- 1Phycology Section, Collections and Research, Canadian Museum of Nature, Ottawa, ON, Canada
- 2Centre Eau Terre Environnement, Institut National de la Recherche Scientifique, Quebec City, QC, Canada
- 3Geological Survey of Canada, Natural Resources Canada, Ottawa, ON, Canada
Monitoring changes in environmental conditions is increasingly important as the Canadian economic infrastructure ramps up exploration and mining development in the more inaccessible northern regions of Canada. Governments are concurrently assessing effects from past mining activities and absorbing the economic cost to society with on-going remediation and monitoring initiatives. The abandoned Aldermac mine in northwestern Quebec, mined from 1932 to 1943, is an excellent case study for assessing the state of environmental and economic effects of past mining operations. A paleolimnological approach, using diatoms as environmental proxies, was used to evaluate the spatial and temporal impacts on aquatic receiving environments. Based on the inferences drawn from diatom assemblages in Lake Arnoux, prior to mining activity, lake water pH was similar to that of surrounding lakes (circumneutral to weakly acidic). After mining operations terminated, changes in pH and alkalinity in Lake Arnoux coincided with distinct increases in sediment sulfur content. Across a 30- to 40-year span (circa 1940–1970s) a significant decline in phytoplankton flora coincided with lake acidification and increased clarity of the water column. This resulted in an increase in the benthic diatom population (>90%), replacing the planktonic diatoms. Observed shifts in environmental proxies are concurrent with one, and possibly two, reported tailings pond breaches at the abandoned mine site. Adverse effects of the abandoned Aldermac mine on nearby ecosystems, combined with pressure from local citizens and environmental groups, forced responsible accountability for site restoration led by the Quebec government. Based on the historical period of economic growth, the financial benefits of the Aldermac mine were significant and justify the current pay-it-backward costs for environmental remediation. However, it has now been documented that the pay-it-backward model is not sustainable in the modern economy. New pay-it-forward approaches, addressing our gray water footprint, are required to merge economic and environmental sustainability for future prosperity.
Introduction
In the early 1900s, resource development and exploration were key drivers for economic development in Canada. At the same time, the Great Depression of North America (1929–1939) had a significant negative effect on resource development. The gross national product (GNP) dropped by 40% before the end of the Depression era and the beginning of the Second World War (Amaral, 2002). Despite the difficult economic period, the mining sector still developed in the province of Quebec during the 1920s and 1930s. In particular, mining development in the Abitibi-Timiskaming region significantly expanded after gold was discovered in the 1920s. This lead to the opening of numerous mines and the creation of two major mining towns: Val-d'Or (“valley of gold”) and Rouyn-Noranda. The mining, pulp and paper, and tourism industries continue to be key economic activities in the region, with gold, as well as copper, zinc, lead, and other metal commodities as important resources. In 2013, mining investment in the Abitibi-Timiskaming region totalled US$0.8 B (Institut de la Statistique du Québec, 2014). Despite being an active mining region, a number of mines established in the mid-1900s were abandoned, leaving a vast legacy of acid-generating tailings [Ministère de l'Énergie et des Ressources Naturelles, 2015]. For example, the Aldermac mine site (1932–1943) had the second largest tailing facility and was one of the most contaminated sites in the area (Ministère de l'Énergie et des Ressources Naturelles, 2015). During its operation, the Aldermac mine exploited a group of massive sulfide lenses which produced copper, gold, silver, silica, and pyrite (Ministère de l'Énergie et des Ressources Naturelles, 2015). To generate these products, 1,500,000 tons of tailings were created and deposited over 76 hectares (Ministère de l'Énergie et des Ressources Naturelles, 2015). This was at a time when there were no guidelines for environmental risk management. The tailings were composed of approximately 50% sulfide minerals with high concentrations of sulfur, arsenic, cadmium, copper, molybdenum, and zinc (Ministère de l'Énergie et des Ressources Naturelles, 2015). The low pH observed in the region was caused by exposing sulfide minerals (e.g., pyrite FeS2) to air and water and generating sulfuric acid: acid mine drainage. The two common chemical reactions in pyrite degradation are (Chandra and Gerson, 2010):
Production of H+ decreases pH and increases acidity. Atmospheric sulfur deposition (e.g., from smelter stacks in Rouyn-Noranda and Sudbury, or from long range atmospheric transport) also adds to the production of sulfuric acid affecting aquatic and terrestrial ecosystems.
Past mining activities and residual tailings left on site have seriously affected nearby aquatic receiving environments, principally the Arnoux River and Lake Arnoux. Restoration activities at the Aldermac site were initiated in 2008, 65 years after mine closure, and have since amounted to an estimated cost of more than US$16.5M (Ministère de l'Énergie et des Ressources Naturelles, 2015). Despite rehabilitation, the Arnoux River and one of its small tributaries (directly flowing through the tailings) were still acidic, with high concentrations of dissolved metals and high conductivities (measurements taken in July 2012; Leguay et al., in press). However, in the surface water of Lake Arnoux, pH seemed to recover during the course of this study from measured values of 4.03–5.37 in 2011 and 5.30–6.25 in 2012 to 6.58–6.77 in the fall of 2013 (unpublished data).
As more economic initiatives look to exploration and mining development in Canada's north, spatial, and temporal monitoring will be of increasing importance to assess environmental conditions. Governments are compelled to assess the environmental legacy of past mining practices in addition to the economic cost to society for remediation, while promoting mining in relatively pristine northern regions (e.g., the Quebec Plan Nord, the Ontario Ring of Fire, and the Northwest Territories Mining Initiative). As of 1995, the contemporary mining industry in Quebec must provide financial guarantees to ensure environmental remediation post-closure. In the province of Quebec alone, more than 100 abandoned mining sites are under the responsibility of the government, which has already injected millions of dollars into restoration (Ministère de l'Énergie et des Ressources Naturelles, 2015). In 2010, the mining legacy to the province reached an environmental debt estimated to be over US$600M (Ministère des Ressources Naturelles et Faune, 2011). With extensive new mining developments in northern Quebec expected in the near future, it is fundamental to understand their potential effects on aquatic ecosystems and to develop performance evaluation techniques for mine site rehabilitation in advance. The amount of public money invested in resource development should include scientific evidence of whether or not an adversely affected ecosystem has recovered or is recovering from mining contamination post-remediation.
Algae, particularly diatoms, are susceptible to changes in environmental conditions and are excellent indicators of past and present ecosystem integrity (Stoermer and Smol, 1999). Diatom assemblages, preserved in lake sediments, can provide valuable information about reference conditions and reference assemblages prior to mining activities. Studies from northern Quebec examined the impact of mining on diatoms and water quality and consistently recorded elevated metal concentrations in the sediments with altered diatom assemblages (Cattaneo et al., 2004, 2008; Laperrière et al., 2008). Diatom-based models, developed in northwestern Quebec, examined correlations between water quality and diatom assemblages (Fallu et al., 2000; Enache and Prairie, 2002; Dixit et al., 2007). The studies by Dixit et al. (2007) and Enache and Prairie (2002) specifically found strong correlations between diatoms and pH. Fallu et al. (2000) demonstrated strong linkages between diatom assemblages, alkalinity, and dissolved organic carbon in northern Quebec and Labrador. Environmental shifts can also have significant impacts on the higher trophic levels and the overall biological health and stability of freshwater systems.
The objective of this study was to investigate the downstream response of diatom assemblages to water quality changes caused by mining activities at the Aldermac mine. A sediment core was collected from Lake Arnoux in 2011, which is the most proximal lentic environment to the Arnoux River, directly downstream from the Aldermac site (Figure 1). Temporal changes in the fossil diatom assemblage composition were assessed in the Lake Arnoux core. The diatom profile from Lake Arnoux was also compared with the assemblages from sediment cores sampled at three sampling stations farther downstream to assess spatial changes with distance from the Aldermac site. In addition, the economic benefits of the Aldermac mine were examined with specific reference to profits gleaned vs. the costs of environmental damage and remediation. The sustainability of this model of government-led rehabilitation of abandoned mines in the public interest was explored.
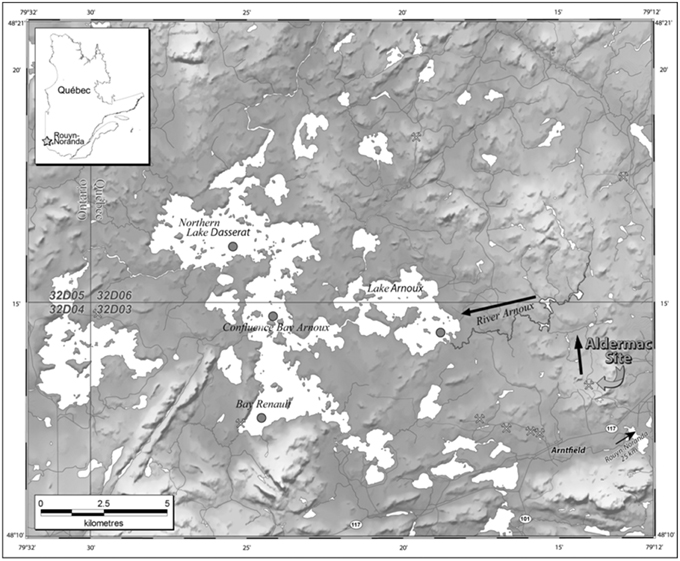
Figure 1. Locations of the former Aldermac mine and the sampling stations (gray dots) downstream in Lake Arnoux and Lake Dasserat. Black arrows identify surface water drainage flow from the abandoned mining site. Map sheets for this figure are 32D03, 04, 05, 06.
