- Urban Forest Research and Ecological Disturbance (UFRED) Group, Geography and Environmental Studies, Ryerson University, Toronto, ON, Canada
Management decisions grounded in ecological understanding are essential to the maintenance of a healthy urban forest. Decisions about where and what tree species to plant have both short and long-term consequences for the future function and resilience of city trees. Through the construction of a theoretical damage index, this study examines the legacy effects of a street tree planting program in a densely populated North American city confronting an invasion of emerald ash borer (Agrilus planipennis). An investigation of spatial autocorrelation for locations of high damage potential across the City of Toronto, Canada was then conducted using Getis-Ord Gi*. Significant spatial clustering of high damage index values affirmed that past urban tree planting practices placing little emphasis on species diversity have created time-lagged consequences of enhanced vulnerability of trees to insect pests. Such consequences are observed at the geographically local scale, but can easily cascade to become multi-scalar in their spatial extent. The theoretical damage potential index developed in this study provides a framework for contextualizing historical urban tree planting decisions where analysis of damage index values for Toronto reinforces the importance of urban forest management that prioritizes proactive tree planting strategies that consider species diversity in the context of planting location.
Introduction
Widely recognized for providing important ecological, economic, and social benefits to city residents (Dwyer et al., 1992; McPherson, 1992), urban tree cover is a living resource and therefore inherently dynamic (Nowak and Greenfield, 2012). Beyond the loss of city trees from new development and urban densification (Sanders, 1984; Nowak and Greenfield, 2012), significant loss of tree cover also results from natural events such as storms (Sisinni et al., 1995; Burley et al., 2008; Wiersma et al., 2012) or sustained conditions of drought (Nowak, 1993). The urban forest is also susceptible to unexpected tree loss from diseases and pests that can affect a specific genus or target an individual species (Raupp et al., 2006). With an increasing number of large North American cities like New York and Los Angeles investing in planting programs to protect and enhance their urban forests (City Plants, 2015; Million Trees NYC, 2015), it is important to investigate how city tree management choices driven by short-term considerations (e.g., financial constraints, nursery stock availability, aesthetics) can result in delayed and unexpected consequences magnifying the susceptibility of the urban forest to canopy decline and tree mortality, thus limiting the overall resilience of the urban forest. This study considers an example of these consequences that are, in part, a legacy of past planting decisions concerning the street tree resource in Toronto, Canada, currently facing sudden and rapid canopy loss resulting from Emerald Ash Borer or “EAB” (Agrilus planipennis), an invasive, phytophagous, insect pest.
As the vast majority of North American street trees are not naturally occurring, the structure of a city's street tree resource is in part resultant from species selection and the planting/replanting rate (stocking rate); both of these choices can have short- and long-term consequences for the urban forest resource and ultimately the resource's potential to deliver desirable benefits. More challenging urban growing conditions narrow the selection of species to those more tolerant of urban stresses (Richards, 1983; Whitlow and Bassuk, 1988). Selecting a tree species less capable of withstanding harsh urban growing conditions increases its probability of mortality, and reduces the average longevity of that species subject to these conditions (Foster and Blaine, 1978; Sanders, 1981; Richards, 1983). Subsurface infrastructure and overhead conflicts (e.g., electrical wires) as well as proximity to impervious surfaces (e.g., sidewalks and roads) results in chronically inadequate soil quantity and quality, which directly inhibit and prohibit root development and mainstem elongation (Bassuk and Whitlow, 1988; Lindsey and Bassuk, 1992). Avoidance of high rates of street tree mortality (Foster and Blaine, 1978; Lu et al., 2010) and stultified tree growth (Close et al., 1996; Quigley, 2004; Smiley et al., 2006) are practical and attractive reasons for selecting fast growing tree species that are also more tolerant of urban conditions. Such decision making serves to improve the short-term efficiency of urban tree planting programs.
There are, however, longer-term consequences of a narrowed street tree species selection, some of which can be magnified based upon decisions related to spatial planting/growing proximity. Preferentially planting many individuals of a single species decreases species diversity and may extend to reduced genetic diversity if very few cultivars of the species are planted. This reduced species and genetic diversity potentially creates conditions for increased susceptibility of city trees to pests or disease (Sanders, 1981; Alvey, 2006; Laćan and McBride, 2008). The plight of the American elm (Ulmus americana) provides a good cautionary example of not prioritizing tree species diversity in urban settings. Planted in large numbers along streets in many North American cities due to its fast growth, appearance, and tolerance of a wide range of conditions (Raupp et al., 2006), populations of American Elm have been catastrophically reduced by Dutch elm disease (or “DED”) (Ophiostoma spp.) both in the United States and Canada. Rather than replace this species-specific loss with greater tree diversity, city planting selections have regularly been limited to a small selection of tree species.
Another genus of tree species often found with elevated frequencies within North American cities is ash (Fraxinus spp.). Prior to being a common choice for replacement of American elms lost to DED (Poland and McCullough, 2006) ash had become a common shade tree due to the “Marshall Seedless” cultivar of the green ash (Fraxinus pennsylvanica) introduced in the early 1940s (MacFarlane and Meyer, 2005), reducing the genetic diversity of this particular ash species in some urban locales. The resulting popularity and increased spatial concentration of ash in some cities has recently resulted in a new problem not anticipated at the time of planting—susceptibility to EAB. Originally identified in Detroit, Michigan in 2002 (Cappaert et al., 2005; MacFarlane and Meyer, 2005), this insect native to Asia has spread from Detroit to 22 US states (Siegert et al., 2010) and two Canadian provinces (Poland and McCullough, 2006; Siegert et al., 2010). EAB is considered to be a significant threat to the urban forest for a number of reasons. While ash species are considered to be more resistant to the pressures of the urban environment than many broadleaf tree species, they still experience exposure to urban stressors and their growing environment is similarly constrained when compared with other city tree species.
