- 1Departamento de Antropología, Universidad de Chile, Santiago, Chile
- 2Centro de Estudios Avanzados en Zonas Áridas, Universidad de la Serena, La Serena, Chile
- 3Centro de Estudios del Hombre Austral, Instituto de la Patagonia, Universidad de Magallanes, Punta Arenas, Chile
- 4Departamento de Antropología, Universidad Alberto Hurtado, Santiago, Chile
- 5Instituto de Geografía, Departamento de Geografía Física, Pontificia Universidad Católica de Chilev, Santiago, Chile
The forest-steppe ecotone of the eastern slope of the Andes in Central Western Patagonia (43°40′–49°15′ S, Chile, South America) provides a unique area for assessing long and short term dynamics between humans and past environments. Central Western Patagonia was a demographically marginal zone inhabited intermittently and with low intensity by hunter-gatherers during the Holocene. This paper adopts a novel approach in order to assess the relationship between trends in the archeological, pollen, and charcoal records. The recognition of temporal and spatial scales in both archeology and paleoecology is crucial for defining roles in paleofire records. The main goal of the paper is to assess the role of climate and human beings as potential ignitors of wildfires by acknowledging the scales in which they operate and the different roles either one played in paleofire trends. We investigated a case study in the Cisnes River Valley (CRV) where the frequencies and magnitudes of fire episodes—reconstructed from macro-charcoal particles from the Lake Shaman intermoraine sequence—can be attributed to human action, while acknowledging the driving role of climate over broader time scales. The Lake Shaman charcoal record spanning the last 19,000 cal years is compared to the archeological record starting at 11,500 cal years BP. After comparing paleofires, reconstructed from the charcoal record, with peaks and troughs in the radiocarbon record and archeological evidence at local and site scales, we argue that this approach provides insights for assessing the timing and magnitude of human effects on the environment. We examine collation and correlation scenarios for comparative trends between the archeological, pollen, and charcoal records. The correlation of occupational events at the El Chueco 1 archeological site and other sites along the CRV with the results obtained at Lake Shaman is suggestive of a combination of human agency and climate drivers in the occurrence of fires during most of the Holocene.
Introduction
Fire plays an important role in the global carbon cycle, in atmospheric chemistry, in large and small scale dynamics of terrestrial ecosystems and in biodiversity (Whitlock et al., 2007; Power et al., 2008). The occurrence, frequency and extent of fires are a complex admixture of climate, available fuels and ignition sources (Power et al., 2008). There is little doubt of the dominant role played by climate in fire ignition and maintenance at large spatial and time scales (Power et al., 2008). However, there is much contention over the role(s) played by humans. One of the most significant effects by humans on their local environments is through the use of fire, but the role of humans as wildfire ignition agents has not been fully explored (Alaback et al., 2003). Although the timing of hominid fire use has been discussed, together with how it was used and controlled (Weiner et al., 1998; Stahlschmidt et al., 2015), there is little doubt that it plays a major role in modern human societies. For instance, fire has been used for driving game, creating artificial temperatures, clearing underbrush, among other uses. For some of these purposes humans ignited open and forest vegetation, thereby establishing or maintaining fire-successional vegetation communities (e.g., Dincauze, 2000; Bliege Bird et al., 2012). However, convincing scenarios of human agency in past fires are often elusive.
The extent and impact of deliberate fires seems to vary greatly between geographic zones. This is the case for the Northwestern coast of North America, as well as for the Rocky Mountains, where the role of humans in fire dynamics since the late Pleistocene seems to have been spatially variable (Moss et al., 2007; McWethy et al., 2013). In Santa Rosa Island, California, human presence seems not to have altered fire regimes (Rick et al., 2012). In Australia, the analysis at continental and regional scales shows no robust correlations between human 14C records and the occurrence of fires (Williams et al., 2015). However, the arrival of Polynesian populations in New Zealand appears to be correlated with dramatic changes to forests through burning (McWethy et al., 2014). Heusser (1995, 2003) attributed a major role in fire incidence and in shaping vegetation in southern South America to the arrival of humans in the late Pleistocene. In the absence of the strong convective air movement necessary for lightning to take place, and given the low capability of volcanic eruptions to ignite fires in humid environments, the author sustained that hunter-gatherers were “a leading vector of burning” (Heusser, 1994:436). On the other hand, the spatial and temporal occurrence of postglacial fires in Patagonia has been interpreted as a consequence of differential ecological responses to climate variability (Markgraf and Anderson, 1994), with no main role played by humans. The extent and magnitude of fires have varied significantly since the end of the Pleistocene, an aspect that has led some authors to argue in favor of climate as a main driver of paleofires (e.g., Huber et al., 2004; Whitlock et al., 2007), directly linked to the local availability of combustible biomass (Iglesias and Whitlock, 2014).
Assessing dynamics between past human and environmental records is a complex process requiring multiple analytical approaches, the recognition of spatial and temporal scales, and the selection of appropriate comparable datasets (Grosjean et al., 2003; Anderson et al., 2007). Defining spatial and temporal scales is a chief concern for assessing the role of humans in fire occurrence. At the scale of an individual fire episode, climate, available fuel and local topography are decisive in determining its characteristics (McWethy et al., 2013). On the other hand, fire regimes imply patterns at larger spatial and temporal scales (Whitlock et al., 2010). In order to ascertain the role of humans in fire occurrence, the archeological record cannot be directly compared to the paleofire reconstructions derived from charcoal particle records of sedimentary archives. In fact, using archeological site frequencies without detail or using radiocarbon dates without properly weighing the data may provide an incomplete or even biased picture of human activity in time and space. Aspects such as regional survey coverage, representativeness, proximity, alternative sites, and the accuracy of the radiocarbon data need to be considered in order to formulate comparable datasets. Furthermore, mobility, technology, and subsistence economy need to be considered in the discussion of the potential influence of humans in modulating fire records, since human-fire relationships vary with the economic orientation that a society has developed (McWethy et al., 2013; Iglesias and Whitlock, 2014). Thus, selecting appropriate case studies, such as areas inhabited solely by hunter-gatherers, may provide a better case-control than including territories with shifting populations and different lifeways.
This paper addresses the relationship between the archeological and charcoal records in the Cisnes River Valley (CRV, 44°40′ S), located in Central Western Patagonia (CWP, Aisén Region, Chile). We examine the role of ancient Patagonian hunter-gatherers in transforming their habitats by starting fires throughout the Holocene. The archeological record of this region is ideal for this comparison because local occupations were not continuous or intense at any given time during the Holocene. The advantage of such punctuated occupation is that human presence may be considered as “measurable” pulses followed by periods devoid of humans, thus archeologically sensitive and comparable to other fluctuating datasets. This paper compares episodic—event-type—records defining the presence or absence of humans with continuous environmental records where paleofire dynamics have already been established (de Porras et al., 2012). The main goal of the paper is to assess the role of climate and human beings as potential ignitors of wildfires in CWP, by acknowledging the scales in which they operate and the different roles either one played in paleofire trends in CRV during the Holocene.
Materials and Methods
Environment and Wildfire Natural Occurrence in Central Western Patagonia
CWP (~44 to 49° S) is located at the western margin of southern South America (Figure 1). It is characterized by four main geomorphologic units (from west to east): the archipelagos and channels of the Pacific, the Andean mountain range which includes volcanoes and ice fields, the sub-Andean mountain range of lower steepness, and extensive sedimentary plains with glacial and fluvio-glacial landforms. The region is located at the northern border of the core of the Southern Westerlies (Garreaud, 2009). It is characterized by a marked west-to-east precipitation gradient resulting from forced air mass subsidence on the lee side of the Andes and a resulting rain-shadow effect (Garreaud et al., 2009). Precipitation ranges from 3500 mm on the Pacific coast to 450 mm close to the Chilean-Argentinean border. Present day vegetation distribution follows the decreasing west-east precipitation gradient. Thus, Pilgerodendron uviferum and Astelia pumila evergreen forest and Nothofagus betuloides and Desfontainia spinosa evergreen forest dominate the western slope of the Andes (lower and middle CRV, respectively; Luebert and Pliscoff, 2006). On the lee side, plant communities include the deciduous forest of Nothofagus pumilio and Berberis illicifolia, the forest-steppe transition of Nothofagus antarctica and Berberis microphylla, and the grass steppe of Festuca pallescens close to the international border (upper CRV; Luebert and Pliscoff, 2006). Native vegetation has been particularly disturbed by logging and intentional fires since European settlement during the early twentieth century, when sheep-herding was introduced (Martinic, 2005). The modern pollen signal is dominated by the Nothofagus dombeyi type pollen throughout the vegetation gradient. Thus, the evergreen forest assemblage is dominated by N. dombeyi type pollen, accompanied by evergreen forest indicators such as Pilgerodendron uviferun, Podocarpus nubigena, and Tepualia stipularis (Haberle and Bennett, 2001). The deciduous forest assemblage is also dominated by N. dombeyi type pollen, however accompanied by Poaceae, represented in higher proportions toward the east. Other pollen taxa that characterize the vegetation gradient are Embothrium within the deciduous forest, Mulinum in the forest-steppe transition and Empetrum in elevated areas. In the upper CRV, the grass steppe of F. pallescens is represented by Poaceae, Asteraceae, and N. dombeyi type reaching 30%; proportion attributed to presence of small forest patches located in protected areas, such as intermoraine depressions.
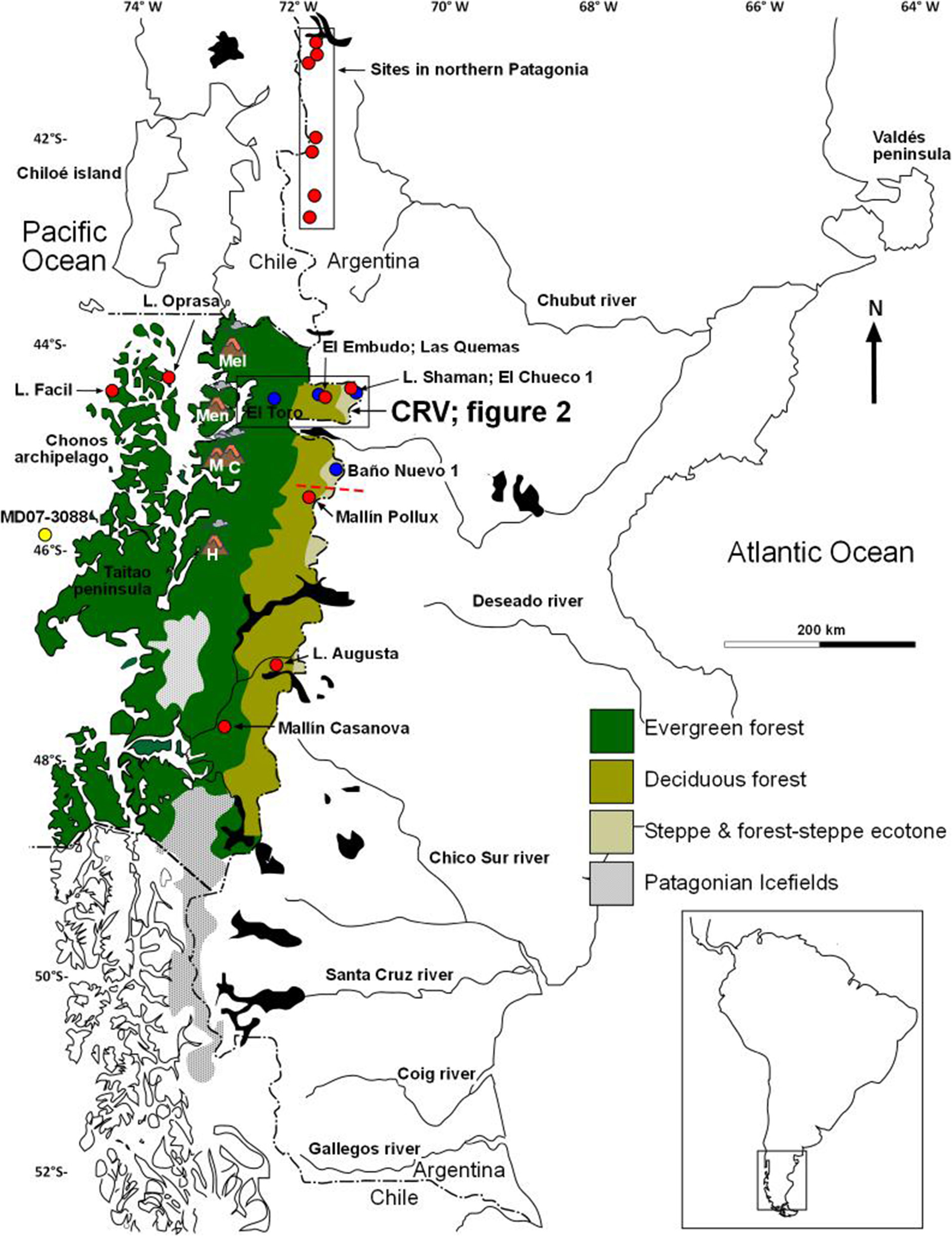
Figure 1. Map of Southern South America showing the CWP, main vegetation communities, the location of the CRV, the main archeological sites (blue), the terrestrial (red), and marine (yellow) sedimentary records discussed in the text, and the location of the Melimoyu (Mel), Mentolat (Men), Maca (M), Cay (C), and Hudson (H) volcanoes.
