- Department of Ecology and Evolutionary Biology, University of Arizona, Tucson, AZ, United States
A crucial step in adapting to urban habitat is switching to novel, often man-made, resources. Switching to a novel resource can influence the dynamics of species coexistence, particularly if it alters trade-offs in performance. While such switches are frequently documented, their influence on species coexistence has been difficult to assess because it requires knowledge of performance trade-offs in the context of both historical and novel resource use. Western and mountain bluebirds provide a unique system in which to investigate the effects of a resource switch on species coexistence because both depend on secondary nest cavities to breed and, across a large part of their range, have switched to using man-made nest boxes. Western bluebirds are less dispersive, but more aggressive, than mountain bluebirds leading to a successional pattern of species replacement in many nest box populations; however, there is evidence of continued coexistence in natural post-fire habitat. Nest boxes differ from natural cavities in both distribution, which may affect the dynamics of interference competition between the species, and thermal conductance, which may impact competition by altering survival of ectothermic young. Here, we use a combination of experimental manipulations of nest box density and more than a decade of fitness and incubation temperature data to investigate whether altered resource distribution or thermal environment best explain patterns of species replacement in nest box populations. In both species, we found that females breeding in nest boxes were unable to maintain normal incubation temperatures during inclement weather and experienced similar offspring mortality patterns. Moreover, climatic variation across populations did not predict species' relative abundance. Instead, experimental manipulation of nest box density showed that mountain bluebirds persisted longer when nest boxes were distributed farther apart, suggesting that nest box distribution may be a key factor in understanding how human-created habitat impacts coexistence of bluebird species. These results emphasize that knowledge of species interactions in the historical habitat is crucial to understanding population dynamics as species transition to novel, man-made habitat.
Introduction
Urbanization has eliminated habitat and resources that many species require, leading to their disappearance from areas where they were once common (Marzluff, 2001; Shochat et al., 2006). Although many species are lost as a consequence of urbanization, changes in habitat structure and resource availability often provide a boon to a small subset of species making community composition in urban areas distinct from more natural environments (Devictor et al., 2007; Chamberlain et al., 2009). Yet, the processes underlying shifts in community composition are poorly understood (Shochat et al., 2010). In particular, it is unclear why some species excel in human-altered landscapes and other species rapidly decline.
One explanation for such differential success is that the changes in resource quality and availability in urban and agricultural areas may affect species' relative competitive ability. For example, superior interference competitors, which dominate in direct interactions for scarce resources (Schoener, 1983), may be able to monopolize resources more efficiently in resource-rich habitat patches and this can alter competitive dominance across patches that vary in resource density (Tessier and Woodruff, 2002; Palmer, 2003). Interference ability may also interact with variation in resource quality to influence patterns of coexistence (Amarasekare, 2002). Strong interference competitors can monopolize the highest quality resources, but may ignore lower quality resources, providing the opportunity for a subdominant competitor to coexist locally (Alatalo and Moreno, 1987). Moreover, interference ability often comes at the expense of investment in other traits such as dispersal, abiotic tolerances, reproductive investment or efficiency of resource use (Tilman, 1994; Hughes et al., 2003; Pfennig and Pfennig, 2005; Cadotte et al., 2006). Such trade-offs may allow species that are poor interference competitors to persist because the costs of strong interference ability may not be supported on low quality resources. Thus, differences in both the distribution and quality of resources in man-made vs. natural habitat can alter species composition in novel environments (Chesson, 2000; Amarasekare and Nisbet, 2001; Kneitel and Chase, 2004).
While a switch to a novel resource is frequently documented in species using human-altered habitat (Lancaster and Rees, 1979; Blair, 1996; Robb et al., 2008), its influence on species coexistence has been difficult to assess because it requires a detailed knowledge of performance trade-offs, how they may change in a novel environment and species' relative interference abilities. Cavity nesting birds provide a unique opportunity to examine how such a switch influences species coexistence. Urban development, such as the clearing of a forest, typically eliminates natural nesting holes on which many cavity nesting species depend and as a consequence can cause rapid population declines in cavity nesting species (Newton, 1994). Such declines have led to widespread implementation of nest box programs where people provide man-made nesting cavities in urban parks and agricultural areas (Semel and Sherman, 1995; Rodríguez et al., 2011; Brazill-Boast et al., 2013). These programs have been quite successful and nest boxes have largely replaced natural cavities as the main breeding resource for cavity nesting birds in many agricultural and highly populated areas (Griffith et al., 2008; Davies et al., 2009). However, man-made nest boxes differ from natural cavities in their distribution and quality in ways that can influence species' relative competitive ability (Duckworth, 2014).
In this study, we investigate mechanisms underlying variable patterns of species coexistence in two species of Sialia bluebirds. Mountain and western bluebirds (S. currucoides and S. mexicana) compete for nest cavities and hold interspecific territories in areas where their ranges overlap in the Northwestern United States. Before the widespread placement of nest boxes, new bluebird habitat in this region was largely created by forest fire, which generates suitable habitat by opening up understory vegetation and creating dead snags. Primary cavity nesters, such as woodpeckers, excavate cavities in these snags that are eventually used by secondary cavity nesters. This habitat lasts for up to 30 years until regrowth of the forest eliminates the open meadows bluebirds depend on to forage for insect prey (Power and Lombardo, 1996; Guinan et al., 2000). The successional nature of post-fire habitat means that historically, the persistence of bluebirds depended on their ability to continually recolonize new habitat patches (Schieck and Song, 2006). Mountain bluebirds are more dispersive than western bluebirds and are among the earliest colonizers following forest fires (Hutto, 1995; Schieck and Song, 2006), whereas western bluebirds often show delayed patterns of colonization (Kotliar et al., 2007; Saab et al., 2007; Hutto and Patterson, 2016), yet are better interference competitors (Duckworth and Badyaev, 2007; Duckworth et al., 2015).
The two species show similar patterns of colonization in both natural and nest box populations with mountain bluebirds arriving first and western bluebirds arriving later (Saab et al., 2007; Duckworth, 2014), however, the ability of these species to coexist differs among populations. Studies of nest box populations of bluebirds have documented several instances of the complete and rapid replacement of mountain bluebirds by western bluebirds (Duckworth and Badyaev, 2007), yet studies in post-fire habitat found that mountain bluebirds persist at high numbers for at least 10 years after western bluebirds' arrival (Saab et al., 2007) suggesting that complete species replacement may be less common in natural habitat. Moreover, rapid species replacement does not always occur in nest box populations—in some populations the two species have been co-occurring stably for over a decade (Duckworth, 2014).
