- 1Department of Ecology, Bioscience Institute, University of São Paulo, São Paulo, Brazil
- 2Department of Ecology and Evolutionary Biology, University of California, Los Angeles, Los Angeles, CA, United States
- 3Department of Biogeography and Global Change, BGC-Museo Nacional de Ciencias Naturales-CSIC, Madrid, Spain
- 4Department of Zoology and Laboratory of Ornithology, Palacky University, Olomouc, Czechia
- 5Behavioral and Physiological Ecology Group, Centre for Ecological and Evolutionary Studies, University of Groningen, Groningen, Netherlands
- 6Department of Wetland Ecology, Estación Biológica de Doñana, CSIC, Sevilla, Spain
- 7Nature Inventory and EIA-Services, Arctic Centre, University of Lapland, Rovaniemi, Finland
- 8Department of Zoology, Institute of Ecology and Earth Sciences, University of Tartu, Tartu, Estonia
- 9Ecology Research Group, Hungarian Academy of Sciences, Hungarian Natural History Museum, Eötvös Loránd University, Budapest, Hungary
- 10Behavioural Ecology Group, Department of Systematics, Zoology and Ecology, Eötvös Loránd University, Budapest, Hungary
- 11Department of Plant Pathology, Szent István University, Budapest, Hungary
- 12Institute of Zoology, Poznań University of Life Sciences, Poznan, Poland
- 13Ecologie Systématique Evolution, Centre National de la Recherche Scientifique, Université Paris-Sud, AgroParisTech, Université Saclay, Orsay, France
Behavioral adjustment is a key factor that facilitates species' coexistence with humans in a rapidly urbanizing world. Because urban animals often experience reduced predation risk compared to their rural counterparts, and because escape behavior is energetically costly, we expect that urban environments will select for increased tolerance to humans. Many studies have supported this expectation by demonstrating that urban birds have reduced flight initiation distance (FID = predator-prey distance when escape by the prey begins) than rural birds. Here, we advanced this approach and, for the first time, assessed how 32 species of birds, found in 92 paired urban-rural populations, along a 3,900 km latitudinal gradient across Europe, changed their predation risk assessment and escape strategy as a function of living in urban areas. We found that urban birds took longer than rural birds to be alerted to human approaches, and urban birds tolerated closer human approach than rural birds. While both rural and urban populations took longer to become aware of an approaching human as latitude increased, this behavioral change with latitude is more intense in urban birds (for a given unit of latitude, urban birds increased their pre-detection distance more than rural birds). We also found that as mean alert distance was shorter, urban birds escaped more quickly from approaching humans, but there was no such a relationship in rural populations. Although, both rural and urban populations tended to escape more quickly as latitude increased, urban birds delayed their escape more at low latitudes when compared with rural birds. These results suggest that urban birds in Europe live under lower predation risk than their rural counterparts. Furthermore, the patterns found in our study indicate that birds prioritize the reduction of on-going monitoring costs when predation risk is low. We conclude that splitting escape variables into constituent components may provide additional and complementary information on the underlying causes of escape. This new approach is essential for understanding, predicting, and managing wildlife in a rapidly urbanizing world.
Introduction
Urbanization is one of the main drivers of the current global biodiversity crisis (Foley et al., 2005; Grimm et al., 2008; McDonald, 2008). More than half of the world's humans live in cities and this proportion is expected to increase over the next decades (United Nations, 2014). In addition, the urban areas are increasing at an even greater rate than the urban population (United Nations, 2014). Given a rapidly urbanizing world, we need to better understand the traits that permit species to coexist with humans, which are often perceived as predators by animals (Frid and Dill, 2002). In this sense, behavioral plasticity is one of the key elements that permits species to coexist with humans (Sol et al., 2013). For instance, because escape from non-threatening humans is costly (Ydenberg and Dill, 1986; Cooper and Frederick, 2007; Samia et al., 2016), and because humans in cities rarely hunt or otherwise intentionally kill animals (Berger, 2007), urban prey are expected to respond to humans by reducing costly anti-predator behavior. In addition, humans in urban areas often displace predators (Møller, 2012), creating human shields where prey are relatively safe (Berger, 2007; Ibáñez-Álamo et al., 2012; Møller, 2012). If animals in urban areas experience reduced risk of predation by their native predators, this should select for reduced anti-predator responses.
In line with these expectations, a growing body of literature has shown that animals generally have relaxed anti-predator behavior in urban environments (e.g., Møller and Ibáñez-Álamo, 2012). In particular, individuals in urban populations generally tolerate more human disturbance by reducing their flight initiation distances (FID = predator-prey distance when escape of the prey begins; Figure 1A) compared to rural populations (Samia et al., 2015c). Despite this apparently ubiquitous pattern, the drivers of the rural-urban difference in human tolerance have just begun to be explored. Recent studies indicate an important role of a species' body size on urbanization with larger birds being more tolerant (Samia et al., 2015c) and having increased survivorship in cities (Brown and Graham, 2015). Brain size also plays a key role: successful urban invaders and exploiters are characterized by having larger brains (Sol et al., 2005, 2013; Maklakov et al., 2011) and several studies have shown a negative correlation between brain mass and FID (Møller and Erritzøe, 2014; Samia et al., 2015a; but see Carrete and Tella, 2011). Studies also suggest that big-brained species are more innovative and flexible in their ability to deal with the novel challenges imposed by urban environments, such as the chronic disturbance caused by human presence. Because predation risk is expected to decrease with group size (Hamilton, 1971; Pulliam, 1973; Alexander, 1974), birds in larger flocks might tolerate more human disturbance than birds alone or in smaller flocks (Dill and Ydenberg, 1987; Reimers et al., 2006), although current evidence does not show a substantial effect of flock size on tolerance to humans of urban birds (Møller, 2015; Samia et al., 2015c). Some studies also suggest that increased tolerance to humans is a function of disturbance frequency (Levey et al., 2009; Engelhardt and Weladji, 2011; McGiffin et al., 2013), which suggests that either the individual habituation process (i.e., phenotypic plasticity; Blumstein, 2016), differential sorting according to individual's personalities (Carrete and Tella, 2013), or strong selection against fearful individuals (Carrete et al., 2016) may be involved in generating tolerance.
