- 1Department of Geosciences, Biogeology, University of Tübingen, Tübingen, Germany
- 2Senckenberg Research Centre for Human Evolution and Palaeoenvironment, University Tübingen, Tübingen, Germany
Megaherbivores fulfilled a number of important ecological functions in terrestrial ecosystems and behaved as ecological engineers since 300 million years until around 12,000 years ago. These essential ecological functions include opening vegetation cover, selective seed dispersal and nutrient recycling and spreading. Thanks to these effects, megaherbivores change the vegetation structure where they live, with cascading effects on smaller herbivores and also on climate. The late Pleistocene extinction strongly impacted the megaherbivores almost all over the world and led to the loss of these important ecological functions in terrestrial ecosystems. These functions were partially restored by agriculturist humans through an ecological replacement that occurred through an ecological shift within the species Homo sapiens. A better understanding of the differences and similarities between the ecological impacts of megaherbivores and those of agricultural humans should help to predict the future of terrestrial ecosystems.
Introduction
Nowadays, very large terrestrial herbivores with adult body weights larger than 1,000 kg (the so-called megaherbivores, in the sense of Owen-Smith, 1988) occur only in some tropical areas of the world, in Africa and Asia, and they are counting only a handful of species (Owen-Smith, 1988). Even if one considers the historical distribution of these megaherbivores, before the recent shrinkage of their distribution due to intensified encroachment of their habitat and active hunting by humans, these very large terrestrial herbivores had still a relatively restricted distribution on the continents before the invention of firearms. However, this restricted geographical and climatic distribution of terrestrial very large herbivores is an exception when one looks at the terrestrial biosphere during geological times. Since the successful colonization of emerged land by vertebrates and the achievement of terrestrial herbivory in tetrapods, about 300 millions years ago, megaherbivores have played a key role in the functioning of almost all types of terrestrial ecosystems (e.g., Doughty, 2017; Mondanaro et al., 2017). This was the case almost everywhere on emerged land (except for glaciated and desertic areas) until around 50,000 years ago. Since then, most of the megaherbivores have disappeared, the exact causes and scenarios are still debated but two main causes are recognized, climate change and human impact (e.g., Barnosky et al., 2004; Stuart, 2015). The chronological coincidence between megaherbivore extinctions and the arrival of modern humans in Australia, North and South America, as well as on large islands such as Madagascar and New Zealand tends to support the idea that humans have something to do with these extinctions (e.g., Bunzel-Drüke, 2000; Barnosky et al., 2004; Crowley, 2010; Holdaway et al., 2014; Perry et al., 2014; Saltré et al., 2016). However, the hypothesis of an overkill by human hunters is not the most favored scenario, but rather an additional predation pressure of human hunter-gatherers on some keystone species during a period of climatic instability that led to a collapse of the trophic system, with some species, especially the megaherbivores, declining so much that no ecological recovery was possible for them (e.g., Ripple and Van Valkenburgh, 2010; Bartlett et al., 2016; Araujo et al., 2017; Haynes, 2018).
In any case, this worldwide extinction led to a strong decrease of mean body mass of terrestrial mammals, almost symmetrical to the increase seen at the beginning of the Cenozoic (Smith et al., 2016b), only much faster, occurring in a few thousand years instead of a few million years. The ecological consequences of these extinctions are increasingly investigated (e.g., Bunzel-Drüke et al., 2001; Catling, 2001; Putshkov, 2003; Brault et al., 2013; McClure, 2013; Gill, 2014; Bakker et al., 2016; Doughty et al., 2016a). After the extinction of most terrestrial megaherbivores around 12,000 years ago (Stuart, 2015), various human populations developed independently in different parts of the world a new way of subsistence by producing their food items instead of collecting them from the surrounding environment (Crawford, 2009). An important aspect of this occurrence of this “Neolithic revolution” is to understand if there is only a chronological coincidence with the extinction of megaherbivores or if both events are causally related. This “neolithic revolution” and the subsequent historical developments had dramatic ecological consequences that have been extensively described (e.g., Köhler-Rollefson, 1988; Butzer and Butzer, 1995; Blondel, 2006; Boivin et al., 2016). In contrast, little consideration has been given to the similarity between the ecological impact of the terrestrial megaherbivores and that of the human agriculturists, raising the possibility of an ecological replacement between both biological entities (Doughty, 2010).
Megaherbivores in the Geological History of Terrestrial Ecosystems
Megaherbivores have been present in terrestrial ecosystems since the early Permian, around 300 Million years ago (Benton, 1979). The taxonomic composition of the megaherbivore guild has changed through time. It was first occupied by primitive reptiles and mammal-like reptiles from the late Palaeozoic until the early Triassic about 200 million years ago, when these groups were progressively replaced by sauropsids, i.e., reptiles of the dinosaur lineage (e.g., Crompton and Attridge, 1986; Weishampel and Norman, 1989). These replacements took place either progressively or abruptly. They coincided roughly with changes in the dominant plant groups, or with mass extinction events, such as the Permian-Triassic and the Triassic-Jurassic boundaries. The taxonomic replacements in the megaherbivore groups seem to have occurred following two mechanisms. In some cases, new megaherbivore groups outcompeted previously dominant groups, while in other cases, the extinction of previously dominant megaherbivore taxa left an ecological vacuum that was filled by the evolution of a new taxonomic group that did not belong originally to the megaherbivores (e.g., Benton, 1979, 1996). Since the late Triassic, all species of megaherbivores belonged to the dinosaurs, until they became eradicated during the Cretaceous-Paleogene mass extinction event, around 66 million years ago (Weishampel and Norman, 1989). This is the best-known example of megaherbivore replacement that led to the global extinction of all late Cretaceous megaherbivores (dinosaurs) and the radiation of large herbivorous mammals that derived from much smaller species (e.g., von Koenigswald et al., 1987; Halliday and Goswami, 2016). This replacement took place through the extinction of the whole megaherbivore guild followed by the evolution of new taxa from other guilds that filled the ecological gap (Smith et al., 2010a). Therefore, there are several occurrences of taxonomic replacements among the megaherbivores, the previously dominant group of megaherbivores usually left its niche to representatives of another tetrapod group.