Wildfires in the forests and forest-steppe ecotone of CWP mainly occur in summer (January to March) when temperatures are warm and fuel conditions are most likely to be dry (CONAF1). Lightning occurrence is quite frequent when Western Patagonia (40–55° S) is immersed in a pool of cold air behind a front that has reached the coast at ~40° S, leading to weakly unstable postfrontal conditions (Garreaud et al., 2014). The forced uplift of the strong westerly winds impinging on the coastal mountains can trigger convection and produce lightning activity, particularly along the coast and, to a lesser degree, on the lee side of the Andes, during summer and late fall (Garreaud et al., 2014). In fact, Holz and Veblen (2012) recorded numerous lightning scars on trees in the CWP evergreen forest that were associated with charcoal, thus indicating that wildfire spread to the surrounding vegetation.
Wildfire occurrence in CWP evergreen forests is climate-limited, given that fire is not restricted by fuel availability in such high biomass environments; rather it is strongly associated with reduced spring precipitation which results in low fuel moisture levels during the summer fire season (Holz and Veblen, 2012). Considering this factor and the fire activity curve along a vegetation gradient (very similar to the west-east gradient in CWP) proposed by Whitlock et al. (2010), wildfires in the deciduous forest would also be climate-limited. Widespread wildfires in Nothofagus deciduous forest are dependent on dry conditions at several time scales that can be a product of decreases in precipitation and/or increases in temperatures (Kitzberger and Veblen, 2003; Lara et al., 2003; González and Veblen, 2006). Moreover, fire occurrence in deciduous forests might be more frequent than in the evergreen forests, given that their eastern distribution along the precipitation gradient would result in drier fuels during the summer fire season under lower (spring) precipitation. On the other hand the forest-steppe ecotone, characterized by a woodland-to-grassland vegetation pattern, presents a climate-limited fire regime influenced in part by a fuel-limited fire regime and appears to be the vegetation unit most prone to anthropogenic influence.
The Physical Setting, Vegetation, and Paleoenvironment of the Cisnes River Valley
The CRV is a 140 km east–west oriented valley excavated by multiple Pleistocene glacial expansions, as testified by well-developed moraine landforms (Figure 2). The valley develops across the Southern Andes, from the Chile-Argentina border to the Pacific Ocean. East of the Andes, the CRV is characterized by outwash plains punctuated by moraines, paleo-lake terraces, and volcanic features (De la Cruz and Cortes, 2011). Deglaciation, after ice occupied CRV during the last glacial period, was characterized by ice front stabilizations and the formation of regional scale proglacial lakes. Series of lake shorelines in the area are suggestive of steady lake recession during deglaciation. Moraine belts and glacio-lacustrine deposits have been reworked by postglacial fluvial erosion.
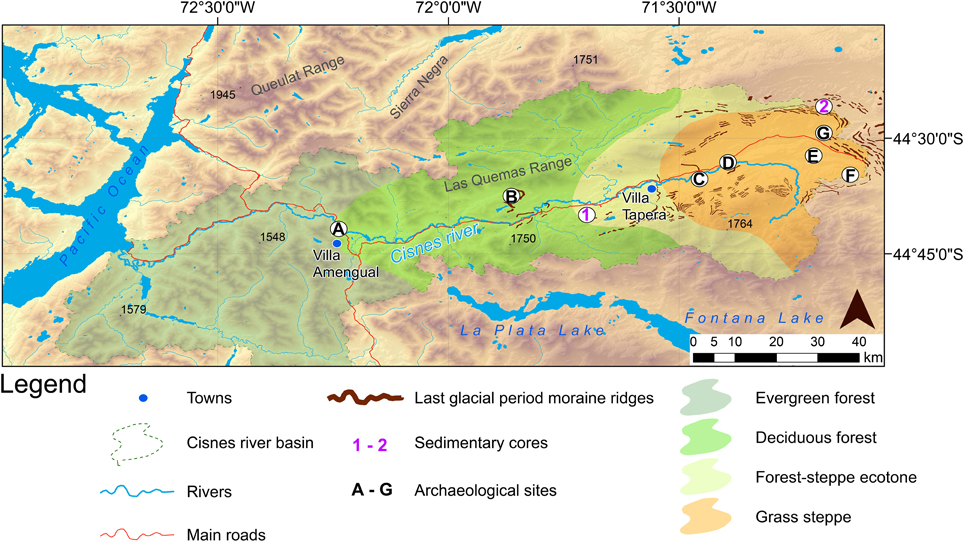
Figure 2. Map of the CRV showing vegetation distribution, main geomorphological features, archeological sites (A, El Toro; B, Las Quemas; C, Chenque Cisnes; D, Winchester 1; E, La Cantera 1; F, Appeleg 1; G, El Chueco 1), and sedimentary cores (1, Mallín El Embudo; 2, Lake Shaman).
Two sedimentary records are available for local climate reconstruction (Figure 3A). The intermoraine Lake Shaman site (44°26′ S; 71°11′ W, 919 masl, Figure 2, sedimentary core 2) located in the forest-steppe ecotone shows organic sedimentation starting at 19,000 cal years BP, thus providing a minimum age for the retreat of the glacial lobe in the upper CRV (de Porras et al., 2012; Figure 4A). Whereas, the Mallín El Embudo site (44°40′ S; 71°42′ W, 686 masl, Figure 2, sedimentary core 1), in the deciduous forest belt, shows a minimum age for local ice-free conditions at 13,000 cal years BP, based on its basal sediments indicating that the basin was no longer flooded by a proglacial lake that occupied the middle CRV (de Porras et al., 2014). Lake Shaman and Mallín El Embudo pollen records show the variability in effective moisture, expressed in the former as fluctuations in the relative abundance of pollen taxa indicative of the location of the forest-steppe boundary, and in the latter as the variability in the canopy density of the deciduous forest. Changes of vegetation in both records are considered to be a consequence of fluctuations in the position and/or strength of the Southern Westerlies since the last glacial period (de Porras et al., 2012, 2014).
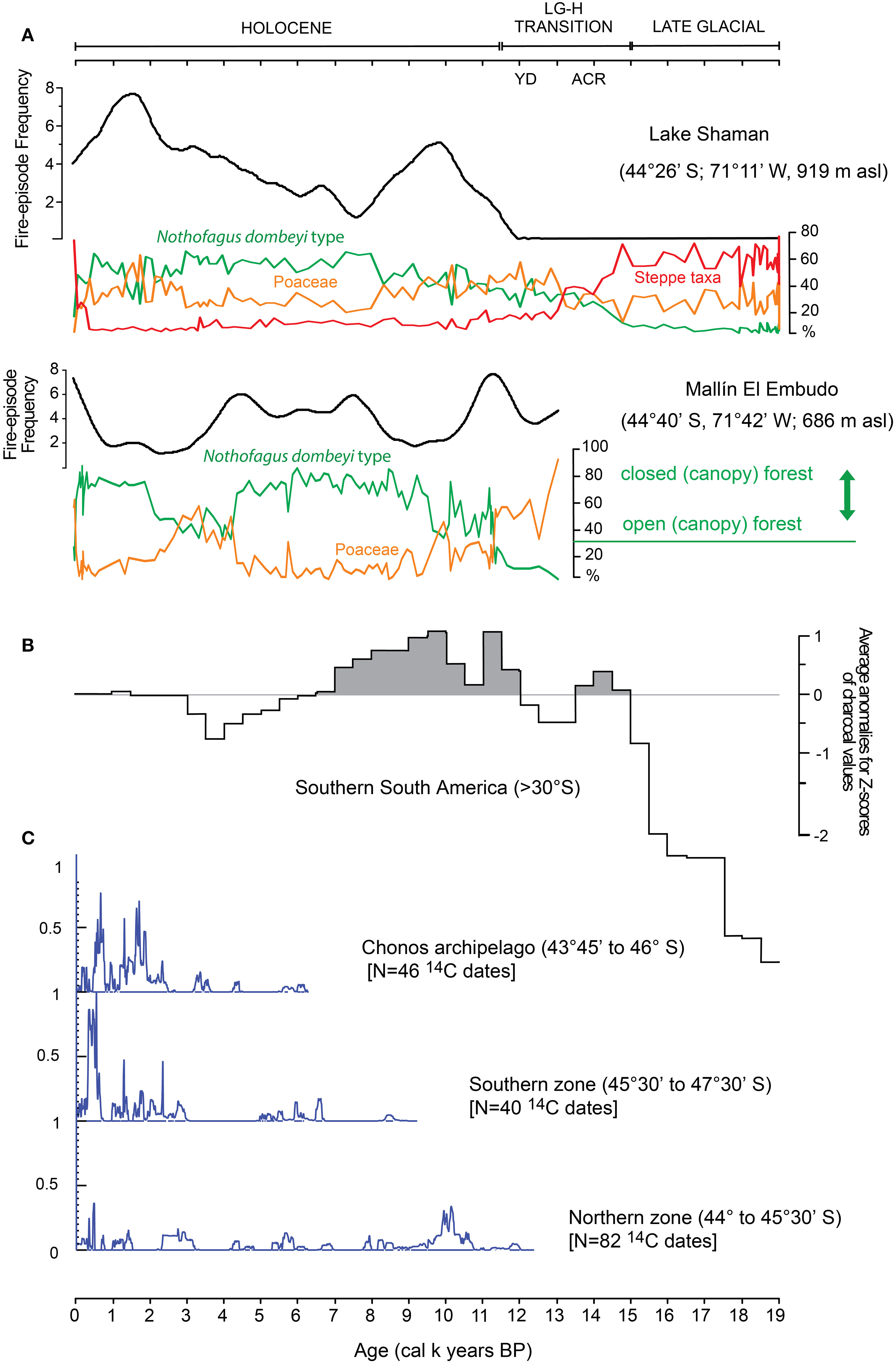
Figure 3. (A) Summary of main pollen taxa and fire-episode frequencies from Mallín El Embudo (de Porras et al., 2014) and Lake Shaman (de Porras et al., 2012) sedimentary records, (B) summary of paleofire activity in western South America (>30° S, after Power et al., 2008), and (C) summed probabilities of the 14C calibrated age distributions for archeological sites in CWP partitioned by sub-regions.
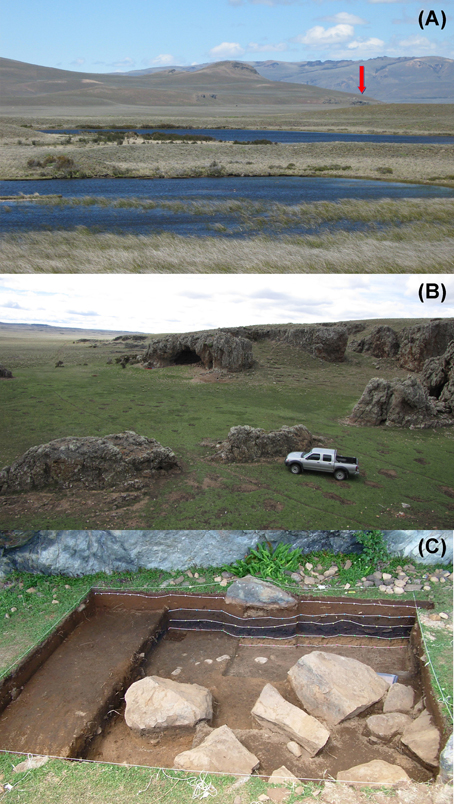
Figure 4. (A) View from Lake Shaman coring site to El Chueco 1 archeological site (red arrow), (B) El Chueco 1 cave site, and (C) excavations at Las Quemas rockshelter showing the distinctive charcoal full stratigraphic unit 4 (black stratum).
