- 1Laboratory of Experimental and Comparative Ethology, University Paris 13-Sorbonne Paris Cité, Villetaneuse, France
- 2Department of Life Sciences and Systems Biology, University of Turin, Turin, Italy
- 3UMR 7261 Centre National de la Recherche Scientifique, Institut de Recherche sur la Biologie de l'Insecte, Université François Rabelais, Tours, France
- 4Departments of Entomology and Chemistry, University of California, Riverside, Riverside, CA, USA
Social insects typically discriminate nestmates from non-nestmates using colony-specific blends of cuticular hydrocarbons, which may be considered as a chemical label. Within a species, the cuticular profile shows approximately the same qualitative set of compounds, although these differ quantitatively among colonies. Thus, the relative proportions of particular hydrocarbons may be higher in individuals of one colony compared to those of another (conspecific) colony. Social insects must perceive these differences in ratios in order to efficiently recognize non-nestmates. However, little is known about the underlying perceptual mechanisms. Here we investigated whether ants can discriminate between different doses of individual linear or methyl-branched hydrocarbons. We used the ant Camponotus aethiops as our study organism and differential conditioning of the maxilla-labium extension response as the experimental procedure, to test olfactory discrimination between two concentrations of the same compound (one rewarded and the other punished), using large (wide range, 1:100) and small differences (narrow range, 1:10) in hydrocarbon concentrations. Ants discriminated well between wide-range concentrations of the same compound, but showed asymmetric generalization between narrow-range concentrations. These results indicate that a certain differential in hydrocarbon concentration is essential for efficient discrimination.
Introduction
The ability to distinguish group members from strangers is a key feature of social life (Hamilton, 1987). In many species, chemicals are used as recognition cues/signals in various contexts, and constitute part of the body odor and/or are emitted by specific glands. Examples range from insects and other invertebrates (d'Ettorre and Moore, 2008; Aquiloni and Tricarico, 2015) to birds (Bonadonna and Sanz-Aguilar, 2012), reptiles (Mason and Parker, 2010; Heathcote et al., 2014) and mammals, including humans (reviewed in Wyatt, 2014). Social insects discriminate nestmates from non-nestmates on the basis of both qualitative and quantitative differences in their cuticular chemical profiles, which are primarily composed of long-chain hydrocarbons (Hefetz, 2007; Bagnères and Lorenzi, 2010; van Zweden and d'Ettorre, 2010; Esponda and Gordon, 2015). Cuticular profiles are complex mixtures of different hydrocarbon classes, including linear alkanes, methyl-branched alkanes, and alkenes, and may contain more than 100 compounds (Blomquist, 2010). Social parasites exploit the recognition code of their hosts by acquiring or mimicking the host-specific cuticular hydrocarbon profile (Bagneres et al., 1996; Lenoir et al., 2001; Lorenzi, 2006; Nash and Boomsma, 2008), further supporting the substantial body of evidence that recognition signals are encoded in the cuticular lipid profile, particularly in social wasps (Lorenzi et al., 1997; Dani et al., 2001) and ants (Martin and Drijfhout, 2009; d'Ettorre and Lenoir, 2010). However, it remains unclear how the information about social identity is encoded in these hydrocarbon mixtures, and how the information is detected and processed by the receiving individual.
Previous studies using binary mixtures of floral odors suggest that in some cases ants do not perceive all blend components within a mixture. The mixture would thus be perceptually reduced to a few key components, without the need to take into account the entire suite of components constituting the olfactory stimulus. Determination of specific odorants as key components would rely on the chemical characteristic of the odorant molecule, such as the functional group and the carbon chain length (Bos et al., 2012, 2013; Perez et al., 2015). In the case of hydrocarbons, the available data suggest that some classes of hydrocarbons are more informative than others for recognition of identity. In particular, methyl-branched alkanes and alkenes may provide more information than linear alkanes as recognition cues/signals (e.g., wasps, Dani et al., 2001; ants, Martin et al., 2008; Guerrieri et al., 2009), although this may not apply to all species that have been investigated. In the ant Formica japonica, both alkenes and linear alkanes are necessary for recognition (Akino et al., 2004) whereas in the Argentine ant Linepithema humile, experimental alteration of the relative ratio of the linear alkanes only elicited a behavioral effect when methyl-branched alkanes and alkenes were also present (Greene and Gordon, 2007). Therefore, at least in some cases, linear alkanes may play a role in recognition, perhaps more specifically for caste and social status (Wagner et al., 1998; Greene and Gordon, 2003; Hefetz, 2007; Liebig, 2010; van Oystaeyen et al., 2014), although linear and methyl-branched alkanes and also alkenes may act as fertility signals or queen pheromones (Holman et al., 2010; van Oystaeyen et al., 2014; Smith et al., 2015).
Individual carpenter ants Camponotus aethiops learned to associate both linear and methyl-branched alkanes with a sucrose reward (Bos et al., 2012), showing that they are able to detect and discriminate among these types of compounds. Conversely, in some cases, ants generalize and thus show perceptual similarity, i.e., they respond similarly to homologs. For example, Argentine ants distinguish between methyl-branched hydrocarbons of the same chain length but differing in the position of the methyl branch (e.g., 17-MeC37 and 15-MeC37), although these ants generalize between hydrocarbons having the same methyl branch position and differing in chain length by two carbon atoms (e.g., 17-MeC37 and 17-MeC35, van Wilgenburg et al., 2012). Generalization can be rationalized on the basis that hydrocarbon perception might follow an “inclusion criterion” (Guerrieri et al., 2009; Bos et al., 2012). That is, in conditioning experiments, if a novel compound presented during the memory tests (in absence of reinforcement) is similar in structure to that presented as the conditioned stimulus but has a shorter carbon chain, this compound may be perceived as similar to the conditioned stimulus. For linear alkanes, generalization is observed when the novel hydrocarbon (e.g., C20) is shorter than the conditioned one (e.g., C22, Bos et al., 2012), possibly because it can be “included” in the same receptor site as the conditioned compound, or coded in a similar way at higher levels of information processing (e.g., in the antennal lobes). This inclusion criterion would also explain why series of homologs, which are regularly found on the cuticle of ants (Martin and Drijfhout, 2009), might be perceived as similar and trigger comparable levels of aggression (e.g., in Argentine ants, van Wilgenburg et al., 2010). However, when the difference in chain length between the two stimuli is sufficiently large (for example n-C28/n-C20), C. aethiops ants do not generalize (Bos et al., 2012).
Many experiments aiming to identify the role of hydrocarbons as recognition signals have been performed by supplementing the insect chemical profile with synthetic hydrocarbons. Indeed, in the context of nestmate recognition, it appears that it is the presence, and not the absence of a particular hydrocarbon on the cuticle that triggers aggression. Thus, carpenter ants supplemented with an extra hydrocarbon via their food are treated aggressively by their nestmates, which lack this particular hydrocarbon on the cuticle. However, the opposite is not true: ants that differ from the discriminators only by the absence of a given hydrocarbon on the cuticle are not treated aggressively (Guerrieri et al., 2009). These results prompted the idea of a new model for nestmate recognition, the Undesirable-present (U-present) model (reviewed in van Zweden and d'Ettorre, 2010; see also Esponda and Gordon, 2015). The template-label matching model is the classical model for nestmate recognition (Lenoir et al., 1999; Starks, 2004). It is based on a stimulus identification/generalization task, consisting of the evaluation of a stimulus-similarity (i.e., how much an individual's own colony odor matches the opponent's odor). According to Sherman et al. (1997), matching is achieved by evaluating the presence of desirable cues (D-present) or the absence of undesirable cues (U-absent). In contrast, the U-present model is based on a more parsimonious assumption, and does not require a point-by-point matching: only extra, but not missing, components elicit aggression. The U-present model is also compatible with the hypothesis of a “pre-filter mechanism” for nestmate recognition (Ozaki and Hefetz, 2014) and with the “distributed nestmate recognition model” (Esponda and Gordon, 2015). The study by Guerrieri et al. (2009) only tested the effects of adding extra hydrocarbons that are not naturally present on the cuticle of the test species. This explains how recognition could be effective among different species, which typically have a number of different compounds on their cuticle. However, for the U-present model to work between colonies of the same species and thus explain discrimination between nestmates and conspecific non-nestmates (which usually show quantitative rather than qualitative differences in their cuticular profiles), ants should be able to detect differences in the relative concentrations of compounds, and/or between different signal intensities of the same compound. In this way, an individual ant could detect and perceive those compounds that are present in higher abundance on a stranger's cuticle compared to its own. The ant will thus treat each of these relatively high concentration compounds as an “undesirable stimulus.”