Given that nest cavities are the main limiting resource for these species (Brawn and Balda, 1988; Power and Lombardo, 1996), differences in nest cavity quality and distribution between natural post-fire forest and nest box populations may play an important role in explaining variable patterns of species replacement. In the United States, many conservation organizations encourage people to set up “nest box trails” by placing nest boxes along roads in suburban and agricultural areas. Typically, these trails are in highly open parks and ranchland where a lack of natural cavities limits the number of bluebirds breeding in these areas. Therefore, people essentially create new bluebird habitat when they set up “nest box trails.” While the distribution of natural nest cavities in post-fire forest is often highly variable, nest boxes in these human-modified suburban and agricultural landscapes are often distributed evenly in a grid or linear transect (Lehmkuhl et al., 2003; Remm and Lõhmus, 2011; Duckworth, 2014). Moreover, in post-fire forests, nest cavity quality is highly variable depending on how it was created (e.g., by rot or various woodpecker species; (Robles and Martin, 2013); whereas, in human-created nest box trails, nest boxes are typically the same shape, size, and thickness. Thus, human-created bluebird habitat differs in both the distribution and extent of variation in quality of nest sites compared to natural populations.
To test the idea that variable patterns of species replacement across populations are due to changes in resource quality (Table 1), we first test experimentally whether natural cavities used by bluebirds are more insulated than nest boxes. We consider more insulated nest cavities to be of higher quality because the main source of offspring mortality in nest box populations is inclement weather during early spring cold snaps (Duckworth, 2006b). The difference in insulation properties of natural and man-made cavities might influence coexistence of mountain and western bluebirds because mountain bluebirds breed at both higher elevations and latitudes than western bluebirds (Power and Lombardo, 1996; Guinan et al., 2000), making them more likely to be adapted to cold weather conditions. If so, this might explain their ability to persist in some populations. We first test the assumption that mountain bluebirds are less impacted by cold snaps in general and then compare offspring survival of both species during cold snaps. Finally, we use elevational variation as a proxy for climatic conditions across populations and examine whether it is associated with changes in species abundances over time (see Table 1 for predictions).
Alternatively, the dynamics of species replacement may be most strongly influenced by differences in the distribution of nest boxes across populations. In an observational study, a strong dependency of species settlement patterns on the density at which nest boxes were placed suggested that mountain bluebirds were more likely to persist in habitat patches where nest box density is low compared to patches where it is high (Duckworth, 2014). Mountain bluebirds are larger and require larger territories than western bluebirds (Pinkowski, 1979; Duckworth, 2014). If nest cavity density is low, the distance between nest sites exceeds the maximum territory size of both species, which limits the importance of interference competition. However, in areas where nest cavity density is high, western bluebirds breed close to mountain bluebirds limiting their territory size to below the species optimal size, essentially “crowding them out” (Duckworth, 2008, 2014). Here, we conducted a long-term experiment manipulating local variation in nest box density to determine whether altered resource distribution explains mountain bluebirds' ability to persist in some areas (Table 1).
Methods
Data were collected from multiple nest box populations of western and mountain bluebirds in western Montana, including four sites surrounding Missoula, MT, one site near St. Regis, MT, two sites in the Blackfoot Valley and one natural post-fire site outside of Missoula (see Table 2 for site names, locations, and sampling details). Three of the nest box sites near Missoula were suburban recreation areas comprised of open space with housing developments on at least one side. The rest of the nest box sites were agricultural with nest boxes placed along roadways bordering open ranchland. Thus, there were very few natural cavities in the nest box populations. All nest boxes in this study were of similar design and size—they were cut from 2 cm thick wood with interior dimensions of 14.5 × 12 cm and a 4 cm hole diameter. Both species of bluebirds show high breeding site fidelity and although their breeding phenology is very similar, western bluebirds generally lay larger clutches than mountain bluebirds (Power and Lombardo, 1996; Guinan et al., 2000).
Comparing Insulation Properties of Natural Cavities and Nest Boxes
To determine whether the insulation properties of natural and man-made nest cavities differed, in 2015 (N = 4) and 2016 (N = 15), we used a paired design to directly compare the thermal microclimate of nest boxes and natural cavities relative to outside ambient temperature in the same location. Natural cavities were found primarily at the post-fire site but a few natural cavities found near the Blackfoot Valley sites were also included (Table 2). Briefly, we found active nests in natural cavities, determined species identity of the pair and monitored nest progress. Once the natural cavity was vacated, we placed a nest box directly below the natural cavity with the box entrance in the same orientation as that of the natural cavity. Holes of the nest boxes were covered by a wire screen to prevent their use during the experiment (Figure S1). As this experiment was conducted after nesting attempts by bluebirds, the majority of the paired comparisons were established late in the breeding season (late June and July). Old nests were placed in nest boxes to standardize for possible temperature effects of nest material between natural cavities and nest boxes. We placed a thermistor in the nest box and natural cavity, ~2 inches above the nest, and an additional thermistor outside the nest box to gather ambient temperature data. We connected all three thermistor wires to a single Hobo Stowaway multi-port datalogger (Onset Computer, Bourne, MA), which allowed for concurrent recording of temperatures for both cavity types relative to each other and ambient temperature. Thermistor readings were taken every 30 s for 7–21 days to capture the range of variation that may occur in a typical bluebird nesting attempt (~5–6 weeks from egg laying to fledge for a successful nest). We excluded days where temperature spikes indicated the presence of an animal using the natural cavity or readings where the temperature fluctuated due to thermistor failure. All Hobo datalogger temperature data were reviewed in HOBOware (Version 3.7.2, Onset Computer, Bourne, MA).
Testing for Species-Specific Effects of Cold Snaps
We monitored female incubation patterns by placing iButton (Dallas Semiconductor, Sunnyvale, CA) thermocouples directly under the eggs in a subset of nests at five sites (Table 2). These thermocouples were programmed to record temperatures every 5 min for the duration of the incubation period, allowing for detection of the presence or absence of the incubating female through the associated degree of temperature change of the incubated eggs. For both western and mountain bluebirds, we assessed variability of incubation temperatures (measured as standard deviation) for females that experienced a cold snap vs. females that did not. We defined cold snaps as periods in which the daily maximum temperature was <15°C for at least 2 days over which at least 10 mm of rain fell. These criteria were chosen as prior work has shown them to be meaningful thresholds at which nests begin to fail due to inclement weather (Duckworth, 2006b).