The pollen record at Lake Shaman indicates the dominance of taxa associated with cool, dry steppe from 19,000 to 14,800 cal years BP (de Porras et al., 2012), followed by a gradual increase in forest taxa. This is consistent with the beginning of the pollen record of Mallín El Embudo at 13,000 cal years BP, where grass taxa accompanied by herbs and shrubs occur alongside low percentages of Nothofagus pollen (de Porras et al., 2014). The expected pollen assemblage for the forest-steppe ecotone appears in these records after 11,500 cal years BP, associated with an increase in effective moisture and a rise in summer temperatures, but still below modern values (de Porras et al., 2012, 2014). According to the record at Lake Shaman, a major eastward expansion of forest into the steppe occurred between 8000 and 3000 cal years BP, associated with higher effective moisture conditions (de Porras et al., 2012). This matches the inference drawn from the Mallín El Embudo record, which shows the development of a closed forest between 9500 and 4200 cal years BP (de Porras et al., 2014). After 3000 cal years BP, a retraction of forest with minimum values around 1800 cal years BP suggests less effective moisture (de Porras et al., 2012), and open forest conditions in Mallín El Embudo between 4000 and 2000 cal years BP (de Porras et al., 2014). The last 2000 cal years BP were characterized by a high variability of the records, while the last 200 years show dramatic vegetation disturbances due to European colonization (de Porras et al., 2012).
Archeology of the Cisnes River Valley
Systematic archeological research in different Andean valleys of CWP has shown contrasting patterns. North of 45°30′ S chronological trends in the archeological record show intermittent presence of human records since the onset of the Holocene (Mena and Stafford, 2006; Méndez and Reyes, 2008). However, most research has been site-based, as is the case with Baño Nuevo 1, the only site reported in the Ñirehuao River Valley (45°15′ S; Mena et al., 2003). This site was occupied as a campsite by highly mobile steppe hunter-gatherers, as suggested by raw material selection, prey choices and stable isotope markers (Méndez et al., 2012, 2014). Available 14C dates indicate a minimum of 28 statistically different occupational events between 11,100 and 3000 cal years BP (Mena and Stafford, 2006), the most significant of which is the burial of 10 individuals whose direct dates overlap at 2σ between 10,180 and 9920 cal years BP (Reyes et al., 2012). On the other hand, south of 45°30′ S there is no available radiocarbon record covering the Holocene (Figure 3C). The earliest dates indicate an initial settlement at ~6000 cal years BP and a marked chronological signal only after 3000 cal years BP (Mena, 2000; Reyes, 2002; Fuentes et al., 2012).
The most detailed archeological information to date at a local (i.e., valley) scale has been gathered at the CRV (Reyes et al., 2009; Figure 2). Surface surveys along the valley have produced a total of 51 archeological sites (plus a higher number of isolated artifacts) showing a marked east–west diminishment in the distribution (grass steppe: 26; forest-steppe transition 18; deciduous forest: 6; evergreen forest: 1). Although this geographic pattern may be partially attributable to vegetation cover, it has also proven to be a reflection of the preferential intensity in the use of different biomes (Méndez et al., 2016).
Given the fact that most sites in the valley are surface lithic scatters (Reyes et al., 2006), providing an accurate radiocarbon chronology for all of them continues to be an incomplete task (Méndez and Reyes, 2015). However, the study conducted at El Chueco 1 site (Figure 4B) has been crucial for understanding the chronological dimension of this valley. Located in the grass steppe plains of the upper CRV (44°29′36″S; 71′11′13″W; 914 masl), it is a cave within a welded rhyolite tuff outcrop (Reyes et al., 2007a). Excavations at El Chueco 1 (16 m2), revealed an ordered, continuous depositional sequence with occupations extending from ~11,500 to 180 cal years BP (Méndez et al., 2011; Table 1; Figure 5). Combustion features, marking discrete localized events, are associated with very few lithic artifacts and bones limited to the upper excavated levels. The occupational events at El Chueco 1 have been interpreted as intermittent, low-intensity use by steppe hunter-gatherers, possibly during summers as suggested by macrobotanical remains at the site (Méndez et al., 2011). Other open-air and rockshelter sites in the upper CRV have yielded ages between 1500 and 400 cal years BP. These have been tested and radiocarbon dated, and as a whole they are indicative of a broad functional variability (Reyes et al., 2009) including both large and small open-air camps (e.g., Appeleg 1, Winchester 1), chenque-type burials (e.g., Pampa El Frío), and lithic workshops (e.g., La Cantera 1; Reyes et al., 2007b; Velásquez et al., 2007; Reyes and Méndez, 2010; Gómez and Méndez, 2015). Along with the larger overall extent of some of these sites when compared to cave sites, a much wider diversity of activities is suggested by larger lithic assemblages, higher frequency of formal tool types, evidence of grinding stone tools, and a more diverse selection of raw materials (Contreras et al., 2016). These, with other undated surface sites in the area, suggest a more intense occupation for the upper CRV during this time period. No contemporary dates have been obtained for sites in the middle and lower CRV (Reyes et al., 2009).
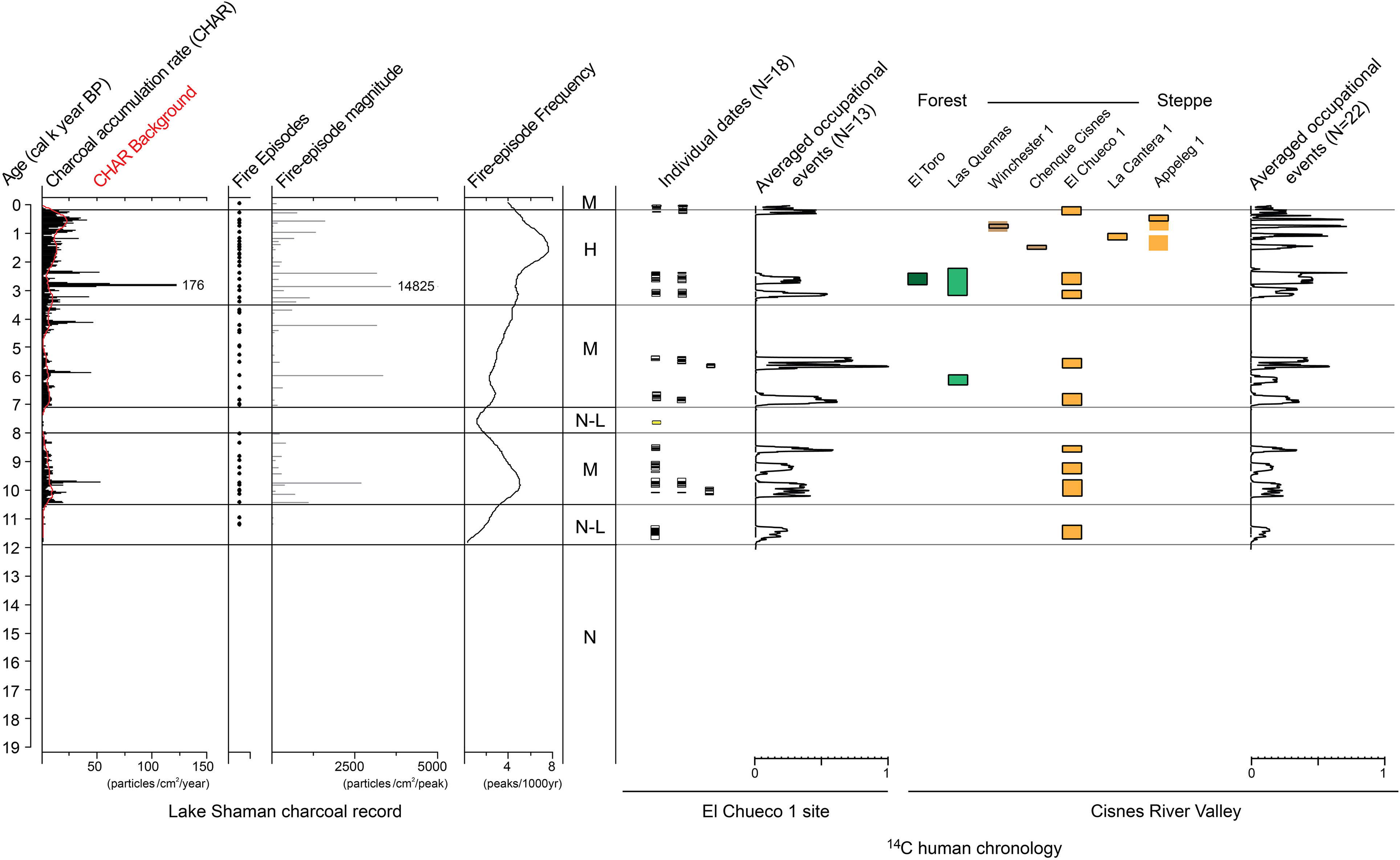
Figure 5. Lake Shaman charcoal record (de Porras et al., 2012) plotted against 14C dates from archeological sites in CRV. The charcoal record was divided into four categories: null (N), null-low (N-L), moderate (M), and high (H) fire activity. Individual dates are graphed in bar plots and the averaged occupational events in a summed probability curve for El Chueco 1 site. Occupational blocks (2σ 14C dates) and the averaged occupational events in a summed probability curve are graphed for CRV (N = 7 dated sites). Archeological sites are organized from west to east showing the environments where the sites are located. Thermoluminiscence dates (Appeleg 1, N = 2; Winchester 1, N = 1) appear unframed.
In the deciduous forest zone, Las Quemas rockshelter site (44°37′42″ S; 71°51′37″ W; 724 masl) affords the main source of archeological evidence (Méndez and Reyes, 2006). The shelter provides a horizontal protected area in excess of 200 m2 and excavations performed in the central area covered 13 m2. Seven 14C dates have been used to define three statistically different occupational events between 2950 and 2400 cal years BP, plus an earlier one at 5990–6270 cal years BP identified at the base of the cultural deposition (Méndez et al., 2016). Bones are inexistent, most probably due to lack of preservation, and lithics are infrequent but evenly dispersed throughout the excavation. Lithics consist mainly of locally procured toolstone types used in a variety of tasks including wood processing. Based on its occupations, Las Quemas site has been interpreted as a redundant, low-intensity, transitory camp occupied while traversing the river axis (Méndez et al., 2016). A macro-charcoal particle recovered beneath the archeological deposition, at the base of the charcoal full stratigraphic unit 4 and directly below a roof fall feature (Figure 4C), was dated to 12,070–11,810 cal years BP. This evidence has been interpreted as a major fire occurring in the area before humans occupied the site (Méndez et al., 2016).
In the westernmost sector of the study area, in the evergreen forest, excavations totaling 14 m2 were conducted at El Toro rockshelter site (44°41′59″S; 72°14′12″W; 219 masl; Bate and Mena, 2005; Méndez et al., 2010). This basaltic cavity, located ~16 m above the Cisnes River course, was filled with fluvial sands, probably attesting to a perched river bed. Despite the hyperhumid setting, the dryness of sediments within the cave has allowed good preservation of bones, consisting of the remains of the cervids huemul (Hippocamelus bisulcus) and pudu (Pudu pudu; Mena et al., 2004) as well as other organic material including charcoal, freshwater shells, wood, and animal feces. Lithics are abundant and include both local and exotic toolstones (Méndez et al., 2006). Three 14C dates constrain the occupation to an averaged time span between 2360 and 2700 cal years BP. The occupations at El Toro suggest a very brief time span, with repeated—possibly seasonal—incursions of hunter-gatherers into the densely vegetated forest to seek for local prey (Méndez et al., 2006).
The earliest human presence found in the CRV to date is that recorded at El Chueco 1, consisting of the deposition of a single tool associated with charcoal dated to 11,500 cal years BP (Méndez et al., 2009). These data are consistent with the expectations for an “exploration phase” implying the low visibility, short-span use of sheltered sites with locally procured toolstones (Borrero and Franco, 1997). During the early to middle Holocene, low intensity occupational events were recorded in this site indicating redundant seasonal visits (Méndez et al., 2011). One single date indicates a forest incursion at 6100 cal years BP, as suggested by the evidence at Las Quemas (Méndez et al., 2016). Between 3000 and 2300 cal years BP, hunter-gatherer mobility ranges expanded into open and close-canopy forests to the west of CRV as expressed by the coincidence of occupational events at El Chueco 1, Las Quemas, and El Toro (Figure 5). It has been suggested that this reorganization occurred in response to a decrease in effective moisture which affected resource distribution in the steppe and the forest-steppe ecotone (Méndez and Reyes, 2008; Reyes et al., 2009; de Porras et al., 2012). While the human occupations in Las Quemas occurred in an open-canopy forest, as suggested by the Mallín El Embudo pollen record (de Porras et al., 2014; Méndez et al., 2016), the occupational event at El Toro may have been under similar to current ecological conditions. Forest occupations were brief but recurrent, and possibly seasonal, according to the faunal record at El Toro (Mena et al., 2004). After this incursion into the evergreen and deciduous forests, no other occupations have been detected; this may be interpreted as a retraction of hunter-gatherers into steppe territories and adjacent areas, similar to ethno-historical patterns (Méndez and Reyes, 2008).
