- Fundación Miguel Lillo and Consejo Nacional de Investigaciones Científicas y Técnicas, Unidad Ejecutora Lillo, San Miguel de Tucumán, Argentina
In seed-dispersal mutualisms, the number of fruit a plant displays is a key trait, as it acts as a signal for seed dispersers that entails fruit removal and exportation of reproductive units (fruit crop size hypothesis). Although this hypothesis has gained general acceptance, forces driving the shape and strength of natural selection exerted by birds on fruit crop size remains an unresolved matter. Here, we propose that ecological filters promoting high functional equivalence of interacting partners (similar functional roles) translate into similar selection pressures on fruit crop size, enhancing selection strength on this trait. We performed a meta-analysis on 50 seed-dispersal systems to test the hypothesis that frugivorous birds exert positive selection pressure on fruit crop size, and to assess whether different factors expected to act as filters (fruit diameter, fruit type, fruiting season length, bird functional groups, and latitude) influence phenotypic selection regimes on this trait. Birds promote larger fruit crop sizes as a general pattern in nature. Short fruiting seasons and a high proportion of species belonging to the same functional group showed higher selection strength on fruit crop size. Also, selection strength on fruit crop size increased for large-fruited species and toward the tropics. Our results support the hypothesis that fruit crop size represents a conspicuous signal advertising the amount of reward to visually driven interacting partners, and that both plant and bird traits, as well as environmental factors, drive selection strength on fruit display traits. Furthermore, our results suggest that the relationship among forces impinged by phenology and frugivore functional roles may be key to understand their evolutionary stability.
Introduction
A major challenge in evolutionary ecology is to understand how mutualistic interactions between plants and animals drive the evolution of plant phenotype (Strauss and Irwin, 2004). Among plant traits involved in mutualistic interactions, the fruits that plants display are a major target of natural selection. Generally, these structures advertise the amount of reward (for example, the larger the fruit, the higher the reward) to their interacting partners (Snow, 1971; Schaefer and Ruxton, 2011). Under this scenario of signal-reward correlation (Benitez-Vieyra et al., 2010), the expected outcome is directional selection on the number of fruit (Snow, 1971; Carr, 1992; Ortiz-Pulido and Rico-Gray, 2000), as well as other fruit and seed traits (e.g., size, color, water and nutrient content, coat thickness; Jordano, 2000; Herrera, 2002). However, this selection regime may be modified by different factors, such as the costs of fruit production or selection pressures imposed by antagonistic interactors (Jordano, 1987; Parciak, 2002; Russo, 2003; Burns, 2015; Muñoz et al., 2017). In this sense, the underlying mechanism of the evolution of repeated plant structures (leaves, flowers, and fruits) is still poorly understood (Herrera, 2009, 2017).
In seed dispersal mutualisms, frugivores act as natural selection agents on different fruit display traits (e.g., fruit and seed size, nutrient content, color), including the number of ripe fruits (i.e., fruit crop size; Jordano, 1995; Sobral et al., 2010; Palacio et al., 2017a). Indeed, fruit crop size has been advocated as the principal target of bird-mediated selection (Snow, 1971; Howe and Estabrook, 1977). In his seminal work, David Snow proposed that plants offering a relatively high number of fruit should increase fruit removal (the “fruit crop size hypothesis”; Snow, 1971; McKey, 1975; Howe and Estabrook, 1977). Several surrogates for fruit removal have been used to test this hypothesis, mainly the number of fruit removed, the proportion of fruit removed and bird visitation rate (Davidar and Morton, 1986; Foster, 1990; Ortiz-Pulido and Rico-Gray, 2000). The fruit crop size hypothesis also assumes that fruit removal bears a positive relationship with effective seed dispersal away from the mother plant (Howe and Estabrook, 1977). Although there is field experimental evidence supporting this hypothesis (Christensen et al., 1991; Ortiz-Pulido et al., 2007), evidence from field studies have had mixed results. For instance, in a qualitative review, Carr (1992) found positive linear relationships between fruit crop size and removal (number of fruit removed) in 18 out of 19 temperate and tropical seed dispersal systems. By contrast, only 3 out of 19 seed dispersal systems showed a positive relationship between fruit crop size and removal (proportion of fruit removed), whereas no relationships in the remaining 16 were found. Further, the shape and intensity of bird-mediated selection on fruit crop size may vary within plant populations in different selection episodes. Foster (1990), for instance, found a positive relationship between fruit crop size and bird visitation in 1 year, but found no relationship in previous years in a population of Allophylus edulis. Similarly, Ortiz-Pulido and Rico-Gray (2000) found that the covariation between fruit crop size and bird visitation varied drastically from non-significant to positive in a population of Bursera fagaroides. These studies have proposed multiple ecological factors to explain fruit removal by birds and their potential evolutionary consequences on seed dispersal (see also Jordano, 2000; Herrera, 2002 for reviews). However, there has not been as yet a quantitative review of the relationship between fruit crop size and fruit removal, nor of the drivers of variation in selection regimes on fruit crop size to disentangle their underlying mechanisms.
As we see it, factors characterizing seed dispersal systems (Snow, 1971; Howe and Estabrook, 1977) that will drive the shape and intensity of selection on fruit crop size fall into three categories: (1) plant traits, such as plant size, fruit size, pulp and nutrient content, and fruiting phenology (Wheelwright, 1993; Burns, 2004, 2005; Sobral et al., 2010; Palacio et al., 2014); (2) bird assemblage traits, related to the functional role of frugivores (Galetti et al., 2013; Morales et al., 2013; Dehling et al., 2016; Palacio et al., 2017b), and (3) environmental or abiotic factors (Hampe, 2003; Márquez et al., 2004; Sobral et al., 2013). We hypothesized that the strength of selection on fruit crop size stems from ecological filtering by constraining certain bird species to interact with a plant population. Basically, ecological filters promoting high functional equivalence (i.e., species with similar roles for the interaction) would translate into similar selection pressures on fruit display traits (Zamora, 2000; Strauss and Irwin, 2004; hereafter “functional equivalence hypothesis”; Figure 1).
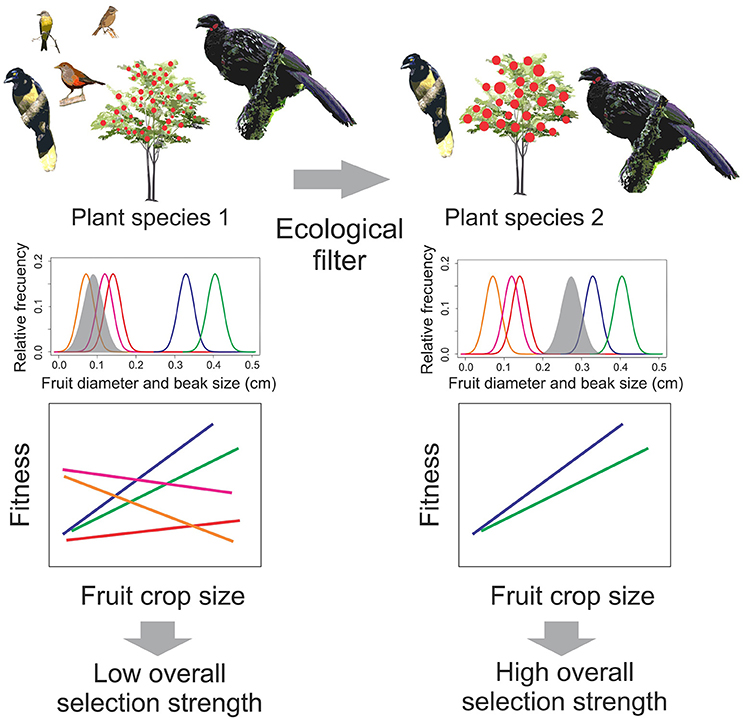
Figure 1. Functional equivalence hypothesis and bird-mediated selection strength on fruit crop size. Ecological filters promoting high functional equivalence would translate into similar selection pressures on fruit display traits increasing selection strength on fruit crop size. As an example, suppose a plant species interacts with an assemblage of frugivorous birds. This plant produces fruits with an average size that may be swallowed by most frugivores from the assemblage, reflected by the frequency distributions of beak sizes (colored lines) and fruit diameter (shaded areas). Under this scenario, birds exert different selection pressures on fruit crop size, some of which may be even conflicting. The expected outcome is a dilution of all selection regimes, resulting in a low overall (assemblage level) selection strength on fruit crop size. When the same frugivore assemblage interacts with another plant species of a larger mean fruit size, some species (small frugivores) are forbidden to interact with the plant due to fruit size constraints (ecological filter), leaving a subset of species playing similar roles for the interaction (large frugivores). Under this scenario, birds are expected to select similar fruit traits and exert similar selection regimes on fruit crop size, thus leading to high overall selection strength. Other ecological filters proposed in this study are fruit type, length of the fruiting season, proportion of gulper and seed predator species, and latitude (see Table 1). Artwork: F. X. Palacio.
There are two central processes increasing selection strength on fruit crop size. The first is related to the fruit crop size itself. Fruit crop size is a key trait for conspicuousness and fruit consumption by birds (Ordano et al., 2017; Palacio et al., 2017a), so plants with more fruits are consumed more. Fruit consumption by birds leads to a positive effect on seed germination, because seed gut passage increases the probability of successful seed dispersal (Traveset and Verdú, 2002; Verdú and Traveset, 2004). If we also consider that fruit crop size is a heritable trait (Wheelwright, 1993; Hancock, 2008), its evolutionary rate will increase with increased selection strength. The second process is that increased functional equivalence involves several species and hence more interacting individuals. Although seed dispersal effectiveness is usually highly variable between species (Ruggera et al., 2016), increased abundance of fruit-consuming species results in a greater number of fruit removed and seeds dispersed (Blendinger, 2017). Therefore, interaction strength will increase, which predicts an increase in the strength of selection (selection intensity for standardized values; Benkman, 2013). A greater number of individuals interacting and playing the same role (i.e., functional equivalent species) will lead to higher interaction strength. The regime (or shape) of the relationship between the interaction strength and the selection intensity will be thus a function of the interacting fruit-consuming birds. If a subset of species after events of ecological filtering is composed by a higher proportion of effective seed dispersers, selection intensity will increase. On the other hand, if the subset of species is composed by a higher proportion of seed predators or non-effective seed dispersers, selection on fruit crop size will be weakened. Altogether, the positive feedback among generations leads selection to a run-away process, increasing the strength of selection on fruit crop size.