Social insects learn their colony odor, i.e., colony-specific combinations of hydrocarbons at specific relative concentrations, and exhibit behavioral outputs as a response to perception of these blends (e.g., Guerrieri and d'Ettorre, 2008). Ants, for instance, have been shown to exhibit conditioned responses to hydrocarbons and floral odors (e.g., Guerrieri et al., 2009; Bos et al., 2010, 2012; Perez et al., 2015). Conditioning paradigms are robust and reliable means for studying learning and memory in invertebrates (Giurfa and Sandoz, 2012) and differential conditioning of proboscis extension reflex (PER) in honeybee workers was already used to evaluate chemical cues potentially involved in kin recognition in this species (Getz et al., 1988). However, the ability of social insects to learn and discriminate among different concentrations of hydrocarbons within a blend has rarely been tested, although these insects discriminate quantitative differences in hydrocarbon blends overall (Dani et al., 2001; Lorenzi et al., 2004; Guerrieri et al., 2009). Here, we tested whether Camponotus aethiops ants can learn different concentrations of hydrocarbons that are typically present on the cuticle and discriminate between them.
The relatively recent development of an associative learning paradigm for harnessed ants, involving the conditioning of the maxilla-labium extension response (MaLER, Guerrieri and d'Ettorre, 2010), which is similar to the proboscis extension response (PER) in honey bees (for review see: Giurfa, 2007; Sandoz, 2011), allows olfactory conditioning of ants under fully controlled laboratory conditions. The binary response (MaLER: YES/NO) is simple and unambiguous and ants show excellent learning performances (Guerrieri and d'Ettorre, 2010; Guerrieri et al., 2011; Bos et al., 2013; Perez et al., 2013, 2015). Here, we used the MaLER paradigm to test whether ants were able to discriminate between different concentrations of the same hydrocarbon (linear and branched alkanes) in a differential conditioning paradigm. We used two different concentration ranges of each hydrocarbon: a “wide range” in which the low concentration was a 100 times smaller than the high concentration, and a “narrow range,” in which the low concentration was 10 times smaller, although the low concentration was the same (10 ng/ml) in the two different ranges. These concentrations are above the detection threshold, and within the range of concentration variation between ant colonies (van Zweden et al., 2009; Ichinose and Lenoir, 2010).
Materials and Methods
Study Organism
Eight queenright colonies of the ant Camponotus aethiops (Latr.) were collected near Toulouse, France, in 2012 and 2013 (Pompertuzat, latitude 43.5°N, longitude 1.5°E). Colonies (size 400–500 workers) were kept under laboratory conditions (24°C, 60% humidity, light-dark cycle = 12:12 h) in artificial nests consisting of two plastic boxes interconnected by a plastic hose. One of the boxes (25.5 × 18 × 9 cm) was covered with a lid and paved with plaster, serving as the nest area; the other box (27.5 × 28 × 8 cm) was exposed to light and served as a foraging arena, and its walls were coated with Fluon® to prevent ants from escaping. Colonies in standard conditions received a diet consisting of agar, whole egg, honey, vitamins, and minerals (Bhatkar and Whitcomb, 1970) and water ad libitum. Two weeks before starting the experiments, ants were deprived of carbohydrate, in order to increase their motivation for a sugar reward during conditioning, but still received water and protein (crickets).
Experimental Procedure
Handling
Medium-size worker ants were taken from the foraging arena, chosen because they were likely to be foragers and therefore motivated to find food. For each experimental session, we used 10 individuals from three different colonies. Ants were anesthetized on ice and then harnessed individually in a holder consisting of an inverted 0.2 ml Eppendorf® microcentrifuge tube, from which the tip was cut off to create a second smaller opening. The ant's head was passed through the apical hole of the tube and fixed with adhesive tape, in order to leave the mouthparts and the antennae free to move. The harnessed ants were left undisturbed for at least 3 h to recover from the anesthesia and habituate to the harness.
Olfactory Stimuli
Camponotus aethiops ants have a complex cuticular profile composed of at least 65 methyl-branched alkanes and 5 linear alkanes in colony-specific proportions (van Zweden et al., 2009).
We tested two linear and two branched alkanes. Three of the four hydrocarbons tested (octacosane, n-C28; 3-methylheptacosane, 3-MeC27; 5-methylheptacosane, 5-MeC27) are present on the cuticle of C. aethiops. In particular, 5-MeC27 has a high diagnostic power (Table 1 in van Zweden et al., 2009) and we choose 3-MeC27 because it is a branched alkane with the same chain length but with a different position for the methyl group. Moreover, these hydrocarbons (both branched and linear) are learned well in an appetitive context (Bos et al., 2012). Docosane (n-C22) was used to test a hydrocarbon with a relatively short chain length compared to octacosane (n-C28). Linear alkanes (purity 99%) were obtained from Sigma-Aldrich, France. Racemic 3-MeC27 (97% purity) was synthesized as previously described (van Oystaeyen et al., 2014). 5-MeC27 (98% purity) was synthesized as follows. A solution of sodium hexamethyldisilazide (2M in THF) was added dropwise to a slurry of docosanyltriphenylphosphonium bromide (3.51 g, 5.4 mmol) in dry THF at 0°C under Ar atmosphere until the solution just turned orange, then an additional 3.0 ml (6 mmol) were added. The resulting orange slurry was warmed to room temperature and stirred 1 h, then cooled to 0°C again, and a solution of 2-hexanone (0.45 g, 4.5 mmol) in 5 ml THF was added dropwise. The mixture was warmed to room temperature and stirred overnight, then quenched with water and extracted twice with hexane. The combined hexane extracts were washed with brine, dried over anhydrous Na2SO4, concentrated, and purified by flash chromatography on silica gel, eluting with hexane, yielding 1.35 g (77%) of 5-methyl-5-heptacosene. This was taken up in 15 ml hexane and stirred with 0.3 g of 5% Pd on carbon under a hydrogen atmosphere for 1.5 h, at which time GC analysis indicated that all the starting material had been consumed. The mixture was filtered through a plug of Celite, rinsing well with hexane, and the filtrate was concentrated and recrystallized from 30 ml acetone at 4°. Filtration of the cold mixture yielded 1.12 g (83%) of racemic 5-MeC27. EI mass spectrum (70 eV) (m/z, abundance): 394 (M+, trace), 379 (5), 365 (4), 337 (35), 336 (20), 309 (5), 308 (7), 85 (100), 84 (69), 71 (57), 57 (82), and 43 (69).
Conditioning Procedure
To investigate whether ants were able to discriminate between two different concentrations of the same compound, ants (n = 480) were subjected to olfactory differential conditioning with two different concentration ranges of each hydrocarbon. These concentration differentials were: a) “wide range,” in which the high concentration was 1 mg/ml of a given hydrocarbon diluted in pentane, and the low concentration was 100 times smaller (0.01 mg/ml); b) “narrow range,” in which the high concentration was 0.1 mg/ml and the low concentration was 10 times smaller (0.01 mg/ml). The concentrations used corresponded respectively to 30 ant equivalents (1 mg/ml); 3 ant equivalents (0.1 mg/ml) and 0.3 ant equivalents (0.01 mg/ml). These are well above ants' detection threshold (10−4 ant equivalents; Ichinose and Lenoir, 2010) and within the range of concentration variation between colonies (e.g., the average proportion of octacosane varied > 40 times among ants from different colonies; van Zweden et al., 2009).
To perform differential conditioning, one odor concentration, the conditioned stimulus (CS+), was paired with the appetitive unconditioned stimulus (US+), consisting of aqueous sucrose solution (50% w/w), while a different concentration of the same odor, the CS−, was punished with an aversive US− consisting of quinine (purity 90%, Sigma-Aldrich, France) solution (1% w/w in water). The concentration of quinine was derived from previous conditioning experiments (Guerrieri and d'Ettorre, 2010). For each concentration range tested, we conditioned two different groups of ants: for one group, a given CS concentration was associated with the US+ stimulus, and for the other group of ants the same CS concentration was associated with the US− stimulus. In this way we had a balanced design.