During the last large-scale taxonomic replacement among the megaherbivore guild that occurred 66 million years ago, it took about 10 million years after the global extinction of non-avian dinosaurs for some mammals to reach body weights of megaherbivores, more or less simultaneously on all continents (Smith et al., 2010a). During Cenozoic times, there was always at least one species of megaherbivore on each continent and in all climatic conditions, as well as during the Pleistocene outside the range of modern humans Homo sapiens (Schüle, 1992). The taxonomic composition of the megaherbivore guild during the Cenozoic was dynamic, with migrations, extinctions and replacements of some groups by others (Putshkov, 2001). However, except for short periods of time following mass extinction events, terrestrial ecosystems have always included a megaherbivore guild since 300 million years.
Ecological Functions of Megaherbivores
Megaherbivores are not only larger versions of their smaller relatives, their large size allows them to perform different ecological functions that are poorly performed by smaller herbivores (e.g., Fritz, 1997; Owen-Smith, 2013), even when these smaller herbivores are present in equivalent biomass as the megaherbivores (Owen-Smith, 1988). The ecological effects of megaherbivores can be observed in the modern ecosystems where they still survive, as well as by monitoring the ecological changes in areas where they were recently extirpated, essentially in African and southern Asian forests and savannas (e.g., Cowling et al., 2009; Bernard et al., 2014; Cromsigt and Beest, 2014; Asner et al., 2016). They behave as ecosystem engineers, thanks to their large size and strength. Their main ecological functions include changing the structure of vegetation through feeding behavior, destructive power and seed dispersal, impacting on the recycling and spread of nutrients, as well as impacting on the climate (e.g., Schüle, 1992; Doughty et al., 2013, 2016a,b; Bernard et al., 2014; Cromsigt and Beest, 2014; Asner et al., 2016; Malhi et al., 2016; Doughty, 2017).
One of the most spectacular effects of megaherbivores is to promote open landscapes at the expense of forested ones (e.g., Bakker et al., 2016; Doughty et al., 2016a), in a range of climatic conditions that represents around 30% of the current Earth surface (Bond, 2005). This impact reduces carbon storage in wood but increases carbon storage in underground organs of plants and also increases the amount of plant biomass suitable for herbivore consumption (Retallack, 2001, 2013). Instead of being trapped in woody plant tissues for decades or centuries, as it is the case in forested ecosystems, organic carbon in the form of grass blades, seeds and underground storage organs can be easily consumed by different kinds of herbivores (e.g., Dominy et al., 2008), and the CO2 incorporated by the plants is rapidly recycled as CO2 in the atmosphere, with an impact on the greenhouse gas content and the temperature. On the other hand, a significant amount of carbon is stored in the biomass of the megaherbivores themselves, which are organisms with long lifespans and essentially beyond the reach of animal predators (Owen-Smith, 1989).
In addition, megaherbivores promote the dispersal of plants with very large seeds and fleshy fruits compared to frugivores of smaller size (e.g., Janzen and Martin, 1982; Guix, 2009; Beaune et al., 2013; Corlett, 2013). Moreover, they disperse more seeds and on larger distances than smaller frugivores, leading to changes in the structure of forested ecosystems (e.g., Campos-Arceiz and Blake, 2011; Bueno et al., 2013; Bunney et al., 2017). Therefore, they have a significant impact on the species composition of the ecosystems where they live (e.g., Bernard et al., 2014), with consequences on the carbon storage by the plant communities (e.g., Bello et al., 2015).
Megaherbivores recycle and spread nutrients far away from nutrients hotspots and counteract the effects of gravity that tend to move nutrients from high areas where erosion takes place to low altitude areas and ultimately to oceanic basins (Doughty et al., 2013, 2016b). In the absence of megaherbivores, large trees tend to trap for long periods in unpalatable woody tissues precious nutrients that are removed from the nutrient cycles (Owen-Smith, 1988). As a consequence, these nutrients are not any more available for the growth of other plants, which are more accessible for small and large herbivores. Therefore, megaherbivores promote a higher biodiversity where they are present (Owen-Smith, 1988; Catling, 2001).
Indirectly, megaherbivores have an impact on climate through the changes they produce on the landscape and vegetation cover, modifying the albedo (e.g., Doughty et al., 2010) and the local humidity level (e.g., Brault et al., 2013), as well as through indirect changes in carbon storage by plants (Doughty et al., 2016c). Moreover, emissions of methane by megaherbivores may have had an effect on greenhouse gas concentration in the atmosphere and therefore on climate (Smith et al., 2010b, 2016a; Brook and Severinghaus, 2011).
Ecological Functions of Agricultural Humans
The start of food production by humans coinciding with the adoption of agriculture was a major step in the expansion of the anthroposphere, leading to a qualitative and quantitative increase of environmental changes due to human activities. When human populations shifted their subsistence strategy from foraging to producing their own food resources using essentially domesticated plant and animal species under their control, they also changed dramatically the ecological functions the human species had on the ecosystems they belonged to Owen-Smith (1987), Boivin et al. (2016). These changes are quite similar to those attributed to megaherbivores (Table 1).
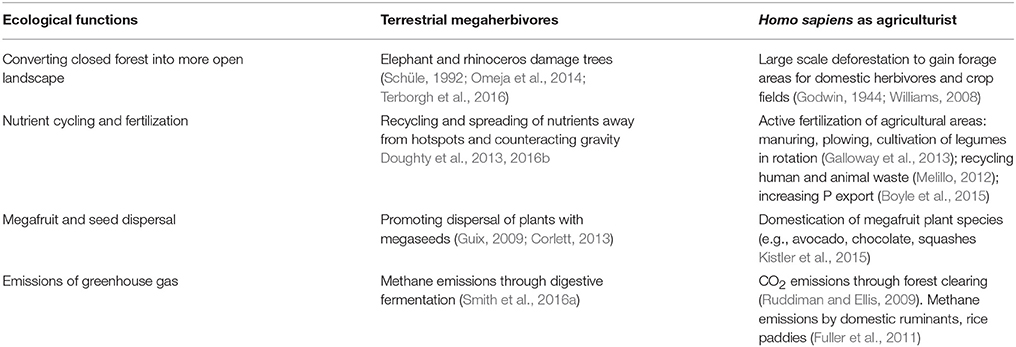
Table 1. Main ecological functions fulfilled by terrestrial megaherbivores and subsequently by humans as agriculturists.