Methods
Study Area
The mining region of Abitibi-Timiskaming in northwestern Quebec (along the Ontario border) is underlain by the Precambrian Shield, covered by the boreal forest in Abitibi and a mixed forest in Timiskaming. Generally, natural waters in this region are low in nutrients, slightly acidic, and have high concentrations of dissolved organic carbon (DOC). The Aldermac mining site (derived from the names of the two prospectors Alderson and Mackay) is located near Arntfield, 25 km west of Rouyn-Noranda. Seventy-six hectares of tailings were left exposed on the site and were drained by small effluents, which reached the Arnoux River. The Arnoux River discharges into Lake Arnoux, a shallow water body ( < 5 m deep). In turn, Lake Arnoux feeds into Bay Arnoux and Lake Dasserat, a well-known lake in the area for recreational fishing, hunting, boating, and cottages. In the immediate vicinity, there were also eight smaller mining operations at varying times during the 1900s.
Sediment cores from Lake Arnoux, Northern Lake Dasserat, Bay Renault, and Confluence Bay Arnoux were collected in September 2011 (Figure 1). The Lake Arnoux coring station is the most proximal to the Aldermac site near the mouth of the Arnoux River (48°14.2843′N/79°19.0407′W). The entire lake is about 4.5 m deep and has no discernable depocentre for sediment focusing. The station was selected for its proximity to the mouth of the Arnoux River, the shores of which remain rich in tailings post-restoration. The other three sites are located in Lake Dasserat and have been named Northern Lake Dasserat (48°16.2658′N/79°25.6293′W), Bay Renault (48°12.5763′N/79°24.6881′W), and Confluence Bay Arnoux (48°14.7120′N/79°24.1266′W). Northern Lake Dasserat is the deepest point in Lake Dasserat (15.2 m). Confluence Bay Arnoux (approximately 9.5 m water depth) is just downstream from where Bay Arnoux drains into Lake Dasserat. Finally, Bay Renault is the deepest site in the southwestern quadrant of Lake Dasserat (approximately 14.5 m).
Sample Collection
At the three sampling stations in Lake Dasserat (Bay Renault, Confluence Bay Arnoux, and Northern Lake Dasserat), scuba divers from Environment Canada retrieved sediment cores (Mudroch and MacKnight, 1991). The cores were hand-taken by the divers to avoid or minimize compaction, smearing, tilting, and mixing of unconsolidated hydrous sediments, and to preserve the sediment–water interface (e.g., Baxter et al., 1981; Glew et al., 2001). Core lengths varied from 40 to 54 cm. The sediment core at Lake Arnoux was recovered by a modified gravity corer due to poor water quality conditions for diving. A 10-cm diameter gravity corer gently penetrated the sediments to permit preservation of both the core integrity and the sediment-water interface. The technique was appropriate for this shallow site and closely simulated the high quality of diver-taken cores. The length of the recovered core in Lake Arnoux was approximately 40 cm. Several cores were taken adjacent to each other at every station with each dedicated to complementary analyses after sub-sampling. Cores were extruded on site and sectioned at 0.5–1.0 cm depth intervals using either a mechanical extruder or a hydraulic extruder with water pressure advancing the core. Sediments cored in this study were either organic-rich (e.g., Lake Arnoux gyttja) or mixed glaciolacustrine clays from glacial Lake Barlow-Ojibway (e.g., Lake Dasserat; Veillette, 1994).
Samples were stored at 4°C on site, during transport, and upon return to headquarters. They were frozen at −60°C before they were transferred into a freeze-dryer and dried under a vacuum of less than 170 × 10−3 mBar for approximately 2–3 days. Samples from the same core were divided for diatom analyses and 210Pb dating. Adjacent cores taken at the same time and sites were treated similarly before submission for analyses of sulfur content.
Diatom Sampling, Processing, and Analyses
Diatoms collected from the four sediment cores were processed following the procedure of Gajewski et al. (1997); a minimum of 600 valves were counted from subsamples, documenting core sections of known dry weights. Taxonomic identifications were made to the lowest possible level. In this study, selected depths from each core were identified based on a general timeline of pre- and post-mining activity. Core samples were selected mainly from recent times (>1930s) with one sample from the early-1900s and one sample from the bottom of each core dating back to before 1850.
Diatom data were expressed as relative abundances to reflect changes in the assemblage structure, indicating potential fluctuations in water quality. Absolute values were also available and were used to support observations from the relative abundances. Taxonomic diatom species identifications were performed by comparison with photomicrographs published in floras from similar geographic regions and environmental conditions (Camburn and Charles, 2000; Fallu et al., 2000; Siver et al., 2005). Additional references were also used to assist in the identifications of rarer species (e.g., Krammer and Lange-Bertalot, 1985, 1986, 1988, 1991a,b). Photomicrographs of the prominent diatoms in this study are presented in Figure S1. Diatom profiles were generated with the software C2 version 1.7.6 (Juggins, 2014), including only the species present with relative abundance ≥ 5% in at least two core depth intervals.
Determinations of past pH and alkalinity in this study were based on the development of transfer function models using C2 and data from Siver et al. (2004, 2005). In their studies, Siver et al. (2004, 2005) developed weighted average models with boot-strapping validation using pH and alkalinity data (non-transformed) from 56 lakes. Taxa not present in at least two lakes were excluded, and in total, 218 taxa were included in the refined models used in this study. Although calibration models are optimal when developed from the region of study, Siver et al.'s (2004, 2005) data were used because they contained more taxa optima estimates for lower pH ranges (< 5.5), had key taxa found in this study (photographically documented), and were publically available. For discussion, additional taxonomic comparisons were made with average weighted mean optima developed from the eastern USA and northwestern Quebec (Camburn and Charles, 2000; Fallu et al., 2000; Gaiser and Johansen, 2000; Dixit et al., 2007). These studies were selected because of their locations, photomicrographic validations of taxa, and similar environmental conditions.
Sediment Dating
A total of 40 freeze-dried sediment samples (10 from each of the four cores) was analyzed for 210Pb (46.5 keV), 137Cs (661 keV), 214Bi (609 keV), and 214Pb (295 and 351 keV) by low-background HPGE gamma spectrometry (Appleby et al., 1986). Analysis was completed after storage for one month in sealed polypropylene vials. Sample storage prior to analysis was to achieve equilibration between 222Rn, 214Bi, and 214Pb with 226Ra. The excess 210Pb activity (210Pbex) was calculated by subtracting the 226Ra activity, as determined by the gamma emissions of 214Bi and 214Pb (its daughter isotopes), from that of measured 210Pb. Counting efficiencies were determined using standard solutions of mixed nuclides (QCYB40 from AEA Technology) and sediment material certified for 226Ra activity (IAEA-314 from the International Atomic Energy Agency). The measured activities were corrected for sample geometry and self-absorption (Appleby et al., 1992). In all cores, the vertical profiles of 210Pbex activity were characterized by an exponential decrease as a function of depth in the sediment (Figure 2; Figure S2). Assuming no mixing, constant sedimentation rate, and constant input flux of unsupported Pb (CF:CS model; Appleby and Oldfield, 1978), the sedimentation rate, ω, can be calculated using the following relationships:
where A and Ao are the activities of the excess 210Pb at depth z and at the top of the core, respectively, β is the slope of ln A vs. z, and γ is the decay constant for 210Pb (i.e., 0.03114 a−1). Sedimentation rates thus determined range between 0.16 and 0.29 cm a−1 depending on the sample site (Figure 2). Figure 3 shows 137Cs profiles for each core with peaks corresponding to the maximum atmospheric fallout of 137Cs as a result of nuclear weapons testing (1963–1964). The well-defined 137Cs peaks observed in all the cores coincide closely to the time period at which the atmospheric fallout of 137Cs reached a maximum (1963–1964).
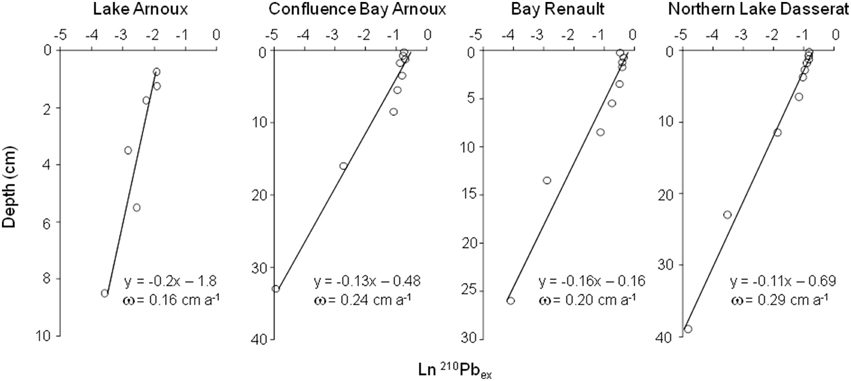
Figure 2. Ln210Pbex as a function of depth (cm) for each of the four cores. Due to some variability in the 210Pb profiles from Lake Arnoux, only the values that followed the expected decreasing trend in concentration as a function of depth were included in the model (4 data points excluded).
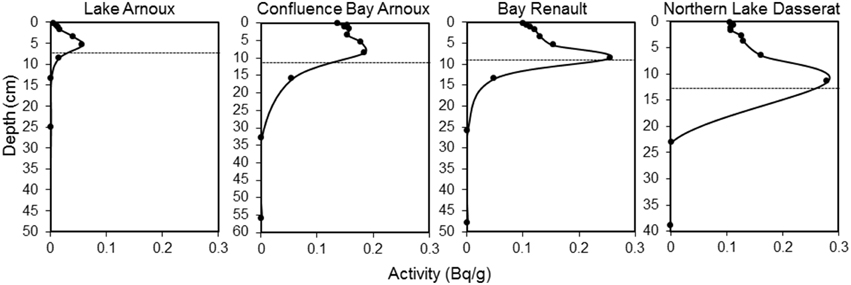
Figure 3. Plots of 137Cs activity as a function of depth (cm) for each of the four cores. The dotted line corresponds to the year 1963–1964 based on sedimentation rates calculated from 210Pb data.