It is known that ants can detect long-chain hydrocarbons from a short distance away (Brandstaetter et al., 2008). The CS was presented by approaching (without touching) the ants' antennae with the tip of a Pasteur pipette coated with the CS dissolved in paraffin oil (Sigma-Aldrich, France), used as a carrier for odorants in several studies on bees (e.g., Pelz et al., 1997). Each hydrocarbon was first dissolved in pentane at the desired concentration (see above), then 50 μl of the solution were transferred into a 2 ml glass vial, the pentane was allowed to evaporate for about 1 min, and 100 μl of paraffin oil was added, to give solutions containing 0.5, 0.05, and 0.005 mg/ml respectively in paraffin oil. The tip of the Pasteur pipette was dipped in this solution for 30 s and then left resting for at least 1 min before use. The US was presented by touching the mouthparts with a toothpick with a drop of the solution (sucrose or quinine). Ants typically displayed MaLER to sucrose but not to quinine. Only individuals that spontaneously responded with MaLER to the sugar reward before conditioning (>99%) were used for the experiments (Guerrieri and d'Ettorre, 2010).
Differential conditioning consisted of 12 trials, during which the ant received the same number of stimulations with the US+ and the US−: 6 CS+ trials at high (or low) concentration and 6 CS− trials at low (or high) concentration. The trials followed a pseudo-random sequence but the conditioning procedure always started with a CS+ trial. To observe MaLER, ants were placed under a stereomicroscope. Each conditioning trial lasted 1 min: 25 s after placing the ant under the microscope, the CS was presented for 5 s. US presentation started 3 s after the onset of the CS, and lasted for 5 s, thus creating an overlap of 2 s between the CS and the US (Guerrieri and d'Ettorre, 2010; Guerrieri et al., 2011). Lastly, the ants remained 27 s in the set-up. An air extractor was placed behind the ant during the experiments to remove any possible residual odor stimulation. Ten individuals were tested in series during the same experimental session; therefore, the inter-trial interval was 10 min. Only those individuals that responded to US+ at least three times during the training phase were included in the statistical analyses (n = 466 from a total of 476 tested ants).
Learning was scored by observing the presence or absence of MaLER upon CS presentation, therefore resulting in a binary response variable (yes = 1/no = 0).
Retention Test
One hour after training, the ants' responses to the stimuli used as CS+ and CS− were assessed in a retention test. The retention test to the CS+/CS− lasted 1 min and followed the same procedure as the conditioning phase, but without USs delivery. Half of the individuals were tested first with the CS+ and then with the CS− and vice versa for the other half. After this retention test, the ant's motivation for the sucrose was tested again by presenting only the US+ to the mouthparts (Guerrieri and d'Ettorre, 2010). Only those individuals that responded with MaLER (97.9%) were used in statistical analyses.
Both the conditioning procedures and the retention tests were performed at 24°C, 60% humidity. We used a freshly prepared Pasteur pipette for each training protocol on 10 ants. We used another freshly prepared Pasteur pipette for each retention test performed 1 h later.
Statistical Analyses
Statistical analyses were performed with R-2.15.01 (Development Core Team). Generalized linear mixed models (GLMM, package lme4; Bates et al., 2011) with a binomial error structure (logit-link) were used to analyse the acquisition data. The test ant's response (0/1) was used as the response variable. Conditioning trials were used as the predictor variable (covariate) and the conditioned stimulus (CS+ and CS−) as a fixed factor. In order to allow repeated measurements and adjust for colony origin, individuals and colony identity were coded as random factors. Interaction between trials and conditioned stimulus was included in the model to detect differences in slopes along the successive trials for the two types of CS. Variations in the response to the CS+ or the CS− along trials (acquisition) were revealed with post-hoc tests by applying the same GLMM without the factor stimulus to the respectively reduced set of data (i.e., responses to the CS+ or to the CS−).
Differences in the level of response between the CS+ and the CS− at the last conditioning trial were assessed with post-hoc tests consisting of the same GLMM without the factor “trial” applied to the respectively reduced set of data (i.e., responses to the CS+ and CS− in the last trial). Differences in the ants' responses to the conditioned odor (CS+ and CS−) between the last conditioning trial and the retention test, and differences in responses to the CS+ and the CS− in the retention test were detected with a McNemar's Chi Square test.
To test whether the concentration used as CS+/CS− influenced the learning rate of ants during the course of the successive trials (i.e., low+/high− vs. high+/low−, with low and high indicating the stimulus concentration and + and − indicating whether this was rewarded or punished) we used a GLMM with a binomial error structure (logit-link). The ants' responses were used as the response variable and individuals and colony of origin as random factors for the same reasons as above. Trials were used as predictor variable (covariate), with the conditioning stimulus (CS+ and CS−) and the concentration of the CS as fixed factors. Interactions between trials, conditioning stimulus, and concentration of the CS+ were included in the model to detect differences in slopes during the trials for the two types of CS and for the two concentrations of the same compound. Non-significant interactions were removed from the models and the models were then recalculated.
Results
Wide Range
Generally, ants learned to discriminate well between two different concentrations of the same hydrocarbon under the wide range conditions (high: 0.5 mg/ml; low: 0.005 mg/ml).
Linear Alkanes
Successful differential conditioning using two concentrations of the same n-alkane (either C22 or C28) is indicated by a significant interaction between trial and CS (44.55 < χ2 < 53.35; p < 0.001 in all cases; Figures 1A,C). Moreover, in the last trial ants discriminated both CSs well, as shown by a high level of conditioned responses to the CS+ (approximately 85%) and a low level of conditioned responses to the CS− (approximately 16%; 15.55 < χ2 < 25.13; p < 0.001 in all cases; Figures 1A,C). Also, ants increased their response to the CS+ during the course of successive trials (49.49 < χ2 < 55.86; p < 0.001 in all cases), whereas there was either a non-significant effect of trial for the CS− (when low+/high−: χ2 = 2.03 and χ2 = 3.52; p > 0.05 in both cases) or a significant decrease in response to the CS− (when high+/low−: χ2 = 4.04 and χ2 = 4.25; p < 0.05 in both cases), indicating that ants learned to respond to the CS+ and not to respond to the CS− (Figures 1A,C). One hour after training, ants remembered the rewarded and the punished concentrations of the same linear alkane well. For both CSs, the level of responses remained stable in the retention test compared to that of the last conditioning trial (0 < χ2 < 2.25; p > 0.13). Moreover, ants responded significantly more to the CS+ (approximately 84%) than to the CS− (approximately 23%) in the retention test (11.53 < χ2 < 19.36; p < 0.001 in all cases; Figures 1B,D).
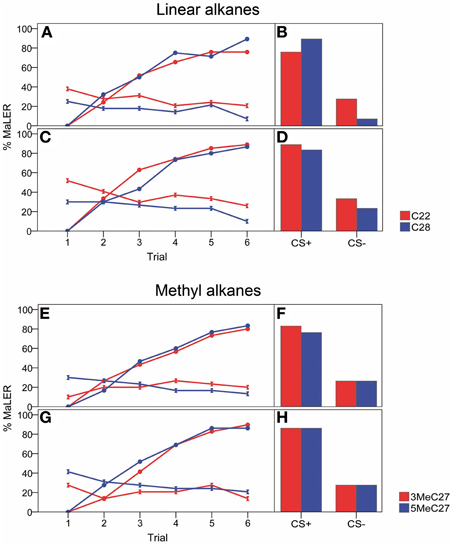
Figure 1. Wide range: acquisition curves and retention tests for linear (C22, C28) and methyl alkanes (3-MeC27 and 5-MeC27) showing the response probability to the wide concentration range expressed as the proportion of ants extending their maxilla-labium (MaLER). In the acquisition curves (A,C,E,G), responses to the conditioned stimulus are shown by circles (CS+) or double triangles (CS−). Retention tests show the proportion of ants responding to both CS+ and CS−, 1 h after training. (A,B,E,F) low concentration rewarded, high concentration punished (low+/high− vs. high+/low−). (C,D,G,H) high concentration rewarded, low concentration punished (high+/low− vs. low+/high).
The concentration of the CS (i.e., whether the concentration was high or low) did not influence the ants' responses to the conditioned stimuli. Indeed, the interaction between CS and CS−concentration was not significant, for both C22 and C28. The 3-way interaction: trial × CS × CS−concentration was also non-significant.