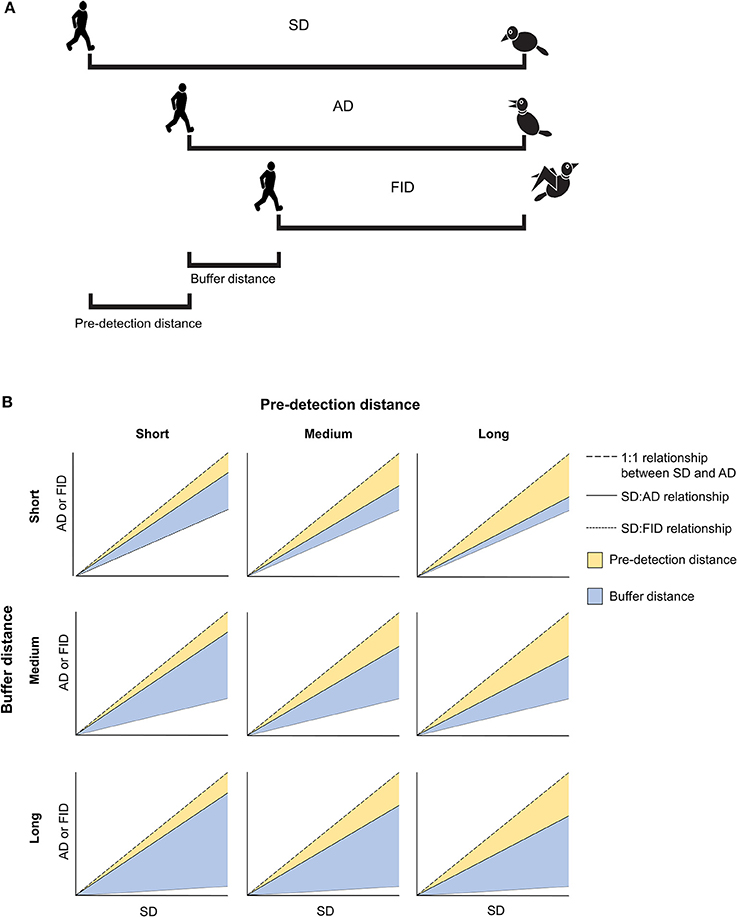
Figure 1. (A) Illustration of the five anti-predator behaviors considered in the present study. Starting distance (SD) is measured as the distance at which an approaching human begins to approach a prey. Alert distance (AD) is measured as the distance at which prey responds by orienting toward the approaching human. Flight initiation distance (FID) is measured as the human-prey distance when the prey begins to flee. Pre-detection distance is a measure of how long a prey takes to become aware of an approaching human (i.e., the difference between SD and AD). Buffer distance is an estimate of tolerance calculated as the distance at which a prey fled after being alerted by an approaching human (i.e., the difference between AD and FID). (B) Some hypothetical relationships between SD, AD, and FID as a function of short, medium, or long pre-detection distances and short, medium, or long buffer distances.
Among many recognized correlates of human tolerance, latitude has recently been identified as a potentially important correlate of tolerance in rural and urban environments. Latitude is used as a proxy for predation risk since predator density tends to decrease with increasing latitude (Laurila et al., 2008; Schemske et al., 2009). In a study of 159 species of European birds, FID decreased as latitude increased, in parallel with a clinal reduction in raptor abundance (raptors are key avian predators; Díaz et al., 2013). Other interspecific studies of FID across latitudinal gradients—or among continents—have recently revealed novel patterns in escape behavior (Møller et al., 2014, 2016; Samia et al., 2015b). However, these large scale studies have not considered a main predictor of FID, the prey's alert distance (AD; Figure 1A), which is the predator-prey distance when an individual prey becomes aware of and begins to monitor the predator (Cooper and Blumstein, 2015). Along with body mass, AD explains most of variation in FID in taxa studied to date (Samia et al., 2013; Cooper et al., 2015). A strong relationship between FID and AD implies that they both reflect the general level of fear that individuals experience while both scanning for predators and fleeing following a predator's approach. Variation in the strength of this relationship will however reflect different escape tactics in response to varying levels of predation risk (Cooper and Blumstein, 2014).
While a robust body of evidence has shown that FID is generally smaller in urban than rural places (review in Samia et al., 2015c), latitudinal studies of rural-urban differences in FID that account for variation in AD should permit us, for the first time, to assess how birds modify their predation risk assessment and escape strategy (in addition to modifying their FID) when living in cities. These anti-predatory decisions can be inferred from two variables. First, the prey's pre-detection distance (Figure 1A), which is a measure of how long a prey takes to become aware of an approaching human [i.e., the distance between starting distance (SD) and AD; where SD is the distance to the bird when approach begins]. Second, the prey's buffer distance (Figure 1A), which is a more rigorous estimate of tolerance estimated as the distance at which a prey fled “after being alerted by an approaching human” (i.e., the distance between AD and FID; Cooper and Blumstein, 2015). Using this approach, we can explore whether the previously identified reduction in urban birds' FID is traded-off with an increase in other costly anti-predatory behaviors. For instance, a shorter FID associated with a larger AD might imply increased vigilance costs since the prey's attention cannot simultaneously be focused on other fitness-enhancing activities, such as foraging. By contrast, a shorter FID associated with a shorter AD would result in a shorter buffer distance, implying that birds escape sooner after detecting an approaching human. If this reduction in tolerance is associated with a longer pre-detection distance, it may illustrate a strategy by which birds reduce their monitoring costs. Figure 1B illustrates these and other hypothesized scenarios.
We studied the difference in escape decisions of urban and adjacent rural populations of 32 bird species along a large latitudinal gradient across Europe (3,900 km). We investigated whether rural and urban birds differ in their awareness and tolerance along this gradient by estimating their pre-detection distance and buffer distance. We also studied the factors potentially related to rural-urban differences in these anti-predatory indicators by exploring seven potential correlates of pre-detection distance and buffer distance: latitude, species' body mass, species' brain mass, number of humans living in the city, rural-urban difference in mean SD (hereafter, ΔSD), rural-urban difference in mean AD (hereafter, ΔAD), and rural-urban difference in mean flock size (hereafter, Δflock size). Our hypotheses concerning the effect of these predictor variables are summarized in Table 1.
Materials and Methods
Field Data Collection
We collected data from April to September 2015 (breeding season) using a standard protocol (Blumstein, 2006). Data were collected in urban and adjacent rural areas of 10 cities from eight European countries: Czech Republic, Denmark, Estonia, Finland, France, Hungary, Poland, and Spain (data set available in Supplementary Material 1). Observers used binoculars to identify birds that were foraging or engaged in “relaxed behavior” (i.e., roosting or preening). Highly vigilant or obviously alarmed individuals, or individuals near their nests were not approached. Subsequently, each individual bird was approached in a straight line by a human walking at a constant speed (0.5 m/s). The distance at which the experimenter started to approach the focal bird was recorded as SD, while the distance at which the bird first oriented toward the approaching human and stopped its previous activity was recorded as AD, and the distance at which the animal began to flee was recorded as FID. The number of birds within a radius of 10 m around the focal individual was recorded as flock size. We avoided sampling the same individual twice by moving to another site immediately after samples were taken. If the same general area was visited, only individuals of different species, sex or age than those sampled before were tested. A modest degree of re-sampling subjects, however, has been shown to not influence the results of studies like this (Runyan and Blumstein, 2004).
We used a paired study design with one urban and one rural area in each study location. The distance between each pair of urban and rural site varied between 1 and 20 km. All urban study sites included both urban centers, characterized by areas with multi-story buildings, as well as suburban areas, characterized by areas with single-family houses. Rural areas were dominated by open farmland with scattered houses. Definitions of urban (at least 50% of built-up area, building density >10 buildings/ha and, a residential human density >10 humans/ha) and rural habitats (5–20% built-up areas, a building density <2.5 buildings/ha, and residential human density between 1 and 10 humans/ha) follow Marzluff et al. (2001). The benefit of this paired study design is that neighboring study sites will share most potentially confounding environmental characteristics (e.g., weather, altitude, soil), yet philopatry and assortative mating may decrease, or even prevent, movements between urban and rural study sites (Møller, 2009, 2015).
Additional Data
Information about latitude and human population size of the cities were extracted from Wikipedia (https://www.wikipedia.org). Body mass information was extracted from Cramp and Perrins (1977–1994). Brain mass information was extracted from Møller and Erritzøe (2014). As explained above, mean flock size was estimated by field observations of individual birds.
