Infection of Anaplasma phagocytophilum and Ehrlichia spp. in Opossums and Dogs in Campeche, Mexico: The Role of Tick Infestation
- 1Departamento de Conservacion de la Biodiversidad, El Colegio de la Frontera Sur-ECOSUR, Campeche, Mexico
- 2Unidad de Investigacion Medica de Enfermedades Infecciosas y Parasitarias, Centro Medico Nacional Siglo XXI, Instituto Mexicano del Seguro Social, Mexico City, Mexico
In recent years, some tick-borne diseases such as anaplasmosis and ehrlichiosis became widespread worldwide, threatening the health of humans, domestic animals and wildlife. The aims of this study were to determine the presence of Anaplasma phagocytophilum, Ehrlichia canis, and Ehrlichia chaffeensis in 102 opossums (Didelphis spp.) and 44 owned free-ranging dogs in southeastern Mexico using a specific polymerase chain reaction (PCR). A. phagocytophilum was detected in opossums and dogs with a prevalence of 3 and 27%, respectively. E. canis was only present in 7% of dogs, while we didn't detect E. chaffeensis in any host. We report the first evidence of infections of A. phagocytophilum in Didelphis virginiana and D. marsupialis in Mexico. The infection rates and patterns we found of A. phagocytophilum suggest that dogs are more directly involved in the ecology of this pathogen than opossums. Despite the small prevalence found, our results are of public health concern because of the zoonotic capabilities of A. phagocytophilum, the high tick infestation rates found and because both opossums and free-ranging dogs can achieve high population densities in the region.
Introduction
Anaplasma phagocytophilum, Ehrlichia canis, and Ehrlichia chaffeensis are tick-borne pathogens of a wide range of vertebrate hosts (McQuiston et al., 2003; Yabsley, 2010; Atif, 2015). Their ecology involves hard ticks (Ixodidae) as vectors and wildlife and domestic animals or human-associated fauna (D. virginiana, rodents) as hosts (McQuiston et al., 2003; Yabsley, 2010; Atif, 2015) (Figure 1). The emerging zoonotic pathogens E. chaffeensis and A. phagocytophilum are increasingly implicated as a human pathogen worldwide. The diseases they cause are human monocytic ehrlichiosis (HME) and human granulocytic anaplasmosis (HGA), respectively (Yabsley, 2010; Atif, 2015). Both pathogens cause similar clinical illnesses that involve malaise, gastrointestinal disorders, high fever, and severe headache (Dahlgren et al., 2011; Atif, 2015). During 2000–2007, the cases-fatality rate in the USA was 1.9 and 0.6%, respectively (Dahlgren et al., 2011). E. canis is not common in humans and his reservoir hosts are canids worldwide, especially domestic dogs (Sainz et al., 2015). It is a pathogen of high veterinary importance for dog health.
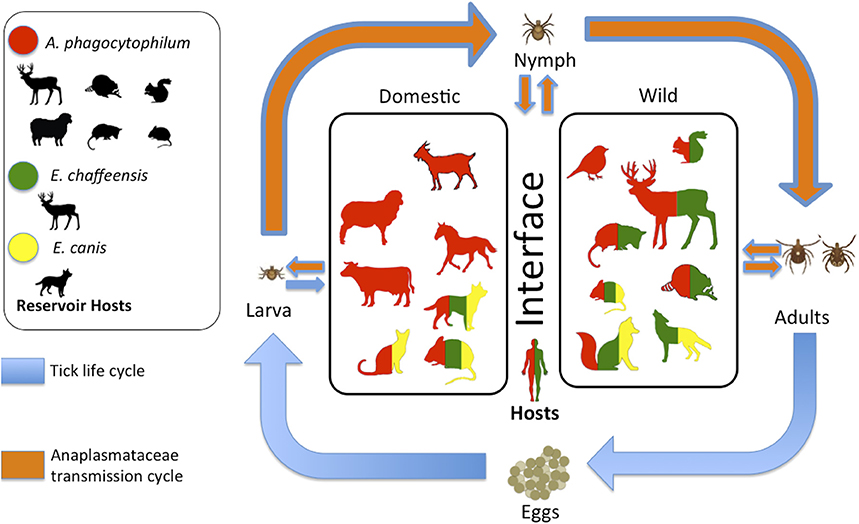
Figure 1. General scheme that integrates the Ixodes tick life cycle, the transmission cycle of Anaplasmataceae, and the hosts of both in the domestic-wildlife interface. Vertebrates in the center of the scheme have been positive at least once, via some method of diagnosis (serological or molecular). The color in the vertebrate indicates which pathogen can infect them. Image modified from the originally published by Estrada-Peña and de la Fuente (2014).