Filters such as large fruits (physical constraints on fruit-handling and ingestion; Wheelwright, 1985; Levey, 1987), low pulp-to-seed ratio fruits (benefit-cost ratio based on optimal foraging; Hegde et al., 1991), short fruiting seasons (reduced temporal species overlap; Howe and Estabrook, 1977; Ting et al., 2008), and high latitudes (environmental constraints; Kissling et al., 2009), would restrict some bird species' ability to interact with a plant species. This would leave as interacting partners those species with similar functional roles (increased functional equivalence), promoting higher selection strength on fruit crop size, particularly effective seed dispersers (Figure 1). In other words, these factors would filter bird species on the basis of ecological traits reflecting resource use, increasing both functional equivalence and selection strength on fruit crop size (Figure 1). This idea is partly analogous to the “environmental or niche filtering hypothesis” in community ecology, which proposes that coexisting species are more similar to one another than would be expected by chance because environmental conditions act as a filter allowing a set of species traits to persist (Zobel, 1997; Laliberté et al., 2014; Kraft et al., 2015). Under this premise, a high interaction frequency of species belonging to the same functional group, such as gulper, pulp consumer, or seed predator species (differences in fruit choice criteria; Levey, 1987; Foster, 1990; Palacio et al., 2017b) will also promote higher selection strength on fruit crop size. However, this hypothesis assumes that the filtered subset of bird species includes species with positive consequences on plant fitness, i.e., effective seed dispersers, yet there could also be non-legitimate seed dispersers or even a combination of seed predators and seed dispersers.
Although each of these factors propose specific ecological predictions (Table 1), little attempt has been made to quantitatively synthesize the patterns of phenotypic selection on fruit crop size related to them. Here, we assessed the strength of bird-mediated phenotypic selection on fruit crop size with a meta-analysis. First, we evaluated the widespread assumption about whether birds exert selection pressures on fruit crop size. We then evaluated how much variation in the strength of phenotypic selection on fruit crop size is accounted for plant traits (fruit type and size, length of the fruiting season), bird assemblage traits (fruit-handling behavior) and environmental factors (latitude). Under the premise of signal-reward correlation, our main predictions are that (1) birds exert positive directional selection on fruit crop size, and (2) ecological filters leading to high functional equivalence of the frugivores (large fruits, fruit types with low pulp-to-seed ratio, short fruiting phenologies, similar fruit-eating behavior, high latitudes) promote higher selection strength.
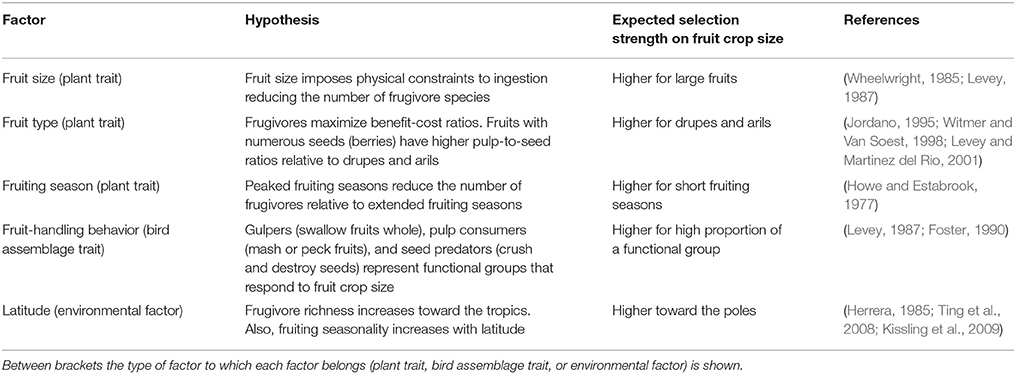
Table 1. Ecological hypotheses and predictions proposed for bird-mediated phenotypic selection strength on fruit crop size.
Materials and Methods
Search and Selection Protocol
We carried out a review of the literature available at the database ISI Web of Science (Thompson Scientific, 2009) using “fruit,” “crop,” “selection,” and “birds” as keywords to find published papers in any year (until 1 September 2015) or language. We then checked out the references of these studies to find earlier publications. With this list and the studies carried out by us and collaborators (Palacio et al., 2017a,b) we completed a list of 100 references (Tables S1, S2, Figure S1). We used the Pearson product-moment correlation coefficient as effect size measure to determine the strength and direction of bird-mediated selection on fruit crop size. Although directional selection is properly measured using the standardized directional selection differential i, which represents the covariance between relative fitness (mean = 1) and a standardized phenotypic trait (mean = 0, variance = 1; Brodie et al., 1995), the advantage of Pearson's correlation is that it is widespread as a statistical descriptor, and well-developed meta-analytical methods exist (Hedges and Olkin, 1985). Moreover, r is proportional to i:, where I is the variance in relative fitness (i.e., opportunity for selection) and is a measure of the power to detect directional selection (Hersch and Phillips, 2004). We therefore selected studies that reported data on fruit crop size and any of three quantitative estimators deemed as surrogate for fitness measures (Brodie and Janzen, 1996; McGraw and Caswell, 1996; Kingsolver et al., 2001; Schupp et al., 2010): bird visitation rate, the number of fruit removed or the proportion of fruit removed. References without this information were excluded (Figure S1).
We defined a visit as the event in which a bird approached a plant and consumed at least one fruit. Several studies have widely used these three fitness components to assess bird-mediated selection on fruit display traits (e.g., Jordano, 1995; Martínez et al., 2007; Sobral et al., 2010; Palacio et al., 2014). We also assumed a positive covariation between short-term dispersal success (a fitness component) and long-term plant fitness (McGraw and Caswell, 1996; Muñoz et al., 2017). Values for Pearson's r were also obtained from one tail P-values when sample size was known (Rosenthal and DiMatteo, 2001), and Spearman's ρ were converted into Pearson's r following Rupinski and Dunlap (1996) as: r = 2sin(ρ × π/6). In several cases, we were able to compute r from raw data. In those studies that raw data were not reported, we estimated approximate values by processing published plots with DATA THIEF III version 1.6 (Tummers, 2010). The final database and the complete list of corresponding references are shown as Supplementary Material (Tables S1, S2).
Effect Sizes and Publication Bias
Correlation coefficients were transformed to Zr using Fisher's algorithm (Hedges and Olkin, 1985). A single mean effect size was computed for species with more than one effect size by averaging Zr values (Rosenthal and DiMatteo, 2001) to account for dependence among species. Once we obtained and transformed effect sizes per species, we computed mean effect sizes for the three fitness components (bird visitation rate, number, and proportion of fruit removed). Within-species variances were computed as 1/(n−3), where n is sample size (Hedges and Olkin, 1985). For comparative purposes, and whenever possible, we computed standardized directional selection differentials. Overall effect sizes were considered statistically significant if 95% confidence intervals did not include zero.
We assessed publication bias both graphically, by funnel plots, and statistically by rank correlations between effect sizes and samples variances (Begg and Mazumdar, 1994). Spearman's rank correlations between effect size and sampling variance were non-significant for the proportion of fruit removed (ρ = −0.050, P = 0.779), but it was significant for the bird visitation rate (ρ = 0.450, P = 0.040) and the number of fruit removed (ρ = 0.511, P = 0.003), indicating the presence of publication bias (Figure S2). We therefore used the Copas selection model (Copas, 1999) to correct mean effect sizes. This model has two components: a model for the effect size, and a “selection” model that gives the probability that study i is published. A Pearson correlation between these two components estimated by maximum likelihood quantifies the magnitude of publication bias; the stronger the correlation, the higher the probability that more extreme effect sizes are published.
Phylogenetic Relationships Between Plant Species
Phylogenetic relationships may lead to non-independence in effect sizes, due to shared phylogenetic history among taxa (Lajeunesse, 2009). We therefore constructed phylogenetic hypotheses using S.Phylomaker, based on the megaphylogeny of vascular plants PhytoPhylo (Qian and Jin, 2016; Figure S3). This phylogeny includes all extant families of gymnosperms and angiosperms, provides ages of all branches, and includes five times as many genera and 55 times more species than does the newest version of angiosperm supertrees derived from Phylomatic (i.e., tree version R20120829; Webb and Donoghue, 2005). We estimated phylogenetic signal in effect sizes with Pagel's λ according to a Brownian motion model of evolution where λ = 0 indicates no phylogenetic signal, and λ = 1 indicates high phylogenetic signal (Pagel, 1999). P-values were computed with 999 randomizations of species across the tips of the phylogeny. Pagel's λ values for correlations between fruit crop size and visitation rate, the number of fruit removed and the proportion of fruit removed were λ = 1.000 (P = 0.151), λ = 0.325 (P = 1.000), and λ = 0.406 (P = 1.000), respectively. This indicates that no effect size expressed phylogenetic inertia, and a phylogenetically controlled meta-analysis is thus unnecessary (Lajeunesse, 2009; Chamberlain et al., 2012).
