The mildly context-sensitive computational power of CS itself, which allows nested patterns of representation of indeterminate depth—e.g., phrases within phrases, and so on, as well as intricate crossing relations among units located at different nested levels—see (1) and (2) above.
Taken together, these two traits seem to suffice to set language apart from other functional and formal variants of the same organ in different animals, as the comparative perspective supports the view that (ii) seems to be almost exceptional, save for some intriguing cases of knot tying observed in the constructive abilities of some avian species, where nevertheless (i) seems to be lacking (Balari et al., 2012; Balari and Lorenzo, 2013). Let us use CCCHuman as a fancy name apt to differentiate language-as-an-organ both from its homologs and from its language particular external correlates.
The main task of evolutionary linguistics may now be redefined as the quest for origins of the Type II novelties that define CCCHuman, obviously enough not an easy one, for it involves a complex array of motives, ranging from fine-grained molecular details to gross environmental considerations capable of jointly explaining how the corresponding character states have emerged and stabilized in the species. No such a task can be seriously undertaken in the few closing pages of a single paper. In any event, let us devote some space to sketch what we consider to be a very promising line of research that could fit the bill. Our main suggestion is that the character states (i) and (ii) above can be explained as outcomes of a single evolutionary event, which makes us think of them as inextricably linked in the way that according to Wagner (2014) defines a “variational modality.”
For concreteness, our specific take on this matter boils down to the idea that both states derive from a general increase of the cortical matter traversing anterior and posterior brain areas—habilitating more working memory resources as a consequence, concurrently with a general reconfiguration process affecting the cranial box—giving place to a characteristically convoluted array of new inter-areas connections, including new ways of access to the working memory facilities (Balari and Lorenzo, 2013). A proposal along similar lines has been recently put forth by Boeckx and Benítez-Burraco (2014a), who also try to make sense of the two processes together under the tag of “globularity.” Such a name is aimed at referring to the characteristic shape of the brain of Anatomically Modern Humans relatively to the elongated configuration of the Neandertal/Denisovan counterpart. According to the authors, such an innovative brain configuration has the structural and functional reflexes of a more extensive and fluid fronto-pariental brain connectivity, as well as an enhanced modulative regime governed from the thalamus (Boeckx and Benítez-Burraco, 2014a, p. 7). Computationally speaking, this neural architecture basically translates into an “unrestricted combinatorial operator” (Boeckx and Benítez-Burraco, 2014a, p. 5)—a.k.a. merge, which enables the brain to connect distantly encoded percepts and concepts in a cross-modular fashion. We find certain aspects of Boeckx and Benítez-Burraco's (henceforth, BBB) model attractive and worth pursuing, but we also differ from their ideas on certain crucial issues. Let us expound them as a way of clarifying our own thesis based on the “variational modality” concept.
One of the strengths of BBB's model is that they have been able to reconstruct a network of genetic sequences and products that they hypothetically relate to “globularity” as the site of the “syntax-semantics interface”—namely, the RUNX2 network, according to their terminology. In BBB's interpretation, it is this network—through its expression in the globular shape of the brain—that ultimately endows humans with their “readiness” to acquire and use external systems of grammatical conventions (Boeckx and Benítez-Burraco, 2014a, p. 1). Note that the position entails that no organ proper—say, a CCC—is required as an intermediary devoted to the effect. It is not our opinion that this Piagetian stance (Boeckx, 2014) is necessarily wrong. In any event, we also think that the position is neither right away supported by the authors' genetic database, which still deserves a closer scrutiny in order to decide whether the RUNX2 network can be interpreted as a ChIN, or it rather is a relatively more unstructured array of “realizing” machinery. What is clear is that according to BBB's preferred interpretation, “globularity” is a shape-related “state” (Müller and Wagner, 1991) attributable to no other character “identity” than the brain itself, thus preventing the formulation of homological claims at other putatively intermediate levels. Let us put this question aside for a moment.
For the time being, let us focus on a spin-off of Boeckx and Benítez-Burraco (2014a), where they comment on two further language-related genetic networks—namely the FOXP2 and ROBO/SLIT networks, already referred to in this paper—and how they relate to the RUNX2 network (Boeckx and Benítez-Burraco, 2014b). Here we find another critical point of departure from BBB's model, for they contend that these networks are alien to the computational procedure in charge of the construction of linguistic expressions—merge—and embrace the idea instead that they relate to the “externalization component” of language (Boeckx and Benítez-Burraco, 2014a, p. 14), conforming with an idea epitomized by the following quote by Berwick and Chomsky (2011):
FOXP2 is more akin to the blue-print that aids in the construction of a properly functioning input-output system for a computer, like its printer, rather than the construction of the computer's central processor itself [… ] If so, then the entire FOXP2 story, and motor externalization generally, is even further removed from the picture of core syntax/semantics evolution. The evidence comes from the finding that all mammals tested (people, dogs, cats, seals, whales, baboons, tamarin monkeys, mice) and unrelated vertebrates (crows, finches, frogs, etc.,) possess what was formerly attributed just to the human externalization system: each of the vocal repertoires of these various species is drawn from a finite set of distinctive phonemes (or, more accurately, songmemes in the case of birds, barkemes in the case of dogs, etc.,) (Berwick and Chomsky, 2011, pp. 23–24).