Methods: Charcoal Particle Quantification and Archeology
Human agency in fire occurrence (i.e., human beings as igniters of wildfires) is better understood in small spatial (e.g., watershed) and temporal (e.g., fire episodes) scales. Thus, valley scale archeological information and radiocarbon records need to be fully understood on a site-by-site basis. In order to evaluate the potential links between human agency and charcoal production by fire we compare the El Shaman charcoal record (de Porras et al., 2012) with the available archeological record in the upper CRV, and specifically the set of 14C dates and bone/artifact depositions from El Chueco 1 site.
Charcoal particles from the Lake Shaman sedimentary record were quantified by determining low frequency (CHAR base) and high frequency (CHAR peak) components, in order to segregate light-weight particles suspended in years without fires and/or transported by winds from long distances from charcoal particles directly derived from local fire episodes (Long et al., 1998; Whitlock and Larsen, 2001). The macro-charcoal particles (125 and 250 mm) were quantified in 2 cm3 of sediment at contiguous 1 cm-intervals following methods outlined in Whitlock and Larsen (2001). Charcoal concentration (particles cm−3) was calculated from raw data and then interpolated to 31 year bins using Char Analysis software (Higuera et al., 2009); the charcoal accumulation rate (CHAR; particles cm−2yr−1) was then calculated. The magnitudes and frequencies of fire episodes were inferred based on a 1000 year moving window. Thus, the fire record was divided into four major categories based on the fire episode frequency values: null (absent), null (absent) to low (N-L; 0–3 peaks 1000 yr−1), moderate (M; 3–5 peaks 1000 yr−1), and high (H; >5 peaks 1000 yr−1).
Recovery methods at the archeological sites focused on excavating continuous plans to understand horizontal relations, identify features and record the three-dimensional location of all artifacts >3 cm. Distinctive stratigraphic units were recorded through 10-cm artificial excavation levels. All sediments were sieved with 4 mm mesh. We used 14C AMS dates to establish the chronology of human occupations and to understand site formation processes. Chronological saturation at a stratigraphic level was established whenever two or more dates were statistically indistinguishable at α = 0.05. In those cases, 14C results were averaged (Ward and Wilson, 1978) in order not to overestimate the chronological signature and to provide calculated occupational events at the site level (Méndez, 2013).
All of the radiocarbon dates discussed in this paper were calibrated into years before present (cal years BP) with Calib 7.0.0 (Stuiver et al., 2013) applying the ShCal13 curve (Hogg et al., 2013) and are expressed as 2σ. Summed probability plots at site and CRV scales were used for comparing human chronological trends with the charcoal particle dataset. For El Chueco 1 site, an age depth model was produced with OxCal 4.2.4 software (Bronk Ramsey, 2008), also applying the ShCal13 curve (Hogg et al., 2013).
Results
Lake Shaman Charcoal Record
The Lake Shaman sedimentary record is 613 cm long and is broadly composed of silt in the lower ~150 cm, gyttja between 356 and 38 cm and peat in the upper section of the core. This sequence is constrained by eight 14C dates (de Porras et al., 2012). Continuous deposition is indicated since 19,000 cal years BP, with minor sedimentation rate variations and no discontinuities. This depth age model allows us to discuss fire occurrences since the postglacial for the upper CRV. The Lake Shaman record shows the first macro-charcoal particles at 11,500 cal years BP (Figure 5), while fire-episode frequencies are characterized as absent before 12,000 cal years BP and absent to low before 10,500 cal years BP. Moderate fire episode frequencies are observed between 10,500 and 8000 cal years BP and again between 7000 and 3500 cal years BP. High frequencies are found between 3500 cal years BP and the end of the record. In contrast, absent to low fire-episode frequencies were recorded in a constrained period between 8000 and 7000 cal years BP (de Porras et al., 2012). Maximum magnitude fire-episodes (exceeding 2500 particles/cm2/peak) occur only around ~9800, ~6000, ~4200, ~2800, and ~2300 cal years BP.
Periods characterized by major fire occurrence could have been favored by high biomass (fuel) availability associated with high summer temperatures between 10,500 and 9000 cal years BP and by short-scale climatic variability between 3000 and 1200 cal years BP (de Porras et al., 2012). Around 1500 cal years BP a peak in the charcoal record is stratigraphically associated with a decrease in percentages of Nothofagus (dombeyi-type) which indicate a retraction of the forest. Particularly, low Nothofagus values in the pollen record around 1800 cal years BP have been interpreted as a consequence of fire-induced changes in view of the coincidence with the highest fire-episode frequencies for the whole record (de Porras et al., 2012).
Thirteen distinctive tephra layers were recorded in the Lake Shaman sediment core (de Porras et al., 2012). Geochemical trace-element analyses indicate that all the sources for tephra are volcanoes along the Liquiñe-Ofqui fault system (Stern et al., 2015). These volcanoes are located distant enough from Lake Shaman as to rule them out as potential forest fire igniters (Melimoyu volcano >140 km distant; Mentolat volcano >150 km; Hudson volcano >210 km; Figure 1). Ash-cloud lightning due to ongoing volcanic eruptions does occur along the southern Andes, but its incidence on wildfires has been dismissed since eruptions are rare (Garreaud et al., 2014). Moreover, tephra layers do not correlate with fire events or with charcoal peaks throughout the Lake Shaman record (de Porras et al., 2012).
El Chueco 1 Stratigraphy, Chronology, and Human Evidence
El Chueco 1, located 4.9 km south of Lake Shaman is considered as representative of human occupations in the CRV because there are no other habitable cave/rockshelter sites in the area (Reyes et al., 2006). Around 50% of its available surface has been excavated so the retrieved sample should be regarded as a representative proxy for human presence at valley scale (Figure 6). The uppermost stratigraphic zone at El Chueco 1 consists of a semi-uniform sheep dung unit (SU1 in Figure 7). The second stratigraphic unit is of limited extent and characterized by relatively recent bioturbation. Stratigraphic unit 3 is a dark-brown, organic clay layer divided into two areas; a low energy area close to the western profile, with small clasts in low frequencies, and a high energy area with abundant clasts, occasionally expressed as clast-supported flows in the southern and eastern profiles. The fourth stratigraphic unit is an organic dark-brown clay and silt-clay matrix with abundant small clasts, with incipient localized pedogenetic processes. Stratigraphic unit 5 is a very dark brown clay layer with low frequencies of clasts. Anthropogenic deposits are expressed between the lower limit of stratigraphic unit 3 and the lower limit of stratigraphic unit 5, and represent ~11,300 years of redundant human occupations. The lowermost stratigraphic unit (SU6) is expressed only in the western profile (deepest excavation sector) and corresponds to light brown-reddish organic clays.
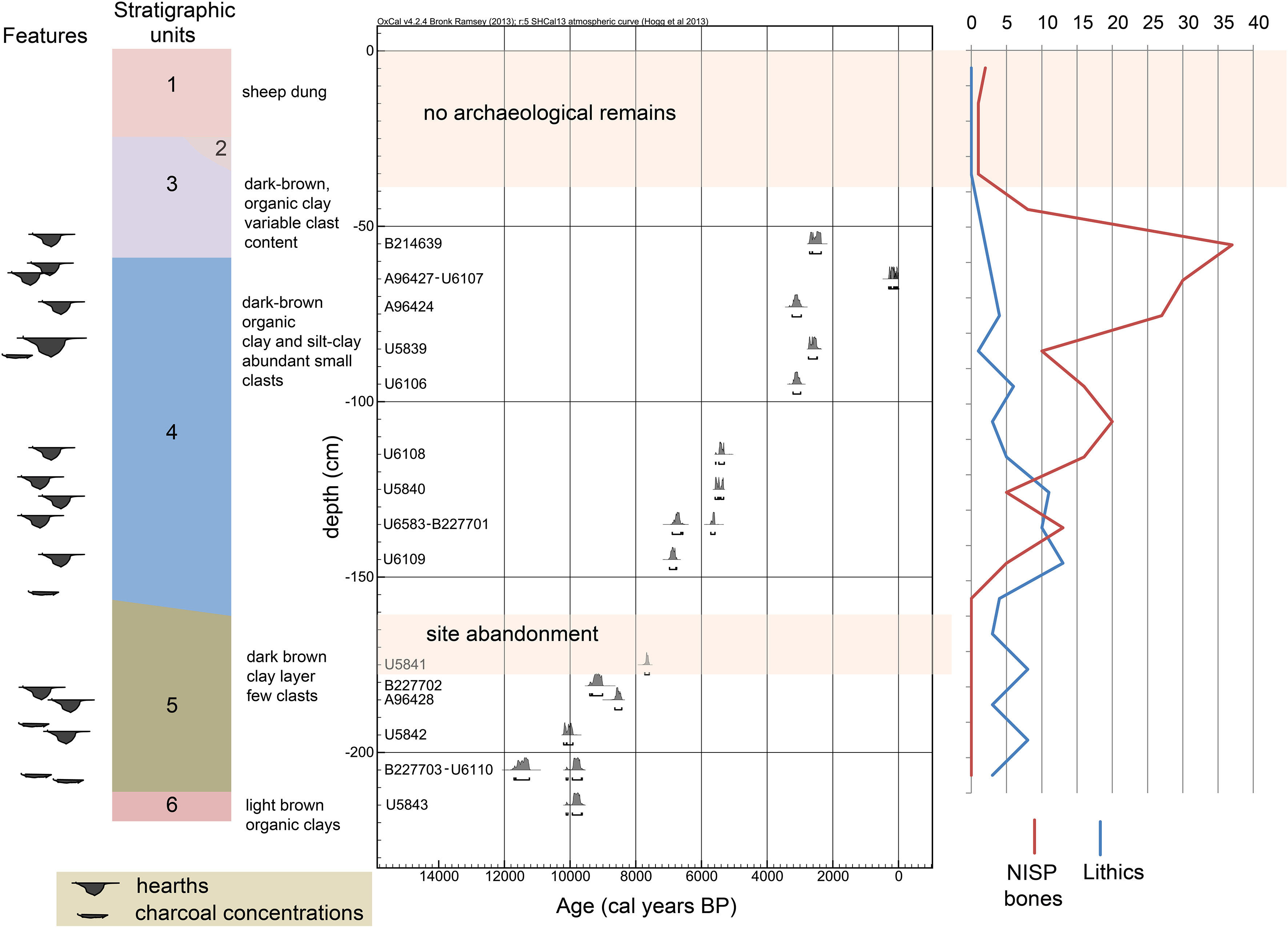
Figure 7. El Chueco 1 schematic stratigraphy, features, 14C age depth plot, and frequencies of lithic artifacts and bone remains (NISP).
The excavated sequence yielded charcoal concentrations of varying size which were labeled as hearths whenever they exhibited high charcoal particle counts, well-defined margins and/or rubified sediments in the base. Other minor concentrations of charcoal or ash were also identified. They total 19 combustion features, the majority of which (78.9%) were located close to the walls and other areas with low exposure. This pattern contrasts with the spatial distribution of >95% of the bone remains which were discarded near the cave entrance.
Based on all available 14C ages we developed a depth/age model for the site and compared it to the deposition rates of lithic artifacts and bone remains. The model shows an ordered stratigraphic succession as had been previously suggested (Reyes et al., 2007a). However, large roof fall particles and differences in depth due to slope may account for some minor inversions (Méndez et al., 2011). Bone remains (Lama guanicoe comprising 58% of total specimens found) and artifact counts are significantly low. While bones total 425 specimens distributed from ~6900 cal years BP onwards, lithics comprise 88 pieces including tools and chipping debris distributed in all occupations since 11,500 cal years BP. These small assemblages are by no means an anomaly, as is indicated by other sites in the region which also yielded scarce archeological evidence (e.g., Velásquez and Mena, 2006; García, 2007; Méndez et al., 2016).
A combination of combustion features, discarded bones and lithics allows us to define nearly continuous archeological deposition defined by low-intensity occupations at El Chueco 1 site. Using 18 14C ages we defined a minimum of 13 occupational events at the site (Figure 5). Only the levels constrained between 7700 and 6930 cal years BP show no combustion features or bone deposition, together with a marked decrease in lithics. Given their small size, lithics are considered more prone to vertical migration and thus their stratigraphic position should not be regarded as in situ deposition, and thus proof of occupation in that time frame. The 7700 cal years BP age is from unburned Festuca sheaf preserved naturally below a rock collapse, which along with the rest of the evidence suggests abandonment of the site for ~800 years (Méndez et al., 2011).