Factors Affecting Phenotypic Selection on Fruit Crop Size
To test for the heterogeneity of effect sizes we used Cochran's Q, which is computed as the weighted sum of squared differences between individual study species and the mean effect size (Hedges and Olkin, 1985). As a complement, we also computed the I2 index to quantify the degree of heterogeneity in a meta-analysis, which measures the percentage of the total variability in effect sizes due to between-studies heterogeneity (Higgins and Thompson, 2002; Huedo-Medina et al., 2006). We then assessed the influence of factors on mean effect sizes to explain variation in phenotypic selection on fruit crop size. We fitted mixed-effects models using both categorical (fruit type: aril, drupe, or berry) and continuous variables (length of the fruiting season, mean fruit diameter, proportion of gulper, pulp consumer and seed predator species, and latitude) as fixed effects. Mixed- or random-effects meta-analysis assumes that the studies analyzed represent a random sample of effect sizes by including a component of between-study variation (study-specific random effect) into the uncertainty of the effect size parameters and their estimates (Hedges and Olkin, 1985). We selected these variables since most studies included information on these factors. The proportion (relative richness) of gulpers, pulp consumers, and seed predators were used instead of interaction frequency, as most studies did not report the latter. We considered “fleshy fruit” as any fleshy diaspore (dispersal unit of seeds plus fleshy structures covering the seeds; Herrera, 2002), so aril was categorized as a fruit type. Regarding bird foraging behavior, we categorized gulpers as species that swallow fruits whole, pulp consumers as those that mash or peck fruits, and seed predators as those that crush and typically destroy seeds (Foster, 1987; Levey, 1987). The length of the fruiting season and fruit diameter were log-transformed due to right-skewed distributions. The proportion of gulper and pulp consumer species were highly correlated (r < −0.90), so we only included the former. To assess the importance of explanatory variables, we adopted an information-theoretic approach based on Akaike's Information Criterion corrected for small sample sizes (AICc), which allows identifying best models given a certain set of plausible hypotheses (Johnson and Omland, 2004; Burnham et al., 2011). We fitted four non-nested main models per fitness component (bird visitation rate, number and proportion of fruit removed), which represented a trade-off between the classification of factors proposed here (plant traits, bird assemblage traits and environmental factors) and differences in sample sizes: a “plant trait” model 1 (fruit type + fruit diameter), a “plant trait” model 2 (length of the fruiting season), a “bird assemblage trait” model (proportion of gulper species + proportion of seed predator species), and an “environmental model” (latitude). For each of the four main models, all the possible nested models were ranked based on their AICc, and those models with the lowest AICc in a range such that ΔAICc <2 were identified as the best models. To complement this analysis and test the support of our results, we fitted a model for bird visitation rate, number and proportion of fruit removed including all variables.
All analyses were performed in R 3.4.2 (R Core Team, 2017), using the packages phytools (function phylosig; Revell, 2012), metafor (function rma; Vietchtbauer, 2010), metasens (function copas; Schwarzer et al., 2016), and glmulti (function glmulti; Calcagno, 2013).
Results
Bird Mediated-Selection on Fruit Crop Size
Data of 50 plant species from 27 families (44 published and 5 unpublished studies) met the selection criteria to assess phenotypic selection on fruit crop size (see Figures S3, S4). Most families were represented by 1 or 2 species, but Rosaceae, Burseraceae, and Myristicaceae were represented by 7, 6, and 5 species, respectively. The median number of plants monitored per species within a publication was 17 (mean = 43.7, SD = 61.9, range = 5–278). Most species were represented by only one study; only data for Olea europaea (Oleaceae), Virola surinamensis (Myristicaceae), and Crataegus monogyna (Rosaceae) were available from 2, 3, and 4 studies, respectively. All continents (except for Asia and Antarctica) were represented in the meta-analysis, with most data from the Americas (31 studies) and Europe (12 studies; Figure S4). Significant positive effects (z-transformed values) of bird-mediated selection on fruit crop size were detected for bird visitation rate (mean bias-uncorrected effect size = 0.584, n = 21, 95% CI = 0.385–0.783; mean bias-corrected effect size = 0.400, n = 21, 95% CI = 0.188–0.613) and the number of fruit removed (mean bias-uncorrected effect size = 1.640, n = 32, 95% CI = 1.390–1.890; mean bias-corrected effect size = 1.300, n = 32, 95% CI = 1.181–1.419), but not for the proportion of fruit removed (mean bias-uncorrected effect size = 0.098, n = 34, 95% CI = −0.017 to 0.213; mean bias-corrected effect size = 0.097, n = 34, 95% CI = −0.011 to 0.211). Moreover, between-species heterogeneity was high (bird visitation rate I2 = 80.10%, number of fruit removed I2 = 94.59%; proportion of fruit removed I2 = 71.94%) and significant for the three overall effect sizes (bird visitation rate Q = 73.573, df = 20; number of fruit removed Q = 497.432, df = 31; proportion of fruit removed Q = 98.103, df = 33; all P's < 0.0001). These results therefore justified our subsequent analyses of factors explaining variation in bird-mediated selection on fruit crop size.
Directional selection differentials were consistent with results using Pearson's correlation as effect size. Differentials showed positive directional selection for the three fitness components (bird visitation rate, number, and proportion of fruit removed). Selection strength on fruit crop size was stronger for the number of fruit removed (median = 1.030, range = 0.033–2.336, n = 31) and bird visitation rate (median = 0.473, range = −0.483–1.581, n = 23), and it was lowest for the proportion of fruit removed (median = 0.041, range = −0.261 to 0.586, n = 36). It should be noted that selection differentials measure both direct selection on a given phenotypic trait and indirect selection through correlations with other traits (Lande and Arnold, 1983), so these values should be interpreted with some caution.
Factors Affecting Phenotypic Selection on Fruit Crop Size
Both plant (length of the fruiting season, mean fruit diameter) and bird (proportion of species belonging to the same functional group) traits, as well as environmental factors (latitude) explained variation in bird-mediated selection on fruit crop size (Tables 2, 3, Figure 2). For the three fitness measures higher selection strength was detected in plants with shorter fruiting seasons and higher proportion of gulper species (Table 3, Figure 2). Also, for the proportion of fruit removed, higher selection strength was detected in plants with larger fruits (Table 3, Figure 2). For the number and proportion of fruit removed, the proportion of seed predators explained variation in phenotypic selection on fruit crop size but showed opposite trends (Table 3, Figure 2). In particular, seed-dispersal systems with higher proportion of seed predators showed either higher or lower selection strength on fruit crop size for the proportion and number of fruit removed, respectively. Finally, selection strength increased toward the equator for bird visitation rate (Table 3, Figure 2). Models with all variables gave similar qualitative results despite their low sample size (Table S3).
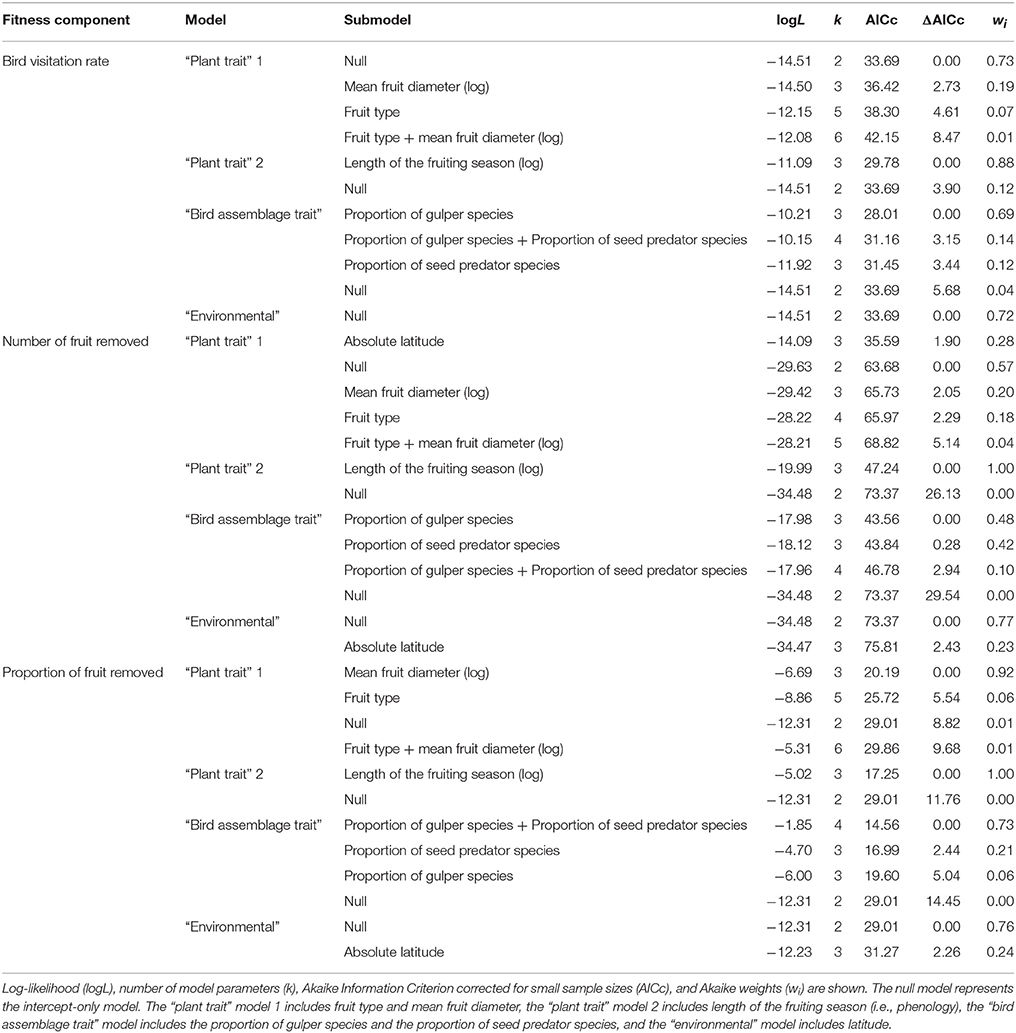
Table 2. Set of models relating explanatory variables with bird-mediated selection on fruit crop size.