We believe that the conclusion of the first part of this quote is not really sustained by the observations of the second part, for as we argued in Section The Quest for “Humaniqueness,” the latter are twofold deceiving: On the one hand, the fact that human phonetics/phonology seems to fit in terms of simplicity the suggested list most probably is an artifact of the externalization channel; and on the other hand, simplicity does not preclude the thesis of a computational processor, based on iterative applications of merge, being at work in the referred abilities, for as we observed in that subsection, merge actually is as simple an operation as you can get.
So putting all the pieces together, we believe that the picture based on the idea that FOXP2-ROBO/SLIT are crucially implied in the ChIN that aids in the construction of the “computer's central processor itself,” along the lines suggested in the previous subsection, is better grounded than BBB's preferred alternative.
Note also that emphasizing the RUNX2-FOXP2-ROBO/SLIT connection is one of the main motivations of BBB's project. But they interpret it as the outcome of the evolutionary encounter of an old “externalization component” (FOXP2-ROBO/SLIT) with a brand new “conceptual-intentional component” (RUNX2) that specifically contributed the merge operation. We believe that this interpretation, while attractive, is not clearly sustained by their battery of empirical evidence, which seems compatible with many other scenarios. According to our view, merge, understood as the basic operative principle of natural computational activities, must be there from the very evolutionary origins of CCC—which we relate with the ChIN putatively comprising the FOXP2-ROBO/SLIT network(s), while its complexification is an event specific to human evolution and plausibly exclusive of the anatomically modern branch—which has credibly to do with the recruitment of the modern RUNX2 net. This is, obviously enough, an idea in need of more elaboration. But let us simply note here some relevant developmental details that in our opinion give support to our view:
(i) It has been replicated in several experimental settings that pre-linguistic children manage to accomplish relatively complex computational operations in language related-tasks in which no semantic parameter seems to be at work—for example, the identification of patterns of organization in syllabic stimuli (Marcus et al., 1999; Gervain et al., 2012); and
(ii) It is also a well-attested fact that children start unraveling the subtleties of the complex organization of linguistic expressions aided by means mostly provided by the “phonetics/phonology interface”—or “externalization component;” the red square in Figure 1 (Kuhl et al., 2008).
So computational activity seems to be at work very early, independent of semantic concerns and in close concert with the phonetics/phonology interface. It is thus our conclusion that the growth of CCChuman works differently and goes in a direction opposite to the one privileged by BBB's model, at least developmentally and very plausibly, also evolutionarily.
To conclude, let us briefly go back to the issue we left pending above: i.e., the best way of making sense of the evolutionary expansion of CCC that, following BBB, we are putatively associating to their RUNX2 network. Two alternative possibilities seem equally compatible with the perspective put forward in this paper:
(i) The RUNX2 network just comprises an interconnected array of “realizer genes” evolutionarily recruited by the CCC's ChIN, so the resulting new pattern of neural connectivity and degree of computational power are correspondingly just the defining “states” of this new “variational modality” of the organ; or
(ii) The RUNX2 network qualifies as a bona fide new ChIN, for it conforms to the typical organizational pattern of this biological entity as described by Wagner (2014), very much like the ChIN that we postulate as associated to the FOXP2-ROBO/SLIT net(s). Note that this is a possibility actually contemplated in Wagner's model: i.e., that the “states” making up a particular “character” qualify in their turn as characters (Wagner, 2014, p. 355), as for example the autopod relatively to tetrapod limb. Were this interpretation the correct one, the new pattern of neural connectivity and degree of computational power would correspondingly qualify as a brand new organ associated to CCChuman, itself an embracing organ. Note that such a scenario opens an extra dimension of the combinatorial approach to homology that we are advocating for here, namely that the “states” making up a particular “character” qualify in their turn as characters (Wagner, 2014, p. 355), as for example the autopod relatively to tetrapod limb.
We are not in the position of settling the question in one or another direction, but the take-home message is that this—as many others reviewed in this paper—is an empirical matter, and probably one apt to be answered in the short run by a close technical scrutiny of the inventory of gene products pinpointed by BBB.
Conclusion
“Language” is a loaded term, with different meanings for different people. In this paper we have strived to restrict its meaning to refer to the set of brain areas and circuits that make it possible that humans acquire and use at least one “language” like Italian, Russian, or Nicaraguan Sign Language. Already this last sentence shows the kinds of terminological and ontological difficulties faced by any attempt to build solid and biologically grounded linguistic and cognitive sciences. Our point of departure here has been that, necessarily, “language,” as a technical term, has an organic referent. Most of the paper is devoted to uncovering what this organic referent is or might be. To this end, we applied the strategy of asking to what extent language possesses an identity as an organic character and in which sense it can be deemed an evolutionary novelty. The natural foundation on which these questions should be framed was, in our opinion, the long tradition in theoretical biology devoted to the study of homologies and, in particular, Wagner's seminal contribution to the topic. In many ways, then, our project was not too different from Richard Owen's cogitations as to whether the word “vomer” is an appropriate term to designate a bone found in the skeletons of humans and whales, because it is the same bone (Owen, 1848).
