- 1Chemical Ecology, Federal Research Centre for Cultivated Plants, Institute for Plant Protection in Fruit Crops and Viticulture, Julius Kühn-Institut, Dossenheim, Germany
- 2Chemical Ecology, Institute of Evolutionary Ecology and Conservation Genomics, University of Ulm, Ulm, Germany
Apple proliferation (AP) and pear decline (PD) are the most severe diseases in pome fruit growing areas. AP-infected trees show typical symptoms such as witches' broom, enlarged stipules, tasteless, and dwarf fruits. PD-infected pears show a progressive weakening, reduced terminal growth, smaller fruits, and die within weeks (quick decline) or years (slow decline). The diseases are caused by the cell-wall lacking bacteria Candidatus Phytoplasma mali (AP phytoplasma) and Ca. P. pyri (PD phytoplasma), respectively. In previous studies it has been shown that AP-infected apple trees emitted higher amounts of the sesquiterpene β-caryophyllene, an attractant of the insect vector Cacopsylla picta (Hemiptera: Psyllidae), thereby facilitating the dispersal of AP phytoplasma. In the present study, volatile organic compounds (VOCs) occurring in the headspace of plants infected with Ca. P. mali strains causing different severity of symptoms in apple plants were collected, analyzed, and identified. Headspace samples from healthy and AP-infected model plant tobacco (Nicotiana occidentalis) and apple (Malus domestica) as well as from healthy and PD-infected pear (Pyrus communis) were investigated via thermodesorption and GC-MS analysis. Significantly higher concentrations of ethyl benzoate were produced in all phytoplasma-infected plants compared to healthy ones and an as yet unidentified sesquiterpene differed between the odor bouquets of healthy and by Ca. P. mali infected tobacco plants. Additionally, statistically significant higher amounts of both compounds were measured in the headspace of plants infected by the virulent AP strain. In apple, significantly higher concentrations of ethyl benzoate and methyl salicylate were observed for trees infected with strains of Ca. P. mali. Ethyl benzoate was also detected in the headspace of pear trees infected with Ca. P. pyri.
Introduction
Phytoplasmas are prokaryotes of the class Mollicutes and obligatory parasitize two different hosts, plants and vector insects. They occur in plants mainly in the phloem tissue (Doi et al., 1967). Phytoplasmas lack a cell wall and require insects for transmission. The insect vectors of phytoplasmas are phloem feeders of the order Hemiptera, mostly leafhoppers (Cicadellidae), planthoppers (Fulgoromorpha) and psyllids (Psyllidae; Weintraub and Beanland, 2006). The vector insects feed from the nutrient-rich phloem sap containing the phytoplasmas and acquire them in their digestive system (acquisition feeding). Infected insects can transmit the phytoplasmas again to the host plants by feeding on healthy plants (inoculation or transmission feeding). Some phytoplasmas have high vector specificity, being transmitted by only one or a few vectors whereby others can be transmitted by a huge range of insect vectors (Seemüller et al., 2002; Mann et al., 2012). For the transmission of European fruit tree phytoplasmas different psyllid species belonging to the taxon Cacopsylla are responsible. Pear decline (PD) caused by Candidatus Phytoplasma pyri can be transmitted by C. pyricola and C. pyri (Davies et al., 1992; Carraro et al., 1998). In contrast, the apple proliferation (AP) disease caused by Ca. Phytoplasma mali is exclusively transmitted by C. picta (Mayer et al., 2009) which forms a highly specialized relationship to its host plant of the genus Malus spp. (Mayer et al., 2008a,b, 2011). Phytoplasmas replicate intracellularly in both plant and insect tissues and have different impacts on the fitness of their hosts. In most instances, plants are negatively affected but infection of insect vectors may have positive or no adverse effects on their fitness (Beanland et al., 2000; Bressan et al., 2005; Mayer et al., 2011).
AP and PD are the most severe diseases in pome fruit growing areas. The diseases are caused by the cell-wall lacking bacteria Candidatus Phytoplasma mali (AP phytoplasma) and Ca. P. pyri (PD phytoplasma), both belonging to the apple proliferation (16SrX) group. AP-infected trees (Malus ssp.) show typical symptoms such as witch's broom, enlarged stipules, tasteless and dwarf fruits. PD-infected pear trees (Pyrus spp.) show progressive weakening characterized by reduced terminal growth, smaller fruits, and die within weeks (quick decline) or years (slow decline; Carraro et al., 2001). Seemüller and Schneider (2007) divided a set of 24 strains of Ca. P. mali into three categories due to their AP-disease severity in apple trees. Virulent and severe strains induce the typical symptoms such as witch's broom and overall dwarfism. Moderate virulent strains elicit milder symptoms, for example enlarged stipules. Avirulent strains, on the contrary, induce unspecific symptoms, or none at all. Avirulent and virulent strains exhibit considerable differences. For example, virulent strains have larger genomes than avirulent strains (Seemüller and Schneider, 2007). Additionally there are suggestions that the severity of symptoms could be influenced by environmental conditions or the titer of the phytoplasmas (Seemüller et al., 1984). However, Seemüller and Schneider (2007) showed that the symptoms differed between several strains although they found identical numbers of phytoplasma cells.
Some psyllids use chemical cues for orientation and host identification (Soroker et al., 2004; Gross and Mekonen, 2005; Martini et al., 2014a). The proteobacterium Ca. Liberibacter asiaticus modifies the odors released by its host plant (citrus trees) to attract its vector, the Asian citrus psyllid (Diaphorina citri). Infection of citrus plants with this pathogen induces release of methyl salicylate which specifically attracts its vector (Mann et al., 2012). This pathogen causes huanglongbing, an incurable disease that threatens commercial citrus industries worldwide (Grafton-Cardwell et al., 2013). The proteobacterium Ca. Liberibacter solanacearum is vectored by the tomato/potato psyllid (Bactericera cockerelli) and causes Zebra chip disease in potatoes. Infected tomato plants emit a qualitatively and quantitatively different blend of VOCs compared to uninfected plants. Infected psyllids preferred to settle on uninfected plants and vice versa (Mas et al., 2014). Apple trees infected by a virulent strain of Ca. Phytoplasma mali showed an overproduction of β-caryophyllene (Mayer et al., 2008a), resulting in increased attraction of “emigrant” adults of the vector Cacopsylla picta (Mayer et al., 2008b, 2011). Through increased feeding on infected plants, the probability of phytoplasma acquisition increases after overwintering adults (“remigrant”) colonize healthy plants. Behavioral changes of the vectors promote both pathogen acquisition and transmission because remigrants prefer uninfected plants for oviposition while emigrants prefer infected host plants (Mayer et al., 2011). Consequently, the question arises whether strains of Ca. P. mali of different virulence possess the same ability to change plants' VOCs emissions and, if so, what are the differences?
In this study, the influence of two strains of Ca. P. mali, one eliciting pronounced symptoms (virulent strain) and the other less pronounced symptoms (avirulent strain), on volatile emissions of the model plant tobacco (Nicotiana occidentalis) were examined. Headspace samples from healthy and infected tobacco were investigated via thermodesorption followed by GC-MS analysis. The data were confirmed by collecting and analyzing VOCs of both AP phytoplasma infected and healthy apple trees and compared to VOC emissions of pear trees infected by Ca. P. pyri.
Material and Methods
Plant Material and Phytoplasma Cultivation: Tobacco
The model plant tobacco (N. occidentalis) was used in the experiments because this plant grows much faster than woody pome fruit trees (apple and pear), but the phytoplasmas cause similar symptoms in the model plant. In the summer and autumn of 1993, samples from symptomatic and non-symptomatic apple trees infected by Ca. P. mali were collected (Seemüller and Schneider, 2007). Infected shoot scions were grafted on healthy rootstocks (M 11) followed by top-grafting with cv. Golden Delicious and maintained by periodic grafting (Seemüller and Schneider, 2007). For permanent cultivation of these phytoplasma strains, they were transmitted to periwinkle (Catharanthus roseus) by regular grafting in an insect-proof greenhouse. For transmission from periwinkle to tobacco, the phytoplasma strains Ca. P. mali 1/93 and 12/93 (Seemüller and Schneider, 2007) were transmitted via the parasitic plant dodder (Cuscuta europaea) which built phloem bridges between the two plants; method described by Mikona and Jelkmann (2010). Infection was proven through DNA extraction of phloem tissue followed by real-time PCR (s.b.). The tobacco plants either infected by the avirulent strain (1/93), by the virulent strain (12/93), or without an infection, were examined every week over a period of 5 weeks beginning with the week in which the first symptoms were detected in plants infected by the virulent strain (= 8 weeks after inoculation with the phytoplasma). Volatiles from the headspace of at least six plant individuals were collected at each examination from every treatment group.