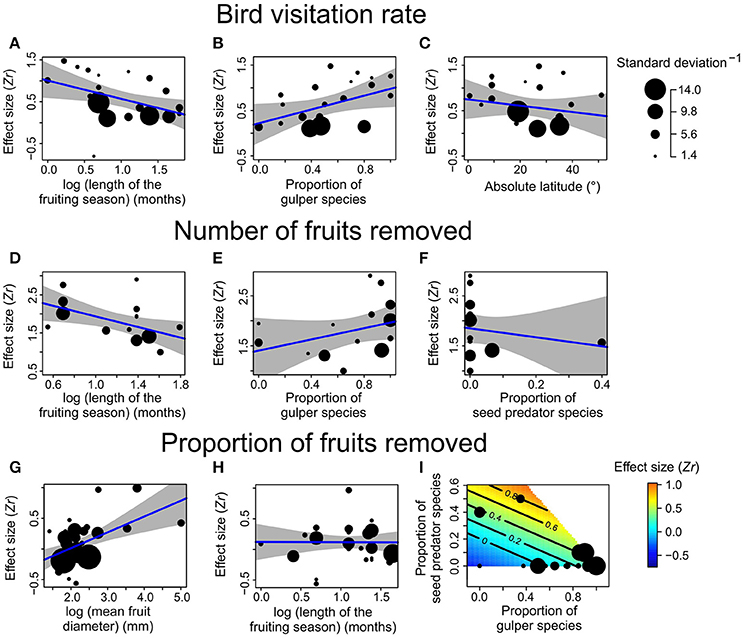
Figure 2. Effect sizes for factors explaining variation of bird-mediated selection on fruit crop size for three fitness components: (A–C) visitation rate, (D–F) number of fruit removed, and (G–I) proportion of fruit removed. (A–H) show simple meta-regression models (blue lines) and 95% confidence intervals (shaded areas), whereas (I) shows a response surface between the proportion of gulper species, the proportion of seed predator species (explanatory variables) and the effect size as response variable (contour lines and scale bar). The length of the fruiting season and mean fruit diameter represent a plant trait, the proportion of gulper and seed predator species represent bird assemblage traits, and latitude represents environmental factors.
Discussion
As Snow (1971) proposed in the first study on the evolutionary ecology of seed dispersal by birds, our meta-analysis suggests that fruit crop size represents a prominent target of bird-mediated selection, and that birds promote larger fruit crop sizes as an overall pattern in nature. After Snow (1971), numerous authors have found a positive association between fruit crop size and fruit removal (Howe and Vande Kerchove, 1981; Davidar and Morton, 1986; Jordano, 1987; Murray, 1987; Foster, 1990; Carr, 1992; Sallabanks, 1992; Laska and Stiles, 1994; Ortiz-Pulido and Rico-Gray, 2000; Palacio et al., 2017b; see also Tables S1, S2). Although the underlying mechanism of this hypothesis is still unclear, it has been traditionally assumed that frugivores perceive fruit display as a signal that determines fruit choice and, consequently, fruit removal (Snow, 1971; Murray, 1987; Willson and Whelan, 1990; Schmidt et al., 2004; Ordano et al., 2017). For instance, in a recent study of fruit traits of 62 plant species of a subtropical Andean forest, Ordano et al. (2017) found that fruit consumption was explained by a positive interaction between fruit crop size and chromatic contrast, supporting the idea that conspicuousness of fruit display acts as a strong signal for visually driven frugivores. However, this hypothesis remains contentious, as several studies have shown no evidence that fruit's signals (e.g., color) are adapted to maximize fruit conspicuousness (Schaefer et al., 2007; Burns et al., 2009; Stournaras and Schaefer, 2017). In this sense, our results do support the hypothesis that fruit crop size represents a conspicuous signal at the plant level that increases fruit detectability, but also reveals the need to assess the interplay with other fruit traits advertising rewards to seed dispersers.
Moreover, the positive directional selection regime on fruit crop size depended on the fitness component. In particular, both the number of fruit removed and visitation rate would be fitness components of fruit removal under selection pressures, contrary to the proportion of fruit removed. This agrees with a few studies quantifying the three fitness components within the same study system (Howe and De Steven, 1979; Howe and Vande Kerckhove, 1979; Howe, 1980) and supports the view that bird behavior promotes absolute rather than proportional dispersal success (Davidar and Morton, 1986; Izhaki, 2002; Stournaras and Schaefer, 2017). From a theoretical perspective, the proportion of fruit removed has been considered a benefit-cost ratio for the plant, since it relates the reproductive investment to the number of dispersed seeds (Herrera, 1991; Willson and Whelan, 1993). Our results suggest that the number of fruit removed is the principal fitness component, at least at this reproductive stage. From the plant's perspective, fruit production should be maximized at the limit of the costs of fruit production to guarantee seed dispersal in a competitive scenario for seed dispersers (Howe and Estabrook, 1977; Albrecht et al., 2015; Donoso et al., 2017). It is worth mentioning that a greater dispersal success (absolute and relative) does not necessarily entails greater recruiting success, due to uncoupling between successive recruitment stages (Jordano and Herrera, 1995; Wang and Smith, 2002; Palacio et al., 2014; but see Blendinger et al., 2011).
Factors Affecting Bird-Mediated Selection Strength on Fruit Crop Size
The functional equivalence hypothesis proposes that different ecological filters (e.g., phenology, plant traits, environmental factors) remove species and leave those with similar trait values and functional roles, promoting similar selection regimes on plant traits (Zamora, 2000). Several studies have found an increase in selection strength resulting from an increase in the abundance of species with shared functional traits. For instance, Alcántara et al. (2007) found that ant assemblages with similar-sized species exerted similar selection pressures on elaiosome and seed size in 12 populations of Helleborus foetidus. In another study, Galetti et al. (2013) found that the functional extinction of large-gape seed dispersers correlated with an evolutionary reduction of seed size in 22 populations of Euterpe edulis in the Atlantic Forest from Brazil. In this case, defaunation acted as a filter on large species changing the evolutionary trajectory of seed size (Galetti et al., 2013). These studies suggest that changes in the abundance of functionally equivalent interacting partners stemming from ecological filters may be an important evolutionary force driving phenotypic trait variation, and therefore deserves further research.
For the three fitness components studied, two plant and bird traits (fruiting phenology and fruit-handling behavior) consistently played a role in bird-mediated selection strength on fruit crop size. As predicted by the functional equivalence hypothesis, factors that would filter bird functional traits promoted higher selection strength on fruit crop size. The timing of fruiting has been shown to play a role in regulating the abundance and diversity of frugivores in many communities (Fenner, 1998; Burns, 2004; Albrecht et al., 2015). A fruit display in a short time period would decrease assemblage diversity relative to extended fruiting seasons, acting as a temporal filter and increasing selection strength on fruit crop size (McKey, 1975; Howe and Estabrook, 1977; Howe, 1993; Fenner, 1998). Higher proportions of species from the same functional group also supported the functional equivalence hypothesis, under the idea that an increase in the proportion of species with shared functional traits promotes higher selection pressure on fruit traits. One limitation of our approach is that we considered the effects of legitimate seed dispersers and seed predators on plant fitness as equivalent. It is known that the effect of fruit removal by seed dispersers and predators are opposite in all aspects of plant recruitment, in which seed predators have a detrimental effect on plant fitness, contrary to seed dispersers (Levey, 1987; Schupp et al., 2010; but see Loayza and Knight, 2010; Tella et al., 2015). From our results, we can only assert that both gulpers and seed predators favor larger fruit crop sizes but, under the assumption that seed predators negatively affect fitness, higher proportion of seed predators entails a negative directional selection pattern on fruit crop size. This highlights the role of antagonistic interactions in shaping fruit display traits in multispecies assemblages (e.g., Alcántara and Rey, 2003; Gómez, 2004; Martínez et al., 2007; Siepielski and Benkman, 2007).
Another plant trait, i.e., mean fruit diameter, also explained variation in selection strength on fruit crop size. In particular, larger fruits promoted higher selection strength on fruit crop size for the proportion of fruit removed. Fruit size is considered a key trait at the fruit unit level in mutualistic interactions of frugivores and fleshy-fruited plants, as it constraints fruit-handling behavior and fruit consumption by seed dispersers (“the fruit size hypothesis”; Wheelwright, 1985; Levey, 1987). Under this hypothesis, it would be expected a decrease of frugivore richness with increased fruit size (Wheelwright, 1985; Jordano, 2000). Indeed, plant species of our database with the largest mean fruit sizes (Aglaia flavida: 150 mm, Pinus edulis: 45 mm) were consumed by one and two frugivores, respectively. In contrast, mean frugivore richness per plant species in those systems ranging from 1 to 10 mm in fruit diameter was 10.2 ± 6.4 bird species. Therefore, larger fruits would promote higher strength on fruit crop size, as a result of coupled selection pressures of a reduced bird assemblage (Herrera, 1985). A latitudinal effect on phenotypic selection on fruit crop size would also be expected, as both frugivore richness (Kissling et al., 2009) and fruiting seasons (Ting et al., 2008) increases toward the tropics which, interestingly, is explained by the same climatic variable (actual evapotranspiration). Nonetheless, we found a negative latitudinal trend of selection strength on fruit crop size, although of low magnitude. A possible explanation is that an unmeasured variable covaries with latitude that decreases selection strength toward the tropics. Recently, Dalsgaard et al. (2017) found opposed latitudinal patterns of network-derived measures of specialization and dietary specialization in bird-frugivore interactions. In particular, the proportion of obligate frugivores increased toward the tropics, but frugivorous birds divided the niche of fruiting plants more finely at high latitudes (Dalsgaard et al., 2017). Higher proportion of obligate frugivores within a seed-dispersal assemblage (potentially fulfilling similar functional roles; Loiselle et al., 2007) may drive higher selection strength on fruit crop size, an idea that deserves further research.