But our project was also different, because the debate on the biology of language has been largely vitiated by too many a priori functional considerations that, among other things, already turned the homology question into a preposterous one: There clearly is nothing like “language” in the organic world. As we hope to have shown here, this statement is only partially true. There certainly is nothing comparable to “language” understood as the organic structure that facilitates the acquisition and use of languages, and in this sense “language” is a term only applicable to refer to this particular structure in humans. Once we forgo such “what-is-it-for” considerations and focus on the kinds of basic activities that characterize “language,” what we see is a natural system of computation with many possible homologs in the natural world. If we were to establish a parallelism, our term “CCC” would be comparable to the term “vertebrate limb,” while “language” would perhaps be similar to the term “bird's wing.” Terminological questions aside, we believe that this proposal has the virtue of overcoming a number of conceptual and theoretical hurdles that placed evolutionary cognition on the verge of absolute stagnation. For example, a paradigmatic side-effect of the uniqueness claims has been the excessive attention paid by neurolinguistic studies on cortical areas of the human brain under (the often hidden) assumption that since language is unique it must have something to do with the also unique development of the human cortex. As expounded in Section One Identity in Search of Homologs, more attention should be paid to the role of other, very ancient, brain structures, like the basal ganglia or the thalamus, with clear homologs in the brains of other organisms at the anatomical and, quite plausibly, also the activity level (Fedorenko and Thompson-Schill, 2014; Hagoort, 2014).
We wouldn't like to close these concluding remarks without identifying a number of open questions the field still needs to face, in addition to those already made explicit in the preceding sections.
(i) The problem of selection. Wagner (2014) contends that the role of selection is negligible in Type I novelties but (with varying degrees) inescapable in their diversification (Type II novelties); we alternatively suspect that the distinguishing character states of language as a particular instantiation of CCC may also be relatively alien to a strictly selective logic (Balari and Lorenzo, 2013). This particular point is illustrative of the important role that language may play in helping to fine tune a general theory of evo-devo.
(ii) The problem of ontology. The interpretation of “language” we have been advocating for here appears to imply a sharp dichotomy between a neurobiological entity, on the one hand, and a cultural/social entity on the other. To be sure, some authors seem to take this dualism to heart (e.g., Christiansen and Chater, 2008; Boeckx and Benítez-Burraco, 2014a, p. 1), as if, much in the Piagetian/Vygotskian tradition, linguistic neural structures were just the appropriate receptacle of the linguistic knowledge to be developed during the process of language acquisition (Bickerton, 2014, for criticisms of this view). We ourselves have appealed to this dichotomy for methodological reasons (Balari and Lorenzo, 2013, Chap. 1), but we have also emphasized that such a dualistic conception has no place in a truly evolutionary developmental approach, for there is no real distinction between the development of the brain and the acquisition of language (Dove, 2012; Balari and Lorenzo, 2015, for a congenial view). We are in fact convinced that the ontological problem can be overridden by taking seriously the agenda of developmental models of cultural evolution like those proposed by Griesemer and Wimsatt, incorporating such notions as “developmental hybrid” (Wimsatt and Griesemer, 2007; Wimsatt, 2013; Griesemer, 2014a,b; Minelli, 2014), to give rise to a more integrative interdisciplinary approach (Love and Lugar, 2013).
(iii) The problem of representation(s). The granularity problem (Poeppel and Embick, 2005) can in fact be broken down into two subproblems: (i) The problem of computational primitives, and (ii) The problem of representations (Embick and Poeppel, 2015). In this paper, we have mostly concentrated on the first, while we almost totally neglected the second, apart from a swift comment on the issue of words in natural language. The case of words is just an example of a much broader problem posing two hard questions: (i) What are representations?, or What format do they have?, and (ii) How do representations represent? This is a very old puzzle, dating back at least to Aristotle, but it is also a very hard one, for which an acceptable solution in biological terms is still wanting—Cummins (1989), for a good exposition of the several issues involved.
These issues have been present in one or another form for a long time. We believe however that the conceptual tools provided by evolutionary developmental biology may help us approach them from a new angle, one capable of dispelling the new wave of skepticism that is again haunting the field, as witnessed by the publication of Hauser et al. (2014), a paper with some eminent scholars among its authors, which contends that the question of the origins of language remains as mysterious as ever.
Hopefully, we expect to have shown otherwise.
Conflict of Interest Statement
The authors declare that the research was conducted in the absence of any commercial or financial relationships that could be construed as a potential conflict of interest.
Acknowledgments
This paper is dedicated to the memory of Werner Callebaut (1952–2014), who was an unexpected supporter of our homological project when it was not even clear to us that it was worth the attention of a biologist. For their comments and suggestions we also want to express our gratitude to Piera Filippi, James Griesemer, Alessandro Minelli, Günter Wagner, and two reviewers. Any remaining errors are our own. This work has been partially supported by the Generalitat de Catalunya through grant 2014-SGR-1013 to the Centre de Lingüística Teòrica of the Universitat Autònoma de Barcelona (SB).
