- 1Department of Plant Pathology, The Ohio State University, Columbus, OH, United States
- 2Department of Entomology, University of Wisconsin-Madison, Madison, WI, United States
- 3Dorena Genetic Resource Center, USDA Forest Service, Cottage Grove, OR, United States
- 4Department of Entomology, Ohio Agricultural Research and Development Center, The Ohio State University, Wooster, OH, United States
- 5Northern Research Station, USDA Forest Service, Morgantown, WV, United States
- 6School of Forest Resources and Conservation, University of Florida, Gainesville, FL, United States
• Invasive pathogens that cause stem cankers and wilts of trees, and insects that bore into bark and wood have proven extremely destructive to the world's forests and particularly difficult to manage once established
• Such pests are especially devastating when evolutionarily naïve host trees lack adequate natural defenses
• Modern tree improvement programs are increasingly capable of restoring such defenses and providing trees for planting that are capable of withstanding alien insects and pathogens
• Careful target selection, early implementation and sustained support of these programs are important for their success
• We provide a simplified framework to guide responses to invasive insects and pathogens and rapidly identify promising pests to target for development of host resistance.
Introduction
Invasions by non-native phytophagous insects and phytopathogens (henceforth, non-native PIPs) have inflicted dramatic economic and ecological damage on forest ecosystems worldwide (Figures 1a,b) (e.g., Lovett et al., 2016). Many such PIPs, including most pathogens as well as adelgids, bark-, and wood-boring insects, are embedded in plant tissues, resulting in intimate associations with their hosts that hinder early detection and eradication (Figures 1c–f). This is particularly true for pathogens and insects that lack long-distance pheromones that could otherwise be used in detection and monitoring (Liebhold et al., 2016). Indeed, many such non-native PIPs are discovered only once eradication or containment are no longer feasible. These intimately associated non-native PIPs are often lethal to trees because they damage critical host tissues in non-adapted hosts that lack adequate defenses (Mattson et al., 1988) (Figures 1g,h). In many cases, effective strategies for management and impact mitigation of such non-native PIPs remain elusive despite intense concerted efforts.
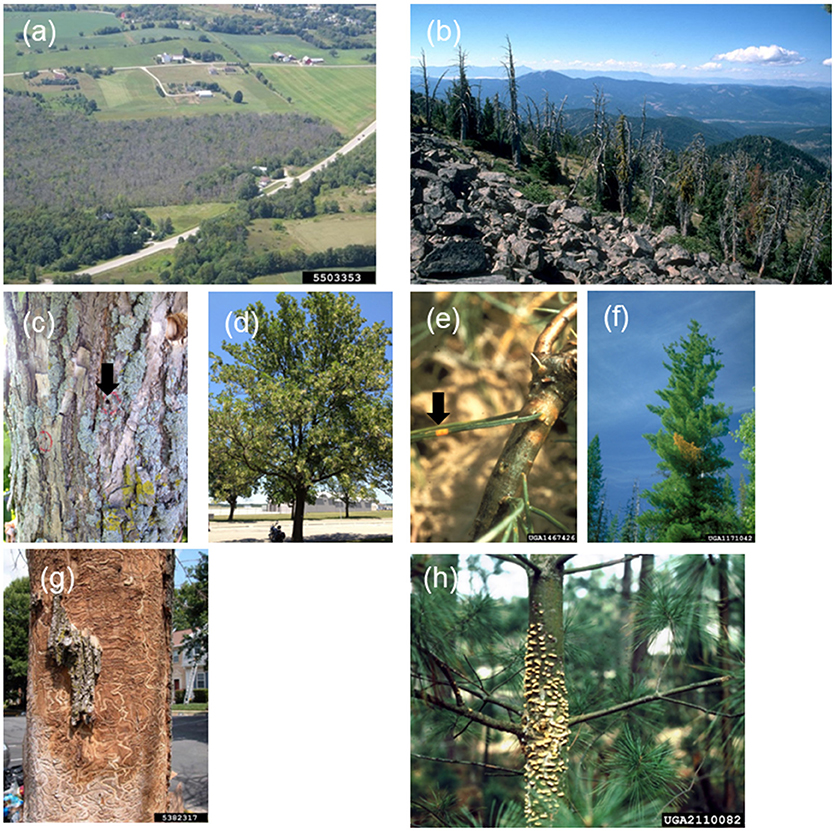
Figure 1. Examples of landscape-, tree-, and tissue-scale presentations of tree-killing non-native phytophagous insects and phytopathogens. Landscape-scale tree mortality resulting from (a) emerald ash borer and (b) white pine blister rust. Problematic hidden nature of infestations, such as (c) small adult beetle emergence holes and (d) non-descript canopy thinning seen in early emerald ash borer infestation, or (e) small lesions and (f) branch flagging seen in early white pine blister rust disease development. (g,h) Damage to critical vascular tissues or meristems leads to rapid decline and death of trees without adequate defenses. Image credits: (a) Bill McNee, Wisconsin Dept of Natural Resources, Bugwood.org. (b) Dave Powell, USDA Forest Service (retired), Bugwood.org. (e) USDA Forest Service – Ogden, Bugwood.org. (f) Chris Schnepf, University of Idaho, Bugwood.org. (g) Eric R. Day, Virginia Polytechnic Institute and State University, Bugwood.org. (h) Frantisek Soukup, Bugwood.org.
The iconic cases of chestnut blight, caused by the introduced fungus Cryphonectria parasitica (Anagnostakis, 1987), and more recently emerald ash borer (Agrilus planipennis, EAB) (Herms and McCullough, 2014) exemplify such devastating non-native PIPs in North America, while red turpentine beetle (Dendroctonus valens) (Yan et al., 2005) and pine wood nematode (Bursaphelenchus xylophilus) (Fielding and Evans, 1996) are examples that have invaded Eurasian forests. Additionally, outbreaks of native and/or exotic PIPs in plantations comprised of exotic tree species present major challenges to commercial forestry (Wingfield et al., 2015).