Despite the concerns for human health, the information about the presence of those pathogens in Mexico is scarce, and most reports have been focused on E. canis, domestic dogs as well as performed with preliminary serological tests (Pat-Nah et al., 2015; Movilla et al., 2016). Only recently the first Human fatality by HME in Mexico has been reported, suggesting the emergence of E. chaffeensis in Mexico (Sosa-Gutiérrez et al., 2016). Another recent study, reported the presence of these three pathogens in wild rodents from central Mexico, highlighting the potential risk to humans and wildlife exposed to these tick-borne rickettsiae (Sosa-Gutiérrez et al., 2014). Only domestic dogs have been reported as a host for E. canis (Pat-Nah et al., 2015), but no other definitive report has been made on wild vertebrates or humans in the study area. The domestic-wildlife interface is important in the transmission of zoonotic pathogens and represents an increasing and a significant threat to global health. Generally, emerging infectious diseases (EIDs) events are dominated by zoonosis (60.3% of EIDs) and the majority of these (71.8%) originate in wildlife and are increasing significantly over time (Jones et al., 2008). Similar conclusions are shown in Daszak et al. (2000), Morens et al. (2004), Weiss and McMichael (2004), and King et al. (2006). For this specific system, several studies identify the importance of the domestic-wildlife interface in the transmission of these three pathogens, associating factors to the risk of infection to A. phagocytophilum and E. chaffeensis. Such as the presence and abundance of vertebrate host species and vectors, forest cover, and high-risk outdoor activities (Paddock and Yabsley, 2007; Dahlgren et al., 2011; Bayles and Allan, 2014; Atif, 2015). Even though E. canis appears to be host-specific, one human case has been reported (Perez et al., 1996) and other wildlife canids, such as foxes, could be involved in the transmission of this pathogen (Guerrero-Sánchez and Weber, 2012). Thus, monitoring the presence of those pathogens in the most common and abundant host of this interface is relevant for understanding the disease dynamics, taking protection measures and preventing epidemiological outbreaks (Gompper, 2014). In this sense, species that are common and abundant in this interface, such as opossums (both Didelphis virginiana and D. marsupialis) and dogs, which in turn could be potential hosts for those pathogens (Figure 1), can be elements that facilitated zoonotic and anthropomorphic processes. It has been reported that only D. marsupialis, represent 40% of the total biomass of small mammals (Colchero et al., 2014), and human: dog ratio in rural towns of the study area can be as high as 1.7:1 (Ortega-Pacheco et al., 2007). Therefore, the objective of our base line study was to identify the role of opossums (D. virginiana and D. marsupialis) and owned, free-ranging dogs in the epidemiology of A. phagocytophilum, E. canis, and E. chaffeensis in a rural area near the city of Campeche, Mexico. To accomplish this objective, first, we determined the prevalence of those three pathogens in opossums and dogs through a polymerase chain reaction (PCR) diagnosis method, as well as assessing the abundance of Ixodidae ticks in both hosts. Then we identify factors associated with the abundance of ticks and the prevalence of these pathogens within the hosts.
Materials and Methods
Study Area
This study was conducted in a rural area near the city of Campeche at the Peninsula of Yucatan. It comprises the villages of Bobolá (19°46′13.46″N; 90°25′12.37″O), Chiná (19°45′49.09″N; 90°29′39.66″O), Xcampeu (19°47′45.80″N; 90°23′51.64″O), and the experimental camp of INIFAP, a research institute in Chiná, Campeche (19°45′2.53″N; 90°27′3.03″O) (Figure 2). The locations differ in human population density and therefore in dog population density, as the two are strongly correlated (Gompper, 2014). Economic activities vary in relation to the villages, predominating cattle ranching at Xcampeu, mechanized agriculture in Bobolá and commerce in Chiná. The landscape is composed of agricultural fields, pastures for livestock purposes and villages immersed in a continuum of tropical lowland forest and secondary vegetation (Noriega-Trejo and Arteaga-Aguilar, 2010). The area has a wet and dry season from June-September and January-May respectively (Villalobos-Zapata and Mendoza-Vega, 2010).
Sample Collection
The sample collection was carried out from January 26 to April 15, 2016. Opossums were captured with Tomahawk traps in three different sites at each location. We categorized the sites for trapping as anthropogenic, natural, or in the edge zones. Anthropogenic sites included human structures and areas adjacent to buildings, this kind of site where mostly in the villages but also in isolated farms. Traps cataloged as “natural,” were placed on the primary or secondary forest, and to avoid any potential edge effect on tick abundance in opossums, traps were placed at least 50 m from the nearest edge. We also captured opossums in “edge zones” between forest and agricultural fields, livestock pastures, and main roads.
From the 150 reactions, we had available for the molecular diagnosis, our intention was to dedicate at least 100 to the opossums and the rest of the dogs. We not only collected the ticks of those dogs and opossum, but also from the majority of dogs per village that were owned but free-ranging as well as the greatest number of opossums we could capture. From all captured opossums and dogs sampled, we collected whole blood in a 3 ml EDTA BD Vacutainer tubes and then stored at −20°C until use, detailed methods of trapping and sample collection have been reported elsewhere (Guerrero-Sánchez and Weber, 2012). All procedures were conducted after approval from El Colegio de la Frontera Sur Ethic Committee and trapping permits granted by SGPA-DGVS-SEMARNAT No. 007765/15. Consent from all dog owners was provided before handling and sampling their animals. Finally, we collected and counted feeding ticks (larvae, nymph, and adults) on all the opossums and dogs sampled. For those opossums and dogs that were highly tick-infested, we counted the ticks located on the bare body parts (legs, tail, and ears) and in two areas (circles with 5-cm diameter) located on the ventral and dorsal area to extrapolate the number of ticks as a function of the body size.