Methyl-branched Alkanes
The results of differential conditioning with methyl-branched alkanes over a wide range of concentrations (high: 0.5 mg/ml; low: 0.005 mg/ml) were very similar to those obtained with linear alkanes. Ants successfully learned to differentiate between two concentrations of the same branched alkane (either 3-MeC27 or 5-MeC27) during the successive conditioning trials, as shown by the significant interaction between trial and CS (20.95 < χ2 < 54.46; p < 0.001 in all cases; Figures 1E,G). In the last trial, ants showed high response levels to the CS+ (approximately 85%) and low response levels to the CS− (approximately 17%) as shown in Figures 1E,G (18.45 < χ2 < 24.08; p < 0.001 in all cases). Also, ants increased their responses to CS+ during the conditioning trials (49.83 < χ2 < 54.64; p < 0.001 in all cases). By contrast, the ants' responses to the CS− did not change (3-MeC27: χ2 = 0.23 and χ2 = 1.45; p > 0.05 in both cases) or decreased (5-MeC27: χ2 = 4.37 and χ2 = 4.41; p < 0.05 in both cases) during the successive trials. One hour after training, ants remembered the rewarded and the punished concentrations of the same methyl-branched alkane. In the retention test, the level of responses to both CSs remained stable compared to that obtained for the last conditioning trial (0 < χ2 < 2.25; p > 0.13) and ants responded more to the CS+ (approximately 83%) than to the CS− (approximately 27%), as shown in Figures 1F,H (10.32 < χ2 < 15.06; p < 0.01 in all cases).
Similar to n-alkanes, the concentration of the CS (high or low) did not influence the ants' responses to the conditioned stimuli in the case of 5-MeC27; indeed, there was a non-significant interaction CS × CS−concentration (the 3-way interaction was also non-significant). However, the 2-way interaction (CS × CS−concentration) tended toward significance in the case of 3-MeC27(χ2 = 3.23; p = 0.072), and the 3-way interaction (trial × CS × CS−concentration) was significant (χ2 = 4.35; p = 0.037).
Narrow Range
Overall, ants also learned to differentiate between two concentrations of the same hydrocarbon under the narrow range conditions (high: 0.05 mg/ml; low 0.005 mg/ml), but efficient discrimination depended on which concentration (high or low) was used as CS+ or CS−.
Linear Alkanes
For n-alkanes, ants learned to discriminate the CS+ from the CS− during the successive conditioning trials when the CS+ was the low concentration. However, when the CS+ was the high concentration, ants did not learn to discriminate between the two concentrations of the same hydrocarbon, but instead generalized. In all cases, the interaction between trial and CS was significant (9.26 < χ2 < 23.98; p < 0.01; Figures 2A,C). In the last trial, when low+/high−, ants showed high levels of conditioned responses to the CS+ (approximately 61%) and low levels of conditioned responses to the CS− (approximately 26%), as depicted in Figure 2A (χ2 = 5.42 and χ2 = 7.38; p < 0.05 in both cases). By contrast, when high+/low−, the levels of conditioning response to the CS+ and to the CS− were similar (approximately 65%), and ants did not discriminate between the two CSs (Figure 2C; χ2 = 0 and χ2 = 0.08; p > 0.05 in both cases).
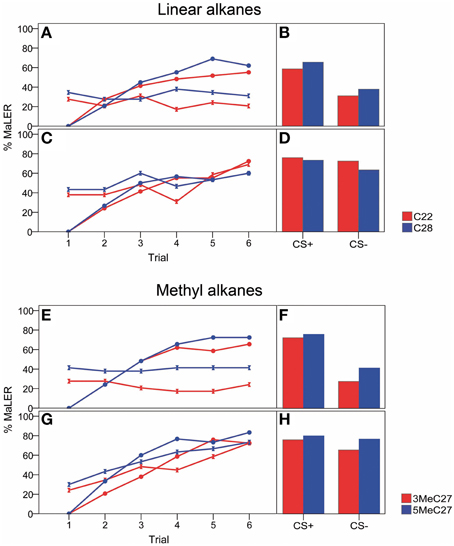
Figure 2. Narrow range: acquisition curves and retention tests for linear (C22, C28) and methyl alkanes (3-MeC27 and 5-MeC27) showing the response probability to the narrow concentration range expressed as the proportion of ants extending their maxilla-labium (MaLER). In the acquisition curves (A,C,E,G), responses to the CS are shown by circles (CS+) or double triangles (CS−). Retention tests show the proportion of ants responding to both CS+ and CS− 1 h after the training. (A,B,E,F) low concentration rewarded, high concentration punished (low+/high− vs. high+/low−); (C,D,G,H) high concentration rewarded, low concentration punished (high+/low− vs. low+/high−).
Post-hoc tests revealed that ants learned to respond to the CS+ during the successive trials (27.37 < χ2 < 37.46; p < 0.001 in all cases). In the low+/high− case, the level of response remained stable during the successive trials (χ2 = 0.06 and χ2 = 0.38; p > 0.05 in both cases). However, when the ants had to solve the high+/low− task, high levels of generalization were observable in the first trial (approximately 41%; Figure 2C). The high level of response to the CS− remained stable along the trials in the case of C28 (χ2 = 2.40; p > 0.05) but varied in the case of C22 (χ2 = 7.80; p < 0.01), although this level remained high at the last conditioning trial (around 65%). One hour after training, when the task was low+/high−, ants remembered the rewarded and the punished concentrations of the same linear alkane, as indicated by a high (approximately 62%) and a low (approximately 35%) level of conditioned responses in the retention test for the CS+ and the CS− respectively (χ2 = 4.08, p < 0.05 in both cases) (Figure 2B). However, in the case of high+/low−, ants did not discriminate the two concentrations of the same linear alkane in the retention test (χ2 = 0 and χ2 = 0.44; p > 0.05 in both cases, Figure 2D). The level of responses to both CSs remained stable in the retention test compared to that of the last conditioning trial (0 < χ2 < 0.1; p > 0.05 in all cases). Taken together, these results show that 1 h after conditioning, ants discriminated a narrow range of two concentrations of the same linear alkane only in the low+/high− case (Figures 2B,D). Indeed, the interaction between CS and concentration of CS was significant (χ2 = 7.66 and χ2 = 8.76; p < 0.01 in both cases).
Methyl-branched Alkanes
Differential conditioning in the narrow concentration range (0.05 vs. 0.005 mg/ml) using branched alkanes showed similar results as for linear alkanes. In all cases, the interaction between trial and CS was significant (χ2 = 7.74 and χ2 = 31.08; p < 0.01 in both cases; Figure 2E) and ants learned to respond to the CS+ along the successive trials (post-hoc tests: 39.46 < χ2 < 50.42; p < 0.001 in all cases). In particular, ants learned to differentiate between the CS+ and the CS− during the successive conditioning trials when low+/high−, as revealed by the difference in the level of response between the CS+ and the CS− in the last conditioning trial (CS+: approximately 69%; CS−: approximately 35%; χ2 = 5.48 and χ2 = 9.37; p < 0.05 in both cases). In the case of low+/high−, the level of response to the CS− was constant along successive trials (χ2 = 0.65 and χ2 = 0.04; p > 0.05 in both cases). However, this level of response was higher in the case of 5-MeC27 (approximately 41%) than in the case of 3-MeC27 (approximately 24%). By contrast, when presented with high+/low−, ants did not learn to discriminate between the two concentrations and showed similar levels of response in the last conditioning trial (CS+: approximately 78%; CS−: approximately 73%; χ2 = 0, 0.87; p > 0.05 in both cases). For the task high+/low−, ants increased their responses to the CS− during the training, as shown by the significant effect of the CS− along the successive trials (χ2 = 15.97 and χ2 = 17.42; p < 0.001 in both cases). One hour after training, when using low+/high−, ants remembered the rewarded and the punished concentrations of the hydrocarbon, as indicated by a high (approximately 69%) and a low (approximately 34%) level of conditioned responses in the retention test respectively for the CS+ and the CS− (χ2 = 9.6 and χ2 = 6.75; p < 0.01 in both cases; Figure 2F). However, if the task was high+/low−, ants did not discriminate (CS+: approximately 78%; CS−: approximately 71%; χ2 = 0, 0.36; p > 0.05 in both cases; Figure 2G). The level of responses to both CSs remained stable in the retention test compared to that of the last conditioning trial (0 < χ2 < 0.25; p > 0.05 all cases), and there was a high level of generalization between CSs in retention tests (χ2 = 0, 0.36; p > 0.05 in both cases, Figure 2H). The concentration used as CS (high or low) significantly influenced the ants' responses to the CSs in the case of 3-MeC27 (interaction CS × concentration of CS: χ2 = 11.50; p < 0.001), but this interaction was not significant in the case of 5-MeC27. The 3-way interaction (trial × CS × concentration of CS) was not significant in both cases. Taken together, these results indicate that ants were able to discriminate two concentrations of the same branched alkane in the narrow range only when the task was low+/high−.