Calculating the Phi Index
The relationship between SD, AD, and FID is constrained by an envelope. This means that AD can only assume values ≤ SD (according to methodology applied to study escape behavior, an individual is never experimentally approached if already alerted by an approaching observer; see Section Field Data Collection) and FID can only assume values ≤ AD (a prey individual cannot escape from a predator before it has detected it). The phi index (Φ) is a goodness-of-fit metric that was originally developed to provide estimates, unbiased by this envelope relationship, of how close FID is to AD, which corresponds to our definition of buffer distance (Samia and Blumstein, 2014). Formally,
where, ei is the AD, oi is the FID, and n is the sample size. Nevertheless, Φ can also be used to estimate how close AD (in this case, oi) is to SD (ei) and then estimate the pre-detection distance. Importantly, Φ can be used as an effect size measure, which provides the magnitude of the effect of SD on AD (hereafter, pre-detection-Φ) and of AD on FID (hereafter, buffer-Φ; Samia and Blumstein, 2014). Φ is a standardized metric ranging from 0 to 1. Pre-detection-Φ values closer to 1 imply that birds were aware of approaching humans as soon as the human started to approach the focal bird, while buffer-Φ values closer to 1 imply that birds escape as soon as they were alerted to an approaching human.
Statistical Methods
We fitted a phylogenetically informed mixed-effect model using the rma.mv function of the metafor R package (Viechtbauer, 2010). Because there were multiple observations for each species, and because a shared evolutionary history makes data statistically dependent (Garamszegi, 2014), we incorporated species identity as a random factor while controlling for the phylogenetic dependence among species using a recent phylogenetic hypothesis (Jetz et al., 2012; Supplementary Figure 1).
Our dependent variables were the rural-urban difference in Φ estimated for a species from a single city (e.g., Φ of the rural population of Passer domesticus from around Madrid minus the Φ of the urban population of P. domesticus from the city of Madrid); hereafter termed Δpre-detection-Φ and Δbuffer-Φ. The use of these dependent variables emphasizes our aim of studying rural-urban differences in these antipredator behaviors. We only included populations with at least eight observations per urban site and at least eight observations per rural site (i.e., at least 16 observations per species' population) in the analyses to avoid estimates based on very small sample sizes.
We tested the effect of the predictors on Δpre-detection-Φ and Δbuffer-Φ using models that were weighted by sample size to account for differences in sampling effort among populations (Garamszegi and Møller, 2011; Garamszegi, 2014). The inverse sample size of a given species' population (= contrast) was used as a proxy for variance in the rma.mv function (Garamszegi, 2014). Continuous variables were log10-transformed before analyses to ensure normality of residuals.
We performed stepwise backward model selection based on corrected Akaike Information Criteria (AICc), using a threshold AICc value of 2. We present both full and minimum adequate models. We assessed the importance of each predictor based on their effect sizes calculated as partial correlation coefficients (Nakagawa and Cuthill, 2007). We followed criteria listed by Cohen (1997) for small (r = 0.10, explaining 1% of the variance), intermediate (r = 0.3, explaining 9% of the variance) or large effect sizes (r = 0.5, explaining 25% of the variance). As expected, brain mass was strongly positively correlated with body mass (r = 0.9), but the remaining predictor variables had low multicollinearity (all variance inflation factors, VIFs, <1.21). Multiple regression is the best approach to control for undesirable confounding effects among correlated covariates (such as body and brain masses), yielding unbiased coefficient estimates (Freckleton, 2002). For this reason, we retained body mass and brain mass in the same model to control for their confounded effect. Moreover, we found no relationship between body mass and latitude (model's P = 0.36; effect size r = −0.07), or body mass and an interaction between latitude and site (rural × urban; model's P = 0.94; effect size r = −0.005), suggesting that any effect of latitude on the escape variables was not confounded by a potential latitudinal increase in avian body mass. All analyses were conducted with R version 3.2.2 (R Core Team, 2014).
Results
Our final data set contained 5,987 observations of 32 bird species, resulting in 92 paired comparisons of rural and urban populations (Supplementary Material 1). Paired t-tests showed that, on average, SD, AD, FID, and buffer-Φ were smaller in urban than in matched rural populations, all with large effect sizes (Figure 2). Although the SD used to approach urban birds was much smaller than the SD for rural birds (with a large effect size, r = 0.64), urban birds took longer than rural birds to be alerted to an approaching human, as evidenced by a smaller pre-detection-Φ in urban populations (the rural-urban difference had a large effect size; Figure 2). Flock size did not differ between rural and urban populations (with a very small effect size; Figure 3), suggesting that urban birds did not take longer to detect humans because of fewer eyes to detect predators.
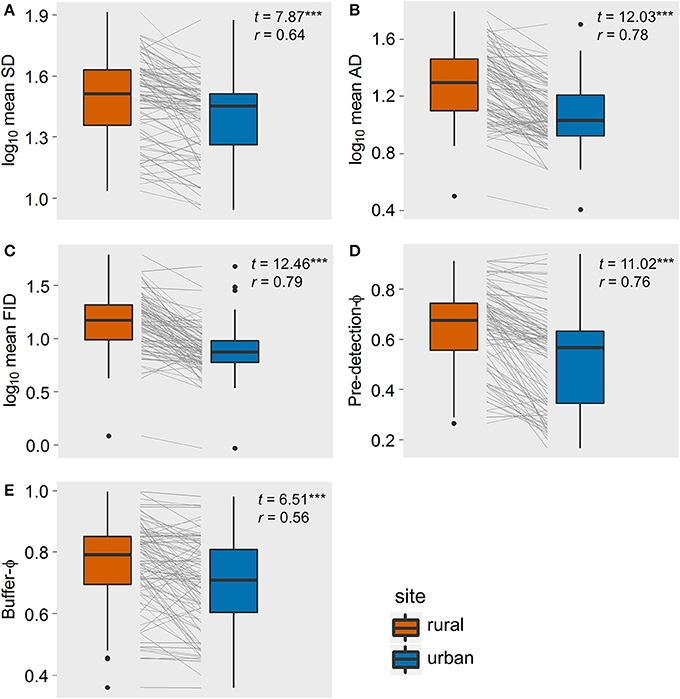
Figure 2. The rural-urban differences of five components of escape behavior of the 92 bird populations studied in Europe: (A) starting distance, SD; (B) alert distance, AD, (C) flight initiation distance, FID; (D) pre-detection-Φ (lower values mean that birds took longer to be alerted to an approaching human); (E) buffer-Φ (lower values mean that birds tolerate more human approach after detection). Box-plots show median, quartiles, 5- and 95-percentiles and extreme values. Each population's trajectory is illustrated with a gray line. t-values and effect sizes calculated as partial correlation coefficients are shown. All t-values were highly significant (***P < 0.001).
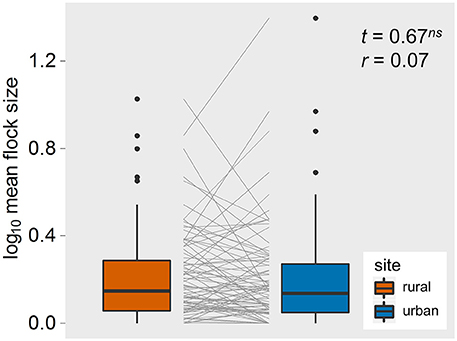
Figure 3. The rural-urban differences in flock size of the 92 bird populations studied in Europe. Box-plots show median, quartiles, 5- and 95-percentiles and extreme values. Each species' trajectory is illustrated with a gray line. t-values and effect sizes calculated as partial correlation coefficients are shown. ns, non-significant.
The minimum adequate model of Δpre-detection-Φ retained only one variable, latitude, which had a large effect size (Table 2). We found that the rural-urban difference in pre-detection distance increased with latitude (Table 2, Figure 4A). Specifically, we found that although both rural and urban populations tended to take longer to be alerted to an approaching human as latitude increased (i.e., both have smaller pre-detection-Φ values as latitude increased), urban birds took even longer than rural birds as latitude increased (Figures 4A,B).