References
Aboitiz, F., Brinetti, E., and Bosman, C. (2006). “The origins of Broca's Area and its connection from an ancestral working-memory network,” in Broca's Region, eds Y. Grodzinsky and K. Amunts (Oxford: Oxford University Press), 3–16. doi: 10.1093/acprof:oso/9780195177640.003.0001
Amundson, R. (2005). The Changing Role of the Embryo in Evolutionary Thought: roots of Evo-Devo. Cambridge: Cambridge University Press. doi: 10.1017/CBO9781139164856
Balari, S., Benítez-Burraco, A., Camps, M., Longa, V. M., and Lorenzo, G. (2012). Knots, language, and computation: a bizarre love triangle? Replies to objections. Biolinguistics 6, 79–111.
Balari, S., and Lorenzo, G. (2008). Pere Alberch's developmental morphospaces and the evolution of cognition. Biol. Theory 3, 297–304. doi: 10.1162/biot.2008.3.4.297
Balari, S., and Lorenzo, G. (2013). Computational Phenotypes. Towards an Evolutionary Developmental Biolinguistics. Oxford: Oxford University Press.
Balari, S., and Lorenzo, G. (2015). The end of development. Biol. Theory 10, 60–72. doi: 10.1007/s13752-014-0180-0
Benítez-Burraco, A., and Boeckx, C. (2014). Universal Grammar and biological variation: an EvoDevo agenda for comparative biolinguistics. Biol. Theory 9, 122–134. doi: 10.1007/s13752-014-0164-0
Berwick, R. C., Beckers, G. J. L., Okanoya, K., and Bolhuis, J. J. (2012). A bird's eye view of human language evolution. Front. Evol. Neurosci. 4:5. doi: 10.3389/fnevo.2012.00005
Berwick, R. C., and Chomsky, N. (2011). “The biolinguistic program: the current state of its development,” in The Biolinguistic Entreprise. New Perspectives on the Evolution and Nature of the Human Language Faculty, eds A. M. Sciullo and C. Boeckx (Oxford: Oxford University Press), 19–41.
Berwick, R. C., Friederici, A. D., Chomsky, N., and Bolhuis, J. J. (2013). Evolution, brain, and the nature of language. Trends Cogn. Sci. 17, 89–98. doi: 10.1016/j.tics.2012.12.002
Berwick, R. C., Okanoya, K., Beckers, G. J. L., and Bolhuis, J. J. (2011). Songs to syntax: the linguistics of birdsong. Trends Cogn. Sci. 15, 113–121. doi: 10.1016/j.tics.2011.01.002
Bickerton, D. (2013). “Language and natural selection,” in The Cambridge Handbook of Biolinguistics, eds C. Boeckx and K. K. Grohmann (Cambridge: Cambridge University Press), 478–488. doi: 10.1017/CBO9780511980435.027
Bickerton, D. (2014). More than Nature Neeeds. Language, Mind, and Evolution. Cambridge, MA: Harvard University Press.
Boeckx, C. (2013a). Biolinguistics: forays into human cognitive biology. J. Anthropol. Sci. 91, 63–89. doi: 10.4436/JASS.91009
Boeckx, C. (2013b). “The I-language mosaic,” in Language, from a Biological Point of View, eds C. Boeckx, M. C. Horno-Chéliz, and J. L. Mendívil-Giró (Newcastle upon Tyne: Cambridge Scholars Publishing), 23–51.
Boeckx, C. (2014). The roots of current biolinguistic thought. Revisiting the ‘Chomsky-Piaget Debate’ in the context of the revival of biolinguistics. Teorema 33, 83–94.
Boeckx, C., and Benítez-Burraco, A. (2014a). The shape of the human language-ready brain. Front. Psychol. 5:282. doi: 10.3389/fpsyg.2014.00282
Boeckx, C., and Benítez-Burraco, A. (2014b). Globularity and language-readiness: generating new predictions by expanding the set of genes of interest. Front. Psychol. 5:1324. doi: 10.3389/fpsyg.2014.01324
Boeckx, C., and Grohmann, K. K. (eds.). (2013). The Cambridge Handbook of Biolinguistics. Cambridge: Cambridge University Press. doi: 10.1017/CBO9780511980435
Bolhuis, J. J., Brown, G. R., Richardson, R. C., and Laland, K. N. (2011). Darwin in mind: new opportunities for evolutionary psychology. PLoS Biol. 9:e1001109. doi: 10.1371/journal.pbio.1001109
Bolhuis, J. J., Okanoya, K., and Scharff, C. (2010). Twitter evolution: converging mechanisms in birdsong and speech. Nat. Rev. Neurosci. 11, 747–759. doi: 10.1038/nrn2931
Bolhuis, J. J., Tattersall, I., Chomsky, N., and Berwick, R. C. (2014). How could language have evolved?. PLoS Biol. 12:e1001934. doi: 10.1371/journal.pbio.1001934
Bolhuis, J. J., and Wynne, C. D. L. (2009). Can evolution explain how minds work. Nature 458, 832–833. doi: 10.1038/458832a
Bonelli, R. M., and Cummings, J. L. (2007). Frontal-subcortical circuitry and behavior. Dialogues Clin. Neurosci. 9, 141–151.
