- Department of Agricultural Sciences, University of Naples Federico II, Portici, Italy
Long term survival of a species relies on maintenance of genetic variability and natural selection by means of successful reproduction and generation turnover. Although, basic to monitor the conservation status of a plant species, life history data are rarely available even for threatened species due to the gap between the large amount of information required and the limits in terms of time and available economic resources to gather these data. Here, the focus on bottlenecks in life-cycle of rare endangered plant species is proposed as a resolving approach to address the challenges of feasible conservation actions. Basic considerations for this approach are: (a) all biological and ecological studies on plant species can be scientifically important, but not all of them are equally relevant to conservation planning and management requirements; (b) under a changing environment, long term survival of a species relies on generation turnover; (c) for conservation purposes, priority should be given to studies aimed to focus on bottlenecks in the succession of generations because they prevent, or slow down natural selection processes. The proposed procedure, named Systematic Hazard Analysis of Rare-endangered Plants (SHARP), consists of a preliminary survey of the already available information on the species and two main components. The first component is the identification of the bottlenecks in the life cycle by means of field surveys. The second is the diagnosis of the causes of the bottleneck by appropriate experimental methods. The target is to provide researchers, managers and practitioners with substantiated indications for sustainable conservation measures.
Introduction
Plant species are assessed as rare if they have a restricted range, if they occur only in one or few specific habitats, and/or if their populations are always small (Rabinowitz, 1981; Rabinowitz et al., 1986). Some types of rarity can be considered a natural state while others are associated to a high risk of extinction. Plant species become rare due to both natural and anthropogenic selective pressures (Frankel and Soulé, 1981; Pegtel, 1998; Briggs, 2009). These plants are considered less able to adapt to changing environmental conditions because their populations lack the necessary genetic variability to survive and reproduce (Spielman et al., 2004). As a consequence, their populations can demographically decline and enter into extinction vortices (Gilpin and Soulé, 1986). Populations of rare plants that fail to be self-sustainable in the long-term period deserve conservation concerns (Briggs, 2009). Species defined as rare and endangered are included in Red Lists and are protected by local, national or international laws.
Although, practical conservation is concentrated on the management of the habitat, a significant part of conservation activity is focused on the management of individual species. New approaches for conservation priorities using life history traits are widely accepted for animals (Loyola et al., 2009; Hidasi-Neto et al., 2015). For plants, although data on species biology and ecology are considered basic to many methods aimed at establishing factors responsible for species rarity (e.g., Kunin and Gaston, 1993; Kunin and Shmida, 1997; Murray et al., 2002; Pilgrim et al., 2004; Farnsworth, 2007; Gabrielová et al., 2013, the species based approach is frequently applied to assess the species biological status and the population viability analysis (Schemske et al., 1994; Crone et al., 2011). The application of these detailed research methods to conservation management, are more and more frequently considered too costly in terms of scientific effort, time and economic resources (e.g., Frankel et al., 1995; Cook et al., 2010). Within this scenario, disputes are growing between academics and practitioners and to trigger a proactive dialogue further innovative proposals are urgently requested (e.g., Laurance et al., 2012).
The present approach was developed in this framework and based on the following considerations: (a) no conservation biologist would disagree in principle with the value of species specific knowledge for conservation planning; (b) both scientists and practitioners involved in conservation, call for more studies on genetics, demography, ecology, and life history of threatened plant species (e.g., Briggs, 2009). Revaluating the species based approach, this proposal is aimed at answering the following question: is it possible to formulate a sustainable species-based approach able firstly to pinpoint the bottleneck in the life cycle of a rare and endangered species, then to identify reasons for its status and finally to provide specific indications for conservation actions? The challenge is taken not pretending to be exhaustive but triggering and with the awareness that any new proposal for single species conservation must be feasible. In the following paragraphs, the rationale of this proposal is described and discussed.
Objective of this paper is to describe the rationale of Systematic Hazard Analysis of Rare-endangered Plants (SHARP), an exploratory tool to be applied to single rare endangered species to identify possible bottlenecks in the generation turnover and their causes. Its final aim is to provide substantiated indications for feasible conservation actions.