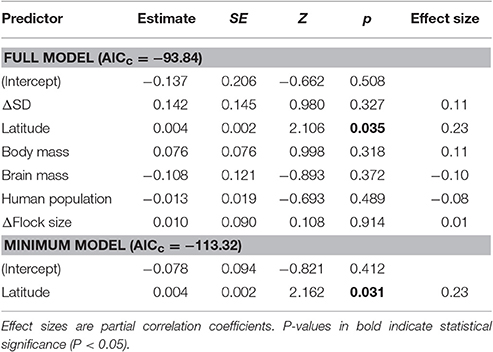
Table 2. Full and minimum adequate models explaining the rural-urban differences in pre-detection distance (Δpre-detection-Φ) of European birds.
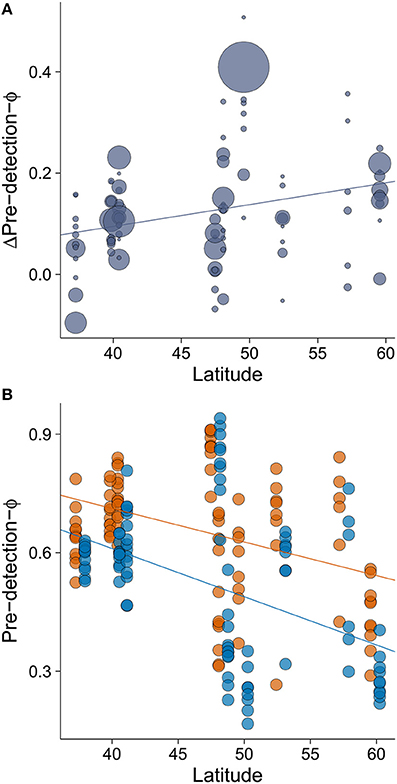
Figure 4. The relationship between (A) latitude and rural-urban difference in pre-detection-Φ (Δpre-detection-Φ) and (B) the relationship between latitude and pre-detection-Φ by site. Lower values of pre-detection-Φ mean that birds took longer to be alerted to an approaching human. The size of the circles in plot A is proportional to sample size for a given population (min = 19, max = 358). In plot (B), orange points and orange line represent rural populations whereas blue points and blue line represent urban populations. Circle size was fixed but points representing urban birds have been slightly displaced to the right (0.7° of latitude) to improve data visualization. Lines illustrate linear regressions.
The minimum adequate model of Δbuffer-Φ retained two variables: ΔAD (intermediate to large effect size) and latitude (small to intermediate effect size; Table 3). We found that the rural-urban differences in buffer distance increased with the difference in their mean AD (Table 3, Figure 5A). When we excluded an outlier with high leverage from analysis, we found that rural birds did not have a significant relationship between mean AD and buffer-Φ (Figure 5B), while urban birds had a negative relationship between these variables (P = 0.019; Figure 5C). The latter relationship was marginally significant when the outlier was kept in the analysis (P = 0.053; Supplementary Figure 2). We also found that rural-urban differences in buffer distance decreased as latitude increased (Table 3, Figure 6A). Although both rural and urban populations tended to escape sooner once alerted, as latitude increased (i.e., both have larger Φ-values as latitude increased), urban birds tended to delay their escape more at low latitudes when compared with rural birds (Figure 6B).
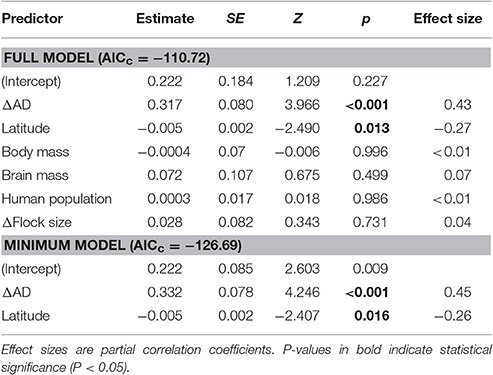
Table 3. Full and minimum adequate models explaining the rural-urban differences in buffer distance (Δbuffer-Φ) of European birds.
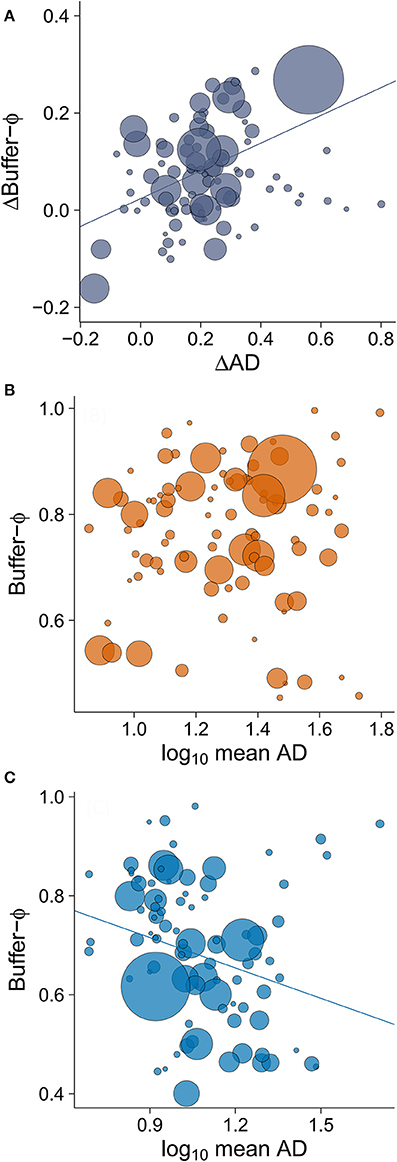
Figure 5. The relationship between (A) rural-urban difference in mean alert distance (ΔAD) and rural-urban difference in buffer-Φ (Δbuffer-Φ), and the relationship between mean AD and buffer-Φ in (B) rural and (C) urban sites. Lower values of buffer-Φ mean that birds tolerate more human approach after detection. The size of the circles is proportional to sample size for a given population (plot A: min = 19, max = 358; plot B: min = 8, max = 122; plot C, min = 8, max = 275). Lines illustrate linear regressions.
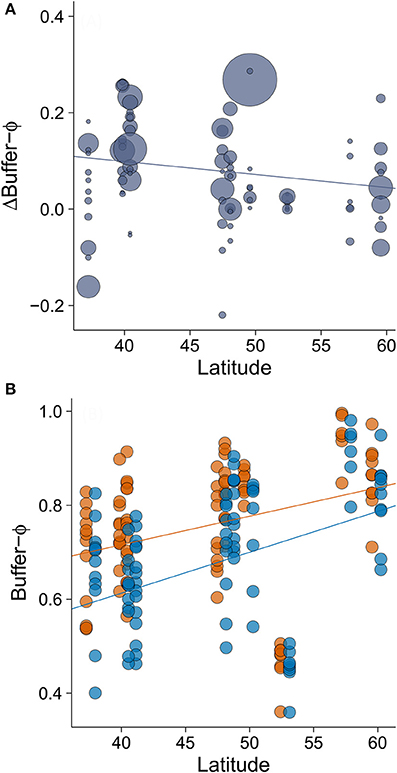
Figure 6. The relationship between (A) latitude and rural-urban difference in buffer-Φ (Δbuffer-Φ) and (B) the relationship between latitude and buffer Φ by site. Lower values of buffer-Φ mean that birds tolerate more human approach after detection. Circle size in plot A is proportional to the sample size for a given population (min = 19, max = 358). In plot B, orange points and orange line represent rural populations whereas blue points and blue line represent urban populations. Circle size was fixed and points representing urban birds have been slightly displaced to right (0.7 degrees of latitude) to improve data visualization. Lines illustrate linear regressions.