Molecular Diagnosis
DNA was extracted from whole blood by using DNeasy Blood and Tissue Kit (QIAGEN, Valencia, CA, USA) according to the manufacturer's protocol. DNA was analyzed by PCR using primers 15F and 842R, which are specific for the 16S rRNA of Anaplasmataceae and generate an 800 pairs bases fragment (Figure 3). This product was amplified in a nested PCR with species-specific primers for A. phagocytophilum, E. canis, and E. chaffeensis who amplified approximately a 390 pairs bases fragment (Anderson et al., 1992). PCR was performed on a Thermocycler Applied Biosystem 2720. We used extracted DNA from dogs naturally infected with each pathogen as a positive control and ultrapure water as negative controls. The nested PCR product was electrophoresed in 1.5% agarose gels, stained with bromide ethidium and photographed. The PCR preparation mix, the reaction in the thermocycler and the electrophoresis were performed in different rooms.
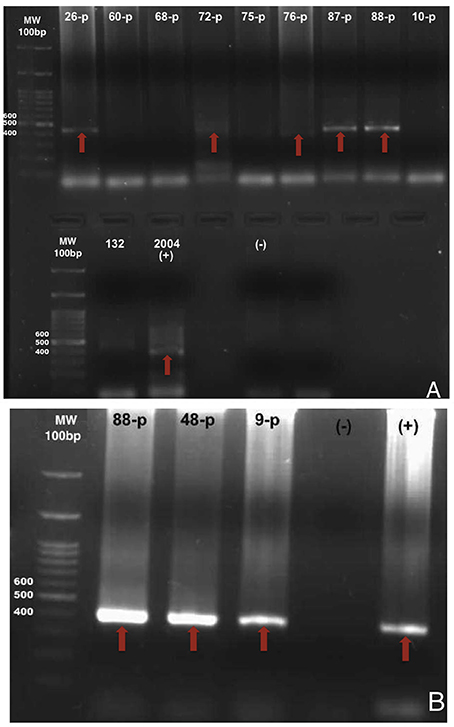
Figure 3. Photos of agarose gels with products amplified by nested PCR. (A) shows the fragments of 400 base pairs of the 16s rDNA gene of A. phagocytophilum from samples of dog 26-p, 72-p, 76-p, 87-p, 88-p, and the 2004 positive control. (B) shows the fragments of 400 base pairs of the 16s rDNA gene from E. canis from samples of dog 88-p, 48-p, 9-p, and positive control. Red arrows indicate bands (thresholds) for positive detection of pathogens.
Data Analysis
Values of prevalence of infection and tick abundance in hosts were established as the number of hosts infected with a particular pathogen species divided by the number of hosts examined for that pathogen species, and the total number of individuals of Ixodes ticks in the host sample divided by the total number of hosts examined (Bush et al., 1997).
We related tick abundance with the variable of kind of host (dogs or opossums), and tick abundance in the opossum with the independent variables of sex, localities, and the kind of site (anthropogenic, natural, or edge zone), using either a U-Mann-Whitney (when the independent variable has two groups, such as sex), or a Kruskal-Wallis test (when the independent variable has more than two groups) (Zar, 1984). A generalized linear model with a negative binomial distribution and a logarithmic link function was subsequently performed to determine the influence of locality, distance to the closest farm, kind of site, sex, and weight-length rate on the tick abundance in the opossums. We opted for the negative binomial distribution due to high levels of over dispersion in the data when models were fitted with a Poisson distribution.
Chi-square with a Yates correction test and Fisher's exact tests were used on contingency tables to compare prevalence related to the categorical risk factor of infection. We used kind of host, tick presence (infested or not infested), locality, and the kind of site as risk factors. Relative risk (Rr) was calculated as the fraction of the incidence rate of infection in the exposed between the incidence rates of infection in unexposed hosts. We defined the exposed group in each risk factor as the group more likely to be infected based on epidemiological hypothesis. Probabilities of p-value < 0.05 were considered statistically significant (Zar, 1984), but biological or epidemiological significance was considered and analyzed independently of p values.
Based on the mean human: dog ratio derived from a human household survey of 14 different rural localities in México, we estimated the owned dog abundance in each locality and in the study area which is a polygon delimited by the principal routes and that comprise the four localities presented in this study (Gompper, 2014). Then, we used localities (with higher or lower dog abundance) as a variable that explains the tick abundance of the hosts and as a risk factor for infection.
Results
Infections and Prevalence
A total of 102 opossums and 44 dog blood samples were examined for A. phagocytophilum, E. canis, and E. chaffeensis in this study. Only DNA of A. phagocytophilum was found in both species of opossums, one D. marsupialis and two D. virginiana were infected (Figure 3). In addition to this last pathogen, we also found DNA of E. canis in dogs. Total prevalence with A. phagocytophilum was 3% in opossums and 27% in dogs, while the prevalence of E. canis in dogs was 7%. None of the samples was positive for E. chaffeensis. The infections with those agents and the prevalence according to the localities and species of the host are presented in Table 1. Only one dog was co-infected with A. phagocytophilum and E. canis.