Despite their tolerance of harsh conditions, there are still many individual ash trees whose vigor is compromised by chronic exposure to urban stressors. Several recent studies have demonstrated that stressed ash trees tend to be preferentially colonized by EAB (McCullough et al., 2009; Siegert et al., 2010). Moreover, low genetic diversity among urban ash tree plantings and the lack of a co-evolutionary pathway to develop some resistance to EAB (i.e., EAB is non-native) means even healthy trees are at risk of EAB infestation (Sydnor et al., 2007; Siegert et al., 2010). Once infested with EAB, the death of a tree occurs within 3–4 years for a large ash tree (Poland and McCullough, 2006). With the potential to migrate up to 20 km per year (Prasad et al., 2010), when established in the urban forest of a city, EAB can disperse very quickly and has the potential to extirpate ash trees throughout the tree canopy. In many eastern North American cities, extirpation of the genus Fraxinus from the urban tree canopy seems most likely given the mixed success of containment strategies applied in locations where EAB infestations have been identified (Herms and McCullough, 2014). It should be noted that the threat of EAB is not restricted to urban settings, and has been identified in both managed and natural forest systems beyond the cities in which the original EAB infestation has been identified (Hodge et al., 2015). Recent aerial surveys by the Ontario Ministry of Natural Resources identified a cumulative area of 63,421 ha in southern Ontario exhibiting severe decline or mortality related to EAB infestation (Scarr et al., 2012). Sudden canopy gaps resulting from rapid decline and mortality are expected to have a number of consequences for these forest systems, including shifting species composition and local impacts on biogeochemical cycling (Kenis et al., 2009; Gandhi and Herms, 2010).
The study area for this research is the City of Toronto, Ontario, Canada. With a population of 2.8 million people (most recent Canadian census), Toronto is now the fourth largest city in North America by population (O'Toole, 2013). The city's current political boundaries are the result of the amalgamation of six contiguous municipalities, which occurred in 1998 (Schwartz, 2004). Pre-amalgamation, these independent municipalities had their own planning departments, each of which had its own objectives and priorities that have resulted in considerable spatial heterogeneity of urban form. Among the variability in planning priorities was the provision and maintenance of green space and, immediately relevant to this research, planning and investment in street tree programs. It is reasonable to infer that each of these former Toronto municipalities shows some variability in how reliant street tree programs have been on ash (Fraxinus spp.) within their past planting selections. While ash is commonly encountered in every city ward across amalgamated Toronto (see Figure 1), there does appear to be areas of higher ash concentrations (spatial clustering), particularly in Scarborough and Old Toronto.
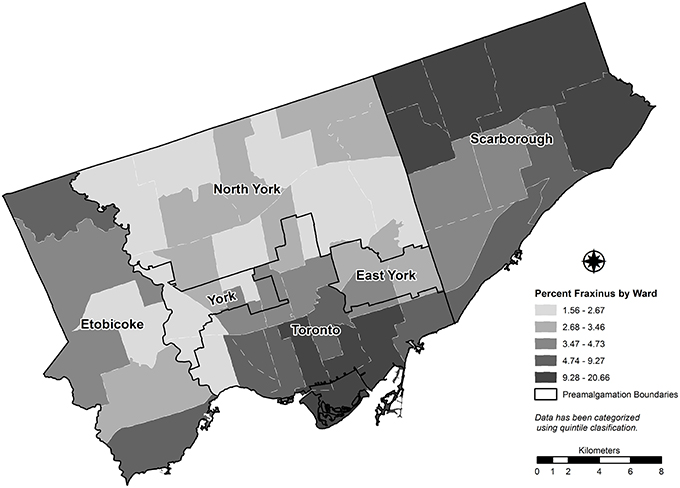
Figure 1. Proportion of street tree stems that are species of the genus Fraxinus by city ward for Toronto, Canada (2012).
Toronto was chosen as the context for this study for a number of reasons. With 10.2 million trees and a structural value of $7.1 billion (Nowak et al., 2013), Toronto's urban forest is comparable to that of other large North American cities such as New York (5.2 million trees, structural value of $5.2 billion; see Nowak et al., 2007) and Los Angeles (6 million trees, structural value of $12.4 billion; see Nowak et al., 2011). Furthermore, though Toronto's street tree population contains a considerable number of species, there is a strong dominance of a small subset, most of which have been selected based upon short-term economic considerations (i.e., fast growing, tolerant of urban conditions). Of the 530,190 street trees inventoried in Toronto, 21.1% are a cultivar of Norway maple (Acer platenoides); 7.2% are Gleditsia spp. (e.g., Gleditsia triacanthos or honey locust); and, 5.2% are ash (Fraxinus spp.) With three tree genera accounting for 33.5% of the street tree population by stem count, and comprising an even greater proportion by leaf area (City of Toronto Parks Forestry and Recreation, Urban Forestry, 2012), Toronto's municipal forest is especially vulnerable to threats in the form of insect pests and pathogens.
Empirically, the study location offers the opportunity to examine the consequences of past tree planting decisions across a large urban forest with an established EAB infestation. At the time of this writing, high levels of ash decline and mortality from EAB are observable within Toronto's street tree population. While Toronto is attempting to delay infestation in as many as 13,000 trees (mostly along streets and in parks) through injection of insecticide in “high value trees” (Patterson, 2011), recent estimates indicate that all ash trees (an estimated total of 860,000 individuals across all land cover categories) could be lost, representing just over 2 percentage points of Toronto's current cumulative urban forest leaf area, or the equivalent of $570 million in structural value (City of Toronto Parks Forestry and Recreation, Urban Forestry, 2013). This loss of canopy is expected to coincide with the early stages of Toronto's renewed commitment to expanding tree canopy (from ~ 27% at present to 40% over 3–5 decades) (City of Toronto Parks Forestry and Recreation, Urban Forestry, 2013).
While the impact of EAB on Toronto's urban forest cover, and associated lost benefits, has been quantified from an aspatial perspective (i.e., structurally and monetarily), there is considerable value in identifying and describing how this urban forest is likely to be impacted spatially by EAB. Moreover, the relationship between diversity and the resilience of managed ecosystems has received limited attention in the literature. In this study, a damage potential index was constructed to provide a spatially explicit framework to evaluate the consequences of past tree planting decisions, particularly the ecological, economic, and social trade-offs that result from those original management considerations. By understanding the time-lagged consequences of past planting decisions, and how they manifest across urban space, important input to proactive approaches of future urban tree planting decisions is possible. Constructing a theoretical metric to identify high-risk locations for EAB infestation and tree mortality can contribute to developing strategic tree planting objectives, including where to prioritize ash replacement. Ideally, a theoretical damage potential metric would be developed from a complete inventory of trees that includes those growing on public and private land. While Toronto, similar to most cities, does not have a full inventory of all of its trees, it does have a complete inventory of street trees. This inventory provides an opportunity to construct a preliminary model to identify, on a local scale, where the street tree resource is likely to be damaged most by EAB. While derived from a street tree inventory, the methodology developed in this study allows for the subsequent inclusion of additional tree datasets, allowing for a more comprehensive understanding of the threat posed by EAB. Finally, this research documents an approach that is transferrable to other urban geographies and to other tree genera that may be vulnerable to current or future threats posed by insects or disease.
