- Department of Biology, Texas State University, San Marcos, TX, United States
Larval amphibians are frequently exposed to multiple stressors in aquatic environments, so understanding how individual stressors and synergisms of multiple stressors affect amphibians is integral to conservation efforts. Glyphosate, the active ingredient in Roundup™, is a common pollutant found in aquatic environments. Exposure to glyphosate induces morphological, behavioral, and physiological changes in tadpoles possibly via infodisruption. Urban aquatic environments may have environmentally relevant levels of glyphosate, as well as higher concentrations of exogenous corticosterone (CORT) than rural areas. Elevated CORT levels also affect the morphology and physiology of tadpoles. Dragonfly larvae are common predators of tadpoles, and tadpoles often show elevated CORT and reduced activity in the presence of dragonfly larvae. We tested the hypothesis that combined effects of exogenous CORT and Roundup™ exposure would affect the antipredator behavior, morphology, and stress hormone responses of Gulf coast toad, Incilius nebulifer, tadpoles. We exposed tadpoles to one of four treatments: Roundup™, CORT, Roundup™+CORT, or control, for 7 days. Tadpoles exposed to CORT or Roundup™+CORT had elevated CORT release rates. Tadpoles exposed to exogenous CORT had lower tail depth compared to tadpoles in other treatments. Subsequently, we exposed tadpoles to dragonfly diet cues. Tadpoles increased activity after predator cue exposure when they had previously been exposed to Roundup™+CORT. Taken together, our results suggest that there may be synergistic effects between Roundup™ and exogeneous CORT on organismal behavior but not their physiology or morphology. It appears that glyphosate is an infordisruptor, that prevents tadpoles from demonstrating adaptive antipredator responses, which may contribute to population declines.
Introduction
Worldwide, amphibian biological diversity is decreasing; 32% of the world's amphibian species are threatened with extinction and 43% of amphibian populations are experiencing declines (Stuart et al., 2004). Environmental pollutants such as herbicides and pesticides not only have a direct effect on amphibian population recruitment, but they also have indirect effects via their immunosuppressive effects on individual organisms (Hayes et al., 2010). Immunosuppression is linked to an increase in glucocorticoid hormones after exposure to chronic stressors. The link between exposure to pollutants and immunosuppression in an organism is likely mediated via the infodisruptive effects of the pollutant that disrupt chemical communication modalities used by the organism. Pollutants also often act as endocrine disruptors that affect hormones and internal communication in organisms (Lurling and Scheffer, 2007). When infodisruptors affect the production of glucocorticoid stress hormones, organisms may show altered immunosuppression in the long-term and altered growth and behavior in both the short- and long-term (McEwen and Wingfield, 2003; Romero, 2004; Denver, 2009; Middlemis Maher et al., 2013). In addition, the breakdown in detection of chemical signals between senders and receivers due to infodisruptors in predator prey systems may increase prey mortality.
Amphibians are exposed to many stressors throughout their life cycle, but frequently early in life aquatic-dependent life stages are particularly susceptible to the effects of exposure to aquatic pollutants (Hayes et al., 2006). During chronic exposure to stressors, the hypothalamic-pituitary interrenal (HPI) axis may become disrupted, and the effects of increased corticosterone (CORT) become immunosuppressive. In frogs and toads, tadpoles show elevated CORT when exposed to pollutants such as atrazine and glyphosate (Burraco and Gomez-Mestre, 2016; McMahon et al., 2017; Gabor et al., 2018), and in the presence of predators (Middlemis Maher et al., 2013; Bennett et al., 2016). In addition, elevated CORT levels in tadpoles induced by exposure to exogenous CORT for 8 days is associated with deeper tails and shorter trunks (Middlemis Maher et al., 2013). Deeper tails may improve the ability of tadpoles to evade predators (Van Buskirk et al., 1997). Tadpoles are exposed to exogenous CORT in their habitat as it is continually released into the water by aquatic vertebrates via urine, feces, and gills (Scott and Ellis, 2007). Tadpoles may uptake the free steroids that are released from the gills (Ellis et al., 2004). Amphibians occupying habitats where they are exposed to more stressors may further release more CORT into the water. For example, the Jollyville Plateau salamander, Eurycea tonkawae, releases more CORT and is also exposed to higher exogenous CORT (“background CORT”) in urban habitats than in rural habitats (Gabor et al., 2018). This suggests that amphibian habitats in urban areas (that often have higher levels of pollutants such as glyphosate) may also have higher levels of exogenous CORT which may affect the endogenous CORT of the amphibians residing in those waters.