Plant Material and Phytoplasma Cultivation: Apple and Pear
The apple plants (Var. Golden Delicious on rootstock M 9) were grafted with scions from already infected apple trees (Ca. P. mali) in 2012. Here the virulent strain AT 3/6 was used for infection. Pear trees (Var. Williams Christ on root stock Kirchensaller) were also inoculated by grafting with already infected pear plants (Ca. P. pyri strain PDW). Infection was checked via DNA extraction from the phloem tissue of plants and amplified by specific primers (s.b.). From February to June, volatiles from infected and healthy apple and pear trees were collected each with four replications once a week. The plants were cultivated in an insect-safe plastic-mesh greenhouse in order to provide natural conditions (day length, light intensity, temperature following natural fluctuations during a season) but without the threat of herbivorous pest insects. A fungicide against apple mildew was applied maintain the health of apple trees. The withholding period between fungicide application and headspace sampling was at least 1 week. Infected and healthy plants were cultivated under the same conditions. Headspace sampling of apple and pear plants started at phenological stage 00 following the BBCH-scale (Meier, 1997) and stopped when emigration from the reproductive host began. For direct comparisons, plants were grouped into phenological growth stages according to the BBCH scale for pome fruits (Meier, 1997): 2 = BBCH 7-11 (beginning of bud break—first leaves unfolded), 3 = BBCH 19-33 (first leaves fully expanded—shoots about 30% of final length), 4 = BBCH 34-35 (shoots about 40–50% of final length), 5 = BBCH = 35 (shoots more than 50% of final length, and 6 = remigration. For the comparison of apple odor the growth stages 2–5, for pear odor comparison all six growth stages were analyzed.
Headspace Sampling
An innovative headspace sampling device enabling an exact collection of relative quantities of VOCs in headspace samples was engineered for these measurements by the author JG together with Ralph Kunath (Wagner Mess- und Regeltechnik GmbH, Offenbach, Germany). The device consisted of five parallel odor collection systems which were mounted on a plate, connected by tubes and wired electrically (Figure 1). Each odor collection system was constructed by a vacuum pump (KNF Neuberger GmbH, Freiburg, Germany) connected with a mass flow controller (M+W Instruments GmbH, Leonhardsbuch, Germany). The controller measured both flow rate and total collected air volume which was programmed using the integrated TFT display or by connecting with a laptop computer. Flow rate regulated the pump throughput constantly but, in case of reduced air flow, the throughput of the vacuum pump was increased automatically. Thus, an exact and comparable flow rate between all five collecting systems was ensured. Between the single odor collection systems no connections by tube were made which prevented cross contamination. Single branches of apple trees or tobacco plants were carefully wrapped in oven plastic bags made of polyethylene terephthalate (20 cm diameter, Melitta, Minden, Germany). A stream of purified air (1000 ml/min), controlled by the headspace sampling device, was pumped through each bag until it reached the final volume of 100 L. The air stream was purified by passing through a gas washing bottle filled with charcoal (granulated 4–8 mm, AppliChem GmbH, Darmstadt, Germany). An empty oven bag not enclosing plant material connected to the sampling device served as a procedural control. After each trial, the washing bottles and tubes were rinsed with 70% ethanol (p.a.; Merck Millipore, Germany) and baked at 100°C for at least 2 h. Volatiles from headspace sampling were trapped in stainless steel, prepacked sample tubes with Tenax® TA60/80 sorbent (PerkinElmer, Markes). Used tubes were closed with Teflon-coated brass compression caps (Swagelok, PerkinElmer) and stored for a maximum of 1 week before being thermodesorbed. Headspace samples from tobacco plants infected by the virulent (N = 42) or the avirulent Ca. P. mali strain (N = 40) and headspace samples from healthy plants (N = 27) were analyzed by GC-MS.
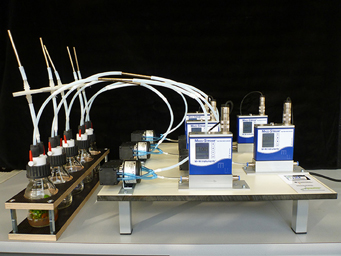
Figure 1. Newly developed five-channel headspace sampling device enabling an exact quantitative measurement of VOCs in headspace samples from both in vitro plants (picture, left) or bigger plants. For detailed description compare material and methods. Photograph: Sabine Wetzel, JKI.
Thermodesorption-GC-MS
Samples were analyzed using an automated thermal desorber (TurboMatrix™ ATD 650, PerkinElmer) connected to a GC-MS (gas chromatograph coupled with mass spectrometer) instrument. The thermal desorption details were as follows: Tube desorption 10 min at 250°C and cold trap (Tenax TA) was held at −20°C throughout the tube desorption process, and then heated at a rate of 99°C/s to 250°C; cold trap desorption time 1 min. The desorbed volatile compounds were separated using a PerkinElmer® Clarus® 680 GC system coupled to a Perkin Elmer quadrupole inert mass selective detector for molecular structure analysis. A nonpolar Elite-5 (Crossbond 5% diphenyl −95% dimethyl polysiloxane, PerkinElmer) capillary column (30 × 0.25 mm id × 0.25 μm film thickness) was used for the GC separation. Splitless injection was employed using helium as the carrier gas (Helium, Air Liquide, Germany) at a flow rate of about 5 ml/min (column head pressure 150 kPa). The initial oven temperature was 40°C and was held for 1 min, followed by a linear programmed temperature from 40 to 180°C at a rate of 5°C/min, and a rate of 20°C/min from 180 to 280°C. The final temperature of 280°C was held for 6 min. This temperature program was used for the analysis of all samples. The ion source temperature was 180°C and GC inlet line temperature was 200°C. Quadrupole mass detector was operated in the electron-impact (EI) mode at 70 eV. The electron multiplier was set to the auto tune procedure. All data were obtained by collecting the full-scan mass spectra within the range of 35–350 m/z. The volatile compounds were identified by comparing the characteristic ion fragmentation pattern (mass spectrum) with data from mass spectra libraries (NIST 08 Mass Spectral Library, National Institute of Standards and Technology, Wiley; JKI-OW Library) and by comparing retention times of standard compounds according to Weintraub and Gross (2013). For relative quantification, the peak areas were integrated, relative proportions of detected compounds were calculated and used for further analyzing, in which the sum of the selected compounds were set as 100%.
Statistical Analysis
After excluding peaks of irregular occurrence, such as contaminants and compounds not separable on the elite five column, multivariate techniques of data analysis—principal component analysis (PCA) and discriminant function analyses (DFA)—were employed to detect volatile compounds of tobacco capable of differentiating between the three infection conditions investigated. The relative proportions of the compounds, which were consistent in all replicates of plant samples were used in a PCA, followed by a DFA (Backhaus et al., 1987) using SPSS 21.0 (IBM). All DFAs were performed with seven principal components (PCs) with an Eigenvalue greater than one. The standardized discriminant function coefficient and the component loadings were used to assess the importance of individual compounds. The two PCs with the highest component loadings were considered to be the most influential components. The chemical compounds which contributed significantly to variations in plant odors were tested for significant differences between respective groups using Kruskal-Wallis non-parametric tests followed by a post-hoc Mann-Whitney U-test and correction of α-levels for multiple comparisons [following Bonferroni-Holm; Holm (1979)]. Hence, p1 < 0.0166; p2 < 0.025; p3 < 0.05 for a global significance level of 0.05. Comparison of relative volatile amounts released by infected and healthy pome fruits were analyzed using Mann-Whitney U-test. Significance level was set at p < 0.05.