Materials and Methods
Datasets
At the time of analyses, current cadastral data for the City of Toronto was not publically available as a complete dataset. A property boundaries feature dataset containing 476,904 individual parcels was created by Ryerson University's Urban Forest Research & Ecological Disturbance (UFRED) Group by merging 922 AutoCAD tiles originally developed for the purpose of emergency service response. An examination of this dataset revealed that the size distribution of city lots varied from a minimum of less than 1 m2 to a maximum of 2.03 km2, with a mean of 1086 m2 and a standard deviation of 10,780 m2. The Toronto street tree inventory was obtained through the Open Toronto portal (City of Toronto Parks Forestry and Recreation, Urban Forestry, 2012). A total of 530,190 trees composed of approximately 185 species in 65 genera were represented by points and georeferenced to parcel centers rather than at discrete coordinates. The following attributes were available for each tree: property address, common name, scientific name, diameter at breast height, and a position code to differentiate multiple trees present on a lot. Street centerlines were obtained from DMTI Spatial Inc. Cartographic boundaries for the 531 census tracts (or “CT”) for the 2006 Census were obtained from Statistics Canada. The size distribution of the CT boundaries range from a minimum area of 13,167 m2 to a maximum area of 29.02 km2, with a mean of 1.19 km2 and standard deviation of 1.71 km2.
Damage Potential Index
In order to produce a spatial distribution of the theoretical damage index, the data were aggregated to a common zonation or analytical frame. Translating the data to a common analytical frame allowed for the standardization of data from spatially implicit forms to spatially explicit forms, which permits better comparison between and among locations. Although, an argument could be made for an analysis that used an arbitrary grid with quadrants of equal size, most planning and policy decisions target only quasi-regular shaped geographic areas that are based on socio-demographic or political boundaries such as dissemination areas, CTs, neighborhoods, and wards. Furthermore, the impact on the street tree resource may disproportionately affect some groups of residents over others (e.g., by income or ethnicity). As a result, CTs were chosen as the common spatial frame for analysis to allow comparability between locations throughout the city, while at the same time maintaining the ability to conduct future analysis that includes socio-demographic variables.
EAB damage to the street tree resource in any CT is expected to be a function of several variables. The first is the frequency of individual trees belonging to the genus Fraxinus as a function of the total number of street trees (relative abundance) (Figure 2). The greater the proportion of tree canopy area contributed by ash trees, the higher the aggregate or “global” damage to the tree canopy is expected within a CT. In this context “global” is intended to contribute to understanding the potential overall magnitude of the damage, but provides little insight concerning the localized (street level) effects to tree canopy (e.g., the loss of clusters of ash individuals opening large gaps in the tree canopy). It is important to note that a measure of ash leaf area represents a superior choice in calculating the proposed index. These data, however, were not available for the target study area and stem density was necessary to apply as a proxy measure to represent the frequency of ash species.
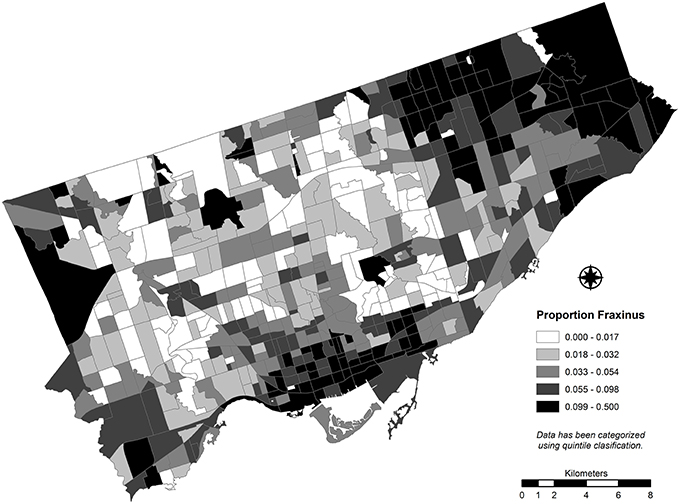
Figure 2. The proportion of street trees that belong to the Fraxinus genus calculated for each census tract for Toronto, Canada (2012).
Similarly, the magnitude of potential EAB damage is also a function of an interaction of both the overall street tree density (Figure 3) and the relative proportion of ash leaf area making up that street tree canopy within a CT. Where there is a high-density of street tree canopy and a large proportion of ash trees contributing to that canopy, there will be a greater magnitude of canopy loss. Therefore, the count of street trees within a CT is not adequate alone for comparison between CTs due to the irregular sizes and shapes of tracts. Therefore, a density of street trees was calculated to facilitate this comparison. If a complete inventory of all trees was available, a simple density of trees by unit area would be adequate. In contrast, trees in this dataset are linked directly to the street network within each CT and, therefore, a different standardization process is necessary. For each CT, the number of street trees per 1000 m of street length was calculated. Primary roadways were excluded from the standardization process as trees growing along major expressways and their associated access ramps are uncommon in Toronto.
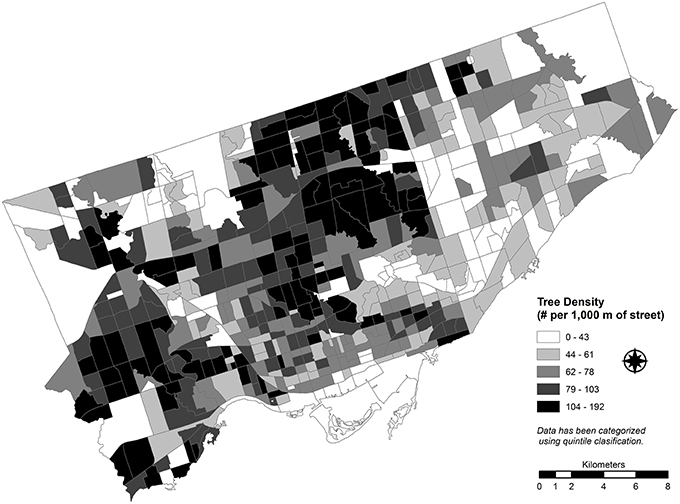
Figure 3. Street tree stem density standardized as a function of the street network density and calculated for each census tract for Toronto, Canada (2012).