Roundup™ is the most commonly used herbicide in the United States for both agricultural and domestic use (Giesy et al., 2000). Glyphosate, the primary active ingredient in Roundup™, and the other associated ingredients enter many aquatic ecosystems via runoff, leaching, and mis-use of the product (Annett et al., 2014), thus affecting non-target organisms living in aquatic habitats. Further, repeated applications of Roundup™ contributes to the persistence of glyphosate in aquatic ecosystems (Giesy et al., 2000). Environmentally relevant concentrations range from 0.1 to 2.7 mg a.e./L (Solomon and Thompson, 2003), and acute toxicity of glyphosates for amphibians based on 96-h LC50 estimates range from 1.8 to 3.5 mg a.e./L (Annett et al., 2014). Therefore, amphibians in many aquatic ecosystems are exposed to sub-lethal to lethal concentrations of glyphosate.
Based on results of long-term mesocosm studies we know that tadpoles can exhibit differential responses to exposure to glyphosate. However, it is unclear if there are synergistic effects of exposure to predator cues and glyphosate on tadpole development, morphology, behavior, and physiology. For example, exposure to 2–3 mg a.e./L of glyphosate or to the cues of a dragonfly (Anax junius) larvae predator is associated with increased tail depth in two species of Rana tadpoles. The increase in tail depth is additive when tadpoles are exposed to both glyphosate and predator cues (Relyea, 2012). In contrast, glyphosate exposure is not associated with morphological changes similar to predator induced changes for tadpoles of Hyla versicolor (Relyea, 2018). In addition to its morphology-based interactions with predator cues, glyphosate may interact with behaviors involved in antipredator responses, as environmentally relevant levels of glyphosate interfere with chemical cue detection and processing (e.g., Tierney et al., 2006). Again, there are no clear patterns emergent from the literature on the direction of effects. For example, tadpoles of Rana sylvatica exposed to 0.5 mg a.e./L glyphosate do not exhibit a behavioral antipredator response (do not reduce activity) when exposed to predator chemical cues (Moore et al., 2015). The effect of glyphosate on disrupting anti-predator behavior may be concentration dependent; R. dalmatina tadpoles exposed to 2 mg a.e./L glyphosate do not differ from control tadpoles in response to predators, but tadpoles exposed to 6.5 mg a.e./L glyphosate exhibited elevated antipredator behavior (reduced activity) (Miko et al., 2017). These results indicate that antipredator response and morphological changes, as a result of glyphosate exposure, vary by exposure level and are species specific in amphibians.
One potential source of variation mediating the relationship between exposure to glyphosate and effects on tadpole morphology and antipredator behavior is the variation in the direction of the stress response. In Pelobates cultripes tadpoles whole-body CORT increases with exposure to 1 and 2 mg/L of glyphosate, but CORT decreases with predator exposure (Burraco and Gomez-Mestre, 2016). These results indicate that the interaction of exposure to exogenous CORT and glyphosate may drive additional changes unlike those predicted for each alone. There is a gap in our understanding of the synergistic effects of glyphosate and exogenous CORT on amphibian morphology, antipredator behavior, and physiological stress in amphibians. In this study, we tested the hypothesis that the combined effects of exogenous CORT exposure and Roundup™ exposure would affect antipredator behavior, morphology, and hormonal responses of Gulf coast toad, Incilius nebulifer, tadpoles.
