- Instituto de Ecología Regional, Universidad Nacional de Tucumán—Consejo Nacional de Investigaciones Científicas y Técnicas, Tucumán, Argentina
Most fleshy-fruited plants establish strong local interactions with a few fruit-eating species across their distribution range, which can differ among sites and have a major impact for the plant population dynamics. In turn, human disturbances alter both the original animal assemblage with which plants interact and the outcome of the mutualistic interaction. Negative consequences of human disturbances can be weakened when different seed dispersers exert similar effects on plant populations, being functionally equivalent. To understand the consequences of variability in seed dispersers on the recruitment of a long-lived tree species, I assessed changes in the assemblages of avian dispersers of Podocarpus parlatorei in subtropical Andean cloud-forests, and how these changes affect the outcome of the interaction at different spatial scales. The seed dispersal effectiveness (SDE) concept, defined as the likelihood of a seed removed by a fruit-eating bird to be dispersed to a suitable site for seed survival and germination, provides the framework to compare the contributions of different birds to seed dispersal. I compared the SDE in two old-growth forests dominated by P. parlatorei and a human disturbed forest, and in the main habitat types of these sites. In all sites, highest SDE values were provided by “gulpers” that swallow the whole fleshy cone (“fruit”), predominantly Elaenia and Turdus species. SDE was highest in forest edges and secondary forests, and negligible in other habitats. Equivalence in SDE was relatively low both within and between forest sites. Human forest disturbance modified the functional equivalence, the generalization in mutualistic interactions and the strength of SDE. Secondary forests showed the higher SDE and the greater richness of dispersers high in SDE; as a consequence, the ecological equivalence increased in the most suitable habitat for recruitment. This could lead to greater resilience of plant populations to local extinctions of dispersers, and allows the recovery of human disturbed forests. This study shows that in the replacement of functional equivalent species, the outcomes of the interactions are strongly affected by disperser abundance and habitat use, at the expense of dispersers' trait redundancy on plant population dynamics and community structure.
Introduction
Numerous terrestrial plants depend on fruit-eating animals as dispersal vectors of their seeds. Seeds are ingested with the fruit pulp and moved away from the mother plant where the progeny would face high density-dependent intraspecific competition and predation risk (Fleming and Estrada, 1993; Levey et al., 2002). In turn, the animal strips the pulp of the seed, with this treatment reducing the risk of attack by pathogens while favoring germination by chemical and physical scarification and removal of germination inhibitors (Robertson et al., 2006; Traveset et al., 2007). However, the efficiency of each of these processes varies even within plant species. For example, individual plants are not equally successful in attracting dispersers, giving rise to a variable proportion of crop size removal (Blendinger et al., 2008; Prasad and Sukumar, 2010). Moreover, some fruit-eating animals only consume the pulp and discard the seeds without ingesting them; thus, seeds are deposited close to the mother plant and dropped with adhered pulp remnants, making them more susceptible to pathogens. Consequently, once the fruit is removed, seeds can be dispersed to varying distances from the mother plant up to their deposition site, where biotic and physical characteristics will affect the following successive stages of recruitment, from the survival of the seed on the ground to the establishment of a new individual plant (Wang and Smith, 2002).
Plant species with fleshy fruits are involved in generalized mutualisms, interacting with different and sometimes numerous species of seed dispersers (Herrera, 1985; Howe, 1993; Donatti et al., 2011). However, the strength of the linkages between a plant species and its mutualistic partners is usually very variable, showing quantitatively strong relationships with only some species of their seed dispersers (Jordano, 1987; Bascompte and Jordano, 2007; Ruggera et al., 2016). These strong links are often established with the most abundant mutualistic partners, whose numerical dominance leads to a higher frequency of visits (Vázquez et al., 2005), and may result in a greater number of removed fruits and dispersed seeds. In other cases, high foraging efficiency could be more likely to influence the quantity of seeds removed, as in large-sized species that require a greater daily food intake than small-sized species with high visitation frequency (Carlo and Yang, 2011; Rother et al., 2016). This quantitative component of the seed dispersal effectiveness (SDE; Schupp et al., 2010) does not necessarily translate into higher dispersion quality. The treatment given to the seed and the characteristics of the deposition site often differ among seed disperser species and can therefore deeply modify the recruitment pattern defined by the quantitative SDE (Schupp, 2007; Loayza and Knight, 2010; McConkey et al., 2015). Therefore, both the quantitative and qualitative components of SDE must be taken into account in order to establish the consequences of seed dispersal by a particular class of fruit-eating animal on the recruitment of a plant species. The spatial context in which the interaction occurs may also largely influence SDE (Schupp et al., 2010; Lavabre et al., 2016). At a regional scale, different populations of a plant interact with different sets of species, since the composition and abundance of seed-disperser assemblages usually vary across the geographic range of a plant species (Jordano, 1993; Thompson, 2006; Robledo-Arnuncio et al., 2014). At the local scale, abundances- and composition- of seed dispersers differ between habitat types as a result of species-specific habitat selection and their interactions with the physical and biotic environment.
The functional importance of seed dispersers could depend on their abundance and phenotypic traits relevant to the interaction (Schleuning et al., 2015). Species of fruit-eating birds, a major group of seed dispersers, differ in mobility, habitat requirements, foraging behavior, size, shape and digestive capacity, and all of these factors influence the number of dispersed seeds and the quality of the dispersal service. However, in plant-disperser mutualistic systems, coevolved interactions would lead to trait convergence between species of the same trophic level (Guimarães et al., 2011; Eklöf et al., 2013; Dehling et al., 2014). Therefore, SDE may depend on species-specific attributes of the dispersers, but disperser species that are similar in ecology or behavior can yield similar interactive outcomes. It is also possible that different combinations in the number of dispersed seeds and in the quality of the seed treatment may result in similar SDE. Thus, the uniqueness of fruit-eating species does not necessarily translate into the uniqueness of interactive outcomes (Zamora, 2000). Trophically similar species might be functionally equivalent, meaning that they play the same role in regulating an ecological process. When they coexist, these species can be functionally redundant and able to take over the functions performed by other species if these become extinct, providing resilience to the community and maintaining the ecosystem functions (Lawton and Brown, 1993). In a broad sense, fruit-eating species are functionally equivalent as they play the same role of seed dispersal. In a narrower sense, establishing functional equivalence between dispersers of a plant species requires knowledge of the consequences of interaction outcomes in terms of dispersal effectiveness, and ultimately, their influence on plant demography. The SDE concept defines the functional importance of the fruit-eating species in their role as seed dispersers of individual plant species. Given the multiplicity of factors affecting the ecology of fruit-eating species, it could be expected that SDE would vary in time and space, both within a community and among communities (Wellnitz and LeRoy Poff, 2001; Schupp et al., 2010). Therefore, the demography of a plant species that interacts with functionally diverse and spatially variable disperser assemblages may depend locally on different species, which may be equivalent or not in their SDE.
Despite the increasing number of studies that evaluate quantitative and qualitative components of SDE in individual plant species (e.g., Godínez-Alvarez et al., 2002; Schupp et al., 2010; Blendinger et al., 2011; McConkey et al., 2015; Rother et al., 2016), empirical evidence on the spatial variability in seed dispersal roles of functionally similar species is still extremely scarce (Calviño-Cancela, 2002; Bueno et al., 2013; Schleuning et al., 2015). The limited evidence suggests that in simple systems defined by plants with a low number of disperser species, functional equivalence is at least low, habitat-specific, and context-dependent. Thus, it could be expected that a few fruit-eating species can have a major impact for most plant population dynamics (e.g., Carlo and Morales, 2016). Here, I use a conifer (Podocarpus parlatorei Pilg., Podocarpaceae) occurring in subtropical Andean cloud forests and the bird species that consume its fleshy cones (hereafter “fruits”) to assess the spatial variability in the functional equivalence of seed dispersers. To achieve this main objective, (1) I explored spatial changes in the assemblages of avian dispersers; (2) evaluated the importance of the trophic morphology and foraging behavior to explain the functional similarity of bird species; (3) assessed the consequences of these changes on the outcome of the plant-disperser interaction. To this end, I studied different subcomponents of the quantitative (QC) and qualitative (QL) components of the SDE in the main habitat types of three localities. The analysis of successive stages of seed dispersal, from fruit production to recruitment of new individuals, allows us to combine the immediate quantitative effects and the delayed qualitative effects of dispersers (Schupp et al., 2010). Then, I integrated these components to determine the relative contribution of each disperser to the initial recruitment of a long-lived tree species and the functional equivalence and redundancy in fruit-eating bird assemblages. I found that variability in functional equivalence between assemblages of seed dispersers affects the outcome of the interaction and the recruitment dynamics of a dominant long-lived tree species in Andean forests.