Finally, the potential for additional localized damage due to the planting arrangement of street trees must be considered. If there are clusters of ash trees in close proximity, mortality of those trees from EAB infestation may result in significant holes appearing in the tree canopy. If the same number of trees are planted at larger distances between individuals with other tree species interspersed between ash plantings, the extent of gaps in the tree canopy is expected to be less extensive. Because discrete coordinate locations were not available, lot boundaries were used as a proxy to calculate the mean distance between ash trees for each CT (Figure 4). As mean distance increases (i.e., mean proximity decreases), localized damage is expected to decrease as the affected ash individuals are more dispersed. As mean distance decreases (i.e., mean proximity increases), individuals in the CT are more likely to be grouped more closely and the potential for localized damage increases.
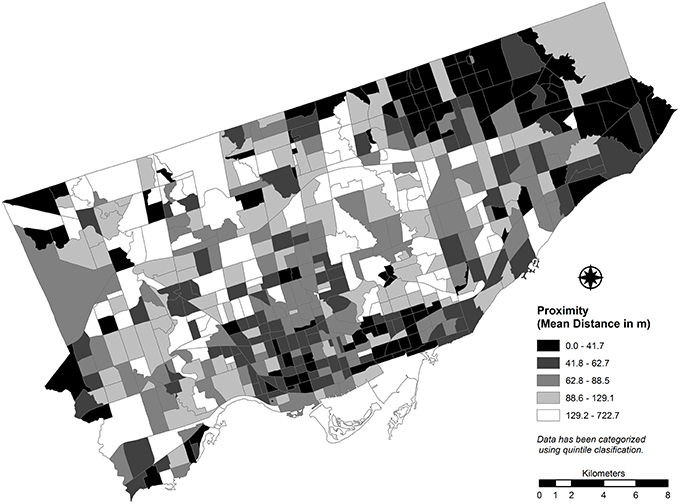
Figure 4. Average proximity of street trees that are ash species calculated for each census tract for Toronto, Canada (2012).
Therefore, the potential damage in a CT is a function of the interaction of the spatial density of street trees and the relative proportion of ash trees, which in turn is augmented by the mean distance between those individuals. This damage potential index (DPI) is operationalized in the following equation:
Where:
The numerator denotes an estimation of global damage; as the value of the numerator increases the potential for overall damage to the municipal forest increases. The denominator represents a modifier for localized structural damage and represents an inverse relationship. Holding the numerator constant (e.g., two CTs with the same street tree density and relative proportion of ash), as the mean distance between ash trees becomes larger there is a reduction in the calculated DPI. As the mean distance between ash trees becomes smaller, there is an increase in the calculated DPI. Examining the input variable ranges for maximum and minimum values, the theoretical maximum DPI value possible for this distribution is 19.2 based on the observed spatial density maximum of 192.2, the relative proportion of ash species maximum of 0.5, and the minimum mean distance between individual ash species of 5 m.
Descriptive statistics for the DPI output revealed a distribution of index values with extreme skew (skewness = +4.103). Consequently the DPI was re-expressed through a log base 10 transformation (assume base 10 for any subsequent reference to log) to better approximate normality and to meet the assumptions necessary for application of spatial statistics. The spatial distribution of re-expressed DPI values was then evaluated for clustering of extreme values using a local indicator of spatial autocorrelation or LISA (Getis and Ord, 1992; Anselin, 1995). For this analysis the Getis-Ord Gi* statistic was chosen because of its ability to detect spatial clusters representing locations that deviate from the overall variable average. These clusters are commonly referred to as significant “hotspots” (i.e., similar high values above the distribution mean) and “cold spots” (i.e., very low values below the distribution mean) in the presence of global spatial autocorrelation (Ord and Getis, 1995, 2001). The identification of significant clusters of high magnitude DPI values expands the spatial extent of investigation beyond individual CTs to identify broader, more regional geographic areas containing ash trees that are likely to become infested with EAB. The presence of these clusters may indicate a multi-scalar impact of local planting decisions; in other words, choices made at the local scale results in local vulnerability to EAB, but may propagate and result in vulnerability at a wider geographic scale.
Results
Prior to transformation, DPI values calculated for study area CTs range from 0 to 1.85 (see Figure 5), with higher values indicating higher damage potential due to EAB. The spatial distribution of log-transformed DPI values by CT is presented in Figure 6. Though the spatial distributions of the original values and log transformed values are similar, the scale of the transformed variable is more difficult to interpret. Because many observed DPI values are positive but less than 1, a log function results in a negative value as an output. Though this outcome is not expected to appreciably affect the results of the LISA cluster analysis, the interpretability of this re-expression should be made carefully when applied in a policy setting.
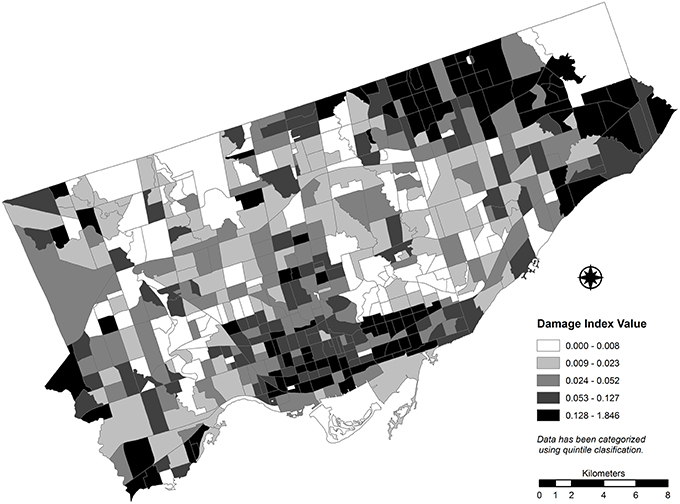
Figure 5. Spatial distribution of theoretical damage potential index values calculated for census tracts. Increasing damage to the street tree resource is expected as index values increase.
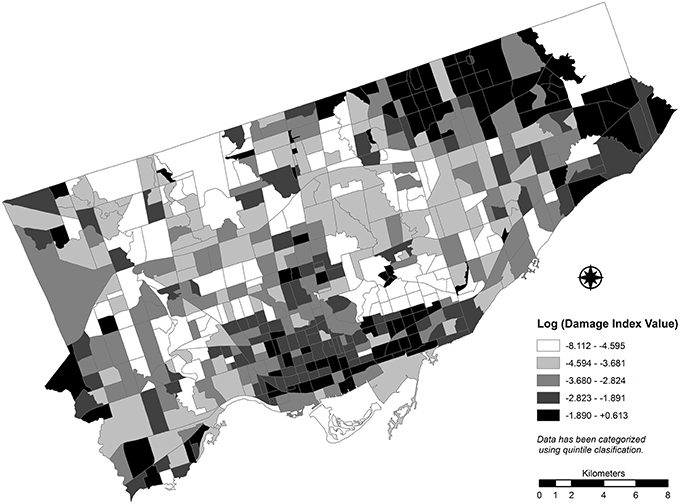
Figure 6. Spatial distribution of log-transformed theoretical damage potential index values calculated for census tracts for Toronto, Canada (2012). Increased damage to the street tree resource is expected as absolute values of the DPI increase.