Materials and Methods
Gulf coast toad (Incilius nebulifer) tadpoles were collected from a pond in Prospect Park, San Marcos, TX (29.874°N, 97.963°W) in May 2017 and maintained in the laboratory at Texas State University. The tadpoles were maintained in 6 L containers with aged tap water and on a 14 h light: 10 h dark cycle at 19°C until Gosner stage 25 (Gosner, 1960). Tadpoles were fed ad libitum a mixture of spirulina and fish flakes suspended in an agar medium.
Experiment 1: Stress Response to Glyphosate and CORT Exposure
We haphazardly assigned tadpoles (N = 120) that were at or near Gosner stage 25 to one of four experimental treatments that varied in what tadpoles were exposed to for 7 days (12–19 May 2017). Tadpoles were reared in either aged tap water or Roundup™ mixed with aged tap water (final concentration of glyphosate was 0.736 mg a.e./L, analyzed by Mississippi State Chemical Lab). Our target concentration of Roundup™ was 0.5 mg a.e./L based on Moore et al. (2015). The water was also dosed with either 125 nM CORT in 37.5 μL ethanol as the solvent, or only 37.5 μL ethanol. We chose a concentration of glyphosate (from Roundup™) that represented a low, but environmentally relevant, sub-lethal dosage (Relyea, 2012; Moore et al., 2015) and chose a CORT dosage of 125 nM following Middlemis Maher et al. (2013). The four treatments were: (1) “Control,” aged tap water and 37.5 μL ethanol, (2) “Roundup™,” Roundup™ water and 37.5 μL ethanol, (3) “CORT,” 125 nM CORT in 37.5 μL ethanol, and (4) “Roundup™ + CORT,” Roundup™ water and 125 nM CORT in 37.5 μL ethanol. We replicated each treatment with 5 tanks, each containing 1.5 L of water (either 24 h aged tap water or aged tap water mixed with Roundup™), that each housed 6 tadpoles. Rearing containers were labeled by code, so the authors were blind to treatments. The volume of ethanol added to each tank was 0.0025% of the total water volume. Prior studies have detected no effects on any measured trait of ethanol at this level so no water control was included (reviewed by Rohr et al., 2013). On the third day, a full water change was completed for all the treatments. Treatments were re-dosed by someone other than the authors. After 7 days of treatment exposure (Day 7), we collected water-borne CORT from 3 of the 6 tadpoles from each of the 5 replicate tanks (N = 15 tadpoles per treatment; except 2 control and 2 CORT tadpoles died in 4 different tanks). To collect water-borne CORT, tadpoles were individually placed into a Nalgene sleeve (with holes on the bottom) fit within 250 ml glass beakers containing 50 ml spring water for 1 h. We then lifted the tadpole from the water using the Nalgene sleeve, leaving the water-borne hormone sample in the glass beaker. We collected water borne-hormones between 0800 and 1400 h to control for circadian variation.
Following water-borne hormone collection, tadpoles were individually photographed from the side in water with a ruler placed below the tadpole, using a digital camera. We used Image J to measure snout vent length (SVL) and tail depth from the photos. After taking photos and mass, we euthanized the tadpoles by overdose of Oragel (active ingredient 1% benzocaine) placed on the ventral side. We preserved the tadpoles in microcentrifuge tubes with 1 ml of 95% ethanol for later Gosner stage determination using a dissecting scope. The water-borne hormone samples were all placed in individual 118 ml HDPE containers and stored at −20°C until hormone extraction (see below). We standardized hormone release rates by dividing by SVL of the given individual (following Gabor et al., 2016). We log transformed CORT release rates but figures present untransformed values. We measured SVL (mm), tail depth (mm), and mass (g) for all tadpoles.
To test the hypothesis that Roundup™ and exogenous CORT affect tadpole endogenous CORT and morphology, we used a linear mixed model with treatment as a fixed effect, and tadpole as a random effect. The response variables were: SVL, tail depth and Ln CORT release rate. All analyses were performed using JMP 14 pro software (SAS Institute, Inc).