We argue that when integrated into an ecologically-informed proactive management response framework, modern programs to develop host resistance can enable long-term management of non-native PIP invasions and the conservation of affected tree species and forest ecosystems. Recent reviews have described the promise of harnessing naturally occurring host resistance of affected tree populations (e.g., Telford et al., 2015) and the process of resistance development through selection and breeding (e.g., Sniezko and Koch, 2017). Others have described successful programs against non-native invasive forest PIPs (Sniezko, 2006), and outlined scientific and technological advances that make discovery and development of host resistance increasingly feasible (Boshier and Buggs, 2015; Woodcock et al., 2017). In this context, we seek to provide a more robust framework for early identification of candidate non-native PIP-host systems for resistance development. We maintain that prospects for host resistance are most promising when:
• The invading non-native PIP appears to be controlled primarily by bottom-up regulation via host defenses in its native region;
• There are substantial differences in host resistance and/or tolerance between trees in the introduced vs. native regions;
• There are practical methods for deploying resistant genotypes across the host species range;
• Stakeholders are committed to preserving or restoring long-term cultural, ecological, and/or economic values unique to the affected tree species or forest ecosystem.
Conceptual Framework for Management Response
Host resistance development is part of a larger response to forest pest invasions. Such invasions progress through introduction, establishment, spread, and population outbreak stages (Blackburn et al., 2011). However, for non-native PIPs discovered after they have established and spread within naïve forest ecosystems, constraints to population outbreaks are critical for minimizing tree mortality and ecosystem impacts.
In general, herbivore populations are constrained by top-down forces exerted by natural enemies (predators, parasitoids, pathogens), bottom-up forces exerted by quantity and quality of food, and general forces associated with abiotic factors. Two complementary hypotheses that explain population outbreaks of invading herbivores following release from biotic resistance are the enemy-free space hypothesis in which invader populations are weakly regulated by natural enemies (Jeffries and Lawton, 1984), and the defense-free space hypothesis in which population growth and spread of the invader is facilitated by low resistance of evolutionarily naïve host plants (Gandhi and Herms, 2010). Along with other factors, like spread potential (Gonthier and Garbelotto, 2013) and host connectivity (Prospero and Cleary, 2017), the degree to which each hypothesis explains the population dynamics of an invading organism can inform how to best manage that invasion once populations become established.
Ideally, agencies and policy-makers attempt to use evidence-based decision processes to respond rapidly to high-impact non-native PIPs. Actual implementation is fraught with difficulties, however, in part because the urgency and need for emergency responses can lead to relatively ad hoc reactions, whereas a firmer framework could improve responses to the long-term challenge. This response framework, summarized in Figure 2, can be applied on an international level, and most elements can be scaled down to management of individual forests. The proposed framework aims to simplify and unify previous frameworks (Dodd et al., 2005; Keane et al., 2012; Dix et al., 2013; Jacobs et al., 2013; Nelson et al., 2014; Millar and Stephenson, 2015; Sniezko and Koch, 2017; Woodcock et al., 2017).
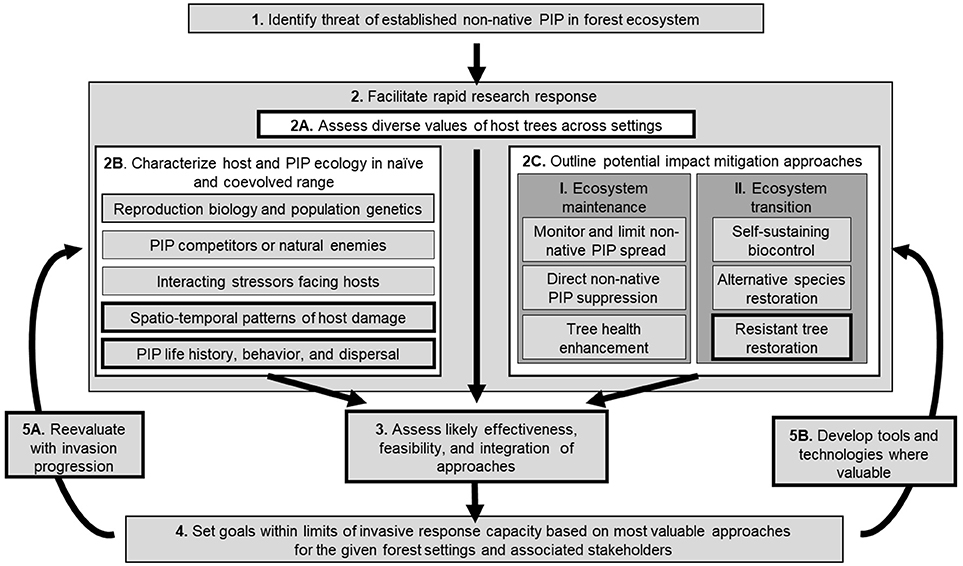
Figure 2. Contextualizing host resistance development within the generalized response framework for established, non-native forest phytophagous insects and phytopathogens (non-native PIPs). (1) Once a non-native PIP is identified as established in a forest ecosystem, research responses (2) must assess cultural, economic, and ecological value of hosts in various settings (2A), characterize the invasion ecology in native and introduced ranges to assess impact risk (2B), and outline available short- and long-term impact mitigation approaches (2C). (3) Likely costs and benefits of various approaches are estimated with available data, and (4) goals are set within limits of current response capacity for the forest ecosystem under consideration. Response goals are periodically revised through the framework (5), with reevaluation of invasion progression (5A) and the development of new mitigation strategies or technologies (5B). Bolded boxes identify key steps in identifying good non-native PIP candidates for host resistance development: non-native PIPs threaten substantial long-term ecological and economic value; non-native PIPs are intimately associated with hosts and damage critical tissues resulting in rapid tree mortality; capacity for restoration using improved resistant germplasm exists or can be developed; mechanisms exist to reevaluate responses as invasion progresses and technologies develop.