To provide enough territories for allowing their crop plants to grow and their livestock to feed, agriculturalist humans have cleared originally forested areas (e.g., Godwin, 1944; Iversen, 1956; Williams, 2000, 2008; Smith et al., 2010c; Alenius et al., 2013), in many cases with the help of fire (e.g., Iversen, 1956; Bennett et al., 1990; Moore, 2000). Improving the recycling of soil nutrients through plowing or adding animal organic waste (manuring) modified the local nutrient balance to increase the productivity and yield of the planted crops (e.g., Bogaard, 2005; Bogaard et al., 2013; Lauer et al., 2014).
In some cases, humans have limited the negative impact of the loss of megaherbivores for the plant species producing very large fruits and they behaved as a partial replacement of large fruits dispersers (Guimarães et al., 2008). For plant species such as gourds and squashes, chocolate and avocado that became cultivated by humans, changing fruit and seed disperser led probably to an increase of the range of the species (Landon, 2009; Kistler et al., 2015).
Moreover, early agricultural humans contributed to CO2 emissions through forest clearance (Ruddiman and Ellis, 2009) and to methane emissions through the expansion of methane-producing livestock and rice paddies (Fuller et al., 2011), increasing the amount of this powerful greenhouse gas in the atmosphere and therefore contributing to climate warming (Ruddiman, 2003). These effects mimic those of megaherbivores respiring and fermenting large amounts of vegetation that also contributed to the the greenhouse gas composition of the atmosphere (e.g., Smith et al., 2010b, 2016a).
The ecological functions fulfilled by agricultural humans that partially replaced the ecological role of megaherbivores were performed by the humans rather than through domestic herbivores. Livestock species are smaller than the extinct megaherbivores and their biomass until 1800 AD was much lower than the biomass of the megaherbivores before the end-Pleistocene megafauna extinction (Doughty, 2013). Their impact on nutrient recycling and dispersal is much more limited than for free ranging megaherbivores (Doughty et al., 2016b). The partial restoration of the ecological functions of the extinct megaherbivores was therefore essentially a consequence of the diversity of human impacts on their surrounding environment through the whole range of their activities.
In areas where climatic conditions were too extreme for human agriculture due to too cold temperatures (arctic and periarctic regions) or with too much or too little humidity (flooded and desertic regions), the ecological functions of megaherbivores were not restored by human activities. One of the most spectacular examples of the ecological consequences of this lack of ecological replacement is the disappearance of the mammoth steppe biome. This biome was a productive cold ecosystem and the largest terrestrial biome during the cold periods of the late Pleistocene, and it disappeared at the expense of low productivity biomes such as tundra and boreal forests that cover the periarctic regions today (Zimov et al., 2012).
The Transition Phase
The ecological shift of humans from foragers to agriculturalists (the so-called “neolithic revolution”) took place independently in different areas of the world at different times, but always after the functional extinction of the local megaherbivores (Doughty, 2010). The earliest evidence for agriculture dates to the Pleistocene-Holocene transition, in Southwest Asia, North China, Mexico and South America (Crawford, 2009). Therefore, the shift of the ecological niche of humans from omnivorous predators into omnivorous herbivores with megaherbivore-like ecological functionality occurred after the functional extinction of the megaherbivores. This ecological replacement did not take place through competitive exclusion but through the occupation of a niche left empty by the extinction of the previous occupants of this niche. An investigation of the timing of independent origin of agriculture in comparison with the timing and percentage of megaherbivore extinction in different regions of the world suggested that agriculture could develop only after the extinction of megaherbivores, removing the competitive pressure of the later and allowing the occupation of the empty ecological niche by agricultural humans (Doughty, 2010).
Interestingly, this extinction of the megaherbivores that seems to have been necessary for the change of the human ecological niche was, at least partially, caused by the impact of humans as they were still ecologically behaving as omnivorous predators with the ability to actively hunt and consume megaherbivores that were until then virtually immune from predation (e.g., Brook and Bowman, 2004; Sandom et al., 2014b; Bartlett et al., 2016). This predation pressure may have contributed significantly to the local decline of megaherbivore populations (e.g., Owen-Smith, 1999; Pushkina and Raia, 2008; Surovell and Waguespack, 2009; Drucker et al., 2015), even if climate change also played a role in fragmenting the range of megaherbivores and making them more vulnerable to human impact (e.g., Araujo et al., 2017; Haynes, 2018). Therefore, the same species, Homo sapiens, with two different ecological modes could (1) contribute to the extinction of a guild (megaherbivores) probably through predation pressure and then (2) occupy a large part of the ecological niche of the extinct guild by shifting its own ecological niche. In contrast to previous ecological replacements among megaherbivores during the geological times in terrestrial ecosystems, the last one did not involve the biological evolution of new species that takes millions of years, but rather a phenotypic and behavioral change of an already existing species and took only a few thousand years.
Some Perspectives for Future Research
So far, the ecological impact of megaherbivores as well as that of agricultural humans has been considered globally and in general terms. However, since megaherbivore species, despite their common biological features, belonged to different mammal groups, some diversity in their impacts is to be expected. Such diversity is already observed in modern ecosystems, where the ecological impacts of megaherbivores are not homogeneous. Indeed, the ecological effects are species-dependent and also differ according to the abiotic context, such as temperature, local precipitations and soil characteristics (e.g., Fritz and Loison, 2006). Even closely related species, such as African and Asian elephants, have different impacts on the rainforests they live in Terborgh et al. (2018). When considering extinct groups without any modern equivalent among the latest surviving megaherbivore species of the Late Pleistocene, such as giant rhinoceroses (e.g., Elasmotherium) in Asia, giant ground sloths (e.g., Megatherium, Scelidotherium, Paramylodon and many others) and mastodons (e.g., Mammut, Cuvieronius, Stegomastodon) in North and South America, giant meriungulates (e.g., Toxodon, Macrauchenia) in South America or giant wombats (e.g., Diprotodon) in Australia, the exact impact that they could have had on their respective ecosystems is still under evaluation (e.g., Gill, 2014; Bakker et al., 2016; Doughty et al., 2016c; Malhi et al., 2016). Their respective ecological impact would depend on many factors, such as their foraging habits, i.e., grazing, browsing, or mix-feeding (e.g., Owen-Smith, 1988), their digestive physiology (e.g., McDonald and Pelikan, 2006; Clauss et al., 2007), their dependence upon the availability of surface water (Owen-Smith, 1988), if they used to dig burrows or not (e.g., Dondas et al., 2009), and the extant of their mobility pattern (e.g., McDonald and Pelikan, 2006; Price et al., 2017). Therefore, further investigations of the palaeobiology of these extinct taxa, as individual taxa but also as a community, should be necessary to quantify more accurately the possible ecological effects in different contexts.