Finally, phylogenetic relationships played a minor role in bird-mediated selection strength on fruit crop size. Indeed, no phylogenetic signal was detected for any fitness component. This also supports the fruit crop size hypothesis, which states that birds promote positive selection on fruit crop size, regardless of plant identity.
Evolutionary Consequences of Selection Imposed by Frugivores on Fruit Crop Size
Several conditions have been advocated to constrain reciprocal coadaptation among plants and seed dispersers, leading to diffuse coevolution (Wheelwright and Orians, 1982; Howe, 1984; Herrera, 1985; Tewksbury, 2002): (1) low selection intensity of dispersers on fruit traits, (2) inconsistent spatiotemporal bird-mediated selection, (3) antagonistic selection by fruit or seed predators, (4) correlations among fruit traits that prevent the evolution of mutual adaptations, and (5) differences in evolutionary rates between plants and dispersers. Two of these conditions, however, are not supported by our results. First, selection coefficients on fruit crop size fell within the range of other reported values of phenotypic selection in natural populations, yet for the number of fruit removed it would be considerably strong (most linear gradients range between −1 and +1, |median| = 0.16; Kingsolver et al., 2001). Therefore, fruit crop size represents a phenotypic trait under strong selection. Furthermore, it has shown to be highly heritable (h2 = 83.0–99.0%; e.g., Manju and Sreelathakumary, 2002; de Moraes et al., 2005; Denton and Nwangburuka, 2011; Meena and Bahadur, 2014) indicating a strong response to selection. Second, we did not find strong negative correlations among fruit crop size and other fruit traits that may prevent reciprocal adaptations. Indeed, we found only positive correlations between fruit crop size and other fruit traits, such as mean fruit diameter (rPsychotria carthagenensis = 0.11, n = 72; rVassobia breviflora = 0.10, n = 27; rJodina rhombifolia = 0.28, n = 17; rPassiflora caerulea = 0.35, n = 8) and mean sugar concentration (rPsychotria carthagenensis = 0.03, n = 72; rVassobia breviflora = 0.22, n = 27). Other studies in natural conditions have found no associations between fruit crop size and other fruit level traits (Michaels et al., 1988; Martínez et al., 2007; Palacio et al., 2014; but see Sallabanks, 1993). Although an increase in fruit production could compromise fruit quality (fruit size, pulp mass, nutrient content), this has been shown under experimental conditions (Barone et al., 1994; Berman and DeJong, 1996; Naor et al., 1999, 2008; Stopar et al., 2002; Roussos et al., 2011). Negative correlations between fruit crop size and other fruit traits may be obscured by the fact that fruit crop size is a complex trait constrained by several factors (Lee, 1988) including resource availability (Lescourret and Génard, 2003), plant age (Mechlia and Carroll, 1989; Marshall et al., 2010), flower display and pollination service (Herrera, 1991; Cunningham, 2000; Benavidez et al., 2013; Stournaras and Schaefer, 2017). The interaction between different factors affecting fruit crop size may reduce correlations with other fruit traits, potentially relaxing indirect selection on fruit crop size in nature (e.g., Jordano, 1995; Sobral et al., 2010; Palacio et al., 2014). By contrast, counteracting selection pressures exerted by seed predators may dilute the overall selection pressure imposed on fruit crop size, favoring the process of diffuse coevolution (Herrera, 1985). Our results support this idea, as an increase in the proportion of seed predators favored large fruit crops. Finally, seed dispersers may shape the evolution of seed size and number, as a result of a trade-off between fruit crop size, seed size, and seed number (Smith and Fretwell, 1974; Eriksson and Jakobsson, 1999; Sadras, 2007). In particular, fruit crop size is positively correlated with the total seed number of a plant, but seed number within a fruit is negatively correlated with mean seed size (Westoby et al., 1992; Parciak, 2002; Sadras, 2007). Therefore, selection on large fruit crop sizes should promote lower mean seed size. This suggests that seed dispersal may be more important than seed size for successful offspring recruitment (Parciak, 2002), although the existence of conflicting selection by other selection agents (e.g., seed predators) might explain the occurrence of an optimal seed size in some plant species without invoking a seed number-size trade-off (Gómez, 2004).
Concluding Remarks and Prospects
As Snow (1971) proposed in his seminal study, the number of fruit a plant displays is a key trait subject to bird-mediated natural selection. The positive directional selection on fruit crop size would be a common regime in nature, and the strong intensity on this trait suggests that it may be more important than other fruit display traits (e.g., Jordano, 1995; Sobral et al., 2010; Palacio et al., 2017a). Moreover, both plant and bird traits play major roles in natural selection regimes on fruit crop size. As we have shown, the strength of selection on fruit crop size is driven mainly by plant fruiting phenology and functional equivalence. Being the first meta-analysis on this matter, it opens new questions and suggests there are still important gaps of studies measuring phenotypic selection on fruit crop size in natural populations, the first step in understanding its evolution (Endler, 1986).
After 45 years of the fruit crop size hypothesis, only four studies have reported selection coefficients of natural selection on fruit crop size (Jordano, 1995; Sobral et al., 2010; Palacio et al., 2014, 2017a). Future studies should report selection coefficients not only on fruit crop size (linear and quadratic selection), but also on combinations of fruit crop size with other fruit traits (correlational selection). This information would give us a broader picture of the patterns and causes of bird-mediated phenotypic selection on fruit crop size, as well as the relationship between fruit crop size and individual fruit level traits.
Overall, as the main ecological consequence, the maximization in fruit production would increase seed dispersal. As an evolutionary one, birds would impose constraints on fruit display development, providing plants enhanced opportunities for the evolution of reproductive assurance mechanisms. Furthermore, the relationship among forces impinged by phenology and frugivore functional roles may be key to understand their evolutionary stability.
Author Contributions
FP and MO: Contributed substantially to the conception and design of the study; FP: Performed the meta-analysis and wrote the first draft of the manuscript. Both authors contributed to ideas and revisions.
Conflict of Interest Statement
The authors declare that the research was conducted in the absence of any commercial or financial relationships that could be construed as a potential conflict of interest.
Acknowledgments
We thank Analía Benavidez and Pedro G. Blendinger for kindly providing data of their study systems. Three reviewers provided valuable comments to the manuscript. This study was partially funded by Fundación Miguel Lillo (Ministerio de Educación, Argentina) and Consejo Nacional de Investigaciones Científicas y Técnicas (CONICET, Ministerio de Ciencia y Tecnología, Argentina).
Supplementary Material
The Supplementary Material for this article can be found online at: https://www.frontiersin.org/articles/10.3389/fevo.2018.00018/full#supplementary-material
References
Albrecht, J., Bohle, V., Berens, D. G., Jaroszewicz, B., Selva, N., and Farwig, N. (2015). Variation in neighbourhood context shapes frugivore-mediated facilitation and competition among co-dispersed plant species. J. Ecol. 103, 526–536. doi: 10.1111/1365-2745.12375
Alcántara, J. M., and Rey, P. J. (2003). Conflicting selection pressures on seed size: evolutionary ecology of fruit size in a bird-dispersed tree, Olea europaea. J. Evol. Biol. 16, 1168–1176. doi: 10.1046/j.1420-9101.2003.00618.x
Alcántara, J. M., Rey, P. J., Manzaneda, A. J., Boulay, R., Ramírez, J. M., and Fedriani, J. M. (2007). Geographic variation in the adaptive landscape for seed size at dispersal in the myrmecochorous Helleborus foetidus. Evol. Ecol. 21, 411–430. doi: 10.1007/s10682-006-9110-3
Barone, E., Gullo, G., Zappia, R., and Inglese, P. (1994). Effect of crop load on fruit ripening and olive oil (Olea europea L.) quality. J. Hortic. Sci. 69, 67–73. doi: 10.1080/14620316.1994.11515250
Begg, C. B., and Mazumdar, M. (1994). Operating characteristics of a rank correlation test for publication bias. Biometrics 50, 1088–1101. doi: 10.2307/2533446
Benavidez, A., Ordano, M., and Valoy, M. (2013). Autocompatibilidad y selección mediada por polinizadores sobre el despliegue floral en una población de Psychotria carthagenensis (Rubiaceae). Lilloa 50, 10–19. Available online at: http://lillo.org.ar/publicaciones/lilloa/v50n1/a02
Benitez-Vieyra, S., Ordano, M., Fornoni, J., Boege, K., and Domínguez, C. A. (2010). Selection on signal–reward correlation: limits and opportunities to the evolution of deceit in Turnera ulmifolia L. J. Evol. Biol. 23, 2760–2767. doi: 10.1111/j.1420-9101.2010.02132.x
Benkman, C. W. (2013). Biotic interaction strength and the intensity of selection. Ecol. Lett. 16, 1054–1060. doi: 10.1111/ele.12138
Berman, M. E., and DeJong, T. M. (1996). Water stress and crop load effects on fruit fresh and dry weights in peach (Prunus persica). Tree Physiol. 16, 859–864. doi: 10.1093/treephys/16.10.859
Blendinger, P. G. (2017). Functional equivalence in seed dispersal effectiveness of Podocarpus parlatorei in Andean fruit-eating bird assemblages. Front. Ecol. Evol. 5:57. doi: 10.3389/fevo.2017.00057
Blendinger, P. G., Blake, J. G., and Loiselle, B. A. (2011). Connecting fruit production to seedling establishment in two co-occurring Miconia species: consequences of seed dispersal by birds in upper Amazonia. Oecologia 167, 61–73. doi: 10.1007/s00442-011-1956-6
Brodie, E. D., and Janzen, F. J. (1996). On the assignment of fitness values in statistical analyses of selection. Evolution 50, 437–442. doi: 10.1111/j.1558-5646.1996.tb04505.x
Brodie, E. D., Moore, A. J., and Janzen, F. J. (1995). Visualizing and quantifying natural selection. Trends Ecol. Evol. 10, 313–318. doi: 10.1016/S0169-5347(00)89117-X
Burnham, K. P., Anderson, D. R., and Huyvaert, K. P. (2011). AIC model selection and multimodel inference in behavioral ecology: some background, observations, and comparisons. Behav. Ecol. Sociobiol. 65, 23–35. doi: 10.1007/s00265-010-1029-6
Burns, K. C. (2004). Scale and macroecological patterns in seed dispersal mutualisms. Global Ecol. Biogeogr. 13, 289–293. doi: 10.1111/j.1466-822X.2004.00108.x
Burns, K. C. (2005). Is there limiting similarity in the phenology of fleshy fruits? J. Veg. Sci. 16, 617–624. doi: 10.1111/j.1654-1103.2005.tb02404.x
Burns, K. C. (2015). The colour of plant reproduction: macroecological trade-offs between biotic signaling and abiotic tolerance. Front. Ecol. Evol. 3:118. doi: 10.3389/fevo.2015.00118
Burns, K. C., Cazetta, E., Galetti, M., Valido, A., and Schaefer, H. M. (2009). Geographic patterns in fruit colour diversity: do leaves constrain the colour of fleshy fruits? Oecologia 159, 337–343. doi: 10.1007/s00442-008-1227-3
Calcagno, V. (2013). glmulti: Model Selection and Multimodel Inference Made Easy. Available online at: https://CRAN.R-project.org/package=glmulti
Carr, D. E. (1992). The relationship between crop size and fruit removal and its implication for the evolution of dioecy. Can. J. Bot. 70, 1784–1789. doi: 10.1139/b92-221
Chamberlain, S. A., Hovick, M. S., Dibble, C. J., Rasmussen, N. L., Van Allen, B. G., Maitner, B. S., et al. (2012). Does phylogeny matter? Assessing the impact of phylogenetic information in ecological meta-analysis. Ecol. Lett. 15, 627–663. doi: 10.1111/j.1461-0248.2012.01776.x
Christensen, K. M., Whitham, T. G., and Balda, R. P. (1991). Discrimination among pinyon pine trees by Clark's Nutcrackers: effects of cone crop size and cone characters. Oecologia 86, 402–407.