Roundup™ Preparation
Roundup™ Ready to Use, Fast Act Technology was purchased from an agricultural supply store in San Marcos, TX USA in 2017. The glyphosate concentration was 20 g acid equivalents (a.e.)/L., so 0.45 ml of Roundup™ base solution was diluted in 18 L of dechlorinated aged tap water to create a stock solution. The glyphosate concentration in the final Roundup™ solution was analyzed from two samples from the stock solution by Mississippi State Chemical Laboratory and determined to be 0.736 mg a.e./L.
Water-Borne Corticosterone Assay
We extracted CORT from the water-borne samples by priming C18 solid phase extraction (SPE) columns (Waters Technology Corporation) with 4 ml of methanol and 4 ml of distilled water (following Gabor et al., 2016). We then pulled the water samples through the column using a vacuum manifold. Samples were eluted with 4 ml of methanol and then the methanol was dried with nitrogen in a 37°C bath. The samples were reconstituted using 5% ethanol, followed by vortexing. We then added 95% enzyme immune-assay (EIA) buffer (from Cayman Chemical Company) up to a volume of 260 μl. All CORT values were multiplied by the resuspension volume. We assayed samples in duplicate using corticosterone EIA plates (No 501320, Cayman Chemical Company) using a spectrophotometer plate reader at 405 nm (BioTek 800XS). Inter-plate variation for four plates was 10.06% and intra-plate variation ranged from 0.22 to 2.91%.
Experiment 2: Antipredator Behavior
On day 7, the remaining tadpoles from the treatments (up to 3 tadpoles in each tank) were placed in 20 clean tanks (the same size as they had been in before) filled with 1.5 L of untreated aged tap water (thus no longer exposed to Roundup™ or CORT). To test for anti-predator behavior, we first collected diet-based chemical cues released by tadpole predators (late instar dragonfly nymphs, genus: Anax sp.). The dragonfly nymphs were collected from the same pond as the tadpoles in May 2017 and were kept in individual containers filled with aged tap water and a perch. On day 0 of Experiment 1, we began feeding Anax nymphs 2 conspecific tadpoles per day for 7 days. On day 7, Anax (n = 5) were placed into separate clean beakers with 230 ml water per gram of Anax for 24 h. The water samples were then pooled together and mixed. This water-based “diet cue” was frozen at −20°C in 120 ml aliquots (Epp and Gabor, 2008). We defrosted the amount of diet cue needed for the antipredator behavior experiment by letting the aliquot come to room temperature before use.
On days 8, 9, and 10 of the experiment, we set up 20 individual tanks with 1.5 L of aged tap water and with two 60 ml syringes connected to airline tubing with a two-way spout that was placed inside the tank to evenly disperse the water and diet cue. One syringe was filled with aged tap water and the other with the predator diet cue. Individual tadpoles were placed in the tanks and allowed to acclimate for 8 min. We then injected 10 ml of water into the tank at a rate of 1 ml per second and recorded the “pre” exposure activity rate of the tadpole for 8 min using a timer. Activity was defined as any tadpole movement. Then, we injected 10 ml of the diet cue into the tank at the same rate and recorded “post” exposure activity (anti-predator behavior) for 8 min. Syringe tips and tubing were cleaned between trials with 3% hydrogen peroxide and rinsed with deionized water. After the predator cue trial, we collected water-borne CORT samples as outlined previously. Antipredator behavior testing occurred from 0800 to 1200 h with water-borne hormone extraction immediately following predator cue exposure. We also euthanized and collected body measurements from these tadpoles, as outlined in experiment 1.
First, we tested for any significant variation in pre-predator cue exposure activity across the tadpoles from the different treatments using a linear mixed effect model with tank as a random effect and treatment, the day (1, 2, or 3 days after the end of experiment 1) of the behavioral trials, and the treatment X day interaction as the fixed effects. We used a linear mixed effect model on the difference in activity (pre-exposure activity—post-exposure activity) with tank as a random effect, the day of the activity behavior trials, and the treatment X day interaction as the fixed effects. We also used a linear mixed effect model on Ln CORT (post predator cue exposure) release rate with tank as a random effect, the day of the activity behavior trials, and the treatment X day interaction as the fixed effects. All significant treatment effects were further explored with post-hoc multiple comparisons. All analyses were performed using JMP 14 pro software (SAS Institute, Inc.).