Comparison between Charcoal and Archeological Records
Summed probability plots based on the averaged occupational events at El Chueco 1 site and at valley scale were used for comparing human occupations with fire-episode frequencies and magnitudes reconstructed from the Lake Shaman record (Figure 5). The following was observed from the fire-episode frequencies: (1) despite the availability of fuel, no fires were recorded prior to the onset of human presence in the CRV at 11,500 cal years BP; (2) from 10,500 to 8000 cal years BP, moderate fire frequencies occur, coinciding with redundant, low-intensity occupational events at El Chueco 1 site; (3) between 8000 and 7000 cal years BP, no fire episodes or absent to low fire-episode frequency were recorded, coinciding with no 14C-based occupations and a lack of human-made features, bone remains and artifact deposition at El Chueco 1; (4) at 7000 cal years BP fire episodes reappear at a moderate frequency alongside human signs at El Chueco 1 and other sites in the upper CRV; (5) high fire-episode frequencies were observed concomitant with the occurrence of multiple occupational events in the upper and middle CRV between 3000 and 2300 cal years BP (Méndez and Reyes, 2008; Méndez et al., 2016), and (6) high fire-episode frequencies were observed associated with higher intensity of occupations e.g., larger sites (Velásquez et al., 2007), higher artifact frequencies (Contreras et al., 2016), more site variability (Reyes et al., 2009) including local burials (Reyes and Méndez, 2010), and the use of local lithic resources (Gómez and Méndez, 2015) in the upper CRV between 1500 and 400 cal years BP.
The highest peaks in the macro-charcoal record occur synchronically with different human occupational events. At ~9800 and ~6000 cal years BP, high fire-episode magnitudes coincide with single site occupational events at El Chueco 1 and Las Quemas sites respectively. At ~2800 (the highest fire-episode magnitude in the record) and at ~2300 cal years BP, peaks appear alongside contemporaneous occupations at multiple areas of CRV, signaling the major east–west human expansion recorded in CWP (Méndez and Reyes, 2008). Only one high magnitude fire episode, at ~4200 cal years BP, does not match any archeological occupation record at CRV.
Discussion
Most studies of the relationship between environment and human dynamics have addressed the effects of climate change on human societies. There are fewer studies emphasizing the role that past human populations have had in modifying the territories they inhabited (e.g., Messerli et al., 2000). Consequently, studies of the relationship between human populations and environmental change in Patagonia have examined primarily how changes in climate may have affected population distribution or economic practices (e.g., Franco et al., 2004; McCulloch and Morello, 2009; Morales et al., 2009; Reyes et al., 2009). In forested regions such as CWP, one of the most straightforward ways of assessing the incidence of human actions upon the environment is through the identification of their role in the production of fires. Both climate and humans have been argued as potential drivers in paleofire trends at various temporal and spatial scales for the broader region of Patagonia (e.g., Veblen et al., 1999; Heusser, 2003; Whitlock et al., 2007; Moreno et al., 2012). Heusser (2003) was among the first to propose the primary role of humans in paleofires in Patagonia. Lightning and volcanic eruptions were considered to have a minor role in fire occurrence when compared to the collation between the first appearance of charcoal particles in sedimentary records and the first dated human occupations at a regional scale (Heusser, 1994). Several later papers have challenged this interpretation and attributed the rise in the frequencies of charcoal, and therefore of past fires, to natural causes in Patagonia as a whole (Whitlock et al., 2007) and CWP in particular (Markgraf et al., 2007), considering available combustible biomass as a product of variable and dry periods. However, defining the role of these agents requires not only a detailed climate reconstruction but also an archeological perspective allowing comparison between environmental and human records.
Humans have historically affected the occurrence of wildfires by encouraging or suppressing them (McWethy et al., 2013). Their economic organization and landscape use are crucial in deliberate decisions or involuntary practices, which may or may not activate fires beyond natural fire occurrence. Involuntary burning of huts has been documented ethnographically in southern Patagonia, as well as the deliberate use of fire in communal hunting and other open-air activities (Musters, 1871; Prieto et al., 2011). Likewise, evidences of fire in the earliest human occupations in Patagonia have been acknowledged as significant indicators of human activity at the site scale (Massone, 2002; Frank, 2012).
Cross-regional comparisons by McWethy et al. (2013) suggest that the sensitivity of forests to human-induced wildfires is modulated by the tendency toward natural fires in the environment, effective moisture, and the characteristics of fuel types. Forests are especially sensitive when fires are infrequent, the forest is poorly adapted to fire and combustible biomass is available in large quantities. Given net primary productivity in CWP, wildfires are most likely to occur in the forest-steppe ecotone and in deciduous forests with open canopies, rather than in the highly humid evergreen forests or on open grass steppes where fuel is discontinuous (McWethy et al., 2013; Whitlock et al., 2015).
Paleoecological and glaciological evidence from CWP supports the hypothesis of a position closer to the equator of the Southern Westerlies during the Last Glacial Maximum (LGM), around 41°S (e.g., Moreno et al., 1999; Rojas et al., 2009). This may have limited the availability of combustible biomass, in turn limiting fire occurrence and propagation (Power et al., 2008; Figure 3B). During the Late Glacial, two possible scenarios for the behavior of the Southern Westerlies have been posited for CWP. On the one hand, a gradual increase in moisture levels recorded in terrestrial records supports a southward migration of the Southern Westerlies to their modern position about 1500 years after the LGM termination (Markgraf et al., 1992; McCulloch et al., 2000). On the other hand, the offshore MD07-3088 pollen record indicates a restricted expansion of magellanic moorland in the Taitao Peninsula area during the Antarctic Cold Reversal, which has been interpreted as a northward shift, during the southward migration of the Southern Westerlies from their LGM to their Holocene (similar to modern) position (Montade et al., 2013).
The Lake Shaman pollen record shows the establishment of the forest-steppe ecotone between 11,500 and 8000 cal years BP, suggesting an increase in effective moisture during the beginning of the Holocene, along with an increase in summer temperatures (de Porras et al., 2012). Under this scenario, high biomass availability and higher summer temperatures may have enhanced the availability of combustible fuel during the early Holocene (de Porras et al., 2012). At the regional scale, eastern (Lake Shaman, Mallín El Embudo, Mallín Pollux; Markgraf et al., 2007; Lake Augusta, Villa-Martínez et al., 2012) and western (Chonos archipelago; Haberle and Bennett, 2004) CWP charcoal records indicate that fire-episode frequency in the early Holocene was high. This pattern agrees with most charcoal records south of 40° S, which indicate that widespread fire activity (positive anomalies) between 12,000 and 8000 cal years BP was climate-driven (Whitlock et al., 2007; Power et al., 2008; Moreno et al., 2010a). However, the scattered presence of humans on the eastern flank of the Andes during this period (Méndez, 2013) suggests that from the onset of the Holocene, hunter-gatherers should be also regarded as possible ignition agents contributing to fire activity. Vegetation and charcoal records combined indicate a minor increase in precipitation and/or higher summer temperatures during the early Holocene. Increased precipitation would be a result of weaker and more southerly Southern Westerlies as a consequence of the reduction in the latitudinal temperature gradient driven by higher-than-present insolation in high southern latitudes (Liu et al., 2003; Whitlock et al., 2007; de Porras et al., 2014).
Between 8000 and 3000 cal years BP, the higher-than-present effective moisture is indicated by the Lake Shaman pollen assemblage through the maximum eastward position of the forest during the last deglaciation cycle (de Porras et al., 2012). Fuel was abundant, but the increased effective moisture conditions combined with moderate dry summers may have triggered persistent but low-magnitude fire episodes that did not severely affect the forest-steppe ecotone. Other pollen and charcoal records from CWP suggest wetter-than-present conditions coupled with an unmarked seasonality (moderate, dry summers), matching the increasing values of the smectite/illite+chlorite index recorded in the MD07-3088 core which also suggest a small, gradual increase in precipitation (Siani et al., 2010). The increase in precipitation in CWP is synchronous with that in northern (e.g., Moreno and León, 2003; Abarzúa et al., 2004; Moreno, 2004) and southern Patagonia (e.g., Moreno et al., 2010b). This reflects an intensification of the Southern Westerlies during the middle Holocene as a result of the steepening of the pole-equator ocean gradient around 6000 cal years BP according to climate modeling (Rojas and Moreno, 2011).
A decrease in arboreal taxa in Lake Shaman at 3000 cal years BP reflects a westward displacement of the forest-steppe boundary, suggesting lower effective moisture levels and therefore higher availability of combustible fuel. From 1200 cal years BP onwards, the current vegetation distribution was established under similar-to-modern climatic conditions (de Porras et al., 2012). Environmental records of CWP show high variability after 3000 cal years BP due to the effect of short climatic variability sources, such as the Southern Annular Mode (SAM) or the El Niño Southern Oscillation (ENSO; Haberle and Bennett, 2004; Markgraf et al., 2007; de Porras et al., 2012, 2014; Moreno et al., 2014). Both of these help to alter temperatures and precipitation at seasonal to interannual scales by changing the strength and latitudinal position of the Southern Westerlies (Garreaud et al., 2009). Modern wildfires in CWP are strongly enhanced by a positive phase of SAM associated with a poleward shift in westerly storm tracks and therefore a reduction in precipitation (Garreaud et al., 2009). The positive phase of the Pacific decadal oscillation, as well as positive NINO3 (ENSO index; D'Arrigo et al., 2005), would also have enhanced wildfire occurrence in CWP but only during positive phases of SAM (Holz and Veblen, 2012; Holz et al., 2012b).
During the twentieth century, extensive human occupation of CWP had important consequences for the physical setting at a regional scale through the introduction of exotic plant taxa (Markgraf et al., 2007; de Porras et al., 2012), extensive sheep herding (Martinic, 2005), and the logging and burning of forests to open up productive land. This history of land-use produced changes in the composition and distribution of vegetation such as a westward position of the forest-steppe ecotone as well as a patchy forest distribution (Szeicz et al., 2003; Haberle and Bennett, 2004; Markgraf et al., 2007).
Most Patagonian records are consistent with low human incidence in fire trends, suggesting that human impact on vegetation was limited in magnitude and geographic extent (McWethy et al., 2013). Thus, fuel availability is one of the major factors affecting fire occurrence at large temporal and spatial scales, and fire-conducive climate conditions can be considered as the major driver (Huber et al., 2004; Iglesias and Whitlock, 2014; Iglesias et al., 2015). However, fires cannot be attributed to a single reason, especially not in such a vast area as Patagonia where population distribution is heterogeneous (Borrero, 2004). In fact, charcoal particle records themselves are not indicative of causal mechanisms of fire occurrence (McWethy et al., 2013).
Sandweiss and Quilter (2008, 2012) have recently discussed the fundamental principles of how to evaluate environmental and human interactions through defining collation, correlation, and causation as: “steps in a chain of increasingly significant activities leading to plausible explanations for past events” (Sandweiss and Quilter, 2012:121). The simultaneous occurrence of human and climatic events (collation) is followed by the demonstration of a case of covariance (correlation) and by the often remote possibility of providing a justifiable casual explanation (Sandweiss and Quilter, 2008, 2012). Comparative evidence between the Lake Shaman charcoal record and the archeological data from El Chueco 1 and in the broader CRV are suggestive of the collation of multiple events throughout the Holocene that may be labeled as a correlation. Charcoal particles are first shown in the Lake Shaman record at the time when humans arrived in the CVR, even though there are indications in the pollen record of fuel availability earlier. Although the fuel-rich environment of the early Holocene may have been ideal for fires to occur, peaks of paleofires occur contemporaneously with human occupations. The onset of humid conditions at 8000 cal years BP is synchronic with a millennium of absence to low fire activity, and also the absence of humans at site and valley scale, as indicated not only by the 14C record but also by the lack of archeological deposition and of feature production at El Chueco 1. Although the mid Holocene should be regarded as poor in fire occurrence, peaks in the charcoal record are again associated with occupational events detected within the CRV. The record of the highest frequency in fire episodes around 3000 cal years BP, peaking at ~2800 cal years BP, is coeval with the onset of a distinguishable change in hunter-gatherers' mobility (Méndez and Reyes, 2008). Occupational ranges were extended into the westernmost locations within the CRV probably as consequence of changes in resource distribution and harsher winter seasons in the grass steppes, where hunter-gatherers dwelt more redundantly (Reyes et al., 2009; Méndez and Reyes, 2015). It is difficult to state whether the unintentional opening of the forest canopy due to wildfires during the Late Holocene generated “time/space windows of opportunity” for humans to exploit the forests, or whether the human population burned the forest deliberately for that purpose. The onset of modern climatic conditions after 1200 cal years BP is broadly associated with a widespread use of the forest-steppe ecotone and grass steppe in higher intensities. Combustible fuel was variable, and high fire frequencies are indicated for the whole period.