Rationale of Systematic Hazard Analysis of Rare-endangered Plants (SHARP)
SHARP is a species-based approach aimed to provide information on biological/ecological constraints of single rare and endangered plant species to be used for action planning and conservation management. It consists of a systematic assessment of bottleneck in the life cycle of a single plant species in a given geographical area and the identification of factors limiting the generation turnover. SHARP first identifies with field surveys and logic deductions the main bottlenecks in the life cycle of the plant species. Subsequently, it converges appropriate scientific expertise only on the highlighted bottlenecks to understand reasons for the constraints. Final results are used to suggest specific conservation measures.
The SHARP approach is based on four leading considerations described in the following paragraphs. Scientists must accept that all biological and ecological studies, although comparable for scientific importance, are not equally relevant to conservation planning and management requirements. Setting the targets for species conservation, priority should be given to applied research projects focusing on biological traits that constitute bottlenecks in natural selection processes because they prevent, or slow down, the succession of generations.
Under a changing environment, sexual reproduction is the only natural process able to improve genetic variability of the offspring and enhance the probability of obtaining new genotypes better adapted to the modified conditions (e.g., Frankel et al., 1995). The rate of successful sexual reproduction and generation turnover constitutes key information to evaluate the conservation perspectives of endangered plant species even when extended clonal growth occurs (Tepedino, 2012; Berjano et al., 2013).
Presence/absence of an angiosperm species in a given area is based on records of the adult sporophyte occurrence. Consequently, when the species distribution approach is used for conservation purposes, information on the regular fulfillment of each stage in the life cycle is neglected. Therefore, data on local occurrence of cloning and/or reproductive process to develop a number of new sporophytes (seeds), genetically diverse and ready to be dispersed away from the mother plant, is not taken into account.
Given that the whole plant life cycle is a continuous gradual phenomenon, the occurrence of one stage in the cycle is evidence of the successful development of the previous one, while failure to achieve a specific phase indicates a critical problem somewhere along the processes that should have taken place after the previous successful step.
SHARP is an exploratory tool aimed to identify hazards in the regular succession of life cycles of a single species. Within the life cycle of a generic angiosperm, SHARP identifies four major stages that are at the same time representative of critical phases and easily detectable in the field: (a) flowering, (b) seed production and dispersal, (c) seedling recruitment, (d) cloning (Figure 1). The achievement of each stage in the process is highly dependent from specific environmental conditions and some of them also rely on the interactions with biotic vectors (e.g., pollinators, seed dispersers).
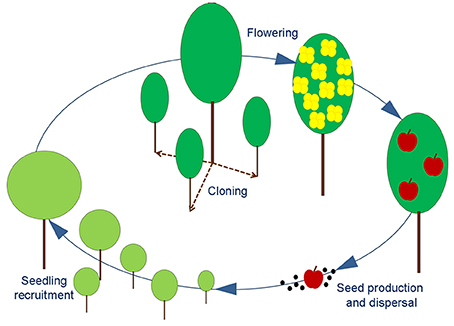
Figure 1. Schematic representation of an angiosperm life cycle. Adult plant can produce new individuals either by vegetative propagation (dashed line) or by reproduction (solid line). The stages of Flowering, Seed production, Seedling recruitment and Cloning are easily detectable with field surveys and used to identify the main bottlenecks for generation turnover.
The activity of SHARP is based on a preliminary phase (Step 0) and two main steps: identification of the main bottlenecks (Step 1) and Diagnoses of bottleneck causes (Step 2). Step 0 is an apriori phase, consisting of a preliminary and comprehensive literature review and subsequent elaboration of any biological, ecological, and socio-economic information on the species and its close relatives. Information on life history, genetic diversity, taxonomic distinctness, phenology, abundance, range size, habitat specificity, populations size, etc., have to be gathered. Scientific literature databases, IUCN bibliography or even direct contact to IUCN assessors and reviewers can be used as basic information sources. The aim of this preliminary survey is to meet practical requirements such as indication of how, where and when the surveys need to be undertaken. This knowledge can be elaborated by a botanist in a few weeks and used to prepare species specific guidelines. The latter include timelines for periodic (from weekly to monthly basis) surveys and protocols aimed to spot and assess every critical stage.