Brigandt, I., and Love, A. C. (2010). Evolutionary novelty and the Evo-Devo synthesis: field notes. Evol. Biol. 37, 93–99. doi: 10.1007/s11692-010-9083-6
Callebaut, W. (2005). “The ubiquity of modularity,” in Modularity: Understanding the Development and Evolution of Natural Complex Systems, eds W. Callebaut and D. Rasskin-Gutman (Cambridge, MA: The MIT Press), 3–28.
Carandini, M. (2012). From circuits to behavior: a bridge too far?. Nat. Neurosci. 15, 507–509. doi: 10.1038/nn.3043
Christiansen, M. H., and Chater, N. (2008). Language as shaped by the brain. Behav. Brain Sci. 31, 489–509. doi: 10.1017/S0140525X08004998
Cummings, J. L. (1993). Frontal-subcortical circuits and human behavior. Arch. Neurol. 50, 873–880. doi: 10.1001/archneur.1993.00540080076020
Denisenko-Nehrbass, N. I., Jarvis, E., Scharff, C., Nottebohm, F., and Mello, C. V. (2000). Site-specific retinoic acid production in the brain of adult songbirds. Neuron 27, 359–370. doi: 10.1016/S0896-6273(00)00043-X
Denisenko-Nehrbass, N. I., and Mello, C. V. (2001). Molecular targets of disulfiram action on song maturation in zebra finches. Mol. Brain Res. 87, 246–250. doi: 10.1016/S0169-328X(01)00002-X
Devanna, P., Middelbeek, J., and Vernes, S. C. (2014). FOXP2 drives neuronal differentiation by interacting with retinoic acid signaling pathways. Front. Cell. Neurosci. 8:305. doi: 10.3389/fncel.2014.00305
Doupe, A. J., and Kuhl, P. K. (1999). Birdsong and human speech: common themes and mechanisms. Annu. Rev. Neurosci. 22, 567–631. doi: 10.1146/annurev.neuro.22.1.567
Dove, G. (2012). Grammar as a developmental phenomenon. Biol. Philos. 17, 615–637. doi: 10.1007/s10539-012-9324-4
Embick, D., Marantz, A., Miyashita, Y., O'Neill, W., and Sakai, K. L. (2000). A syntactic specialization for Broca's area. Proc. Natl. Acad. Sci. U.S.A. 97, 6150–6154. doi: 10.1073/pnas.100098897
Embick, D., and Poeppel, D. (2015). Towards a computational(ist) neurobiology of language: correlational, integrated and explanatory neurolinguistics. Lang. Cogn. Neurosci. 30, 357–366. doi: 10.1080/23273798.2014.980750
Erwin, D. H., and Krakauer, D. C. (2004). Insights into innovation. Science 304, 117–119. doi: 10.1126/science.1099385
Fedorenko, E., and Thompson-Schill, S. L. (2014). Reworking the language network. Trends Cogn. Sci. 18, 120–126. doi: 10.1016/j.tics.2013.12.006
Filippi, P. (2014). Specifically human: going beyond perceptual syntax. Biosemiotics 7, 111–123. doi: 10.1007/s12304-013-9187-3
Fitch, W. T., and Friederici, A. D. (2012). Artificial grammar learning meets formal language theory: an overview. Philos. Trans. R. Soc. B 367, 1933–1955. doi: 10.1098/rstb.2012.0103
Fitch, W. T., Friederici, A. D., and Hagoort, P. (2012). Pattern perception and computational complexity: introduction to the special issue. Philos. Trans. R. Soc. B 367, 1925–1932. doi: 10.1098/rstb.2012.0099
Fitch, W. T., Hauser, M. D., and Chomsky, N. (2005). The evolution of the language faculty: clarifications and implications. Cognition 97, 179–210. doi: 10.1016/j.cognition.2005.02.005
Gervain, J., Berent, I., and Werker, J. F. (2012). Binding at birth: the newborn brain detects identity relations and sequential position in speech. J. Cogn. Neurosci. 24, 564–574. doi: 10.1162/jocn_a_00157
Griesemer, J. R. (2014a). “Reproduction and the scaffolded development of hybrids,” in Developing Scaffolds in Evolution, Culture, and Cognition, eds L. R. Caporael, J. R. Griesemer, and W. C. Wimsatt (Cambridge, MA: The MIT Press), 23–44
Griesemer, J. R. (2014b). “Reproduction and scaffolded developmental processes: an integrated evolutionary perspective,” in Towards a Theory of Development, eds A. Minelli and T. Pradeau (Oxford: Oxford University Press), 183–202.