To assess whether inclement weather causes differential reproductive success among species, we compared reproductive success of nests that had experienced a broad range of weather conditions (across years and populations). For this analysis, we included nests initiated between 2003 and 2015. We excluded any nests that were subjected to an experimental treatment that might reasonably have impacted reproductive success (e.g., egg swap, clutch size manipulation, yolk sampling), and also required that nests had been checked with sufficient frequency to enable an approximation of nest stage. When nest stage was not precisely known, we used knowledge of a single reproductive event to approximate the others. For example, nestling age was used to estimate hatch date, hatching was assumed to occur 14 days after the onset of incubation, and incubation onset was assumed to occur on the day of clutch completion. Because females typically lay one egg each day until their clutch is complete, any observation of a nest during laying allowed us to estimate first egg date.
To measure reproductive success, we determined the percent of offspring that fledged from each nest. We used percent fledged because the species differ in average clutch size with western bluebirds typically having slightly larger clutches than mountain bluebirds (Power and Lombardo, 1996; Guinan et al., 2000) and we were interested in understanding the extent to which cold snaps affected reproductive success while controlling for baseline differences in clutch size among the species. Nestlings were assumed to have fledged if they were present in the nest during the last active nest check (typically on day 15) and the nest was empty during the next check (day 21). The nestling period is typically 21 days for these two species. Nests were considered to have experienced a cold snap if any portion of incubation or the nestling period overlapped with any portion of a defined cold snap (see above). We excluded nests that were initiated after June 10th because there were no cold snaps after that time in any of our populations.
Influence of Elevation on Rate of Species Replacement across Populations
To examine whether variable climatic conditions across populations influenced the rate at which western bluebirds replace mountain bluebirds in nest box populations, we used variation in elevation as a proxy for variable climatic conditions. Table S1 shows weather station data for our lowest and highest elevation site, showing that the highest elevation site has consistently lower temperatures across the breeding season and across all years compared to the lowest elevation site. We compared change in species abundances across five sites (Table 2) which varied in elevation and for which we had at least 5 years of nest box occupancy data on both western and mountain bluebirds. BMT and STR sites were excluded from this analysis because mountain bluebirds were largely absent from these sites during the study period.
For each site, we calculated the percent of the western bluebirds out of all bluebirds breeding there and used this to generate standardized regression coefficients from a linear regression analysis with percent western bluebird breeders as the dependent variable and year as independent variable. We then used these standardized regression coefficients as a measure of the rate of species replacement. We were unable to do a similar meaningful comparison with nest box density as only one site (UMC) differed substantially in nest box density (Table 2). Moreover, bluebirds breeding in natural cavities were rare at all sites due to the placement of nest boxes in open meadows or ranchland.
Experimental Test of Nest Box Density on Dynamics of Species Replacement
In 2008, to examine the influence of nest cavity density on patterns of species replacement, we created replicated linear transects of high and low density (Table 2; Figure S2) at two sites (PAU, OVD). High density transects had four nest boxes placed 75–100 m apart and low density transects had four nest boxes placed 150–200 m apart. These distances were chosen based on observed average territory sizes for each species (Duckworth, 2014). Adjacent transects were at least 300 m apart and high and low density transects were interspersed throughout the study sites (Figure S2). We recorded the identity of species breeding in each box for every year of the study (2008–2016), with the exception of 2010 and 2011. Because birds on the edges of transects might be subject to less crowding compared to birds breeding in middle boxes, position in each transect was recorded as exterior if a pair was breeding in the first or last box and interior if they were in either of the middle boxes. Within each year, we only used data from the first nesting attempt because interference competition for nest boxes and territories is most relevant early in the breeding season when all individuals are simultaneously acquiring territories.
Statistical Analysis
All analysis was completed in SAS® software V9.4. To determine whether insulation of natural cavities differed from nest boxes, we used paired t-tests to compare the average maximum and minimum daily temperatures recorded in each. We used linear mixed models (PROC MIXED in SAS) to determine the effect of cold snaps on variability of female incubation temperatures with standard deviation of incubation temperatures as the dependent variable, cold snap presence/absence, species identity and their interaction as fixed factors. We included population as a random factor. To determine the influence of cold snaps and species identity on nest success, we used only first nests as these are the most likely ones to overlap cold snaps. We excluded nests that failed due to predation. Because cold snaps may influence nest success differently during distinct stages of the nesting cycle, we categorized cold snaps that occurred during incubation and nestling periods separately. We used mixed models with percent of offspring fledged as the dependent variable and incubation and nestling period cold snap occurrence and species identity as fixed factors. Brood size differed between the species [F(1, 434) = 41.95, P < 0.001] and we included it as a covariate. We included population and year as random factors. To determine whether the species were affected differentially by cold snaps, we also included the interactions between nestling and incubation period cold snap occurrence and species identity. Initially, we included nest initiation date as a covariate, but as the two species did not differ in their mean nest initiation dates (t = 0.56, P = 0.58) and it was not significantly related to the percent of offspring fledged (P > 0.15), we excluded it from the model. We used a Spearman rank correlation to examine whether variable elevational variation across populations was related to the rate of species replacement (measured as standardized regression coefficients).
For analysis of the nest box density experiment, we used generalized linear mixed models (PROC GLIMMIX in SAS) fitting a binomial distribution with a logit-link function. The dependent variable was either mountain or western bluebird presence per number of boxes available (using events/trials syntax) with density treatment (high vs. low), position in transect (interior vs. exterior) and year as fixed effects. Site was included as a random effect. We analyzed mountain and western bluebird nest box occupancy separately as we expected that nest box density might affect their settlement patterns in different ways. We fitted year as a linear trend for western bluebirds and as a second order polynomial for mountain bluebirds. We excluded boxes from this analysis if they were not occupied by either species of bluebird over the 9 years of the study. Lack of occupancy of particular boxes likely reflected microhabitat preferences of the species (e.g., a few boxes adjacent to lakes or wetlands were never occupied by bluebirds) and thus were not informative about competitive dynamics and settlement patterns. In a subset of boxes, the bluebird species occupying them switched across years. We used a sign test to assess whether box occupancy was more likely to switch from mountain to western or western to mountain across years.
Results
Testing the Assumption That Natural Cavities and Nest Boxes Differ In Insulation Properties
Natural cavities were more insulated than nest boxes (Figure 1) as they had a lower average daily maximum temperature (mean maximum temperature for natural nest cavities = 25.48°C ± 1.13 SE vs. for nest boxes = 26.75°C ± 1.15; t = −2.13, P = 0.049) and a higher average daily minimum temperature compared to nest boxes (mean minimum temperature for natural nest cavities = 11.96°C ± 0.87 SE vs. for nest boxes = 8.70°C ± 0.91; t = 6.03, P < 0.0001; Figure 1). The temperature difference of nest boxes and natural cavities did not differ in relation to which species used the cavity for either maximum temperature differences (mean ± SE nest box minus natural cavity temperatures for mountain vs. western bluebirds: 5.41°C ± 1.55 vs. 3.08°C ± 1.41, t = 1.11, P = 0.28) or minimum temperature differences (−7.05°C ± 1.05 vs. −5.46°C ± 0.86, t = −1.19, P = 0.25).