DNA Extraction and Amplification
For identification of phytoplasma infections in plants, DNA was extracted from the phloem tissue and amplified by specific primers. Phloem tissue from the midrib of a leaf of respective plant (tobacco, apple, pear) was abraded. Between 0.1 and 0.3 g of midrib tissue was selected for DNA-extraction according to Doyle and Doyle (1990). The extraction was performed under standardized conditions. The nucleic acid pellets were resuspended in 50 μl of sterile distilled water and stored at −20°C. Amplification of DNA was performed in 25 μl reactions containing 5 pmol of each primer, 0.1 mM of each dNTP, 1 U of heat-stable polymerase (KAPA Biosystems) and 1 x polymerase buffer. The reaction was cycled in a thermocycler (Eppendorf) with the following parameters: initial denaturation 95°C, 2 min; 35 cycles of denaturation (95°C, 45 s), annealing (52°C, 45 s), elongation (72°C, 1 min); final elongation 72°C 5 min. The PCR-products were electrophoresed on a 1% agarose gel containing ethidium bromide (0.3 μg/ml) in 1x TAE buffer (40 mM GTris, 20 mM acetic acid, 1 mM EDTA, pH 8.0). PCR-products were visualized and photographed under UV light. For identification of the phytoplasmas belonging to the apple proliferation group, the primer pair fO1/rO1 (5′-CGGAAACTTTTAGTTTCAGT-3′ and 5′-AAGTGCCCAACTAAATGAT) were used (Lorenz et al., 1995). By the use of a Ca. P. mali specific primer pair fAT/rAS (5′-CATCATTTAGTTGGGCACTT-3′ and 5′-GGCCCGGACCATTATTTATT-3′) a 400 bp sequence in the 16S–23S ribosomal ribonucleic acid spacer region was amplified and used to identify phytoplasma DNA from Ca. P. mali (Smart et al., 1996).
Real-Time PCR
Real-Time PCR was performed with the iCycler IQ (Bio-Rad Laboratories GmbH, Munich, Germany) only in tobacco to differentiate between the two phytoplasma strains (virulent and avirulent) analyzed. The amplification was performed in 25 μl reactions containing 25 pmol of each primer (for strain 1/93: f460_B_1-93: 5′-GTGCCCGAAATCCCTACAAAAG-3′ & r460short_1-93: 5′-GAAGGGGTAAATTTTATCTTTTTTT-3′; for strain 12/93: f460_B_12-93: 5′-GTGCCCGAAATCCCTCCAAAAA-3′ & r460_12-93: 5′-GTTTGAAGAGGTGAAATTTATATTTTTTC-3′), 2 pmol of Taqman probe (for strain 1/93: 5′-TTGGAACATCATTTAATTTTTTTTC-3′; for strain 12/93: 5′-CTGGAGCATCAGTTAATTTTT TTG-3′) with a reporter fluorescence dye (for strain 1/93: Cy5; for strain 12/93: FAM) at the 5′ end and a quencher dye (for strain 1/93: BHQ3; for strain 12/93: BHQ1) at the 3′ end, 0.2 mM of each dNTP, 1 U of heat-stable polymerase (Tempase, Amplicon), 1 × polymerase buffer, and 1 μl of DNA. PCR conditions were as follows: 15 min at 95°C, followed by a two-step protocol consisting of 39 cycles at 95°C for 15 s and 54°C for 30 s. The primer pairs, as well as the marked probe, are very specific for a target sequence of an AAA + ATPase (APT00460) of each strain (Schneider, personal communication).
Results
Identification of Volatile Compounds Emitted by Model Plant (Nicotiana occidentalis)
Thirty compounds were recognized by GC analysis. Twenty-eight of these compounds were consistent in all replicates of 109 plant samples and considered for statistical analysis (Figure 2; Table 1). Twenty compounds could be identified with confidence. For six compounds, only the substance class has been identified and two compounds remained unknown. The substance subset is composed by several plant volatiles such as amino acid derivatives, esters, mono-, and sesquiterpenes. The most abundant compounds found in all tobacco plant headspace samples were green leaf volatiles (GLV) such as n-hexanal (2), (Z)-3-hexene-1-ol (3), and (Z)-3-hexene-1-ol acetate (12) in addition to an unidentified butanoic acid ester (14), ocimene (17), a second unidentified butanoic acid ester (19), and β-caryophyllene (25) with different compositions within the tested odor bouquets (numbers following Figure 2). An analysis of the relative concentrations of the compounds revealed differences between infection status (virulent infected, avirulent infected, healthy), as well as between stage of infection (8–12th week after infection). The most obvious identifiable VOC's difference between the infected and the healthy tobacco plants was the higher occurrence of ethyl benzoate (22; RT 14.2) and an unidentified sesquiterpene at RT 23.4 (28) in infected plants as opposed to healthy tobacco plants (Figure 2).
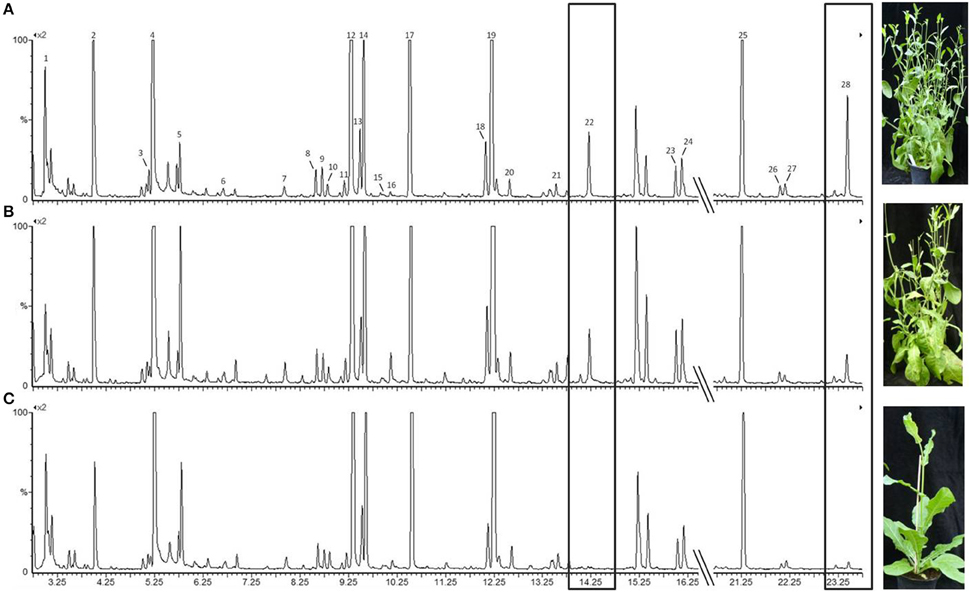
Figure 2. Total ion current chromatogram obtained from GC-MS analysis of odor spectra of tobacco plants under different infection conditions. The visible symptoms (shoot proliferation) caused by the respective phytoplasma strain are shown in the photograph. (A) infected by the virulent strain; (B) infected by the avirulent strain; (C) healthy tobacco plant. Amplification: 1*109. Ethyl benzoate (22) and an unidentified sesquiterpene at 23.4 min (28) were present in the odor of infected plants but not in the one of uninfected plants. Numbering of relevant peaks follows the numbering in Table 1. Photographs by Margit Rid, JKI.
For the statistical analysis of both qualitative and quantitative differences between the three treatments, the calculated relative concentrations of the respective compounds were used (given as percentages). Canonical linear discriminant function analysis (DFA) was performed with seven PCs with an Eigenvalue above one explaining 77.17% of the variance to test for differences in tobacco VOC spectrum within different infection conditions. For every week after infection, a unique DFA was obtained (Figure 3). Up until 9 weeks after infection, the plants' odor pattern did not differ—as revealed by the non-significant discriminant functions (Figure 3A: e.g., 8th week: DFA: discriminant function 1: χ2 = 20.360, df = 14, p = 0.11; discriminant function 2: χ2 = 7.295, df = 6, p = 0.294). After the 10th week, the volatile pattern of the three conditions of tobacco plants could be statistically separated with respect to relative concentrations of individual compounds according to their infection condition (Figure 3B: 10th week: DFA: discriminant function 1: χ2 = 34.074, df = 14, p = 0.002; discriminant function 2: χ2 = 11.508, df = 6, p = 0.074; Figure 3C: 11th week: DFA: discriminant function 1: χ2 = 29.207, df = 14, p = 0.010; discriminant function 2: χ2 = 7.776, df = 6, p = 0.255; Figure 3D: 12th week: DFA: discriminant function 1: χ2 = 30.821, df = 14, p = 0.006; discriminant function 2: χ2 = 5.177, df = 6, p = 0.521). From the 11th week infection, the odor compositions of the two infected plants were also separated statistically. It is important to mention that the group centroid based on the odor of the plant infected with the avirulent strain is located intermediate with respect to the x-axis between the one infected with the virulent strain and the healthy one (Figures 3C,D).