Not all areas of CWP present similar trends between paleofire records and archeological evidence, which we think responds to the complex climate-fire-vegetation relationships occurring in the different vegetation units (Whitlock et al., 2010) coupled with the sustained or episodic presence of human beings. For instance charcoal particles in Laguna Oprasa and Laguna Facil records in the Los Chonos archipelago appear from ~17,000 and ~16,000 cal years BP respectively (Haberle and Bennett, 2004). However, 14C evidence associated with human presence in the same area starts at 5700 cal years BP (Reyes et al., 2015; Figure 3C). Peaks and troughs in these records do not occur in phase with the shifts in archeological dates recorded in the archipelago, which suggest higher human densities after 2000 cal years BP (Méndez and Reyes, 2015). Similarly, the Mallín Casanova charcoal record in the Baker River Valley shows peaks between 11,000 and 7000 cal years BP, in an area and a time period where archeological records are absent (Holz et al., 2012a). In the CRV, Las Quemas rockshelter in the deciduous forest shows a distinctively burnt stratigraphic unit dated at 12,070–11,810 cal years BP (Figure 4C) which coincides with the maximum magnitude fire-episode detected in the Mallín El Embudo core (de Porras et al., 2014), located only 13.5 km east of the site. This has been interpreted as a natural fire episode since there is no evidence of human intervention either at the site or in the immediate vicinity (Méndez et al., 2016). On the other hand, Mallín Pollux shows extremely high fire activity between 10,500 and 9500 cal years BP, associated with a period of dry summers and high combustible fuel availability (Markgraf et al., 2007). Remarkably, within this time frame, the Baño Nuevo 1 site in the Ñirehuao River Valley, located 50 km away from Mallín Pollux, yielded a high intensity of human presence, including the evidence for the earliest concentration of burials in Patagonia (Mena and Stafford, 2006; Reyes et al., 2012). Whether this constitutes a collation of events needs to be further tested using the analytical approach presented here.
For North Western Patagonia, Iglesias and Whitlock (2014) have proposed that the mismatch between the highest regional fire activity between 3000 and 1900 cal years BP and population increase at local and regional scales implies that human use of fire was not linearly dependent on population density. In the CRV there is no indication of a population increase at 3000 cal years BP based on the identification of occupational events at the local scale. In this case, distributional changes in the archeological record have been interpreted as modifications in mobility and the differential use of biomes between 3000 and 2300 cal years BP. The increase in local populations, as suggested by the archeological record, occurs at ~1500 cal years BP and is coeval with the peak in fire activity (Figure 5).
Our data supports the hypothesis of McWethy et al. (2013), which suggests that abundant and continuous fine fuels promote high fire activity in intermediate-productivity/moisture settings, such as the forest-steppe ecotone present in the CRV. Human action may alter the timing of fires but does little to increase fire frequency in these fire-prone environments. We do not argue here that burning by past Patagonian hunter-gatherers was a deliberate strategy to modify the landscape or prey availability. It cannot be determined at this point whether burning was a deliberate strategy in CWP. Rather we observe the correlation of multiple events between paleofire and archeological records at a local spatial scale, which implies that both climate and humans played a role in the occurrence of past fires. Changes in vegetation and landscape due to fire may have influenced a reconfiguration of mobility and use of space by humans along the CRV. Thus, the difficulties for human displacement posed by the abrupt topography of the CRV and the low landmark visibility in the closed canopy forest of CWP may have been counteracted by a high fire-episode frequency as occurred during the late Holocene.
Conclusions
The role of humans and climate in fire patterns in southern South America is still not well resolved because of the lack of detailed archeological surveys of some regions and our incomplete chronological understanding of the variations linked to population dynamics. Although climate provides the main control for widespread occurrence and maintenance of fires at large spatial and temporal scales, in cases such as the eastern Andean flank of CWP where there is a combination of climate-limited and fuel-limited fire regimes both the unintentional spread of human campfires and deliberate burning can be considered as potential contributors to wildfires. In this paper we have attempted to distinguish collation, correlation, and causation between the human and paleofire records at the scale of the CRV. The results are far from proving causality, but they provide a defensible case of correlation between two comparable sets of data. In order to assess potential causal relations we would need to move beyond this initial correlation by working on new valleys sharing the same intermittent occupational mode as that described for CRV and the sites identified there.
If we consider Holocene population dynamics, the easternmost section of the CRV, like most of the forest-steppe ecotone of CWP, can be understood as marginal in a geographical sense (Borrero, 2004). It lies distant from the demographic nuclei to the east (e.g., Miotti and Salemme, 2004; Prates et al., 2013), as suggested by artifact and site frequencies, artifact depositional rates and the 14C data. We propose that areas with infrequent archeological records, although used repeatedly and intermittently, are appropriate for measuring human/environment interactions because such low human densities are highly sensitive for showing the alternation between occupation and abandonment at different scales. This study shows that at small spatial scales there is a strong correlation between indicators of human presence and fire activity in the CRV, and that at different points throughout the Holocene, especially during periods of high fuel availability, humans may have contributed to the onset of wildfires. In this case, human beings can be indicated as potential agents in the transformation of the landscapes they inhabited, probably not by deliberate action.
Author Contributions
CM conceived the idea, conducted archeological surveys and excavations, performed analyses, and wrote the manuscript; MD and AM collected the sedimentary cores and performed pollen and charcoal analysis; OR and AN conducted archeological surveys and excavations; JG performed geomorphological analysis. All authors contributed to writing this article.
Funding
Funded by FONDECYT 1130128 grant. The NSF Arizona AMS Facility at the University of Arizona provided partial support with three radiocarbon dates. CM and JG received support from INQUA for attending the XIX INQUA Congress in Nagoya.
Conflict of Interest Statement
The authors declare that the research was conducted in the absence of any commercial or financial relationships that could be construed as a potential conflict of interest.
Acknowledgments
An earlier version of the article was presented in the “Identifying human activity in palaeoecological records” session at the XIX INQUA Congress in Nagoya, Japan 2015. We thank the organizers Simon Haberle, Alistair Seddon, and David McWethy for the invitation to participate in this Research Topic. We thank Estancia Río Cisnes and Stephanie Buckaert for granting permission for our archeological research. We thank Mauricio González for constructing Figure 2 and Bárbara Thompson and William Barne for providing editorial assistance.
Footnotes
References
Abarzúa, A. M., Villagrán, C., and Moreno, P. I. (2004). Deglacial and postglacial climate history in east-central Isla Grande de Chiloé, southern Chile (43° S). Quat. Res. 62, 49–59. doi: 10.1016/j.yqres.2004.04.005
Alaback, P., Veblen, T. T., Whitlock, C., Lara, A., Kitzberger, T., and Villalba, R. (2003). “Climatic and human influences on fire regimes in temperate forest ecosystems in North and South America,” in How Landscapes Change, Human Disturbance and Ecosystem Fragmentation in the Americas, eds G. A. Bradshaw and P. A. Marquet (Verlag: Springer), 49–87.
Anderson, G., Sandweiss, D., and Maasch, K. A. (2007). Climate Change and Cultural Dynamics: A Global Perspective on Holocene Transitions. San Diego, CA: Academic Press.
Bate, L. F., and Mena, F. (2005). “Alero El Toro: un campamento indígena en el bosque siempreverde cercano al litoral en Aisén,” in Actas del XVI Congreso Nacional de Arqueología Chilena, ed M. Massone (Concepción: Sociedad Chilena de Arqueología and Museo de Historia Natural de Concepción), 675–680.
Bliege Bird, R., Codding, B. F., Kauhanen, P. G., and Bird, D. W. (2012). Aboriginal hunting buffers climate-driven fire-size variability in Australia's spinifex grasslands. Proc. Natl. Acad. Sci. U.S.A. 109, 10287–10292. doi: 10.1073/pnas.1204585109
Borrero, L., and Franco, N. (1997). Early Patagonian hunter-gatherers: subsistence and technology. J. Anthropol. Res. 57, 219–239. doi: 10.1086/jar.53.2.3631277
Borrero, L. A. (2004). “The archaeozoology of Andean ‘dead ends’ in Patagonia: living near the continental Ice Cap,” in Colonisation, Migration and Marginal Areas. A Zooarchaeological Approach, eds M. Mondini, A. S. Muñoz, and S. Wickler (Oakville, ON: Oxbow Books), 55–61.
Bronk Ramsey, C. (2008). Deposition models for chronological records. Quat. Sci. Rev. 27, 42–60. doi: 10.1016/j.quascirev.2007.01.019
Contreras, C., Méndez, C., and Reyes, O. (2016). Tecnología lítica de cazadores recolectores en la estepa aysenina. Gestión de recursos y organización espacial en los valles de los ríos Cisnes y Simpson. Magallania 44, 167–185. doi: 10.4067/S0718-22442016000100010
D'Arrigo, R., Cook, E. R., Wilson, R. J., and Mann, M. E. (2005). On the variability of ENSO over the past six centuries. Geophys. Res. Lett. 32:L03711. doi: 10.1029/2004GL022055
De la Cruz, R., and Cortes, J. (2011). Geología del Área Oriental de la Hoja Puerto Cisnes. Región de Aysén del General Calos Ibañez del Campo. Santiago: SERNAGEOMIN.
de Porras, M. E., Maldonado, A., Abarzúa, A. M., Cárdenas, M., Francois, J. P., Martel-Cea, A., et al. (2012). Postglacial vegetation, fire and climate dynamics at Central Chilean Patagonia (Lake Shaman, 44°S), Chile. Quat. Sci. Rev. 50, 71–85. doi: 10.1016/j.quascirev.2012.06.015
de Porras, M. E., Maldonado, A., Quintana, F., Martel-Cea, A., Reyes, O., and Méndez, C. (2014). Environmental and climatic changes at central Chilean Patagonia since the Late Glacial (Mallín El Embudo, 44°S). Clim. Past 10, 1063–1078. doi: 10.5194/cp-10-1063-2014
Dincauze, D. (2000). Environmental Archaeology. Principles and Practice. Cambridge: Cambridge University Press.
Franco, N. V., Borrero, L. A., and Mancini, M. V. (2004). Environmental changes and hunter-gatherers in southern Patagonia: Lago Argentino and Cabo Vírgenes (Argentina). Before Farm. 3, 1–17. doi: 10.3828/bfarm.2004.3.3
Frank, A. (2012). Los fogones en la meseta central de Santa Cruz durante el Pleistoceno Final. Magallania 40, 145–162. doi: 10.4067/S0718-22442012000100009
Fuentes, F., Mena, F., Blanco, J., and Contreras, C. (2012). Excavaciones en Alero Gianella, curso medio del valle de Chacabuco (Andes Centro Patagónicos). Magallania 40, 259–265. doi: 10.4067/S0718-22442012000200013
García, C. (2007). “Conjuntos líticos y estrategias tecnológicas de las ocupaciones humanas de la cuenca de Baño Nuevo-1 (XI Región de Aisén, Chile),” in Arqueología de Fuego-Patagonia. Levantando Piedras, Desenterrando Huesos Develando Arcanos, eds F. Morello, M. Martinic, A. Prieto, and G. Bahamonde (Punta Arenas: CEQUA), 493–502
Garreaud, R. D. (2009). The Andes climate and weather. Adv. Geosci. 22, 3–11. doi: 10.5194/adgeo-22-3-2009
Garreaud, R. D., Nicora, M. G., Bürgesser, R. E., and Ávila, E. E. (2014). Lightning in Western Patagonia. J. Geophys. Res. 119, 1–15. doi: 10.1002/2013jd021160
Garreaud, R. D., Vuille, M., Compagnucci, R., and Marengo, J. (2009). Present-day South American climate. Palaeogeogr. Palaeoclimatol. Palaeoecol. 281, 180–195. doi: 10.1016/j.palaeo.2007.10.032
Gómez, M. L., and Méndez, C. (2015). “Aprovisionamiento lítico en Alto Río Cisnes, el caso del sitio La Cantera 1,” in Actas del XIX Congreso Nacional de Arqueología Chilena, eds M., Sepúlveda, C. Alday, C. Castillo, and A. Oyaneder (Arica: Sociedad Chilena de Arqueología and Universidad de Tarapacá), 577–584.