The proposed framework begins with the establishment of a non-native PIP (Figure 2, box 1), i.e., when eradication efforts are predicted or concluded to be unsuccessful, at which point three research goals are pursued. The first is to determine the value of products and services under threat (Figure 2, box 2A) so that a proportionate response can be implemented and stakeholders identified. Valuations include timber, recreation, ecosystem services, biodiversity, cultural significance, and contribution to ecosystem resilience (Ellison et al., 2005; Ferguson, 2006; Freer-Smith and Webber, 2017).
The second research goal is to characterize PIP ecology in its native and introduced ranges (van Kleunen et al., 2010) (Figure 2, box 2B). Little is known about many PIPs in their native range, where they often are much less damaging and therefore little studied (GAO, 2006). Key knowledge gaps at this stage that will guide management decisions include PIP life history and behavior, dispersal mechanisms, taxonomic host range, symbionts, generalist and specialist natural enemies, and patterns of host connectivity, damage and mortality over time and space (Dodd et al., 2005; Gonthier and Garbelotto, 2013; Prospero and Cleary, 2017; Woodcock et al., 2017). Specifically, it is important to determine the degrees to which PIP population dynamics, and resulting tree damage and mortality in native and introduced ecosystems, are associated with bottom-up factors (tree genetics, ontogeny, drought stress) and top-down factors (natural enemy population dynamics and behavior).
The third research goal is to identify available impact mitigation approaches, and to assess their feasibility and likely effectiveness in preserving the value of the threatened host. Forest health managers employ combinations of two disturbance impact mitigation approaches (Millar and Stephenson, 2015): ecosystem maintenance and ecosystem transition (Figure 2, box 2CI, II).
Ecosystem maintenance is a short-term strategy intended to temporarily strengthen barriers to non-native PIP spread, suppress non-native PIP populations below outbreak/epidemic levels, and preserve populations of healthy trees, thereby delaying (but not preventing) costs associated with tree death and with transitions to a new forest community. Such an approach may employ chemical or biological pesticides, pheromone-based mating disruption or trap-out, removal of infested hosts (sanitation), augmentative/ non-persistent introductions of competitors or natural enemies, and/or silvicultural practices intended to enhance existing host defenses and tolerances and/or inhibit non-native PIP growth or spread (Figure 2, box 2CI).
An ecosystem transition approach seeks to facilitate conversion of the forest ecosystem to one that is less prone to non-native PIP outbreaks, and/or restore cultural, ecological and/or economic value lost to the invasion. These approaches revolve around identifying, developing, and deploying resistant populations of the affected host tree species and/ or establishing self-sustaining populations of antagonists or natural enemies of non-native invasive PIPs (classical biocontrol). (Figure 2, box 2CII). Additionally, forest ecosystems may be restored with alternative non-host species, though at the expense of any unique cultural or ecological values of the original affected species.
Assessments of host tree value and invasion ecology provide the basis for predicting the effectiveness, feasibility, and integration of proposed mitigation approaches (Figure 2, box 3). Effectiveness is measured as the ability to reduce invasion impact by preventing, delaying, or restoring diverse values lost to tree damage or mortality. For example, restoration using resistant trees can preserve biodiversity and/or restore cultural value or ecosystem resilience associated with a species or population, but is generally not effective in preventing costs associated with tree mortality, such as removal or lost timber yield.
Feasibility represents the capacity required to implement effective mitigation, including time, labor, capital, expertise, and political will. For instance, resistant tree restoration requires both available resistant germplasm and mechanisms for deployment in affected ecosystems (Jacobs et al., 2013). Integration of mitigation tactics can improve effectiveness and/or feasibility (Suckling et al., 2012). An example is the SLow Ash Mortality (SLAM) pilot program for EAB, which integrates multiple maintenance tactics to reduce and delay costs associated with EAB-induced ash mortality in urban and natural forests (McCullough and Mercader, 2012). As a transition approach, deployment of resistance could increase the probability of establishing or enhancing self-sustaining populations of natural enemies of non-native invasive PIPs (classical biological control) (Price et al., 1980; Barbosa et al., 2009).
Public and private stakeholders should be engaged to select approaches within the available response capacity for a given forest, and those approaches should be prioritized using criteria of effectiveness, feasibility and integration (Figure 2, box 4). A balance is required between maintenance and transition approaches that reflects the short- and long-term value of the affected tree species and forest. For urban or plantation forests, this may require primarily a maintenance approach that preserves existing trees and delays costs associated with their mortality. However, in natural forests, especially those in which foundational or keystone tree species are directly affected by the invasion (e.g., high elevation white pines affected by white pine blister rust in the Rocky Mountains) the importance of long-term resilience of the forest ecosystem may more strongly favor an ecosystem transition approach (Schoettle and Sniezko, 2007). The research and goal-setting process should be repeated as new information is gathered while the invasion progresses (Figure 2, box 5A), and/or as new mitigation tools and technologies become available (Figure 2, box 5B). In addition to advocating for periodic re-evaluation as an invasion progresses, in the following section we identify early invasion characteristics that may help determine when host resistance development and deployment should be pursued.
Decision Making With Limited Information
Non-native PIP Traits That Constrain Mitigation Approaches
Features of non-native PIP biology and invasion ecology may help predict the effectiveness of ecosystem transition approaches. By limiting outbreaks and reducing tree mortality, classical biological control has been an effective ecosystem transition response against approximately 13 phytophagous insects in native US forests and a partial success against four more (Van Driesche et al., 2010; MacQuarrie et al., 2016). There are additional examples worldwide. However, these successes have come primarily against foliage-feeding insects, which are outside the focus of this paper. In contrast, there are fewer examples of effective biological control against bark- and wood-boring insects (Fielding and Evans, 1997; Slippers et al., 2015), and canker- and wilt-inducing pathogens (Milgroom and Cortesi, 2004). In an extensive review, the success rate of introduced parasitoids of agricultural and forest insect pests was twice as high against leaf-feeding Lepidoptera and Coleoptera as against wood-borers and root-feeders, although ecosystem and pest taxonomy exerted strong interacting effects (Gross et al., 2005). Specialist natural enemies are preferred for biological control due to their limited non-target impacts on other organisms (MacQuarrie et al., 2016), and these specialists often exert negative density-dependent effects on PIP populations (Elkinton, 2008). However, population responses of specialist natural enemies to their prey usually exhibit time lags. When density dependent effects of natural enemies are delayed and/or their population increase is slow, they may not regulate tree-killing non-native PIPs effectively or slow tree mortality during outbreaks, especially when host resistance or tolerance to the invader is low.