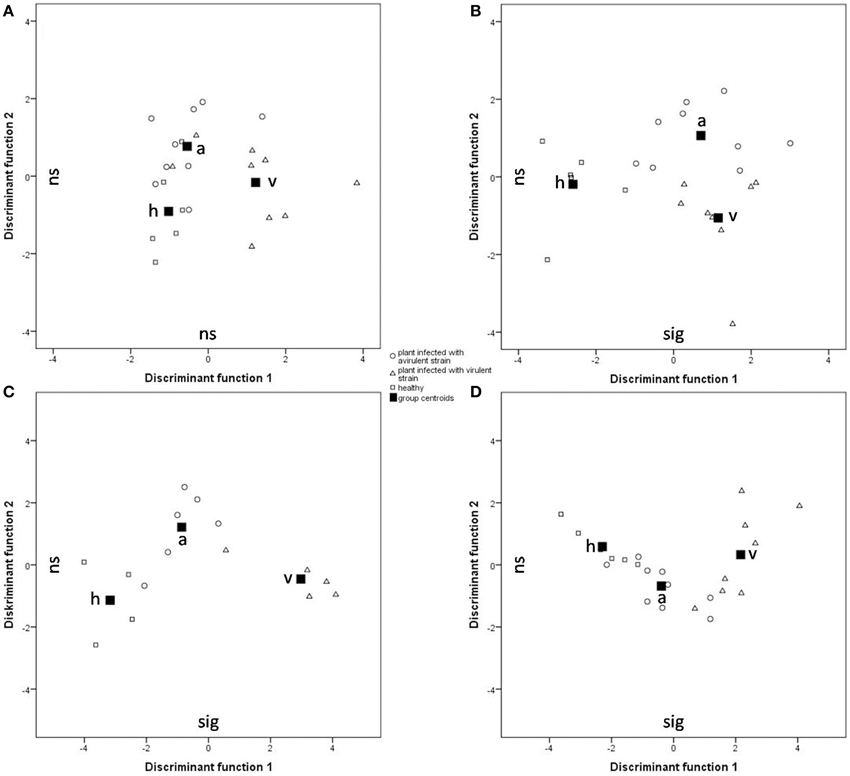
Figure 3. Comparison of the odor composition of tobacco plants' headspace according Ca. P. mali infection conditions using a DFA. Group centroids are depicted as squares. h, healthy plants (squares); a, tobacco plants infected by the avirulent strain (circles); v, tobacco plants infected by the virulent strain (triangles). (A) 8 weeks after grafting (Nh = 6, Na = 9, Nv = 9; discriminant function (df) 1: ns, df 2: ns); (B) 10 weeks after grafting (Nh = 6, Na = 8, Nv = 9; df 1: sig, df 2: ns); (C) 11 weeks after grafting (Nh = 4, Na = 6, Nv = 6; df 1: sig, df 2: ns); (D) 12 weeks after grafting (Nh = 6, Na = 9, Nv = 8; df 1: sig, df 2: ns).
Discriminant functions were constructed using up to seven PCs and every PC received an individual component loading to assess the importance of individual compounds. Function 1 was always strongly correlated with PC4 which consisted of ethyl benzoate, the unidentified sesquiterpene at RT 23.4 (ST 23.4) and α-caryophyllene. Additionally, significant differences were found between these particular compounds within tested plants (Kruskal-Wallis, p < 0.05). Ethyl benzoate contributed to a greater extent to the scent bouquet of infected plants relative to healthy plants (Figure 4A; Mann-Whitney, p < 0.05, following Bonferroni-Holm correction) and contributed to the separation of infected and healthy plants. Among the two infected plant treatments, there was no general difference of the relative amount of ethyl benzoate. In contrast, the relative amount of ST 23.4 differed statistically significant between the three tested infection conditions of plants 11 weeks after infection (Mann-Whitney, p < 0.05, following Bonferroni-Holm correction; Figure 4B). Hence, prior to this time, it is possible to distinguish between infected and healthy plants as well as between the two infected plant treatments based on ST 23.4.
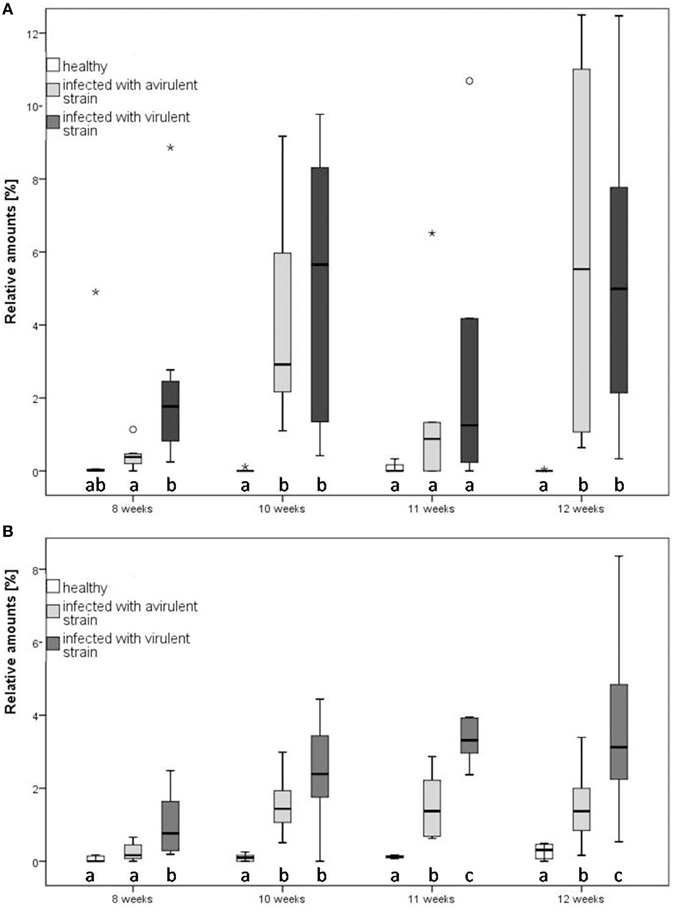
Figure 4. Relative amounts of two compounds of tobacco (Nicotiana occidentalis) at three plant infection conditions at different infection states in direct comparison. (A) Ethyl benzoate, (B) sesquiterpene 23.4. Different letters indicate statistical differences (Mann-Whitney, p < 0.05, followed by Bonferroni-Holm correction). Notice that outliers are indicated by circles and extremes by asterisks. The relative amounts of emitted ethyl benzoate and ST 23.4 are at every time point higher in the infected plants, compared to healthy ones.
Classification by cross validation, a statistical method to verify the LDA model, confirmed these results, as 56.5–68.8% of cross validated grouped cases were classified correctly (Table 2). Ten weeks after infection, plants infected with the virulent strain were never wrongly classified as healthy plants according to their odor patterns and vice versa. In contrast, incorrect classification of tobacco plants infected with the avirulent strain (mistaken for plants infected with the virulent strain or healthy plants) was common (Table 2). The VOCs of the plant infected with the avirulent strain had similarities with both the VOCs of the healthy plants and plants infected with the virulent phytoplasma strain. However, the longer a tobacco plant had been infected (>9 weeks), the more distinctly a differentiation between infected and healthy conditions could be detected. From the 10th week onwards, even the influence of the type of strain (virulent or avirulent) on the odor pattern could be detected.