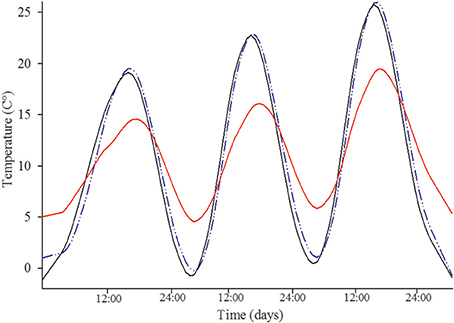
Figure 1. Example showing that natural nest cavities are more insulated than man-made nest boxes. Shown are daily fluctuations for ambient temperature (black solid line), unoccupied man-made nest box interior temperature (blue dashed line) and unoccupied natural nest cavity interior temperature (red solid line). Ambient temperature was recorded directly outside the natural nest cavity. Nest box was placed directly below the natural nest cavity.
Influence of Cold Snaps on Incubation Patterns and Nest Success
During the study period, 71% of nests experienced a cold snap during either the incubation or nestling period. Females that experienced a cold snap during incubation had greater fluctuations in incubation temperatures compared to females that did not [F(1, 149) = 11.19, P = 0.001] but the species did not differ in how variable their incubation temperatures were [F(1, 149) = 0.87, P = 0.35]. Moreover, the species did not differ in how cold snaps affected their incubation patterns as there was no significant interaction between presence of a cold snap and species identity on variability of incubation temperatures [F(1, 149) = 0.43, P = 0.51]. Population affiliation did not affect female incubation temperatures (Z = 0.97, P = 0.16).
Cold snaps had a negative impact on offspring survival, but only when they occurred during the nestling stage [F(1, 428) = 8.65, P = 0.003]. Nests that experienced a cold snap only during incubation did not differ in survival compared to nests that did not experience a cold snap during incubation [F(1, 428) = 0.80, P = 0.37]. There was no difference among the species in percent of offspring fledged for pairs that did and did not experience either incubation or nestling period cold snaps [interaction between incubation stage cold snap presence and species identity: F(1, 428) = 0.41, P = 0.52; interaction between nestling stage cold snap presence and species identity: F(1, 428) = 2.31, P = 0.13] indicating that the two species were affected by cold snaps similarly. Overall, mountain bluebirds fledged a higher percentage of offspring compared to western bluebirds [F(1, 428) = 5.10, P = 0.024] despite having lower brood size (mean ± SE brood size, mountain = 4.99 ± 0.09 vs. western = 5.06 ± 0.08). Year explained a significant amount of the variance in percent offspring fledged (Z = 1.97, P = 0.024), but population did not (Z = 1.23, P = 0.11).
Influence of Elevation on Dynamics of Species Replacement
The five nest box populations varied in the percent of western bluebirds breeding in the population over time [interaction between population and year: F(9, 30) = 8.88, P = 0.0002; Figures 2A–E]. PAU had the greatest increase of western bluebirds relative to mountain bluebirds [F(1, 5) = 28.80, P = 0.006, BST = 0.937], followed by OVD [F(1, 7) = 7.66, P = 0.04, BST = 0.778], UMC [F(1, 7) = 7.86, P = 0.031, BST = 0.753], and WWH [F(1, 4) = 0.18, P = 0.696, BST = 0.241]. At the MTJ site, the percent of breeding western bluebird pairs decreased over time [F(1, 4) = 13.53, P = 0.035, BST = −0.905]. However, elevation, our proxy for variation in climatic conditions, was not correlated to the strength of change over time in the percent of western bluebirds breeding in these populations (r = −0.14, P = 0.82; Figure 2F).
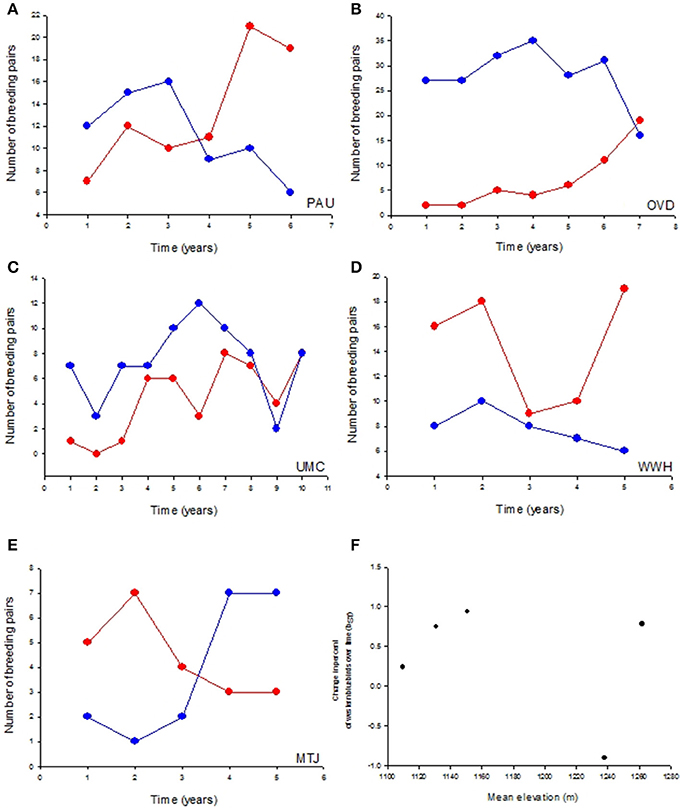
Figure 2. Dynamics of species replacement were not related to variable climatic conditions across populations. (A) The number of breeding pairs of western bluebirds (red lines) increased over time compared to mountain bluebirds (blue lines) in four nest box populations (A–D) but declined (E) in one population. (F) The relative change in western bluebird numbers over time was unrelated to population elevation, a proxy for variable climatic conditions.