Copas, J. (1999). What works? Selectivity models and meta-analysis. J. R. Stat. Soc. A 162, 95–109. doi: 10.1111/1467-985X.00123
Cunningham, S. A. (2000). Depressed pollination in habitat fragments causes low fruit set. Proc. R. Soc. Lond. B Biol. 267, 1149–1152. doi: 10.1098/rspb.2000.1121
Dalsgaard, B., Schleuning, M., Maruyama, P. K., Dehling, D. M., Sonne, J., Vizentin-Bugoni, J., et al. (2017). Opposed latitudinal patterns of network-derived and dietary specialization in avian plant–frugivore interaction systems. Ecography 40, 1395–1401. doi: 10.1111/ecog.02604
Davidar, P., and Morton, E. S. (1986). The relationship between fruit crop sizes and fruit removal rates by birds. Ecology 67, 262–265. doi: 10.2307/1938529
Dehling, D. M., Jordano, P., Schaefer, H. M., Böhning-Gaese, K., and Schleuning, M. (2016). Morphology predicts species' functional roles and their degree of specialization in plant–frugivore interactions. Proc. R. Soc. B 27:1823. doi: 10.1098/rspb.2015.2444
de Moraes, M. C., Geraldi, I. O., de Pina Matta, F., and Vieira, M. L. C. (2005). Genetic and phenotypic parameter estimates for yield and fruit quality traits from a single wide cross in yellow passion fruit. HortScience 40, 1978–1981. Available online at: http://hortsci.ashspublications.org/content/40/7/1978.short
Denton, O. A., and Nwangburuka, C. C. (2011). Heritability, genetic advance and character association in six yield related characters of Solanum anguivi. Asian J. Agric. Res. 5, 201–207. doi: 10.3923/ajar.2011.201.207
Donoso, I., García, D., Martínez, D., Tylianakis, J. M., and Stouffer, D. B. (2017). Complementary effects of species abundances and ecological neighborhood on the occurrence of fruit-frugivore interactions. Front. Ecol. Evol. 5:133. doi: 10.3389/fevo.2017.00133
Eriksson, O., and Jakobsson, A. (1999). Recruitment trade-offs and the evolution of dispersal mechanisms in plants. Evol. Ecol. 13, 411–423. doi: 10.1023/A:1006729311664
Fenner, M. (1998). The phenology of growth and reproduction in plants. Perspect. Plant Ecol. Evol. Syst. 1, 78–91. doi: 10.1078/1433-8319-00053
Foster, M. S. (1987). Feeding methods and efficiencies of selected frugivorous birds. Condor 89, 566–580. doi: 10.2307/1368645
Foster, M. S. (1990). Factors influencing bird foraging preferences among conspecific fruit trees. Condor 92, 844–854. doi: 10.2307/1368720
Galetti, M., Guevara, R., Côrtes, M. C., Fadini, R., Von Matter, S., Leite, A. B., et al. (2013). Functional extinction of birds drives rapid evolutionary changes in seed size. Science 340, 1086–1090. doi: 10.1126/science.1233774
Gómez, J. M. (2004). Bigger is not always better: conflicting selective pressures on seed size in Quercus ilex. Evolution 58, 71–80. doi: 10.1111/j.0014-3820.2004.tb01574.x
Hampe, A. (2003). Large-scale geographical trends in fruit traits of vertebrate-dispersed temperate plants. J. Biogeogr. 30, 487–496. doi: 10.1046/j.1365-2699.2003.00852.x
Hancock, J. F. (2008). Temperate Fruit Crop Breeding: Germplasm to Genomics. New York, NY: Springer. doi: 10.1007/978-1-4020-6907-9
Hedges, L. V., and Olkin, I. (1985). Statistical Methods for Meta-Analysis. Orlando, FL: Academic Press.
Hegde, S. G., Ganeshaiah, K. N., and Shaanker, R. U. (1991). Fruit preference criteria by avian frugivores: their implications for the evolution of clutch size in Solanum pubescens. Oikos 60, 20–26. doi: 10.2307/3544987
Herrera, C. M. (1985). Determinants of plant-animal coevolution: the case of mutualistic dispersal of seeds by vertebrates. Oikos 44, 132–141. doi: 10.2307/3544054
Herrera, C. M. (1991). Dissecting factors responsible for individual variation in plant fecundity. Ecology 72, 1436–1448. doi: 10.2307/1941116
Herrera, C. M. (2002). “Seed dispersal by vertebrates,” in Plant-Animal Interactions an Evolutionary Approach, eds C. M. Herrera and O. Pellmyr (Padstow: Blackwell Scientific Publications), 185–208.
Herrera, C. M. (2009). Multiplicity in Unity: Plant Subindividual Variation and Interaction with Animals. Chicago, IL: Chicago University Press. doi: 10.7208/chicago/9780226327952.001.0001
Herrera, C. M. (2017). The ecology of subindividual variability in plants: patterns, processes, and prospects. Web Ecol. 17:51. doi: 10.5194/we-17-51-2017
Hersch, E. I., and Phillips, P. C. (2004). Power and potential bias in field studies of natural selection. Evolution 58, 479–485. doi: 10.1111/j.0014-3820.2004.tb01671.x
Higgins, J. P., and Thompson, S. G. (2002). Quantifying heterogeneity in a meta-analysis. Stat. Med. 21, 1539–1558. doi: 10.1002/sim.1186
Howe, H. F. (1980). Monkey dispersal and waste of a neotropical fruit. Ecology 61, 944–959. doi: 10.2307/1936763
Howe, H. F. (1984). Constraints on the evolution of mutualisms. Am. Nat. 123, 764–777. doi: 10.1086/284238
Howe, H. F. (1993). Specialized and generalized dispersal systems: where does ‘the paradigm’ stand? Vegetatio 107/108, 3–13.
Howe, H. F., and De Steven, D. (1979). Fruit production, migrant bird visitation, and seed dispersal of Guarea glabra in Panama. Oecologia 39, 185–196. doi: 10.1007/BF00348067
Howe, H. F., and Estabrook, G. F. (1977). Bird activity and seed dispersal of a tropical wet forest tree. Ecology 58, 539–550. doi: 10.2307/1939003
Howe, H. F., and Vande Kerckhove, G. A. (1979). Fecundity and seed dispersal of a tropical tree. Ecology 60, 180–189. doi: 10.2307/1936479
Howe, H. F., and Vande Kerchove, G. A. (1981). Removal of wild nutmeg (Virola surinamensis) crops by birds. Ecology 62, 1093–1106. doi: 10.2307/1937007
Huedo-Medina, T. B., Sánchez-Meca, J., Marín-Martínez, F., and Botella, J. (2006). Assessing heterogeneity in meta-analysis: Q statistic or I2 index? Psychol. Methods 11:193. doi: 10.1037/1082-989X.11.2.193
Izhaki, I. (2002). “The role of fruit traits in determining fruit removal in East Mediterranean ecosystems,” in Seed Dispersal and Frugivory: Ecology, Evolution, and Conservation, eds D. J. Levey, W. R. Silva, and M. Galetti (Wallingford: CAB International), 161–175.