EAB provides a good example of this phenomenon: biological control agents will likely be insufficient to allow North American ashes to persist as dominant tree species without improved tree resistance (Liu et al., 2003; Herms and McCullough, 2014). Woodpeckers and native and introduced parasitoids have caused some EAB larval mortality in late stages of EAB infestation (Duan et al., 2014; Jennings et al., 2015), and additionally parasitoids may promote survival of ash saplings following outbreak (Margulies et al., 2017), but the long-term impacts on ash populations are still unknown (Duan et al., 2017). Despite the presence of a full complement of coevolved natural enemies in Asia, EAB has caused high mortality of North American ash species planted there (Yang et al., 2010; Duan et al., 2015). EAB population models predict that both increased rates of parasitism by natural enemies and higher levels of host resistance are required to maintain EAB populations at equilibrium levels low enough to support reproducing populations of North American ash species (Duan et al., 2015). As a further example, biological control of Sirex noctilio by entomopathogenic nematodes is especially successful when integrated with silvicultural practices (Slippers et al., 2015) that may improve innate host resistance (Dodds et al., 2007). Therefore, biological control may act synergistically with effective tree defenses to prevent or reduce mortality caused by tree-killing non-native PIPs (Price et al., 1980; Duan et al., 2015). Biological control is best applied to systems in which the hosts can at least partially resist or tolerate non-native PIP attack, especially if negative density-dependent responses of natural enemies are slow relative to how long it takes the non-native PIP to kill trees.
Non-native PIP Traits Associated With Outbreaks in Defense-Free Space
As introduced above, decisions about how to manage an established invasive forest non-native PIP population can be guided by the enemy-free space (Jeffries and Lawton, 1984) and/or the defense-free space (Gandhi and Herms, 2010) hypotheses. These two hypotheses describe a continuum of top-down and bottom-up biotic forces that constrain the population growth of heterotrophic organisms (Figure 3A). Mattson et al. (1988) associated certain plant defense syndromes, and thus importance of top-down or bottom-up factors, with key host and herbivorous insect traits. We propose an extension of these same concepts to phytopathogens (Figure 3B).
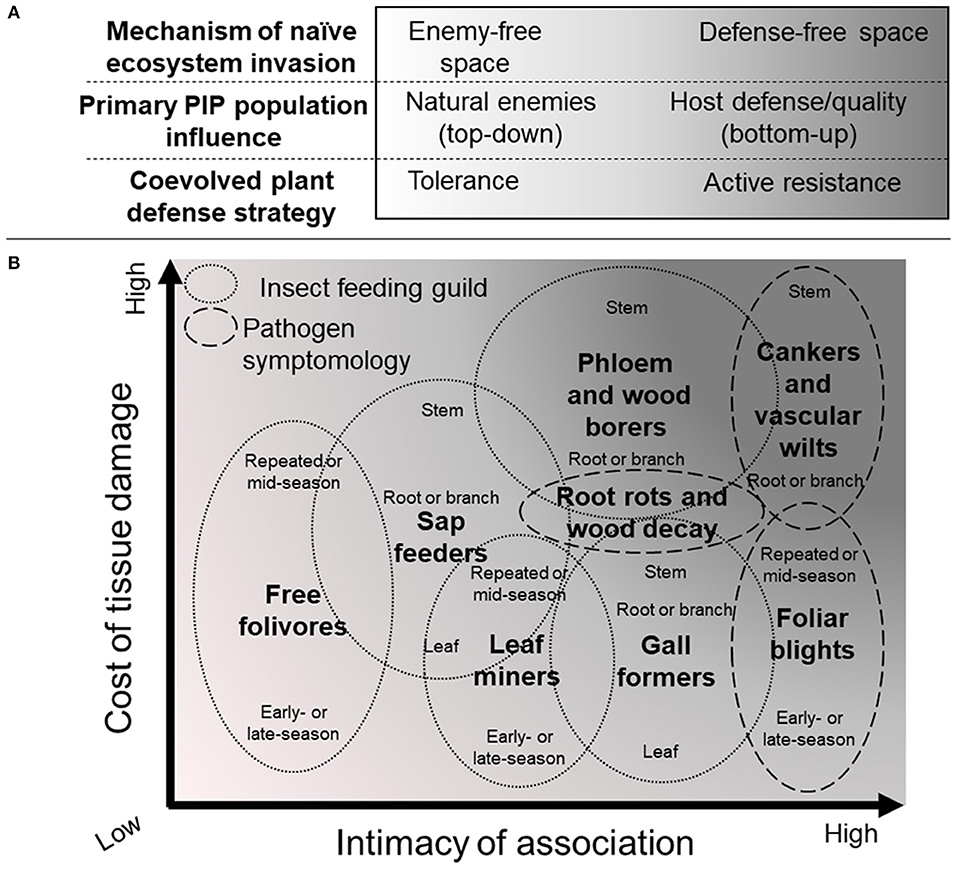
Figure 3. Effective plant defense strategies and associated phytophagous insect and phytopathogen (PIP) traits. (A) Plant defense strategies and corresponding non-native PIP population influences and invasion mechanisms vary along a continuum. (B) Features of the PIP-host interaction, intimacy and damage, are associated with the strategy/influence/mechanism continuum, and with feeding guild, symptomology, phenology, and host tissue. Increasing shading indicates the likely importance of host resistance development in managing invasions of defense-free space. The pests emphasized in this review, i.e., phloem- and wood-borers and cankers and vascular wilts, occupy the darkest shaded, upper right region. Synthesized and adapted from Mattson et al. (1988).