Some works are already providing interesting possible directions for future research. For instance, it is possible to quantify differences in vegetation openness and composition during previous interglacial periods in Europe. Investigations using different approaches, such as pollen analysis, beetle assemblages and carbon isotopic abundances in large mammal teeth, suggest more open conditions than during the early Holocene (e.g., Cheddadi et al., 2005; Pushkina et al., 2014; Sandom et al., 2014a). In a completely different context, the Pleistocene savannas from South America, more open vegetation covers have been predicted by modelization when megaherbivores are included rather than excluded (Doughty et al., 2016a). Such a prediction might be tested using carbon stable isotopes of large herbivores from the abundant fossil records from these regions (e.g., Domingo et al., 2012; Dantas et al., 2017).
In the same way that different megaherbivores under different abiotic conditions may have variable ecological impacts, it is expected that different agricultural human societies of the past, using different domestic species, using different agricultural practices and living under different climatic and soil conditions, would certainly have a variety of ecological impacts on their respective environments. Moreover, depending on the history of megaherbivore impact in the same area, the ecological consequences might be different. All these factors should be investigated to document the changes in terrestrial ecosystems from different key regions of the world across the transition from the ecological dominance of megaherbivores to the ecological dominance of agricultural humans to evaluate the respective roles of abiotic, biotic and anthropic factors in the different ways this transition took place and provide a test for the generalization of the model suggested here.
In areas where the transition to agriculture did not take place, it seems that the ecological functions previously performed by the megaherbivores were not restored, such as in the case of the mammoth steppe (Zimov et al., 1995; Zimov, 2005), or to a lesser degree for temperate deciduous forests Bunzel-Drüke et al., 2001; Borkowski, 2011. In such contexts a megafauna rewilding strategy could restore some ecological functions, especially increase the nutrient cycling and therefore productivity and possibly the climatic impact of vegetation structure and landscape changes (Kintisch, 2015).
Conclusion
Understanding the transition that the terrestrial biosphere has experienced during the last thousand years is essential to anticipate the current and future changes relevant for human societies. These transformations did not just start with the Neolithic revolution, but earlier, when most terrestrial biomes lost the ecological impact of their respective megaherbivores. The “natural” baseline for terrestrial ecosystems is not that of environments shortly before the adoption of agriculture by prehistoric societies, it is the time when megaherbivores were still around and contributed significantly in shaping the terrestrial biomes and impacted on the biotic and abiotic components of the Earth System.
Author Contributions
The author confirms being the sole contributor of this work and approved it for publication.
Conflict of Interest Statement
The author declares that the research was conducted in the absence of any commercial or financial relationships that could be construed as a potential conflict of interest.
References
Alenius, T., Mökkönen, T., and Lahelma, A. (2013). Early farming in the Northern Boreal Zone: reassessing the history of land use in Southeastern Finland through high-resolution pollen analysis. Geoarchaeology 28, 1–24. doi: 10.1002/gea.21428
Araujo, B. B., Oliveira-Santos, L. G. R., Lima-Ribeiro, M. S., Diniz-Filho, J. A. F., and Fernandez, F. A. (2017). Bigger kill than chill: the uneven roles of humans and climate on late Quaternary megafaunal extinctions. Quat. Int. 431, 216–222. doi: 10.1016/j.quaint.2015.10.045
Asner, G. P., Vaughn, N., Izak, P. J., and Levick, S. (2016). Ecosystem-scale effects of megafauna in African savannas. Ecography 39:240–252. doi: 10.1111/ecog.01640
Bakker, E. S., Gill, J. L., Johnson, C. N., Vera, F. W., Sandom, C. J., Asner, G. P., et al. (2016). Combining paleo-data and modern exclosure experiments to assess the impact of megafauna extinctions on woody vegetation. Proc. Natl. Acad. Sci. U.S.A. 113, 847–855. doi: 10.1073/pnas.1502545112
Barnosky, A. D., Koch, P. L., Feranec, R. S., Wing, S. L., and Shabel, A. B. (2004). Assessing the causes of Late Pleistocene extinctions on the continents. Science 306, 70–75. doi: 10.1126/science.1101476
Bartlett, L. J., Williams, D. R., Prescott, G. W., Balmford, A., Green, R. E., Eriksson, A., et al. (2016). Robustness despite uncertainty: regional climate data reveal the dominant role of humans in explaining global extinctions of Late Quaternary megafauna. Ecography 39, 152–161. doi: 10.1111/ecog.01566
Beaune, D., Fruth, B., Bollache, L., Hohmann, G., and Bretagnolle, F. (2013). Doom of the elephant-dependent trees in a Congo tropical forest. For. Ecol. Manage. 295, 109–117. doi: 10.1016/j.foreco.2012.12.041
Bello, C., Galetti, M., Pizo, M. A., Magnago, L. F., Rocha, M. F., Lima, R. A., et al. (2015). Defaunation affects carbon storage in tropical forests. Science Adv. 1:e1501105. doi: 10.1126/sciadv.1501105
Bennett, K. D., Simonson, W. D., and Peglar, S. M. (1990). Fire and man in post-glacial woodlands of eastern England. J. Archaeol. Sci. 17, 635–642. doi: 10.1016/0305-4403(90)90045-7