Johnson, J. B., and Omland, K. S. (2004). Model selection in ecology and evolution. Trends Ecol. Evol. 19, 101–108. doi: 10.1016/j.tree.2003.10.013
Jordano, P. (1987). Avian fruit removal: effects of fruit variation, crop size, and insect damage. Ecology 68, 1711–1723. doi: 10.2307/1939863
Jordano, P. (1995). Frugivore-mediated selection on fruit and seed size: birds and St. Lucie's cherry, Prunus mahaleb. Ecology 76, 2627–2639. doi: 10.2307/2265833
Jordano, P. (2000). “Fruits and frugivory,” in Seeds: the Ecology of Regeneration in Plant Communities, ed M. Fenner (Wallingford: CAB International Publishing), 125–166.
Jordano, P., and Herrera, C. M. (1995). Shuffling the offspring: uncoupling and spatial discordance of multiple stages in vertebrate seed dispersal. EcoScience 2, 230–237 doi: 10.1080/11956860.1995.11682288
Kingsolver, J. G., Hoekstra, H. E., Hoekstra, J. M., Berrigan, D., Vignieri, S. N., Hill, C. E., et al. (2001). The strength of phenotypic selection in natural populations. Am. Nat. 157, 245–261. doi: 10.1086/319193
Kissling, W. D., Böhning-Gaese, K., and Jetz, W. (2009). The global distribution of frugivory by birds. Global Ecol. Biogeogr. 18, 150–162. doi: 10.1111/j.1466-8238.2008.00431.x
Kraft, N. J., Adler, P. B., Godoy, O., James, E. C., Fuller, S., and Levine, J. M. (2015). Community assembly, coexistence and the environmental filtering metaphor. Funct. Ecol. 29, 592–599. doi: 10.1111/1365-2435.12345
Lajeunesse, M. J. (2009). Meta-analysis and the comparative phylogenetic method. Am. Nat. 174, 369–381. doi: 10.1086/603628
Laliberté, E., Zemunik, G., and Turner, B. L. (2014). Environmental filtering explains variation in plant diversity along resource gradients. Science 345, 1602–1605. doi: 10.1126/science.1256330
Lande, R., and Arnold, S. J. (1983). The measurement of selection on correlated characters. Evolution 6, 1210–1226.
Laska, M. S., and Stiles, E. W. (1994). Effects of fruit crop size on intensity of fruit removal in Viburnum prunifolium (Caprifoliaceae). Oikos 69, 199–202. doi: 10.2307/3546138
Lee, T. H. (1988). “Patterns of fruit and seed production,” in Plant Reproductive Ecology: Patterns and Strategies, eds J. L. Doust and L. L. Doust (New York, NY: Oxford University Press), 179–202.
Lescourret, F., and Génard, M. (2003). A multi-level theory of competition for resources applied to fruit production. Ecoscience 10, 334–341. doi: 10.1080/11956860.2003.11682782
Levey, D. (1987). Seed size and fruit-handling techniques of avian frugivores. Am. Nat. 129, 471–485. doi: 10.1086/284652
Levey, D., and Martinez del Rio, C. (2001). It takes guts (and more) to eat fruit: lessons from avian nutritional ecology. Auk 118, 819–831. doi: 10.2307/4089834
Loayza, A. P., and Knight, T. (2010). Seed dispersal by pulp consumers, not “legitimate” seed dispersers, increases Guettarda viburnoides population growth. Ecology 91, 2684–2695. doi: 10.1890/09-0480.1
Loiselle, B. A., Blendinger, P. G., Blake, J. G., and Ryder, T. B. (2007). “Ecological redundancy in seed dispersal systems: a comparison between manakins (Aves: Pipridae) in two tropical forests,” in Seed Dispersal: Theory and Its Application in a Changing World, eds A. J. Dennis, E. W. Schupp, R. J. Green, and D. A. Westcott (Wallingford: CABI), 178–195.
Manju, P. R., and Sreelathakumary, I. (2002). Quality parameters in hot chilli (Capsicum chinense J.). J. Trop. Agric. 40, 7–10. Available online at: http://jtropag.kau.in/index.php/ojs2/article/view/66
Márquez, A. L., Real, R., and Vargas, J. M. (2004). Dependence of broad-scale geographical variation in fleshy-fruited plant species richness on disperser bird species richness. Global Ecol. Biogeogr. 13, 295–304. doi: 10.1111/j.1466-822X.2004.00100.x
Marshall, D. J., Heppell, S. L., Munch, S. B., and Warner, R. R. (2010). The relationship between maternal phenotype and offspring quality: do older mothers really produce the best offspring? Ecology 91, 2862–2873. doi: 10.1890/09-0156.1
Martínez, I., García, D., and Obeso, J. R. (2007). Allometric allocation in fruit and seed packaging conditions the conflict among selective pressures on seed size. Evol. Ecol. 21, 517–533. doi: 10.1007/s10682-006-9132-x
McGraw, J. B., and Caswell, H. (1996). Estimation of individual fitness from life-history data. Am. Nat. 147, 47–64. doi: 10.1086/285839
McKey, D. (1975). “The ecology of evolved seed dispersal systems,” in Coevolution of Animals and Plants, eds L. E. Gilbert and P. H. Raven (Austin, TX: University of Texas Press), 151–159.
Mechlia, N. B., and Carroll, J. J. (1989). Agroclimatic modeling for the simulation of phenology, yield and quality of crop production. Int. J. Biometeorol. 33, 36–473. doi: 10.1007/BF01045896
Meena, O. P., and Bahadur, V. (2014). Assessment of genetic variability, heritability and genetic advance among tomato (Solanum lycopersicum L.) germplasm. Bioscan 9, 1593–1597. Available online at: http://thebioscan.in/Journal%20Supplement/94Sup03%20OM%20PRAKASH%20MEENA.pdf
Michaels, H. J., Benner, B., Hartgerink, A. P., Lee, T. D., Rice, S., Willson, M. F., et al. (1988). Seed size variation: magnitude, distribution, and ecological correlates. Evol. Ecol. 2, 157–166. doi: 10.1007/BF02067274
Morales, J. M., García, G., Martínez, M., Rodríguez-Pérez, J., and Herrera, J. M. (2013). Frugivore behavioural details matter for seed dispersal: a multi-species model for Cantabrian thrushes and trees. PLoS ONE 8:e65216. doi: 10.1371/journal.pone.0065216
Muñoz, M. C., Schaefer, H. M., Böhning-Gaese, K., and Schleuning, M. (2017). Positive relationship between fruit removal by animals and seedling recruitment in a tropical forest. Basic Appl. Ecol. 20, 31–39. doi: 10.1016/j.baae.2017.03.001
Murray, K. G. (1987). Selection for optimal fruit-crop size in bird-dispersed plants. Am. Nat. 129, 18–31. doi: 10.1086/284620
Naor, A., Klein, I., Hupert, H., Grinblat, Y., Peres, M., and Kaufman, A. (1999). Water stress and crop level interactions in relation to nectarine yield, fruit size distribution, and water potentials. J. Amer. Soc. Hortic. Sci. 124, 189–193.
Naor, A., Naschitz, S., Peres, M., and Gal, Y. (2008). Responses of apple fruit size to tree water status and crop load. Tree Physiol. 28, 1255–1261. doi: 10.1093/treephys/28.8.1255
Ordano, M., Blendinger, P. G., Lomáscolo, S. B., Chacoff, N. P., Sánchez, M. S., Núñez-Montellano, M. G., et al. (2017). The role of trait combination in the conspicuousness of fruit display among bird-dispersed plants. Funct. Ecol. 31, 1718–1727. doi: 10.1111/1365-2435.12899
Ortiz-Pulido, R., Albores-Barajas, Y. V., and Díaz, S. A. (2007). Fruit removal efficiency and success: influence of crop size in a neotropical treelet. Plant Ecol. 189, 147–154. doi: 10.1007/s11258-006-9175-7
Ortiz-Pulido, R., and Rico-Gray, V. (2000). The effect of spatio-temporal variation in understanding the fruit crop size hypothesis. Oikos 91, 523–527. doi: 10.1034/j.1600-0706.2000.910314.x
Pagel, M. (1999). Inferring the historical patterns of biological evolution. Nature 401, 877–884. doi: 10.1038/44766
Palacio, F. X., Girini, J. M., and Ordano, M. (2017a). Linking the hierarchical decision-making process of fruit choice and the phenotypic selection strength on fruit traits by birds. J. Plant Ecol. 10, 713–720. doi: 10.1093/jpe/rtw063
Palacio, F. X., Lacoretz, M., and Ordano, M. (2014). Bird-mediated selection on fruit display traits in Celtis ehrenbergiana (Cannabaceae). Evol. Ecol. Res. 16, 51–62. Available online at: http://www.evolutionary-ecology.com/abstracts/v16/2873.html
Palacio, F. X., Valoy, M., Bernacki, F., Sánchez, M. S., Núñez-Montellano, M. G., Varela, O., et al. (2017b). Bird fruit consumption results from the interaction between fruit-handling behaviour and fruit crop size. Ethol. Ecol. Evol. 29, 24–37. doi: 10.1080/03949370.2015.1080195
Parciak, W. (2002). Environmental variation in seed number, size, and dispersal of a fleshy-fruited plant. Ecology 83, 780–793. doi: 10.1890/0012-9658(2002)083[0780:EVISNS]2.0.CO;2
Qian, H., and Jin, Y. (2016). An updated megaphylogeny of plants, a tool for generating plant phylogenies and an analysis of phylogenetic community structure. J. Plant Ecol. 9, 233–239. doi: 10.1093/jpe/rtv047
R Core Team (2017). R: A Language and Environment for Statistical Computing. Vienna: R foundation for Statistical Computing. Available online at: http://www.R-project.org/
Revell, L. J. (2012). phytools: An R package for phylogenetic comparative biology (and other things). Methods Ecol. Evol. 3, 217–223. doi: 10.1111/j.2041-210X.2011.00169.x
Rosenthal, R., and DiMatteo, M. R. (2001). Meta-analysis: recent developments in quantitative methods for literature reviews. Annu. Rev. Psychol. 52, 59–82. doi: 10.1146/annurev.psych.52.1.59
Roussos, P. A., Sefferou, V., Denaxa, N. K., Tsantili, E., and Stathis, V. (2011). Apricot (Prunus armeniaca L.) fruit quality attributes and phytochemicals under different crop load. Sci. Hortic. 129, 472–478. doi: 10.1016/j.scienta.2011.04.021
Ruggera, R. A., Blendinger, P. G., Gomez, M. D., and Marshak, C. (2016). Linking structure and functionality in mutualistic networks: do core frugivores disperse more seeds than peripheral species? Oikos 125, 541–555. doi: 10.1111/oik.02204
Rupinski, M. T., and Dunlap, W. P. (1996). Approximating Pearson-product moment correlations from Kendall's tau and Spearman's rho. Educ. Psychol. Meas. 56, 419–429.