Finally, regression between buffer-Φ and pre-detection-Φ by site revealed an interesting pattern (Figure 7). In rural environments, populations that were alerted soon after a human began approaching them responded by delaying or anticipating flight, whereas all but one population that took longer to become alerted to humans escaped soon afterwards (Figure 7A). By contrast, in urban environments, the significant negative relationship between buffer-Φ and pre-detection-Φ (small to intermediate effect size, r = −0.26; intercept = 0.783, b = −0.203, P = 0.012) suggests a trade-off between these escape decisions (Figure 7B).
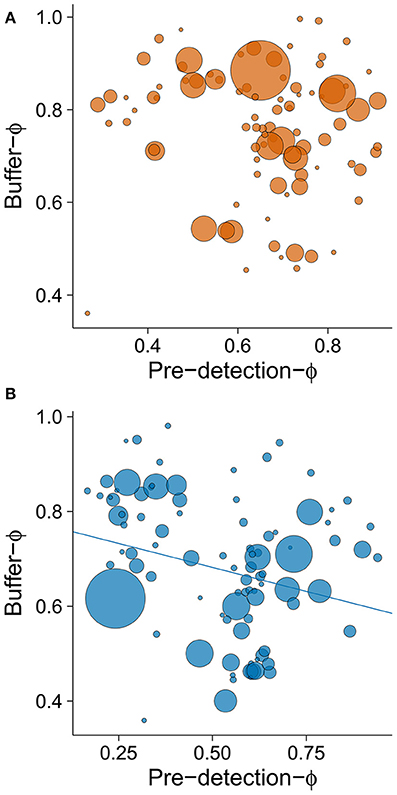
Figure 7. The relationship between pre-detection-Φ and the buffer-Φ for (A) rural and (B) urban populations. Lower values of pre-detection-Φ mean that birds took longer to be alerted to an approaching human, whereas lower values of buffer-Φ mean that birds tolerate more human approach after detection. Circle size is proportional to sample size for a given population (plot A: min = 8, max = 122; plot B: min = 8, max = 275). Line illustrates linear regression.
Discussion
When compared with rural birds, urban European birds have shorter alert distances (AD), flight initiation distances (FID), and delay escape more (as reflected by smaller buffer-Φ values). Despite the fact that the starting distance (SD) used to approach individual birds was much smaller in urban sites, urban birds took longer to be alerted by an approaching human (as reflected by a smaller pre-detection-Φ values), making our conclusions even more robust. Despite the fact that the observer is partly determining SD, it is also a feature of habitats and species associated with specific habitats. In urban habitats, birds may observe an approaching human or predator later because of high building density or because of urban noise, and therefore urban birds may have less time to make their escape decisions. Importantly, since AD and FID were also substantially reduced in urban habitats (both with large effect sizes), the large reduction in pre-detection distance and buffer distances in urban locations were not an artifact caused by Φ, a metric which calculates the relative, not the absolute, difference between these behavioral estimates (Samia and Blumstein, 2014).
The great reduction in anti-predatory responses in urban environments suggests that urban birds in Europe live under lower predation risk than their rural counterparts, a causal hypothesis supported by previous studies (e.g., Anderies et al., 2007; Møller and Ibáñez-Álamo, 2012; Díaz et al., 2013). Economic escape theory postulates that prey should counterbalance predation risk and the costs of fleeing (which includes the loss of foraging opportunities) when deciding to escape from a potential predator (Ydenberg and Dill, 1986; Cooper and Frederick, 2007). By reducing awareness and delaying flight after detecting a potential threat, urban birds are expected to be able to allocate more of their limited energy to other key activities, such as foraging and reproduction (Ydenberg and Dill, 1986; Cooper and Frederick, 2007). Indeed, urban birds often have longer reproductive periods and more breeding bouts when compared with their rural counterparts, although climatic and ecological factors are also involved in this process (Deviche and Davies, 2013; Møller et al., 2015).
Besides the difference in predation risk, there are at least two other possible drivers of these rural-urban differences in anti-predatory responses. First, it is possible that urban birds live in “heat islands” and this climatic effect, rather than variation in predation risk, drives the patterns in the life history decisions reported here. We find this possible, but not likely because of the effect sizes we identified and because of the relationship between predation and FID that we (and others) reported elsewhere (Stankowich and Blumstein, 2005; Díaz et al., 2013; Cooper and Blumstein, 2015; Samia et al., 2015c). Second, variation in flock size, which could influence risk perception, could also explain these patterns. Differences in flock size between rural and urban populations could contribute to differential awareness and risk taking by birds that results from the effects of many eyes scanning for predators (Pulliam, 1973). Individuals in larger flocks could also tolerate more human disturbance because of a reduction in per capita risk of predation (Alexander, 1974). And, if tolerance of non-lethal human disturbance is socially transmitted, one might expect the effect of social transmission to be enhanced in larger groups (Griffin, 2004). However, the rural and urban bird populations we studied in Europe did not differ in their flock sizes. Although, a recent study found that wild birds increased tolerance to humans as group size increased (Samia et al., 2015a), flock size was not important in explaining rural-urban differences in tolerance in a meta-analysis of 180 bird species distributed around the globe (Samia et al., 2015c), nor was it important in a meta-analysis of all estimates of FID in birds (Møller, 2015). Nevertheless, the similarly-sized flocks in rural and urban bird populations in our study suggests alternative explanations for rural-urban differences in these anti-predatory responses.
To understand the changes in escape strategies of rural and urban birds we need an integrative view of the patterns that arise from the main drivers of pre-detection distance and buffer distance. As latitude increases, pre-detection distances increase and buffer distances decrease in both rural and urban birds. If we assume that predation risk decreases with increasing latitude (Schemske et al., 2009; Díaz et al., 2013), this implies that as risk decreases, birds take longer to become aware of approaching humans while they take flight more rapidly following detection. This pattern suggests that birds prioritize the reduction of monitoring costs when facing lower predation pressure, which occurs at higher latitudes and in urban areas. Because attention is a limited entity (Dukas, 1998, 2004), prey that allocate attention so that they can detect predators must reduce or divert their attention from other fitness-enhancing activities, such as foraging or social activities. For this reason, ongoing monitoring is costly because of lost opportunities. In fact, a recent meta-analysis showed that factors associated with opportunity costs had the largest effect size on escape decisions in lizards (Samia et al., 2016). This raises the question whether it is reasonable to expect selection on animals to reduce monitoring costs in areas with reduced predation risk. Future studies that report a negative relationship between latitude (or other environmental correlates of predation risk) and vigilance behavior in birds would be consistent with the causal hypothesis of reduction of attentional costs.
While larger pre-detection distances at higher latitudes is consistent with the reduced predation risk hypothesis, the more immediate escape of both rural and urban birds at higher latitudes might sound counterintuitive. However, this observation can be explained by the spatial constraints associated with escape. Escape variables are constrained so that SD ≥ AD ≥ FID, with FID always being > 0 for prey which flee an approaching threat at any given moment (Cooper et al., 2015). The larger a prey's pre-detection distance, the closer is AD to the minimal safe distance (Blumstein, 2003; Cooper and Blumstein, 2015). In economic escape theory, the minimal safe distance (or Zone I sensu Blumstein, 2003) represents a distance at which prey flee immediately because the risk of being depredated is maximal given its very close distance to the predator. Thus, it is not expected that prey tradeoff risks against costs, but rather flee immediately (Blumstein, 2003; Cooper and Blumstein, 2015). Therefore, because birds seemingly increase their pre-detection distance to avoid ongoing monitoring costs at high latitudes, their AD shifts closer to their minimal safe distance, resulting in even more immediate escape at higher latitudes. Although the existence of a minimal safe distance has empirical support (e.g., Cooper, 2005, 2008), future studies identifying the factors that determine it, and whether the minimal safe distance changes over time and context, will help us to understand the processes leading to the success of urban populations.