Volatiles Emitted by Infected and Healthy Apple (Malus communis) and Pear (Pyrus communis) Plants
Twenty VOCs consistently present in chromatograms in all replicates of apple and pear were selected for statistical analysis. At the beginning of the experiment, apple trees infected by Ca. P. mali showed earlier sprouting than healthy trees. While the AP infected plants attained BBCH 07-09, healthy apple trees remained in dormancy. Additionally, significant higher concentrations of (Z)-3-hexene-1-ol, benzaldehyde and linalool were produced by healthy apple plants at BBCH 19-33 (Mann-Whitney, p < 0.05; data not shown). At BBCH 34-35, benzaldehyde, heptanal, and hexyl acetate occurred more often in healthy plants (Mann-Whitney, p < 0.05; data not shown). In contrast, a significant higher release of ethyl benzoate (at BBCH 34-35; Figure 5A) and methyl salicylate (at BBCH 19-33; Figure 5B) was observed for apple trees infected with Ca. Phytoplasma mali (Mann-Whitney, p < 0.05). At the beginning of sprouting (BBCH 7-11) there were no noticeable differences in the occurrence of ethyl benzoate. From BBCH 34-35 onwards, the relative concentration of this volatile ester increased steadily. Increased release of methyl salicylate by infected apple trees was confirmed for all phenological stages except BBCH 7-11. In pear trees infected with Ca. P. pyri, the expression of ethyl benzoate began at BBCH 19-33, differed significantly between infected and healthy trees at BBCH 34-35 but was not expressed at any other stages (Figure 6).
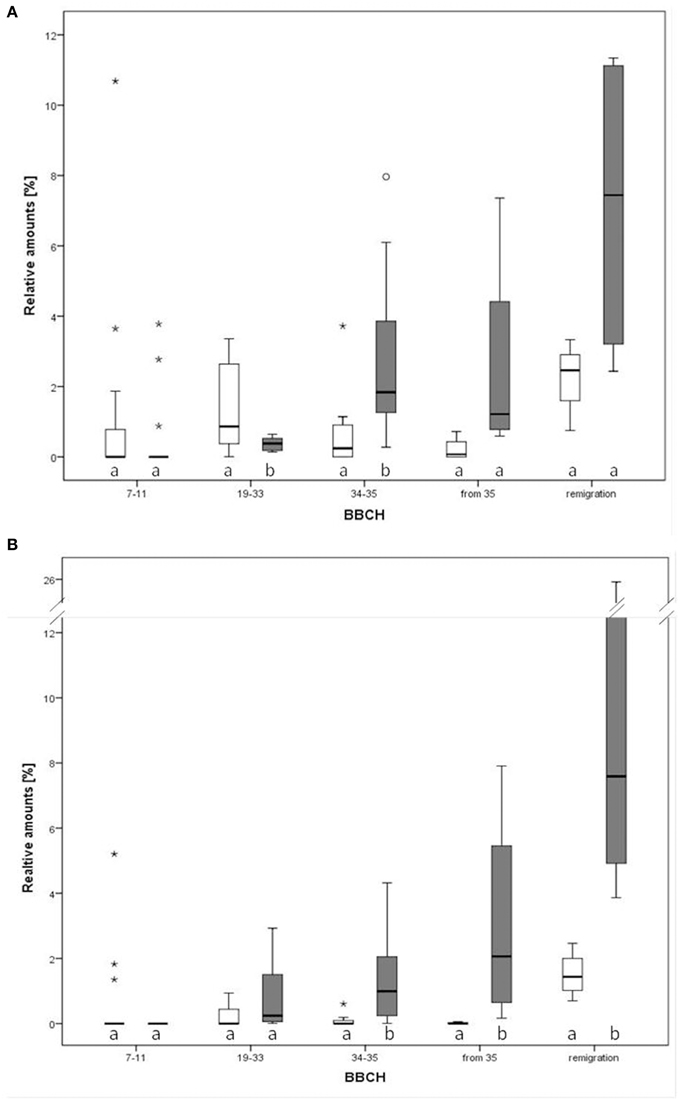
Figure 5. Relative amounts of two compounds in the direct comparison of infected and healthy apple plants (Malus domestica) at different phenological growth stages. (A) Ethyl benzoate, (B) methyl salicylate. Different letters denote statistical differences (Mann-Whitney, p < 0.05). Notice that outliers are indicated by circles and extremes by asterisks.
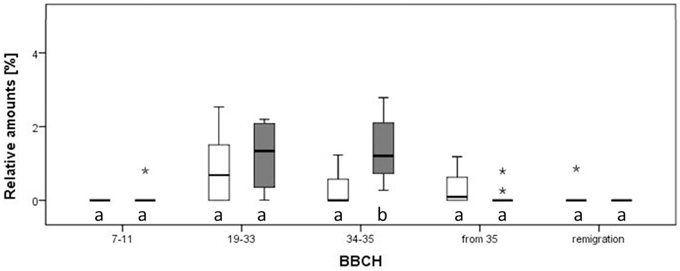
Figure 6. Relative amounts of ethyl benzoate in the direct comparison of infected and healthy pear plants (Pyrus communis) at different phenological stages. Different letters denote statistical differences (Mann-Whitney, p < 0.05). Notice that outliers are indicated by circles and extremes by asterisks.
Discussion
This is the first indication that different strains of a phytoplasma, differentiated by their virulence, possess different abilities to modulate the emission of volatiles by hosts (tobacco and apple). Significantly higher concentrations of ethyl benzoate were produced by all phytoplasma-infected plants compared to healthy plants and the concentration of an unidentified sesquiterpene (ST 23.4) differed between the bouquets of healthy and Ca. P. mali infected tobacco (Figure 4). Both ethyl benzoate and ST 23.4 occur in infected tobacco plants in higher concentrations independent of the virulence of the strain of phytoplasma. Additionally, ethyl benzoate was also detected in statistically higher concentrations in the odor of pear trees (Pyrus communis) infected by Ca. Phytoplasma pyri (Figure 6).
Ethyl benzoate is an ester formed by the condensation of benzoic acid and ethanol. It is very common in flower and fruit odors of many plants. Some animal species utilize ethyl benzoate in their chemical communication system. For example, it is part of the pheromone mix of the interdigital gland of male white-tailed deer (Gassett et al., 1996) and it is an attractant for some tephritids like the oriental fruit fly Bactrocera dorsalis and the West Indian fruit fly Anastrepha oblique (Chu et al., 1996; Cruz-López et al., 2006). While ethyl benzoate is not a common volatile of leaves, it was produced by apple leaves after infestation by the spider mite Tetranychus urticae (Takabayashi et al., 1991). In our tobacco model, as well as in the host plants apple and pear, a higher emission of ethyl benzoate by infected plants was observed. It seems that there is a direct link between infection with phytoplasmas and ethyl benzoate production. Ethyl benzoate may be an active compound for the vector of the apple proliferation disease C. picta. The concentration of ethyl benzoate emitted by apple leaves is higher when infected with Ca. P. mali than by healthy apple plants. Ethyl benzoate is capable of eliciting reactions from insects of other genera (Chu et al., 1996; Cruz-López et al., 2006; Beck et al., 2011) but to confirm whether psyllids, especially C. picta, recognize and are responsive to it will require that additional EAG (electroantennography) and olfactometer experiments are performed.