Results
Experiment 1: Stress Response to Glyphosate and CORT Exposure
After 7 days of exposure to treatments, CORT release rates were significantly higher in the CORT only and the Roundup™ + CORT treated tadpoles than in the control treatment tadpoles [Treatment: F(3, 16) = 41.21, p < 0.0001; Figure 1a]. Tadpoles in the CORT only treatment had significantly lower tail depth than the control tadpoles [F(3, 16) = 4.39; p = 0.02; Figure 2a]. None of the treatments had significantly different SVL from the control treatment, but tadpoles in the CORT treatment had a significantly smaller SVL than those in the Roundup™ only treatment [Treatment: F(3, 16) = 4.75; p = 0.01; Figure 2b).
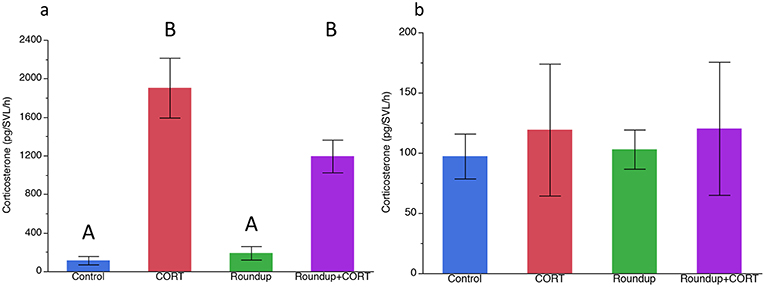
Figure 1. Mean (±SE) corticosterone (pg/SVL/h) by treatment (a) after 7 days in the exposure treatments, and (b) after predator cue exposure for Gulf coast toad, Incilius nebulifer. Different letters indicate significant differences based on Tukey's HSD post-hoc test.
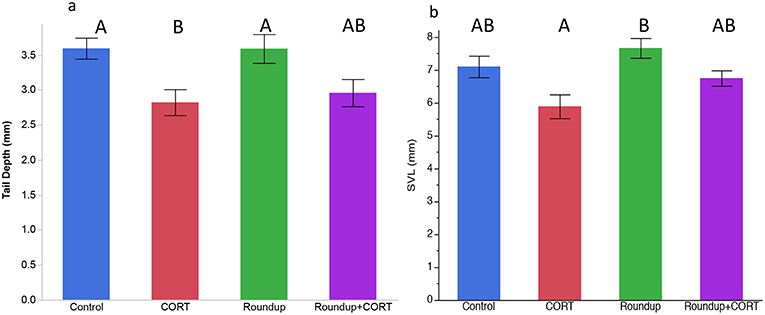
Figure 2. Mean (±SE) (a) tail depth and (b) snout-vent length (SVL) by treatments on day 7 for Gulf coast toad, Incilius nebulifer. Different letters indicate significant differences based on post-hoc student's t-tests.
Experiment 2: Antipredator Behavior
After exposure to the treatments, but before exposure to any predator cues, there was no significant variation in the activity level of the tadpoles (Table 1). After predator exposure, there was a significant effect of the treatment on the change in activity of the tadpoles; tadpoles in the Roundup™ + CORT treatment had a significant increase in their activity after predator cue exposure. Tadpoles from all other treatments decreased activity [Treatment: F(3, 17) = 3.36, p = 0.043; Figure 3]. CORT release rates did not differ across treatments after exposure to predator diet cues [Treatment: F(3, 15) = 0.34, p = 0.80; Figure 1b].
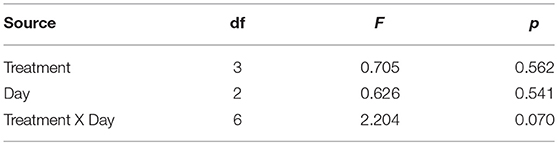
Table 1. Fixed effects tests from LME model on post-treatment, but pre-exposure to predator cue activity (s) of Gulf coast toad, Incilius nebulifer, tadpoles.