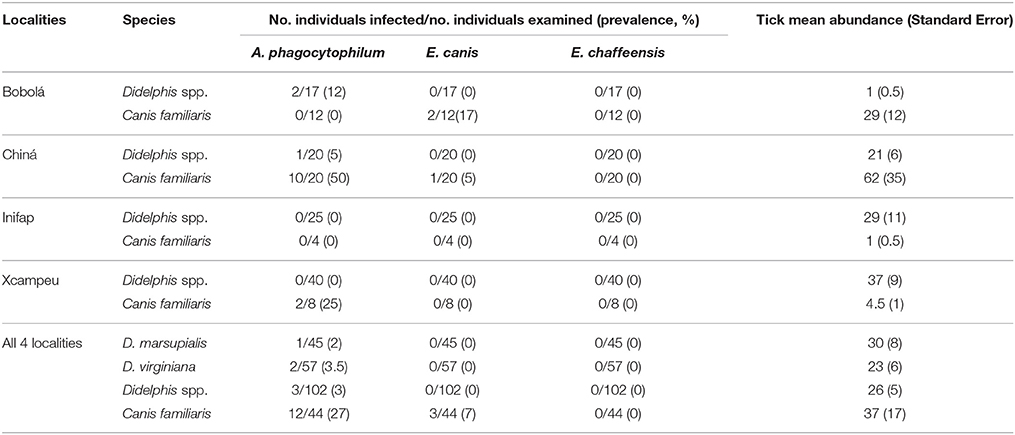
Table 1. Prevalence of A. phagocytophilum, E. canis, and E. chaffeensis and tick mean abundance among dogs and opossum (Didelphis. spp.) in four localities near Campeche, Mexico.
Tick Abundance in Hosts
The tick abundance in opossums was not statistically different between both species (U = 1116.5, Z = −1.46, p = 0.14, U-Mann-Whitney), neither between the different capture sites (anthropogenic, natural, and edge zone) (U = 950.5, Z = −0.66, p = 0.5, U-Mann-Whitney). Tick abundance in opossums only was marginally different between sexes (X22 = 1.54, p = 0.46, Kruskal-Wallis), with the males having higher tick abundance than females (35.6 vs. 12.7 respectively) and by the locality (X23 = 25.6, p = 0.001, Kruskal-Wallis), where opossums from Bobolá had lower tick abundance than the rest of the localities (Table 1). We also found several opossums with an estimate of >200 feeding ticks on their bodies. The GLM show the same pattern as the non-parametric statistic, where sex and locality were the variables that explain tick abundance in opossums (Table 2). Estimation of owned dog abundance per locality is shown in Figure 4.
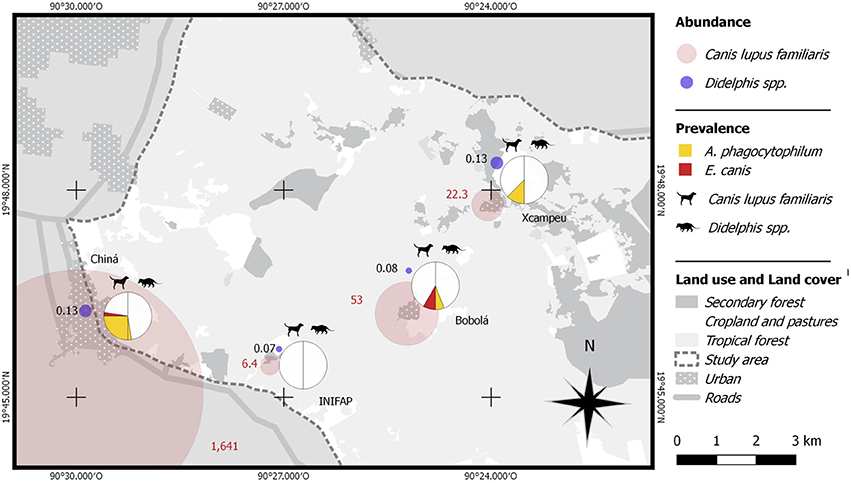
Figure 4. Spatial representation of dog and opossum relative abundance by locality and the prevalence of A. phagocytophilum and E. canis in hosts.
Prevalence and Risk Factors
None of the pathogens where associated with the tick presence in the host (X2 = 1.6, GL = 1, p = 0.1) or the kind of site where opossums were captured (X2 = 0.27, GL = 2, p = 0.55). Infections with A. phagocytophilum and E. canis were higher in dogs than in opossums (X2 = 17.2, gl = 1, p < 0.000; X2 = 4.1, gl = 1, p = 0.026 respectively) (Figure 4), therefore dogs had more risk of infection by A. phagocytophilum than opossums (Rr = 9.3). The relative risk of infection by E. canis per host species was not calculated, because none of the opossums was infected. In terms of the risk factor of locality, infections by A. phagocytophilum were higher in Chiná (X2 = 12.7, gl = 3, p < 0.000) (Figure 3), which in turn it is also the “exposed group” who had a higher risk of infection than the “non-exposed group” comprised by Bobolá, INIFAP and Xcampeu (Rr = 6.6). There were no association of infection by E. canis within any locality (X2 = 0.17, GL = 3, p = 1).