Influence of Experimental Manipulation of Nest Box Density on Settlement Patterns
At sites where we carried out the nest box density experiment, western bluebirds' box occupancy strongly increased over time [F(1, 73) = 42.04, P < 0.0001], while mountain bluebird numbers were lower in the first and the last year of the study compared with all other years [F(1, 72) = 20.44, P < 0.001; Figure 3A]. Across years, there were 41 changes in box occupancy from one bluebird species to the other. Western bluebirds were more likely to replace mountain bluebirds (N = 30) than vice versa (N = 11; P = 0.004). Western bluebird nest box occupancy was not influenced by either transect density [F(1, 73) = 0.01, P = 0.925], box location within a transect [F(1, 73) = 0.15, P = 0.697] or their interaction [F(1, 73) = 1.57, P = 0.214]. There was a significant interaction between transect density and box location on mountain bluebird box occupancy [F(1, 72) = 5.47, P = 0.022] such that mountain bluebirds were more common in low density transects but only for nest boxes located in the middle of transects (Figure 3B). Moreover, after accounting for the interaction effect, there was an independent influence of nest box location within transects on mountain bluebird settlement patterns with a higher prevalence of mountain bluebirds in middle transect positions [F(1, 72) = 6.65, P = 0.012].
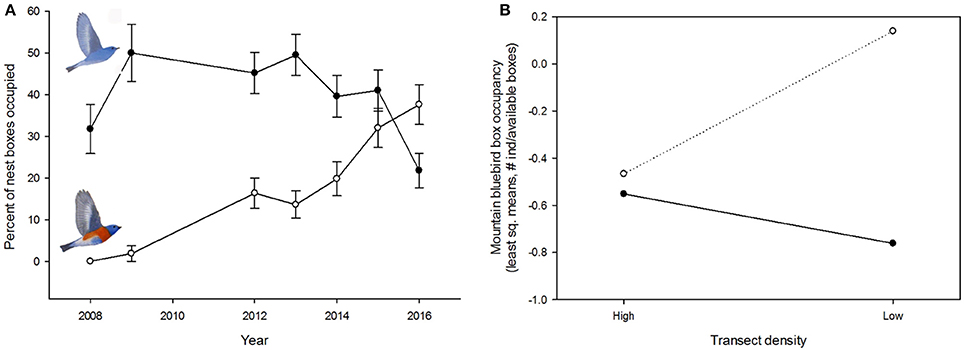
Figure 3. Colonization dynamics of mountain and western bluebirds in a nest box population where nest box density was experimentally manipulated. (A) Western bluebirds' (open circles) rapid increase in box occupancy in later years of the experiment was accompanied by a decrease in mountain bluebird (closed circles) box occupancy. (B) Mountain bluebirds were more common in low density transects but only in boxes that were in interior positions (dotted line) of transects. In high density transects, box occupancy by mountain bluebirds did not differ between interior and exterior (solid line) positions. Shown are the least square means from a generalized linear mixed model (see Section Methods for details).
Discussion
A long-standing paradox of human-modified habitats is that they are characterized by simultaneously high population densities and low biodiversity compared to more natural populations (Shochat et al., 2010). While high population densities suggest that resources are plentiful for some species in human-altered landscapes, one potential explanation for low species diversity is a lack of heterogeneity in resource distribution and quality compared to natural ecosystems (Shochat et al., 2010). Resource heterogeneity is a main mechanism for the maintenance of species diversity (Hutchinson, 1959; Tilman and Pacala, 1993; Rosenzweig, 1995) and studies of urban populations often show a link between complexity of urban habitat and avian species diversity (Lancaster and Rees, 1979; Melles et al., 2003; Devictor et al., 2007; Evans et al., 2009). Yet, the mechanisms underlying these patterns are poorly understood.
This study of the mechanisms underlying variable species replacement across populations of two cavity nesting birds breeding in human-altered environments clearly supported a role of variable resource distribution impacting coexistence dynamics, but did not support a role of variable resource quality. Specifically, we found that, in a multi-year experiment manipulating nest box density, mountain bluebirds were more common in the presence of western bluebirds when resource density was low (Figure 3B). However, the effects of nest box distribution on mountain bluebird settlement patterns cannot be fully explained by competition with western bluebirds. We had predicted that mountain bluebirds would persist longer in exterior positions of transects because they would be less likely to be crowded out by western bluebirds in these positions. However, we found the opposite pattern, suggesting that colonization dynamics are influenced by both competition among species and nest site selection preferences within each species. Second, we did not find evidence that differences in nest cavity insulation between natural and nest box populations explained variable patterns of species coexistence. In contrast to our prediction (Table 1), mountain bluebirds breeding in nest boxes were just as likely to show highly variable incubation temperatures and high offspring mortality during cold snaps as western bluebirds. Finally, the rate of change in species' abundances was not related to variation in elevation of populations.
Given that mountain bluebirds occur across a much wider elevational and latitudinal range compared to western bluebirds, our finding that they show similar offspring mortality patterns during cold snaps is surprising (Power and Lombardo, 1996; Guinan et al., 2000). One potential explanation for this finding is that the insulation properties of natural nest cavities are so superior to nest boxes that, even at high elevations, mountain bluebirds breeding in natural cavities rarely experience strong selection for high cold tolerance of eggs and young nestlings. Multiple studies (Coombs et al., 2010; Amat-Valera et al., 2014), including this study (Figure 1), have found that natural cavities are well-insulated while internal temperatures of nest boxes do not differ from ambient temperatures. Thus, it will be interesting in future studies to investigate whether selection on offspring survival due to cold snaps differs between nest box and natural post-fire populations. In fact, in contrast to nest box populations, several studies have found nest predation rates in natural cavity-nesting populations to be the dominant source of nest mortality (see Johnson and Kermott, 1994 for review), suggesting that selection pressures in natural vs. nest box populations differ. Alternatively, cold snaps may primarily influence offspring survival by making it difficult for parents to find enough insects to feed offspring, making insulation properties of nest cavities less important than availability of food resources. If this is true, it may explain the differential influence of cold snaps during the incubation and nestling periods. Cold snaps may have a stronger impact during the nestling period because of the added difficulty of keeping offspring fed in addition to keeping them warm. Moreover, we detected a difference in offspring survival when a nest experienced a cold snap, but were not able in this study to test for any differential effects of cold snap length. Therefore, it will be important in future studies to determine whether there are also more nuanced influences of cold snaps on offspring survival that vary with cold snap severity.
Interestingly, even though cold snaps did not differentially affect reproductive success of the species, mountain bluebirds overall fledged a higher percentage of offspring than western bluebirds. This differential reproductive success may reflect a trade-off between interference ability and reproductive investment (Case and Gilpin, 1974; Vance, 1984; Holway, 1999). Such a trade-off has been documented within western bluebirds where less aggressive males invest more in offspring care compared to more aggressive males (Duckworth, 2006b). It will be interesting to see whether this trade-off also holds at the interspecific level for these species.