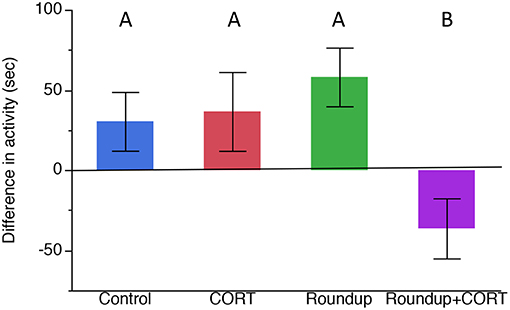
Figure 3. Mean (±SE) difference in activity (time in seconds spent moving) before predator cue exposure and after predator cue exposure across treatments (pre-cue time minus post-cue time). Different letters indicate significant differences based on Tukey's HSD post-hoc test.
Discussion
Anthropogenic stressors, such as pollutants, contribute to amphibian population declines (Stuart et al., 2004). Because amphibians are often simultaneously exposed to multiple stressors, we evaluated here if multiple stressors can have synergistic effects on tadpole stress response, morphology, and antipredator behavior. We found that Gulf coast toad tadpoles exposed to exogenous CORT and Roundup™ + CORT had significantly higher CORT release rates than those exposed to Roundup™ alone or the control. Our results were consistent with Glennemeier and Denver (2002) who found elevated whole-body CORT in leopard frogs, Rana pipiens, after exposure to 125 nM exogenous CORT. Aquatic habitats in urban areas may be more likely to have higher levels of exogenous sources of CORT (background CORT) in the water from other aquatic vertebrates, run-off, terrestrial animals, or sewage line seepage (e.g., Gabor et al., 2018). Because tadpoles can uptake exogenous CORT through their skin, exposure to higher concentrations of exogenous CORT may push tadpoles away from homeostasis and could ultimately result in immunosuppression of the organism. Such indirect effects on organisms as a result of exposure to anthropogenic stressors are associated with greater disease susceptibility (Blaustein et al., 2012), and can ultimately lead to decreases in population recruitment. However, we did not find an infodisruptive effect of a low but environmentally relevant concentration of Roundup™.
We exposed tadpoles to a very low, concentration of Roundup™ and their CORT release rates did not increase with this exposure. In contrast, (Burraco and Gomez-Mestre, 2016) found that tadpoles exposed to 1 or 2 mg a.e./L glyphosate had increased whole-body CORT after a 10-day exposure period. Future studies will address if higher levels of Roundup™ and/ or longer exposure affect CORT release rates in Gulf coast toads.
Despite no effects of exposure to 0.736 mg a.e./L glyphosate for 7 days on Gulf coast toad tadpole CORT release rates, these tadpoles did have significantly greater SVL than CORT exposed tadpoles. Exposure to CORT also affected Gulf coast toad tadpole tail morphology: tadpoles in the CORT only treatment had significantly lower tail depth than the control tadpoles. Exposure to elevated CORT is associated with tadpoles of R. sylvatica and R. pipiens developing deeper tails (an antipredator morphology; Hossie et al., 2010; Middlemis Maher et al., 2013). At higher, but still environmentally relevant, concentrations of Roundup™ the antipredator morphology of deeper tails is induced in wood frog and leopard frog tadpoles (Relyea, 2012) but not in gray tree frog tadpoles, Hyla versicolor (Relyea 2018). Deeper tails aid in escaping from predators as deeper tails deflect lethal predator attacks toward the tail instead of the body of the tadpole (Van Buskirk et al., 1997). We hypothesize that Gulf coast toad tadpoles do not show antipredator morphology because the tadpoles are noxious to predators (Adams et al., 2011) and thus do not rely on this deflection behavior as an anti-predator strategy.