Discussion
High densities of dogs inhabiting the human-wildlife interface may facilitate their potential reservoir role for multihost pathogens (Gompper, 2014). The positive results we found support previous reports of dogs as a host for A. phagocytophilum (Woldehiwet, 2010; Atif, 2015; Çetinkaya et al., 2016). The previous report of A. phagocytophilum in dogs by PCR worldwide (serological studies excluded) vary from 0.5 to 6.3% (Çetinkaya et al., 2016), which is much less than the 27% prevalence we found. This difference could be due to: (1) A. phagocytophilum strain, (2) the presence of this pathogen in the rest of the vertebrate host community in the study area, and (3) previous studies have found that dogs with access to veterinary care might have low prevalence values, opposite to our study area (Jensen et al., 2007; Bowman et al., 2009; Çetinkaya et al., 2016; Movilla et al., 2016). We need further investigation to support the first two hypotheses and to discard that the high prevalence are due to the low sample size. The high density of free-ranging dogs and the unusually high prevalence we found suggest their role as a major reservoir host in the area. However, it is difficult to assess this conclusion, since there wasn't an exhaustive survey on dog population and on other potential hosts, such as squirrels and racoons (Keesing et al., 2010). We found that both species of opossums are potential hosts for A. phagocytophilum in southeastern Mexico. D. virginiana has already been reported as a competent host for A. phagocytophilum in the USA with a higher prevalence (36%) than the one we found (Keesing et al., 2012). This difference could be explained because opossums were captured in an area considered as of high risk of infection by this pathogen due to the population growth of his main reservoir host and vector (Dugan et al., 2006; Paddock and Yabsley, 2007; Dahlgren et al., 2011). To the best of our knowledge, this is the first study screening D. marsupialis for the presence of the three pathogens using molecular tools (PCR analysis) in southeastern Mexico.
The absence of E. canis and E. chaffeensis in opossums in the present study is an argument in favor that these pathogens cannot infect them. E. canis has never been detected in opossums. E. chaffeensis, has only been detected by serological methods in D. virginiana in two different studies, which may have cross-reacted with a related Ehrlichial agent (Yabsley, 2010). Furthermore, we need experimental studies to determine if both species of Ehrlichia can infect the Didelphidae. The prevalence of E. canis that we found in dogs is lower than the 36% reported previously in a city near the study area and despite they are considered as reservoir hosts for this pathogen (Pat-Nah et al., 2015). However, they did the study with dogs from animal shelters from the city, and this type of provenance is considered a risk factor of infection by E. canis de facto (Pat-Nah et al., 2015). Furthermore, infections by tick-borne pathogens may appear aggregated among hosts collected from closely spaced geographical sites (Paddock and Childs, 2003; Estrada-Peña and de la Fuente, 2014). Further research is needed in order to infer the low prevalence of E. canis in dogs and the absence of E. chaffeensis in the dog population from the study area.
Opossums from Bobolá had lower tick abundance than the rest of the localities. In part, this can be explained by the environmental history of capture sites. The proximity of the capture sites in this locality with the agricultural fields could affect tick abundance, since this activity is negatively associated with questing tick and tick abundance in the host because it excludes the hosts of ticks and the loss of habitat for these (Pfäffle et al., 2013). In contrast, cattle practices in Xcampeu and high dog densities in Chiná, may have favored the abundance of ticks in opossums. Nevertheless, it is important to mention that the environmental history is not sufficient to explain the observed pattern, since host-tick relationships are complex and depend on many local factors (microclimate, structure, and composition of vegetation and host community) (Pfäffle et al., 2013) and factors that occur at the landscape level (proportion of agricultural, livestock and vegetation areas, vegetation patch size, and connectivity with other patches) (Estrada-Peña and de la Fuente, 2014). The sex also influenced the abundance of ticks in opossums, being females the ones with the lower tick abundance. Even if this pattern hasn't been observed in opossums, it is common in other vertebrates such as deer and rodents (Brunner and Ostfeld, 2008; Ruiz-Fons et al., 2013). One of the main hypotheses to explain this, is the difference in behavior between males and females: for the case of opossums, it has been reported that males travel more distances during the day, even covering the territory of several females (Sunquist et al., 1987; Zarza and Medellín, 2014), which makes them more vulnerable to tick infestations. On the other hand, opossums had a lower tick abundance than dogs. This can be explained because the dogs had a higher body surface than opossums. Furthermore, dogs are present in high population densities in the study area, and it is well-known that tick infestations are density dependent. The habitat use of owned free-ranging dogs in the study area may facilitate tick infestation because they usually frequent open areas of the towns with high probability to be infested by the tick Rhipicephalus sanguineus, the domestic dog's main tick (Pat-Nah et al., 2015). In addition, the little veterinary health care that dogs receive here, makes them vulnerable to contracting high tick infestations as we found.
The only risk factors associated with infections by A. phagocytophilum and E. canis in the present study were the kind of host and locality. Opossums had a lower risk of infection by both pathogens than dogs. This result was to be expected because: (1) dogs are considered a common reservoir host for these two pathogens, (2) because of the greater abundance of ticks in dogs than opossums and; (3) the little (or none) veterinary health care they receive (Beall et al., 2012; Atif, 2015). There was also a locality effect: Chiná had the highest dog abundance, and this could have caused the infections with A. phagocytophilum to be concentrated there because dogs are considered the common host of this pathogen (Atif, 2015). Instead, in the rest of the localities, where the abundance and density of dogs are lower, so were the prevalence values found. In addition, the distribution of the three positive opossums, suggests that the localities with a greater abundance of dogs, also have an increased risk of infection by A. phagocytophilum.