Discussion
Our results demonstrate that ants can learn and discriminate between different concentrations of long-chain hydrocarbons, the chemical compounds that constitute the majority of their cuticular lipids, and confirm that ants can perceive hydrocarbons through olfaction, i.e., without any contact between the stimulus and the antenna. These findings should help to elucidate the mechanisms at the basis of nestmate recognition in ants and other social insects based on the following arguments.
During the last couple of decades there has been considerable progress in understanding both the production of cuticular components, and the behavioral responses that they mediate in the context of nestmate recognition mechanisms in social insects (Starks, 2004; Blomquist and Bagnères, 2010). However, the third key component—the perception component—remains relatively unexplored. The nestmate recognition template could be at the periphery, where odorant molecules are detected by specific antennal sensilla (Ozaki et al., 2005). Here, odorant binding proteins can selectively bind specific odorants and transport them to the olfactory receptors. The receptors then transmit signals to the antennal lobes (AL), the first integration relay of the central nervous system (Hildebrand and Shepherd, 1997). Thus, the template-label matching could also occur at the level of the ALs (Leonhardt et al., 2007; Guerrieri et al., 2009; Stroeymeyt et al., 2010; Bos and d'Ettorre, 2012; Ozaki and Hefetz, 2014), where signals from olfactory receptors elicit specific patterns of glomerular activity (Galizia and Szyszka, 2008). As a final alternative, the template could be stored in higher brain centers (e.g., the mushroom bodies), using long-term memory to match the new recognition signal with the colony template. To our knowledge, it remains unclear as to which one or which set of these alternatives may be correct.
Specifically, it remains unclear how the information about colony identity is encoded in the cuticular hydrocarbon profile (Martin and Drijfhout, 2009; van Zweden and d'Ettorre, 2010), and how this information is perceived and processed by the insect nervous system (Bos and d'Ettorre, 2012; d'Ettorre, 2013; Ozaki and Hefetz, 2014). Particularly within a species, differences in the cuticular chemical profile are usually quantitative rather than qualitative, with non-nestmate conspecifics having the same compounds present in their profiles, but in different relative proportions (review in d'Ettorre and Lenoir, 2010). It has been hypothesized that social insects form a neural template based on the relative proportions of hydrocarbons characterizing their colony odor, and use this template in the nestmate recognition process. The relative proportions of hydrocarbons detected reflect the different concentrations of the hydrocarbons in the overall cuticular chemical profile. The ability of social insects to form a recognition template based on the relative concentrations of various hydrocarbons, and to discriminate between different proportions, was indirectly tested in several studies (reviewed in van Zweden and d'Ettorre, 2010). For instance, when nests, nest wax, or nest soil were swapped between conspecific colonies, the insects typically modified their colony label/template following the colony odor swap and became generally more tolerant toward individuals from the alien colony (i.e., the donor of colony odor) (d'Ettorre et al., 2006; Couvillon et al., 2007; Lorenzi et al., 2011; Costanzi et al., 2013). This suggests that their template included information about hydrocarbon proportions. In C. aethiops ants, nest soil indirectly transfers hydrocarbons between ants, with consequences on recognition behavior. In particular, non-nestmates exposed to soil from a conspecific colony received significantly less aggression from ants belonging to the colony supplying the soil (i.e., nest soil contributes to the colony odor label; Bos et al., 2011) than did sham-treated non-nestmates. The present study helps clarifying to what extent ants detect and perceive information about cuticular hydrocarbon proportions.
Notwithstanding the fact that ants generally learned well in our conditioning experiments, we observed significant differences in ants' discriminating abilities depending on whether the concentration difference between the two stimuli (CS+ and CS−) was wide or narrow. It is worth noting that the lowest hydrocarbon concentration was the same in both concentration ranges (0.005 mg/ml); therefore the observed results cannot be a consequence of the insects' detection thresholds. In the case of the wide concentration range, both for linear and branched alkanes, differential conditioning was fully achieved, and the results were independent of whether the rewarded (or respectively, the punished) concentration was low or high. This shows that ants treat two concentrations of one odorant as qualitatively different stimuli when the concentration differential is sufficiently high. By contrast, when conditioning involved the narrow concentration range, successful discrimination between the two stimuli was significantly influenced by the interaction between CS and concentration of the CS, i.e., whether the rewarded (or respectively the punished) concentration was the high or low one. Specifically, when the tested concentration range was narrow, the ants discriminated between different concentrations only when the CS+ was the low concentration. When the CS+ was the high concentration, the ants did not discriminate well (i.e., they generalized between the two stimuli). Interestingly, honeybees appear not to be able to discriminate between different concentrations of one component of floral odors (the monoterpenoid linalool; Pelz et al., 1997). The bees could not solve the task “low+/high−” but they were successful in discriminating high+/low−. Irrespective of which was the rewarded stimulus, the bees' response levels to the high odorant concentration were always higher than the response levels to the lower concentrations, indicating that honeybees process different concentrations as differentially salient variants of one odorant (Pelz et al., 1997). The results of the present study show exactly the opposite trend for the narrow concentration range: the ants solved the task low+/high− but failed to discriminate between the two concentrations when the task was high+/low−. This discrepancy between odor discriminating ability in ants and bees is likely due to the nature of the odorants used in the experiments and the context in which these odors are encountered in nature. Hydrocarbons are constantly present on the body of ants and act as recognition cues/signals, whereas floral odors indicate the presence of flowers and are relevant when the bees are foraging. Honeybees should follow a floral odor concentration gradient to locate pollen and nectar, whereas ants (and other social insects) may rely on “U-present” as a simple rule to evaluate both qualitative and quantitative differences in cuticular hydrocarbon profiles.
Under the “U-present” hypothesis, non-nestmates may be readily detected because they bear “undesirable” cues, either qualitatively (additional compounds, as in case of heterospecific encounters) or quantitatively (different amounts of compounds present on the evaluator's profile, as in case of homospecific encounters). In this view, the “U-present” model would help to explain why “chemical insignificance,” i.e., the absence (or the very low amount) of recognition cues on the cuticle (Lenoir et al., 2001), might help social parasites to infiltrate host colonies (d'Ettorre and Errard, 1998; Johnson et al., 2001; Lorenzi and Bagnères, 2002; Lorenzi et al., 2004; Lambardi et al., 2007; Uboni et al., 2012), as well as explaining why the experimental removal (by solvent washing) of cuticular hydrocarbons significantly decreases aggression of non-nestmates (e.g., Lorenzi et al., 1997 for social wasps; Morel et al., 1988 for ants). Cini et al. (2009) have documented that the concentration of recognition cues (relative proportions of hydrocarbons being equal) has a role in eliciting aggressive behavior by resident social wasps against non-nestmates. They found that the higher was the concentration, the longer was the time spent by resident wasps attacking non-nestmates. Similar results were obtained with honeybees (Cappa et al., 2014). However, the hypothesis that higher concentrations of hydrocarbons are perceived as undesirable cues (whereas lower concentrations are not) should be further tested experimentally as alternative hypotheses exist (e.g., the template-label point-by-point matching).
The undesirable stimulus “U” may be represented by a hydrocarbon that is present on the cuticle of the opponent but absent on their own cuticle (qualitative difference, Guerrieri et al., 2009; van Zweden and d'Ettorre, 2010; Bos and d'Ettorre, 2012; Ozaki and Hefetz, 2014), or by a hydrocarbon present on the cuticle of the opponent in higher relative proportions than on their own cuticle (quantitative difference). A differential response to stimuli differing in concentration is a basic and widespread ability in organisms such as those exhibiting positive or negative chemotaxis (asymmetric movement in response to chemicals; e.g., in bacteria and nematodes, Wadhams and Armitage, 2004; Rassmann et al., 2012). However, social insects exhibit more complex outputs in response to different concentrations of hydrocarbons, manifested as asymmetric expression of aggressive behavior (they attack non-nestmates and tolerate nestmates) triggered by a U-present stimulus. We hypothesize that when concentration differences are narrow, ants might be unable to produce asymmetric expression of aggressive behavior in response to U-lower (or U-absent) stimuli, because social insects usually show low aggression (or no aggression at all) when exposed to nestmates with reduced amounts of recognition cues (see Section Introduction, and also Cini et al., 2009). This hypothesis would explain why we observed that ants were less successful when the task was high+/low− (the asymmetrical response) than when the task was low+/high−. We interpret this as an ability to discriminate between different concentrations. In natural conditions, when ants habituate to a relatively “high concentration” of a given hydrocarbon as one of the properties of their colony odor (i.e., they learn a high+/low− task), they might tolerate non-nestmate ants bearing lower amounts of the given hydrocarbon, all else being equal. Conversely, when ants are used to a relatively “low concentration” of a given hydrocarbon as their colony odor (i.e., a low+/high− task), they might attack non-nestmate ants bearing amounts of the given hydrocarbon higher than their own, all else being equal. In our experiment, ants were more efficient in performing the task low+/high− (in natural conditions, when two individuals meet, this task involves two behavioral responses: attack or tolerance) than in performing the task high+/low− (involving only one behavioral response: tolerance). Although, this interpretation supports the U-present model applied to quantitative differences in recognition cues, it is still a suggestion and further empirical investigations testing specifically the nestmate recognition mechanism are needed to confirm this hypothesis.