Step 1 consists of field surveys aimed to check the achievement of the above mentioned main stages (Figure 1) by answering the following questions: (a) Do plants flower? (b) Are seeds produced? (c) Does seedling recruitment occur? (d) Does cloning occur? (Figure 2). After a short training, any conservation botanist or ecologist will be ready to conduct Step 1 activities. The operator, on the basis of the guidelines prepared at the end of Step 0, will survey the plants in the wild populations to check all stages in sequence. A positive answer is considered evidence of the successful development of all previous stages in the cycle and no priority will be given to further investigations on these processes. The identification of a breakdown at a specific stage (negative answer) will evidence that a bottleneck is occurring along the processes from the former positive check (Figure 2). Some species might not show a total breakdown of a specific stage within a population, but a limitation. The available knowledge on the species, on its close relatives and on the habitat, can be used to define threshold levels in order to discriminate bottleneck from natural variation. The indication of the threshold levels for the first assessment of the weakness points, can be evaluated in Step 0 and reported in the specific guidelines.
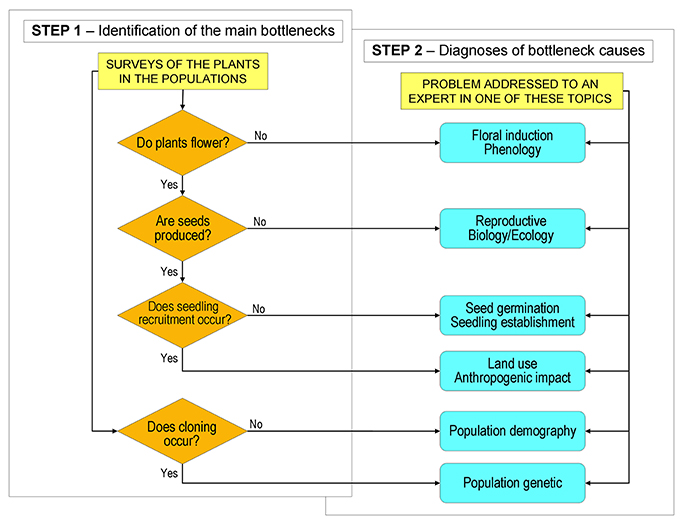
Figure 2. Illustrative path diagram of Step 1 and Step 2 of the SHARP procedure. Step 1 is aimed to check with field surveys the successful achievement of the main stages of the life cycle. Failure to reach one of the stages suggests the occurrence of a bottleneck. In Step 2, according to the bottlenecks identified in Step 1, a researcher with specific expertise is involved to clarify the causes of the bottlenecks and suggest applicable countermeasures. Reported bottlenecks have to be considered as an illustrative, yet incomplete list of cases.
When the bottlenecks have been identified, Step 1 is concluded. At this point, Step 2 starts and further investigations are carried out only on those processes that have been pinpointed as bottlenecks. Step 2 is aimed to refine the diagnosis of the specific bottleneck clarifying the causes of the constraints and interactions between biological phenomena and environmental conditions. This work is committed to scientists with appropriate expertise. Appointed researchers will apply current scientific methods to clarify the limiting processes. Depending on individual cases, experiments can be performed in the field and/or under controlled conditions. The final aim of scientists' investigations must be suggestions of countermeasures for sustainable conservation of the species.
Simulated Application of SHARP to Case Species
Possible scenarios after Step 1 and resulting indication for priority follow-up activities in Step 2 are outlined in Table 1 only as illustrative examples. For each scenario a case species, explicatory of the specific life cycle bottleneck is also reported.