Methods
Study Area
Field work was conducted during 2005–2007 in three sites of northwester Argentina: (1) peaks of Taficillo (26°42′S and 65°21′W, 1,600–1,800 m a.s.l.) in San Javier mountain range, province of Tucumán, an area located inside the Sierra de San Javier Park (hereafter “Taficillo”); (2) Nogalar de los Toldos National Reserve (22°16′S and 64°43′W, 1,700–2,000 m a.s.l.), province of Salta (hereafter “Nogalar”), 490 km north of Taficillo; (3) Los Toldos (22°18′S and 64°42′W, 1,500–1,600 m a.s.l.), valley of Los Toldos, province of Salta (hereafter “Los Toldos”), 3 km east of Nogalar. Taficillo and Nogalar are old-growth forests in good conservation condition, with moderate livestock density. They are located on slopes close to the upper limit of the forest, occupying a mosaic of forests, grasslands, or shrubby grasslands, and the treeline ecotone characterized by the presence of a narrow vegetation strip dominated by shrubs (Supplementary Figure S1). Los Toldos corresponds to secondary forests in rural areas in the surroundings of the town of Los Toldos, growing mainly in abandoned crops and pastures of different age (Supplementary Figure S1). All sites are located in cloud forests, the upper vegetation level of the Southern Yungas, with dominance of P. parlatorei trees. The climate is subtropical with dry season. Rainfall is determined orographically, highly seasonal, concentrated in summer but with an important water input by fog interception throughout the year (Hunzinger, 1997). Frosts are frequent and snowfalls occasional in winter. Mean annual rainfall is 1,300 mm and mean annual temperature is 15°C (Easdale et al., 2007).
P. parlatorei is the unique native conifer in northwestern Argentina and southern Bolivia, and is considered an emblematic species of cloud forests in the region. It has a fragmented distribution along a narrow latitudinal strip of more than 1,000 km of the eastern flanks of the Andes, from Catamarca and Tucumán in Argentina to Cochabamba and La Paz in Bolivia, extending marginally to the south of Peru (Quiroga et al., 2012). It occupies the upper vegetation level of the cloud forests, growing in mixed forests at lower altitudes, and in almost monospecific forests at higher elevations toward the treeline. It is a long-lived canopy species that can live for several centuries and reach 25–30 m in height and up to 1.5 m diameter at breast height (Carilla and Grau, 2010; Quiroga et al., 2012). This dioecious tree requires more than 15 years to initiate reproductive activity and nearly half a century to reach its largest size. The solitary fruits are pedunculate and consist of two clearly differentiated segments, a fleshy purple receptacle formed by the basal scales of the cone, and a single seed covered with a thin, fleshy, green, modified scale attached to the apex of the receptacle (Figure 1A). The fleshy fruit is 0.36 g in weight and 12.2 × 7.6 mm in size; the single seed is ellipsoidal and weighs 65.4 mg.
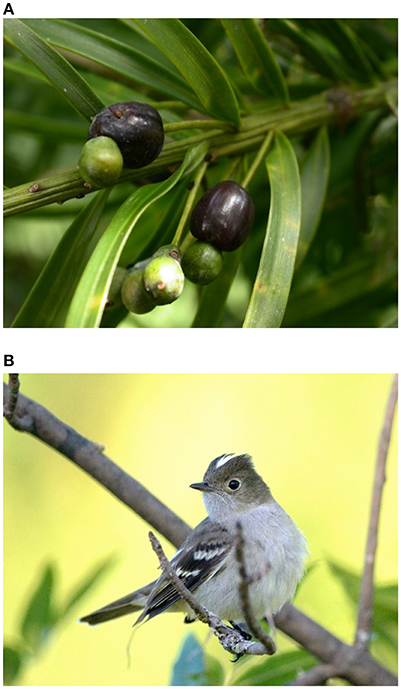
Figure 1. (A) Ripe cone (“fruit”) of Podocarpus parlatorei showing the fleshy basal receptacle and the single seed attached to the apex of the receptacle (photo of the author). (B) Elaenia albiceps was the largest contributor to the overall seed dispersal effectiveness of P. parlatorei in old-growth forests (photo by Rodrigo Aráoz).
Sampling Design
In Taficillo and Nogalar, there are three potentially important habitat types for the recruitment of P. parlatorei: the interior of the forest (“forest”), the shrubby grassland (“grassland”) and the shrubby ecotone between the forest and the grassland (“forest edge”). In each type of habitat, I evaluated the abundance of fruit-eating birds, post-dispersal seed survival, and germination. I evaluated fruit consumption in the forest and in the forest edge, since there were no trees of reproductive age in the grassland. I measured the abundance of fruit-eating birds and their fruit consumption in a single habitat type in Los Toldos, an open secondary forest represented by interspersed patches of trees and shrubby grasslands, which is physiognomically and functionally similar to the forest edge.
Birds: Abundance, Foraging Behavior, and Functional Traits
To determine the abundance of birds, I performed 20 point counts (20-m fix-radius and 7 min long) in each habitat type in the three sites (N = 140, Table 1) during February-March, when P. parlatorei is fruiting. I made counts during the first 3 h after sunrise and 1 h before sunset, avoiding rainy or windy days. Point counts were 200 m or more apart to avoid double counts. I recorded all individuals of fruit-eating species seen or heard at each point. I visited each counting point twice, and calculated the average value per species (expressed as number of birds per ha) for statistical analysis.

Table 1. Sampling design to the study of direct and indirect effects of Podocarpus parlatorei seed dispersal by birds at three sites of subtropical Andean cloud-forest.
I conducted focal samples on trees with mature fruits to estimate the relative importance of each fruit-eating bird species in the dispersal of P. parlatorei seeds. Since the large volume of the tree crown and the simultaneous presence of several individuals made it difficult to record all the required data, I split the 20-min focal sample into two consecutive 10-min periods. During the first 10 min, I recorded all the individuals that visited the tree and their permanence time in seconds, and during the next 10 min I recorded the number of seeds ingested per visit and the treatment given to the fruits. I considered a visit when a fruit-eating bird arrived to a tree and consumed one or more fruits, and considered a foraging event the consumption of each individual fruit. According to the handling behavior given to the fruit, I classified each foraging event as “gulping” (when the whole fruit was swallowed including the seed), “mashing” (the bird plucked the fruit and chewed it in the beak, being able to swallow or not the seed together with the pulp) and “pecking” (the bird removed pieces of pulp without ingesting the seed; usually it did not pluck the fruit; Foster, 1987; Levey, 1987). I recorded the number of seeds swallowed and dropped during mashing; no seed was ingested during pecking and I assumed that all seeds were ingested during gulping. I made 12 20-min focal samples on 5–8 trees with mature fruits on forests and forest edges at each site (N = 414; Table 1). Samples at each tree were distributed homogeneously during 4.5 h from sunrise, in two blocks of six successive focal samples on non-consecutive days during February-March.
In order to functionally characterize the bird species that consumed P. parlatorei fruits, I averaged the measurements of four adult specimens (two females and two males) from Southern Yungas forests of Argentina available at the ornithological collection of the Fundación Miguel Lillo (Tucumán, Argentina). From each specimen, I measured beak length from the tip to the commissural point, beak width between the commissural points with the closed beak, beak height at the nostrils with the closed beak, wing chord as the length of the folded wing, and wing pointedness calculated with the Kipp's index (i.e., the distance from the tip of the first secondary to the wing tip measured on the folded wing). Body mass was averaged from numerous mist-netted birds from Southern Yungas forests captured by the author and from data available at the national banding center (CENAA) of Argentina. I used these data to calculate the following five morphological functional traits for statistical analyses: “body mass” as an indicator of energy requirements; “beak length:wing chord ratio” and “beak width:wing chord ratio” as indicators of the relative size of the beak; “beak length:beak height ratio” as an expression of beak shape; “Kipp's index” as a surrogate of flight capacity. I also classified all bird species in three functional groups according to their fruit handling behavior (see above) as gulpers, mashers and peckers.
Seed Survival to Predation and Seed Germination
I used an experimental approach to assess the importance of seed predation and seed germination to plant recruitment. Seeds used in the tests were removed from ripe fruits collected from different parent plants at the sites; only apparently healthy seeds were used.
Rodents are the main cause of post-dispersal loss of P. parlatorei seeds (PGB, unpublished data). I studied post-dispersal seed survival to predation by seed-eating rodents using field tests conducted in the three habitat types of Taficillo and Nogalar and in forest edges of Los Toldos (Table 1). A seed survival test consisted of five seeds stuck firmly with low-odor rainproof glue on 10 × 3 cm metallic mesh, nailed to the ground (see García et al., 2005 for similar methods). In February 2006, during the peak of the seed dispersal season, I established 361 tests in the field and monitored the number of remaining seeds after 45 and 90 days. A seed was considered predated if it was either missing from the metallic mesh or gnawed; disappearance owing to other factors was considered negligible. Although some missing seeds could be secondarily dispersed by rodents, I did not found evidence of this.
I studied germination experimentally in the three habitat types of Taficillo and Nogalar and in secondary forests in Los Toldos (Table 1), by means of tests with enclosures that prevented seed predation by rodents. Each test consisted of 20 seeds deposited directly on the soil, covered with a mesh to prevent seed removal by rodents. The enclosure consisted of a 3-mm metallic mesh, 30 × 20 cm side and 10 cm in depth, firmly nailed to the soil. The mesh size prevented the loss of seeds by water runoff. In March 2006, I established 150 germination tests in the field. Germination tests were placed in two to four different microhabitats (below fruiting trees, non-fruiting trees, shrubs and grasses, in the open) within each type of habitat, but for the purposes of this study all samples were combined by habitat type. Germination started after1 month; therefore, to evaluate germination I monitored emerging seedling after 45 and 90 days. A seed was considered germinated when the radicle emerged. A few tests were lost due to trampling by cows and soil movements by underground rodents (Ctenomys sp.); these tests were excluded from statistical analysis as the seed fate was unknown.