At one end of the host/PIP interaction spectrum, plants tolerate PIP damage while environmental factors or natural enemies reduce PIP populations and associated damage (Figure 3A). Under this scenario, non-native PIP outbreaks may occur in naïve forest ecosystems if effective natural enemies are not present, i.e., in enemy-free space. At the other end of the continuum, plant defense traits constrain the population growth of the PIP, thereby limiting damage (Figure 3A). When non-native PIPs that have evolved under these constraints become established in a naïve forest ecosystem, outbreaks may occur in the absence of host defenses sufficient to reduce populations below damaging levels, i.e., in defense-free space. Plant defense traits –such as expression of specific recognition and signaling proteins, or expression and activation of biosynthetic enzymes for toxin production or cell wall strengthening– can incur fitness costs of varying magnitude. Therefore, a corresponding fitness benefit –such as reduced herbivory or resource depletion– must be present for the traits to persist in a host population (Herms and Mattson, 1992; Heil, 2002). Thus, the consequences of herbivory or infection, including fitness cost or resulting mortality rate, is the first useful criterion for estimating the likelihood that host resistance traits are present in a coevolved plant-insect or plant-microbe interaction.
If defense traits are important in regulating a coevolved interaction, then restoring them will be important for effective management of invasions in defense-free space (Figure 3B). A number of PIP traits can influence consequences of host damage, but the type of host tissue affected is among the most important (Mattson et al., 1988) (Figure 3B). Foliage feeders and foliar blights are usually among the least damaging, as defoliation can be tolerated relatively easily, especially by deciduous plants (Krause and Raffa, 1996). Next most impactful are PIPs that affect branches or peripheral roots, either through nutrient removal or tissue destruction, as in the case of root rots. The most damaging PIPs affect the main stem and/or main roots, which can lead to rapid tree decline and death. Particularly damaging pests are those that interfere with the potential for recovery by damaging meristematic tissue (e.g., buds, root tips, or vascular cambium) at critical junctures in the tree developmental cycle or, in the case of insects, by vectoring or facilitating infection by phytopathogens or egesting toxins. Bark- and wood-boring insects, as well as vascular wilt- or canker-inducing pathogens, are among the most damaging classes of invasive non-native PIPs, evidenced by their ability to rapidly kill host trees with ineffective defenses.
The intimacy of the association between host and PIP is another criterion useful for predicting impact (Figure 3B). Intimacy of the interaction may be less precise, but can be estimated as the proportion of the PIP lifecycle during which the PIP is in direct contact with living host tissue, the amount and nature of PIP tissues in direct contact with the host, and the extent to which plant losses extend beyond the point of actual nutrient transfer between host and parasite (e.g., some galls represent highly intimate but relatively benign associations) (Mattson et al., 1988). For example, some less intimately associated PIPs may interact with host tissues for a large portion of their life cycle but only via feeding structures, be they mouth-parts of leaf chewing insects, or appressoria and haustoria of powdery mildew fungi. In more intimate associations, such as those involving bark and wood-boring insects as well as vascular wilt- or canker-inducing pathogens, PIPs may be entirely surrounded by living host tissue for a large portion of their life cycles, thus extending the opportunity to exchange specific molecular information (effectors, phytoalexins, etc.) that may be integrated into signaling, defense, and counter-defense responses. These intimate and damaging associations are well explained by the defense-free space hypothesis. Therefore, development and deployment of host resistance is likely to be highly beneficial.
Actionable Recommendations and Conclusions
Non-native phytophagous insects and phytopathogens in defense-free space continue to devastate the world's forests. Reestablishment of bottom-up regulation of these non-native PIPs through development and deployment of host resistance is essential to preserving the long-term value of healthy forests. Timely and accelerated study of PIP and host ecology in the native and introduced ranges as soon as an invasive non-native PIP is recognized can help determine when defense-free space is the primary driver behind damage, and thereby prioritize interventions accordingly. Such rapid response is increasingly feasible with modern tree improvement programs (Boshier and Buggs, 2015; Sniezko and Koch, 2017). However, to achieve genetically diverse, resilient tree populations requires substantial investment in programs aimed at developing host resistance as soon as eradication of lethal non-native PIPs invading defense-free space is determined to be impossible. In addition to protecting trees directly, resistance may also improve the efficacy of biological control as part of an integrated response to invasive forest insects (Price et al., 1980; Duan et al., 2015).
Knowledge gaps include challenges associated with the integration of improved germplasm into naturally regenerating forest ecosystems that are managed by diverse stakeholders. Thus, resistance development programs should be carefully linked to restoration research and planning to ensure that improved germplasm is matched ecologically and silviculturally to management objectives (Jacobs et al., 2013; Sniezko and Koch, 2017; Woodcock et al., 2017). There is also a need to incorporate emerging understanding of associational (Plath et al., 2012) and landscape (Haas et al., 2015) resistance into restoration strategies.
Experience has shown that relying on short-term emergency funding for research and development reduces the prospects for effective, long-term, ecosystem protection from non-native PIPs invading defense-free space. The few historical examples of successful operational deployment of trees resistant to such non-native PIPs have benefitted from long-term support necessary for program continuity (Sniezko, 2006). The prospects of integrating host resistance into responses against future invasive insect pests and pathogens will be increased where an established system is in place to:
• Proactively adopt or amend a clearly defined response framework to minimize ad hoc responses;
• Rapidly facilitate research to fill knowledge gaps in invasive non-native PIP-host tree ecology with the objective of informing the management response framework;
• Apply a long-term capacity of expertise, infrastructure, and public engagement to enable rapid and sustained resistance discovery and development when such a response is deemed valuable;
• Prioritize early integration of management responses, including biological control, host resistance development, and ecosystem restoration, to maximize compatibility and efficiency.
The above capabilities will be more effective where infrastructure is supported on a sustained and global scale, including field sites and biosafety level facilities staffed with tree geneticists and breeders collaborating with pathologists and entomologists. Success requires early and sustained support of host resistance programs through feasibility assessment, trait discovery, development, and sustainable deployment. Significantly greater commitment and resolve from government agencies and the public than presently available are essential (Boshier and Buggs, 2015; Nelson and Koch, 2017; Sniezko and Koch, 2017; Woodcock et al., 2017).