Finally, our results corroborate previous studies showing that ants learn efficiently when subjected to differential conditioning of the MaLER (Guerrieri and d'Ettorre, 2010; Perez et al., 2013), thus allowing a fine-tuned investigation of their discrimination ability between two odor stimuli, one appetitive (sucrose) and the other aversive (quinine, for a discussion about the aversive valence of quinine for ants, see Guerrieri and d'Ettorre, 2010). We also confirmed that C. aethiops ants retained the associations between CSs and USs for at least 1 h (Guerrieri and d'Ettorre, 2010). Indeed, the retention test performed 1 h after conditioning revealed that the ants, in all cases (with one exception, see below), showed a higher response to the hydrocarbon concentration previously associated with sucrose than to the hydrocarbon concentration associated with quinine. Further, studies are needed to clarify how long the ants are able to remember these learned associations, and whether long-term olfactory memories can be formed with hydrocarbon concentrations as stimuli, similar to what was found when using two different linear hydrocarbons as stimuli (i.e., CS+ and CS−, Guerrieri et al., 2011).
Taken together, the present results provide information about the resolution with which ants discriminate between two different concentrations of one hydrocarbon. Although there is mixed evidence about whether different classes of hydrocarbons, such as linear and branched alkanes, are equally relevant for nestmate recognition (see Section Introduction), we observed the same pattern of results for both hydrocarbon classes tested here (see also Bos et al., 2012). Moreover, we did not observe large differences in discriminating ability depending on chain length (C22 vs. C28) or position of the methyl-branch (3-MeC27 vs. 5-MeC27). That is, each test compound appeared to be perceived with similar efficacy, and to elicit analogous learning and behavioral responses. A previous study on a Camponotus species showed that the nature of the hydrocarbon (linear or methyl-branched) is indeed relevant for the recognition process, with branched alkanes affecting recognition behavior more than linear alkanes when experimentally added to the ant cuticular profile (Guerrieri et al., 2009). Therefore, that study documented that the molecular structure plays a role in nestmate recognition in at least some species. The present study clearly indicates that the stimulus represented by the hydrocarbon concentration is an important feature in hydrocarbon perception and learning. Ants treat two concentrations of one hydrocarbon as two qualitatively different stimuli, at least when the concentration differential is high (wide range), and to some extent when the differential is low (narrow range).
Nest-mate recognition in social insects needs to be strict enough to allow the rejection of individuals if they come from a different colony, while at the same time flexible enough to accept natural variations in the colony odor composition, thus avoiding rejection of nestmates (Reeve, 1989; Downs and Ratnieks, 2000; van Zweden and d'Ettorre, 2010). Individuals within a colony show variations in their chemical profile depending on their age, caste, functional role, social status, and diet (Hefetz, 2007; d'Ettorre and Lenoir, 2010; Liebig, 2010; Esponda and Gordon, 2015), requiring further plasticity in the overall perception and discrimination of the colony odor. The results of the present study show that ants discriminate large differences in hydrocarbon concentration more easily than small ones, suggesting that they might be more likely to reject individuals with more quantitatively dissimilar profiles than those with less dissimilar profiles compared to their own. Further studies are needed to test this hypothesis.
Author Contributions
PD and ML conceived the study; GD, FG and PD designed the study; JM provided the synthetic hydrocarbons; GD and MP carried out the experimental work; MP, GD, ML, and PD analyzed the data; GD, ML, JM, and PD wrote the manuscript and all authors read commented and approved the manuscript.
Conflict of Interest Statement
The authors declare that the research was conducted in the absence of any commercial or financial relationships that could be construed as a potential conflict of interest.
Acknowledgments
We would like to thank Chloé Leroy and Paul Devienne for technical support. This study was supported by the ERASMUS student exchange programme between University of Turin and University of Paris 13, and partially by a grant from the French National Research Agency (ANR-14-CE18-0003-03 PheroMod) to PD.
References
Akino, T., Yamamura, K., Wakamura, S., and Yamaoka, R. (2004). Direct behavioral evidence for hydrocarbons as nestmate recognition cues in Formica japonica (Hymenoptera: Formicidae). Appl. Entomol. Zool. 39, 381–387. doi: 10.1303/aez.2004.381
Aquiloni, L., and Tricarico, E. (2015). Social Recognition in Invertebrates. Springer International Publishing Switzerland. doi: 10.1007/978-3-319-17599-7
Bagnères, A.-G., and Lorenzi, M.C. (2010). “Chemical deception/mimicry using cuticular hydrocarbons,” in Insect Hydrocarbons: Biology, Biochemistry and Chemical Ecology, eds G. Blomquist and A.-G. Bagnères (Cambridge: Cambridge University Press), 282–324.
Bagneres, A. G., Lorenzi, M. C., Dusticier, G., Turillazzi, S., and Clement, J. L. (1996). Chemical usurpation of a nest by paper wasp parasites. Science 272, 889–892. doi: 10.1126/science.272.5263.889
Bates, D., Maechler, M., and Bolker, B. (2011). lme4: Linear Mixed-effects Models Using S4 Classes. R package version 0.999375–999339.
Bhatkar, A. P., and Whitcomb, W. H. (1970). “Artificial diet for rearing various species of ants,” in Insect Hydrocarbons: Biology, Biochemistry and Chemical Ecology, Florida Entomol, Vol. 53, eds G. Blomquist and A.-G. Bagnères (Cambridge: Cambridge University Press), 229–232.
Blomquist, G. J. (2010). “Structure and analysis of insect hydrocarbons,” in Insect Hydrocarbons: Biology, Biochemistry and Chemical Ecology, eds G. J. Blomquist and A.-G. Bagnères (Cambridge: Cambridge University Press), 19–34.
Blomquist, G. J., and Bagnères, A.-G. (2010). Insect Hydrocarbons: Biology, Biochemistry, and Chemical Ecology. Cambridge: Cambridge University Press.
Bonadonna, F., and Sanz-Aguilar, A. (2012). Kin recognition and inbreeding avoidance in wild birds: the first evidence for individual kin-related odour recognition. Anim. Behav. 84, 509–513. doi: 10.1016/j.anbehav.2012.06.014
Bos, N., Dreier, S., Jørgensen, C. G., Nielsen, J., Guerrieri, F. J., and d'Ettorre, P. (2012). Learning and perceptual similarity among cuticular hydrocarbons in ants. J. Insect. Physiol. 58, 138–146. doi: 10.1016/j.jinsphys.2011.10.010
Bos, N., Grinsted, L., and Holman, L. (2011). Wax on, wax off: Nest soil facilitates indirect transfer of recognition cues between ant nestmates. PLoS ONE 6:e19435. doi: 10.1371/journal.pone.0019435
Bos, N., Guerrieri, F. J., and d'Ettorre, P. (2010). Significance of chemical recognition cues is context dependent in ants. Anim. Behav. 80, 839–844. doi: 10.1016/j.anbehav.2010.08.001
Bos, N., and d'Ettorre, P. (2012). Recognition of social identity in ants. Front. Psychol. 3:83. doi: 10.3389/fpsyg.2012.00083
Bos, N., d'Ettorre, P., and Guerrieri, F. J. (2013). Chemical structure of odorants and perceptual similarity in ants. J. Exp. Biol. 216, 3314–3320. doi: 10.1242/jeb.087007
Brandstaetter, A. S., Endler, A., and Kleineidam, C. J. (2008). Nestmate recognition in ants is possible without tactile interaction. Naturwissenschaften 95, 601–608. doi: 10.1007/s00114-008-0360-5
Cappa, F., Bruschini, C., Cipollini, M., Pieraccini, G., and Cervo, R. (2014). Sensing the intruder: a quantitative threshold for recognition cues perception in honeybees. Naturwissenschaften 101, 149–152. doi: 10.1007/s00114-013-1135-1
Cini, A., Gioli, L., and Cervo, R. (2009). A quantitative threshold for nest-mate recognition in a paper social wasp. Biol. Lett. 5, 459–461. doi: 10.1098/rsbl.2009.0140
Costanzi, E., Bagnères, A.-G., and Lorenzi, M. C. (2013). Nestmate recognition in social wasp is based on the relative proportions of cuticular hydrocarbons within species-specific ranges of hydrocarbon concentrations. PLoS ONE 8:e65107. doi: 10.1371/journal.pone.0065107
Couvillon, M. J., Caple, J. P., Endsor, S. L., Kärcher, M., Russell, T. E., Storey, D. E., et al. (2007). Nest-mate recognition template of guard honeybees (Apis mellifera) is modified by wax comb transfer. Biol. Lett. 3, 228–230. doi: 10.1098/rsbl.2006.0612
Dani, F. R., Jones, G. R., Destri, S., Spencer, S. H., and Turillazzi, S. (2001). Deciphering the recognition signature within the cuticular chemical profile of paper wasps. Anim. Behav. 62, 165–171. doi: 10.1006/anbe.2001.1714
d'Ettorre, P. (2013). “Learning and recognition of identity in ants,” in Invertebrate Learning and Memory, eds R. Menzel and P. R. Benjamin (London: Elsevier), 501–513.