Grodzinsky, Y., and Amunts, K. (eds.). (2006). Broca's Region. Oxford: Oxford University Press. doi: 10.1093/acprof:oso/9780195177640.001.0001
Haesler, S., Rochefort, C., Georgi, B., Licznerski, P., Osten, P., and Scharff, C. (2007). Incomplete and inaccurate vocal imitation after knockdown of FoxP2 in songbird basal ganglia nucleus area X. PLoS Biol. 5:e321. doi: 10.1371/journal.pbio.0050321
Haesler, S., Wada, K., Nshdejan, A., Morrisey, E., Lints, T., Jarvis, E. D., et al. (2004). FoxP2 expression in avian vocal learners and non-learners. J. Neurosci. 24, 3164–3175. doi: 10.1523/JNEUROSCI.4369-03.2004
Hagoort, P. (2005). On Broca, brain, and binding: a new framework. Trends Cogn. Sci. 9, 416–423. doi: 10.1016/j.tics.2005.07.004
Hagoort, P. (2013). MUC (Memory, Unification, Control) and beyond. Front. Psychol. 4:416. doi: 10.3389/fpsyg.2013.00416
Hagoort, P. (2014). Nodes and networks in the neural architecture for language: Broca's region and beyond. Curr. Opin. Neurobiol. 28, 136–141. doi: 10.1016/j.conb.2014.07.013
Hauser, M. D. (2009). The possibility of impossible cultures. Nature 460, 190–196. doi: 10.1038/460190a
Hauser, M. D., Chomsky, N., and Fitch, W. T. (2002). The Faculty of Language: What is it? Who has it? How did it evolve?. Science 298, 1569–1579. doi: 10.1126/science.298.5598.1569
Hauser, M. D., Yang, C., Berwick, R. C., Tattersall, I., Ryan, M. J., Watumull, J., et al. (2014). The mystery of language evolution. Front. Psychol. 5:401. doi: 10.3389/fpsyg.2014.00401
Heinz, J. (2014). “Computational theories of learning and developmental psycholinguistics,” in The Oxford Handbook of Developmental Linguistics, eds J. Lidz, W. Snyder, and J. Pater (Oxford: Oxford University Press). (in press).
Heinz, J., and Idsardi, W. (2011). Sentence and word complexity. Science 333, 295–297. doi: 10.1126/science.1210358
Heinz, J., and Idsardi, W. (2013). What complexity differences reveal about domains in language. Top. Cogn. Sci. 5, 111–131. doi: 10.1111/tops.12000
Jackendoff, R., and Pinker, S. (2005). The nature of the language faculty and its implications for the evolution of language (Reply to Fitch, Hauser, and Chomsky). Cognition 97, 211–225. doi: 10.1016/j.cognition.2005.04.006
Kiya, T., Itoh, Y., and Kubo, T. (2008). Expression analysis of the FoxP homologue in the brain of the honeybee, Apis mellifera. Insect Mol. Biol. 17, 53–60. doi: 10.1111/j.1365-2583.2008.00775.x
Konopka, G., Bomar, J. M., Winden, K., Coppola, G., Jonsson, Z. O., Gao, F., et al. (2009). Human-specific transcriptional regulation of CNS development genes by FOXP2, Nature 462, 213–217. doi: 10.1038/nature08549
Kuhl, P. K., Conboy, B. T., Coffey-Corina, S., Padden, D., Rivera-Gaxiola, M., and Nelson, T. (2008). Phonetic learning as a pathway to language: new data and native language magnet theory expanded (NLM-e), Philos. Trans. R. Soc. B 363, 979–1000. doi: 10.1098/rstb.2007.2154
Lai, C. S., Fisher, S. E., Hurst, J. A., Vargha-Khadem, F., and Monaco, A. P. (2001). A forkhead-domain gene is mutated in a severe speech and language disorder. Nature 413, 519–523. doi: 10.1038/35097076
Lai, C. S., Gerrelli, D., Monaco, A. P., Fisher, S., and Coop, A. J. (2003). FOXP2 expression during brain development coincides with adult sites of pathology in a severe speech and language disorder. Brain 126, 2455–2462. doi: 10.1093/brain/awg247
Lawton, K. J., Wassmer, T. L., and Deitcher, D. L. (2014). Conserved role of Drosophila melanogaster FoxP in motor coordination and courtship song. Behav. Brain Res. 268, 213–221. doi: 10.1016/j.bbr.2014.04.009
Li, G., Wang, J., Rossiter, S. J., and Zhang, S. (2007). Accelerated FoxP2 evolution in echolocating bats. PLoS ONE 2:e900. doi: 10.1371/journal.pone.0000900
Lieberman, P. (2006). Toward an Evolutionary Biology of Language. Cambridge, MA: Harvard University Press.
Liégeois, F., Badelweg, T., Connelly, A., Gadian, D. G., Mishkin, M., and Varga-Khadem, F. (2003). Language fMRI abnormalities associated with FOXP2 gene mutation. Nat. Neurosci. 6, 1230–1237. doi: 10.1038/nn1138
Love, A. C. (2007). Functional homology and homology of function: biological concepts and philosophical consequences. Biol. Philos. 22, 691–708. doi: 10.1007/s10539-007-9093-7
Love, A. C., and Lugar, G. L. (2013). Dimensions of integration in interdisciplinary explanations of the origin of evolutionary novelty. Stud. Hist. Philos. Biol. Biomed. Sci. 44, 537–550. doi: 10.1016/j.shpsc.2013.09.008
Marcus, G. F., Vijayan, S., Ban di Rao, S., and Vishton, P. M. (1999). Rule learning by seven-month-old infants. Science 283, 77–80. doi: 10.1126/science.283.5398.77
Mayr, E. (1960). “The emergence of evolutionary novelties,” in Evolution After Darwin. The University of Chicago Centennial, Vol.1: The Evolution of Life. Its Origin, Evolution and Future, ed S. Tax (Chicago, IL: University of Chicago Press), 349–380.