The joint influence of nest box density and location within a transect suggests that interference competition and microhabitat preferences may interact to determine mountain bluebird settlement patterns. If mountain bluebirds prefer territories with multiple nest cavities, as has been shown in both western and eastern bluebirds (S. sialis; Plissner and Gowaty, 1995; Duckworth, 2006a), this may explain their propensity to select interior transect positions. Interior positions have the potential to provide access to three nest cavities, assuming a bird's territory is large enough to encompass neighboring nest boxes. Alternatively, mountain bluebirds' overrepresentation in interior transect positions may reflect the dominant western bluebirds' preference for exterior transect positions. The earliest arriving western bluebirds benefit from acquiring large territories in recently colonized populations (Duckworth, 2008). By choosing nest boxes at the edges of transects, western bluebirds are able to expand their territory boundary well beyond the average territory size before encountering a neighbor. However, if this latter explanation were correct, we would expect western bluebirds to be overrepresented in these positions, but we did not find any effect of either transect density or nest box position on western bluebird settlement patterns. Thus, given these patterns, we suggest that mountain bluebirds' settlement patterns reflect a combination of their own microhabitat preferences and western bluebirds' ability to crowd them out of areas where resource density is high.
In three out of the five nest box populations, western bluebird population density increased linearly over time and was accompanied by a concomitant decrease in mountain bluebird numbers (Figure 2). The nest box density experiment provided strong evidence for a causal link between increases in western bluebird numbers and the decline of mountain bluebirds. In cases where species occupancy of a nest box changed across consecutive years, western bluebirds were three times more likely to replace a mountain bluebird than vice versa. However, despite evidence for the importance of variable resource density, it cannot be the sole explanation for the patterns that we observe across populations. In two of our nest box populations, mountain bluebirds either remained steady or increased over time despite similar densities of nest boxes across sites (Table 2), patterns that are similar to what has been observed in natural post-fire habitat (Saab et al., 2007).
What other factors might influence species coexistence in this system to produce such variable patterns of species replacement in both nest box and post-fire populations? In post-fire habitat, there is immense variation in nest cavities along multiple axes of quality, including cavity height, depth, and hole size (Robles and Martin, 2013). Among secondary cavity nesters, dominant species often occupy the highest quality cavities pushing subdominant species into low quality cavities (Martin et al., 2004). Thus, it is possible that, in post-fire habitat, western bluebirds occupy the highest quality nest cavities and mountain bluebirds' ability to persist is enabled by their occupancy of lower quality nest cavities that western bluebirds ignore. Even though nest boxes are poorer in quality in terms of their insulation properties, they may be perceived by bluebirds as high quality given their small hole size, an attribute that is important protection against nest predators (Newton, 1994).
These findings have important implications for our understanding of patterns of species coexistence in both natural and human-influenced environments. It has been suggested that lower species diversity in human-modified environments is due to a combination of low predation pressure and a stable and abundant resource base (Anderies et al., 2007). Our results suggest that resource distribution and heterogeneity may be additional important factors influencing patterns of diversity in wildland vs. human-modified habitats. Moreover, we suggest that, given our ability to manipulate a key resource, comparisons of cavity-nesting birds in natural vs. man-made habitat have the potential to provide particularly novel insight into the mechanisms by which resource heterogeneity can influence patterns of species diversity.
Ethics Statement
This study was carried out in accordance with the recommendations and guidelines of the University of Arizona IACUC committee. The protocol was approved by the University of Arizona IACUC committee.
Author Contributions
RD conceived of and designed the study, wrote the manuscript, analyzed, and collected the data. AP designed the nest box microclimate experiment, wrote the manuscript, analyzed, and collected the data. KH wrote the manuscript and analyzed data. NH analyzed and extracted data on incubation patterns and offspring survival.
Conflict of Interest Statement
The authors declare that the research was conducted in the absence of any commercial or financial relationships that could be construed as a potential conflict of interest.
Acknowledgments
We thank Erin Morrison, Katie Chenard, Georgy Semenov, and Alex Badyaev for discussion and comments which improved this manuscript. We are also grateful to members of Mountain Bluebird Trails for allowing us to work on their nest box trails and Jay Kolbe, Paws Up staff and landowners in the Blackfoot Valley for permission to set up experimental transects on both the Blackfoot-Clearwater Game Range and private property. We thank the National Forest Service for permission to conduct part of this work on Forest Service property. This work was supported by US National Science Foundation grants (DEB-0918095 and DEB-1350107) to RD and grant from the American Museum of Natural History and Rosemary Grant, and the US National Science Foundation Graduate Research Fellowship Program (NSF DGE-1143953) to AP.
Supplementary Material
The Supplementary Material for this article can be found online at: http://journal.frontiersin.org/article/10.3389/fevo.2017.00072/full#supplementary-material
Figure S1. An example of the paired nest box/natural nest cavity temperature measurement experiment. This particular set-up was located at the BKMT post-fire natural habitat site. The nest box hole is covered to prevent its use by cavity nesting species.
Figure S2. Aerial photo showing example of two experimental nest box trails at the OVD site with high (red lines) and low (blue lines) density transects. White circles in the two bottommost transects indicate locations of nest boxes which are 75–100 m apart in the high density treatment and 150–200 m apart in the low density treatment. Adjacent transects were separated by at least 300 m. High and low transects were interspersed evenly in a linear fashion.
Table S1. Temperature and rainfall data from weather stations in Missoula and Blackfoot Valley during the study period. The Missoula weather station is located closest to WWH, the lowest elevation site and the Blackfoot Valley station is located closest to OVD, the highest elevation site. In all time periods, at the lower elevation site, the average daily maximum temperature was higher and the average daily minimum temperature was lower than at the higher elevation site.