Sulfur Content
After sediment samples were frozen and freeze-dried, they were sieved through an 80-mesh (177 μm) screen. Sediment dissolution was a modified aqua-regia treatment (1F Exploration Geochemistry aqua-regia ultra-trace analysis; Acme Analytical Laboratories, Limited, Vancouver, British Columbia; currently Bureau Veritas Mineral Labs AQ250/AR250 analytical package). Pulp-splits of 0.5 g samples were leached with a 6 ml mixture of HCl, HNO3, and distilled/deionized water (2:2:2 ratio by volume) at 95°C for 1 h. The sample solution was diluted to 20 ml and analyzed by inductively-coupled plasma mass spectrometry (ICP-MS; Perkin–Elmer Elan instrument) for sulfur contents with a detection limit of 0.02%. Certified reference materials (CRMs; LKSD-1 and LKSD-4), in addition to blind duplicates, were inserted into sediment sample runs for QA/QC. Sulfur (S) contents were expressed as % weight.
Results from aqua-regia digestion are considered a partial digest and were compared with those from multi-acid digestion, which represent near-total dissolution (1T Exploration Geochemistry multi-acid ultra-trace analysis by Acme Labs; equivalent to the current Bureau Veritas Mineral Labs MA250 analytical package; detection limit of 0.04%). Sediments splits of 0.25 g were heated in 10 ml of HNO3-HClO4-HF solution until fuming and were dried. The resulting residue was dissolved in 4 ml of 50% HCl and heated. After cooling, the solution was loaded into polypropylene test tubes and filled to make a 10 ml volume with 5% HCl before analysis by ICP-MS.
Although measurements of S content after the 4-acid digestion (near total) is consistently higher than those for aqua-regia, the mean difference in S content between the two methods is small (0.02% weight). The two datasets show identical trends in S content with depth at all sediment coring stations. For the purpose of this study, ICP-MS measurements from the aqua-regia digestion are presented.
Relevant Temporal Data Retrieved from Archives
Citizen letters of complaint reported tailings containment failure and environmental degradation of lakes and water courses downstream of the Aldermac mine to different branches of the Quebec government (Ministère du Développement Durable de l'Environnement et de la Lutte Contre les Changements Climatiques, 2015; obtained by access to information request). Two letters reported dead fish in Lake Arnoux dating back to 1948. In addition, two letters reported development of a rusty brown-colored bathtub ring along rocky shorelines of Lake Arnoux, which were observed during low water levels and are still evident today. One letter cites pH measurements in the Arnoux River and the center of Lake Arnoux, which were acidic in 1950 (pH of 3.6 and 4.5, respectively). Water analyses, also conducted in 1957 at different sites in the region, measured surface water pH as low as 2.4 in small tributaries of the Arnoux River (on the mine property; Ministère du Développement Durable de l'Environnement et de la Lutte Contre les Changements Climatiques, 2015). Additional pH data from 1957 to 1961, reported in citizen letters (Ministère du Développement Durable de l'Environnement et de la Lutte Contre les Changements Climatiques, 2015), record pH measurements ranging from 3.3 in Lake Arnoux (near Provencher Creek) to 3.9 in the northern portion of the lake. For this time period, surface water of Lake Dasserat (unspecified location) had a pH between 5.8 and 7.1, whereas Bay Arnoux (before discharging into Lake Dasserat) had low pH of 4.2–5, suggesting acidification downstream of Lake Arnoux (Figure 1). Another citizen, in a letter written subsequently in the 1970s, refers to Lake Arnoux as a dead lake, devoid of fish (Ministère du Développement Durable de l'Environnement et de la Lutte Contre les Changements Climatiques, 2015). Apart from reported fish observations, there was little to no evidence that the biology and ecosystem health of water systems discharging from the mining site were monitored.
Results
Sediment Sulfur Content
Sediments below 20 cm depth had consistent sulfur (S) values of < 0.06%S. The S content profiles for the three cores in Lake Dasserat showed consistent patterns with %S peaks observed at sediment depths of 8–10 cm (Figure 4), occurring after 1965. In all three cores from Lake Dasserat, the initial increase in %S occurred after 1943. The S peak in Lake Arnoux occurred at a depth of 4–6 cm after 1965, but %S started to increase at ~15–17 cm (early 1900s). The highest %S (2.5%) was observed in Lake Arnoux. Peak %S contents correspond to 7- to 64-fold increases from the late 1950s to the 1970s. All profiles indicated an increase and subsequent decline in %S over a 60-year period. Current S content of approximately 0.1% is evident in Northern Lake Dasserat and Confluence Bay Arnoux. Concentrations in Bay Renault persisted at 0.7% and Lake Arnoux registered 0.4%S. At all sites, the rate of decline was equal to the rate of the initial increase, with the steepest increase and decrease in Lake Arnoux.
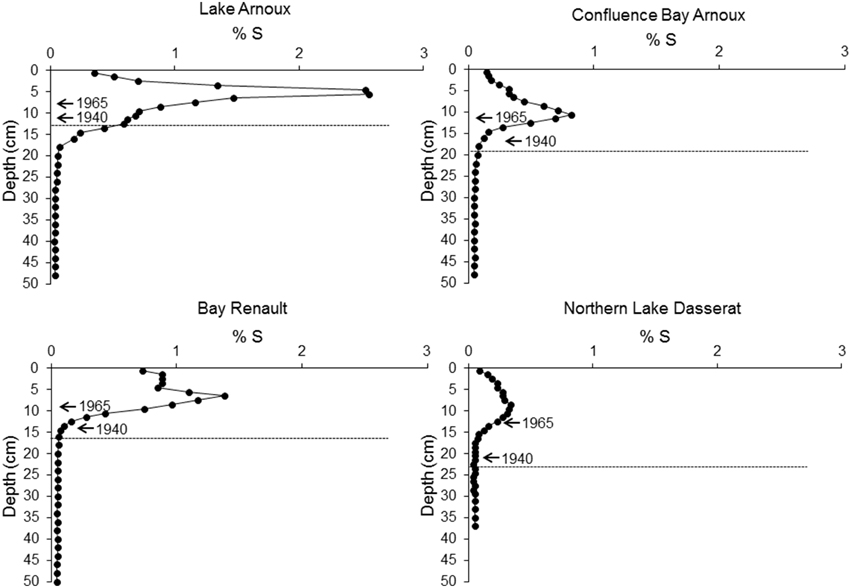
Figure 4. Profiles of total percent sulfur concentrations in sediments for each of the four cores. 210Pb dates aligned with sediment depth are presented with arrows. The dotted line corresponds to the pre-mining period based on sedimentation rates determined with 210Pb data.
Diatom Assemblages through Time and Space
The total number of taxa found in all four core sediments combined was 327, representing 64 diatom genera. The genera with the most species were benthic, represented by Navicula Bory, Nitzschia Hassall, and Pinnularia Ehrenberg. Sixteen percent of the taxa were planktonic, predominantly from the genera Aulacoseira Thwaites and Fragilaria Lyngbye. Calculated valve densities, indicative of diatom productivity, ranged from 159 to >1650 million cells g dry weight−1. The lowest average cell densities were observed in Lake Arnoux at 251 million cells g dry weight−1. The highest average densities were 671 million cells g dry weight−1 recorded from Bay Renault.
Overall, Asterionella formosa Hassall, Tabellaria flocculosa (Roth) Kütz. sensu lato, and Aulacoseira subarctica (O.Müller) Haworth were the dominant taxa, in terms of occurrence and relative abundance, in all four cores (Figure S1 and Data Sheet 1). However, these taxa were absent or quasi absent in the top 6 cm of Lake Arnoux sediments and did not reach a relative abundance of ≥5% in this core. Aulacoseira pusilla (Meister) Tuji and Houli was present in all four cores (Figure S1 and Data Sheet 1) but only found with abundances ≥12% in Lake Arnoux with the exception at the top 6 cm where it was completely absent (Figures 5–8). Cyclotella lemanensis O.Müller sensu lato and Discostella stelligera (Cleve and Grunow) Houk and Klee sensu lato were also present in all four cores with abundances generally ≤10% (Figures 5–8). These two taxa were also absent in the top 6 cm of the Lake Arnoux sediments (Figure 5). The abundance of T. flocculosa increased in Lake Arnoux from 16 to 7 cm and then disappeared (Figure 5). In Confluence Bay Arnoux and Bay Renault, T. flocculosa increased after the initiation of the mine and stabilized (Figures 6, 7). In contrast, T. flocculosa decreased from 13 cm depth to present in Northern Lake Dasserat (Figure 8). A. formosa was present in larger numbers (>5%) at all the Lake Dasserat sites and was < 5% in Lake Arnoux. Prior to 1940, A. formosa relative abundances in Northern Lake Dasserat, Bay Renault, and Confluence Bay Arnoux were lower with an increase during the mining and post-mining period. Recently there has been a reduction in A. formosa similar to pre 1940s levels (Figures 6–8). In Lake Arnoux, A. formosa was absent in the counts after 1975 (Figure 5). The proportion of benthic diatom species was relatively constant throughout the cores (20–30% of the assemblages), with the exception of Lake Arnoux, where a sharp increase, up to 99%, was observed in the top 6 cm (Figure 5). Brachysira microcephala and Fragilaria crotonensis were prominent spatially-distributed tychoplanktonic/benthic species in Lake Dasserat (Confluence Bay Arnoux, Bay Renault, and Northern Lake Dasserat; Figure S1 and Data Sheet 1 and Figures 6–8), whereas Frustulia saxonica and Pinnularia subcapitata var. elongata dominated in Lake Arnoux (Figure 5). Numerous other benthic species were observed in this study, but their relative abundances were < 5%.