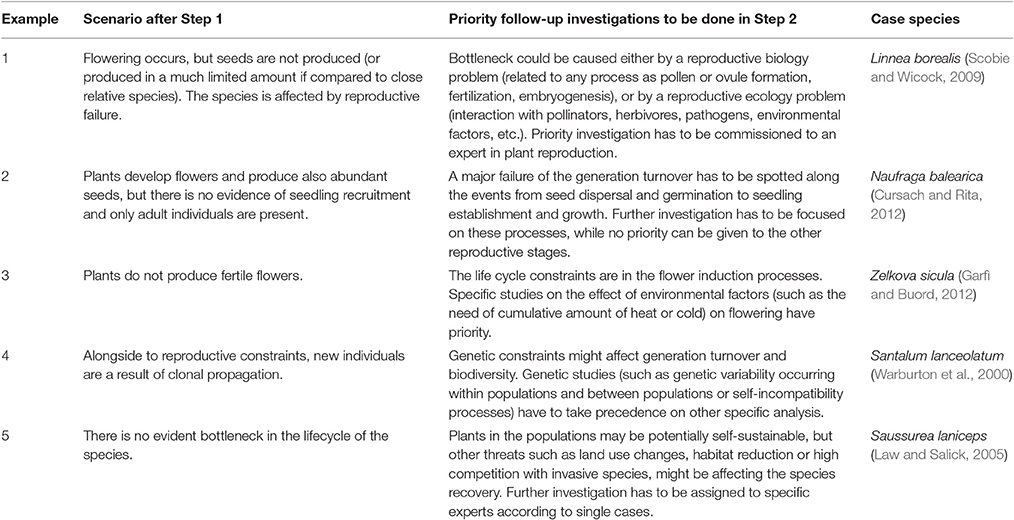
Table 1. Illustrative examples of (a) possible scenarios after Step 1, (b) indication for priority follow-up activities in Step 2 and (c) single case species whose bottleneck is reported in literature.
SHARP application to Naufraga balearica would have followed a setup similar to that utilized by Cursach and Rita (2012). Surveys in Step 1 would have disclosed that seed germination occurs under natural conditions, but seedling mortality is high due to many factors including seasonal environmental conditions, suitability of the germination site, herbivore pressure and competition for space. Nevertheless, to suggest substantiated indications for conservation actions, the application of SHARP procedure would have given less priority to studies on pollen/ovule ratio, mating system and pollinators (Cursach and Rita, 2012) focusing soon on seed germination and seedling establishment.
Application of SHARP procedure to Zelkova sicula would have suggested a priority conservation approach quite different from that really used and based on prioritization of the research studies aimed to understand causes of the breakdown of generation turnover.
Z. sicula is a relict tree species of the Tertiary flora, endemic to the mountain of SE Sicily (Italy) and consisting of only two populations of a few hundred trees (Garfì et al., 2011). In 2011 a project was funded by a composite (from local to international) partnership to ensure the survival of Z. sicula through in situ and ex situ conservation actions (http://www.zelkovazione.eu/). With a total budget of more than 2 million euro, the project is aimed to eliminate the threat factors in order to obtain the protection of the species through concrete activities of conservation, standard legal protection acts, information campaigns and awareness raising. Countermeasures (such as fencing against grazing/misuse disturbances, an emergency irrigation system and pilot planting tests) have been already realized.
From the scientific point of view, after the discovery of Z. sicula in 1991 (Di Pasquale et al., 1992), various studies have been reported regarding its origin and systematic, including caryology and dendroecology (Quézel et al., 1993; Garfì, 1998), but also flowering (Garfì, 1997), pollen morphology (Nakagawa et al., 1998), leaf anatomical traits (Wang et al., 2001), plant architecture and growth patterns (Garfì et al., 2002), genetic characterization (Fineschi et al., 2002, 2004; Denk and Grimm, 2005), plant interactions with other organisms (Barbagallo, 2002; Sidoti and Granata, 2005; Barbagallo and Cocuzza, 2008). Despite the numerous studies, it has been reported that much still needs to be understood both in terms of basic knowledge and issues for conservation planning of this species (Garfì and Buord, 2012).