Even though the small prevalence of A. phagocytophilum found in both opossums in the study area, it is evident that they can be infected. Those small frequencies of infection could be difficult to interpret, as the abundance that opossums can achieve in some regions is very high, therefore potentially increasing the chances of higher infection rates regionally. Because of the extremely high tick infestation rates we found, our data suggest that both opossums and dogs may favor tick populations regionally (Table 1, Figure 4). From 102 opossums, 25 had tick infestation rates greater than 25 ticks/individual. From this group, 9 were hosting 100 ticks or more (up to 215); that is an extremely high infestation rate considering the opossum's body size. This finding makes us wonder about their role in tick population dynamics and therefore their role in the ecology of tick-borne pathogens. In this sense, our data contradicts the assumption that “Virginia opossum kill the vast majority of ticks that attempt to feed on them” (Keesing et al., 2010).
The large dog abundance in the study area and the little health care they receive, place them as risk species in the transmission of the tick-borne pathogens for which they may be hosts. Due to the unusually high prevalence of A. phagocytophilum in dogs, they could even be considered reservoir hosts for this Ricketsial. In this context, the role of owned free-ranging dogs in the transmission of A. phagocytophilum becomes important because it is a pathogen that can infect a wide variety of vertebrates, including humans, and because they are an active species at the domestic-wildlife interface (Gompper, 2014). Furthermore, the study area is situated on The Yucatan Peninsula, which is a major stopover site for migratory birds, potentially connecting ticks and pathogen populations from North and Central America (Viana et al., 2016). Within this context, the ecology of A. phagocytophilum as a zoonotic pathogen, involve the diversity of local vertebrate hosts and human health through North and Central America. Further research is needed to determine which vertebrate species are involved in such dynamics; their host-competence; and which tick species from the high extent diversity are competent vectors of these and other tick-borne pathogens.
Understanding the dynamics of disease transmission in the human-domestic-wildlife interface has become a major priority in current ecological and epidemiological research. Under this approach, we found evidence of the presence of A. phagocytophilum in opossums and dogs in a rural area of The Yucatan Peninsula. Despite of the low prevalence of this pathogen in opossums, or null (for E. canis and E. chaffeensis), they could be indirectly involved in the dynamic transmission of tick-borne pathogens due to the high abundance of ticks that they presented. Males may have a major role in the transmission of those pathogens due to the greater average abundance of ticks in relation to the females. Other regional factors also appear to affect the abundance of ticks, such as livestock practices, which may favor it, or agriculture that may decrease it; and that eventually translates into a greater or lesser risk of infection. Regarding the high prevalence of A. phagocytophilum in dogs we found as well as previous reports in Mexico and in the world, they could be considered as a reservoir host in this region. This is a finding that cannot be overlooked in terms of public human and animal health, nor for future research with rickettsiosis in this and other Neotropical socio-ecosystems.
Author Contributions
ER-V and MW: study design, field work, and data analysis; ER-V and GG-P: laboratory analysis; ER-V, GG-P, and MW: funding and manuscript writing.
Conflict of Interest Statement
The authors declare that the research was conducted in the absence of any commercial or financial relationships that could be construed as a potential conflict of interest.
Acknowledgments
We thank Dr. Abel Gutiérrez and Carla Sánchez for support in DNA extraction; Tadeo Mateos and Irwin Te for field assistance; Jorge Gaona, Ethan Romero, and Carolina Jiménez for laboratory assistance; Alonso Castillo for facilitating access to his family ranch. Special thanks to Gabriela García Marmolejo for support with the maps. El Colegio de la Frontera Sur, CONACYT (M.Sc scholarship to the ER-V) and the Instituto Mexicano del Seguro Social IMSS provided financial support, FIS-IMSS G13-1192.
References
Anderson, B. E., Sumner, J. W., Dawson, J. E., Tzianabos, T., Greene, C. R., Olson, J. G., et al. (1992). Detection of the etiologic agent of human ehrlichiosis by polymerase chain reaction. J. Clin. Microbiol. 30, 775–780.
Atif, F. A. (2015). Anaplasma marginale and Anaplasma phagocytophilum: Rickettsiales pathogens of veterinary and public health significance. Parasitol. Res. 114, 3941–3957. doi: 10.1007/s00436-015-4698-2
Bayles, B. R., and Allan, B. F. (2014). Social-ecological factors determine spatial variation in human incidence of tick-borne ehrlichiosis. Epidemiol. Infect. 142, 1911–1924. doi: 10.1017/S0950268813002951
Beall, M. J., Alleman, A. R., Breitschwerdt, E. B., Cohn, L. A., Couto, C. G., Dryden, M. W., et al. (2012). Seroprevalence of Ehrlichia canis, Ehrlichia chaffeensis and Ehrlichia ewingii in dogs in North America. Parasit. Vectors 5, 2–11. doi: 10.1186/1756-3305-5-29
Bowman, D., Little, S. E., Lorentzen, L., Shields, J., Sullivan, M. P., and Carlin, E. P. (2009). Prevalence and geographic distribution of Dirofilaria immitis, Borrelia burgdorferi, Ehrlichia canis, and Anaplasma phagocytophilum in dogs in the United States: results of a national clinic-based serologic survey. Vet. Parasitol. 160, 138–148. doi: 10.1016/j.vetpar.2008.10.093
Brunner, J. L., and Ostfeld, R. S. (2008). Multiple causes of variable tick burdens on small–mammal hosts. Ecology 89, 2259–2272. doi: 10.1890/07-0665.1
Bush, A. O., Lafferty, K. D., Lotz, J. M., and Shostak, A. W. (1997). Parasitology meets ecology in its own terms: Margolis et al. revisited. J. Parasitol. 83, 575–583. doi: 10.2307/3284227
Çetinkaya, H., Matur, E., Akyazi, I., Ekiz, E. E., Aydin, L., and Toparlak, M. (2016). Serological and molecular investigation of Ehrlichia spp. and Anaplasma spp. in ticks and blood of dogs, in the Thrace región of Turkey. Ticks Tickborne Dis. 7, 706–714. doi: 10.1016/j.ttbdis.2016.02.021
Colchero, F., O'Farril, G., and Medellín, R. A. (2014). “Common opossum,” in Mammals of Mexico, ed G. Ceballos (Baltimore, MD: Johns Hopkins Univerity Press), 74–76.