Author Contributions
PB and JS conceived of the manuscript which was developed and prepared by DS. All authors contributed to writing the manuscript, provided critical review during development, and read and approved the submitted version.
Conflict of Interest Statement
DH was employed by The Ohio State University during the conception and writing of this manuscript, and joined The Davey Tree Expert Company shortly before submission.
The remaining authors declare that the research was conducted in the absence of any commercial or financial relationships that could be construed as a potential conflict of interest.
Acknowledgments
We are grateful to the attendees of the 2015 Conference on the Genetics of Tree-Parasite Interactions in Orleans, France for thoughtful discussion and debate of the concepts in this manuscript. This work was partially supported by state and federal funds appropriated to The Ohio State University, and the Ohio Agricultural Research and Development Center, and by the University of Wisconsin Vilas and Sorenson Foundations.
References
Anagnostakis, S. L. (1987). Chestnut blight: the classical problem of an introduced pathogen. Mycologia 79, 23–37. doi: 10.2307/3807741
Barbosa, P., Hines, J., Kaplan, I., Martinson, H., Szczepaniec, A., and Szendrei, Z. (2009). Associational resistance and associational susceptibility: having right or wrong neighbors. Ann. Rev. Ecol. Evo. Sys. 40, 1–20. doi: 10.1146/annurev.ecolsys.110308.120242
Blackburn, T. M., Pyšek, P., Bacher, S., Carlton, J. T., Duncan, R. P., Jarošík, V., et al. (2011). A proposed unified framework for biological invasions. Tr. Ecol. Evol. 26, 333–339. doi: 10.1016/j.tree.2011.03.023
Boshier, D., and Buggs, R. J. A. (2015). The potential for field studies and genomic technologies to enhance resistance and resilience of British tree populations to pests and pathogens. Forestry 88, 27–40. doi: 10.1093/forestry/cpu046
Dix, M. E., Bass, B., Covel, S. A., Bautista, S., Britton, K. O., Finch, D. M., et al. (2013). Forest Service National Strategic Framework for Invasive Species Management FS-1017. Available online at: http://www.fs.fed.us/foresthealth/publications/Framework_for_Invasive_Species_FS-1017.pdf (accessed August 10, 2016).
Dodd, R., Frankel, S., Grünwald, N., Hansen, E., Hayden, K., Kubisiak, T., et al. (2005). “Utilizing genetic information to address Phytophthora ramorum/Sudden Oak Death,” in A whitepaper of the USDA Forest Service, Pacific Southwest Research Station. Available online at: http://www.fs.usda.gov/Internet/FSE_DOCUMENTS/stelprdb5280971.pdf (accessed August 18, 2016).
Dodds, K. I., Cooke, R. R., and Gilmore, D. W. (2007). Silvicultural options to reduce pine susceptibility to attack by a newly detected invasive species, Sirex Noctilio. North. J. Appl. For. 24,165–167. doi: 10.1093/njaf/24.3.165
Duan, J. J., Abell, K. J., Bauer, L. S., Gould, J., and Van Driesche, R. G. (2014). Natural enemies implicated in the regulation of an invasive pest: a life table analysis of the population dynamics of the emerald ash borer. Ag. For. Entomol. 16, 406–416. doi: 10.1111/afe.12070
Duan, J. J., Bauer, L. S., and Van Driesche, R. G. (2017). Emerald ash borer biocontrol in ash saplings: the potential for early stage recovery of North American ash trees. For. Ecol. Manag. 394, 64–72. doi: 10.1016/j.foreco.2017.03.024
Duan, J. J., Van Driesche, R. G., Bauer, L. S., Kashian, D. M., and Herms, D. A. (2015). “Risk to ash from emerald ash borer: can biological control prevent the loss of ash stands?” in Biology and Control of Emerad Ash Borer, eds R. G. Van Driesche, R. R. Reardon (USDA Forest Service, Forest Health Technology Enterprise), 153–163. Available online at: http://www.fs.fed.us/foresthealth/technology/pdfs/FHTET-2014-09_Biology_Control_EAB.pdf (Accessed August 10, 2016).
Elkinton, J. (2008). “The role of population ecology and population models in biological control,” in Control of Pests and Weeds by Natural Enemies: An Introduction to Biological Control, eds R. G. Van Driesche, M. Hoddle, and T. Center (Malden, MA: Blackwell Publishing Ltd.), 97–114.
Ellison, A. M., Bank, M. S., Clinton, B. D., Colburn, E. A., Elliott, K., Ford, C. R., et al. (2005). Loss of foundation species: consequences for the structure and dynamics of forested ecosystems. Front. Ecol. Env. 3, 479–486. doi: 10.1890/1540-9295(2005)003[0479:LOFSCF]2.0.CO;2
Ferguson, K. (2006). The true value of forests. Front. Ecol. Env. 4, 456–456. doi: 10.1890/1540-9295(2006)4[452:D]2.0.CO;2
Fielding, N. J., and Evans, H. F. (1996). The pine wood nematode Bursaphelenchus xylophilus (Steiner and Buhrer) Nickle (= B. lignicolus Mamiya and Kiyohara): an assessment of the current position. Forestry 69, 35–46. doi: 10.1093/forestry/69.1.35
Fielding, N. J., and Evans, H. F. (1997). Biological control of Dendroctonus micans (Scolytidae) in Great Britain. BioContr. News Info. 18, 51–60.
Freer-Smith, P. H., and Webber, J. F. (2017). Tree pests and diseases: the threat to biodiversity and the delivery of ecosystem services. Biodiv. Conserv. 26, 3167–3181. doi: 10.1007/s10531-015-1019-0
Gandhi, K., and Herms, D. A. (2010). Direct and indirect effects of alien insect herbivores on ecological processes and interactions in forests of eastern North America. Biol. Inv. 12, 389–405. doi: 10.1007/s10530-009-9627-9
GAO (Government Accountability Office) (2006). Invasive Forest Pests: Lessons Learned From Three Recent Infestations May Aid in Managing Future Efforts. Available online at: http://www.gao.gov/assets/250/249776.pdf (accessed August 10, 2016).