Components of Seed Dispersal Effectiveness
To determine how different birds and bird groups (species, functional groups, assemblages) affected the outcome of the interaction with P. parlatorei, first I estimated the contribution of each bird species to the different components and subcomponents of SDE. I calculated the quantitative SDE, at the individual tree level, as QC = VD, where V is the number of birds consuming fruits in a focal tree during 120 min of observation, and D the number of seeds dispersed per visit. In turn, I estimated the number of dispersed seeds as D = CI, where C is the mean number of fruits consumed per visit (i.e., the product between mean consumption rate and permanence time) and I is the proportion of swallowed seeds of the total fruit consumed. I calculated the qualitative SDE as QL = ASG, where A is the probability of depositing seeds in a particular habitat type estimated as bird species abundance (birds ha−1) times the proportion of swallowed seeds (I), S is the seed survival rate calculated for each habitat as the proportion of seed survival, and G is the germination rate calculated as the proportion of seeds that germinated in each habitat. I used A as a proxy to estimate seed deposition in the absence of empirical data on the spatial distribution of seed deposition by each bird species. Post-dispersal seed survival and germination were constant in each habitat type; therefore, I estimated QL at the population level. Finally, I combined the quantitative and qualitative components to calculate the effectiveness of seed dispersal as SDE = QC × QL. When more than one bird species were involved (i.e., functional group; assemblage), I calculated the effect of all of them acting together as the sum of the values estimated for each species.
Landscapes of Seed Dispersal Effectiveness
I plotted all bird species (or functional groups) per habitat combinations on SDE landscapes. These are two-dimensional graphs that show the possible combinations of QC (x-axis) and QL (y-axis) values, where isoclines connect all QC and QL-values yielding the same SDE (Schupp et al., 2010). The position occupied by each species or functional group in the SDE landscapes explains fundamental issues in seed dispersal ecology, such as their contribution to the overall SDE, knowledge of the dispersers that provide highly effective seed dispersal to populations of P. parlatorei, identification of functionally equivalent highly-effective seed dispersers, and the sensitivity of the overall SDE to changes in different components or subcomponents of SDE.
Statistical Analysis
In order to test for the existence of spatial changes in fruit-eating bird assemblages, I constructed matrices of sites or habitats (columns) by abundances of species that consumed fruits of P. parlatorei (rows). I used a beta diversity-based approach to explore the extent of change among assemblages, disentangling the contribution of spatial turnover and nestedness to beta-diversity patterns (Baselga, 2010). Spatial turnover implies the replacement of some species, while nestedness occurs when the assemblages of sites with smaller numbers of species are subsets of the assemblages at richer sites. To differentiate both components of beta diversity, I used the Sørensen dissimilarity index (βsor) and the Simpson dissimilarity index (βsim), following the procedure proposed by Baselga (2010). Accordingly, species turnover is described by βsim and nestedness by βnes = βsor − βsim.
To explore the distribution of fruit-eating species in the morpho-functional space that is relevant for the consumption of P. parlatorei fruits, I performed a principal component analysis (PCA) to order all species as a function of the five morphological traits. I used the species-site (habitat) × species-trait data matrix for analysis of similarity (ANOSIM) to compare the variation in morpho-functional traits between fruit-eating assemblages (sites or habitats). ANOSIM is a non-parametric permutation procedure based on a standardized rank correlation between two distance matrices, and tests the hypothesis of no differences between sites (habitats) in the morpho-functional traits of bird species, by calculating the statistic R of global difference among all the assemblages as well as the level of difference among all the pairs of assemblages analyzed. In all the analyses, I used the Euclidean similarity distance without data transformation.
To test for functional traits affecting the quantitative SDE provided by birds, I fitted a linear mixed-effect model, with QC-values per bird species in each assemblage as the dependent variable. I performed a similar model by replacing QL as the dependent variable. I included body mass, beak length:wing chord ratio, beak width:wing chord ratio, beak length:beak height ratio, Kipp's index and the behavioral functional group trait as fixed factors. I included bird species as a random factor, since some of them occurred in more than one assemblage. Before fitting the model, trait variables were log-10 transformed and standardized to bring all to compatible units from a distribution with a mean of 0 and a standard deviation of 1. I did not included sites and habitats as random effects because variables were scaled within them. I used the Type V sums of squares method for testing effects in incomplete designs, and explore the outcome of different orders of effects.
Results
Spatial Variation in Bird Assemblages
I recorded a total of 26 species of birds consuming fruits of P. parlatorei (Supplementary Table S1). The overall total dissimilarity between pairs of fruit-eating bird assemblages was moderate but quite variable (βsor: rank = 0.20–0.91). Overall dissimilarity due to spatial turnover was slightly higher than nestedness (Table 2), although differences were not significant (T-test: t = 1.51, df = 40, P = 0.14). Nestedness and spatial turnover were the main source of dissimilarity in intra-site and intra-habitat comparisons, respectively (Table 2).
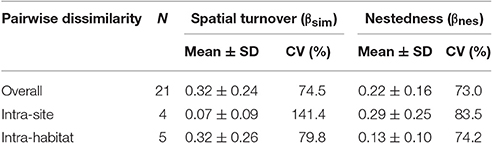
Table 2. Components of dissimilarity between assemblages of birds that consumed fruits of Podocarpus parlatorei in three sites and three habitats in subtropical Andean cloud-forests.
Functional Diversity between Fruit-Eating Birds
The principal components PC1 and PC2 explained 63.1% of the variation in the functional diversity of the morphological traits. In the two-dimensional plot defined by PC1-2, the species of gulpers differed from the remaining fruit-eating species in the shape of the beak, which is proportionally larger in gap size (larger beak width:wing chord ratio) and/or more sharpened (larger beak length:beak height ratio) than in the others. Gulpers also have high to medium values of wing pointedness (Figure 2). Species of peckers were located on the opposite side of the two-dimensional ordination plot, characterized by their small size and short and blunt beaks or short beaks with narrow gap size (Figure 2). Mashers are a heterogeneous group of species, morphologically intermediate between gulpers and peckers (Figure 2).
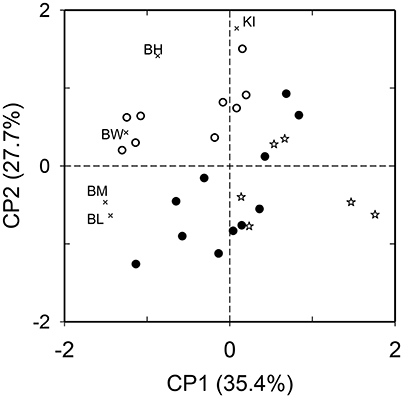
Figure 2. Ordination of 26 bird species that consumed Podocarpus parlatorei fruits in the functional space defined by five morphological traits of size and shape (depicted with thin x; BM, body mass; BL, beak length to wing chord ratio; BW, beak width to wing chord ratio; BH, beak length to beak height ratio; KI, Kipp's index) in a principal component analysis. Bird functional groups defined by their fruit handling behavior are depicted with open circles (gulpers), filled circles (mashers), and open stars (peckers).
Morpho-functional similarity did not differ between assemblages of fruit-eating birds. The global difference among assemblages was smaller than expected by chance both based on sites (N = 3 sites, ANOSIM Global R = −0.042, P = 0.98) and habitat types (N = 3 habitats, Global R = −0.06, P = 0.96).
Seed Dispersal Effectiveness
Site and Habitat
Overall SDE per site, calculated as the sum of all partial SDE-values in Table 1, was higher in Los Toldos (13.558) than in Taficillo (0.838) and Nogalar (0.923). Overall SDE in grasslands equaled QL due to lack of fruiting trees, and was null in forests because seeds did not germinate there (Table 3). As a consequence, intra-site SDE (calculated as the sum of all SDE-values of a particular site) was always maximum in forest edges (>99%), being negligible in grasslands and absent in forests (Table 3).