Dahlgren, F. S., Mandel, E. J., Krebs, J. W., Massung, R. F., and McQuiston, J. H. (2011). Increasing incidence of Ehrlichia chaffeensis and Anaplasma phagocytophilum in the United States, 2000-2007. Am.J. Trop. Med. Hyg. 85, 124–131. doi: 10.4269/ajtmh.2011.10-0613
Daszak, P., Cunningham, A. A., and Hyatt, A. D. (2000). Emerging infectious diseases of wildlife Threats to biodiversity and human health. Science 287, 443–449. doi: 10.1126/science.287.5452.443
Dugan, V. G., Yabsley, M. J., Tate, C. M., Mead, D. G., Munderloh, U. G., Herron, M. J., et al. (2006). Evaluation of a prototype Anaplasma phagocytophilum surveillance system using white-tailed deer (Odocoileus virginianus) as natural sentinels. Vector Borne Zoonot. Dis. 6, 197–207. doi: 10.1089/vbz.2006.6.192
Estrada-Peña, A., and de la Fuente, J. (2014). The ecology of ticks and epidemiology of tick-Borne viral diseases. Antiviral Res. 108, 104–128. doi: 10.1016/j.antiviral.2014.05.016
Gompper, M. E. (2014). Free-Ranging Dogs and Wildlife Conservation, Vol. 1, 1st. Edn. Oxford: Oxford University Press.
Guerrero-Sánchez, S., and Weber, M. (2012). “Riesgo zoonótico y antropozoonótico en carnívoros silvestres peque-os y mediados en Calakmul, Campeche,” in El Huerto Familiar en el Sureste de México, ed R. Mariaca (Tabasco: Secretaría de Recursos Naturales y Protección Ambiental del estado de Tabasco; ECOSUR), 269–291.
Jensen, J., Simon, D., Murua-Escobar, H., Soller, J. T., Bullerdiek, J., Beelitz, P., et al. (2007). Anaplasma phagocytophilum in dogs in Germany. Zoonoses Public Health 54, 94–101. doi: 10.1111/j.1863-2378.2007.01028.x
Jones, K. E., Patel, N. G., Levy, M. A., Storeygard, A., Balk, D., Gittleman, J. L., et al. (2008). Global trends in emerging infectious diseases. Nature 451, 990–993. doi: 10.1038/nature06536
Keesing, F., Belden, L. K., Daszak, P., Dobson, A., Harvell, C. D., Holt, R. D., et al. (2010). Impacts of biodiversity on the emergence and transmission of infectious diseases. Nature 468, 647–652. doi: 10.1038/nature09575
Keesing, F., Hersh, M. H., Tibbetts, M., McHenry, D. J., Duerr, S., Brunner, J., et al. (2012). Reservoir competence of vertebrate hosts for Anaplasma phagocytophilum. Emerg. Infect. Dis. 18, 2013–2016. doi: 10.3201/eid1812.120919
King, D. A., Peckham, C., Waage, J. K., Brownlie, J., and Woolhouse, M. E. (2006). Infectious diseases: preparing for the future. Science 313, 1392–1393. doi: 10.1126/science.1129134
McQuiston, J. H., McCall, C. L., and Nicholson, W. L. (2003). Ehrlichiosis and related infections. Zoonosis Update 223, 1750–1756. doi: 10.2460/javma.2003.223.1750
Morens, D. M., Folkers, G. K., and Fauci, A. S. (2004). The challenge of emerging and reemerging infectious diseases. Nature 430, 242–249. doi: 10.1038/nature02759
Movilla, R., García, C., Siebert, S., and Roura, X. (2016). Countrywide serological evaluation of canine prevalence for Anaplasma spp., Borrelia burgdorferi (sense lato), Dirofilaria immitis and Ehrlichia canis in Mexico. Parasit. Vectors 9, 421–433. doi: 10.1186/s13071-016-1686-z
Noriega-Trejo, R., and Arteaga-Aguilar, M. (2010). “Ecosistemas continentales: Síntesis de los tipos de vegetación terrestre,” in La Biodiversidad en Campeche: Estudio de Estado, Villalobos–Zapata, eds G. J. Mendoza and J. Vaga (Campeche: CONABIO; UAC y ECOSUR), 148–155.