This causal hypothesis of the relationship between buffer distance and latitude is supported by two other findings. First, the observation of different relationships between AD and buffer distance that varied across sites. In rural areas, with larger average AD, which were further from bird's minimal safe distances, there was no relationship between mean AD and buffer distance. By contrast, this relationship was negative in urban areas where mean AD was shorter and thus closer to the bird's minimal safe distances. Second, the observation of the inverse relationship of pre-detection distance and buffer distance that varied with latitude. This relationship suggests that the minimal safe distance is a potential threshold for escape in birds. The rural-urban difference in buffer distance is larger in Southern Europe, the region where the difference in pre-detection distance is smaller. Conversely, the rural-urban difference in buffer distance is smaller in Northern Europe, where the difference in pre-detection distance is larger.
Although the observed latitudinal variation in behavior is consistent with the predation risk hypothesis (when not distinguishing rural from urban populations), the observed “rural-urban difference” in these behaviors with latitude is challenging to explain, particularly since some expect patterns were not observed (see Table 1).
Contrary to our expectation, rural-urban differences in pre-detection distance increased at higher latitudes, with urban birds taking even longer to be alerted of approaching humans in higher latitudes. There are at least two hypotheses that may explain these patterns. First, in Northern Europe, rural villages are small and birds may effectively avoid people because they have a reduced tolerance of people (e.g., because they are less exposed, they are less likely to habituate to humans) and because of a weaker human shield effect in these areas (Berger, 2007; Ibáñez-Álamo et al., 2012; Møller, 2012). Thus, the difference between urban centers and rural areas may be exacerbated given the size of rural villages. Second, the differences between Northern and Southern Europe could result by their different types of predator communities. Predator assemblages in Northern towns are less diverse and abundant than assemblages in the Southern towns. For example, no stray cats and red foxes live in Northern European towns (e.g., Jokimäki et al., 2005), despite both of them being common in Southern European towns. In addition, many raptor species (e.g., Falco peregrinus and F. tinnunculus) are urbanized in the South but have not become urbanized in the North. These differences are partly related to much longer history of urbanization in Southern than in Northern Europe. Thus, urban birds in the North may live in even safer environments and this could enhance the pre-detection differences between rural and urban areas. However, it is also possible that both human presence/disturbance (Carrete and Tella, 2010) as well as differences in the predator assemblages might cause differences in avian anti-predatory behavior.
Brain size was not a main driver of rural-urban differences in escape decisions in this set of European birds. Brain size was a main driver of escape decisions of North American and Australian birds when interspecific differences were investigated (Samia et al., 2015a). At the species level, differences in brain size are often associated with variation in cognitive abilities and/or behavioral flexibility (Sol et al., 2005, 2013; Maklakov et al., 2011). However, as we found, a species' brain size may not have a substantial effect on the rural-urban changes in avian escape decisions within species. Despite that, the effect of brain size on rural-urban difference in escape decisions of European birds might differ from that in North American and Australian birds due to a longer history of close interactions between birds and humans in the Old World, dating back more than 30,000 years. For example, Møller et al. (2014) showed that the relationship between FID and population trends differed between Europe and Australia on one hand and North America on the other. Whether this difference is based on cognitive, and hence brain size, differences among continents still remains to be determined. Future studies that explicitly analyze the relationship between rural-urban differences in escape decisions and brain size on continents that vary in their history of exposure to human activity are warranted. Such differences in behavior may have consequences for life history, which are already known to differ between the Old and the New World with reduced nest predation and increased fecundity and iteroparity in the Old World (Martin and Clobert, 1996; Ghalambor and Martin, 2001). The present study indicates that effects of urbanization, acting through selection on life history decisions, may be more profound than previously realized.
We conclude by noting that, while the study of FID has played a crucial role in the development of risk assessment theory and has been widely used to study the effects of urbanization, we have shown that other components of escape behavior can respond differently (both to urbanization and latitude) and provide a complementary perspective to the study of antipredator responses. This new approach is essential if we are to understand, predict and manage wildlife in a rapidly urbanizing world.
Ethics Statement
Data were collected on public and private land after acquiring any required permits. By design, experimental approaches were designed to create only a brief disturbance and we are not aware of any lasting harm caused by the experimental approaches. In addition, and to reduce the likelihood of any negative effects, endangered species were not targeted, and we only targeted birds away from their nests. In fact, the disturbance produced to birds by our methodology did not differ from standard “background” disturbance caused by any persons walking outdoors.
Authors Contributions
Conceived the study: DS and AM. Collected data: All authors, except DS and DTB. Analyzed data: DS. Discussed the results: All authors. Wrote the first draft of the manuscript: DS, DTB, and AM. All authors edited the manuscript.
Funding
DS was supported by Fundação de Amparo à Pesquisa do Estado de São Paulo (FAPESP 2015/06734-1). DTB was supported by NSF. TG was supported by the Human Frontier Science Program award (RGY83/2012). JI was funded by a postdoctoral contract (TAHUB-104) from the program “Andalucía Talent Hub” (co-funded by the European's Union Seventh Framework Program Marie Skłodowska-Curie actions—COFUND—and the regional Government of Andalucía). KT was supported by the Estonian Research Council (institutional research funding IUT number 34-8). GM was supported by National Research, Development, and Innovation Office (NKFIH, K-115970).
Conflict of Interest Statement
The authors declare that the research was conducted in the absence of any commercial or financial relationships that could be construed as a potential conflict of interest.
Acknowledgments
We thank Tomás Pérez-Contreras for his help during the field work and R. Viitanen for designing the Figure 1A.
Supplementary Material
The Supplementary Material for this article can be found online at: http://journal.frontiersin.org/article/10.3389/fevo.2017.00066/full#supplementary-material
References
Alexander, R. D. (1974). The evolution of social behavior. Annu. Rev. Ecol. Syst. 5, 325–383. doi: 10.1146/annurev.es.05.110174.001545
Anderies, J. M., Katti, M., and Shochat, E. (2007). Living in the city: resource availability, predation, and bird population dynamics in urban areas. J. Theor. Biol. 247, 36–49. doi: 10.1016/j.jtbi.2007.01.030
Berger, J. (2007). Fear, human shields and the redistribution of prey and predators in protected areas. Biol. Lett. 3, 620–623. doi: 10.1098/rsbl.2007.0415
Blumstein, D. T. (2003). Flight initiation distance in birds is dependent on intruder starting distance. J. Wildl. Manage. 67, 852–857. doi: 10.2307/3802692
Blumstein, D. T. (2006). Developing an evolutionary ecology of fear: how life history and natural history traits affect disturbance tolerance in birds. Anim. Behav. 71, 389–399. doi: 10.1016/j.anbehav.2005.05.010
Blumstein, D. T. (2016). Habituation and sensitization: new thoughts about old ideas. Anim. Behav. 120, 255–262. doi: 10.1016/j.anbehav.2016.05.012
Brown, L. M., and Graham, C. H. (2015). Demography, traits and vulnerability to urbanization: can we make generalizations? J. Appl. Ecol. 52, 1455–1464. doi: 10.1111/1365-2664.12521
Carrete, M., and Tella, J. L. (2010). Individual consistency in flight initiation distances in burrowing owls: a new hypothesis on disturbance-induced habitat selection. Biol. Lett. 6, 167–170. doi: 10.1098/rsbl.2009.0739
Carrete, M., and Tella, J. L. (2011). Inter-individual variability in fear of humans and relative brain size of the species are related to contemporary urban invasion in birds. PLoS ONE 6:e18859. doi: 10.1371/journal.pone.0018859
Carrete, M., and Tella, J. L. (2013). High individual consistency in fear of humans throughout the adult lifespan of rural and urban burrowing owls. Sci. Rep. 3:3524. doi: 10.1038/srep03524
Carrete, M., Martínez-Padilla, J., Rodríguez-Martínez, S., Rebolo-Ifrán, N., Palma, A., and Tella, J. L. (2016). Heritability of fear of humans in urban and rural populations of a bird species. Sci. Rep. 6, 1–6. doi: 10.1038/srep31060
Cohen, J. (1997). Statistical Power Analysis for the Behavioral Sciences. New York, NY: Academic Press.