The LISA cluster analysis identified two clusters of significantly high index values (see Figure 7). The first cluster is located in the southern portion of the study area, which is within the pre-amalgamation municipality of Old Toronto (containing the downtown core and central business district of present day amalgamated Toronto). These CTs exhibit higher proportions of ash trees along their streets in addition to having a higher geographic proximity between ash individuals (i.e., a lower mean distance to neighboring ash trees). Overall, the street tree density within these CTs is moderate, many falling within the second and third categorical quartiles of the distribution.
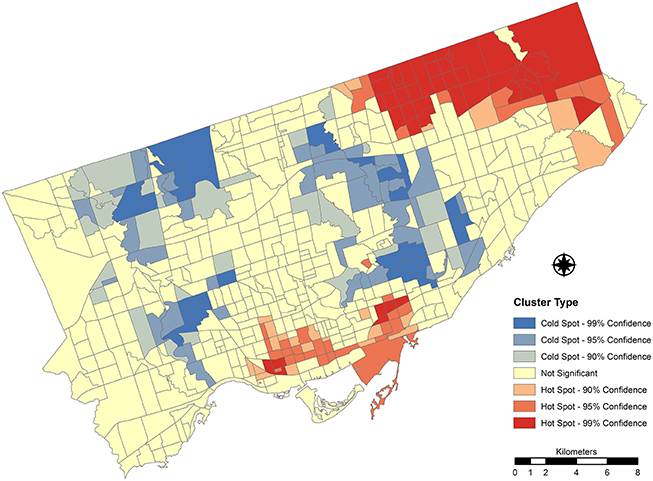
Figure 7. Getis-Ord local Gi* cluster analysis of the log transformed theoretical damage potential index values calculated for each census tract for Toronto, Canada (2012). Hotspots and cold spots represent clusters of transformed index values significantly higher and lower than the distribution mean.
The second notable cluster of elevated DPI values is located in the northeastern portion of the study area and is contained within the pre-amalgamation municipality of Scarborough. This large contiguous group of CTs exhibits similar street tree characteristics to the previously discussed cluster observed in the downtown core—greater proportions of ash trees as well as closer mean spatial proximity values between individuals.
Discussion
Results of this analysis indicate that past management decisions can have an important influence on the multi-scale vulnerability of city trees, in this case susceptibility to an invasive insect pest. In addition to individual CTs being more vulnerable to potential EAB damage, the presence of broader clusters of adjacent (neighboring) CTs with higher DPI values suggests past planting patterns have inadvertently left much larger geographic coverages of street trees at risk to canopy damage. Even with the assumption that all ash trees will be lost to EAB, if the same number of individual trees were more dispersed (i.e., greater distances between individuals), localized structural damage to the canopy is likely to be lessened. More specifically, the gaps in the canopy from lost ash trees are expected to be larger when individual trees are planted in close proximity than if the same number of individuals were separated by several individuals of other species with no vulnerability to the EAB threat (Gandhi and Herms, 2010). The importance of increased canopy density in providing improved ecosystem service delivery such as summer temperature moderation (Greene, 2015) and air filtration (Givoni, 1991) has been demonstrated and it is reasonable to expect other ecosystem services may exhibit a similar positive relationship with canopy density.
Furthermore, there are social and economic losses related to urban tree decline and mortality. For some low-income city dwellers, public trees (i.e., street and park trees) represent their primary access to nature and the plethora of benefits associated with such access; many of these residents do not have the resources to purchase and maintain their own private trees (Heynen et al., 2006). Findings from the present study confirm that the amalgamated City of Toronto will be disproportionately affected by the damage to city trees caused by EAB. If these areas of high potential damage coincide with areas more reliant on street trees for access to greenspace, social issues related to distributional inequality are likely to result (Pham et al., 2012; Greene, 2015). However, even if very limited distributional inequality of access emerges as a result of ash tree mortality, if dying ash trees lost to the EAB are not replaced, the benefits once delivered by said trees will be lost to the affected neighborhood and to the city as a whole. Considerable public funds are invested in street tree planting and maintenance; where EAB-caused tree mortality is prevalent this investment represents, to some extent, sunk costs. However, it can also be argued that those sunk costs may not be as high as the original investment if the cumulative historical benefits provided by dead and dying trees are considered. Prior to succumbing to EAB it may be reasonably argued that enough ecological, economic, and social benefit was delivered by these trees in aggregate accrued that, in part, offset the initial investment and maintenance costs associated with the historical choice to plant ash trees.
In Toronto, a specific commitment has been made to replace lost ash trees as a part of the strategic plan to expand the urban tree canopy from approximately 27% at present to 40% over 4–5 decades (City of Toronto Parks Forestry and Recreation, Urban Forestry, 2012). This commitment to canopy expansion, in the face of a relatively sudden 2% point loss of tree canopy by leaf area resulting from EAB mortality, most certainly will slow the rate of tree canopy expansion over the short term. Though Toronto is expecting the tree loss resulting from EAB will begin to subside by 2017 (Doyle, 2015), planned plantings of 105,000 trees in 2015 and 2016, and an additional 110,587 in 2107 will not adequately compensate for the canopy loss occurring over that same time period. Moreover, if priority is given to mitigating this EAB caused tree loss, other planting priorities may be compromised due to opportunity costs. Resources dedicated to managing EAB infestations, and replacing lost ash trees, are resources that cannot be committed to other planting objectives such as decreasing the polarization of access to tree canopy or maximizing the efficiency of important ecosystem services.
Understanding the trade-offs resulting from past planting decisions is critical to informing future management practices, specifically adapting methods toward more proactive tree planting and maintenance efforts in Toronto as well as in other North American cities. Replacing ash trees that are casualties of EAB with a single species, or even a single genus, has the potential to result in new and, yet unknown urban forest vulnerabilities. Ensuring diversity beyond species richness to include considerations of evenness and genetic diversity is one of the most important decisions that can serve to buffer against future urban forest vulnerability to insect pests or disease. Nevertheless, the reason that large aggregations of CTs are susceptible to higher magnitude damage from EAB remains connected to the difficulty associated with establishing alternate tree species that are tolerant of harsh urban growing conditions. Therefore, achieving a more resilient urban forest canopy requires not only planting proactively from the perspective of species diversity to avoid potential crises similar to EAB, but is also dependent upon the will to improve difficult growing conditions for city trees so as to increase the probability of survival and to extend average longevity for a wider range of tree species (e.g., shared rooting zones or engineered solutions such as suspended pavement designs). Assuming a fixed budget for urban forest management that includes both planting and maintenance activities, a shift from more reactive planting (i.e., replacing dead trees with new ones in the same growing conditions and hoping for a different result) to proactive planting (i.e., improving the survival of young trees and focusing on approaches to enhance their longevity) implies less trees are likely to be planted per year in lieu of improving subsurface soil conditions and improved maintenance of existing trees.