The sesquiterpene ST 23.4 occurs in the odor of infected tobacco plants in different concentrations. Since this compound was only emitted by tobacco, we refrained from attempting a full identification which would have been difficult and costly. Apple and tobacco plants are both capable of producing β-caryophyllene. While in apple plants, Ca. P. mali elicited an increase of β-caryophyllene (Mayer et al., 2008a,b), ethyl benzoate and methyl salicylate expression, an increase in another sesquiterpene (the unidentified compound ST 23.4) was induced in tobacco. Both sesquiterpenes may share identical precursors for their biosynthesis (Dudareva et al., 2004) but the reason why Ca. P. mali elicits the emission of different sesquiterpenes in two plant species, which are both able to produce β-caryophyllene, is still elusive. A possible explanation is that in both studies, different strains of Ca. Phytoplasma mali were used. The apple plants analyzed by Mayer et al. (2008a,b) were infected with strain AT and tobacco plants analyzed in this study with strains 1/93 (avirulent) and 12/93 (virulent). Additionally, also different cultivars of apple were used in the two studies. While Mayer et al. (2008a,b) conducted their investigations using the cultivar “Gala,” the cultivar “Golden Delicious” was used in this study. It was shown that β-caryophyllene was emitted by the roots of European maize cultivars infested by subterranean Diabrotica virgifera larvae (Rasmann et al., 2005). Nevertheless, this important defense compound could not be detected in most American maize cultivars (Köllner et al., 2008). Thus, as a consequence of artificial selection for specific traits like size, smell or color, many crop plant varieties underwent genotypic and phenotypic changes during their domestication which may have been associated with the loss of specific VOCs. In the final step of terpene biosynthesis only one enzyme defines the differentiation in a mono- or sesquiterpene. Volatile terpenoid biosynthesis is predominantly regulated at transcription (Sharon−Asa et al., 2003; Wang et al., 2008). Thus, it is likely that pathogen virulence factors may change the overall volatile blend of a plant by regulating gene expression by terpene synthases/cyclases (TPS). Like seen for inducing β-caryophyllene in apple (Mayer et al., 2008b) and ST 23.4 in tobacco, Ca. P. mali possess the ability to change sesquiterpene production in plants. However, silent TPSs, which are not expressed under normal conditions but in pathological circumstances, were reported recently (Orlova et al., 2009). The genes affected and mechanisms remain unknown. Phytoplasma virulence factors have been shown to interfere with gene regulation of infected plants (Hoshi et al., 2009; Sugio et al., 2011b; Maclean et al., 2014). If they are capable of interfering with terpene biosynthesis, they should become the focus of further investigation.
Methyl salicylate was released by infected apple trees of all phenological growth stages, except BBCH 7-11 (Figure 5A). This ester is naturally produced by many species of plants and has been reported in numerous floral scents (Knudsen et al., 1993). It has been shown that methyl salicylate was also released from vegetative tissues attacked by herbivores (Van Poecke et al., 2001). In another plant-pathogen system it was recently shown that methyl salicylate was induced in the leaves of Citrus spp. by Ca. Liberibacter asiaticus (Mann et al., 2012). Moreover, methyl salicylate is attractive to Diaphorina citri and has been suggested to mimic a psyllid pheromone (Mann et al., 2012). Methyl salicylate is also attractive to some insect predators and parasitoids (Van Poecke et al., 2001; Martini et al., 2014b; Jones et al., 2015).
In addition to specific secondary compounds, general (GLVs) contributed a considerable proportion to the odor of infected and healthy tobacco and apple plants in our study. GLVs are C6 molecules which are produced and emitted rapidly following herbivory or pathogen infection by almost every green plant (Scala et al., 2013). Mechanical damage of plant tissues can result in both quantitative and qualitative changes in the volatile emissions of GLVs (Scala et al., 2013). It has been shown that phytoplasmas can interfere with the synthesis of jasmonic acid (JA) (Sugio et al., 2011a). Since both JA and GLV are produced via the lipoxygenase pathway (Dudareva et al., 2004), it is possible that phytoplasmas are also capable of inducing emissions of GLVs. Moreover, because the emission of GLVs was observed in some healthy tobacco plants, it is possible that some of the control plants had been accidently damaged by handling.
Due to the short generation time of tobacco, as opposed to woody apple, greater replication was achieved in a shorter time. Although tobacco exhibited several differences to apple, both the development of disease symptoms and odor alteration due to infection, were able to investigated with this model (Figure 2). While tobacco is not a suitable host for the vector, changes in odor due to infection with Ca. P. mali appear similar to those in apples and provide hints as to the compounds which should be included in future investigations. Presumably, tobacco and apple share some VOC biosynthesis pathways (Dudareva et al., 2004), in particular the production of mono- or sesquiterpenes which rely on identical precursors.
The relative amount of compound ST 23.4 in tobacco varied significantly between the two virulence strains. On one hand, the higher emission of VOCs in plants infected with Ca. Phytoplasma mali could be caused by an increased amount of vascular tissue and leaf surface due to the proliferation associated with the production of witch's broom symptoms—as suggested by Mayer et al. (2008a). On the other hand, the plant tissue infected by the virulent strain may emit higher concentrations of VOCs. The less virulent strain is unable to elicit typical disease symptoms presumably because it lacks a factor which suppresses the plant's immune system. That the immune system can overcome a phytoplasma infection is one explanation of the so-called “recovery phenomenon” in AP-infested trees (Carraro et al., 2004). The recovery phenomenon is accompanied by a higher concentration of JA in asymptomatic but infected plants; in contrast, infected plants exhibiting disease symptoms have reduced JA concentrations (Patui et al., 2013). Thus, the virulent strain may suppress the plant immune system more effectively than the avirulent strain. The variation in symptoms observed in infected plants coincides with differential presence of phytoplasma effector genes (Sugio and Hogenhout, 2012). Whether effector proteins are involved in changing the odor profile is unknown.
Some species of psyllid have been shown to use semiochemicals in interspecific and intraspecific communication (Soroker et al., 2004; Mayer et al., 2011; Gross, 2013; Weintraub and Gross, 2013; Lubanga et al., 2014). While sex pheromones may play a role in mate location in some species of psyllid, it appears that vibrational signals may be even more important and perhaps effective for mate location and selection (Eben et al., 2014; Lubanga et al., 2014; Wood et al., 2015). Psyllid behaviors related to aggregation will be important to elucidate to better understand the epidemiology of phytoplasmas. Nevertheless, olfactory, gustatory and visual plant cues affecting psyllid behavior also need to be documented to understand the movement of psyllids between hosts and among modules within hosts (Farnier et al., 2014, 2015; Orlovskis et al., 2015). The differences in VOCs emitted by infected host plants depending on pathogen virulence add a new dimension to plant attractiveness to C. picta. Ongoing research will aim to clarify the underlying physiological and molecular interactions between phytoplasma and host plant as they relate to the emission of VOCs. Furthermore, the role of VOCs in vector attraction and phytoplasma epidemiology will be investigated.
Author Contributions
MR, JG, and CM designed the study and all authors contributed to the analysis and interpretation of the data. MR conducted the experiments with tobacco plants. CM performed the experiments with apple and pear trees. MR and JG wrote the first draft of the manuscript, which was edited and approved by all authors. JG and MA supervised the project.
Funding
CM was supported by funds of the Federal Ministry of Food and Agriculture (BMEL) based on a decision of the Parliament of the Federal Republic of Germany via the Federal Office for Agriculture and Food (BLE) under the innovation support program number 2815409810.
Conflict of Interest Statement
The authors declare that the research was conducted in the absence of any commercial or financial relationships that could be construed as a potential conflict of interest.
Acknowledgments
We thank Ralph Kunath (Wagner Mess- und Regeltechnik GmbH, Offenbach, Germany) and Achim Gönnawein (JKI Dossenheim, Germany) for help in developing and engineering the quantitative headspace sampling device. We are grateful to Svenja Stein, Sabine Wetzel and Kai Lukat (JKI Dossenheim) for excellent laboratory assistance and Jutta Schmid (University of Ulm, Germany) for statistical advices. Special thanks to Sabine Wetzel for taking the picture of the 5-channel headspace sampling device. We thank Felix Hergenhahn (JKI Dossenheim) for grafting and cultivation of the plants. The authors are grateful to Martin Steinbauer (La Trobe University, Melbourne, Australia) for helpful comments on an earlier draft of the manuscript and language improvements.
References
Backhaus, K., Erichson, B., Plinke, W., Schuchard-Ficher, C., and Weiber, R. (1987). Multivariate Analysemethoden. Eine anwendungsorientierte Einführung. New York, NY: Springer. doi: 10.1007/978-3-662-08891-3
Beanland, L., Hoy, C. W., Miller, S. A., and Nault, L. R. (2000). Influence of aster yellows phytoplasma on the fitness of aster leafhopper (Homoptera: Cicadellidae). Ann. Entomol. Soc. Am. 93, 271–276. doi: 10.1603/0013-8746(2000)093[0271:IOAYPO]2.0.CO;2
Beck, J. J., Higbee, B. S., Gee, W. S., and Dragull, K. (2011). Ambient orchard volatiles from California almonds. Phytochem. Lett. 4, 199–202. doi: 10.1016/j.phytol.2011.03.005
Bressan, A., Clair, D., Sémétey, O., and Boudon-Padieu, É. (2005). Effect of two strains of Flavescence dorée phytoplasma on the survival and fecundity of the experimental leafhopper vector Euscelidius variegatus Kirschbaum. J. Invertebr. Pathol. 89, 144–149. doi: 10.1016/j.jip.2005.03.001
Carraro, L., Ermacora, P., Loi, N., and Osler, R. (2004). The recovery phenomenon in apple proliferation-infected apple trees. J. Plant Pathol. 86, 141–146.