Minelli, A. (1998). Molecules, developmental modules, and phenotypes: a combinatorial approach to homology. Mol. Phylogenet. Evol. 9, 340–347. doi: 10.1006/mpev.1997.0490
Minelli, A. (2000). Limbs and tail as evolutionary diverging duplicates of the main body axes. Evol. Dev. 2, 157–165. doi: 10.1046/j.1525-142x.2000.00054.x
Minelli, A. (2003). The Development of Animal Form. Cambridge: Cambridge University Press. doi: 10.1017/CBO9780511541476
Minelli, A. (2014). Naturalistic perspectives in cognitive sciences: evolution and development. Reti Saperi Linguaggi 1, 37–48.
Minelli, A., and Fusco, G. (2005). Conserved versus innovative features in animal body organization. J. Exp. Zool. B Mol. Dev. Evol. 304B, 520–525. doi: 10.1002/jez.b.21044
Moczek, A. P. (2008). On the origins of novelty in development and evolution. BioEssays 30, 432–447. doi: 10.1002/bies.20754
Müller, G. B., and Newman, S. A. (2005). The innovation triad: an evodevo agenda. J. Exp. Zool. B Mol. Dev. Evol. 304B, 487–503. doi: 10.1002/jez.b.21081
Müller, G. B., and Wagner, G. P. (1991). Novelty in evolution: restructuring the concept. Annu. Rev. Ecol. Syst. 22, 229–256. doi: 10.1146/annurev.es.22.110191.001305
Müller, G. B., and Wagner, G. P. (2003). “Innovation,” in Keywords and Concepts in Evolutionary Developmental Biology, eds B. K. Hall and W. M. Olson (Cambridge, MA: Harvard University Press), 218–227.
Musso, M., Moro, A., Glauche, V., Rijntjies, M., Reichenbach, J., Büchel, C., et al. (2003). Broca's area and the language instinct. Nat. Neurosci. 6, 774–781. doi: 10.1038/nn1077
Owen, R. (1843). Lectures on the Comparative Anatomy and Physiology of the Invertebrate Animals. London: Longman, Brown, Green and Longmans.
Owen, R. (1848). On the Archetype and Homologies of the Vertebrate Skeleton. London: John van Voorst.
Owen, R. (1849). On the Nature of Limbs. A Discourse. London: John van Voorst. doi: 10.5962/bhl.title.50117
Pfenning, A. R., Hara, E., Whitney, O., Rivas, M. V., Wang, R., Roulhac, P. L., et al. (2014). Convergent transcriptional specializations in the brains of humans and song-learning birds. Science 346, 1333–1346. doi: 10.1126/science.1256846
Pinker, S. (2003). “Language as an adaptation to the cognitive niche,” in Language Evolution, eds M. H. Christiansen and S. Kirby (Oxford: Oxford University Press), 16–37.
Pinker, S., and Bloom, P. (1990). Natural language and natural selection. Behav. Brain Sci. 13, 707–727. doi: 10.1017/S0140525X00081061
Pinker, S., and Jackendoff, R. (2005). The Faculty of Language: what's special about it. Cognition 95, 201–236. doi: 10.1016/j.cognition.2004.08.004
Poeppel, D. (2011). Genetics and language: a neurobiological perspective on the missing link (-ing hypotheses). J. Neurodevelop. Disord. 3, 381–387. doi: 10.1007/s11689-011-9097-0
Poeppel, D. (2012). The maps problem and the mapping problem: two challenges for a cognitive neuroscience of speech and language. Cogn. Neuropsychol. 29, 34–55. doi: 10.1080/02643294.2012.710600
Poeppel, D. (2014). The neuroanatomic and neurophysiological infrastructure for speech and language. Curr. Opin. Neurobiol. 28, 142–149. doi: 10.1016/j.conb.2014.07.005
Poeppel, D., and Embick, D. (2005). “Defining the relation between linguistics and neuroscience,” in Twenty-first Century Psycholinguistics: Four Cornerstones, ed A. Cutler (Hillsdale, NJ: Erlbaum), 173–189.