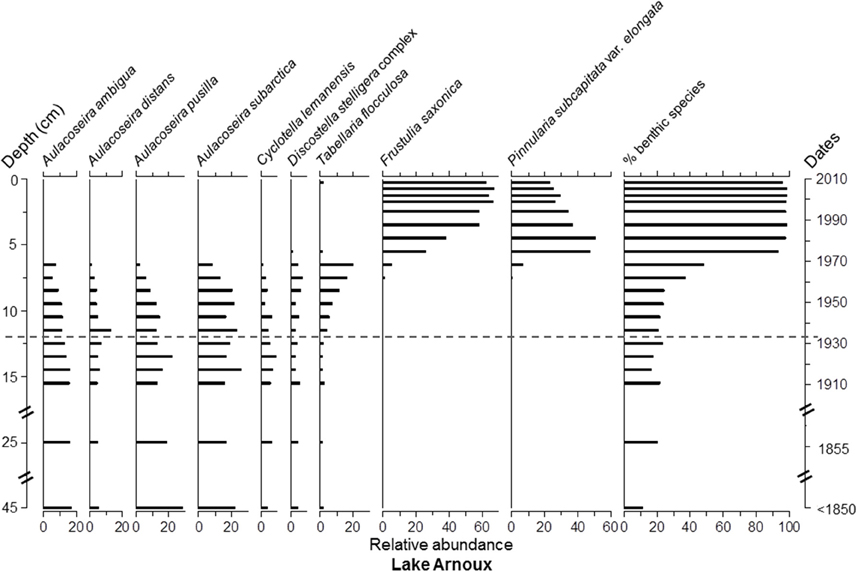
Figure 5. Diatom taxa paleolimnological profiles represented as % abundance in the benthic sediments of Lake Arnoux.
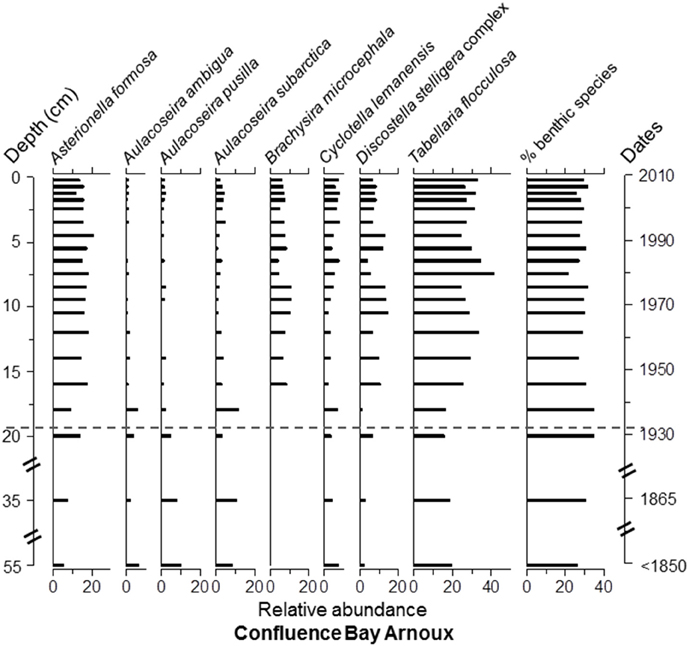
Figure 6. Diatom taxa paleolimnological profiles represented as % abundance in the benthic sediments of Confluence Bay Arnoux. The dotted line corresponds to he pre-mining period based on sedimentation rates determined with 210Pb data.
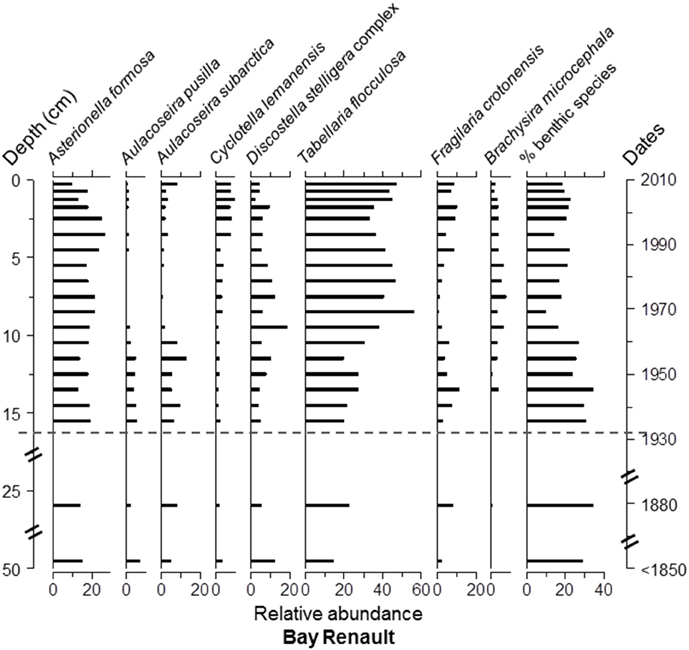
Figure 7. Diatom taxa paleolimnological profiles represented as % abundance in the benthic sediments of Bay Renault. The dotted line corresponds to the pre-mining period based on sedimentation rates determined with 210Pb data.
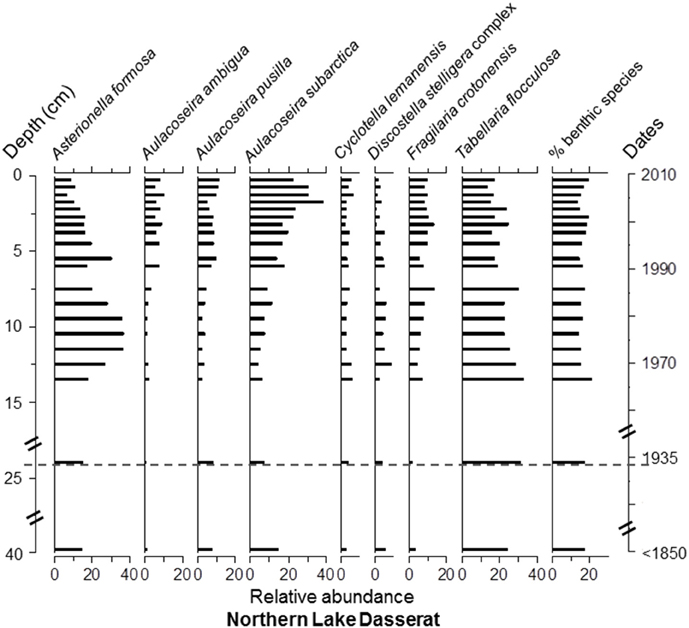
Figure 8. Diatom taxa paleolimnological profiles represented as % abundance in the benthic sediments of Northern Lake Dasserat. The dotted line corresponds to the pre-mining period based on sedimentation rates determined with 210Pb data.
The assemblages from Lake Arnoux showed an abrupt change in taxonomic composition and life form in the top 6 cm of the core (circa 1965), switching from a dominance of planktonic Aulacoseira species to a dominance of benthic acidophilic taxa, including F. saxonica and P. subcapitata var. elongata (Figure 5). This marked shift in the assemblages was preceded by a gradual increase in T. flocculosa and a subtle decrease in A. ambigua and A. pusilla between 12 and 7 cm depth. Chrysophyte cysts (not shown) decreased gradually from 68.4 million at the bottom to 7.9 million cysts g dry weight−1 at the top of the core.
The three cores from Lake Dasserat recorded similar and less variable profiles in the diatom assemblages. Nevertheless, some changes in the structure of the assemblages are worth noting. For example, in the Confluence Bay Arnoux core, the benthic species, B. microcephala, appeared at 16 cm and remained present to the top of the core with relative abundances between 5 and 12% (Figure 6). This observation was accompanied by a decrease in Aulacoseira species, as well as an increase in A. formosa, D. stelligera, and T. flocculosa. These changes correspond to the estimated period of approximately 1940. Despite the sudden appearance of B. microcephala, the overall proportion of benthic species did not increase to a large extent in the core. However, from 16 cm to the top of the core, this species dominated the benthic assemblage, whereas more diverse benthic assemblages were present in the older sediments. In Bay Renault, the most significant modification in the diatom assemblage structure happened circa 1965 showing an increase in T. flocculosa with abundances between 15 and 30% down-core and 30–55% from a depth of 10 cm and up (Figure 7). This increase in T. flocculosa was preceded by the appearance of B. microcephala circa 1945, but with abundances never ≥8%. In Northern Lake Dasserat, the most significant changes through time were the increase in Aulacoseira spp. (particularly of A. subarctica) and F. crotonensis, and the overall general decline of A. formosa and T. flocculosa circa 1990 (Figure 8).
Diatom pH and Alkalinity Reconstructions
The weighted averaging model for pH (data not transformed) using inverse deshrinking was:
with an R2 of 0.85, a root mean square error (RMSE) of 0.35 and a root mean square error of prediction (RMSEP) of 0.44. A selection of the prominent taxa in this study showed a broad spectrum of pH optima estimates (Data Sheet 2). P. capitata var. elongata, B. serians, and F. saxonica had the lowest pH optima, 4.8, 4.82, and 4.92, respectively. In contrast Ulnaria ulna, Stephanodiscus niagare, and A. formosa had higher pH optima determinations of 7.34, 7.29, and 7.00, respectively.
Estimates of pH in Lake Arnoux from < 1850 to present varied from 4.09 to 7.17 (Figure 9). Eight diatom taxa, representing 60–95% of the community, were central to these pH determinations. There was a significant drop in pH between 5.5 and 8.5 cm (circa 1965). Below 8.5 cm the estimated pH ranged from 6.74 to 7.34, whereas between 5.5 cm and 0.25 cm, the estimated pH ranged from 4.09 to 4.42. Between 7 and 31 taxa were used in the pH reconstructions. In contrast, Bay Renault, Confluence Bay Arnoux, and Northern Lake Dasserat had estimated circumneutral pH-values throughout the core sections (pH ~7.0). Confluence Bay Arnoux from 0.25 to 15 cm had estimated pH-values at or below 7.0 (Figure 9), whereas Bay Renault and Northern Lake Dasserat had values at and slightly above 7.0. Confluence Bay Arnoux, Bay Renault, and Northern Lake Dasserat used 19–30, 15–32, and 14–27 taxa in the down-core reconstructions, respectively.