Cooper, W. E. Jr., and Blumstein, D. T. (2014). Novel effects of monitoring predators on costs of fleeing and not fleeing explain flushing early in economic escape theory. Behav. Ecol. 25, 44–52. doi: 10.1093/beheco/art083
Cooper, W. E. Jr., and Blumstein, D. T. (eds.). (2015). Escaping from Predators: An Integrative View of Escape Decisions. New York, NY: Cambridge University Press. doi: 10.1017/CBO9781107447189
Cooper, W. E. Jr., and Frederick, W. G. (2007). Optimal flight initiation distance. J. Theor. Biol. 244, 59–67. doi: 10.1016/j.jtbi.2006.07.011
Cooper, W. E. Jr., Samia, D. S. M., and Blumstein, D. T. (2015). FEAR, spontaneity, and artifact in economic escape theory: a review and prospectus. Adv. Stud. Behav. 47, 147–159. doi: 10.1016/bs.asb.2015.02.002
Cooper, W. E. Jr. (2005). When and how do predator starting distances affect flight initiation distances? Can. J. Zool. 83, 1045–1050. doi: 10.1139/z05-104
Cooper, W. E. Jr. (2008). Strong artifactual effect of starting distance on flight initiation distance in the actively foraging lizard Aspidoscelis exsanguis. Herpetologica 64, 200–206. doi: 10.1655/07-081.1
Cramp, S., and Perrins, C. M. (1977–1994). The Birds of the Western Palearctic. Oxford: Oxford University press.
Deviche, P., and Davies, S. (2013). “Reproductive phenology of urban birds: environmental cues and mechanisms,” in Avian Urban Ecology: Behavioural and Physiological Adaptations, eds D. Gil and H. Brumm (Oxford: Oxford University Press), 98–115. doi: 10.1093/acprof:osobl/9780199661572.003.0008
Díaz, M., Møller, A. P., Flensted-Jensen, E., Grim, T., Ibáñez-Álamo, J. D., Jokimäki, J., et al. (2013). The geography of fear: a latitudinal gradient in anti-predator escape distances of birds across Europe. PLoS ONE 8:e64634. doi: 10.1371/journal.pone.0064634
Dill, L. M., and Ydenberg, R. C. (1987). The group size - flight distance relationship in water striders (Gerris remigis). Can. J. Zool. 65, 223–226. doi: 10.1139/z87-036
Dukas, R. (1998). Cognitive Ecology: The Evolutionary Ecology of Information Processing and Decision Making. Chicago, IL: University of Chicago Press.
Dukas, R. (2004). Causes and consequences of limited attention. Brain Behav. Evol. 63, 197–210. doi: 10.1159/000076781
Engelhardt, S. C., and Weladji, R. B. (2011). Effects of levels of human exposure on flight initiation distance and distance to refuge in foraging eastern gray squirrels (Sciurus carolinensis). Can. J. Zool. 89, 823–830. doi: 10.1139/z11-054
Foley, J. A., DeFries, R., Asner, G. P., Barford, C., Bonan, G., Carpenter, S. R., et al. (2005). Global consequences of land use. Science 309, 570–574. doi: 10.1126/science.1111772
Freckleton, R. P. (2002). On the misuse of residuals in ecology: regression of residuals vs. multiple regression. J. Anim. Ecol. 71, 542–545. doi: 10.1046/j.1365-2656.2002.00618.x
Frid, A., and Dill, L. (2002). Human-caused disturbance stimuli as a form of predation risk. Conserv. Ecol. 6, 1–11. doi: 10.5751/es-00404-060111
Garamszegi, L. Z. (eds.). (2014). Modern Phylogenetic Comparative Methods and Their Application in Evolutionary Biology: Concepts and Practice. New York, NY: Springer. doi: 10.1007/978-3-662-43550-2
Garamszegi, L. Z., and Møller, A. P. (2011). Nonrandom variation in within-species sample size and missing data in phylogenetic comparative studies. Syst. Biol. 60, 876–880. doi: 10.1093/sysbio/syr060
Ghalambor, C. K., and Martin, T. E. (2001). Fecundity-survival trade-offs and parental risk-taking in birds. Science 292, 494–497. doi: 10.1126/science.1059379
Griffin, A. S. (2004). Social learning about predators: a review and prospectus. Learn. Behav. 32, 131–140. doi: 10.3758/BF03196014
Grimm, N. B., Foster, D., Groffman, P., Grove, J. M., Hopkinson, C. S., Nadelhoffer, K. J., et al. (2008). The changing landscape: ecosystem responses to urbanization and pollution across climatic and societal gradients. Front. Ecol. Environ. 6, 264–272. doi: 10.1890/070147
Hamilton, W. D. (1971). Geometry for the selfish herd. J. Theor. Biol. 31, 295–311. doi: 10.1016/0022-5193(71)90189-5
Ibáñez-Álamo, J. D., Sanllorente, O., and Soler, M. (2012). The impact of researcher disturbance on nest predation rates: a meta-analysis. Ibis 154, 5–14. doi: 10.1111/j.1474-919X.2011.01186.x
Jetz, W., Thomas, G. H., Joy, J. B., Hartmann, K., and Mooers, A. O. (2012). The global diversity of birds in space and time. Nature 491, 444–448. doi: 10.1038/nature11631
Jokimäki, J., Kaisanlahti-Jokimäki, M. L., Sorace, A., Fernández-Juricic, E., Rodriguez-Prieto, I., and Jimenez, M. D. (2005). Evaluation of the “safe nesting zone” hypothesis across an urban gradient: a multi-scale study. Ecography 28, 59–70. doi: 10.1111/j.0906-7590.2005.04001.x
Laurila, A., Lindgren, B., and Laugen, A. T. (2008). Antipredator defenses along a latitudinal gradient in Rana temporaria. Ecology 89, 1399–1413. doi: 10.1890/07-1521.1
Levey, D. J., Londoño, G. A., Ungvari-Martin, J., Hiersoux, M. R., Jankowski, J. E., Poulsen, J. R., et al. (2009). Urban mockingbirds quickly learn to identify individual humans. Proc. Natl. Acad. Sci. U.S.A. 106, 8959–8962. doi: 10.1073/pnas.0811422106
Maklakov, A. A., Immler, S., Gonzalez-Voyer, A., Rönn, J., and Kolm, N. (2011). Brains and the city: big-brained passerine birds succeed in urban environments. Biol. Lett. 7, 730–732. doi: 10.1098/rsbl.2011.0341
Martin, T. E., and Clobert, J. (1996). Nest predation and avian life-history evolution in Europe versus North America: a possible role of humans? Am. Nat. 147, 1028. doi: 10.1086/285891
Marzluff, J., Bowman, R., and Donnelly, R. (2001). “A historical perspective on urban bird research: trend, terms, and approaches,” in Avian Ecology and Conservation in an Urbanizing World, eds M. Jm, R. Bowman, and R. Donnelly (New York, NY: Kluwer Academic Publisher), 20–47. doi: 10.1007/978-1-4615-1531-9
McDonald, R. I. (2008). Global urbanization: can ecologists identify a sustainable way forward? Front. Ecol. Environ. 6, 99–104. doi: 10.1890/070038
McGiffin, A., Lill, A., Beckman, J., and Johnstone, C. P. (2013). Tolerance of human approaches by Common Mynas along an urban-rural gradient. Emu 113, 154–160. doi: 10.1071/MU12107
Møller, A. P. (2009). Successful city dwellers: a comparative study of the ecological characteristics of urban birds in the Western Palearctic. Oecologia 159, 849–858. doi: 10.1007/s00442-008-1259-8
Møller, A. P. (2012). Urban areas as refuges from predators and flight distance of prey. Behav. Ecol. 23, 1030–1035. doi: 10.1093/beheco/ars067
Møller, A. P. (2015). “Birds,” in Escaping from Predators: An Integrative View of Escape Decisions, eds W. E. Cooper Jr. and D. T. Blumstein (New York, NY: Cambridge University Press), 88–112.