Simulated outcomes of the SHARP application to Z. sicula soon after its discovery might be synthetized as in the following scenario. In Step 0 all available information on Z. sicula, as well as on its congeneric species, is gathered. Special attention is done to Z. abelicea (Lam.) Boiss., a threatened endemic species from another Mediterranean isle, discovered and studied earlier than Z. sicula (Kozlowski et al., 2014, and cited references). Altogether, these data are used to edit practical guidelines for Step 1 (e.g., indications on where, when, how and how frequently to look for flowers, fruit, seeds, seedlings). In Step 1 the life cycle is monitored by means of field surveys. Most likely, results could have been similar to those reported by Garfì (1997) or by Garfì and Buord (2012): “flowering is irregular, seeds seem sterile and most trees are very probably of clonal origin.” Based on such data, two bottlenecks in the life cycle of Z. sicula would have been identified: the absence of flowering in most of the years and the development of sterile seeds when flowering occurs. In Step 2 priority would have given to research activities aimed to investigate reasons for the two bottlenecks. Scientists with expertise in processes of flower induction and seed development would have been involved to formulate hypotheses, perform tests and clarify why Z. sicula does not flower regularly and why it produces sterile seeds.
At present, according to the available literature, such experimental work was not yet carried out on Z. sicula. Nevertheless, this knowledge would have established if and to which extent it is possible to protect the species through activities aimed to understand, and possibly eliminate, the threat factors limiting the natural turnover of generations.
Discussion
SHARP is aimed to identify the bottlenecks in the life cycle of rare endangered species and narrow further experimental research on factors and processes affecting generation turnover and leading to population decline. The application of the SHARP procedure will allow a direct transfer of scientific knowledge into management actions. The information on the causes of the life cycle constraints can be promptly used to activate specific corrective measures in situ and/or ex situ to reduce the local extinction risk of the species to an acceptable level.
Although at first sight the proposed method might seem similar to that based on an exhaustive study of the species life history, this is far from the case. The object of the SHARP procedure is not to know as much as possible on any perspective of the biology and ecology of the species and possibly establish the cause of its rarity. By means of the identification of the main weakness points in the species biology processes, its target is to prioritize studies aimed at understanding the causes of the breakdown of generation turnover in order to get conservation countermeasures.
The SHARP procedure is certainly not comparable with the biological status assessment. They start from different premises, use different approaches and have different aims. The evaluation of the biological status of rare and endangered plants is based on long-time collection of extensive demographic data, construction of stage-projection matrices and analysis of metapopulation dynamics (Schemske et al., 1994; Briggs, 2009). Together with the population viability analysis, the aim is to get predictive models of rare species dynamics and, consequently, they are an assessment of extinction risk. Differently, the SHARP procedure can be used following any assessment of extinction risk (e.g., Benito et al., 2009). It can be applied virtually to any angiosperm species reported in a checklist of taxa that have undergone an extinction risk assessment. It can be applied to the IUCN Red List of Threatened Species™ independently from its limits in global states of conservation (Martín, 2009). The SHARP procedure can be applied also to the priority lists of species obtained using together criteria of species rarity and of habitat vulnerability (Gauthier et al., 2010).
As an additional benefit, standard results obtained applying the systematic analysis of the SHARP approach can be included in already existing databases such as the IUCN Red List of Threatened Species™. Data from SHARP procedure constitute basic information for systematic reviews of the evidence-based conservation management increasingly proposed for long-term conservation and environmental management (Briggs, 2009; Cook et al., 2010).
Given that field activities for Step 1 of the SHARP approach are simple, they can be repeated regularly to monitor the effects of the applied conservation measures. Moreover, these surveys can be proposed also to comply with the obligations for the periodical assessment of conservation status such as those from the European Habitats Directive (Council of the European Communities, 1992, Article 11 and 17).
As a final remark, the SHARP procedure will create a point where the perspectives of conservation managers, practitioners and scientists can converge. Practitioners can obtain information required for conservation action, managers can handle their limited economic resources with no wastage and scientists can offer their expertise with the awareness that results will have practical application.
Author Contributions
The author confirms being the sole contributor of this work and approved it for publication.
Conflict of Interest Statement
The author declares that the research was conducted in the absence of any commercial or financial relationships that could be construed as a potential conflict of interest.
The reviewer AG and handling Editor declared their shared affiliation, and the handling Editor states that the process nevertheless met the standards of a fair and objective review.