Carraro, L., Loi, N., and Ermacora, P. (2001). Transmission characteristics of the European stone fruit yellows phytoplasma and its vector Cacopsylla pruni. Eur. J. Plant Pathol. 107, 695–700. doi: 10.1023/A:1011923801387
Carraro, L., Loi, N., Ermacora, P., Gregoris, A., and Osler, R. (1998). Transmission of pear decline by using naturally infected Cacopsylla pyri L. Acta Hort. 472, 665–668. doi: 10.17660/ActaHortic.1998.472.89
Chu, Y. I., Li, J. L., Tung, C. H., Lin, S. H., and Chen, S. P. (1996). Attractive efficacy of three attractants for the oriental fruit fly, Bactrocera dorsalis (Hendel) (Diptera: Tephritidae). Plant Prot. Bull. Taipei 38, 59–66.
Cruz-López, L., Malo, E. A., Toledo, J., Virgen, A., Del Mazo, A., and Rojas, J. C. (2006). A new potential attractant for Anastrepha obliqua from Spondias mombin fruits. J. Chem. Ecol. 32, 351–365. doi: 10.1007/s10886-005-9006-7
Davies, D. L., Guise, C. M., Clark, M. F., and Adams, A. N. (1992). Parry's disease of pears is similar to pear decline and is associated with mycoplasma−like organisms transmitted by Cacopsylla pyricola. Plant Pathol. 41, 195–203. doi: 10.1111/j.1365-3059.1992.tb02338.x
Doi, Y., Teranaka, M., Yora, K., and Asuyama, H. (1967). Mycoplasma-or PLT group-like microorganisms found in the phloem elements of plants infected with mulberry dwarf, potato witches' broom, aster yellows, or Paulownia witches' broom. Ann. Phytopathol. Soc. Jpn. 33, 259–266. doi: 10.3186/jjphytopath.33.259
Dudareva, N., Pichersky, E., and Gershenzon, J. (2004). Biochemistry of plant volatiles. Plant Physiol. 135, 1893–1902. doi: 10.1104/pp.104.049981
Eben, A., Mühlethaler, R., Gross, J., and Hoch, H. (2014). First evidence of acoustic communication in the pear psyllid Cacopsylla pyri L.(Hemiptera: Psyllidae). J. Pest Sci. 88, 87–95. doi: 10.1007/s10340-014-0588-0
Farnier, K., Dyer, A. G., and Steinbauer, M. J. (2014). Related but not alike: not all Hemiptera are attracted to yellow. Front. Ecol. Evol. 2:67. doi: 10.3389/fevo.2014.00067
Farnier, K., Dyer, A. G., Taylor, G. S., Peters, R. A., and Steinbauer, M. J. (2015). Visual acuity trade-offs and microhabitat-driven adaptation of searching behaviour in psyllids (Hemiptera: Psylloidea: Aphalaridae). J. Exp. Biol. 218, 1564–1571. doi: 10.1242/jeb.120808
Gassett, J. W., Wiesler, D. P., Baker, A. G., Osborn, D. A., Miller, K. V., Marchinton, R. L., et al. (1996). Volatile compounds from interdigital gland of male white-tailed deer (Odocoileus virginianus). J. Chem. Ecol. 22, 1689–1696. doi: 10.1007/BF02272407
Grafton-Cardwell, E. E., Stelinski, L. L., and Stansly, P. A. (2013). Biology and management of Asian citrus psyllid, vector of the huanglongbing pathogens. Annu. Rev. Entomol. 58, 413–432. doi: 10.1146/annurev-ento-120811-153542
Gross, J. (2013). “Drugs for bugs: The potential of infochemicals mediating insect - Plant - microbe interactions for plant protection and medicine,” in Phytochemicals, Plant Growth, and the Environment, ed D. R. Gang (New York, NY: Springer Science+Business Media), 79–93.
Gross, J., and Mekonen, N. (2005). Plant odours influence the host finding behaviour of apple psyllids (Cacopsylla picta; C. melanoneura). IOBC WPRS Bull. 28, 351–355.
Hoshi, A., Oshima, K., Kakizawa, S., Ishii, Y., Ozeki, J., Hashimoto, M., et al. (2009). A unique virulence factor for proliferation and dwarfism in plants identified from a phytopathogenic bacterium. Proc. Natl. Acad. Sci. U.S.A. 106, 6416–6421. doi: 10.1073/pnas.0813038106
Jones, V. P., Horton, D. R., Mills, N. J., Unruh, T. R., Baker, C. C., Melton, T. D., et al. (2015). Evaluating plant volatiles for monitoring natural enemies in apple, pear and walnut orchards. Biol. Control. doi: 10.1016/j.biocontrol.2015.03.009. [Epub ahead print].
Knudsen, J. T., Tollsten, L., and Bergström, L. G. (1993). Floral scents - a checklist of volatile compounds isolated by head-space techniques. Phytochemistry 33, 253–280. doi: 10.1016/0031-9422(93)85502-I
Köllner, T. G., Held, M., Lenk, C., Hiltpold, I., Turlings, T. C. J., Gershenzon, J., et al. (2008). A maize (E)-β-caryophyllene synthase implicated in indirect defense responses against herbivores is not expressed in most American maize varieties. Plant Cell 20, 482–494. doi: 10.1105/tpc.107.051672
Lorenz, K. H., Schneider, B., Ahrens, U., and Seemüller, E. (1995). Detection of the apple proliferation and pear decline phytoplasmas by PCR amplification of ribosomal and nonribosomal DNA. Phytopathology 85, 771–776. doi: 10.1094/Phyto-85-771
Lubanga, U. K., Guédot, C., Percy, D. M., and Steinbauer, M. J. (2014). Semiochemical and vibrational cues and signals mediating mate finding and courtship in psylloidea (Hemiptera): a synthesis. Insects 5, 577–595. doi: 10.3390/insects5030577
Maclean, A. M., Orlovskis, Z., Kowitwanich, K., Zdziarska, A. M., Angenent, G. C., Immink, R. G., et al. (2014). Phytoplasma effector SAP54 hijacks plant reproduction by degrading MADS-box proteins and promotes insect colonization in a RAD23-dependent manner. PLoS Biol. 12:e1001835. doi: 10.1371/journal.pbio.1001835
Mann, R. S., Ali, J. G., Hermann, S. L., Tiwari, S., Pelz-Stelinski, K. S., Alborn, H. T., et al. (2012). Induced release of a plant-defense volatile “deceptively” attracts insect vectors to plants infected with a bacterial pathogen. PLoS Pathog. 8:e1002610. doi: 10.1371/journal.ppat.1002610
Martini, X., Kuhns, E. H., Hoyte, A., and Stelinski, L. L. (2014a). Plant volatiles and density-dependent conspecific female odors are used by Asian citrus psyllid to evaluate host suitability on a spatial scale. Arth. Plant Interact. 8, 453–460. doi: 10.1007/s11829-014-9326-z
Martini, X., Pelz-Stelinski, K. S., and Stelinski, L. L. (2014b). Plant pathogen-induced volatiles attract parasitoids to increase parasitism of an insect vector. Front. Ecol. Evol. 2:8. doi: 10.3389/fevo.2014.00008
Mas, F., Vereijssen, J., and Suckling, D. M. (2014). Influence of the pathogen Candidatus liberibacter solanacearum on tomato host plant volatiles and psyllid vector settlement. J. Chem. Ecol. 40, 1197–1202. doi: 10.1007/s10886-014-0518-x
Mayer, C. J., Jarausch, B., Jarausch, W., Jelkmann, W., Vilcinskas, A., and Gross, J. (2009). Cacopsylla melanoneura has no relevance as vector of apple proliferation in Germany. Phytopathology 99, 729–738. doi: 10.1094/PHYTO-99-6-0729
Mayer, C. J., Vilcinskas, A., and Gross, J. (2008a). Pathogen-induced release of plant allomone manipulates vector insect behavior. J. Chem. Ecol. 34, 1518–1522. doi: 10.1007/s10886-008-9564-6
Mayer, C. J., Vilcinskas, A., and Gross, J. (2008b). Phytopathogen lures its insect vector by altering host plant odor. J. Chem. Ecol. 34, 1045–1049. doi: 10.1007/s10886-008-9516-1
Mayer, C. J., Vilcinskas, A., and Gross, J. (2011). Chemically mediated multitrophic interactions in a plant–insect vector−phytoplasma system compared with a partially nonvector species. Agric. Forest Entomol. 13, 25–35. doi: 10.1111/j.1461-9563.2010.00495.x
Meier, U. (1997). BBCH-Monograph. Growth Stages of Plants–Entwicklungsstadien Von Pflanzen–Estadios de las plantas–Développement des Plantes. Berlin und Wien: Blackwell Wissenschaftsverlag, 622.