References
Alatalo, R., and Moreno, J. (1987). Body size, interspecific interactions, and use of foraging sites in tits (Paridae). Ecology 68, 1773–1777. doi: 10.2307/1939868
Amarasekare, P. (2002). Interference competition and species coexistence. Proc. R. Soc. Lond. B 269, 2541–2550. doi: 10.1098/rspb.2002.2181
Amarasekare, P., and Nisbet, R. M. (2001). Spatial heterogeneity, source-sink dynamics, and the local coexistence of competing species. Am. Natural. 158, 572–584. doi: 10.1086/323586
Amat-Valera, M., Calero-Torralbo, M. A., Václav, R., and Valera, F. (2014). Cavity types and microclimate: implications for ecological, evolutionary, and conservation studies. Int. J. Biometeorol 58, 1983–1994. doi: 10.1007/s00484-014-0801-0
Anderies, J. M., Katti, M., and Shochat, E. (2007). Living in the city: resource availability, predation, and bird population dynamics in urban areas. J. Theor. Biol. 247, 36–49. doi: 10.1016/j.jtbi.2007.01.030
Blair, R. B. (1996). Land use and avian species diversity along an urban gradient. Ecol. Appl. 6, 506–519. doi: 10.2307/2269387
Brawn, J. D., and Balda, R. P. (1988). Population biology of cavity nesters in northern Arizona: do nest sites limit breeding densities? Condor 90, 61–71. doi: 10.2307/1368434
Brazill-Boast, J., Pryke, S. R., and Griffith, S. C. (2013). Provisioning habitat with custom-designed nest-boxes increases reproductive success in an endangered finch. Austral Ecol. 38, 405–412. doi: 10.1111/j.1442-9993.2012.02424.x
Cadotte, M. W., Mai, D. V., Jantz, S., Collins, M. D., Keele, M., and Drake, J. A. (2006). On testing the competition-colonization trade-off in a multispecies assemblage. Am. Nat. 168, 704–709. doi: 10.1086/508296y
Case, T. J., and Gilpin, M. E. (1974). Interference competition and niche theory. Proc. Natl. Acad. Sci. U.S.A. 71, 3073–3077. doi: 10.1073/pnas.71.8.3073
Chamberlain, D. E., Cannon, A. R., Toms, M. P., Leech, D. I., Hatchwell, B. J., and Gaston, K. J. (2009). Avian productivity in urban landscapes: a review and meta-analysis. IBIS 151, 1–18. doi: 10.1111/j.1474-919X.2008.00899.x
Chesson, P. (2000). Mechanisms of maintenance of species diversity. Annu. Rev. Ecol. Syst. 31, 343–366. doi: 10.1146/annurev.ecolsys.31.1.343
Coombs, A. B., Bowman, J., and Garroway, C. J. (2010). Thermal properties of tree cavities during Winter in a Northern Hardwood Forest. J. Wildlife Manage. 74, 1875–1881. doi: 10.2193/2009-560
Davies, Z. G., Fuller, R. A., Loram, A., Irvine, K. N., Sims, V., and Gaston, K. J. (2009). A national scale inventory of resource provision for biodiversity within domestic gardens. Biol. Conserv. 142, 761–771. doi: 10.1016/j.biocon.2008.12.016
Devictor, V., Julliard, R., Couvet, D., Lee, A., and Jiguet, F. (2007). Functional homogenization effect of urbanization on bird communities. Conserv. Biol. 21, 741–751. doi: 10.1111/j.1523-1739.2007.00671.x
Duckworth, R. A. (2006a). Aggressive behaviour affects selection on morphology by influencing settlement patterns in a passerine bird. Proc. Biol. Sci. 273, 1789–1795. doi: 10.1098/rspb.2006.3517
Duckworth, R. A. (2006b). Behavioral correlations across breeding contexts provide a mechanism for a cost of aggression. Behav. Ecol. 17, 1011–1019. doi: 10.1093/beheco/arl035
Duckworth, R. A. (2008). Adaptive dispersal strategies and the dynamics of a range expansion. Am. Nat. 172, S4–S17. doi: 10.1086/588289
Duckworth, R. A. (2014). “Human-induced changes in the dynamics of species coexistence: an example with two sister species,” in Avian Urban Ecology: Behavioural and Physiological Adaptations, eds D. Gil and H. Brumm (Oxford, UK: Oxford University Press), 181–191.
Duckworth, R. A., and Badyaev, A. V. (2007). Coupling of dispersal and aggression facilitates the rapid range expansion of a passerine bird. Proc. Natl. Acad. Sci. U.S.A. 104, 15017–15022. doi: 10.1073/pnas.0706174104
Duckworth, R. A., Belloni, V., and Anderson, S. R. (2015). Cycles of species replacement emerge from locally induced maternal effects on offspring behavior in a passerine bird. Science 374, 875–877. doi: 10.1126/science.1260154
Evans, K. L., Newson, S. E., and Gaston, K. J. (2009). Habitat influences on urban avian assemblages. IBIS 151, 19–39. doi: 10.1111/j.1474-919X.2008.00898.x
Griffith, S. C., Pryke, S. R., and Mariette, M. (2008). Use of nest-boxes by the Zebra Finch (Taeniopygia guttata): implications for reproductive success and research. Emu 108, 311–319. doi: 10.1071/MU08033
Guinan, J. A., Gowaty, P. A., and Eltzroth, E. K. (2000). Western bluebird (Sialia mexicana). Birds N. Am. 510, 1–31. doi: 10.2173/bna.510
Holway, D. A. (1999). Competitive mechanisms underlying the displacement of native ants by the invasive argentine ant. Ecology 80, 238–251. doi: 10.1890/0012-9658(1999)080[0238:CMUTDO]2.0.CO;2
Hughes, C. L., Hill, J. K., and Dytham, C. (2003). Evolutionary trade-offs between reproduction and dispersal in populations at expanding range boundaries. Biol. Lett. 270, S147–S150. doi: 10.1098/rsbl.2003.0049
Hutchinson, G. E. (1959). Homage to Santa Rosalia, or why are there so many kinds of animals? Am. Nat. 93, 145–159. doi: 10.1086/282070
Hutto, R. L. (1995). Composition of bird communities following stand-replacement fires in northern rocky mountain (U.S.A.) conifer forests. Conserv. Biol. 9, 1041–1058. doi: 10.1046/j.1523-1739.1995.9051033.x-i1
Hutto, R. L., and Patterson, D. A. (2016). Positive effects of fire on birds may appear only under narrow combinations of fire severity and time-since-fire. Int. J. Wildland Fire 25, 1074–1085. doi: 10.1071/WF15228
Johnson, L. S., and Kermott, L. H. (1994). Nesting success of cavity-nesting birds using natural tree cavities. J. Field Ornithol. 65, 36–51.