González, M. E., and Veblen, T. T. (2006). Climatic influences on fire in Araucaria araucana- Nothofagus forests in the Andean cordillera of south central Chile. Ecoscience 13, 342–350. doi: 10.2980/i1195-6860-13-3-342.1
Grosjean, M., Cartajena, I., Geyh, M. A., and Nuñez, L. (2003). From proxy data to paleoclimate interpretation: the mid-Holocene paradox of the Atacama Desert, northern Chile. Palaeogeogr. Palaeoclimatol. Palaeoecol. 194, 247–258. doi: 10.1016/S0031-0182(03)00280-3
Haberle, S. G., and Bennett, K. D. (2001). Modern pollen rain and lake mud-water interface geochemistry along environmental gradients in southern Chile. Rev. Palaeobot. Palynol. 117, 93–107. doi: 10.1016/S0034-6667(01)00079-3
Haberle, S. G., and Bennett, K. D. (2004). Postglacial formation and dynamics of North Patagonian Rainforest in the Chonos Archipelago, Southern Chile. Quat. Sci. Rev. 23, 2433–2452. doi: 10.1016/j.quascirev.2004.03.001
Heusser, C. J. (1994). Paleoindians and fire during the late Quaternary in southern South America. Rev. Chil. Hist. Nat. 67, 435–443.
Heusser, C. J. (1995). Three Late Quaternary pollen diagrams from Southern Patagonia and their palaeoecological implications. Palaeogeogr. Palaeoclimatol. Palaeoecol. 118, 1–24. doi: 10.1016/0031-0182(94)00138-X
Heusser, C. J. (2003). Ice Age Southern Andes: A Chronicle of Paleoecological Events. Amsterdam: Elsevier.
Higuera, P. E., Brubaker, L. B., Anderson, P. M., Hu, F. S., and Brown, T. A. (2009). Vegetation mediated the impacts of postglacial climate change on fire regimes in the south-central Brooks Range, Alaska. Ecol. Monogr. 79, 201–219. doi: 10.1890/07-2019.1
Hogg, A., Hua, Q., Blackwell, P., Niu, M., Buck, C., Guilderson, T., et al. (2013). SHCAL13 Southern hemisphere calibration, 0-50,000 years CAL BP. Radiocarbon 55, 1889–1903. doi: 10.2458/azu_js_rc.55.16783
Holz, A., Haberle, S. G., Veblen, T., De Pol-Holz, R., and Southon, J. (2012a). Fire history in western Patagonia from paired tree-ring fire-scar and charcoal records. Clim. Past 8, 451–466. doi: 10.5194/cp-8-451-2012
Holz, A., Kitzberger, T., Paritsis, J., and Veblen, T. T. (2012b). Ecological and climatic controls of modern wildfire activity patterns across southwestern South America. Ecosphere 3, 1–25. doi: 10.1890/ES12-00234.1
Holz, A., and Veblen, T. T. (2012). Wildfire activity in rainforests in western Patagonia linked to the Southern Annular Mode. Int. J. Wildland Fire 21, 114–126. doi: 10.1071/WF10121
Huber, U. M., Markgraf, V., and Schäbitz, F. (2004). Geographical and temporal trends in Late Quaternary fire histories of Fuego-Patagonia, South America. Quat. Sci. Rev. 23, 1079–1097. doi: 10.1016/j.quascirev.2003.11.002
Iglesias, V., and Whitlock, C. (2014). Fire responses to postglacial climate change and human impact in northern Patagonia (41-43°S). Proc. Natl. Acad. Sci. U.S.A. 111, E5545–E555. doi: 10.1073/pnas.1410443111
Iglesias, V., Yospin, G. I., and Whitlock, C. (2015). Reconstruction of fire regimes through integrated paleoecological proxy data and ecological modeling. Front. Plant Sci. 5:785. doi: 10.3389/fpls.2014.00785
Kitzberger, T., and Veblen, T. (2003). “Influences of climate on fire in northern Patagonia, Argentina,” in Fire and Climatic Changes in Temperate Ecosystems of the Western Americas, eds T. Veblen, W. Baker, G. Montenegro, and T. Swetnam (New York, NY: Springer-Verlag), 290–315.
Lara, A., Wolodarsky-Franke, A., Aravena, J., Cortés, M., Fraver, S., and Silla, F. (2003). “Fire regimes and forest dynamics in the Lake Region of south-central Chile,” in Fire and Climatic Change in Temperate Ecosystems of the Western Americas, eds T. Veblen, W. Baker, G. Montenegro, T. Swetnam (New York, NY: Springer-Verlag), 322–342.
Liu, J., Schmidt, G. A., Martinson, D., Rind, D. H., Russell, G. L., and Yuan, X. (2003). Sensitivity of sea ice to physical parameterizations in the GISS global climate model. J. Geophys. Res. 108, 3053. doi: 10.1029/2001jc001167
Long, C. J., Whitlock, C., Bartlein, P. J., and Millspaugh, S. H. (1998). A 9000-year fire history from the Oregon Coast Range, based on a high-resolution charcoal study. Can. J. For. Res. 28, 774–787. doi: 10.1139/x98-051
Luebert, F., and Pliscoff, P. (2006). Sinopsis Bioclimática y Vegetacional de Chile. Santiago: Editorial Universitaria.
Markgraf, V., and Anderson, L. (1994). Fire history of Patagonia: climate versus human cause. Rev. Inst. Geogr. Sao Paulo 15, 33–47. doi: 10.5935/0100-929x.19940004
Markgraf, V., Dodson, J. R., Kershaw, A. P., McGlone, M. S., and Nicholls, N. (1992). Evolution of late Pleistocene and Holocene climates in the circum-South Pacific land areas. Clim. Dyn. 6, 193–211. doi: 10.1007/BF00193532
Markgraf, V., Whitlock, C., and Haberle, S. G. (2007). Vegetation and fire history during the last 18,000 cal yr B.P. in Southern Patagonia: Mallín Pollux, Coyhaique, Province Aisén (45°41′30″ S, 71°50′30″ W, 640 m elevation). Palaeogeogr. Palaeoclimatol. Palaeoecol. 254, 492–507. doi: 10.1016/j.palaeo.2007.07.008
Martinic, M. (2005). De la Trapananda al Aysén: una Mirada Reflexiva Sobre el Acontecer de la Región de Aysén Desde la Prehistoria Hasta Nuestros Días. Santiago: Pehuén Editores.
Massone, M. (2002). El fuego de los cazadores Fell 1 a fines del Pleistoceno. Anales Instituto Patagonia, Serie Ciencias Humanas 17, 47–60.
McCulloch, R., and Morello, F. (2009). “Evidencia glacial y paleoecológica de ambientes tardiglaciales y del Holoceno temprano. Implicaciones para el poblamiento temprano de Tierra del Fuego,” in Arqueología de Patagonia: Una Mirada Desde el Último Confín, eds M. Salemme, F. Santiago, M. Álvarez, E. Piana, M. Vázquez, and M. E. Mansur (Ushuaia: Editorial Utopías), 119–136.
McCulloch, R. D., Bentley, M. J., Purves, R. S., Hulton, N. R. J., Sugden, D. E., and Clapperton, C. M. (2000). Climatic inferences from glacial and palaeoecological evidence at the last glacial termination, southern South America. J. Quat. Sci. 15, 409–417. doi: 10.1002/1099-1417(200005)15:4<409::AID-JQS539>3.0.CO;2-#
McWethy, D. B., Higuera, P. E., Whitlock, C., Veblen, T. T., Browman, D. M. J. S., Cary, G. J., et al. (2013). A conceptual framework for predicting temperate ecosystem sensitivity to human impacts on fire regimes. Glob. Ecol. Biogeogr. 22, 900–912. doi: 10.1111/geb.12038
McWethy, D. B., Wilmshurst, J., Whitlock, C., Wood, J. R., and McGlone, M. S. (2014). A high-resolution chronology of rapid forest transitions following Polynesian arrival in New Zealand. PLoS ONE 9:328. doi: 10.1371/journal.pone.0111328
Mena, F. (2000). Un panorama de la prehistoria de Aisén oriental; estado del conocimiento a fines del siglo. Rev. Ser. Antropol. 2, 21–41.
Mena, F., Reyes, O., Stafford, T., and Southon, J. (2003). Early human remains from Baño Nuevo-1 (Central Patagonian Andes; Chile). Quat. Int. 109–110, 113–121. doi: 10.1016/S1040-6182(02)00207-0
Mena, F., and Stafford, T. (2006). “Contexto estratigráfico y fechación directa de esqueletos humanos del Holoceno Temprano en Cueva Baño Nuevo 1 (Patagonia Central, Chile),” in Segundo Simposio Internacional del Hombre Temprano en América, eds J. Jiménez, S. González, J. Pompa, and F. Ortíz (Ciudad de México: INAH), 139–154.
Mena, F., Velásquez, H., Trejo, V., and Torres-Mura, J. (2004). “Aproximaciones zooarqueológicas al pasado de Aisén continental (Patagonia central chilena),” in Zooarchaeology of South America, ed G. Mengoni (Oxford: British Archaeological Reports International Series 1298), 99–122.
Méndez, C. (2013). Terminal Pleistocene/early Holocene 14C dates form archaeological sites in Chile: discussing critical chronological issues for the initial peopling of the region. Quat. Int. 301, 60–73. doi: 10.1016/j.quaint.2012.04.003
Méndez, C., Barberena, R., Reyes, O., and Nuevo Delaunay, A. (2014). Isotopic ecology and human diets in the forest-steppe ecotone, Aisén Region, Central-Western Patagonia, Chile. Int. J. Osteoarchaeol. 24, 187–201. doi: 10.1002/oa.2337
Méndez, C., and Reyes, O. (2006). Nuevos datos de la ocupación humana en la transición bosque estepa en Patagonia: Alero Las Quemas (Comuna de Lago Verde, XI Región de Aisén). Magallania 34, 161–165. doi: 10.4067/S0718-22442006000100011
Méndez, C., and Reyes, O. (2008). Late Holocene human occupation of Patagonian forests: a case study at Cisnes river basin (44° S, Chile). Antiquity 82, 560–570. doi: 10.1017/S0003598X00097222
Méndez, C., and Reyes, O. (2015). Archaeology near the southern Ice-End. Current advances in human interdisciplinary research at Central Western Patagonia. SAA Archaeol. Rec. 15, 21–26.
Méndez, C., Reyes, O., Maldonado, A., and Francois, J. P. (2009). “Ser humano y medio ambiente durante la transición Pleistoceno Holoceno en las cabeceras del río Cisnes (~44° S),” in Arqueología de Patagonia: Una Mirada Desde el Último Confín, eds M. Salemme, F. Santiago, M. Álvarez, E. Piana, M. Vázquez, and M. E. Mansur (Ushuaia: Editorial Utopías), 75–83.
Méndez, C., Reyes, O., Nuevo Delaunay, A., Trejo, V., Barberena, R., and Velásquez, H. (2011). Ocupaciones humanas en la margen occidental de Patagonia Central: eventos de poblamiento en Alto Río Cisnes. Magallania 39, 223–242. doi: 10.4067/S0718-22442011000200016
Méndez, C., Reyes, O., Nuevo Delaunay, A., Velásquez, H., Trejo, V., Hormazábal, N., et al. (2016). Las Quemas rockshelter: understanding human occupations of Andean forests of Central Patagonia (Aisén, Chile), Southern South America. Latin Am. Antiquity 27, 207–226. doi: 10.7183/1045-6635.27.2.207
Méndez, C., Reyes, O., Velásquez, H., and Maldonado, A. (2010). Comentario sobre una edad 14C en el límite Pleistoceno/Holoceno de alero El Toro, bosque siempreverde de Aisén. Magallania 38, 281–286. doi: 10.4067/S0718-22442010000100017
Méndez, C., Stern, C., Reyes, O., and Mena, F. (2012). Early Holocene long-distance obsidian transport in Central-South Patagonia. Chung. Rev. Antropol. Chil. 44, 363–375. doi: 10.4067/s0717-73562012000300001
Méndez, C., Velásquez, H., Reyes, O., and Trejo, V. (2006). Tras los moradores del bosque. Análisis de los conjuntos arqueológicos de Alero El Toro (Valle del río Cisnes, Región de Aisén). Werken 8, 101–115.
Messerli, B., Grosjean, M., Hofer, T., Nuñez, L., and Pfister, C. (2000). From nature-dominated to human-dominated environmental changes. Quat. Sci. Rev. 19, 459–479. doi: 10.1016/S0277-3791(99)00075-X
Miotti, L., and Salemme, M. (2004). Poblamiento, movilidad y territorios entre las sociedades cazadoras-recolectoras de Patagonia. Complutum 15, 177–206.