A shift toward more proactive planting is complicated by the political nature of tree planting and the power relationships that exist between established municipal departments and elected officials. Tree planting is a highly visible activity and the number of trees planted in a year is a metric easily interpretable by the electorate. During the most recent municipal election in Toronto the new mayor made a campaign pledge to plant 3.8 million trees by 2025 (Dale, 2014). It is uncertain whether this commitment was made due to a belief that the benefits provided by the urban tree canopy are a critical natural capital resource or whether this commitment was to portray a well-defined contrasting vision to his predecessor who viewed tree planting and maintenance as an unnecessary expenditure of public tax dollars. Though recently amended, the Urban Forestry Financial Plan with projected plantings totaling 1,066,787 trees by 2023 (Doyle, 2015) has not been modified to the extent that the mayor's campaign pledge can reasonably be met. It is foreseeable that a recommendation by urban forestry managers to spend more per tree in order to improve probability of survival and longevity of those trees is likely to be politically unfavorable and be difficult to adopt once presented to city council.
In addition to providing a framework for considering the consequences of past planting decisions and informing future planting efforts for street tree resources, the DPI developed in this research can be enhanced as additional datasets become available to better describe the entirety of urban forest. Adding a new dataset that represents an additional subpopulation of the urban forest (e.g., tree cover in city parks) will not affect how the proportion of ash trees or the mean distance to the next ash individual are calculated, there would simply be more individuals to include in a DPI calculation. Adding new datasets or expanding the inputs to a complete inventory would require a modification of the density variable in the numerator. Despite this requirement, tree density can easily be modified from a measure of trees per linear distance to a measure of trees per area, ideally a measure of leaf area coverage per area. Because tree benefits increase with leaf area (usually correlated with tree maturity; McPherson, 1992; Peper and McPherson, 2003), a greater benefit to cost ratio is typically possible with fewer larger trees than is associated with a greater number of younger trees. Moreover, when expressed as leaf area per unit of geographic area, the DPI would better reflect the magnitude of potential damage to the local stand structure than using stem density. The potential for expansion of this DPI is particularly important to better identifying the potential for ecological impacts related to gap formation resulting from the EAB invasion; in its present form, the DPI does not capture the considerable proportion of leaf area corresponding to Toronto's expansive ravine system. Data describing leaf area by tree species was not available for this study and, therefore, it was necessary to use stem density to represent ash tree density. As a consequence the nature of the relationship (whether linear or multiplicative) between DPI and realized damage to the canopy cannot effectively be evaluated at this time; however, validation of the theoretical DPI, through ground-based measurement, should be the priority of a subsequent study.
It is important to note that, although the methodology is expandable as new data become available, this DPI was developed using a street tree inventory and, therefore, the results of the analyses should not be extrapolated categorically beyond that of the street tree resource. As well, there are potential data limitations related to the dynamic nature of the urban forest that include some uncertainty concerning tree condition and whether the dataset may include trees that have died since the time of inventory. Furthermore, this analysis was not designed to predict how EAB will spread throughout the study area. Developing a model that could predict both the spatial and temporal dimensions of EAB infestation, and resulting damage, requires data that were not available for Toronto. In addition to a complete inventory (or an estimation from a sampling program), more complete information regarding where the EAB infestation originated; the location of first satellite colonies that spread from the origin of infestation; and, the time from infestation to new colony establishment are all required for such a spatio-temporal model.
Construction of the DPI in this research serves as an important framework for understanding how past urban tree planting decisions can create unanticipated consequences as a result of insect pests or pathogens, beyond the immediate example of EAB. A narrow tree species selection and the resulting decrease in diversity, influenced in part by short-term economic considerations, has led to high concentrations of ash trees in parts of Toronto. Subsequently, an uneven distribution of locations with a greater likelihood of tree mortality and associated loss of tree canopy has developed over time. These planting decisions have caused an exacerbation of the EAB impact that is multi-scalar, present at both the local level (i.e., the CT level) and at a meso-scale (i.e., across multiple contiguous CTs) in some parts of the study area.
Like many North American cities confronting EAB infestations, Toronto has made a commitment to replacing trees that succumb to this insect pest. The findings of this study underscore the need for decision-makers to understand the longer-term consequences of the tradeoffs made at the time of planting (i.e., species selection and geographic placement) and point toward the requirement for a systemic change to decision making that moves beyond the more customary, reactive approach of replacing dead or dying trees to one of tree planting and management that is proactive and with a long-term vision of ensuring a resilient future urban forest. Replacing one species or genus with another on a one-to-one basis does not address the risks to the urban forest stemming from the dominance of a small suite of species, lack of genetic diversity, and the increased stress from urban pressures contributing to the current EAB problem. Achieving improved diversity, improved species evenness, and reducing these urban stressors will require greater attention to significantly enhancing growing conditions (i.e., improving soil volume and quality), which is likely to require increased investment per tree in the short-term. Increased short-term investment will yield returns in the form of longer-term benefits delivered by trees of a more mature stature having greater leaf area.
The framework used when developing the DPI in this research has broader applicability than evaluating Toronto's street tree resource. Within the context of the study area, the DPI can be expanded to include additional datasets as they become available to encompass a larger proportion (or the entirety) of the urban forest. Furthermore, there is wider applicability for urban forest managers—this framework can effectively be transferred for application to other tree species that may be vulnerable to other insect pests such as Anoplophora glabripennis (Asian Long-horned Beetle) and Lymantria dispar dispar (Gypsy Moth), or be used in vulnerability assessments of tree pathogens such as Ophiostoma spp. (Dutch Elm Disease).
Author Contributions
CG Underlying concept, data processing, interpretation of results, figure development, manuscript preparation. AM Interpretation of results and editorial support.
Conflict of Interest Statement
The authors declare that the research was conducted in the absence of any commercial or financial relationships that could be construed as a potential conflict of interest.