Benton, M. J. (1979). Ecological succession among Late Palaeozoic and Mesozoic tetrapods. Palaeogeogr. Palaeoclimatol. Palaeoecol. 26, 127–150. doi: 10.1016/0031-0182(79)90145-7
Benton, M. J. (1996). Testing the roles of competition and expansion in tetrapod evolution. Proc. R. Soc. Lond. B Biol. Sci. 263, 641–646. doi: 10.1098/rspb.1996.0096
Bernard, M., Booth, L., and Kielar, A. (2014). The effects of mega-herbivore extinctions on seed dispersal and community structure in an East African Savanna. Consilience 13, 312–326. doi: 10.7916/D8GM87ST
Blondel, J. (2006). The ‘design’ of Mediterranean landscapes: a millennial story of humans and ecological systems during the historic period. Hum. Ecol. 34, 713–729. doi: 10.1007/s10745-006-9030-4
Bogaard, A. (2005). “Garden agriculture” and the nature of early farming in Europe and the Near East. World Archaeol. 37, 177–196. doi: 10.1080/00438240500094572
Bogaard, A., Fraser, R., Heaton, T. H., Wallace, M., Vaiglova, P., Charles, M., et al. (2013). Crop manuring and intensive land management by Europe's first farmers. Proc. Natl. Acad. Sci. U.S.A. 110, 12589–12594. doi: 10.1073/pnas.1305918110
Boivin, N. L., Zeder, M. A., Fuller, D. Q., Crowther, A., Larson, G., Erlandson, J. M., et al. (2016). Ecological consequences of human niche construction: examining long-term anthropogenic shaping of global species distributions. Proc. Natl. Acad. Sci. U.S.A. 113, 6388–6396. doi: 10.1073/pnas.1525200113
Bond, W. J. (2005). Large parts of the world are brown or black: a different view on the “Green World” hypothesis. J. Veg. Sci. 16, 261–266. doi: 10.1111/j.1654-1103.2005.tb02364.x
Borkowski, J. (2011). What did European primeval forests under high herbivore pressure look like? Forest Res. Pap. 72, 183–190. doi: 10.2478/v10111-011-0018-5
Boyle, J. F., Chiverrell, R. C., Davies, H., and Alderson, D. M. (2015). An approach to modeling the impact of prehistoric farming on Holocene landscape phosphorus dynamics. Holocene 25, 203–214. doi: 10.1177/0959683614556381
Brault, M. O., Mysak, L. A., Matthews, H. D., and Simmons, C. T. (2013). Assessing the impact of late Pleistocene megafaunal extinctions on global vegetation and climate. Clim. Past 9:1761. doi: 10.5194/cp-9-1761-2013
Brook, B. W., and Bowman, D. M. J. S. (2004). The uncertain blitzkrieg of Pleistocene megafauna. J. Biogeogr. 31, 517–523. doi: 10.1046/j.1365-2699.2003.01028.x
Brook, E. J., and Severinghaus, J. P. (2011). Methane and megafauna. Nat. Geosci. 4, 271–272. doi: 10.1038/ngeo1140
Bueno, R. S., Guevara, R., Ribeiro, M. C., Culot, L., Bufalo, F. S., and Galetti, M. (2013). Functional redundancy and complementarities of seed dispersal by the last Neotropical megafrugivores. PLoS ONE 8:e56252. doi: 10.1371/journal.pone.0056252
Bunney, K., Bond, W. J., and Henley, M. (2017). Seed dispersal kernel of the largest surviving megaherbivore—the African savanna elephant. Biotropica 49, 395–401. doi: 10.1111/btp.12423
Bunzel-Drüke, M. (2000). “Artenschwund durch Eiszeitjäger?,” in Bayerische Landesanstalt für Wald und Forstwirtschaft: Großtiere als Landschaftsgestalter - Wunsch oder Wirklichkeit? Berichte aus der LWF, Nummer 27, August 2000, Freising, pp 6–20.
Bunzel-Drüke, M., Drüke, J., and Vierhaus, H. (2001). Der Einfluss von Großherbivoren auf die Naturlandschaft Mitteleuropas. Available online at: http://www.abu-naturschutz.de/downloads/finish/8-diverse-veroeffentlichungen/116-der-einfluss-von-grossherbivoren-auf-die-naturlandschaft-mitteleuropas.html
Butzer, K. W., and Butzer, E. K. (1995). “Transfer of the Mediterranean livestock economy to New Spain: adaptation and ecological consequences,” in Global Land Use Change: A Perspective from the Columbian Encounter, eds B. L. Turner II, A. G. Sal, F. G. Bernáldez, F. di Castri (Madrid: Consejo Superior de Investaciones Cientificas; DODECAEDRO), 151–193.
Campos-Arceiz, A., and Blake, S. (2011). Megagardeners of the forest–the role of elephants in seed dispersal. Acta Oecologica 37, 542–553. doi: 10.1016/j.actao.2011.01.014
Catling, P. M. (2001). Extinction and the importance of history and dependence in conservation. Biodiversity 2, 2–14. doi: 10.1080/14888386.2001.9712550
Cheddadi, R., de Beaulieu, J. L., Jouzel, J., Andrieu-Ponel, V., Laurent, J. M., Reille, M., et al. (2005). Similarity of vegetation dynamics during interglacial periods. Proc. Natl. Acad. Sci. U.S.A. 102, 13939–13943. doi: 10.1073/pnas.0501752102
Clauss, M., Jürgen Streich, W., Schwarm, A., Ortmann, S., and Hummel, J. (2007). The relationship of food intake and ingesta passage predicts feeding ecology in two different megaherbivore groups. Oikos 116, 209–216. doi: 10.1111/j.0030-1299.2007.15461.x
Corlett, R. T. (2013). The shifted baseline: prehistoric defaunation in the tropics and its consequences for biodiversity conservation. Biol. Conserv. 163, 13–21. doi: 10.1016/j.biocon.2012.11.012
Cowling, R. M., Kamineth, A., Difford, M., and Campbell, E. E. (2009). Contemporary and historical impacts of megaherbivores on the population structure of tree euphorbias in South African subtropical thicket. Afr. J. Ecol. 48, 135–145. doi: 10.1111/j.1365-2028.2009.01096.x
Crawford, G. W. (2009). Agricultural origins in North China pushed back to the Pleistocene–Holocene boundary. Proc. Natl. Acad. Sci. U.S.A. 106, 7271–7272. doi: 10.1073/pnas.0903375106
Crompton, A. W., and Attridge, J. (1986). “Masticatory apparatus of the larger herbivores during Late Triassic and Early Jurassic times,” in The Beginning of the Age of Dinosaurs. Faunal Change Across Triassic-Jurassic Boundary, ed K. Padian (Cambridge: Cambridge University Press), 223–236.