Russo, S. E. (2003). Responses of dispersal agents to tree and fruit traits in Virola calophylla (Myristicaceae): implications for selection. Oecologia 136, 80–87. doi: 10.1007/s00442-003-1239-y
Sadras, V. O. (2007). Evolutionary aspects of the trade-off between seed size and number in crops. Field Crops Res. 100, 125–138. doi: 10.1016/j.fcr.2006.07.004
Sallabanks, R. (1992). Fruit fate, frugivory, and fruit characteristics: a study of the hawthorn, Crataegus monogyna (Rosaceae). Oecologia 91, 296–304. doi: 10.1007/BF00317800
Sallabanks, R. (1993). Hierarchical mechanisms of fruit selection by an avian frugivore. Ecology 74, 1326–1336. doi: 10.2307/1940063
Schaefer, H. M., and Ruxton, G. D. (2011). Plant-Animal Communication. Oxford: Oxford University Press. doi: 10.1093/acprof:osobl/9780199563609.001.0001
Schaefer, H. M., Schaefer, V., and Vorobyev, M. (2007). Are fruit colors adapted to consumer vision and birds equally efficient in detecting colorful signals? Am. Nat. 169, 159–S169. doi: 10.2307/4125309
Schmidt, V., Schaefer, H. M., and Winkler, H. (2004). Conspicuousness, not colour as foraging cue in plant-animal interactions. Oikos 106, 551–557. doi: 10.1111/j.0030-1299.2004.12769.x
Schupp, E. W., Jordano, P., and Gómez, J. M. (2010). Seed dispersal effectiveness revisited: a conceptual review. New Phytol. 188, 333–353. doi: 10.1111/j.1469-8137.2010.03402.x
Schwarzer, G., Carpenter, J., and Rücker, G. (2016). Metasens: Advanced Statistical Methods to Model and Adjust for Bias in Meta-Analysis. Available online at: http://CRAN.R-project.org/package=metasens
Siepielski, A. M., and Benkman, C. W. (2007). Selection by a predispersal seed predator constrains the evolution of avian seed dispersal in pines. Funct. Ecol. 21, 611–618. doi: 10.1111/j.1365-2435.2007.01261.x
Smith, C. C., and Fretwell, S. D. (1974). The optimal balance between size and number of offspring. Am. Nat. 108, 499–506. doi: 10.1086/282929
Snow, D. W. (1971). Evolutionary aspects of fruit eating by birds. Ibis 113, 194–202. doi: 10.1111/j.1474-919X.1971.tb05144.x
Sobral, M., Guitián, J., Guitián, P., and Larrinaga, A. R. (2013). Selective pressure along a latitudinal gradient affects subindividual variation in plants. PLoS ONE 8:e74356. doi: 10.1371/journal.pone.0074356
Sobral, M. A., Larrinaga, A. R., and Guitián, J. (2010). Do seed-dispersing birds exert selection on optimal plant trait combinations? Correlated phenotypic selection on the fruit and seed size of hawthorn (Crataegus monogyna). Evol. Ecol. 24, 1277–1290. doi: 10.1007/s10682-010-9380-7
Stopar, M., Bolcina, U., Vanzo, A., and Vrhovsek, U. (2002). Lower crop load for cv. Jonagold apples (Malus × domestica Borkh.) increases polyphenol content and fruit quality. J. Agric. Food Chem. 50, 1643–1646. doi: 10.1021/jf011018b
Stournaras, K. E., and Schaefer, H. M. (2017). Does flower and fruit conspicuousness affect plant fitness? Contrast, color coupling and the interplay of pollination and seed dispersal in two Vaccinium species. Evol. Ecol. 31, 229–247. doi: 10.1007/s10682-016-9864-1
Strauss, S. Y., and Irwin, R. E. (2004). Ecological and evolutionary consequences of multispecies plant-animal interactions. Annu. Rev. Ecol. Evol. Syst. 35, 435–466. doi: 10.1146/annurev.ecolsys.35.112202.130215
Tella, J. L., Baños-Villalba, A., Hernández-Brito, D., Rojas, A., Pacífico, E., Díaz-Luque, J. A., et al. (2015). Parrots as overlooked seed dispersers. Front. Ecol. Environ. 13, 338–339. doi: 10.1890/1540-9295-13.6.338
Tewksbury, J. J. (2002). Fruits, frugivores and the evolutionary arms race. New Phytol. 156, 137–139. doi: 10.1046/j.1469-8137.2002.00522.x
Thompson Scientific (2009). ISI Web of Knowledge. Available online at: http://isiwebofknowledge.com
Ting, S., Hartley, S., and Burns, K. (2008). Global patterns in fruiting seasons. Global Ecol. Biogeogr. 17, 648–657. doi: 10.1111/j.1466-8238.2008.00408.x
Traveset, A., and Verdú, M. (2002). “A meta-analysis of the effect of gut treatment on seed germination,” in Seed Dispersal and Frugivory: Ecology, Evolution, and Conservation, eds D. J. Levey, W. R. Silva, and M. Galetti (Wallingford: CAB International), 339–350.
Tummers, B. (2010). Data Thief III. Available online at: http://datathief.org/
Verdú, M., and Traveset, A. (2004). Bridging meta-analysis and the comparative method: a test of seed size effect on germination after frugivores' gut passage. Oecologia 138, 414–418. doi: 10.1007/s00442-003-1448-4
Vietchtbauer, W. (2010). Conducting meta-analyses in R with the metafor package. J. Stat. Softw. 36, 1–48. Available online at: http://brieger.esalq.usp.br/CRAN/web/packages/metafor/vignettes/metafor.pdf
Wang, B. C., and Smith, T. B. (2002). Closing the seed dispersal loop. Trends Ecol. Evol. 17, 379–386. doi: 10.1016/S0169-5347(02)02541-7
Webb, C. O., and Donoghue, M. J. (2005). Phylomatic: tree assembly for applied phylogenetics. Mol. Ecol. Res. 5, 181–183. doi: 10.1111/j.1471-8286.2004.00829.x
Westoby, M., Jurado, E., and Leishman, M. (1992). Comparative evolutionary ecology of seed size. Trends Ecol. Evol. 7, 368–372. doi: 10.1016/0169-5347(92)90006-W
Wheelwright, N. T. (1985). Fruit size, gape width, and the diets of fruit eating birds. Ecology 66, 808–818. doi: 10.2307/1940542
Wheelwright, N. T. (1993). Fruit size in a tropical tree species: variation, preference by birds, and heritability. Vegetatio 107/108, 163–174. doi: 10.1007/978-94-011-1749-4_11
Wheelwright, N. T., and Orians, G. H. (1982). Seed dispersal by animals: contrasts with pollen dispersal, problems of terminology, and constraints on coevolution. Am. Nat. 119, 402–413. doi: 10.1086/283918
Willson, M. F., and Whelan, C. J. (1990). The evolution of fruit color in fleshy-fruited plants. Am. Nat. 136, 790–809. doi: 10.1086/285132
Willson, M. F., and Whelan, C. J. (1993). Variation of dispersal phenology in a bird-dispersed shrub, Cornus drummondii. Ecol. Monogr. 63, 151–172.
Witmer, M. C., and Van Soest, P. J. (1998). Contrasting digestive strategies of fruit-eating birds. Funct. Ecol. 12, 728–741. doi: 10.1046/j.1365-2435.1998.00242.x
Zamora, R. (2000). Functional equivalence in plant-animal interactions: ecological and evolutionary consequences. Oikos 88, 442–447. doi: 10.1034/j.1600-0706.2000.880222.x
Keywords: frugivory, mutualism, phenotypic selection, plant-animal interactions, seed dispersal
Citation: Palacio FX and Ordano M (2018) The Strength and Drivers of Bird-Mediated Selection on Fruit Crop Size: A Meta-Analysis. Front. Ecol. Evol. 6:18. doi: 10.3389/fevo.2018.00018
Received: 20 October 2017; Accepted: 14 February 2018;
Published: 28 February 2018.
Edited by:
Marco A. Molina-Montenegro, University of Talca, ChileReviewed by:
Robert Bagchi, University of Connecticut, United StatesRodrigo S. Rios, University of La Serena, Chile
Constanza Celedón Neghme, Independent Researcher, Spain
Copyright © 2018 Palacio and Ordano. This is an open-access article distributed under the terms of the Creative Commons Attribution License (CC BY). The use, distribution or reproduction in other forums is permitted, provided the original author(s) and the copyright owner are credited and that the original publication in this journal is cited, in accordance with accepted academic practice. No use, distribution or reproduction is permitted which does not comply with these terms.
*Correspondence: Facundo X. Palacio, ZmFjdW5kb19wYWxhY2lvQGZjbnltLnVubHAuZWR1LmFy
†Present Address: Facundo X. Palacio, Sección Ornitología, División Zoología Vertebrados, Facultad de Ciencias Naturales y Museo, La Plata, Argentina