Acknowledgments
We thank the reviewers for providing timely and insightful feedback on this study. The doctoral examination committee for CG dissertation added valuable insights concerning the shaping of this manuscript. UFRED Group research assistants—particularly Adriano Nicolucci—provided vital assistance processing lot boundary data. The authors appreciate the EnSciMan Program's willingness to support open-access publishing.
References
Alvey, A. A. (2006). Promoting and preserving biodiversity in the urban forest. Urban For. Urban Gree. 5, 195–201. doi: 10.1016/j.ufug.2006.09.003
Anselin, L. (1995). Local indicators of spatial association-LISA. Geogr. Anal. 27, 93–115. doi: 10.1111/j.1538-4632.1995.tb00338.x
Bassuk, N., and Whitlow, T. (1988). Environmental stress in street trees. Arboric. J. 12, 195–201. doi: 10.1080/03071375.1988.9746788
Burley, S., Robinson, S. L., and Lundholm, J. T. (2008). Post-hurricane vegetation recovery in an urban forest. Landsc. Urban Plan. 85, 111–122. doi: 10.1016/j.landurbplan.2007.10.003
Cappaert, D., McCullough, D. G., Poland, T. M., and Siegert, N. W. (2005). Emerald ash borer in North America: a research and regulatory challenge. Am. Entomol. 51, 152–165. doi: 10.1093/ae/51.3.152
City of Toronto Parks Forestry Recreation, Urban Forestry. (2012). Street Tree Data [Feature Dataset, Shapefile]. Available online at: http://www1.toronto.ca/wps/portal/contentonly?vgnextoid=5af95104c26f3310VgnVCM1000003dd60f89RCRD&vgnextchannel=1a66e03bb8d1e310VgnVCM10000071d60f89RCRD
City of Toronto Parks Forestry Recreation, Urban Forestry,. (2013). Sustaining and Expanding the Urban Forest: Toronto's Strategic Forest Management Plan. Toronto, ON: City of Toronto.
City Plants (2015). About City Plants. Available online at: http://www.cityplants.org/index.php/about-us-1/about-city-plants
Close, R. E., Nguyen, P. V., and Kielbaso, J. J. (1996). Urban vs. natural sugar maple growth: I. stress symptoms and phenology in relation to site characteristics. J. Arboric. 22, 144–150.
Dale, D. (2014). Mayoral Candidate John Tory Proposes Doubling Toronto's Tree-Planting Budget. Tor. Star. Available online at: http://www.thestar.com/news/city_hall/toronto2014election/2014/04/22/mayoral_candidate_john_tory_proposes_doubling_torontos_treeplanting_budget.html
Doyle, J. (2015). 2015 Operating Budget Briefing Note: Tree Planting Program. (Briefing Note No. BN # 9 – Feb 13). Toronto, ON.
Dwyer, J. F., McPherson, E. G., Schroeder, H. W., and Rowntree, R. A. (1992). Assessing the benefits and costs of the urban forest. J. Arboric. 18, 227–234.
Foster, R. S., and Blaine, J. (1978). Urban tree survival: trees in the sidewalk. J. Arboric. 4, 14–17.
Gandhi, K. J., and Herms, D. A. (2010). Direct and indirect effects of alien insect herbivores on ecological processes and interactions in forests of eastern North America. Biol. Invasions 12, 389–405. doi: 10.1007/s10530-009-9627-9
Getis, A., and Ord, J. K. (1992). The analysis of spatial association by use of distance statistics. Geogr. Anal. 24, 189–206. doi: 10.1111/j.1538-4632.1992.tb00261.x
Givoni, B. (1991). Impact of planted areas on urban environmental quality: a review. Atmos. Environ. B-Urban 25, 289–299. doi: 10.1016/0957-1272(91)90001-U
Greene, C. S. (2015). Integrating Sustainability Subsystems in the Management of Urban Forests. Unpublished PhD Dissertation, Ryerson University. Toronto, ON.
Herms, D. A., and McCullough, D. G. (2014). Emerald ash borer invasion of North America: history, biology, ecology, impacts, and management. Annu. Rev. Entomol. 59, 13–30. doi: 10.1146/annurev-ento-011613-162051
Heynen, N., Perkins, H. A., and Roy, P. (2006). The political ecology of uneven urban green space: the impact of political economy on race and ethnicity in producing environmental inequality in Milwaukee. Urban Aff. Rev. 42, 3–25. doi: 10.1177/1078087406290729
Hodge, J., Scarr, T., Ross, F., Ryall, K., and Barry, L. (2015). Emerald Ash Borer Pest Risk Analysis for Northern Ontario and Manitoba. Canadian Council of Forest Ministers Forest Pest Working Group. Available online at: http://cfs.nrcan.gc.ca/publications?id=35964
Kenis, M., Auger-Rozenberg, M.-A., Roques, A., Timms, L., Péré, C., Cock, M. J. W., et al. (2009). Ecological effects of invasive alien insects. Biol. Invasions 11, 21–45. doi: 10.1007/s10530-008-9318-y
Laćan, I., and McBride, J. R. (2008). Pest vulnerability matrix (PVM): a graphic model for assessing the interaction between tree species diversity and urban forest susceptibility to insects and diseases. Urban For. Urban Gree. 7, 291–300. doi: 10.1016/j.ufug.2008.06.002
Lindsey, P., and Bassuk, N. (1992). Redesigning the urban forest from the ground below: a new approach to specifying adequate soil volumes for street trees. Arboric. J. 16, 25–39. doi: 10.1080/03071375.1992.9746896
Lu, J. W. T., Svendsen, E. S., Campbell, L. K., Greenfield, J., Braden, J., King, K. L., et al. (2010). Biological, social, and urban design factors affecting young street tree mortality in New York City. Cities Environ. 3, 1–15.
MacFarlane, D. W., and Meyer, S. P. (2005). Characteristics and distribution of potential ash tree hosts for emerald ash borer. Forest Ecol. Manag. 213, 15–24. doi: 10.1016/j.foreco.2005.03.013
McCullough, D. G., Poland, T. M., and Cappaert, D. (2009). Attraction of the emerald ash borer to ash trees stressed by girdling, herbicide treatment, or wounding. Can. J. Forest. Res. 39, 1331–1345. doi: 10.1139/X09-057
McPherson, E. G. (1992). Accounting for benefits and costs of urban greenspace. Lands. Urban Plan. 22, 41–51. doi: 10.1016/0169-2046(92)90006-L
Million Trees NYC (2015). Million Trees NYC: About. Available online at: http://www.milliontreesnyc.org/html/about/about.shtml
Nowak, D. J. (1993). Historical vegetation change in Oakland and its implications for urban forest management. J. Arboric. 19, 313–319.