Gonthier, P., and Garbelotto, M. (2013). Reducing the threat of emerging infectious diseases of forest trees. CAB Rev. 8, 1–2. doi: 10.1079/PAVSNNR20138025
Gross, P., Hawkins, B. A., Cornell, H. V., and Hosmane, B. (2005). Using lower trophic level factors to predict outcomes in classical biological control of insect pests. Bas. Appl. Ecol. 6, 571–584. doi: 10.1016/j.baae.2005.05.006
Haas, S. E., Cushman, J. H., Dillon, W. W., Rank, N. E., Rizzo, D. M., and Meentemeyer, R. K. (2015). Effects of individual, community and landscape drivers on the dynamics of a wildland forest epidemic. Ecology 97, 649–660. doi: 10.1890/15-0767.1
Heil, M. (2002). Fitness costs of induced resistance: emerging experimental support for a slippery concept. Tr. Plant Sci. 7, 61–67. doi: 10.1016/S1360-1385(01)02186-0
Herms, D. A., and Mattson, W. J. (1992). The dilemma of plants: to grow or defend. Q. Rev. Biol. 67, 283–335. doi: 10.1086/417659
Herms, D. A., and McCullough, D. G. (2014). Emerald ash borer invasion of North America: history, biology, ecology, impacts, and management. Ann. Rev. Entomol. 59, 13–30. doi: 10.1146/annurev-ento-011613-162051
Jacobs, D. F., Dalgleish, H. J., and Nelson, C. D. (2013). A conceptual framework for restoration of threatened plants: the effective model of American Chestnut (Castanea dentata) reintroduction. New Phytol. 197, 378–393. doi: 10.1111/nph.12020
Jeffries, M. J., and Lawton, J. H. (1984). Enemy free space and the structure of ecological communities. Biol. J. Linn. Soc. 23, 269–286. doi: 10.1111/j.1095-8312.1984.tb00145.x
Jennings, D. E., Duan, J. J., Abell, K., Bauer, L. S., Gould, J. R., Shrewsbury, P. M., et al. (2015). “Life table evaluation of change in emerald ash borer populations due to biological control,” in Biology and Control of Emerad Ash Borer, eds R. G. Van Driesche, R. R. Reardon (USDA Forest Service, Forest Health Technology Enterprise), 153–163. Available online at: http://www.fs.fed.us/foresthealth/technology/pdfs/FHTET-2014-09_Biology_Control_EAB.pdf (accessed August 10, 2016).
Keane, R. E., Tomback, D., Aubry, C., Bower, A. D., Campbell, F. M., Cripps, C. L., et al. (2012). A Range-wide restoration strategy for whitebark pine (Pinus albicaulis). General Techincal Report RMRS-GTR-279. US Forest Service. Available online at: http://www.fs.fed.us/rm/pubs/rmrs_gtr279.pdf (accessed 10 August, 2016).
Krause, S. C., and Raffa, K. F. (1996). Defoliation tolerance affects the spatial and temporal distributions of larch sawfly and natural enemy populations. Ecol. Entomol. 21, 101–111. doi: 10.1046/j.1365-2311.1996.00004.x
Liebhold, A. M., Berec, L., Brockerhoff, E. G., Epanchin-Niell, R. S., Hastings, A., Herms, D. A., et al. (2016). Eradication of invading insect populations: from concepts to applications. Ann. Rev. Entomol. 61, 335–352. doi: 10.1146/annurev-ento-010715-023809
Liu, H. P., Bauer, L. S., Gao, R. T., Zhao, T. H., Petrice, T. R., and Haack, R. A. (2003). Exporatory survey for the emerald ash borer, Agrilus planipennis (Coleoptera: Buprestidae), and its natural enemies in China. Great Lakes Entomol. 36, 191–204.
Lovett, G. M., Weiss, A., Liebhold, A. M., Holmes, T. P., Leung, B., Lambert, K. F., et al. (2016). Nonnative forest insects and pathogens in the United States: impacts and policy options. Ecol. Appl. 26, 1437–1455. doi: 10.1890/15-1176
MacQuarrie, C. J. K., Lyons, D. B., Seehausen, M. L., and Smith, S. M. (2016). A history of biological control in Canadian forests, 1882–2014. Canad. Entomol. S1, S239–S269. doi: 10.4039/tce.2015.66
Margulies, E., Bauer, L., and Ibáñez, I. (2017). Buying time: preliminary assessment of biocontrol in the recovery of native forest vegetation in the aftermath of the invasive emerald ash borer. Forests 8:369. doi: 10.3390/f8100369
Mattson, W. J., Lawrence, R. K., Haack, R. A., Herms, D. A., and Charles, P. J. (1988). “Defensive strategies of woody plants against different insect-feeding guilds in relation to plant ecological strategies and intimacy of association with insects,” in Mechanisms of woody plant defenses against insects, eds W. J. Mattson, J. Levieux, and C. Bernard-Dagan (New York, NY: Springer-Verlag), 3–38.