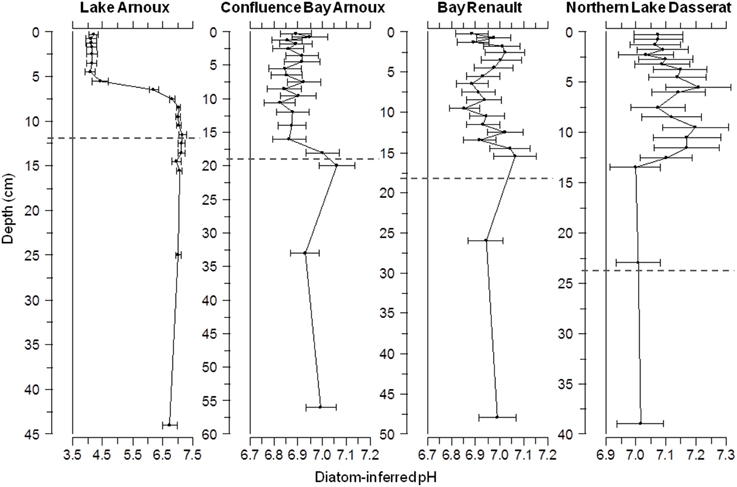
Figure 9. Profiles of diatom-inferred pH-values for Lake Arnoux, Confluence Bay Arnoux, Bay Renault and Northern Lake Dasserat. The dotted line corresponds to the pre-mining period based on sedimentation rates determined with 210Pb data. The dotted line corresponds to the pre-mining period based on sedimentation rates determined with 210Pb data.
The weighted averaging model for alkalinity (data not transformed) was:
with an R2 of 0.73, a RMSE of 33.15 and a RMSEP of 41.9. Estimates of alkalinity from Lake Arnoux ranged from −72 to 109 μeqL−1 (standard errors varied from ± 42 to 90 μeqL−1), and showed consistent values up to the 1950s with estimated concentrations ranging from 73 to 103 μeqL−1 (graph not shown). After the 1950s, alkalinity dropped to negative estimates, with the lowest values between the depths of 0.25 and 5.5 cm. The low buffering capacity of the lake prior to the 1950s changed to no buffering capacity after the reported tailings pond breach. Alkalinity levels were stable and improving slightly at the top of the core (2011). Lake water alkalinity in August 2013 was measured at 160 μeqL−1 (unpublished data), indicating recent improvements in water quality and buffering capacity. In contrast, alkalinity estimates from < 1850 to present from Confluence Lake Arnoux, Bay Renault, and Northern Lake Dasserat ranged from 69 to 107 μeqL−1 with estimated standard errors ± 41 to 44 μeqL−1. The estimated alkalinity profiles were strongly correlated with the estimated pH profiles and, therefore, are not presented here.
Discussion
Sedimentation Rates and Sulfur Content
The sedimentation rates (0.16–0.29 cm a−1) calculated for the Lake Arnoux–Dasserat system were comparable with published studies for other boreal lakes in the region (Moingt et al., 2014). Sedimentation rates estimated using the 137Cs profiles and the dating horizon were relatively similar to values obtained based on the CF:CS model. This indicates that the chronology for this study is reliable at the stations in Lake Dasserat. The 210Pb sedimentation rates for all three sites in Lake Dasserat were similar (0.20–0.29 cm a−1).
In contrast, the coring station in Lake Arnoux has an estimated sedimentation rate of 0.16 cm a−1. Considerations include the nearly uniform shallow bathymetry of Lake Arnoux (4–5 m) and lack of a clear sediment depocentre, so that sedimentation would be spatially distributed in the immediate area of the mouth of the Arnoux River. The approximate correlation of the 137Cs peak with a sediment date of 1963–1964 (Figure 3) further indicates that the higher sedimentation rates recorded at stations located in zones of sediment focussing in Lake Dasserat were not possible at the coring station in Lake Arnoux. However, it is reasonable to expect that sediment mixing (e.g., by wind action or bioturbation) in Lake Arnoux could have compromised the dating to some degree.
Marked increases in S content were observed after 1950 in Lake Arnoux, with the initial increases staring at about 16 cm (15–17 cm sediment section). The age error associated with this 2 cm sediment section represents ± 12 years, which would place the initiation of mining operation at about 1917. This is before the opening of the mine and the difference in chronologies could be associated with some uncertainties with the 210Pb dating for Lake Arnoux (low number of data points). The uncertainties in dating could be simply a problem of sediment mixing in the bottom of Lake Arnoux, which could also account for the presence of increasing %S lower in the sediments (15–17 cm). In addition, there could be a possibility of soluble S migration downward in the sediments resulting in a subtle %S gradient. Finally, we have no information about the occurrence and duration of pre-mining activities which could account for some early increases in %S.
During the mining operation (1932–1943), there was a consistent increase in %S in the sediments of Lake Arnoux which halted briefly before the sharp increase after 1965 peaking at 2.5 %S. The immediate drop in S from the 1960s to present was at the same rate as the increase, which suggests that there was a single large pulse of S influx in the late 1960s and early 1970s. Coupled with recent findings of high S levels in tributaries of the Arnoux River near the Aldermac site (>10000 μM SO2−4, Leguay et al., in press), it appears that the primary source of S is the Aldermac site. Bay Renault had 46%, Confluence Bay Arnoux 60% and Northern Lake Dasserat 85% less S, relative to Lake Arnoux (based on peak concentrations). Sediments from Lake Arnoux recorded increases in S content before 1940, whereas increases at the Confluence Bay Arnoux and Bay Renault stations started after circa 1950. As for Northern Lake Dasserat, increases in S content occurred after 1960, but were much lower. These results support an aquatic vector for the migration of S away from the Aldermac mining site. Although these findings seem logical, S can also be influenced by other vectors (e.g., westerly winds, water discharge rates). The Lake Dasserat region is also within the wind circulation and zones of influence of smelters in Sudbury (Ontario) and Rouyn-Noranda (the Horne smelter; e.g., Olsen et al., 1982; Mayer et al., 2007). Therefore, other industrial sources could contribute to S deposition in the study lakes by atmospheric transport.
Evidence for a tailings pond breach on the Aldermac property was reported by Bédard (2000), highlighting that a mining waste containment structure broke in 1942. Water contamination and dead fish were also reported from Lake Arnoux in 1948 (Ministère du Développement Durable de l'Environnement et de la Lutte Contre les Changements Climatiques, 2015) in addition to a citizen's letter of complaint from 1959 (Ministère du Développement Durable de l'Environnement et de la Lutte Contre les Changements Climatiques, 2015) of a tailings containment failure on the Aldermac property and resultant release of contaminated waters into Lake Arnoux and beyond. A citizen's letter also mentioned that the plume of contaminated waters from Lake Arnoux discharging into Lake Dasserat was visible from the air and looked like the “tentacles of an octopus” (Ministère du Développement Durable de l'Environnement et de la Lutte Contre les Changements Climatiques, 2015). It is not discernible whether these reports were independent records of the same tailings pond breach or were two separate breaches. The change in accumulation rates and sharp increases in S in Lake Arnoux over a short period are consistent with the tailings pond rupture explicitly reported. The sharp decline in %S after the peak indicated a discrete pulse of S input, which abruptly ceased. High 210Pb dating errors limit the precise determination of breach year(s). Similar patterns in S content distributions were also evident at the more distal Lake Dasserat coring stations, but were not as distinctive. In addition to atmospheric transport and other local mining activities, the surface water flow in the Lake Arnoux–Dasserat system was also altered. For example, the regional drainage divide was shifted by damming to accommodate the early logging industry, which relied on wood floating for timber transportation to the province of Ontario, causing a flow reversal in Lake Dasserat (Organisme de Bassin Versant Abitibi-Jamésie, 2015). This reorganization of the watershed became problematic for the hydropower station situated to the north because of reduced flow. A decision was later made to return the drainage divide line to its natural position in 1942 with the construction of multiple dams still in place today (Organisme de Bassin Versant Abitibi-Jamésie, 2015). The surface flow reversals could have affected aquatic transport of S into Bay Renault after mining operations ended. However, flow reversal in Lake Dasserat did not affect S content and diatom composition in Lake Arnoux.
Past Mining Activities and Diatom Assemblages
The striking turnover in diatom composition (planktonic to benthic assemblages) observed in the Lake Arnoux core clearly demonstrates major temporal environmental changes related to a gradual, then rapid decline in pH. The reconstruction of pH from the diatom transfer function model showed pre-industrial pH of about 7.0, similar to the three sampling stations in Lake Dasserat. This level was maintained up to the 1950s, then dropped dramatically in alignment with the pulse of S input from the reported rupture of a tailings pond on the abandoned mine site (Citizen report, Ministère du Développement Durable de l'Environnement et de la Lutte Contre les Changements Climatiques, 2015). pH-values of 3.3 and 3.9 reported in Lake Arnoux between 1957 and 1961 coincide with the reported tailings pond rupture (Ministère du Développement Durable de l'Environnement et de la Lutte Contre les Changements Climatiques, 2015). The pH reconstruction at the top of the core (2011) was 4.2, whereas the modern measured pH value for Lake Arnoux was 4.5, which is within error of the model. Increases in surface water pH from an average of 4.5 in 2011 to 5.7 and 6.7 in 2012 and 2013, respectively, in addition to the early reappearance of the planktonic T. flocculosa, are early indicators of a temporal trend toward less acidic waters.
The changes in diatom assemblages and water quality in Lake Arnoux started from 1930–1940, when the Aldermac mine was operating. However, increases in %S in the sediments peaked in the mid to late 1960s and followed the same increasing trend as T. flocculosa. The peak in %S matched the abrupt changes observed in the diatom community from planktonic to benthic species. Changes in lake water pH were significant from circum-neutral or slightly acidic waters before mining to strongly acidic waters after mining. Lake acidification was reported by local citizens (Ministère du Développement Durable de l'Environnement et de la Lutte Contre les Changements Climatiques, 2015) and by inferred pH from the diatom transfer function model. The similar rates of increase and decrease at all the sampling stations, up to 10 km from the Aldermac site, indicate that there was a regional effect farther downstream. The slow increase and decrease in %S and lower maximum S content at the Northern Lake Dasserat site also suggest a lag response compared to more proximal sites.