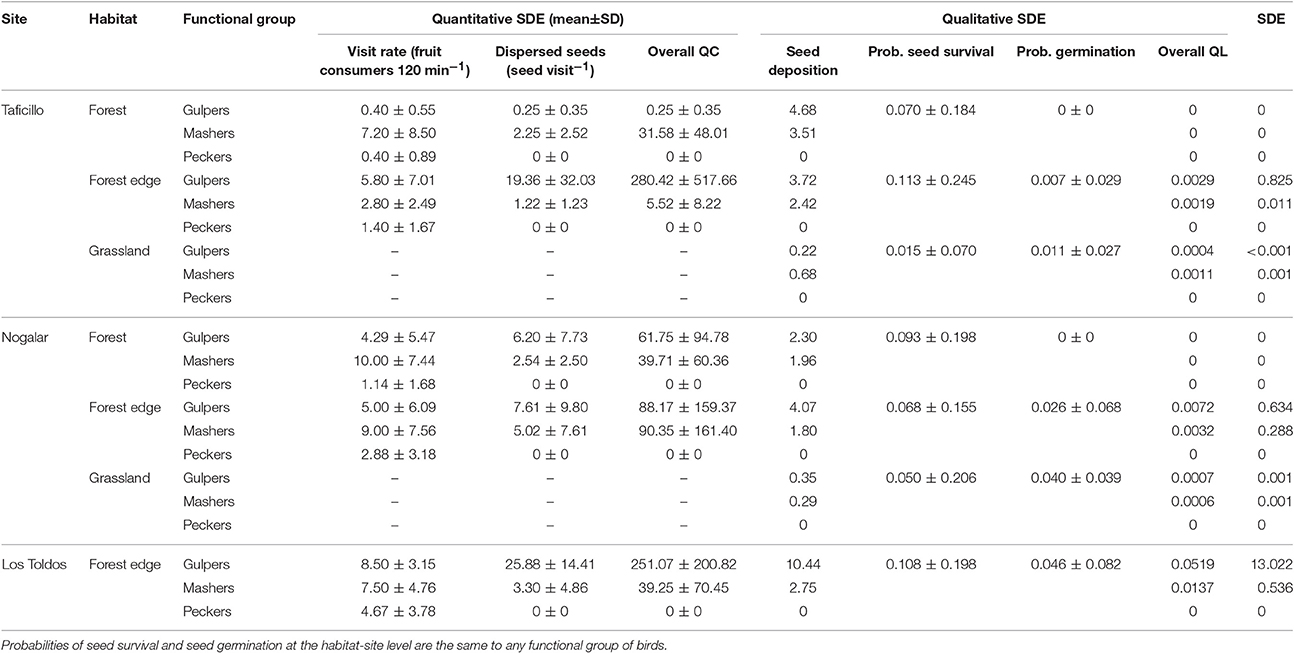
Table 3. Overall seed dispersal effectiveness (SDE) of Podocarpus parlatorei trees, and values of calculated subcomponents of quantitative (QC) and qualitative (QL) SDE by bird functional groups in different habitats of three sites in subtropical Andean cloud-forest.
Functional Group
Average QL and QC components of SDE differed between functional groups [Kruskal-Walis tests, QL: H(2, 21) = 7.96, P = 0.019; QC: H(2, 21) = 10.35, P = 0.006], being significantly larger for gulpers than for peckers (test of multiple comparisons of mean ranks). Similarly, overall SDE was significantly larger in gulpers than in peckers, with mashers showing intermediate values [H(2, 21) = 8.06, P = 0.018]. Peckers cannot be considered dispersers, since they failed to disperse seeds. The overall QC showed less variability than overall QL (coefficient of variation, 151.7 and 287.8% respectively; Table 3).
SDE reached higher values than the mean SDE only in gulpers and mashers of forest edges (except mashers of Taficillo; Figure 3). In particular, effectiveness by gulpers was much higher in a population of P. parlatorei (Los Toldos) than in any other group analyzed. QL values in grasslands were very low for all functional groups, showing the low effectiveness of seed dispersal in this habitat (Table 3, Figure 3).
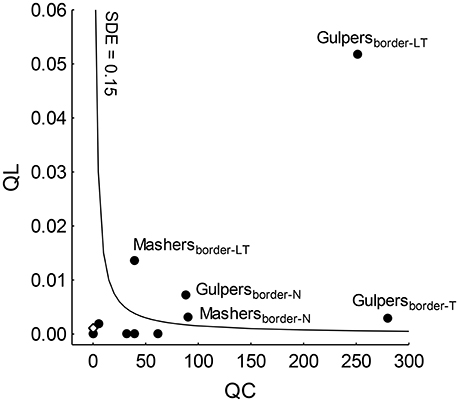
Figure 3. Positions of functional groups (filled circles) of fruit-eating birds on the seed dispersal effectiveness (SDE) landscape. Subscripts show the combination of habitat-site that indicate the assemblage; T, Taficillo; N, Nogalar; LT, Los Toldos. The open diamond shows the highest QL-value reached by a functional group (i.e., mashers from Taficillo) in grasslands, where it is not possible to calculate QC. The isocline indicates the mean SDE across all functional groups.
Bird Species
A few fruit-eating bird species reached values above the mean SDE of the sites (Figure 4). In Nogalar and Taficillo, Elaenia albiceps (White-crested Elaenia; Figure 1B) was the most effective seed disperser, followed by Turdus nigriceps (Slaty Thrush) in Taficillo. In Los Toldos, the most effective seed disperser was Elaenia parvirostris (Small-billed Elaenia), followed by Turdus rufiventris (Rufous-bellied Thrush) and Elaenia obscura (Highland Elaenia). A group of species reached moderately high levels of effectiveness: Atlapetes citrinellus (Yellow-striped Brush-finch) in Taficillo, E. obscura and Atlapetes fulviceps (Fulvous-headed Brush-finch) in Nogalar, and Thraupis sayaca (Sayaca Tanager) and Tyrannus melancholicus (Tropical Kingbird) in Los Toldos (Figure 4). All these species with moderate SDE had high QL but a very low QC component, resulting in SDE-values below the average of the site.
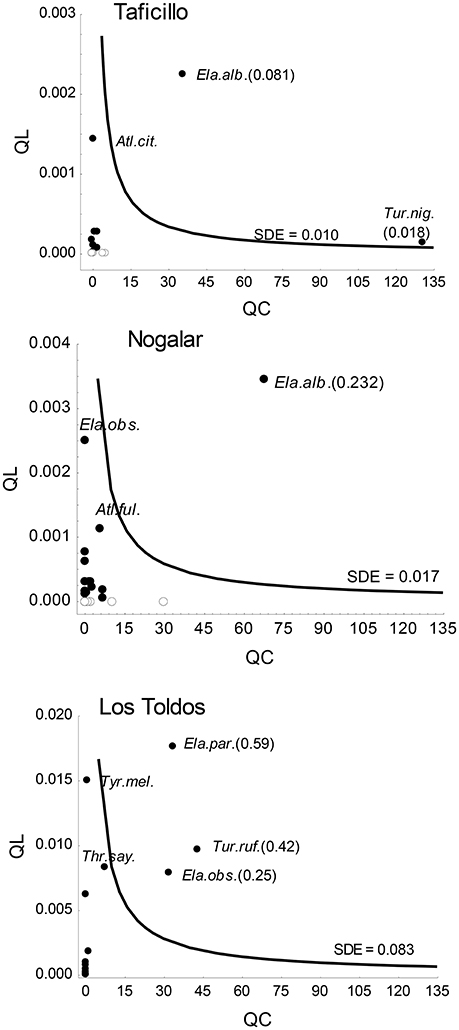
Figure 4. Positions of Podocarpus parlatorei seed dispersers (filled circles: forest edge species; open circles: forest species) on the seed dispersal effectiveness (SDE) landscape in three subtropical Andean cloud-forest sites. Isoclines indicate the mean SDE across all functional groups in each site. The species name and SDE-values between brackets are given only for highly effective seed dispersers; names of moderately effective species with high QL-values are also shown. Acronyms of gulpers are Ela.alb, Elaenia albiceps; Ela.obs, Elaenia obscura; Ela.par, Elaenia parvirostris; Tur.nig, Turdus nigriceps; Tur.ruf, Turdus rufiventris; Tyr.mel, Tyrannus melancholicus; mashers are Atl.cit, Atlapetes citrinellus; Atl.ful, Atlapetes fulviceps; Thr.say, Thraupis sayaca.
All bird species with high SDE were gulpers, which have a high visit rate to fruiting trees in forest edges and which ingested a relatively high number of seeds per visit (Table 4). Among the major seed dispersers of P. parlatorei, the variability of the overall QC and QL components was very similar (Table 4; coefficient of variation, 116.4 and 116.8% respectively).
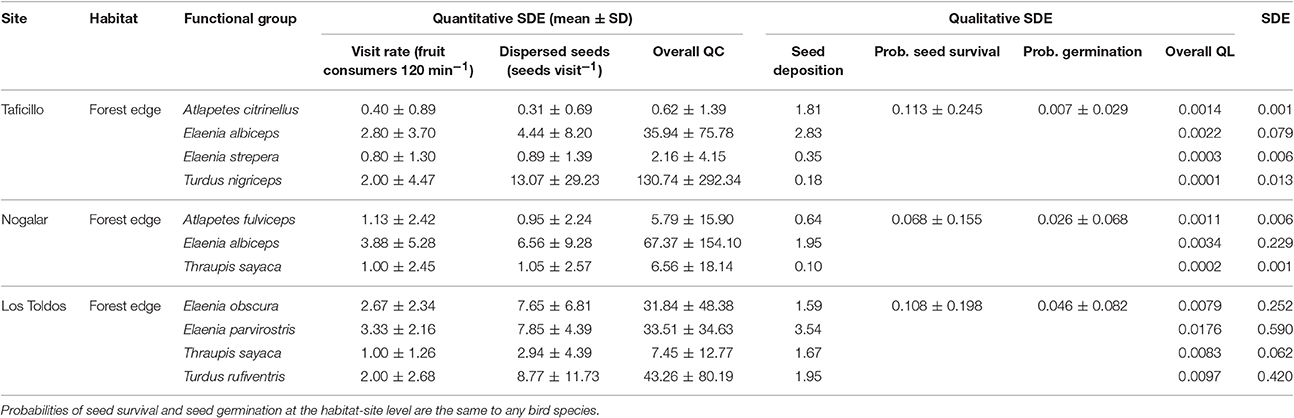
Table 4. Direct and indirect effects of the interaction between Podocarpus parlatorei and its highly effective seed dispersers at three sites in the subtropical Andean cloud-forest.