Mikona, C., and Jelkmann, W. (2010). Replication of grapevine leafroll-associated virus-7 (GLRaV-7) by Cuscuta species and its transmission to herbaceous plants. Plant Dis. 94, 471–476. doi: 10.1094/PDIS-94-4-0471
Orlova, I., Nagegowda, D. A., Kish, C. M., Gutensohn, M., Maeda, H., Varbanova, M., et al. (2009). The small subunit of snapdragon geranyl diphosphate synthase modifies the chain length specificity of tobacco geranylgeranyl diphosphate synthase in planta. Plant Cell 21, 4002–4017. doi: 10.1105/tpc.109.071282
Orlovskis, Z., Canale, M. C., Thole, V., Pecher, P., Lopes, J. R., and Hogenhout, S. A. (2015). Insect-borne plant pathogenic bacteria: getting a ride goes beyond physical contact. Curr. Opin. Insect Sci. 9, 16–23. doi: 10.1016/j.cois.2015.04.007
Patui, S., Bertolini, A., Clincon, L., Ermacora, P., Braidot, E., Vianello, A., et al. (2013). Involvement of plasma membrane peroxidases and oxylipin pathway in the recovery from phytoplasma disease in apple (Malus domestica). Physiol. Plant. 148, 200–213. doi: 10.1111/j.1399-3054.2012.01708.x
Rasmann, S., Köllner, T. G., Degenhardt, J., Hiltpold, I., Toepfer, S., Kuhlmann, U., et al. (2005). Recruitment of entomopathogenic nematodes by insect-damaged maize roots. Nature 434, 732–737. doi: 10.1038/nature03451
Scala, A., Allmann, S., Mirabella, R., Haring, M. A., and Schuurink, R. C. (2013). Green leaf volatiles: a plant's multifunctional weapon against herbivores and pathogens. Int. J. Mol. Sci. 14, 17781–17811. doi: 10.3390/ijms140917781
Seemüller, E., Garnier, M., and Schneider, B. (2002). “Mycoplasmas of plants and insects,” in Molecular Biology and Pathogenicity of Mycoplasmas, eds S. Razin and R. Herrmann (New York, NY: Kluwer Academic/Plenum Publishers), 91–116.
Seemüller, E., Schaper, U., and Zimbelmann, F. (1984). Seasonal variation in the colonization patterns of mycoplasmalike organisms associated with apple proliferation and pear decline. Z. Pflanzenkrankh. Pflanzenschutz 91, 371–382.
Seemüller, E., and Schneider, B. (2007). Differences in virulence and genomic features of strains of ‘Candidatus Phytoplasma mali’, the apple proliferation agent. Phytopathology 97, 964–970. doi: 10.1094/PHYTO-97-8-0964
Sharon-Asa, L., Shalit, M., Frydman, A., Bar, E., Holland, D., Or, E., et al. (2003). Citrus fruit flavor and aroma biosynthesis: isolation, functional characterization, and developmental regulation of Cstps1, a key gene in the production of the sesquiterpene aroma compound valencene. Plant J. 36, 664–674. doi: 10.1046/j.1365-313X.2003.01910.x
Smart, C. D., Schneider, B., Blomquist, C. L., Guerra, L. J., Harrison, N. A., Ahrens, U., et al. (1996). Phytoplasma-specific PCR primers based on sequences of the 16S-23S rRNA spacer region. Appl. Environ. Microbiol. 62, 2988–2993.
Soroker, V., Talebaev, S., Harari, A. R., and Wesley, S. D. (2004). The role of chemical cues in host and mate location in the pear psylla Cacopsylla bidens (Homoptera: Psyllidae). J. Insect Behav. 17, 613–626. doi: 10.1023/B:JOIR.0000042544.35561.1c
Sugio, A., and Hogenhout, S. A. (2012). The genome biology of phytoplasma: modulators of plants and insects. Curr. Opin. Microbiol. 15, 247–254. doi: 10.1016/j.mib.2012.04.002
Sugio, A., Maclean, A. M., Grieve, V. M., and Hogenhout, S. A. (2011a). Phytoplasma protein effector SAP11 enhances insect vector reproduction by manipulating plant development and defense hormone biosynthesis. Proc. Natl. Acad. Sci. U.S.A. 108, 1254–1263. doi: 10.1073/pnas.1105664108
Sugio, A., Maclean, A. M., Kingdom, H. N., Grieve, V. M., Manimekalai, R., and Hogenhout, S. A. (2011b). Diverse targets of phytoplasma effectors: from plant development to defense against insects. Annu. Rev. Phytopathol. 49, 175–195. doi: 10.1146/annurev-phyto-072910-095323
Takabayashi, J., Dicke, M., and Posthumus, M. A. (1991). Variation in composition of predator-attracting allelochemicals emitted by herbivore-infested plants: relative influence of plant and herbivore. Chemoecology 2, 1–6. doi: 10.1007/BF01240659
Van Poecke, R. M. P., Posthumus, M. A., and Dicke, M. (2001). Herbivore-induced volatile production by Arabidopsis thaliana leads to attraction of the parasitoid Cotesia rubecula: chemical, behavioral, and gene-expression analysis. J. Chem. Ecol. 27, 1911–1928. doi: 10.1023/A:1012213116515
Wang, G., Tian, L., Aziz, N., Broun, P., Dai, X., He, J., et al. (2008). Terpene biosynthesis in glandular trichomes of hop. Plant Physiol. 148, 1254–1266. doi: 10.1104/pp.108.125187
Weintraub, P. G., and Beanland, L. (2006). Insect vectors of phytoplasmas. Annu. Rev. Entomol. 51, 91–111. doi: 10.1146/annurev.ento.51.110104.151039
Weintraub, P. G., and Gross, J. (2013). “Capturing insect vectors of phytoplasmas,” in Phytoplasma: Methods and Protocols, eds M. J. Dickinson and J. Hodgetts (New York, NY: Springer Science+Business Media), 61–72.
Keywords: Candidatus Phytoplasma mali, Candidatus Phytoplasma pyri, quantitative headspace sampling device, Nicotiana occidentalis, VOC, Malus spp., Pyrus spp., multitrophic interactions
Citation: Rid M, Mesca C, Ayasse M and Gross J (2016) Apple Proliferation Phytoplasma Influences the Pattern of Plant Volatiles Emitted Depending on Pathogen Virulence. Front. Ecol. Evol. 3:152. doi: 10.3389/fevo.2015.00152
Received: 23 October 2015; Accepted: 15 December 2015;
Published: 07 January 2016.
Edited by:
Lukasz Lech Stelinski, University of Florida, USAReviewed by:
Cesar Rodriguez-Saona, Rutgers University, USAZsofia Szendrei, Michigan State University, USA
Copyright © 2016 Rid, Mesca, Ayasse and Gross. This is an open-access article distributed under the terms of the Creative Commons Attribution License (CC BY). The use, distribution or reproduction in other forums is permitted, provided the original author(s) or licensor are credited and that the original publication in this journal is cited, in accordance with accepted academic practice. No use, distribution or reproduction is permitted which does not comply with these terms.
*Correspondence: Jürgen Gross, anVlcmdlbi5ncm9zc0Bqa2kuYnVuZC5kZQ==