The change of the diatom community in Lake Arnoux, after mining operation terminated, and the coincident peak in S levels, indicates a tolerance of the diatom community to stressors. The pulse of S-rich sediment input into Lake Arnoux during the tailings breach could have blocked the sunlight because of increased suspended particulate matter, reduced buffering capacity and altered pH. In reaction to this stress, the plankton community declined, and more tolerant acid-loving benthic diatoms appeared, like acidophilic F. saxonica and P. subcapitata var. elongata. The new light condition favored benthic diatom development, resulting in a suitable environment for the dominance of acidophilic species like F. saxonica and P. subcapitata var. elongata. Lower abundances of chrysophyte cysts toward the top of the core suggest lower numbers of living planktonic chrysophytes, which is consistent with the disappearance of prominent planktonic diatoms during the same period. The total disappearance of planktonic taxa and abrupt transition to benthic taxa show that primary productivity in Lake Arnoux switched from planktonic to benthic. However, the lake sediment record indicates that the change in diatoms was not likely instantaneous but developed over a period of up to 5 years, which is consistent with a biological lag response. The paleolimnological transitions in diatom composition were in two phases: (1) an increase in the planktonic taxa T. flocculosa (circa 1942–1960); and (2) a change from planktonic to benthic species (circa 1960–1970).
In other sectors of the Abitibi mining region, pH has been highlighted as a significant environmental stressor. The fossil diatom assemblages of Lake Perron (current pH 3.72) and Lake de la Pépinière (current pH 4.7) included the benthic F. saxonica, but not P. subcapitata var. elongata (Dixit et al., 2007). Overall the three lake systems (Arnoux–Dasserat, Perron, and de la Pépinière) had different fossil diatom assemblages, although all had predominantly benthic acidophilic species, indicating clear-water lakes.
Although valve deformities have been observed in low pH and metal-contaminated waters (e.g., Cattaneo et al., 2004; Falasco et al., 2009; Morin et al., 2012), they were rare in this study. Some species are tolerant of high metal concentrations and can be good indicators of elevated metal levels (Morin et al., 2012). For example, a number of taxa within the genus Pinnularia have been reported as acid-tolerant organisms occurring in acidic pit ponds (e.g., P. ferroindulgentissima) and bogs (P. subcapitata var. elongata) (Czarnecki and Cawley, 1997; Krammer, 2000). This can explain, in part, why deformed valves were not observed in Lake Arnoux. Further, Verb and Vis (2000) reported that P. subcapitata was present in acid mine drainage waters and tolerant of elevated metal concentrations (e.g., Al, Fe, Mn). Verb and Vis (2000) also reported that Frustulia rhomboides, a closely aligned taxon to F. saxonica, was dominant in acid mine drainage waters. Finally, the low species richness observed in Lake Arnoux after the mid-1960s can be explained by the highly acidic and elevated metals environment, suggesting that species richness or diversity can also represent an indicator of mining effects.
The paleolimnological diatom assemblages at all three sites in Lake Dasserat were generally invariant over the last 200+ years, with planktonic diatoms dominating the assemblages. The open water site of Northern Lake Dasserat showed some changes in the diatom composition (from A. formosa and T. flocculosa to A. subarctica and F. crotonensis; two more circum-neutral species living in similar nutrient conditions) from the early 1990s to present, but the changes were not evidently linked to pH. Both Bay Renault and Confluence Bay Arnoux sites demonstrated an increase in T. flocculosa from the early 1900s up to 1965 and then became stable to present day. There was also an introduction of the benthic species Brachysira microcephala, an acid-loving species, at these sites (< 10% abundance). These subtle changes in the diatom community possibly suggest minor shifts in lake water alkalinity or pH, but there was no statistical significance to the change. Confluence Bay Arnoux is distal enough from the immediate discharge of Lake Arnoux, and within the buffering capacity of Lake Dasserat, to minimize the environmental effects of S input. Other mining activities near Bay Renault may have affected water quality and induced the presence of B. microcephala.
Mining Economics vs. Environmental Debt
Mining, both past and present, has been a significant contributor to the Canadian economy. Today, mining and associated processing industries contribute an estimated US $34 B a−1 to the economy (Marshall, 2014). Over the last 5 years this represented an average contribution of 5% to the Canadian Gross Domestic Product with tax estimates to federal and provincial/territorial governments at US $7.6 B a−1 (Marshall, 2014). The mining industry directly accounts for approximately one in every 46 Canadian jobs and, indirectly through goods producing sectors, contributes one in every 10 jobs. In 2011, mining added US $10.3 B to Quebec's economy by employing 85,000 in 32 mines and plants (GEreports, 2015). The region of Rouyn-Noranda and Val d'Or in Quebec is considered one of the seven major Canadian frontiers, which were developed through mining.
Since 1995, mining companies operating in Quebec are obligated to submit both a mine rehabilitation plan and closure plan to the government of Quebec for approval (Ministère de l'Énergie et des Ressources Naturelles, 2015). The 1995 Mining Act was intended to protect the government of Quebec from unforeseen site restoration expenditures and ensure that mining companies followed socially and environmentally acceptable practices. A modified version of the Act was enacted in 2013, increasing the liability of the mining company to 100% of the projected rehabilitation costs. This, along with increased royalties and taxation, has dropped Quebec from 1st to 21st in the world's ranking for mining exploration (GEreports, 2015). Both a mine remediation plan and closure plan must be approved before mining leases can be granted. This is in stark contrast to the early 1900s, when no such legislation or environmental risk assessment existed. It is no surprise that mining companies, like the proprietors of the Aldermac mine, abandoned the site after operations ceased, without any notion or obligation in site rehabilitation and further environmental restorations. With respect to older abandoned or orphaned mines in Quebec, it becomes the responsibility of the government to deal with the legacy of environmental degradation and accompanying debt. Large amounts of money need to be invested to reclaim the sites. In the case of the Aldermac property, to date over US $16.5 M in restoration costs were spent since 2008. Adjusted for inflation, this government expenditure for site remediation in 1942 would have been equivalent to circa US$ 1.3 M (United States Department of Labor, 2012). If known at the time, the magnitude of the rehabilitation expense would have been weighed by past governments against revenues from taxes, economic development, and employment.
As a cost-benefit analysis exercise, an estimate of the time-equivalent value of resources extracted from the Aldermac mine can be compared with costs of environmental restoration. Over the 11-year period of operation, the Aldermac mine produced circa 200,000 tons of extracted resources (Ministère de l'Énergie et des Ressources Naturelles, 2015). This grossed an estimated US $130 M based on metals market values of 1943 (Schodde, 2010; Macrotrends, 2015). Presented at today's market values, (inflation adjusted, not market adjusted) these commodities of copper, silver, and gold would represent circa US $1.7 B (United States Department of Labor, 2012). Based on a 7% corporate taxation rate for the mining industry in 1935 (Vallières, 2012), the Aldermac mine returned circa US $9.1 M to the government of Quebec; inflation adjusted to 2010, this represents US $121.7 M. The inflation-adjusted tax, averaged over the lifespan of Aldermac operations, would represent 1.2% of the current mining tax revenue generated for Quebec per year (based on 2011 taxation data). In today's economy, the Adermac mine would be an important contributor to the GDP of Quebec. A simple calculation of profit vs. rehabilitation expenses ($121.7–16.5 M) would further suggest that the government of Quebec ultimately profited from the Aldermac mine even after pay-it-backward remediation activities. It is important to also recognize the unaccounted economics of mining during the development of a young country. In the 1930s, Canada was in the middle of the Great Depression of North America. People needed jobs to survive and governments needed tax dollars. Based on the cost to mine copper in 1943 (Schodde, 2010), the primary metal commodity produced at the Aldermac mine, it is estimated that US $68 M was spent in mining costs to build and operate the mine; a large proportion of this amount in support of both local and Quebec-based business. No additional estimates of economic benefits can be made credibly because data are not available. In total, even with the pay-it-backward environmental costs, the economic benefits justified the extraction of these mineral resources during the 1930s using the technology and ethical values of the period.
However, the results here show a significant environmental alteration in Lake Arnoux with substantive S loading, along with drastic changes in pH and alkalinity. These changes in Lake Arnoux have biologically altered lake health. Remediation expenses of $16.5 M represent costs toward environment stabilization (shoreline reconstruction, containment of seepage, etc.) but not directly toward removal of the problem. The proposed remediation plan is containment and stabilization on the mining property itself, with natural biological (e.g., ecosystem recovery) and physical process (e.g., sediment capping) doing the work of “cleaning the environment” on the site and farther downstream. The cost to remove the contaminants would be prohibitive and change the economic balance-sheet.
Based on modern economics, paying-it-backward in rehabilitation costs and unforeseen future socio-economic costs is not socially or financially acceptable (Ministère du Développement Durable de l'Environnement et de la Lutte Contre les Changements Climatiques, 2012). To assess the cost to global and human sustainability, the economics of conserving and recycling resources must also be considered along with the value of restored ecosystems, including goods and services they supply. New approaches in assessing the economics of our terrestrial and aquatic footprints will effectively merge sustainable environmental conservation with industrial development (e.g., Hoekstra et al., 2011; Zhi et al., 2015). For example, the restoration of the Solbec-Cupra mining site in Quebec during the 1990s, at a cost of US $4.8 M, resulted in a high-quality environment. The restoration created goods and services (tourism, recreation, new development, refugia development for wildlife) with an estimated worth ca. US $0.75–$1 M per year in 2009 (Vittet, 2011). Estimating goods and services of an ecosystem after mine site restoration (sustainable economics) represents an interesting argument for site remediation investments and improved rehabilitation plans.