Ortega-Pacheco, A., Rodríguez-Buenfil, J. C., Bolio-González, M. E., Sauri-Arceo, C. H., Jimenez-Coello, M., Linde, C., et al. (2007). A survey of dog populations in urban and rural áreas of Yucatan, Mexico. Anthrozoos 20, 261–274. doi: 10.2752/089279307X224809
Paddock, C. D., and Childs, J. E. (2003). Ehrlichia chaffeensis: a prototypical emerging pathogen. Clin. Microbiol. Rev. 16, 37–64. doi: 10.1128/CMR.16.1.37-64.2003
Paddock, C. D., and Yabsley, M. J. (2007). Ecological Havoc, the rice of White-tailed deer, and the emergence of Amblyomma americanum-associated zoonoses in the United States. Curr. Top. Microbiol. Immunol. 315, 289–324. doi: 10.1007/978-3-540-70962-6_12
Pat-Nah, H., Rodriguez-Vivas, R. I., Bolio-Gonzalez, M. E., Villegas-Perez, S. L., and Reyes-Novelo, E. (2015). Molecular diagnosis of Ehrlichia canis in Dogs and Ticks Rhipicephalus sanguineus (Acari: Ixodidae) in Yucatan, Mexico. J. Med. Entomol. 52, 101–104. doi: 10.1093/jme/tju010
Perez, M., Rikihisa, Y., and Wen, B. (1996). Ehrlichia canis-like agent isolated from a man in Venezuela: antigenic and genetic characterization. J. Clin. Microbiol. 34, 2133–2139.
Pfäffle, M., Littwin, N., Muders, S. V., and y Petney, T. N. (2013). The ecology of tick-borne diseases. Int. J. Parasitol. 43, 12–13. doi: 10.1016/j.ijpara.2013.06.009
Ruiz-Fons, F., Acevedo, P., Sobrino, R., Vicente, J., Fierro, Y., and Fernández–de–Mera, I. G. (2013). Sex-biased differences in the effects of host individual, host population and environmental traits driving tick parasitism in red deer. Front. Cell. Infect. Microbiol. 3:23. doi: 10.3389/fcimb.2013.00023
Sainz, Á., Roura, X., Miró, G., Estrada-Peña, A., Kohn, B., Harrus, S., et al. (2015). Guideline for veterinary practitioners on canine Ehrlichiosis and Anaplasmosis in Europe. Parasit. Vectors 8:75. doi: 10.1186/s13071-015-0649-0
Sosa-Gutiérrez, C. G., Vargas, M., Torres, J., and Gordillo-Pérez, G. (2014). Tick-borne rickettsial pathogens in rodents from Mexico. J. Biomed. Sci. Eng. 7, 884–889. doi: 10.4236/jbise.2014.711087
Sosa-Gutiérrez, C. G., Vargas-Sandova, M., Torres, J., and Gordillo-Pérez, G. (2016). Tick-borne rickettsial pathogens in questing ticks, removed from humans and animals in Mexico. J. Vet. Sci. 17, 353–360. doi: 10.4142/jvs.2016.17.3.353
Sunquist, M. E., Austad, S. N., and Sunquist, F. (1987). Movement patterns and home range in the common opossum (Didelphis marsupialis). J. Mammal. 68, 173–176. doi: 10.2307/1381069
Viana, D. S., Santamaría, L., and Figuerola, J. (2016). Migratory birds as global dispersal vectors. Trends Ecol. Evol. 31, 763–775. doi: 10.1016/j.tree.2016.07.005
Villalobos-Zapata, G. J., and Mendoza-Vega, J. (2010). La Biodiversidad en Campeche: Estudio de Estado, Campeche: Comisión Nacional para el Conocimiento y Uso de la Biodiversidad (Conabio). Gobierno del Estado de Campeche; Universidad Autónoma de Campeche; El Colegio de la Frontera Sur.
Weiss, R. A., and McMichael, A. J. (2004). Social and environmental risk factors in the emergence of infectious diseases Nature Medicine 10, 570–576. doi: 10.1038/nm1150
Woldehiwet, Z. (2010). The natural history of Anaplasma phagocytophilum. Vet. Parasitol. 167, 108–122. doi: 10.1016/j.vetpar.2009.09.013
Yabsley, M. J. (2010). Natural history of Ehrlichia chaffeensis: Vertebrate hosts and tick vectors from the United States and evidence for endemic transmission in other countries. Vet. Parasitol. 167, 136–148. doi: 10.1016/j.vetpar.2009.09.015
Keywords: domestic-wildlife interface, epidemiology, tick-borne pathogens, vector, PCR, Didelphis marsupialis, Didelphis virginiana, Canis lupus familiaris
Citation: Rojero-Vázquez E, Gordillo-Pérez G and Weber M (2017) Infection of Anaplasma phagocytophilum and Ehrlichia spp. in Opossums and Dogs in Campeche, Mexico: The Role of Tick Infestation. Front. Ecol. Evol. 5:161. doi: 10.3389/fevo.2017.00161
Received: 25 July 2017; Accepted: 28 November 2017;
Published: 21 December 2017.
Edited by:
Mauricio Casas-Martínez, Instituto Nacional de Salud Pública, MexicoReviewed by:
Will Ludington, University of California, Berkeley, United StatesClay Cressler, University of Nebraska Lincoln, United States
Copyright © 2017 Rojero-Vázquez, Gordillo-Pérez and Weber. This is an open-access article distributed under the terms of the Creative Commons Attribution License (CC BY). The use, distribution or reproduction in other forums is permitted, provided the original author(s) or licensor are credited and that the original publication in this journal is cited, in accordance with accepted academic practice. No use, distribution or reproduction is permitted which does not comply with these terms.
*Correspondence: Manuel Weber, mweber@ecosur.mx