The best mixed general linear model (adjusted R2 = 0.33, df = 25, P = 0.031) that explained the QC component of SDE included only bird species identity. Morphological and behavioral traits were excluded from the model (Table 5). Similar results were obtained when the QL component was analyzed (adjusted R2 = 0.74, df = 25, P < 0.0001; Table 6).
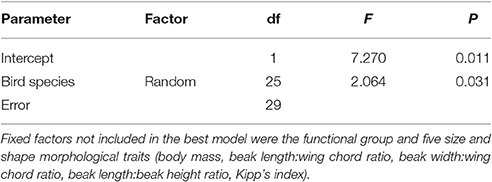
Table 5. Result of mixed general linear model analyzing bird functional traits that affect the QC of SDE.
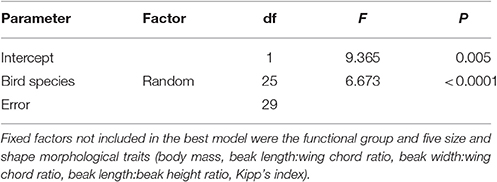
Table 6. Result of mixed general linear model analyzing bird functional traits that affect the QL of SDE.
Discussion
P. parlatorei is a dominant tree in subtropical Andean cloud-forests, whose populations depend on fruit-eating birds to disperse the seeds. Bird species composition and richness varied between disperser assemblages, but the distribution of morpho-functional traits relevant for the mutualistic interaction did not vary. Thus, the main differences in SDE of P. parlatorei populations were explained by the habitat type where the interaction occurred and by the disperser identity. The quality of seed dispersal imposed a major ecological filter to seedling establishment. The insignificant arrival of seeds to the grasslands and unsuccessful seed germination within the forest determined that SDE reached a maximum in the ecotone between forests and grasslands. There, disperser effectiveness was highly species-specific and depended largely on the abundance of dispersers and their fruit handling behavior, which determined the number of dispersed seeds. The most effective dispersers were all gulpers that ingest the whole fruit, but SDE was highly variable between species. In old-growth forest edges, E. albiceps was by far the largest contributor to the overall SDE. It is noteworthy that in secondary forest sites, this function was performed by several congeneric species, including E. parvirostris and E. obscura. These morphologically similar species are mainly ecologically differentiated by their segregation between habitat types. In consequence, Elaenia species replace each other as highly effective dispersers of P. parlatorei in forests with different conservation status (i.e., old growth forests, disturbed forests). Other species of Turdus, Atlapetes, and Thraupis reached moderate to high values of SDE and may contribute in a similar way to the dispersal of P. parlatorei. This is also remarkable, given that these species from different evolutionary lineages provide a similar ecological service despite their morphological and behavioral differences. Equivalent disperser species could be functionally redundant when coexisting in the same assemblage, providing larger resilience to the community against eventual population crashes of a disperser.
Close functional relationships between individual plant and fruit-eating bird species, and trait convergence between functionally similar bird species, where described elsewhere (e.g., Moermond and Denslow, 1985; Dehling et al., 2014). Accordingly, I found morpho-functional differences between the group of birds more efficient in fruit handling (i.e., gulpers) and the group of pulp predators that do not disperse the P. parlatorei seeds (i.e., pulp peckers), but not between mashers and gulpers. Since both gulpers and mashers include species with very different values of SDE, and the wide overlap of SDE-values between both functional groups, it is not surprising that the effectiveness of P. parlatorei seed dispersal does not match the functional traits of fruit-eating species. These results indicate that traits related to a frugivorous diet may partially explain the role of birds as seed disperser or pulp predator, but do not explain their role as effective seed disperser to a plant population. Both the frequency of visits and the estimated deposition of seeds depend strongly on bird abundance, and subordinate to it, the outcome of the plant-frugivore interaction is influenced by behavioral and morphological bird traits. Moreover, even pulp mashers, usually considered inefficient dispersers compared to "legitimate" dispersers like fruit gulpers, can be quantitatively important effective dispersers in Andean forests (Ruggera et al., 2011, 2016). Although species of gulpers are those that reach higher values of SDE of P. parlatorei, also some species of mashers can be very effective dispersers of this species with medium-sized seeds (see Loayza and Knight, 2010, for a similar finding).
The SDE concept is a useful framework to study the equivalence and complementarity of seed dispersers in the establishment of plants (McConkey and Brockelman, 2011; Bueno et al., 2013; Schleuning et al., 2015; Rother et al., 2016). A pattern emerging from most SDE studies is that one or few species are responsible for most effective seed dispersal (Schupp et al., 2010). This can have important consequences on plant population demography, since variations in abundance or foraging behaviors of one disperser may have strong effects on plant recruitment. Highly-effective seed dispersers can be equivalent or complementary in the interaction outcomes. Larger equivalence between seed dispersers may contribute to resilience of dispersal systems, whereas more functional complementary species can lead to a larger representativeness of microhabitats for plant recruitment (McConkey et al., 2015; Rother et al., 2016). The functional equivalence between highly effective dispersers allowed for high SDE in sites differing in human forest use, and increased the overall SDE when these dispersers coexisting in habitats suitable for recruitment. Only one species was the most effective disperser of P. parlatorei in edges of old-growth forests (E. albiceps, 78.9% of the overall SDE in Taficillo, 95.8% in Nogalar), but there were multiple effective dispersers in secondary forests (E. parvirostris, T. rufiventris and E. obscura; 44.3, 31.4, and 18.9%, respectively). All three species share similar fruit handling behavior and habitat use (particularly Elaenia species); therefore, they seem to be functionally equivalent rather than complementary in P. parlatorei dispersal. All of them are short-distance dispersers that favor open secondary cloud forests rather than the interior of old-growth forests where P. parlatorei does not germinate.
Multispecies effective dispersal may be particularly advantageous in the absence of density-dependent mechanisms of regulation, such as resource limitation that could promote competition between dispersers. In populations of P. parlatorei, dispersal is clearly not limited by fruit availability; crop sizes are superabundant and a large amount of fruits falls undispersed under the canopy of the mother plants, suggesting disperser satiation (Hampe, 2008). Seed dispersal by multiple effective species increased overall SDE in secondary forests that grow in abandoned crops and pastures. The individual contribution of each of the most effective dispersers was even greater than overall SDE in the edges of old-growth forests. If the abundance -and the number of visits- of the dispersers is limited by habitat availability in a density-dependent manner (e.g., number of breeding territories), the spatial configuration of secondary forests could be increasing the carrying capacity of dispersers in the habitat that meets the best conditions for recruitment. While the ecotone between old-growth forests and grasslands is a narrow strip distributed linearly, secondary forests are distributed two-dimensionally through abandoned paddocks, where the proportion of “edges” is much higher than in forest-grassland ecotones. Therefore, the spatial configuration of trees and open sites in human disturbed forests would have an indirect effect on P. parlatorei recruitment through its influence on SDE by fruit-eating birds.
Coexisting equivalent species can be redundant (in the sense of compensation responses, see below) in their function as dispersers, although this is particularly difficult to verify, even in a single plant population. Redundancy requires that fruit uneaten by one disperser would be eaten by another that disperses seeds with similar effectiveness, so that the loss of a disperser would result in no significant reduction in the recruitment of seedlings (McConkey and Brockelman, 2011). Bridging practical difficulties to demonstrate changes in a species abundance in response to changes in a potential competitor, the landscapes of SDE allow us to estimate how QC or QL magnitudes should change in one species to replace another in its dispersal function. As explained above, changes in the number of seeds dispersed by moderately high-quality dispersers can have large effects on SDE, but SDE of species with low QC will have a low increase despite large increases in QL. An outstanding result of this study is that the number of potentially redundant species that can reach high SDE is low in any of the assemblages I studied.
The SDE of P. parlatorei populations vary spatially, and the relationship between QC and QL components depends strongly on the habitat type (see Calviño-Cancela, 2002 in Schupp et al., 2010 for a similar example). Between-habitat rather than between-site changes in dispersal components resulted in huge variation in plant establishment. While recruitment of new individuals occurs in young forest stands, very little or no recruitment can be observed in old-growth forests once a tall canopy of this long-lived tree is formed, conducting to extensive recruitment failure (PGB, unpublished data). Effective seed dispersal occurred almost exclusively in forest edges and secondary forests; this result provides empirical support for previous observations suggesting that P. parlatorei requires shrubs in areas with extensive disturbance to recruit (Ramadori, 1998; Navarro and Maldonado, 2002). In these habitats, almost all birds ranking high in the QC component of SDE also exhibited high QL-values, but only some species ranking high in QL did it similarly in QC. The reason for this result is that not all consumers are equally dependent on fruits. All species in the fruit-eating assemblages are frugivorous-insectivorous, varying in ecology and foraging behavior, with fruit representing a quit variable fraction of their diet. Therefore, the abundance of a disperser may affect the frequency of visits (Vázquez et al., 2005) and the potential seed arrival to a habitat type (a QL subcomponent), but may have little influence on the number of seeds actually ingested (a QC subcomponent; Supplementary Table S2). In fact, local abundance of fruit-eating birds (all species, and gulpers and mashers separately) was related to increased visit frequency (Supplementary Table S2), but only the abundance of gulpers was related to the amount of seeds dispersed (Supplementary Table S2). A clear example is T. nigriceps in forest edges of Taficillo (high QL and low QL, Figure 4), a species with low abundance at the edges and a consequent low frequency of visits, but which swallows a comparatively large amount of seeds per visit. A similar relationship between visitation frequency and fruit removal was observed for another species of Turdus, which was interpreted as a warning of consider the most abundant species as quantitatively more important dispersers (Carlo and Yang, 2011). On the other hand, emberizids of the genus Atlapetes are moderately abundant and have high QL-values and visit frequencies, but reach below-average SDE-values because of their low dependence on fruits (Rougès, 2004) and because they discard many seeds during fruit handling (Ruggera et al., 2014).