References
Barbagallo, S. (2002). Zelkovaphis trinacriae, a new eriosomatine aphid genus and species living on Zelkova in Sicily (Rhynchota: Aphididae). Boll. Zool. Agric. Bachic. 34, 281–301
Barbagallo, S., and Cocuzza, G. (2008). Annotazioni bio-ecologiche su Zelkovaphis trinacriae afide galligeno (insecta, hemiptera) della Zelkova sicula (Ulmaceae). Naturalista Sicil S 32, 455–470.
Benito, B. M., Martínez-Ortega, M. M., Muñoz, L. M., Muñoz Lorite, J., and Peñas, J. (2009). Assessing extinction-risk of endangered plants using species distribution models: a case study of habitat depletion caused by the spread of greenhouses. Biodivers. Conserv. 18, 2509–2520. doi: 10.1007/s10531-009-9604-8
Berjano, R., Gauthier, P., Fisogni, A., Doblas, D., Pons, V., and Thompson, J. D. (2013). Mate limitation in populations of the endangered Convolvulus lineatus L.: a case for genetic rescue? J. Nat. Conserv. 21, 334–341. doi: 10.1016/j.jnc.2013.05.001
Briggs, D. (2009). Plant Microevolution and Conservation in Human-Influenced Ecosystems. New York, NY: Cambridge University Press.
Cook, C. N., Hockings, M., and Carter, R. W. (2010). Conservation in the dark? The information used to support management decisions. Front. Ecol. Environ. 8, 181–186. doi: 10.1890/090020
Council of the European Communities (1992). Council Directive 92/43/EEC of 21 May 1992 on the conservation of natural habitats and of wild fauna and flora. Official J. Eur. Commun. 206, 7–50.
Crone, E. E., Menges, E. S., Ellis, M. M., Bell, T., Bierzychudek, P., Ehrlén, J., et al. (2011). How do plant ecologists use matrix population models? Ecol. Lett. 14, 1–8. doi: 10.1111/j.1461-0248.2010.01540.x
Cursach, J., and Rita, J. (2012). Implications of the reproductive biology of the narrow endemic Naufraga balearica (Apiaceae) for its conservation status. Plant Syst. Evol. 298, 581–596. doi: 10.1007/s00606-011-0568-2
Denk, T., and Grimm, G. W. (2005). Phylogeny and biogeography of Zelkova (Ulmaceae sensu stricto) as inferred from leaf morphology, ITS sequence data and the fossil record. Bot. J. Linn. Soc. 147, 129–157. doi: 10.1111/j.1095-8339.2005.00354.x
Di Pasquale, G., Garfì, G., and Quézel, P. (1992). Sur la présence d'un Zelkova nouveau en Sicile sudorientale (Ulmaceae). Biocosme Mésogéen 8–9, 401–409.
Farnsworth, E. J. (2007). Sandplain plant life history traits as guides to multi-species management strategies for rare taxa. Biol. Conserv. 136, 44–52. doi: 10.1016/j.biocon.2006.10.045
Fineschi, S., Anzidei, M., Cafasso, D., Cozzolino, S., Garfì, G., Pastorelli, R., et al. (2002). Molecular markers reveal a strong genetic differentiation between two European relic tree species: Zelkova abelicea (Lam.) Boissier and Z. sicula Di Pasquale, Garfì and Quezel (Ulmaceae). Conserv. Genet. 3, 145–153. doi: 10.1023/A:1015222230887
Fineschi, S., Cozzolino, S., Migliaccio, M., and Vendramin, G. G. (2004). Genetic variation of relic tree species: the case of Mediterranean Zelkova abelicea (Lam.) Boisser and Z. sicula Di Pasquale, Garfì and Quézel (Ulmaceae). Forest. Ecol. Manage. 197, 273–278. doi: 10.1016/j.foreco.2004.05.018
Frankel, O. H., Brown, A. H. D., and Burdon, J. J. (1995). The Conservation of Plant Biodiversity. Cambridge: Cambridge University Press.
Frankel, O. H., and Soulé, M. E. (1981). Conservation and Evolution. Cambridge: Cambridge University Press.