d'Ettorre, P., and Errard, C. (1998). Chemical disguise during colony founding in the dulotic ant Polyergus rufescens Latr. (Hymenoptera, Vespidae). Insect. Soc. Life 2, 71–77.
d'Ettorre, P., and Lenoir, A. (2010). “Nestmate recognition,” in Ant Ecology, eds L. Lach, C. Parr, and K. Abbott (Oxford: Oxford University Press), 194–209.
d'Ettorre, P., and Moore, A. J. (2008). “Chemical communication and the coordination of social interactions in insects,” in Sociobiology of Communication, eds P. d'Ettorre and D. P. Hughes (Oxford: Oxford University Press), 81–96.
d'Ettorre, P., Wenseleers, T., Dawson, J., Hutchinson, S., Boswell, T., and Ratnieks, F. L. W. (2006). Wax combs mediate nestmate recognition by guard honey bees. Anim. Behav. 71, 773–779. doi: 10.1016/j.anbehav.2005.05.014
Downs, S. G., and Ratnieks, F. L. W. (2000). Adaptive shifts in honey bee (Apis mellifera L.) guarding behavior support predictions of the acceptance threshold model. Behav. Ecol. 11, 326–333. doi: 10.1093/beheco/11.3.326
Esponda, F., and Gordon, D. M. (2015). Distributed nestmate recognition in ants. Proc. R. Soc. London B 282, 20142838. doi: 10.1098/rspb.2014.2838
Galizia, C. G., and Szyszka, P. (2008). Olfactory coding in the insect brain: molecular receptive ranges, spatial and temporal coding. Entomol. Exp. Appl. 128, 81–92. doi: 10.1111/j.1570-7458.2007.00661.x
Getz, W. M., Brückner, D., and Smith, K. B. (1988). Variability of chemosensory stimuli within honeybees (Apis mellifera) colonies: differential conditioning assay for discrimination cues. J. Chem. Ecol. 14, 253–264. doi: 10.1007/BF01022545
Giurfa, M. (2007). Behavioral and neural analysis of associative learning in the honeybee: a taste from the magic well. J. Comp. Physiol. A 193, 801–824. doi: 10.1007/s00359-007-0235-9
Giurfa, M., and Sandoz, J. C. (2012). Invertebrate learning and memory: fifty years of olfactory conditioning of the proboscis extension response in honeybees. Cold Spring Harb. Lab. Press 19, 54–66. doi: 10.1101/lm.024711.111
Greene, M. J., and Gordon, D. M. (2003). Cuticular hydrocarbons inform task decisions. Nature 423, 32. doi: 10.1038/423032a
Greene, M. J., and Gordon, D. M. (2007). Structural complexity of chemical recognition cues affects the perception of group membership in the ants Linephithema humile and Aphaenogaster cockerelli. J. Exp. Biol. 210, e905. doi: 10.1242/jeb.02706
Guerrieri, F. J., Nehring, V., Jørgensen, C. G., Nielsen, J., Galizia, C. G., and d'Ettorre, P. (2009). Ants recognize foes and not friends. Proc. R. Soc. London B 276, 2461–2468. doi: 10.1098/rspb.2008.1860
Guerrieri, F. J., and d'Ettorre, P. (2008). The mandible opening response: quantifying aggression elicited by chemical cues in ants. J. Exp. Biol. 211, 1109–1113. doi: 10.1242/jeb.008508
Guerrieri, F. J., and d'Ettorre, P. (2010). Associative learning in ants: conditioning of the maxilla-labium extension response in Camponotus aethiops. J. Insect Physiol. 56, 88–92. doi: 10.1016/j.jinsphys.2009.09.007
Guerrieri, F. J., d'Ettorre, P., Devaud, J. M., and Giurfa, M. (2011). Long-term olfactory memories are stabilised via protein synthesis in Camponotus fellah ants. J. Exp. Biol. 214, 3300–3304. doi: 10.1242/jeb.059170
Hamilton, W. D. (1987). “Discrimination nepotism: expectable, common, overlooked,” in Kin Recognition in Animals, eds D. J. C. Fletcher and C. D. Michener (New York, NY: Wiley), 417–437.
Heathcote, R. J. P., Bell, E., d'Ettorre, P., While, G. M., and Uller, T. (2014). The scent of sun worship: basking experience alters scent mark composition in male lizards. Behav. Ecol. Sociobiol. 68, 861–870. doi: 10.1007/s00265-014-1700-4
Hefetz, A. (2007). The evolution of hydrocarbon pheromone parsimony in ants (Hymenoptera: Formicidae)—interplay of colony odor uniformity and odor idiosyncrasy—a review. Myrmecol. News 10, 59–68.
Hildebrand, J. G., and Shepherd, G. M. (1997). Mechanisms of olfactory discrimination: converging evidence for common principles across phyla. Annu. Rev. Neurosci. 20, 595–613. doi: 10.1146/annurev.neuro.20.1.595
Holman, L., Jørgensen, C. G., Nielsen, J., and d'Ettorre, P. (2010). Identification of an ant queen pheromone regulating worker sterility. Proc. R. Soc. London B 277, 3793–3800. doi: 10.1098/rspb.2010.0984
Ichinose, K., and Lenoir, A. (2010). Hydrocarbons detection levels in ants. Insectes Soc. 57, 453–455. doi: 10.1007/s00040-010-0103-4
Johnson, C. A., Vander Meer, R. K., and Lavine, B. (2001). Changes in the cuticular hydrocarbon profile of the slave-maker ant queen, Polyergus breviceps Emery, after killing a Formica host queen (Hymenoptera: Formicidae). J. Chem. Ecol. 27, 1787–1804. doi: 10.1023/A:1010456608626
Lambardi, D., Dani, F. R., Turillazzi, S., and Boomsma, J. J. (2007). Chemical mimicry in an incipient leaf-cutting ant social parasite. Behav. Ecol. Sociobiol. 61, 843–851. doi: 10.1007/s00265-006-0313-y
Lenoir, A., Fresneau, D., Errard, C., and Hefetz, A. (1999). “Individuality and colonial identity in ants: the emergence of the social representation concept,” in Information Processing in Social Insects, eds C. Detrain, J.-L. Deneubourg, and J. M. Pasteels (Basel: Birkhäuser Verlag), 219–237.
Lenoir, A., d'Ettorre, P., Errard, C., and Hefetz, A. (2001). Chemical ecology and social parasitism in ants. Annu. Rev. Entomol. 46, 573–599. doi: 10.1146/annurev.ento.46.1.573
Leonhardt, S. D., Brandstaetter, A. S., and Kleineidam, C. J. (2007). Reformation process of neural template for nestmate-recognition cues in the carpenter ant Camponotus floridanus. J. Comp. Physiol. A 193, 993–1000. doi: 10.1007/s00359-007-0252-8
Liebig, J. (2010). “Hydrocarbon profiles indicate fertility and dominance status in ant, bee, and wasp colonies,” in Insect Hydrocarbons: Biology, Biochemistry and Chemical Ecology, eds G. Blomquist and A.-G. Bagnères (Cambridge: Cambridge University Press), 254–281.