Large body mass species usually remove larger numbers of fruits and move seeds over longer distances than small species (Nathan et al., 2008; Wotton and Kelly, 2012; Morales et al., 2013). Although fruit-eating assemblages recorded in this study were entirely composed of small-sized birds (<100 g body mass), at least three medium-to-large bird species also dispersed P. parlatorei seeds in the undisturbed forest sites. Patagioenas fasciata (Band-tailed Pigeon) was occasionally recorded consuming P. parlatorei fruits. In the subtropical upper cloud forest, this pigeon is less abundant but is the only fruit-eating species that commutes long distances in short periods, being able to play a key role in long-distance seed dispersal of many fleshy-fruited plants. Intact seeds were found in feces of cracids [Penelope obscura (Dusky-legged Guan) and P. dabbenei (Red-faced Guan)]; although P. parlatorei is less important in their diet and represents an underused resource when other fruits become available (Ruggera et al., 2011). The inclusion of large-sized dispersers could lead to more functionally diverse assemblages, with higher complementary seed dispersal (Schleuning et al., 2015; Rother et al., 2016). This would happen if the large-sized species provide metapopulation benefits by promoting colonization of new patches and maintenance of genetic flow between populations, increasing the overall SDE (Schupp et al., 2010; McConkey and Brockelman, 2011). However, the absence of these species in disturbed forests, particularly guans, is alarming. Guans and other cracids suffer a strong hunting pressure, usually leading to numerical declines and local extinction (Strahl et al., 1997). Defaunation of large-sized animals can drive shifts in disperser assemblages often currently dominated by small-sized species, suppressing gene flow and negatively affecting the recruitment and metapopulation dynamics of plant species (Carvalho et al., 2016; Pérez-Méndez et al., 2016).
Although I did not evaluate temporal changes in P. parlatorei SDE, it could be expected that the functional role of the equivalent bird species within each habitat type reported in this study, will be roughly maintained over time. This is because there would be no reason to suppose that bird species will substantially modify their main habitat use patterns, in addition to the fact that the species that reach higher values of SDE are mostly abundant, for which fruits represent an important dietary item (Ruggera et al., 2016; Nazaro and Blendinger, 2017). Moreover, given the greater functional equivalence observed in the habitats where most of the recruitment occurs, long-term changes in the abundances of particular bird species could have low effect on P. parlatorei population dynamics. On the other hand, current changes in land use can modify over time the distribution and extent of forest successional stages in the landscape. Andean cloud forests in northwestern Argentina show a tendency to the abandonment of agricultural and grazing lands and recovery of secondary forest associated to decreasing rural population (Izquierdo and Grau, 2009; Grau et al., 2010). Under this scenario, secondary forests will progress in the succession to old-growth forests, where recruitment of P. parlatorei is negligible. Moreover, the high SDE of birds that inhabit forest edges and secondary forests can play an important role as catalyst of P. parlatorei recruitment. Given the particularly high SDE recorded in these habitats, one would expect an advance of forests dominated by P. parlatorei into abandoned or underused productive lands, although processes such as fire dynamics and rainfall patterns can limit this advance toward grasslands (Carilla and Grau, 2010).
Implications for Conservation
Forest degradation and fragmentation can result in a loss of fruit-eating species, in particular of old-growth forest specialists, and the consequent decrease in their seed dispersal service (Luck and Daily, 2003; Markl et al., 2012; Albrecht et al., 2013; Fontúrbel et al., 2015). However, secondary forests in Los Toldos showed increased SDE compared to old-growth forests dominated by P. parlatorei. The surprisingly high SDE in secondary forests may be explained by the combination of both a high frequency of fruit consumption by birds and the high availability of microhabitats that are favorable for seedling establishment and located close to seed sources. Assemblages of fruit-eating birds differ from those of old-growth forests in their greater richness given by the turnover by related species that favor more open environments and the persistence in low density of several species typical of old-growth forests. They include the Elaenia species already mentioned and other noteworthy species like T. rufiventris and T. sayaca, two of the main seed dispersers at lower elevation subtropical Andean forests (Blendinger et al., 2015), but less abundant in upper old-growth cloud forests (Ruggera et al., 2016). In summary, dispersal services depend on an enriched set of dispersers in secondary forests, where the high quantitative component of SDE is largely achieved by the spatial turnover of habitat specialists of closely related and morphologically similar habitat generalists, which are able to overcompensate for the loss of effective seed dispersers typical of mature forests.
Regarding the high seedling establishment recorded in secondary forests, Los Toldos valley is a mosaic of productive lands, shrubby grasslands, and patches of secondary forests of different age dominated by P. parlatorei, which grows in abandoned crops and grazing lands (Carilla and Grau, 2010). High rates of seedling establishment seem to occur primarily in habitat patches with discontinuous tree cover in a matrix of shrubs and open areas (PGB, pers. obs.). As in other Neotropical landscapes modified by humans (Sekercioglu et al., 2007; Pizo and dos Santos, 2011), fruiting trees along fences, stream margins, road edges, and old-secondary forest patches are the source of seeds dispersed toward shrubby grasslands and farming plots with a more recent history of abandonment. The existence of numerous seed sources and a habitat structurally similar to the ecotone between forest edges and shrubby grasslands (i.e., where recruitment reaches its maximum value in undisturbed sites) seem to have a synergistic effect on the qualitative component of SDE, favoring forest regeneration. It is noteworthy that in the same valley, P. parlatorei fails to recruit in adjacent grasslands subject to cattle ranching. This is not only explained by reduced SDE. Carilla and Grau (2010) proposed that P. parlatorei forest stands are not expanding over degraded grasslands due to interactive effects between land use, climate, grazing and fire which maintains grasslands as an alternative degraded state highly resistant to tree recruitment. Fire removes the shrubby cover that favor seedling establishment in grasslands, burns saplings, and produces soil degradation and desiccation. In consequence, recruitment of P. parlatorei in open areas results rather episodic, with temporal pulses over decades associated with periods of higher grazing intensity and below-average rainfall that reduces fuel availability to recurrent fires.
My observations have important implications for forest restoration. Cloud forests in the Southern Yungas of Bolivia and Argentina have undergone degradation and transformation as a consequence of logging, grazing, fire management and farming (Navarro and Maldonado, 2002; Grau et al., 2010), leading to the ban of the international trade of P. parlatorei since 1975 (UNEP-WCMC, 2015), among other conservation measures. Being a pioneer but very long-lived tree, which persists as a dominant species thorough most successional stages, this conifer plays an important role in the cloud forest dynamics and in the recruitment of other plant species (Arturi et al., 1998; Ramadori, 1998; Carilla and Grau, 2010), and is a key resource for wildlife (Ruggera et al., 2011; Pidgeon et al., 2015). The high effectiveness of seed dispersal in human disturbed areas found in this study gives P. parlatorei an outstanding management potential for the recovery of forests and lands degraded by overexploitation in the subtropical Andean cloud forests.
Conclusion
Fleshy-fruited plants depend on animals to disperse their seeds to adequate microhabitats, to increase seed and seedling survival probabilities, and to maintaining population viability and genetic flow among populations. To establish interactions with a diverse assemblage of fruit-eating species does not guarantee a successful interaction outcome. Functional equivalence in SDE may be very low, depending on the spatial context and identity of the disperser species. This study shows that compositional changes in fruit-eating assemblages mediated by human forest disturbance modify the functional equivalence, the generalization in mutualistic interactions and the strength of SDE in a tree-seed disperser mutualism. In habitats suitable for recruitment, SDE could strongly depend on attributes of the dispersers linked to their abundance rather than to their morphology or behavior; even not “legitimate” seed dispersers, such as pulp mashers, can be moderately effective dispersers. Even dominant tree species may depend on only one or a few highly effective mutualistic partners. An important message to conservation biology, and in particular to habitat restoration, is that the regional pool of animals may provide new highly effective small-sized dispersers that favor plant establishment in degraded habitats, functionally equivalent to species lost during habitat degradation.
Author Contributions
The author confirms being the sole contributor of this work and approved it for publication.
Funding
This study was funded by Consejo Nacional de Investigaciones Científicas y Técnicas (CONICET, Argentina). This research was partially supported by grants number PIP 592-2015 (CONICET) and PICT 2013-1280 (FONCyT).
Conflict of Interest Statement
The author declares that the research was conducted in the absence of any commercial or financial relationships that could be construed as a potential conflict of interest.
Acknowledgments
I thank Gabriela Núñez Montellano for her active participation in the field work. I want to thank Rodrigo Aráoz by generously sharing the E. albiceps photography. This study complies with the current laws of Argentina. Permission for conducting research in Sierra de San Javier Park was granted by the Universidad Nacional de Tucumán, and in Nogalar de Los Toldos National Reserve by the Administración de Parques Nacionales, Argentina.