Reimers-Kipping, S., Hevers, W., Pääbo, S., and Enard, W. (2011). Humanized Foxp2 specifically affects cortico-basal ganglia circuits. Neuroscience 175, 75–84. doi: 10.1016/j.neuroscience.2010.11.042
Reiner, A., Perkel, D. J., Mello, C. V., and Jarvis, E. D. (2004). Songbirds and the revised avian brain nomenclature. Ann. N. Y. Acad. Sci. 1016, 77–108. doi: 10.1196/annals.1298.013
Roeske, T. C., Scharff, C., Olson, C. R., Nshdejan, A., and Mello, C. V. (2014). Long-distance retinoic signaling in the zebra finch brain. PLoS ONE 9:e111722. doi: 10.1371/journal.pone.0111722
Rogers, J., and Hauser, M. D. (2010). “The use of formal languages in artificial language learning: a proposal for distinguishing the differences between human and nonhuman animal learners,” in Recursion in Human Language, ed H. V. D. Hulst (Berlin: De Gruyter Mouton), 213–232.
Rogers, J., and Pullum, G. K. (2011). Aural pattern recognition experiments and the subregular hierarchy. J. Log. Lang. Inf. 20, 329–342. doi: 10.1007/s10849-011-9140-2
Shu, W., Cho, J. Y., Jiang, Y., Zhang, M., Weisz, D., Elder, G. A., et al. (2007). Altered ultrasonic vocalization in mice with a disruption in the Foxp2 gene. Proc. Natl. Acad. Sci. U.S.A. 102, 9643–9648. doi: 10.1073/pnas.0503739102
Shubin, N., Tabin, C., and Carroll, S. (1997). Fossils, genes and the evolution of animal limbs. Nature 388, 639–648. doi: 10.1038/41710
Shubin, N., Tabin, C., and Carroll, S. (2009). Deep homology and the origins of evolutionary novelty. Nature 457, 818–823. doi: 10.1038/nature07891
Spiteri, E., Konopka, G., Coppola, G., Bomar, J., Odlham, M., Ou, J., et al. (2007). Identification of the transcriptional targets of FOXP2, a gene linked to speech and language, in developing human brain. Am. J. Hum. Genet. 81, 1144–1157. doi: 10.1086/522237
Teramitsu, I., Kudo, L. C., London, S., Geschwind, D. H., and White, S. A. (2004). Parallel FoxP1 and FoxP2 expression in songbird and human brain predicts functional interaction. J. Neurosci. 24, 3152–3163. doi: 10.1523/JNEUROSCI.5589-03.2004
Vernes, S. C., Oliver, P. L., Spiteri, E., Lockstone, H. E., Puliyadi, R., Taylor, J. M., et al. (2011). Foxp2 regulates gene networks implicated in neurite outgrowth in the developing brain. PLoS Genet. 7:e1002145. doi: 10.1371/journal.pgen.1002145
Vernes, S. C., Spiteri, E., Nicod, J., Groszer, M., Taylor, J. M., Davies, K. E., et al. (2007). High-throughput analysis of promoter occupancy reveals direct neural targets of FOXP2, a gene mutated in speech and language disorders. Am. J. Hum. Genet. 81, 1232–1250. doi: 10.1086/522238
Wagner, A. (2011). The Origins of Evolutionary Innovations. A Theory of Transformative Change in Living Systems. Oxford: Oxford University Press. doi: 10.1093/acprof:oso/9780199692590.001.0001
Wagner, G. P. (2014). Homology, Genes, and Evolutionary Innovation. Princeton and Oxford: Princeton University Press. doi: 10.1515/9781400851461
Watumull, J., Hauser, M. D., Roberts, I. G., and Hornstein, N. (2014). On recursion. Front. Psychol. 4:1017. doi: 10.3389/fpsyg.2013.01017
Wimsatt, W. C. (2013). Articulating Babel: an approach to cultural evolution. Stud. Hist. Philos. Biol. Biomed. Sci. 44, 563–571. doi: 10.1016/j.shpsc.2013.09.001
Wimsatt, W. C., and Griesemer, J. R. (2007). “Reproducing entrenchments to scaffold culture: The central role of development in cultural evolution,” in Integrating Evolution and Development: From Theory to Practice, eds R. Sansom and R. Brandon (Cambridge, MA: The MIT Press), 227–323.
Keywords: language, brain, computation, homology, novelty
Citation: Balari S and Lorenzo G (2015) It is an organ, it is new, but it is not a new organ. Conceptualizing language from a homological perspective. Front. Ecol. Evol. 3:58. doi: 10.3389/fevo.2015.00058
Received: 10 April 2015; Accepted: 27 May 2015;
Published: 10 June 2015.
Edited by:
Angelika Stollewerk, Queen Mary University of London, UKReviewed by:
Lucas Leclere, University Pierre and Marie Curie, FranceGunter P. Wagner, Yale University, USA
Copyright © 2015 Balari and Lorenzo. This is an open-access article distributed under the terms of the Creative Commons Attribution License (CC BY). The use, distribution or reproduction in other forums is permitted, provided the original author(s) or licensor are credited and that the original publication in this journal is cited, in accordance with accepted academic practice. No use, distribution or reproduction is permitted which does not comply with these terms.
*Correspondence: Sergio Balari, Departament de Filologia Catalana, Facultat de Filosofia i Lletres, Universitat Autònoma de Barcelona, Edifici B, Bellaterra, Barcelona E-08193, Spain,c2VyZ2kuYmFsYXJpQHVhYi5jYXQ=