Nowak, D. J., and Greenfield, E. J. (2012). Tree and impervious cover change in U.S. cities. Urban For. Urban Gree. 11, 21–30. doi: 10.1016/j.ufug.2011.11.005
Nowak, D. J., Hoehn, R. E. III, Bodine, A. R., Greenfield, E. J., Ellis, A., Endreny, T. A., et al. (2013). Assessing Urban Forest Effects and Values: Toronto's Urban Forest (Research Bulletin No. NRS-79). Newtown Squarte, PA: U.S. Forest Service.
Nowak, D. J., Hoehn, R. E. III, Crane, D. E., Stevens, J. C., and Walton, J. T. (2007). Assessing Urban Forest Effects and Values: New York City's Urban Forest (Research Bulletin No. NRS-9). Newtown Square, PA: U.S. Forest Service.
Nowak, D. J., Hoehn, R. E. III, Crane, D. E., Weller, L., and Davila, A. (2011). Assessing Urban Forest Effects and Values: Los Angeles' Urban Forest (Research Bulletin No. NRS-47). Newtown Square, PA: U.S. Forest Service.
Ord, J. K., and Getis, A. (1995). Local spatial autocorrelation statistics: distributional issues and an application. Geogr. Anal. 27, 286–306. doi: 10.1111/j.1538-4632.1995.tb00912.x
Ord, J. K., and Getis, A. (2001). Testing for local spatial autocorrelation in the presence of global autocorrelation. J. Reg. Sci. 41, 411–432. doi: 10.1111/0022-4146.00224
O'Toole, M. (2013, March 6). “Toronto overtakes Chicago as fourth-largest city in North America, in National Post. Available online at: http://news.nationalpost.com/toronto/toronto-overtakes-chicago-as-fourth-largest-city-in-north-america
Patterson, B. (2011). Strategy for the Management of the Emerald Ash Borer (Staff Report No. PE02-032811-AFS#12934). Toronto, ON.
Peper, P. J., and McPherson, E. G. (2003). Evaluation of four methods for estimating leaf area of isolated trees. Urban For. Urban Gree. 2, 19–29. doi: 10.1078/1618-8667-00020
Pham, T.-T.-H., Apparicio, P., Séguin, A.-M., Landry, S., and Gagnon, M. (2012). Spatial distribution of vegetation in Montreal: an uneven distribution or environmental inequity? Landsc. Urban Plan. 107, 214–224. doi: 10.1016/j.landurbplan.2012.06.002
Poland, T. M., and McCullough, D. G. (2006). Emerald ash borer: Invasion of the urban forest and the threat to North America's ash resource. J. Forest. 104, 118–124.
Prasad, A., Iverson, L., Peters, M., Bossenbroek, J., Matthews, S., Davis Sydnor, T., et al. (2010). Modeling the invasive emerald ash borer risk of spread using a spatially explicit cellular model. Landscape Ecol. 25, 353–369. doi: 10.1007/s10980-009-9434-9
Quigley, M. F. (2004). Street trees and rural conspecifics: will long-lived trees reach full size in urban conditions? Urban Ecosyst. 7, 29–39. doi: 10.1023/B:UECO.0000020170.58404.e9
Raupp, M. J., Cumming, A. B., and Raupp, E. C. (2006). Street tree diversity in eastern North America and its potential for tree loss to exotic borers. Arboric. Urban For. 32, 297–304.
Richards, N. A. (1983). Diversity and stability in a street tree population. Urban Ecol. 7, 159–171. doi: 10.1016/0304-4009(83)90034-7
Sanders, R. A. (1981). Diversity in the street trees of Syracuse, New York. Urban Ecol. 5, 33–43. doi: 10.1016/0304-4009(81)90019-X
Sanders, R. A. (1984). Some determinants of urban forest structure. Urban Ecol. 8, 13–27. doi: 10.1016/0304-4009(84)90004-4
Scarr, T. A., Ryall, K. L., and Hodge, P. (2012). Forest Health Conditions in Ontario, 2011. Available online at: https://www.ontario.ca/document/forest-health-conditions-2011
Schwartz, H. (2004). The relevance of Toronto's new governmental structure for the 21st century. Can. J. Regional Sci. 27, 99–120.
Siegert, N. W., McCullough, D. G., Williams, D. W., Fraser, I., Poland, T. M., and Pierce, S. J. (2010). Dispersal of agrilus planipennis (coleoptera: buprestidae) from discrete epicenters in two outlier sites. Environ. Entomol. 39, 253–265. doi: 10.1603/EN09029
Sisinni, S., Zipperer, W., and Pleninger, A. (1995). Impacts from a major ice storm: street-tree damage in Rochester, New York. J. Arboric. 21, 156–167.
Smiley, E. T., Calfee, L., Fraedrich, B. R., and Smiley, E. J. (2006). Comparison of structural and noncompacted soils for trees surrounded by pavement. Arboric. Urban For. 32, 164–169.
Sydnor, T. D., Bumgardner, M., and Todd, A. (2007). The potential economic impacts of emerald ash borer (agrilus planipennis) on Ohio, U.S., communities. Arboric. Urban For. 33, 48–54.
Whitlow, T. H., and Bassuk, N. L. (1988). Ecophysiology of urban trees and their management: the North American experience. Hortic. Sci. 23, 542–546.
Keywords: urban forest, invasive species, emerald ash borer, environmental decision making, vulnerability
Citation: Greene CS and Millward AA (2016) The Legacy of Past Tree Planting Decisions for a City Confronting Emerald Ash Borer (Agrilus planipennis) Invasion. Front. Ecol. Evol. 4:27. doi: 10.3389/fevo.2016.00027
Received: 13 January 2016; Accepted: 09 March 2016;
Published: 30 March 2016.
Edited by:
Jeremy Lundholm, Saint Mary's University, CanadaReviewed by:
Shuqing Zhao, Peking University, ChinaSusan C. Cook-Patton, AAAS Fellow at United States Department of Agriculture Forest Service, USA
Copyright © 2016 Greene and Millward. This is an open-access article distributed under the terms of the Creative Commons Attribution License (CC BY). The use, distribution or reproduction in other forums is permitted, provided the original author(s) or licensor are credited and that the original publication in this journal is cited, in accordance with accepted academic practice. No use, distribution or reproduction is permitted which does not comply with these terms.
*Correspondence: Christopher S. Greene, Y3NncmVlbmVAYXJ0cy5yeWVyc29uLmNh