Montade, V., Combourieu Nebout, N., Kissel, C., Haberle, S. G., Siani, G., and Michel, E. (2013). Vegetation and climate changes during the last 22,000 yr from a marine core near Taitao Peninsula, southern Chile. Palaeogeogr. Palaeoclimatol. Palaeoecol. 369, 335–348. doi: 10.1016/j.palaeo.2012.11.001
Morales, M., Barberena, R., Belardi, J. B., Borrero, L. A., Cortegoso, V., Durán, V., et al. (2009). Reviewing human-environment interactions in arid regions of southern South America during the past 3000 years. Palaeogeogr. Palaeoclimatol. Palaeoecol. 281, 283–295. doi: 10.1016/j.palaeo.2008.09.019
Moreno, P. I. (2004). Millennial-scale climate variability in northwest Patagonia over the last 15000 yr. J. Quat. Sci. 19, 35–47. doi: 10.1002/jqs.813
Moreno, P. I., Francois, J. P., Moy, C. M., and Villa-Martínez, R. (2010b). Covariability of the Southern Westerlies and atmospheric CO2 during the Holocene. Geology 38, 727–730. doi: 10.1130/G30962.1
Moreno, P. I., Kitzberger, T., Iglesias, V., and Holz, A. (2010a). Paleofires in southern South America since the Last Glacial Maximum. PAGES News 18, 75–77.
Moreno, P. I., and León, A. (2003). Abrupt vegetation changes during the last glacial to Holocene transition in mid-latitude South America. J. Quat. Sci. 18, 787–800. doi: 10.1002/jqs.801
Moreno, P. I., Lowell, T. V., Jacobson, G. L., and Denton, G. H. (1999). Abrupt vegetation and climate changes during the last glacial maximum and last termination in the Chilean Lake District: a case study from Canal de La Puntilla (41°S). Geogr. Ann. 81A, 285–311. doi: 10.1111/j.0435-3676.1999.00059.x
Moreno, P. I., Vilanova, I., Villa-Martínez, R., Garreaud, R. D., Rojas, M., and De Pol-Holz, R. (2014). Southern Annular Mode-like changes in southwestern Patagonia at centennial timescales over the last three millennia. Nat. Commun. 5, 4375. doi: 10.1038/ncomms5375
Moreno, P. I., Villa-Martínez, R., Cárdenas, M. L., and Sagredo, E. A. (2012). Deglacial changes of the southern margin of the southern westerly winds revealed by terrestrial records from SW Patagonia (52° S). Quat. Sci. Rev. 41, 1–21. doi: 10.1016/j.quascirev.2012.02.002
Moss, M., Peteet, D., and Whitlock, C. (2007). “Mid-Holocene culture and climate on the Northwest Coast of North America,” in Climate Change and Cultural Dynamics, eds D. Anderson, K. Maasch, and D. Sandweiss (New York, NY: Academic Press), 491–529. doi: 10.1016/b978-012088390-5.50019-4
Musters, G. C. (1871). At Home with the Patagonians. A Year's Wanderings over Untrodden Ground from the Straits of Magellan to the Rio Negro. London: John Murray.
Power, M. J., Marlon, J., Ortiz, N., Bartlein, P. J., Harrison, S. P., Mayle, F. E., et al. (2008). Changes in fire regimes since the Last Glacial Maximum: an assessment based on a global synthesis and analysis of charcoal data. Clim. Dyn. 30, 887–907. doi: 10.1007/s00382-007-0334-x
Prates, L., Politis, G., and Steele, J. (2013). Radiocarbon chronology of the early human occupation of Argentina. Quat. Int. 301, 104–122. doi: 10.1016/j.quaint.2013.03.011
Prieto, A., Morano, C., and Massone, M. (2011). Clima, fuego y humanos en América austral. Rev. Arqueol. Am. 29, 7–26.
Reyes, O. (2002). Funebria indígena en el curso inferior del valle del río Ibáñez, margen occidental de la estepa centropatagónica (XI Región de Aisén). Anal. Inst. Patag. 30, 87–101.
Reyes, O., and Méndez, C. (2010). Precisando la cronología para la inhumación tipo chenque, valle del río Cisnes (Aisén, Chile), Patagonia Central. Magallania 38, 97–102. doi: 10.4067/S0718-22442010000200008
Reyes, O., Méndez, C., Maldonado, A., Velásquez, H., Trejo, V., Cárdenas, M., et al. (2009). Uso del espacio de cazadores recolectores y paleoambiente Holoceno en el valle del río Cisnes, región de Aisén, Chile. Magallania 37, 91–107. doi: 10.4067/S0718-22442009000200006
Reyes, O., Méndez, C., Mena, F., and Moraga, M. (2012). “The bioanthropological evidence of a ca. 10,000 CALYBP ten-individual group from Central Patagonia,” in Southbound: Late Pleistocene Peopling of Latin America, eds L. Miotti, M. Salemme, N. Flegenheimer, and T. Goebel (Texas: Center for the Study of the First Americans), 39–44.
Reyes, O., Méndez, C., Trejo, V., and Velásquez, H. (2007a). El Chueco 1: un asentamiento multicomponente en la estepa occidental de Patagonia Central (11400 a 2700 años cal ap, 44° s). Magallania 35, 107–119. doi: 10.4067/S0718-22442007000100007
Reyes, O., Méndez, C., Velásquez, H., and Trejo, V. (2006). Distribuciones espaciales y contextos arqueológicos de cazadores recolectores esteparios en Alto río Cisnes (XI Región de Aisén). Magallania 34, 75–90. doi: 10.4067/S0718-22442006000200008
Reyes, O., Méndez, C., Velásquez, H., and Trejo, V. (2007b). Ocupaciones humanas tardías en la transición bosque estepa: la localidad de Winchester (curso alto del Rio Cisnes, XI Región de Aisén). Magallania 35, 145–150. doi: 10.4067/S0718-22442007000200011
Reyes, O., Moraga, M., Méndez, C., and Cherkinsky, A. (2015). Maritime hunter-gatherers in the Chonos Archipelago (43°50′ - 46°50′ S), Western Patagonian Channels. J. I. Coast. Archaeol. 10, 207–231. doi: 10.1080/15564894.2014.1001920
Rick, T., Wah, J., and Erlandson, J. (2012). Re-evaluating the origins of late Pleistocene fire areas on Santa Rosa Island, California, USA. Quat. Res. 78, 353–362. doi: 10.1016/j.yqres.2012.06.006
Rojas, M., and Moreno, P. I. (2011). Atmospheric circulation changes and neoglacial conditions in the Southern Hemisphere mid-latitudes: insights from PMIP2 simulations at 6 kyr. Clim. Dyn. 37, 357–375. doi: 10.1007/s00382-010-0866-3
Rojas, M., Moreno, P. I., Kageyama, M., Crucifix, M., Hewitt, C., Abe-Ouchi, A., et al. (2009). The Southern Westerlies during the last glacial maximum in PMIP2 simulations. Clim. Dyn. 32, 525–548. doi: 10.1007/s00382-008-0421-7
Sandweiss, D. H., and Quilter, J. (2008). “Climate, catastrophe, and culture in the ancient Americas,” in El Niño, Catastrophism, and Culture Change in Ancient America, eds D. Sandweiss and J. Quilter (Washington D.C.: Dumbarton Oaks), 1–11.
Sandweiss, D. H., and Quilter, J. (2012). “Collation, correlation, and causation in the prehistory of coastal Peru,” in Surviving Sudden Environmental Change, eds J. Cooper and P. Sheets (Boulder, CO: University Press of Colorado), 117–139.
Siani, G., Colin, C., Michel, E., Carel, M., Richter, T., Kissel, C., et al. (2010). Late Glacial to Holocene terrigenous sediment record in the Northern Patagonian margin: paleoclimate implications. Palaeogeogr. Palaeoclimatol. Palaeoecol. 297, 26–36. doi: 10.1016/j.palaeo.2010.07.011
Stahlschmidt, M., Miller, C., Ligouisc, B., Hambach, U., Goldberg, P., Berna, F., et al. (2015). On the evidence for human use and control of fire at Schöningen. J. Hum. Evol. 89, 181–201. doi: 10.1016/j.jhevol.2015.04.004
Stern, C. R., de Porras, M. E., and Maldonado, A. (2015). Tephrochronology of the upper Río Cisnes valley (44°S), southern Chile. And. Geol. 42, 173–189. doi: 10.5027/andgeoV42n2-a02
Stuiver, M., Reimer, P., and Reimer, R. (2013). CALIB 7.0.4. Available online at: http://calib.qub.ac.uk/calib/ (Accessed May 01, 2015).
Szeicz, J., Haberle, S. G., and Bennett, K. D. (2003). Dynamics of North Patagonian rainforests from fine-resolution pollen, charcoal and tree-ring analysis, Chonos Archipelago, Southern Chile. Aust. Ecol. 28, 413–422. doi: 10.1046/j.1442-9993.2003.01299.x
Veblen, T. T., Kitzberger, T., Villalba, R., and Donnegan, J. (1999). Fire history in northern Patagonia: the roles of humans and climatic variation. Ecol. Monogr. 69, 47–67. doi: 10.1890/0012-9615(1999)069[0047:FHINPT]2.0.CO;2
Velásquez, H., and Mena, F. (2006). Distribuciones óseas de ungulados en la cueva Baño Nuevo-1 (XI Región, Chile): un primer acercamiento. Magallania 34, 91–106. doi: 10.4067/S0718-22442006000200009
Velásquez, H., Méndez, C., Reyes, O., Trejo, V., Sanhueza, L., Quiroz, D., et al. (2007). Campamentos residenciales tardíos a cielo abierto en el alto río Cisnes (Región de Aisén): Appeleg 1 (CIS 009). Magallania 35, 121–132. doi: 10.4067/S0718-22442007000100008
Villa-Martínez, R., Moreno, P., and Valenzuela, M. (2012). Deglacial and postglacial vegetation changes on the eastern slopes of the central Patagonian Andes (47° S). Quat. Sci. Rev. 32, 86–99. doi: 10.1016/j.quascirev.2011.11.008
Ward, G., and Wilson, S. (1978). Procedures for combining radiocarbon age determinations: a critique. Archaeometry 20, 19–31. doi: 10.1111/j.1475-4754.1978.tb00208.x
Weiner, S., Xu, Q., Goldberg, P., Liu, j., and Bar-Yosef, O. (1998). Evidence for the use of fire at Zhoukoudian, China. Science 28, 251–253. doi: 10.1126/science.281.5374.251
Whitlock, C., Higuera, P. E., McWethy, D. B., and Briles, C. E. (2010). Paleoecological perspective on fire ecology: revisiting the fire regime concept. Open Ecol. J. 3, 6–23. doi: 10.2174/1874213001003020006
Whitlock, C., and Larsen, C. (2001). “Charcoal as a fire proxy,” in Tracking Environmental Change Using Lake Sediments, eds J. Smol, H. J. Briks, and W. Last (Dordrecht: Kluwer Academic Publishers), 75–97.
Whitlock, C., McWethy, D. B., Tepley, A., Veblen, T. T., Holz, A., McGlone, M., et al. (2015). Past and present vulnerability of closed-canopy temperate forests to altered fire regimes: a comparison of the Pacific Northwest, New Zealand, and Patagonia. BioScience 65, 151–163. doi: 10.1093/biosci/biu194
Whitlock, C., Moreno, P. I., and Bartlein, P. (2007). Climatic controls of Holocene fire patterns in southern South America. Quat. Res. 68, 28–36. doi: 10.1016/j.yqres.2007.01.012
Keywords: human environmental dynamics, charcoal analysis, paleofire records, human paleoecology, anthropogenic impacts, hunter-gatherer archeology, holocene paleoclimate, Central Western Patagonia
Citation: Méndez C, de Porras ME, Maldonado A, Reyes O, Nuevo Delaunay A and García J-L (2016) Human Effects in Holocene Fire Dynamics of Central Western Patagonia (~44° S, Chile). Front. Ecol. Evol. 4:100. doi: 10.3389/fevo.2016.00100
Received: 30 April 2016; Accepted: 09 August 2016;
Published: 23 August 2016.
Edited by:
David McWethy, Montana State University, USAReviewed by:
Juan Carlos Berrio, University of Leicester, UKDebra Willard, United States Geological Survey, USA
Copyright © 2016 Méndez, de Porras, Maldonado, Reyes, Nuevo Delaunay and García. This is an open-access article distributed under the terms of the Creative Commons Attribution License (CC BY). The use, distribution or reproduction in other forums is permitted, provided the original author(s) or licensor are credited and that the original publication in this journal is cited, in accordance with accepted academic practice. No use, distribution or reproduction is permitted which does not comply with these terms.
*Correspondence: César Méndez, Y21lbmRlem1AdWNoaWxlLmNs