Møller, A. P., and Erritzøe, J. (2014). Predator-prey interactions, flight initiation distance and brain size. J. Evol. Biol. 27, 34–42. doi: 10.1111/jeb.12272
Møller, A. P., and Ibáñez-Álamo, J. D. (2012). Escape behaviour of birds provides evidence of predation being involved in urbanization. Anim. Behav. 84, 341–348. doi: 10.1016/j.anbehav.2012.04.030
Møller, A. P., Díaz, M., Grim, T., Dvorská, A., Flensted-Jensen, E., Ibáñez-Álamo, J. D., et al. (2015). Effects of urbanization on bird phenology: a continental study of paired urban and rural populations. Clim. Res. 66, 185–199. doi: 10.3354/cr01344
Møller, A. P., Samia, D. S. M., Weston, M. A., Guay, P. J., and Blumstein, D. T. (2016). Flight initiation distances in relation to sexual dichromatism and body size in birds from three continents. Biol. J. Linn. Soc. 117, 823–831. doi: 10.1111/bij.12706
Møller, A. P., Samia, D. S. M., Weston, M. A., Guay, P.-J., and Blumstein, D. T. (2014). American exceptionalism: population trends and flight initiation distances in birds from three continents. PLoS ONE 9:e107883. doi: 10.1371/journal.pone.0107883
Nakagawa, S., and Cuthill, I. C. (2007). Effect size, confidence interval and statistical significance: a practical guide for biologists. Biol. Rev. Camb. Philos. Soc. 82, 591–605. doi: 10.1111/j.1469-185X.2007.00027.x
Pulliam, H. (1973). On the advantages of flocking. J. Theor. Biol. 38, 419–422. doi: 10.1016/0022-5193(73)90184-7
R Core Team (2014). R: A Language and Environment for Statistical Computing. Vienna: R Foundation for Statistical Computing. Available online at: http://www.R-project.org/
Reimers, E., Miller, F. L., Eftestøl, S., Colman, J. E., and Dahle, B. (2006). Flight by feral reindeer Rangifer tarandus tarandus in response to a directly approaching human on foot or on skis. Wildlife Biol. 12, 403–413. doi: 10.2981/0909-6396(2006)12[403:FBFRRT]2.0.CO;2
Runyan, A. M., and Blumstein, D. T. (2004). Do individual differences influence flight initiation distance? J. Wildl. Manage. 68, 1124–1129. doi: 10.2193/0022-541X(2004)068[1124:DIDIFI]2.0.CO;2
Samia, D. S. M., and Blumstein, D. T. (2014). Phi index: a new metric to test the flush early and avoid the rush hypothesis. PLoS ONE 9:e113134. doi: 10.1371/journal.pone.0113134
Samia, D. S. M., Blumstein, D. T., Stankowich, T., and Cooper, W. E. Jr. (2016). Fifty years of chasing lizards: new insights advance optimal escape theory. Biol. Rev. 91, 349–366. doi: 10.1111/brv.12173
Samia, D. S. M., Møller, A. P., and Blumstein, D. T. (2015a). Brain size as a driver of avian escape strategy. Sci. Rep. 5:11913. doi: 10.1038/srep11913
Samia, D. S. M., Møller, A. P., Blumstein, D. T., Stankowich, T., and Cooper, W. E. Jr. (2015b). Sex differences in lizard escape decisions vary with latitude, but not sexual dimorphism. Proc. R. Soc. B 282, 20150050. doi: 10.1098/rspb.2015.0050
Samia, D. S. M., Nakagawa, S., Nomura, F., Rangel, T. F., and Blumstein, D. T. (2015c). Increased tolerance to humans among disturbed wildlife. Nat. Commun. 6, 1–8. doi: 10.1038/ncomms9877
Samia, D. S. M., Nomura, F., and Blumstein, D. T. (2013). Do animals generally flush early and avoid the rush? A meta-analysis. Biol. Lett. 9:20130016. doi: 10.1098/rsbl.2013.0016
Schemske, D. W., Mittelbach, G. G., Cornell, H. V., Sobel, J. M., and Roy, K. (2009). Is there a latitudinal gradient in the importance of biotic interactions? Annu. Rev. Ecol. Evol. Syst. 40, 245–269. doi: 10.1146/annurev.ecolsys.39.110707.173430
Sol, D., Duncan, R. P., Blackburn, T. M., Cassey, P., and Lefebvre, L. (2005). Big brains, enhanced cognition, and response of birds to novel environments. Proc. Natl. Acad. Sci. U.S.A. 102, 5460–5465. doi: 10.1073/pnas.0408145102
Sol, D., Lapiedra, O., and González-Lagos, C. (2013). Behavioural adjustments for a life in the city. Anim. Behav. 85, 1101–1112. doi: 10.1016/j.anbehav.2013.01.023
Stankowich, T., and Blumstein, D. T. (2005). Fear in animals: a meta-analysis and review of risk assessment. Proc. R. Soc. B 272, 2627–2634. doi: 10.1098/rspb.2005.3251
United Nations (2014). World Urbanization Prospects, the 2014 Revision. New York, NY: Department of Economic and Social Affairs, Population Division.
Viechtbauer, W. (2010). Conducting meta-analyses in R with the metafor package. J. Stat. Softw. 36, 1–48. doi: 10.18637/jss.v036.i03
Keywords: alert distance, antipredator behavior, buffer distance, flight initiation distance, phi index, pre-detection distance, rural-urban difference, urbanization
Citation: Samia DSM, Blumstein DT, Díaz M, Grim T, Ibáñez-Álamo JD, Jokimäki J, Tätte K, Markó G, Tryjanowski P and Møller AP (2017) Rural-Urban Differences in Escape Behavior of European Birds across a Latitudinal Gradient. Front. Ecol. Evol. 5:66. doi: 10.3389/fevo.2017.00066
Received: 17 November 2016; Accepted: 02 June 2017;
Published: 26 June 2017.
Edited by:
Caroline Isaksson, Lund University, SwedenReviewed by:
Ximena J. Nelson, University of Canterbury, New ZealandMichael Reichert, University College Cork, Ireland
Copyright © 2017 Samia, Blumstein, Díaz, Grim, Ibáñez-Álamo, Jokimäki, Tätte, Markó, Tryjanowski and Møller. This is an open-access article distributed under the terms of the Creative Commons Attribution License (CC BY). The use, distribution or reproduction in other forums is permitted, provided the original author(s) or licensor are credited and that the original publication in this journal is cited, in accordance with accepted academic practice. No use, distribution or reproduction is permitted which does not comply with these terms.
*Correspondence: Diogo S. M. Samia, ZGlvZ29zYW1pYUBnbWFpbC5jb20=