McCullough, D. G., and Mercader, R. J. (2012). Evaluation of potential strategies to slow ash mortality (SLAM) caused by emerald ash borer (Agrilus planipennis): SLAM in an urban forest. Int. J. Pest Manag. 58, 9–23. doi: 10.1080/09670874.2011.637138
Milgroom, M. G., and Cortesi, P. (2004). Biological control of chestnut blight with hypovirulence: a critical analysis. Ann. Rev. Phytopath. 42, 311–338. doi: 10.1146/annurev.phyto.42.040803.140325
Millar, C. I., and Stephenson, N. L. (2015). Temperate forest health in an era of emerging megadisturbance. Science 349, 823–826. doi: 10.1126/science.aaa9933
Nelson, C. D., and Koch, J. L. (2017). “Institute of forest tree breeding: improvement and gene conservation of iconic tree species in the 21st century,” in Gene Conservation of Tree Species: Banking on the Future. Proceedings of a Workshop. Gen. Tech. Rep. PNW-GTR-963, eds R. A. Sniezko, G. Man, V. Hipkins, K. Woeste, D. Gwaze, J. T. Kliejunas, et al. (Portland, OR: U.S. Department of Agriculture, Forest Service, Pacific Northwest Research Station), 24–27. Available online at: https://www.nrs.fs.fed.us/pubs/55239
Nelson, C. D., Powell, W., Maynard, C., Baier, K. M., Newhouse, A., Merkle, S. A., et al. (2014). “The forest health initiative, American chestnut (Castanea dentata) as a model for forest tree restoration: biological research program,” in Fifth International Chestnut Symposium, eds M. L. Double and W. L. MacDonald (Leuven: International Society of Horticultural Science), 179–189.
Plath, M., Dorn, S., Riedel, J., Barrios, H., and Mody, K. (2012). Associational resistance and associational susceptibility: specialist herbivores show contrasting responses to tree stand diversification. Oecologia 169, 477–487. doi: 10.1007/s00442-011-2215-6
Price, P. W., Bouton, C. E., Gross, P., McPheron, B. A., Thompson, J. N., and Weis, A. E. (1980). Interactions among three trophic levels: influence of plants on interactions between insect herbivores and natural enemies. Ann. Rev. Ecol. Syst. 11, 41–65. doi: 10.1146/annurev.es.11.110180.000353
Prospero, S., and Cleary, M. (2017). Effects of host variability on the spread of invasive forest diseases. Forests 8:80. doi: 10.3390/f8030080
Schoettle, A. W., and Sniezko, R. A. (2007). Proactive intervention to sustain high-elevation pine ecosystems threatened by white pine blister rust. J. For. Res. 12, 327–226. doi: 10.1007/s10310-007-0024-x
Slippers, B., Hurley, B. P., and Wingfield, M. J. (2015). Sirex woodwasp: a model for evolving management paradigms of invasive forest pests. Ann. Rev. Entomol. 60, 601–619. doi: 10.1146/annurev-ento-010814-021118
Sniezko, R. A. (2006). Resistance breeding against nonnative pathogens in forest trees — current successes in North America. Canad. J. Plant Pathol. 28, S270–S279. doi: 10.1080/07060660609507384
Sniezko, R. A., and Koch, J. (2017). Breeding trees resistant to insects and diseases – putting theory into application. Biol. Invas. 19, 3377–3400. doi: 10.1007/s10530-017-1482-5
Suckling, D. M., Tobin, P. C., McCullough, D. G., and Herms, D. A. (2012). Combining tactics to exploit Allee effects for eradication of alien insect populations. J. Econ. Entomol. 105, 1–13. doi: 10.1603/EC11293
Telford, A., Cavers, S., Ennos, R. A., and Cottrell, J. E. (2015). Can we protect forests by harnessing variation in resistance to pests and pathogens? Forestry 88, 3–12. doi: 10.1093/forestry/cpu012
Van Driesche, R., Carruthers, R., Center, T., Hoddle, M. S., Hough-Goldstein, J., Morin, L., et al. (2010). Classical biological control for the protection of natural ecosystems. Biol. Contr. 54, S2–S33. doi: 10.1016/j.biocontrol.2010.03.003
van Kleunen, M., Dawson, W., Schlaepfer, D., Jeschke, J., and Fischer, M. (2010). Are invaders different? A conceptual framework of comparative approaches for assessing determinants of invasiveness. Ecol. Lett. 13, 947–958. doi: 10.1111/j.1461-0248.2010.01503.x
Wingfield, M. J., Brockerhoff, E. G., Wingfield, B. D., and Slippers, B. (2015). Planted forest health: the need for a global strategy. Science 349, 832–836. doi: 10.1126/science.aac6674
Woodcock, P., Cottrell, J. E., Buggs, R. J. A., and Quine, C. P. (2017). Mitigating pest and pathogen impacts using resistant trees: a framework and overview to inform development and deployment in Europe and North America. Forestry 91, 1–16. doi: 10.1093/forestry/cpx031
Yan, Z., Sun, J., Don, O., and Zhang, Z. (2005). The red turpentine beetle, Dendroctonus valens LeConte (Scolytidae): an exotic invasive pest of pine in China. Biodiv. Conserv. 14, 1735–1760. doi: 10.1007/s10531-004-0697-9
Keywords: forest pathogens, forest insects, host plant resistance, biological invasions, defense-free space
Citation: Showalter DN, Raffa KF, Sniezko RA, Herms DA, Liebhold AM, Smith JA and Bonello P (2018) Strategic Development of Tree Resistance Against Forest Pathogen and Insect Invasions in Defense-Free Space. Front. Ecol. Evol. 6:124. doi: 10.3389/fevo.2018.00124
Received: 06 March 2018; Accepted: 02 August 2018;
Published: 03 September 2018.
Edited by:
David Jack Coates, Department of Biodiversity, Conservation and Attractions (DBCA), AustraliaReviewed by:
Luisa Ghelardini, Università degli Studi di Firenze, ItalyPaolo Gonthier, Università degli Studi di Torino, Italy
Thomas Seth Davis, Colorado State University, United States
Copyright © 2018 Showalter, Raffa, Sniezko, Herms, Liebhold, Smith and Bonello. This is an open-access article distributed under the terms of the Creative Commons Attribution License (CC BY). The use, distribution or reproduction in other forums is permitted, provided the original author(s) and the copyright owner(s) are credited and that the original publication in this journal is cited, in accordance with accepted academic practice. No use, distribution or reproduction is permitted which does not comply with these terms.
*Correspondence: David N. Showalter, c2hvd2EwMjhAdW1uLmVkdQ==
†Present Address: David N. Showalter, Department of Plant and Microbial Biology, University of Minnesota, St. Paul, MN, United States
Daniel A. Herms, The Davey Tree Expert Company, Kent, OH, United States