Supplementary Material
The Supplementary Material for this article can be found online at: http://journal.frontiersin.org/article/10.3389/fevo.2017.00057/full#supplementary-material
References
Albrecht, J., Berens, D. G., Blüthgen, N., Jaroszewicz, B., Selva, N., and Farwig, N. (2013). Logging and forest edges reduce redundancy in plant-frugivore networks in an old-growth European forest. J. Ecol. 101, 990–999. doi: 10.1111/1365-2745.12105
Arturi, M. F., Grau, H. R., Aceñolaza, P. G., and Brown, A. D. (1998). Estructura y sucesión en bosques montanos del Noroeste de Argentina. Rev. Biol. Trop. 46, 525–532.
Bascompte, J., and Jordano, P. (2007). Plant-animal mutualistic networks: the architecture of biodiversity. Ann. Rev. Ecol. Evol. Syst. 38, 567–593. doi: 10.1146/annurev.ecolsys.38.091206.095818
Baselga, A. (2010). Partitioning the turnover and nestedness components of beta diversity. Glob. Ecol. Biogeogr. 19, 134–143. doi: 10.1111/j.1466-8238.2009.00490.x
Blendinger, P. G., Blake, J. G., and Loiselle, B. A. (2011). Connecting fruit production to seedling establishment in two co-occurring Miconia species: consequences of seed dispersal by birds in upper Amazonia. Oecologia 167, 61–73. doi: 10.1007/s00442-011-1956-6
Blendinger, P. G., Jiménez, J., Macchi, L., Martín, E., Sánchez, M. S., and Ayup, M. M. (2015). Scale-dependent spatial match between fruits and fruit-eating birds in Andean mountain forests. Biotropica 47, 702–711. doi: 10.1111/btp.12247
Blendinger, P. G., Loiselle, B. A., and Blake, J. G. (2008). Crop size, plant aggregation and microhabitat type affect fruit removal by birds from individual melastome plants in the Upper Amazon. Oecologia 158, 273–283. doi: 10.1007/s00442-008-1146-3
Bueno, R. S., Guevara, R., Ribeiro, M. C., Culot, L., Bufalo, F. S., and Galetti, M. (2013). Functional redundancy and complementarities of seed dispersal by the last Neotropical megafrugivores. PLoS ONE 8:e56252. doi: 10.1371/journal.pone.0056252
Calviño-Cancela, M. (2002). Spatial patterns of seed dispersal and seedling recruitment in Corema album (Empetraceae): the importance of unspecialized dispersers for regeneration. J. Ecol. 90, 775–784. doi: 10.1046/j.1365-2745.2002.00711.x
Carilla, J., and Grau, H. R. (2010). 150 years of tree establishment, land use and climate change in montane grasslands, Northwest Argentina. Biotropica 42, 49–58. doi: 10.1111/j.1744-7429.2009.00565.x
Carlo, T. A., and Morales, J. M. (2016). Generalist birds promote tropical forest regeneration and increase plant diversity via rare-biased seed dispersal. Ecology 97, 1819–1831. doi: 10.1890/15-2147.1
Carlo, T. A., and Yang, S. (2011). Network models of frugivory and seed dispersal: challenges and opportunities. Acta Oecol. 37, 619–624. doi: 10.1016/j.actao.2011.08.001
Carvalho, C. S., Galetti, M., Colevatti, R. G., and Jordano, P. (2016). Defaunation leads to microevolutionary changes in a tropical palm. Sci. Rep. 6:31957. doi: 10.1038/srep31957
Dehling, D. M., Töpfer, T., Schaefer, H. M., Jordano, P., Böhning-Gaese, K., and Schleuning, M. (2014). Functional relationships beyond species richness patterns: trait matching in plantÂŰbird mutualisms across scales. Glob. Ecol. Biogeogr. 23, 1085–1093. doi: 10.1111/geb.12193
Donatti, C. I., Guimarães, P. R., Galetti, M., Pizo, M. A., Marquitti, F. M. D., and Dirzo, R. (2011). Analysis of a hyper-diverse seed dispersal network: modularity and underlying mechanisms. Ecol. Lett. 14, 773–781. doi: 10.1111/j.1461-0248.2011.01639.x
Easdale, T. A., Gurvich, D. E., Sersic, A. N., and Healey, J. R. (2007). Tree morphology in seasonally dry montane forest in Argentina: relationships with shade tolerance and nutrient shortage. J. Veg. Sci. 18, 313–326. doi: 10.1111/j.1654-1103.2007.tb02543.x
Eklöf, A., Jacob, U., Kopp, J., Bosch, J., Castro-Urgal, R., Chacoff, N., et al. (2013). The dimensionality of ecological networks. Ecol. Lett. 16, 577–583. doi: 10.1111/ele.12081
Fleming, T. H., and Estrada, A. (Eds.) (1993). Frugivory and Seed Dispersal: Ecological and Evolutionary Aspects. Dordrecht: Kluwer Academic.
Fontúrbel, F. E., Candia, A. B., Malebrán, J., Salazar, D. A., González-Browne, C., and Medel, R. (2015). Meta-analysis of anthropogenic habitat disturbance effects on animal-mediated seed dispersal. Glob. Change Biol. 21, 3951–3960. doi: 10.1111/gcb.13025
Foster, M. S. (1987). Feeding methods and efficiencies of selected frugivorous birds. Condor 89, 566–580. doi: 10.2307/1368645
García, D., Obeso, J. R., and Martínez, I. (2005). Spatial concordance between seed rain and seedling establishment in bird-dispersed trees: does scale matter? J. Ecol. 93, 693–704. doi: 10.1111/j.1365-2745.2005.01004.x
Godínez-Alvarez, H., Valiente-Banuet, A., and Rojas-Martinez, A. (2002). The role of seed dispersers in the population dynamics of the columnar cactus Neobuxbaumia tetetzo. Ecology 83, 2617–2629. doi: 10.1890/0012-9658(2002)083[2617:TROSDI]2.0.CO;2
Grau, H. R., Carilla, J., Gil-Montero, R., Villalba, R., Araoz, E., Masse, G., et al. (2010). “Environmental history and forest regeneration dynamics in a degraded valley of north-west Argentina's cloud forests,” in Tropical Montane Cloud Forests: Science for Conservation and Management, eds L. A. Bruijnzeel, F. N. Scatena, and L. S. Hamilton (Cambridge: Cambridge University Press), 597–604.
Guimarães, P. R., Jordano, P., and Thompson, J. N. (2011). Evolution and coevolution in mutualistic networks. Ecol. Lett. 14, 877–885. doi: 10.1111/j.1461-0248.2011.01649.x
Hampe, A. (2008). Fruit tracking, frugivore satiation, and their consequences for seed dispersal. Oecologia 156, 137–145. doi: 10.1007/s00442-008-0979-0
Herrera, C. M. (1985). Determinants of plant-animal coevolution: the case of mutualistic dispersal of seeds by vertebrates. Oikos 44, 132–141. doi: 10.2307/3544054
Howe, H. F. (1993). Specialized and generalized dispersal systems: where does 'the paradigm' stand? Vegetatio 107, 3–13. doi: 10.1007/978-94-011-1749-4_1
Hunzinger, H. (1997). Hydrology of montane forests in the Sierra de San Javier, Tucumán, Argentina. Mt. Res. Dev. 17, 299–308. doi: 10.2307/3674020
Izquierdo, A. E., and Grau, H. R. (2009). Agriculture adjustment, land-use transition and protected areas in Northwestern Argentina. J. Environ. Manage. 90, 858–865. doi: 10.1016/j.jenvman.2008.02.013
Jordano, P. (1987). Patterns of mutualistic interactions in pollination and seed dispersal: connectance, dependence asymmetries, and coevolution. Am. Nat. 129, 657–677. doi: 10.1086/284665
Jordano, P. (1993). Geographical ecology and variation of plant-seed disperser interactions: southern Spanish junipers and frugivorous thrushes. Vegetatio 107, 85–104. doi: 10.1007/978-94-011-1749-4_6
Lavabre, J. E., Gilarranz, L. J., Fortuna, M. A., and Bascompte, J. (2016). How does the functional diversity of frugivorous birds shape the spatial pattern of seed dispersal? A case study in a relict plant species. Phil. Trans. R. Soc. B 371:20150280. doi: 10.1098/rstb.2015.0280
Lawton, J. H., and Brown, V. K. (1993). “Redundancy in ecosystems,” in Biodiversity and Ecosystem Function, eds E. D. Schulze and H. A. Mooney (New York, NY: Springer), 255–268.
Levey, D. J. (1987). Seed size and fruit-handling techniques of avian frugivores. Am. Nat. 129, 471–485. doi: 10.1086/284652
Levey, D. J., Silva, W. R., and Galetti, M. (eds.). (2002). Seed Dispersal and Frugivory: Ecology, Evolution and Conservation. Wallingford: CAB International Publishing.