Future-proof mining represents a global initiative of the mining industry to streamline costs, centralize knowledge, and optimize efficiencies (GEreports, 2015). Mining will not be for new resource prospects but for the best new prospects. The new business model will optimize productivity and limit environmental liabilities under the economics of lower profit margins (GEreports, 2015). Investments in post-restoration monitoring will be a fundamental component of future mine site remediation plans. As standard planning, secondary economic benefits should be incorporated into mining initiatives, whereby high-quality environments will be created with accompanying goods and services. This ad hoc assessment should not only be restricted to the directly affected site, but also extend to adjacent receiving environments. The social license to operate is generally perceived to be more crucial in areas downstream of mining operations (e.g., tourism, recreation, local community health). In the current study of the abandoned Aldermac mine, adjacent lake environments (Lake Arnoux, Lake Dasserat) were economically impacted with respect to hunting, fishing, tourism, and recreation. Micro-economics, along with more stringent environmental legislation, will drive the new model of mining economics.
Conclusions
A multi-proxy assessment was used to evaluate the water quality and ecosystem health of lakes downstream of the abandoned Aldermac mine in northwestern Quebec: (1) diatoms were used as paleolimnological bio-indicators to evaluate spatial and temporal environmental effects of mining massive sulfide deposits nearly eight decades ago, (2) sulfur content of lake sediments was analyzed as a pH driving vector, and (3) paleolimnological findings supported testimonial observations, which provided documented evidence for contaminant releases by on-going acid mine discharge and discrete tailings breach(es) after the mine was abandoned. Diatom assemblages in lake sediments indicate that Lake Arnoux was similar in pH, water quality (oligotrophic to weakly mesotrophic) and diatom composition as other lakes in the region before the onset of mining. Post-mining, the surface water of Lake Arnoux became increasingly acidic; after 1940 a shift from circumneutral to strongly acidic conditions were inferred from diatom assemblages. Once acidified, the water column allowed for the development of a poorly-diverse benthic diatom community, dominated by acid-loving metal tolerant species, in place of the planktonic species.
There were potential early signs that restoration activities (initiated in 2008) were starting to return receiving aquatic environments to pre-mining states, from a marked rise in surface water pH in Lake Arnoux and the early appearance of a planktonic diatom. However, it is clear that increased investments in post-restoration environmental monitoring are required. Monitoring, both on the abandoned mine property and in receiving environments downstream, serves as a tool for performance measurement of restoration strategies and adaptive management. The ultimate success of remedial restoration initiatives necessitates performance measurement in both on-going economic costs and long-term health of the environment.
During the Great American Depression, mining initiatives, like the Adermac mine, supported the economy of Quebec and Canada. Based on the historical period of economic growth for Quebec and time-equivalent value, revenues from the Aldermac mine were significant and justify the current costs for environmental remediation. However, this model is not sustainable in the modern economy and does not meet the requirements for environmental sustainability of resource development. New approaches, like the gray water footprint modeling (e.g., Zhi et al., 2015) are required to merge economic and environmental sustainability for future prosperity.
Conflict of Interest Statement
The authors declare that the research was conducted in the absence of any commercial or financial relationships that could be construed as a potential conflict of interest.
Acknowledgments
The study was funded under the Environmental Geoscience Program, Earth Sciences Sector of Natural Resources Canada (ESS Contribution number: 20150051). A RAC 2014–2015 grant from the Canadian Museum of Nature to P. B. Hamilton further supported this research. The authors wish to acknowledge R. J. McNeil and A. Grenier from the Geological Survey of Canada, Natural Resources Canada, for scientific and field support, in addition to L. Grapentine, B. Gray, and the Technical Operations Division of Environment Canada in Burlington, Ontario, for joint field work and diver-taken cores in September 2011. C. Gobeil is gratefully acknowledged for review of sediment dating results as is A. S. Dixit for helpful review of this manuscript. The authors would also like to thank E. Saulnier-Talbot for constructive discussions. Sample preparation was completed in the Sedimentology Laboratory, Geological Survey of Canada in Ottawa; radiometric dating was done by L. Rancourt, Institut nationale de la recherche scientifique, Centre Eau Terre Environnement, in Quebec City; and S content in sediments was analyzed at Acme Analytical Laboratories in Vancouver, British Columbia.
Supplementary Material
The Supplementary Material for this article can be found online at: http://journal.frontiersin.org/article/10.3389/fevo.2015.00099
Figure S1. SEM images of the prominent diatoms in Lake Arnoux. 1, Frustulia saxonica, whole valve external view; 2, Frustulia saxonica, valve apex internal view; 3, Pinnularia subcapitata var. elongata, internal view; 4, Pinnularia subcapitata var. elongata, apex internal view; 5, Tabellaria flocculosa, external view; 6, Asterionella formosa, external view; 7, Aulacoseira subarctica, external view; 8, Aulacoseira pusilla, external view. Scale bars: 1 = 20 μm; 3, 5, 6 = 10 μm; 2 = 5 μm; 4, 7, 8 = 2 μm.
Figure S2. 210Pbex as a function of depth (cm) in the sediments for each core.
Data Sheet 1. Diatom counts (relative abundances) for each of the four cores.
Data Sheet 2. Spectrum of pH optima estimates for the prominent taxa observed in this study.
References
Amaral, P. S. (2002). The great depression in canada and the united states: a neoclassical perspective. Rev. Econ. Dynam. 5, 45–72. doi: 10.1006/redy.2001.0141
Appleby, P. G., Nolan, P. J., Gifford, D. W., Godfrey, M. J., Oldfield, F., Anderson, N. J., et al. (1986). 210Pb dating by low background gamma counting. Hydrobiologia 141, 21–27.
Appleby, P. G., and Oldfield, F. (1978). The calculation of lead-210 dates assuming a constant rate of supply of unsupported 210Pb to the sediment. Catena 5, 1–8. doi: 10.1016/S0341-8162(78)80002-2
Appleby, P. G., Richardson, N., and Nolan, P. J. (1992). Self-absorption corrections for well-type germaniun detectors. Nucl. Inst. Methods B. 71, 228–233. doi: 10.1016/0168-583X(92)95328-O
Baxter, M. S., Farmer, J. G., McKinley, I. G., Swan, D. S., and Jack, W. (1981). Evidence of the unsuitability of gravity coring for collecting sediment in pollution and sedimentation rate studies. Environ. Sci. Technol. 15, 843–846. doi: 10.1021/es00089a014
Bédard, I. (2000). Techniques de Réhabilitation des Zones D'épanchement du Parc à Résidus Miniers Aldermac. Masters thesis, Département des Génies Civil, Géologique et des Mines, École Polytechnique de Montréal, September 2000.
Camburn, K. E., and Charles, D. R. (2000). Diatoms of Low-alkalinity Lakes in the Northeastern United States. Special Publication 18. Acad. Nat. Sci. Philadelphia, PA:Scientific Publications.
Cattaneo, A. Y., Couillard, Y., Wunsam, S., and Courcelles, M. (2004). Diatom taxonomic and morphological changes as indicators of metal pollution and recovery in Lac Dufault (Québec, Canada). J. Paleolim. 32, 163–175. doi: 10.1023/B:JOPL.0000029430.78278.a5
Cattaneo, A. Y., Couillard, Y., and Wunsam, S. (2008). Sedimentary diatoms along a temporal and spatial gradient of metal contamination. J. Paleolim. 40, 115–127. doi: 10.1007/s10933-007-9159-1
Chandra, A. P., and Gerson, A. R. (2010). The mechanisms of pyrite oxidation and leaching: a fundamental perspective. Surf. Sci. Rep. 65, 293–315. doi: 10.1016/j.surfrep.2010.08.003
Czarnecki, D. B., and Cawley, E. T. (1997). A new species of Pinnularia (Pinnulariaceae) from Iron rich, highly acidic strip-mined coal pits of South-Central Iowa. Proc. Acad. Natl. Sci. Philadelphia 147, 111–117.
Dixit, A. S., Alpay, S., Dixit, S. S., and Smol, J. P. (2007). Paleolimnological reconstructions of Rouyn-Noranda lakes within the zone of influence of the Horne Smelter, Québec Canada. J. Paleolim. 38, 209–226. doi: 10.1007/s10933-006-9072-z
Enache, M., and Prairie, Y. T. (2002). WA-PLS diatom-based pH, TP and DOC influence models from 42 lakes in the Abitibi clay belt area (Québec Canada). J. Paleolim. 27, 151–171. doi: 10.1023/A:1014281128544
Falasco, E., Bona, F., Badino, G., Hoffmann, L., and Ector, L. (2009). Diatom teratological forms and environmental alterations: a review. Hydrobiologia 623, 1–35. doi: 10.1007/s10750-008-9687-3
Fallu, M.-A., Allaire, N., and Pienitz, R. (2000). “Freshwater diatoms from northern Quebec and Labrador (Canada). Species-environment relationships in lakes of boreal forest, forest-tundra and tundra regions,” in Bibliotheca Diatomologica, Vol. 45, ed J. Cramer (Stuttgart; Berlin).
Gaiser, E. E., and Johansen, J. (2000). Freshwater diatoms from Carolina bays and other isolated wetlands on the Atlantic coastal plain of South Carolina, U.S.A. with descriptions of seven taxa new to science. Diatom Res. 15, 75–130. doi: 10.1080/0269249X.2000.9705487
Gajewski, K., Hamilton, P. B., and McNeely, R. (1997). A high-resolution proxy climate record from an arctic lake with annually-laminated sediments on Devon Island. J. Paleolim. 17, 215–225. doi: 10.1023/A:1007984617675
GEreports. (2015). Miner Key in Canada. General Electric Online Magazine. (July 23, 2014). Available online at: https://gereports.ca/slideshow/miner-key-in-canada/
Glew, J. R., Smol, J. P., and Last, W. M. (2001). “Sediment core collection and extrusion,” in Tracking Environmental Change using Lake Sediments. Vol. 1: Basin Analysis, Coring, and Chronological Techniques, eds W. M. Last and J. P. Smol (Dordrecht: Kluwer Academic Publishers), 73–105.
Hoekstra, A. Y., Chapagain, A. K., Aldaya, M. M., and Mekonnen, M. M. (2011). The Water Footprint Assessment Manual, Setting the Global Standard. London; Cornwall: Earthscan; TJ International Ltd.