Gabrielová, J., Münzbergová, Z., Tackenberg, O., and Chrtek, J. (2013). Can we distinguish plant species that are rare and endangered from other plants using their biological traits? Folia Geobot. 48, 449–466. doi: 10.1007/s12224-012-9145-x
Garfì, G. (1997). On the flowering of Zelkova sicula (Ulmaceae): additional description and comments. Plant Biosyst. 131, 137–142. doi: 10.1080/11263504.1997.10654174
Garfì G. (1998). Zelkova sicula (Ulmaceae), raro endemita della regione iblea. origine, evoluzione, prospettive di conservazione. Boll. Accad. Gioenia Sci. Nat. S 29, 267–284.
Garfì, G., Barbero, M., and Tessier, L. (2002). Architecture and growth patterns of Zelkova sicula (Ulmaceae) in south-east Sicily as a response to environmental conditions. J. Med. Ecol. 3, 65–76.
Garfì, G., and Buord, S. (2012). Relict species and the challenges for conservation: the emblematic case of Zelkova sicula Di Pasquale, Garfi et Quézel and the efforts to save it from extinction Biodivers. J. 3, 281–296.
Garfì, G., Carimi, F., Pasta, S., Rühl, J., and Trigila, S. (2011). Additional insights on the ecology of the relic tree Zelkova sicula Di Pasquale, Garfì et Quézel (Ulmaceae) after the finding of new population. Flora 206, 407–417. doi: 10.1016/j.flora.2010.11.004
Gauthier, P., Debussche, M., and Thompson, J. D. (2010). Regional priority setting for rare species based on a method combining three criteria. Biol. Conserv. 143, 1501–1509. doi: 10.1016/j.biocon.2010.03.032
Gilpin, M. E., and Soulé, M. E. (1986). “Minimum viable populations: processes of species extinction,” in Conservation Biology: The Science of Scarcity and Diversity, ed M. E. Soulé (Sunderland, MA: Sinauer Associates), 19–34.
Hidasi-Neto, J., Loyola, R., and Cianciaruso, M. V. (2015). Global and local evolutionary and ecological distinctiveness of terrestrial mammals: identifying priorities across scales. Divers. Distrib. 21, 548–559. doi: 10.1111/ddi.12320
Kozlowski, G., Frey, D., Fazan, L., Egli, B., Bétrisey, S., Gratzfeld, J., et al. (2014). The Tertiary relict tree Zelkova abelicea (Ulmaceae): distribution, population structure and conservation status on Crete. Oryx 48, 80–87. doi: 10.1017/S0030605312001275
Kunin, W. E., and Gaston, K. J. (1993). The biology of rarity: patterns, causes and consequences. Trends Ecol. Evol. 8, 298–301. doi: 10.1016/0169-5347(93)90259-R
Kunin, W. E., and Shmida, A. (1997). Plant reproductive traits as a function of local, regional, and global abundance. Conserv. Biol. 11, 183–192. doi: 10.1046/j.1523-1739.1997.95469.x
Laurance, W. F., Koster, H., Grooten, M., Anderson, A. B., Zuidema, P. A., Zwick, S., et al. (2012). Making conservation research more relevant for conservation practitioners. Biol. Conserv. 153, 164–168. doi: 10.1016/j.biocon.2012.05.012
Law, W., and Salick, J. (2005). Human-induced dwarfing of Himalayan snow lotus, Saussurea laniceps (Asteraceae). Proc. Nat. Acad. Sci. U.S.A. 102, 10218–10220. doi: 10.1073/pnas.0502931102
Loyola, R. D., Oliveira-Santos, L. G., Almeida-Neto, M., Nogueira, D. M., Kubota, U., Diniz-Filho, J. A., et al. (2009). Integrating economic costs and biological traits into global conservation priorities for carnivores. PLoS ONE 4:e6807. doi: 10.1371/journal.pone.0006807
Martín, J. L. (2009). Are the IUCN standard home-range thresholds for species a good indicator to prioritise conservation urgency in small islands? A case study in the Canary Islands (Spain). J. Nat. Conserv. 17, 87–98. doi: 10.1016/j.jnc.2008.10.001
Murray, B. R., Thrall, P. H., and Lepschi, B. J. (2002). Relating species rarity to life history in plants of eastern Australia. Evol. Ecol. Res. 4, 937–950.