Cromsigt, J. P., and Beest, M. (2014). Restoration of a megaherbivore: landscape-level impacts of white rhinoceros in Kruger National Park, South Africa. J. Ecol. 102, 566–575. doi: 10.1111/1365-2745.12218
Crowley, B. E. (2010). A refined chronology of prehistoric Madagascar and the demise of the megafauna. Quat. Sci. Rev. 29, 2591–2603. doi: 10.1016/j.quascirev.2010.06.030
Dantas, M., Cherkinsky, A., Bocherens, H., Drefahl, M., Bernardes, C., and França, L. M. (2017). Isotopic palaeoecology of the Pleistocene megamammals from the Brazilian Intertropical Region: feeding ecology (δ13C), niche breadth and overlap. Quat. Sci. Rev. 170, 152–163 doi: 10.1016/j.quascirev.2017.06.030
Domingo, L., Prado, J. L., and Alberdi, M. T. (2012). The effect of paleoecology and paleobiogeography on stable isotopes of Quaternary mammals from South America. Quat. Sci. Rev. 55, 103–113. doi: 10.1016/j.quascirev.2012.08.017
Dominy, N. J., Vogel, E. R., Yeakel, J. D., Constantino, P., and Lucas, P. W. (2008). Mechanical properties of plant underground storage organs and implications for dietary models of early hominins. Evol. Biol. 35, 159–175. doi: 10.1007/s11692-008-9026-7
Dondas, A., Isla, F. I., and Carballido, J. L. (2009). Paleocaves exhumed from the Miramar Formation (Ensenadan Stage-age, Pleistocene), Mar del Plata, Argentina. Quat. Int. 210, 44–50. doi: 10.1016/j.quaint.2009.07.001
Doughty, C. E. (2010). The development of agriculture in the Americas: an ecological perspective. Ecosphere 1, 1–11. doi: 10.1890/ES10-00098.1
Doughty, C. E. (2013). Preindustrial human impacts on global and regional environment. Annu. Rev. Environ. Resour. 38, 503–527. doi: 10.1146/annurev-environ-032012-095147
Doughty, C. E. (2017). Herbivores increase the global availability of nutrients over millions of years. Nat. Ecol. Evol. 1, 1820-1827. doi: 10.1038/s41559-017-0341-1
Doughty, C. E., Faurby, S., and Svenning, J. C. (2016a). The impact of the megafauna extinctions on savanna woody cover in South America. Ecography 39, 213–222. doi: 10.1111/ecog.01593
Doughty, C. E., Roman, J., Faurby, S., Wolf, A., Haque, A., Bakker, E. S., et al. (2016b). Global nutrient transport in a world of giants. Proc. Natl. Acad. Sci. U.S.A. 113, 868–873. doi: 10.1073/pnas.1502549112
Doughty, C. E., Wolf, A., and Field, C. B. (2010). Biophysical feedbacks between the Pleistocene megafauna extinction and climate: the first human-induced global warming? Geophys. Res. Lett. 37:L15703. doi: 10.1029/2010GL043985
Doughty, C. E., Wolf, A., and Malhi, Y. (2013). The legacy of the Pleistocene megafauna extinctions on nutrient availability in Amazonia. Nat. Geosci. 6, 761–764. doi: 10.1038/ngeo1895
Doughty, C. E., Wolf, A., Morueta-Holme, N., Jørgensen, P. M., Sandel, B., Biolle, C., et al. (2016c). Megafauna extinction, tree species range reduction, and carbon storage in Amazonian forests. Ecography 39, 194–203. doi: 10.1111/ecog.01587
Drucker, D. G., Vercoutère, C., Chiotti, L., Nespoulet, R., Crépin, L., Conard, N. J., et al. (2015). Tracking possible decline of woolly mammoth during the Gravettian in the Dordogne and the Swabian Jura using multi-isotope tracking (13C, 14C, 15N, 34S, 18O). Quat. Int. 359–360, 304–317. doi: 10.1016/j.quaint.2014.11.028
Fritz, H. (1997). Low ungulate biomass in west African savannas: primary production or missing megaherbivores or large predator species? Ecography 20, 417–421. doi: 10.1111/j.1600-0587.1997.tb00387.x
Fritz, H., and Loison, A. (2006). “Large herbivores across biomes,” in Large Herbivore Ecology, Ecosystems Dynamics and Conservation, eds K. Danell, R. Bergström, P. Duncan, and J. Pastor (Cambridge: Cambridge University Press), 19–49.
Fuller, D. Q., Van Etten, J., Manning, K., Castillo, C., Kingwell-Banham, E., Weisskopf, A., et al. (2011). The contribution of rice agriculture and livestock pastoralism to prehistoric methane levels: an archaeological assessment. Holocene 21, 743–759. doi: 10.1177/0959683611398052
Galloway, J. N., Leach, A. M., Bleeker, A., and Erisman, J. W. (2013). A chronology of human understanding of the nitrogen cycle. Phil. Trans. Royal Soc. B 368:20130120. doi: 10.1098/rstb.2013.0120
Gill, J. L. (2014). Ecological impacts of the late Quaternary megaherbivore extinctions. New Phytol. 201, 1163–1169. doi: 10.1111/nph.12576
Guimarães, P. R. Jr., Galetti, M., and Jordano, P. (2008). Seed dispersal anachronisms: rethinking the fruits extinct megafauna ate. PLoS ONE 3:e1745. doi: 10.1371/journal.pone.0001745
Halliday, T. J. D., and Goswami, A. (2016). Eutherian morphological disparity across the end-Cretaceous mass extinction. Biol. J. Linn. Soc. 118, 152–168. doi: 10.1111/bij.12731
Haynes, G. (2018). “The evidence for human agency in the Late Pleistocene megafaunal extinctions,” in Encyclopedia of the Anthropocene, eds D. DellaSala and M. Goldstein (Amsterdam: Elsevier), 219–226.