Loayza, A. P., and Knight, T. (2010). Seed dispersal by pulp consumers, not “legitimate” seed dispersers, increases Guettarda viburnoides population growth. Ecology 91, 2684–2695. doi: 10.1890/09-0480.1
Luck, G. W., and Daily, G. C. (2003). Tropical countryside bird assemblages: richness, composition, and foraging differ by landscape context. Ecol. Appl. 13, 235–247. doi: 10.1890/1051-0761(2003)013[0235:TCBARC]2.0.CO;2
Markl, J. S., Schleuning, M., Forget, P. M., Jordano, P., Lambert, J. E., Traveset, A., et al. (2012). Meta-analysis of the effects of human disturbance on seed dispersal by animals. Conserv. Biol. 26, 1072–1081. doi: 10.1111/j.1523-1739.2012.01927.x
McConkey, K. R., and Brockelman, W. Y. (2011). Nonredundancy in the dispersal network of a generalist tropical forest tree. Ecology 92, 1492–1502. doi: 10.1890/10-1255.1
McConkey, K. R., Brockelman, W. Y., Saralamba, C., and Nathalang, A. (2015). Effectiveness of primate seed dispersers for an “oversized” fruit, Garcinia benthamii. Ecology 96, 2737–2747. doi: 10.1890/14-1931.1
Moermond, T. C., and Denslow, J. S. (1985). Neotropical avian frugivores: patterns of behavior, morphology, and nutrition, with consequences for fruit selection. Ornithol. Monogr. 36, 865–897. doi: 10.2307/40168322
Morales, J. M., García, D., Martínez, D., Rodriguez-Pérez, J., and Herrera, J. M. (2013). Frugivore behavioural details matter for seed dispersal: a multi-species model for Cantabrian thrushes and trees. PLoS ONE 8:e65216. doi: 10.1371/journal.pone.0065216
Nathan, R., Schurr, F. M., Spiegel, O., Steinitz, O., Trakhtenbrot, A., and Tsoar, A. (2008). Mechanisms of long-distance seed dispersal. Trends Ecol. Evol. 23, 638–647. doi: 10.1016/j.tree.2008.08.003
Navarro, G., and Maldonado, M. (2002). Geografía Ecológica de Bolivia: Vegetación y Ambientes Acuáticos. Cochabamba: Fundación Simón Patiño.
Nazaro, M. G., and Blendinger, P. G. (2017). How important are arthropods in the diet of fruit-eating birds? Wilson J. Ornithol. 129.
Pérez-Méndez, N., Jordano, P., García, C., and Valido, A. (2016). The signatures of Anthropocene defaunation: cascading effects of the seed dispersal collapse. Sci. Rep. 6:24820. doi: 10.1038/srep24820
Pidgeon, A. M., Rivera, L., Martinuzzi, S., Politi, N., and Bateman, B. (2015). Will representation targets based on area protect critical resources for the conservation of the Tucuman Parrot? Condor 117, 503–517. doi: 10.1650/CONDOR-14-214.1
Pizo, M. A., and dos Santos, B. T. P. (2011). Frugivory, post-feeding flights of frugivorous birds and the movement of seeds in a Brazilian fragmented landscape. Biotropica 43, 335–342. doi: 10.1111/j.1744-7429.2010.00695.x
Prasad, S., and Sukumar, R. (2010). Context-dependency of a complex fruit–frugivore mutualism: temporal variation in crop size and neighborhood effects. Oikos 119, 514–523. doi: 10.1111/j.1600-0706.2009.17971.x
Quiroga, M. P., Pacheco, S., Malizia, L. R., and Premoli, A. C. (2012). Shrinking forests under warming: evidence of Podocarpus parlatorei (pino del cerro) from the subtropical Andes. J. Hered. 103, 682–691. doi: 10.1093/jhered/ess031
Ramadori, D. (1998). Sucesión Secundaria en Bosques Montanos del Noroeste Argentino. Ph.D. dissertation. Universidad Nacional de La Plata.
Robertson, A. W., Trass, A., Ladley, J. J., and Kelly, D. (2006). Assessing the benefits of frugivory for seed germination: the importance of the deinhibition effect. Funct. Ecol. 20, 58–66. doi: 10.1111/j.1365-2435.2005.01057.x
Robledo-Arnuncio, J. J., Klein, E. K., Muller-Landau, H. C., and Santamaría, L. (2014). Space, time and complexity in plant dispersal ecology. Mov. Ecol. 2:16. doi: 10.1186/s40462-014-0016-3
Rother, D. C., Pizo, M. A., and Jordano, P. (2016). Variation in seed dispersal effectiveness: the redundancy of consequences in diversified tropical frugivore assemblages. Oikos 125, 336–342. doi: 10.1111/oik.02629
Rougès, M. (2004). Bird Community Dynamics along an Altitudinal Gradient in Subtropical Montane Forests. Ph.D. dissertation. University of Missouri-St. Louis.
Ruggera, R. A., Blendinger, P. G., Gomez, M. D., and Marshak, C. (2016). Linking structure and functionality in mutualistic networks: do core frugivores disperse more seeds than peripheral species? Oikos 125, 541–555. doi: 10.1111/oik.02204
Ruggera, R. A., Gomez, D., and Blendinger, P. G. (2014). Frugivory and seed dispersal role of the Yellow-striped Brush-Finch (Atlapetes citrinellus), an endemic emberizid of Argentina. Emu 114, 343–351. doi: 10.1071/MU14033
Ruggera, R. A., Álvarez, M. E., and Blendinger, P. G. (2011). Dieta de la Pava de Monte Alisera (Penelope dabbenei) en un bosque montano del noroeste de Argentina. Ornitol. Neotrop. 22, 615–621.
Schleuning, M., Fründ, J., and García, D. (2015). Predicting ecosystem functions from biodiversity and mutualistic networks: an extension of trait-based concepts to plant-animal interactions. Ecography 38, 380–392. doi: 10.1111/ecog.00983
Schupp, E. W. (2007). “The suitability of a site for seed dispersal is context-dependent,” in Seed Dispersal: Theory and its Implications in a Changing World, eds A. Dennis, R. Green, E. W. Schupp, and D. A. Westcott (Wallingford: CABI Publishing), 445–462.
Schupp, E. W., Jordano, P., and Gómez, J. M. (2010). Seed dispersal effectiveness revisited: a conceptual review. New Phytol. 188, 333–353. doi: 10.1111/j.1469-8137.2010.03402.x
Sekercioglu, C. H., Loarie, S. R., Oviedo Brenes, F., Ehrlich, P. R., and Daily, G. C. (2007). Persistence of forest birds in the Costa Rican agricultural countryside. Cons. Biol. 21, 482–494. doi: 10.1111/j.1523-1739.2007.00655.x
Strahl, S. D., Beaujon, S., Brooks, D. M., Begazo, A. J., Sedaghatkish, G., and Olmos, F. (Eds.) (1997). The Cracidae: their Biology and Conservation. Blaine, WA: Hancock House Publishers.
Thompson, J. N. (2006). Mutualistic webs of species. Science 312, 372–373. doi: 10.1126/science.1126904
Traveset, A., Robertson, A. W., and Rodríguez-Pérez, J. (2007). “A review on the role of endozoochory on seed germination,” in Seed Dispersal: Theory and its Implications in a Changing World, eds A. Dennis, R. Green, E. W. Schupp, and D. A. Westcott (Wallingford: CABI Publishing), 78–103.
UNEP-WCMC (2015). The Checklist of CITES Species Website. CITES Secretariat, Geneva, Switzerland. Compiled by UNEP-WCMC, Cambridge, UK. Available online at: http://checklist.cites.org (Accessed Feb 10, 2017).
Vázquez, D. P., Morris, W. F., and Jordano, P. (2005). Interaction frequency as a surrogate for the total effect of animal mutualists on plants. Ecol. Lett. 8, 1088–1094. doi: 10.1111/j.1461-0248.2005.00810.x
Wang, B. C., and Smith, T. B. (2002). Closing the seed dispersal loop. Trends Ecol. Evol. 17, 379–385. doi: 10.1016/S0169-5347(02)02541-7
Wellnitz, T., and LeRoy Poff, N. (2001). Functional redundancy in heterogeneous environments: implications for conservation. Ecol. Lett. 4, 177–179. doi: 10.1046/j.1461-0248.2001.00221.x
Wotton, D. M., and Kelly, D. (2012). Do larger frugivores move seeds further? Body size, seed dispersal distance, and a case study of a large, sedentary pigeon. J. Biogeogr. 39, 1973–1983. doi: 10.1111/jbi.12000
Keywords: disperser effectiveness, Elaenia, fruit-eating birds, neotropical yungas forests, plant recruitment, Podocarpus parlatorei, spatial context, Turdus
Citation: Blendinger PG (2017) Functional Equivalence in Seed Dispersal Effectiveness of Podocarpus parlatorei in Andean Fruit-Eating Bird Assemblages. Front. Ecol. Evol. 5:57. doi: 10.3389/fevo.2017.00057
Received: 01 March 2017; Accepted: 15 May 2017;
Published: 09 June 2017.
Edited by:
Tomás A. Carlo, Pennsylvania State University, United StatesReviewed by:
Marco Aurélio Pizo, Universidade Estadual Paulista Júlio Mesquita Filho, BrazilJordan Karubian, Tulane University, United States
Copyright © 2017 Blendinger. This is an open-access article distributed under the terms of the Creative Commons Attribution License (CC BY). The use, distribution or reproduction in other forums is permitted, provided the original author(s) or licensor are credited and that the original publication in this journal is cited, in accordance with accepted academic practice. No use, distribution or reproduction is permitted which does not comply with these terms.
*Correspondence: Pedro G. Blendinger, blendinger@birdecology.com.ar