Nakagawa, T., Garfì, G., Reille, M., and Verlaque, R. (1998). Pollen morphology of Zelkova sicula (Ulmaceae), a recently discovered relic species of the European Tertiary flora: description, chromosomal relevance, and palaeobotanical significance. Rev. Palaeobot. Palynol. 100, 27–37. doi: 10.1016/S0034-6667(97)00062-6
Pegtel, D. M. (1998). Rare vascular plant species at risk: recovery by seeding? Appl. Veget. Sci. 1, 67–74. doi: 10.2307/1479086
Pilgrim, E. S., Crawley, M. J., and Dolphin, K. (2004). Patterns of rarity in the native British flora. Biol. Conserv. 120, 161–170. doi: 10.1016/j.biocon.2004.02.008
Quézel, P., Di Pasquale, G., and Garfì, G. (1993). Découverte d'un zelkova en sicile sud-orientale. Incidence biogéographiques et historiques. Comp. R. Acad. Sci. Paris 316, 21–26.
Rabinowitz, D. (1981). “Seven forms of rarity,” in The Biological Aspects of Rare Plant Conservation, ed H. Synge (New York, NY: John Wiley and Sons), 205–217.
Rabinowitz, D., Cairns, S., and Dillon, T. (1986). “Seven forms of rarity and their frequency in the flora of the British Isles,” in Conservation Biology, The Science of Scarcity and Diversity, ed E. Soule (Sunderland, MA: Sinauer Associates), 182–204.
Schemske, D. W., Husband, B. C., Ruckelshaus, M. H., Goodwillie, C., Parker, I. M., and Bishop, J. G. (1994). Evaluating approaches to the conservation of rare and endangered plants. Ecology 75, 584–606. doi: 10.2307/1941718
Scobie, A. R., and Wicock, C. C. (2009). Limited mate availability decreases reproductive success of fragmented populations of Linnaea borealis, a rare, clonal self-incompatible plant. Ann. Bot. 103, 835–846. doi: 10.1093/aob/mcp007
Sidoti, A., and Granata, G. (2005). Diplodia sarmentorum e Fusicoccum aesculi, agenti di cancri su Zelkova sicula. Micol. Ital. 3, 44–48.
Spielman, D., Brook, B. W., and Frankham, R. (2004). Most species are not driven to extinction before genetic factors impact them. Proc. Nat. Acad. Sci. U.S.A. 101, 15261–15264. doi: 10.1073/pnas.0403809101
Tepedino, V. J. (2012). Overestimating population size of rare clonal plants. Conserv. Biol. 26, 945–947. doi: 10.1111/j.1523-1739.2012.01886.x
Wang, Y.-F., Ferguson, D. K., Zetter, R., Denk, T., and Garfì, G. (2001). Leaf architecture and epidermal characters in Zelkova, Ulmaceae. Bot. J. Linn. Soc. 136, 255–265. doi: 10.1111/j.1095-8339.2001.tb00571.x
Keywords: plant reproduction, plant conservation, plant generation turnover, plant life-cycle, sustainable conservation, reproductive success, life cycle bottleneck
Citation: Aronne G (2017) Identification of Bottlenecks in the Plant Life Cycle for Sustainable Conservation of Rare and Endangered Species. Front. Ecol. Evol. 5:76. doi: 10.3389/fevo.2017.00076
Received: 02 December 2016; Accepted: 27 June 2017;
Published: 12 July 2017.
Edited by:
Enrique Martínez-Meyer, National Autonomous University of Mexico, MexicoReviewed by:
Andres Garcia, National Autonomous University of Mexico, MexicoCarlos Alberto Lopez Gonzalez, Autonomous University of Queretaro, Mexico
Copyright © 2017 Aronne. This is an open-access article distributed under the terms of the Creative Commons Attribution License (CC BY). The use, distribution or reproduction in other forums is permitted, provided the original author(s) or licensor are credited and that the original publication in this journal is cited, in accordance with accepted academic practice. No use, distribution or reproduction is permitted which does not comply with these terms.
*Correspondence: Giovanna Aronne, YXJvbm5lQHVuaW5hLml0