Holdaway, R. N., Allentoft, M. E., Jacomb, C., Oskam, C. L., Beavan, N. R., and Bunce, M. (2014). An extremely low-density human population exterminated New Zealand moa. Nat. Commun. 5:5436. doi: 10.1038/ncomms6436
Iversen, J. (1956). Forest clearance in the Stone Age. Sci. Am., 194, 36–41. doi: 10.1038/scientificamerican0356-36
Janzen, D. H., and Martin, P. S. (1982). Neotropical anachronisms: the fruits the gomphotheres ate. Science 215, 19–27. doi: 10.1126/science.215.4528.19
Kistler, L., Newsom, L. A., Ryan, T. M., Clarke, A. C., Smith, B. D., and Perry, G. H. (2015). Gourds and squashes (Cucurbita spp.) adapted to megafaunal extinction and ecological anachronism through domestication. Proc. Natl. Acad. Sci. U.S.A. 112, 15107–15112. doi: 10.1073/pnas.1516109112
von Koenigswald, W., Rensberger, J. M., and Pretzschner, H. U. (1987). Changes in the tooth enamel of early Paleocene mammals allowing increased diet diversity. Nature 328, 150–152. doi: 10.1038/328150a0
Köhler-Rollefson, I. (1988). The aftermath of the Levantine Neolithic revolution in the light of ecological and ethnographic evidence. Paléorient 14, 87–93. doi: 10.3406/paleo.1988.4442
Landon, A. J. (2009). Domestication and Significance of Persea americana, the Avocado, in Mesoamerica. Nebraska Anthropol. 47, 62–79.
Lauer, F., Prost, K., Gerlach, R., Pätzold, S., Wolf, M., Urmersbach, S., et al. (2014). Organic fertilization and sufficient nutrient status in prehistoric agriculture? – indications from multi-proxy analyses of archaeological topsoil relicts. PLoS ONE 9:e106244. doi: 10.1371/journal.pone.0106244
Malhi, Y., Doughty, C. E., Galetti, M., Smith, F. A., Svenning, J. C., and Terborgh, J. W. (2016). Megafauna and ecosystem function from the Pleistocene to the Anthropocene. Proc. Natl Acad. Sci. U.S.A. 113, 838–846. doi: 10.1073/pnas.1502540113
McClure, S. B. (2013). Domesticated animals and biodiversity: early agriculture at the gates of Europe and long-term ecological consequences. Anthropocene 4, 57–68. doi: 10.1016/j.ancene.2013.11.001
McDonald, H. G., and Pelikan, S. (2006). Mammoths and mylodonts: exotic species from two different continents in North American Pleistocene faunas. Quat. Int. 142, 229–241. doi: 10.1016/j.quaint.2005.03.020
Melillo, E. D. (2012). The first green revolution: debt peonage and the making of the nitrogen fertilizer trade, 1840–1930. Am. Hist. Rev. 117, 1028–1060. doi: 10.1093/ahr/117.4.1028
Mondanaro, A., Castiglione, S., Melchionna, M., Di Febbraro, M., Vitagliano, G., Serio, C., et al. (2017). Living with the elephant in the room: top-down control in Eurasian large mammal diversity over the last 22 million years. Palaeogeogr. Palaeoclimatol. Palaeoecol. 485, 956–962. doi: 10.1016/j.palaeo.2017.08.021
Moore, J. (2000). Forest fire and human interaction in the early Holocene woodlands of Britain. Palaeogeogr. Palaeoclimatol. Palaeoecol. 164, 125–137. doi: 10.1016/S0031-0182(00)00180-2
Omeja, P. A., Jacob, A. L., Lawes, M., Lwanga, J. S., Rothman, J. M., Tumwesigye, C., et al. (2014). Changes in elephant abundance affect forest composition or regeneration? Biotropica 46, 704–711. doi: 10.1111/btp.12154
Owen-Smith, N. (1989). “The megaherbivore syndrome: alternative life style or different time frame?” in Alternative Life-History Styles of Animals, ed M. N. Bruton (Dordrecht: Kluwer Academic Publishers), 441–457.
Owen-Smith, N. (1999). “The interaction of humans, megaherbivores, and habitats in the late Pleistocene extinction event,” in Extinctions in Near Time, ed R. D. E. MacPhee (New York, NY; Boston, MA; Dordrecht; London; Moscow: Kluwer Academic; Plenum Publishers), 57–69.
Owen-Smith, R. N. (1987). Pleistocene extinctions: the pivotal role of megaherbivores. Paleobiology 13, 351–362. doi: 10.1017/S0094837300008927
Owen-Smith, R. N. (1988). Megaherbivores: The Influence of Very Large Body Size on Ecology. Cambridge: Cambridge University Press.
Owen-Smith, R. N. (2013). “Megaherbivores,” in Encyclopedia of Biodiversity, ed S. A. Levin (Amsterdam: Elsevier), 223–239.
Perry, G. L. W., Wheeler, A. B., Wood, J. R., and Wilmshurt, J. M. (2014). A high-precision chronology for the rapid extinction of New Zealand moa (Aves, Dinornithiformes). Quat. Sci. Rev. 105, 126–135. doi: 10.1016/j.quascirev.2014.09.025
Price, G. J., Ferguson, K. J., Webb, G. E., Feng, Y. x., Higgins, P., Nguyen, A. D., et al. (2017). Seasonal migration of marsupial megafauna in Pleistocene Sahul (Australia–New Guinea). Proc. Royal Soc. B 284:20170785. doi: 10.1098/rspb.2017.0785
Pushkina, D., Bocherens, H., and Ziegler, R. (2014). Unexpected palaeoecological features of the middle and late Pleistocene large herbivores in south-western Germany revealed by stable isotopic abundances in tooth enamel. Quat. Int. 339-340, 164–178. doi: 10.1016/j.quaint.2013.12.033
Pushkina, D., and Raia, P. (2008). Human influence on distribution and extinctions of the late Pleistocene Eurasian megafauna. J. Hum. Evol. 54, 769–782. doi: 10.1016/j.jhevol.2007.09.024
Putshkov, P. V. (2001). “Proboscidean agent of some Tertiary megafaunal extinctions,” in World of Elephants. Proceedings of the 1st International Congress, eds G. Cavarreta, P. Giola, M. Mussi, M. R. Palombo (Rome), 133–136.
Putshkov, P. V. (2003). The impact of mammoths on their biome: clash of two paradigms. Deinsea 9, 365–380.