Kneitel, J. M., and Chase, J. M. (2004). Trade-offs in community ecology: linking spatial scales and species coexistence. Ecol. Lett. 7, 69–80. doi: 10.1046/j.1461-0248.2003.00551.x
Kotliar, N. B., Kennedy, P. L., and Ferree, K. (2007). Avifaunal responses to fire in southwestern montane forests along a burn severity gradient. Ecol. Appl. 17, 491–507. doi: 10.1890/06-0253
Lancaster, R. K., and Rees, W. E. (1979). Bird communities and the structure of urban habitats. Can. J. Zool. 57, 2358–2368. doi: 10.1139/z79-307
Lehmkuhl, J. F., Everett, R. L., Schellhaas, R., Ohlson, P., Keenum, D., Riesterer, H., et al. (2003). Cavities in snags along a wildfire chronosequence in eastern Washington. J. Wildlife Manage. 67, 219–228. doi: 10.2307/3803077
Martin, K., Aitken, K. E. H., and Wiebe, K. L. (2004). Nest sites and nest webs for cavity-nesting communities in interior British Columbia, Canada: nest characteristics and niche partitioning. Condor 106, 5–19. doi: 10.1650/7482
Marzluff, J. M. (2001). “Worldwide urbanization and its effects on birds,” in Avian Ecology and Conservation in an Urbanizing World, eds J. M. Marzluff, R. Bowman, and R. Donnelly (New York, NY: Springer), 19–47.
Melles, S., Glenn, S., and Martin, K. (2003). Urban bird diversity and landscape complexity: Species-environment associations along a multiscale habitat gradient. Conserv. Ecol. 7:5. doi: 10.5751/ES-00478-070105
Newton, I. (1994). The role of nest sites in limiting the numbers of hole-nesting birds - a review. Biol. Conserv. 70, 265–276. doi: 10.1016/0006-3207(94)90172-4
Palmer, T. M. (2003). Spatial habitat heterogeneity influences competition and coexistence in an African acacia ant guild. Ecology 84, 2843–2855. doi: 10.1890/02-0528
Pfennig, K. S., and Pfennig, D. W. (2005). Character displacement as the “best of a bad situation”: fitness trade-offs resulting from selection to minimize resource and mate competition. Evolution 59, 2200–2208. doi: 10.1554/05-263.1
Pinkowski, B. C. (1979). “Foraging ecology and habitat utilization in the genus Sialia,” in The Role of Insectivorous Birds in Forest Ecosystems, eds J. G. Dickson, R. N. Conner, R. R. Fleet, J. C. Kroll, and J. A. Jackson (New York, NY: Academic Press), 165–190.
Plissner, J. H., and Gowaty, P. A. (1995). Eastern Bluebirds are attracted to two-box nest sites. Wilson Bull. 107, 289–295.
Power, H. W., and Lombardo, M. P. (1996). “Mountain bluebird,” in The Birds of North America, Vol. 222, eds A. Poole and F. Gill (Philadelphia, PA: Birds of North America, Inc.), 1–21.
Remm, J., and Lõhmus, A. (2011). Tree cavities in forests – The broad distribution pattern of a keystone structure for biodiversity. For. Ecol. Manage. 262, 579–585. doi: 10.1016/j.foreco.2011.04.028
Robb, G. N., McDonald, R. A., Chamberlain, D. E., and Bearhop, S. (2008). Food for thought: supplementary feeding as a driver of ecological change in avian populations. Front. Ecol. Environ. 6, 476–484. doi: 10.1890/060152
Robles, H., and Martin, K. (2013). Resource quantity and quality determine the inter-specific associations between ecosystem engineers and resource users in a cavity-nest web. PLoS ONE 8:e74694. doi: 10.1371/journal.pone.0074694
Rodríguez, J., Avilés, J. M., and Parejo, D. (2011). The value of nestboxes in the conservation of Eurasian Rollers Coracias garrulus in southern Spain. IBIS 153, 735–745. doi: 10.1111/j.1474-919X.2011.01161.x
Rosenzweig, M. L. (1995). Species Diversity in Space and Time. Cambridge: Cambridge University Press.
Saab, V. A., Russell, R. E., and Dudley, J. G. (2007). Nest densities of cavity-nesting birds in relation to postfire salvage logging and time since wildfire. Condor 109, 97–108. doi: 10.1650/0010-5422(2007)109[97:NDOCBI]2.0.CO;2
Schieck, J., and Song, S. J. (2006). Changes in bird communities throughout succession following fire and harvest in boreal forests of western North America: literature review and meta-analysis. Can. J. For. Res. 36, 1299–1318. doi: 10.1139/x06-017
Schoener, T. W. (1983). Field experiments on interspecific competition. Am. Nat. 122, 240–285. doi: 10.1086/284133
Semel, B., and Sherman, P. W. (1995). Alternative placement strategies for wood duck nest boxes. Wildlife Soc. Bull. 23, 463–471.
Shochat, E., Lerman, S. B., Anderies, J. M., Warren, P. S., Faeth, S. H., and Nilon, C. H. (2010). Invasion, competition, and biodiversity loss in urban ecosystems. Bioscience 60, 199–208. doi: 10.1525/bio.2010.60.3.6
Shochat, E., Warren, P. S., Faeth, S. H., McIntyre, N. E., and Hope, D. (2006). From patterns to emerging processes in mechanistic urban ecology. Trends Ecol. Evol. 21, 186–191. doi: 10.1016/j.tree.2005.11.019
Tessier, A., and Woodruff, P. (2002). Trading off the ability to exploit rich versus poor food quality. Ecol. Lett. 5, 685–692. doi: 10.1046/j.1461-0248.2002.00373.x
Tilman, D. (1994). Competition and biodiversity in spatially structured habitats. Ecology 75, 2–16. doi: 10.2307/1939377
Tilman, D., and Pacala, S. (1993). “The maintenance of species richness in plant communities,” in Species Diversity in Ecological Communities, eds R. E. Ricklefs and D. Schluter (Chicago, IL: University of Chicago Press) 13–25.
Keywords: successional dynamics, species replacement, interference competition, competitive dominance, colonization, resource heterogeneity
Citation: Duckworth RA, Hallinger KK, Hall N and Potticary AL (2017) Switch to a Novel Breeding Resource Influences Coexistence of Two Passerine Birds. Front. Ecol. Evol. 5:72. doi: 10.3389/fevo.2017.00072
Received: 22 February 2017; Accepted: 19 June 2017;
Published: 11 July 2017.
Edited by:
Caroline Isaksson, Lund University, SwedenReviewed by:
Frédéric Angelier, UMR7372 Centre d'Études Biologiques de Chizé (CEBC), FranceRoss Alexander Crates, Australian National University, Australia
Copyright © 2017 Duckworth, Hallinger, Hall and Potticary. This is an open-access article distributed under the terms of the Creative Commons Attribution License (CC BY). The use, distribution or reproduction in other forums is permitted, provided the original author(s) or licensor are credited and that the original publication in this journal is cited, in accordance with accepted academic practice. No use, distribution or reproduction is permitted which does not comply with these terms.
*Correspondence: Renée A. Duckworth, cmFkM0BlbWFpbC5hcml6b25hLmVkdQ==