Lorenzi, M. C. (2006). The result of an arms race: the chemical strategies of Polistes social parasites. Ann. Zool. Fenn. 43, 550–563.
Lorenzi, M. C., and Bagnères, A. G. (2002). Concealing identity and mimicking hosts: A dual chemical strategy for a single social parasite? (Polistes atrimandibularis, Hymenoptera: Vespidae). Parasitology 125, 507–512. doi: 10.1017/S003118200200238X
Lorenzi, M. C., Bagnères, A.-G., Clément, J.-L., and Turillazzi, S. (1997). Polistes biglumis bimaculatus epicuticular hydrocarbons and nestmate recognition (Hymenoptera: Vespidae). Insectes Soc. 44, 123–138. doi: 10.1007/s000400050035
Lorenzi, M. C., Cervo, R., and Bagnères, A.-G. (2011). Facultative social parasites mark host nests with branched hydrocarbons. Anim. Behav. 82, 1149–1157. doi: 10.1016/j.anbehav.2011.08.011
Lorenzi, M. C., Cervo, R., Zacchi, F., Turillazzi, S., and Bagnères, A. G. (2004). Dynamics of chemical mimicry in the social parasite wasp Polistes semenowi (Hymenoptera: Vespidae). Parasitology 129, 643–651. doi: 10.1017/S0031182004005992
Martin, S. J., and Drijfhout, F. P. (2009). Nestmate and task cues are influenced and encoded differently within ant cuticular hydrocarbon profiles. J. Chem. Ecol. 35, 368–374. doi: 10.1007/s10886-009-9612-x
Martin, S. J., Vitikainen, E., Helanterä, H., and Drijfhout, F. P. (2008). Chemical basis of nest-mate discrimination in the ant Formica exsecta. Proc. R. Soc. London B 275, 1271–1278. doi: 10.1098/rspb.2007.1708
Mason, R. T., and Parker, M. R. (2010). Social behavior and pheromonal communication in reptiles. J. Comp. Physiol. A 196, 729–749. doi: 10.1007/s00359-010-0551-3
Morel, L., Vander Meer, R. K., and Lavine, B. K. (1988). Ontogeny of nestmate recognition cues in the red carpenter ant (Camponotus floridanus): behavioural and chemical evidence for the role of age and social experience. Behav. Ecol. Sociobiol. 22, 175–183. doi: 10.1007/BF00300567
Nash, D. R., and Boomsma, J. J. (2008). “Communication between hosts and social parasites,” in Sociobiology of Communication, eds P. d'Ettorre and D. P. Hughes (Oxford: Oxford University Press), 55–79.
Ozaki, M., and Hefetz, A. (2014). Neural mechanisms and information processing in recognition systems. Insects 5, 722–741. doi: 10.3390/insects5040722
Ozaki, M., Wada-Katsumata, A., Fujikawa, K., Iwasaki, M., Yokohari, F., Satoji, Y., et al. (2005). Ant nestmate and non-nestmate discrimination by a chemosensory sensillum. Science 309, 311–314. doi: 10.1126/science.1105244
Pelz, C., Gerber, B., and Menzel, R. (1997). Odorant intensity as a determinant for olfactory conditioning in honeybees: roles in discrimination, overshadowing and memory consolidation. J. Exp. Biol. 200, 837–847.
Perez, M., Giurfa, M., and d'Ettorre, P. (2015). The scent of mixtures: rules of odour processing in ants. Sci. Rep. 5:8659. doi: 10.1038/srep08659
Perez, M., Rolland, U., Giurfa, M., and d'Ettorre, P. (2013). Sucrose responsiveness, learning success, and task specialization in ants. Learn. Mem. 20, 417–420. doi: 10.1101/lm.031427.113
Rassmann, S., Ali, J. G., Helder, J., and van der Putten, W. H. (2012). Ecology and evolution of soil nematode chemotaxis. J. Chem. Ecol. 38, 615–628. doi: 10.1007/s10886-012-0118-6
Reeve, H. K. (1989). The evolution of conspecific acceptance thresholds. Am. Nat. 133, 407–435. doi: 10.1086/284926
Sandoz, J.-C. (2011). Behavioral and neurophysiological study of olfactory perception and learning in the honeybee. Front. Syst. Neurosci. 5:98. doi: 10.3389/fnsys.2011.00098
Sherman, P. W., Reeve, H. K., and Pfennig, D. W. (1997). “Recognition systems,” in Behavioural Ecology: An Evolutionary Approach, eds J. R. Krebs and N. B. Davies (Oxford: Blackwell Science), 69–96.
Smith, A. A., Millar, J. G., and Suarez, A. V. (2015). A social insect fertility signal is dependent on chemical context. Biol. Lett. 11:20140947. doi: 10.1098/rsbl.2014.0947
Starks, P. T. (2004). Recognition systems: From components to conservation. Ann. Zool. Fenn. 41, 689–690.
Stroeymeyt, N., Guerrieri, F. J., van Zweden, J. S., and d'Ettorre, P. (2010). Rapid decision-making with side-specific perceptual discrimination in ants. PLoS ONE 5:e12377. doi: 10.1371/journal.pone.0012377
Uboni, A., Bagnères, A. G., Christidès, J. P., and Lorenzi, M. C. (2012). Cleptoparasites, social parasites and a common host: chemical insignificance for visiting host nests, chemical mimicry for living in. J. Insect Physiol. 58, 1259–1264. doi: 10.1016/j.jinsphys.2012.06.013
van Oystaeyen, A., Oliveira, R. C., Holman, L., van Zweden, J. S., Romero, C., Oi, C. A., et al. (2014). Conserved class of queen pheromones stops social insect workers from reproducing. Science 343, 287–290. doi: 10.1126/science.1244899
van Wilgenburg, E., Sulc, R., Shea, K. J., and Tsutsui, N. D. (2010). Deciphering the chemical basis of nestmate recognition. J. Chem. Ecol. 36, 751–758 doi: 10.1007/s10886-010-9812-4
van Wilgenburg, F. A., Felden, A., Choe, D.H., Sulc, R., Luo, J., Shea, K. J., Elgar, M. A., et al. (2012). Learning and discrimination of cuticular hydrocarbons in a social insect. Biol. Lett. 8, 17–20. doi: 10.1098/rsbl.2011.0643
van Zweden, J. S., Dreier, S., and d'Ettorre, P. (2009). Disentangling environmental and heritable nestmate recognition cues in a carpenter ant. J. Insect Physiol. 55, 158–163. doi: 10.1016/j.jinsphys.2008.11.001
van Zweden, J. S., and d'Ettorre, P. (2010). “The role of hydrocarbons in nestmate recognition,” in Insect Hydrocarbons: Biology, Biochemistry and Chemical Ecology, eds G. Blomquist and A.-G. Bagnères (Cambridge: Cambridge University Press), 222–243.
Wadhams, G. H., and Armitage, J. P. (2004). Making sense of it all: bacterial chemotaxis. Nat. Rev. Mol. Cell Biol. 5, 1024–1037. doi: 10.1038/nrm1524
Keywords: ants, Camponotus, learning, odor salience, recognition label/template
Citation: di Mauro G, Perez M, Lorenzi MC, Guerrieri FJ, Millar JG and d'Ettorre P (2015) Ants Discriminate Between Different Hydrocarbon Concentrations. Front. Ecol. Evol. 3:133. doi: 10.3389/fevo.2015.00133
Received: 21 May 2015; Accepted: 12 November 2015;
Published: 26 November 2015.
Edited by:
Abraham Hefetz, Tel Aviv University, IsraelReviewed by:
Robert Kenneth Vander Meer, United States Department of Agriculture, USAMamiko Ozaki, Kobe University, Japan
Copyright © 2015 di Mauro, Perez, Lorenzi, Guerrieri, Millar and d'Ettorre. This is an open-access article distributed under the terms of the Creative Commons Attribution License (CC BY). The use, distribution or reproduction in other forums is permitted, provided the original author(s) or licensor are credited and that the original publication in this journal is cited, in accordance with accepted academic practice. No use, distribution or reproduction is permitted which does not comply with these terms.
*Correspondence: Patrizia d'Ettorre, ZGV0dG9ycmVAbGVlYy51bml2LXBhcmlzMTMuZnI=