Retallack, G. J. (2001). Cenozoic expansion of grasslands and climatic cooling. J. Geol. 109, 407–426. doi: 10.1086/320791
Retallack, G. J. (2013). Global cooling by grassland soils of the geological past and near future. Annu. Rev. Earth Planet. Sci. 41, 69–86. doi: 10.1146/annurev-earth-050212-124001
Ripple, W. J., and Van Valkenburgh, B. (2010). Linking top-down forces to the Pleistocene megafaunal extinctions. Bioscience 60, 516–526. doi: 10.1525/bio.2010.60.7.7
Ruddiman, W. F. (2003). The anthropogenic greenhouse era began thousands of years ago. Clim. Change 61, 261–293. doi: 10.1023/B:CLIM.0000004577.17928.fa
Ruddiman, W. F., and Ellis, E. C. (2009). Effect of per-capita land use changes on Holocene forest clearance and CO2 emissions. Quat. Sci. Rev. 28, 3011–3015. doi: 10.1016/j.quascirev.2009.05.022
Saltré, F., Rodríguez-Rey, M., Brook, B. W., Johnson, C. N., Turney, C. S., Alroy, J., et al. (2016). Climate change not to blame for late Quaternary megafauna extinctions in Australia. Nat. Commun. 7:10511. doi: 10.1038/ncomms10511
Sandom, C., Ejrnæs, R., Hansen, M. D., and Svenning, J. C. (2014a). High herbivore density associated with vegetation diversity in interglacial ecosystems. Proc. Natl. Acad. Sci. U.S.A 111, 4162–4167. doi: 10.1073/pnas.1311014111
Sandom, C., Faurby, S., Sandel, B., and Svenning, J. C. (2014b). Global late Quaternary megafauna extinctions linked to humans, not climate change. Proc. R. Soc. B 281:20133254. doi: 10.1098/rspb.2013.3254
Schüle, W. (1992). “Vegetation, Megaherbivores, Man and Climate in the Quaternary and the Genesis of Closed Forests,” in Tropical forests in transition, eds J. G. Goldhammer (Basel: Birkhäuser Verlag), 45-76.
Smith, F. A., Boyer, A. G., Brown, J. H., Costa, D. P., Dayan, T., Ernest, S. K., et al. (2010a). The evolution of maximum body size of terrestrial mammals. Science 330, 1216–1219. doi: 10.1126/science.1194830
Smith, F. A., Hammond, J. I., Balk, M. A., Elliott, S. M., Lyons, S. K., Pardi, M. I., et al. (2016a). Exploring the influence of ancient and historic megaherbivore extirpations on the global methane budget. Proc. Natl. Acad. Sci. U.S.A. 113, 874–879. doi: 10.1073/pnas.1502547112
Smith, F. A., Doughty, C. E., Malhi, Y., Svenning, J. C., and Terborgh, J. (2016b). Megafauna in the Earth system. Ecography 39, 99–108. doi: 10.1111/ecog.02156
Smith, F. A., Elliott, S. M., and Lyons, S. K. (2010b). Methane emissions from extinct megafauna. Nat. Geosci. 3, 374–375. doi: 10.1038/ngeo877
Smith, D., Whitehouse, N., Bunting, M. J., and Chapman, H. (2010c). Can we characterise ‘openness’ in the Holocene palaeoenvironmental record? Modern analogue studies of insect faunas and pollen spectra from Dunham Massey deer park and Epping Forest, England. Holocene 20, 215–229. doi: 10.1177/0959683610350392
Stuart, A. J. (2015). Late Quaternary megafaunal extinctions on the continents: a short review. Geol. J. 50, 338–363. doi: 10.1002/gj.2633
Surovell, T. A., and Waguespack, N. M. (2009). “Human prey choice in the Late Pleistocene and its relation to megafaunal extinctions,” in American Megafaunal Extinctions at the End of the Pleistocene, ed G. Haynes (Springer), 77–105.
Terborgh, J., Davenport, L. C., Niangadouma, R., Dimoto, E., Mouandza, J. C., Scholtz, O., et al. (2016). Megafaunal influences on tree recruitment in African equatorial forests. Ecography 39, 180–186. doi: 10.1111/ecog.01641
Terborgh, J., Davenport, L. C., Ong, L., and Campos-Arceiz, A. (2018). Foraging impacts of Asian megafauna on tropical rain forest structure and biodiversity. Biotropica 50, 84–89. doi: 10.1111/btp.12488
Weishampel, D. B., and Norman, D. B. (1989). Vertebrate herbivory in the Mesozoic; Jaws, plants, and evolutionary metrics. Geol. Soc. Am. Spec. Paper 238, 87–101. doi: 10.1130/SPE238-p87
Williams, M. (2000). Dark ages and dark areas: global deforestation in the deep past. J. Hist. Geogr. 26, 28–46. doi: 10.1006/jhge.1999.0189
Williams, M. (2008). A new look at global forest histories of land clearing. Annu. Rev. Environ. Ressour. 33, 345–367. doi: 10.1146/annurev.environ.33.040307.093859
Zimov, S. A. (2005). Pleistocene park: return of the mammoth's ecosystem. Science 308, 796–798. doi: 10.1126/science.1113442
Zimov, S. A., Chuprynin, V. I., Oreshko, A. P., Chapin, I. I. I., F. S., Chapin, M. C., and Reynolds, J. F. (1995). “Effects of mammals on ecosystem change at the Pleistocene-Holocene boundary,” in Arctic and Alpine Biodiversity: Patterns, Causes and Ecosystem Consequences (Berlin; Heidelberg: Springer Verlag), 127–135.
Keywords: megaherbivores, terrestrial biomes, neolithic, ecological replacement, extinction
Citation: Bocherens H (2018) The Rise of the Anthroposphere since 50,000 Years: An Ecological Replacement of Megaherbivores by Humans in Terrestrial Ecosystems? Front. Ecol. Evol. 6:3. doi: 10.3389/fevo.2018.00003
Received: 15 September 2017; Accepted: 09 January 2018;
Published: 24 January 2018.
Edited by:
Florent Rivals, Institució Catalana de Recerca i Estudis Avançats (ICREA), SpainReviewed by:
Chris J. Caseldine, University of Exeter, United KingdomFerran Estebaranz-Sánchez, University of Barcelona, Spain
Copyright © 2018 Bocherens. This is an open-access article distributed under the terms of the Creative Commons Attribution License (CC BY). The use, distribution or reproduction in other forums is permitted, provided the original author(s) or licensor are credited and that the original publication in this journal is cited, in accordance with accepted academic practice. No use, distribution or reproduction is permitted which does not comply with these terms.
*Correspondence: Hervé Bocherens, herve.bocherens@uni-tuebingen.de