After predator exposure, tadpoles in the Roundup™ + CORT treatment were more active than they were before exposure to the dragonfly nymph predator cue. Prior to exposure to the predator cue, there were no significant differences in tadpole activity across the treatments, suggesting that the increase in the activity after predator cue exposure of tadpoles exposed to both Roundup™ and CORT was a result of their prior treatment affecting their responsiveness to a predator cue in a seemingly maladaptive way. Tadpoles from all other treatments decreased activity after exposure to a predator cue. Generally, tadpole antipredator behavior consists of freezing in response to sit-and-wait predators like dragonfly nymphs (Middlemis Maher et al., 2013; Moore et al., 2015; Miko et al., 2017). Wood frog, Rana sylvatic, tadpoles do not reduce activity when they are exposed to Roundup™ alone and predator diet cues (Moore et al., 2015). For Gulf coast toads, Roundup™ alone did not affect their antipredator behavior but the combination of CORT and Roundup™ did affect their antipredator behavior. Toads could be less sensitive to the effects of Roundup™, except when they are exposed to stressors. One hypothesis for this is that Bufonid toads synthesize toxic steroids called bufadienolides that are synthesized from the same chemical pathway that produces CORT and the relationship may result in negative feedback that could have mediated a reduction in CORT production (Webb et al., 2008). Bókony et al. (2017) found that longterm exposure of tadpoles, Bufo bufo, to 4 mg a.e./L of glyphosate in the lab produced more bufadienolides.
We found that CORT release rates did not increase after exposure to predator diet cues for 10 min as these values were similar to the control values for tadpoles after the first (exposure/no exposure to CORT or Roundup™) experiment. Amphibian CORT response to predator diet cues is variable and often time-dependent. Rana sylvatica tadpoles exhibited an acute (10–20 min post-cue exposure) whole-body CORT increase in response to dragonfly larvae predator cues (Bennett et al., 2016). In some populations of R. temporaria, whole-body CORT levels are higher in predator exposed tadpoles at 24 h than in control tadpoles, but this difference is not apparent by 15 days after exposure to predator cues (Dahl et al., 2012). In some cases, there can be initial downregulation of whole-body CORT (within 4 h of non-lethal exposure to predators), but upregulation of CORT after several days (e.g., R. sylvatica, Middlemis Maher et al., 2013). One explanation for our result is that CORT had already decreased in the time since the tadpoles were exposed to the initial treatments (1–3 days), and that the short-term predator cue exposure was not enough to elicit a CORT response. CORT may have also been downregulated in the CORT and CORT + Roundup™ treatments after predator exposure, but we are unable to test this hypothesis with or data. However, CORT release rates in tadpoles in the CORT or Roundup™ + CORT treatments dropped to the same levels as the other treatments after exposure to the predator cues. Whether downregulation of CORT is associated with the observed increase of activity in the Roundup™ + CORT treatment is not clear.
Tadpoles exposed to the exogeneous CORT or the Roundup™ + CORT treatments had higher CORT release rates than tadpoles in the control or Roundup™ only treatments prior to predator cue exposure. One interpretation for this result is that tadpoles can uptake exogenous CORT in the environment, which could affect their endogenous CORT levels and behavior. However, it is not known how high the levels of free exogenous CORT need to be in an organism's environment for it to be absorbed and ultimately affect the organism's CORT levels. We should note that in our experiment when we measured water-borne CORT after exposure to exogenous CORT we did not rinse the tadpoles off. In theory a droplet of water from the treatment could have traveled on the tadpole into the water sample and ultimately inflated the CORT release rate values. However, the amount of CORT in a 0.05 mL droplet from a tadpole removed from the 125 nM treated water (~5.63 pg) would be much lower than the observed difference in CORT release rates between the treatments. In the future it may be better to rinse tadpoles before measuring water-borne CORT after being exposed to exogenous CORT although the additional handling and rinsing could add an additional stressor to the tadpoles.
Our results support the hypothesis that the combined effects of exogenous CORT exposure and environmentally-relevant concentrations of Roundup™ affected antipredator behavior but not morphology or physiology of Gulf coast toads, I. nebulifer. Roundup™ alone does not act as an infodisruptor to Gulf coast toads at the tadpole stage, as tadpoles reared in Roundup™ did show decreased activity after predator cue exposure, which is consistent with other studies. However, our results suggest that the synergistic effect of Roundup™ + CORT exposure may act as an infodisruptor in I. nebulifer tadpoles as they increased activity after introduction of predator cues. Tadpoles of this species exposed to low levels of Roundup™ could be negatively affected by the synergism of glyphosate and exogenous CORT in urban aquatic environments.