Institut de la Statistique du Québec. (2014). L'Investissement Minier au Québec en 2013., Mines en Chiffres. Available online at: http://www.stat.gouv.qc.ca/statistiques/mines/mines-chiffres-2014.pdf (Accessed 22 March).
Juggins, S. (2014). C2 Version 1.7.6: Software for Ecological and Palaeoecological Data Analysis and Visualisation. Newcastle University.
Krammer, K. (2000). “Diatoms of the European inland waters and comparable habitats, Vol. 1, The genus Pinnularia,” in Diatoms of Europe. A. R. G. Gantner Verlag K. G. Ruggell.
Krammer, K., and Lange-Bertalot, H. (1985). “Naviculaceae, Neue und wenig bekannte Taxa, neue Kombinationen und Synonyme sowie Bemerkungen zu einigen Gattungen,” in Bibliotheca Diatomologica, Vol. 9, ed J. Cramer (Stuttgart; Berlin: Lubrecht & Cramer Ltd).
Krammer, K., and Lange-Bertalot, H. (1986). “Bacillariophyceae. 1. Teil: Naviculaceae,” in Süßwasserflora von Mittleuropa, Vol. 2/1, eds H. Ettl, J. Gerloff, H. Heyinig, and D. Mollenhauer (Stuttgart; New York, NY: Gustav Fischer Verlag).
Krammer, K., and Lange-Bertalot, H. (1988). “Bacillariophyceae. 2. Teil: Bacillariaceae, Epithemiaceae, Surirellaceae,” in Süßwasserflora von Mittleuropa, Vol. 2/2, eds H. Ettl, J. Gerloff, H. Heyinig, and D. Mollenhauer (Stuttgart; New York, NY: Gustav Fischer Verlag).
Krammer, K., and Lange-Bertalot, H. (1991a). “Bacillariophyceae. 3. Teil: Centrales, Fragilariaceae, Eunotiaceae,” in Süßwasserflora von Mittleuropa, Vol. 2/3, eds H. Ettl, J. Gerloff, H. Heyinig, and D. Mollenhauer (Stuttgart; Jena: Gustav Fischer Verlag).
Krammer, K., and Lange-Bertalot, H. (1991b). “Bacillariophyceae. 4. Teil: Achnanthaceae Kritische Ergänzungen zu Navicula (Lineolatae) und Gomphonema,” in Süßwasserflora von Mittleuropa, Vol. 2/4, eds H. Ettl, G. Gärtner, J. Gerloff, H. Heynig, and D. Mollenhauer (Stuttgart; New York, NY: Gustav Fischer Verlag).
Laperrière, L., Fallu, M.-A., Hausmann, S., Pienitz, R., and Muir, D. (2008). Paleolimnological evidence of mining and demographic impacts on Lac Dauriat, Schefferville (subarctic Québec, Canada). J. Paleolim. 40, 309–324. doi: 10.1007/s10933-007-9162-6
Leguay, S., Lavoie, I., Levy, J., and Fortin, C. (in press). Protective effects of hardness pH on metal accumulation by stream biofilms. Environ. Toxicol. Chem.
Marshall, B. (2014). The Mining Association of Canada, Facts and Figures of the Canadian Mining Industry 2014, 110. Available online at: http://mining.ca
Mayer, B., Alpay, S., Gould, W. D., Lortie, L., and Rosa, F. (2007). The onset of anthropogenic activity recorded in lake sediments in the vicinity of the Horne smelter in Quebec, Canada: sulfur isotope evidence. Appl. Geochem. 22, 397–414. doi: 10.1016/j.apgeochem.2006.10.001
Ministère de l'Énergie et des Ressources Naturelles (MERN). (2015). Available online at: https://www.mern.gouv.qc.ca/mines/quebec-mines/2008-11/restauration.asp (Accessed March 24, 2015).
Ministère du Développement Durable de l'Environnement et de la Lutte Contre les Changements Climatiques (MDDELCC). (2012). Directive 019 Sur L'industrie Minière. Gouvernement du Québec 105. Available online at: http://www.mddelcc.gouv.qc.ca/milieu_ind/directive019/directive019.pdf (Accessed March, 27).
Ministère du Développement Durable de l'Environnement et de la Lutte Contre les Changements Climatiques (MDDELCC). (2015). Citizen Letters of Complaint Regarding the Aldermac Site 1940-1980 Addressed to Various Branches of the Quebec Government. 21; 9 Letters. Archived unpublished documents held by la Direction Régionale de l'Abitibi-Témiscamingue et du Nord du Québec; obtained through an access to information request to MDDELCC made 26 November 2014; received 30 January 2015. Original authors and classified information were undisclosed for privacy protection.
Ministère des Ressources Naturelles et Faune (MRNF). (2011). Restauration des Sites Miniers. Septembre 2011. Available online at: http://maxxam.ca/wp-content/uploads/2011/10/MRNF_Johanne_CYR_22-septembre2011-MAXXAM.pdf (Accessed March 24, 2015).
Moingt, M., Lucotte, M., Paquet, S., and Ghaleb, B. (2014). Deciphering the impact of land-uses on terrestrial organic matter and mercury inputs to large boreal lakes of central Québec using lignin biomarkers. Appl. Geochem. 41, 34–48. doi: 10.1016/j.apgeochem.2013.11.008
Morin, S., Cordonier, A., Lavoie, I., Arini, A., Blanco, S., Duong, T. T., et al. (2012). “Consistency in diatom response to metal-contaminated environments,” in Emerging and Priority Pollutants in Rivers, eds H. Guasch, A. Ginebreda, and A. Geiszinger (Heidelberg: Springer), 117–146.
Mudroch, A., and MacKnight, S. D. (1991). “Bottom sediment sampling,” in CRC Handbook of Techniques for Aquatic Sediments Sampling, eds A. Mudroch and S. D. MacKnight (Boca Raton, FL: CRC Press), 29–95.
Olsen, M. P., Voldner, E. C., and Oikawa, K. K. (1982). A computerized sulphur budget for the eastern Canadian provinces. Water Air Soil Pollut. 18, 139–155. doi: 10.1007/BF02419409
Organisme de Bassin Versant Abitibi-Jamésie (OBVAJ). (2015). Plan Directeur de L'eau: Diagnostique du Bassin Versant de La Rivière Abitibi. Available online at: http://obvaj.org/evenement/plan-directeur-leau (Accessed 28 March 2015).
Schodde, R. (2010). 100 Years of Resource Growth for Copper—Impact of Costs, Grade and Technology. Available online at: http://www.slideshare.net/RichardSchodde/growth-factors-for-copper-schodde-sme-mems-march-2010-final (Accessed March 16, 2014).
Siver, P. A., Ahrens, T. D., Hamilton, P. B., Stachura-Suchoples, K., and Kociolek, J. P. (2004). “The ecology of diatoms in ponds and lakes on the cape cod peninsula, Massachusetts, U.S.A., with special reference to pH,” in The 17th International Diatom Symposium, ed M. Poulin (Bristol: Biopress Limited), 335–357.
Siver, P. A., Hamilton, P. B., Stachura-Suchoples, K., and Kociolek, J. P. (2005). “Diatoms of North America: the freshwater flora of Cape Cod, Massachusetts, U.S.A,” in Iconographia Diatomologia Vol. 14 (Königstein: A.R.G. Gantner Verlag).
Stoermer, E. F., and Smol, J. P. (1999). The Diatoms: Application for the Environmental and Earth Sciences. Cambridge: Cambridge University Press.
United States Department of Labor. (2012). Available online at: http://www.bls.gov/data/inflation_calculator.htm (Accessed March 25, 2015).
Vallières, M. (2012). Des Mines et des Hommes. Histoire de l'industrie Minérale Québécoise. Des Origines à Aujourd'hui. Québec: Ministère des Ressources naturelles.
Veillette, J. J. (1994). Evolution and paleohydrology of glacial Lakes Barlow and Ojibway. Quat. Sci. Rev. 13, 945–971. doi: 10.1016/0277-3791(94)90010-8
Verb, R. G., and Vis, M. L. (2000). Comparison of benthic diatom assemblages from streams draining abandoned and reclaimed coal mines and nonimpacted sites. J. N. Am. Benthol. Soc. 19, 274–288. doi: 10.2307/1468070
Vittet, C. (2011). Valeurs Écologique et Économique d'un Ancien site Minier Restauré. Masters thesis. Université de Sherbrooke, Québec, Canada.
Keywords: acid mine drainage, Aldermac mine, biomonitoring, diatoms, Lake Arnoux–Dasserat system, paleolimnology, pH, sulfur
Citation: Hamilton PB, Lavoie I, Alpay S and Ponader K (2015) Using diatom assemblages and sulfur in sediments to uncover the effects of historical mining on Lake Arnoux (Quebec, Canada): a retrospective of economic benefits vs. environmental debt. Front. Ecol. Evol. 3:99. doi: 10.3389/fevo.2015.00099
Received: 29 April 2015; Accepted: 07 August 2015;
Published: 01 September 2015.
Edited by:
Isabelle Larocque-Tobler, The L.A.K.E.S. Institute, SwitzerlandReviewed by:
Teresa Vegas-Vilarrúbia, University of Barcelona, SpainDavid Francis Porinchu, The University of Georgia, USA
Chad Steven Lane, University of North Carolina Wilmington, USA
Copyright © 2015 Hamilton, Lavoie, Alpay and Ponader. This is an open-access article distributed under the terms of the Creative Commons Attribution License (CC BY). The use, distribution or reproduction in other forums is permitted, provided the original author(s) or licensor are credited and that the original publication in this journal is cited, in accordance with accepted academic practice. No use, distribution or reproduction is permitted which does not comply with these terms.
*Correspondence: Paul B. Hamilton, Phycology Section, Collections and Research, Canadian Museum of Nature, 240 McLeod St., Ottawa, ON K2P 2R1, Canada,cGhhbWlsdG9uQG11cy1uYXR1cmUuY2E=