Ethics Statement
Institutional Animal Care and Use Committee - IACUC201563714.
Author Contributions
HP and AH executed the experiments with help from CG and ZF. CG and AA analyzed the data. All authors contributed to writing the manuscript. All authors have read and approved the final version of the manuscript.
Funding
This research was funded in part by U.S. Department of Education, HSI STEM Program (84.031c), Award #P031C160036 and the Francis Rose Undergraduate Award for Excellence in Biological Research to HP and Texas State University Honors program funding to AH.
Conflict of Interest Statement
The authors declare that the research was conducted in the absence of any commercial or financial relationships that could be construed as a potential conflict of interest.
Acknowledgments
We thank Diana Kim, Cory Goff, Chloe Trevino for help with the experiment.
References
Adams, C. K., Saenz, D., and Conner, R. N. (2011). Palatability of twelve species of anuran larvae in eastern Texas. Am. Midl. Natural. 166, 211–223. doi: 10.1674/0003-0031-166.1.211
Annett, R., Habibi, H. R., and Hontela, A. (2014). Impact of glyphosate and glyphosate-based herbicides on the freshwater environment. J. Appl. Toxicol. 34, 458–479. doi: 10.1002/jat.2997
Bennett, A. M., Longhi, J. N., Chin, E. H., Burness, G., Kerr, L. R., and Murray, D. L. (2016). Acute changes in whole body corticosterone in response to perceived predation risk: a mechanism for anti-predator behavior in anurans? Gen. Comp. Endocrinol. 229, 62–66. doi: 10.1016/j.ygcen.2016.02.024
Blaustein, A. R., Gervasi, S. S., Johnson, P. T., Hoverman, J. T., Belden, L. K., Bradley, P. W., et al. (2012). Ecophysiology meets conservation: understanding the role of disease in amphibian population declines. Philos. Trans. R. Soc. Lond. B Biol. Sci. 367, 1688–1707. doi: 10.1098/rstb.2012.0011
Bókony, V., Mik,ó, Z., Móricz, Á. M., Krüzselyi, D., and Hettyey, A. (2017). Chronic exposure to a glyphosate-based herbicide makes toad larvae more toxic. Proc. Royal Soc. B 284:20170493. doi: 10.1098/rspb.2017.0493
Burraco, P., and Gomez-Mestre, I. (2016). Physiological stress responses in amphibian larvae to multiple stressors reveal marked anthropogenic effects even below lethal levels. Physiol. Biochem. Zool. 89, 462–472. doi: 10.1086/688737
Dahl, E., Orizaola, G., Winberg, S., and Laurila, A. (2012). Geographic variation in corticosterone response to chronic predator stress in tadpoles. J. Evol. Biol. 25, 1066–1076. doi: 10.1111/j.1420-9101.2012.02493.x
Denver, R. J. (2009). Stress hormones mediate environment-genotype interactions during amphibian development. Gen. Comp. Endocrinol. 164, 20–31. doi: 10.1016/j.ygcen.2009.04.016
Ellis, T., James, J. D., Stewart, C., and Scott, A. P. (2004). A non-invasive stress assay based upon measurement of free cortisol released into the water by rainbow trout. J. Fish Biol. 65, 1233–1252. doi: 10.1111/j.0022-1112.2004.00499.x
Epp, K. J., and Gabor, C. R. (2008). Innate and learned predator recognition mediated by chemical signals in Eurycea nana. Ethology 114, 607–615. doi: 10.1111/j.1439-0310.2008.01494.x|ISSN0179-1613
Gabor, C. R., Davis, D. R., Kim, D. S., Zabierek, K. C., and Bendik, N. F. (2018). Urbanization is associated with elevated corticosterone in Jollyville Plateau salamanders. Ecol. Indica. 85, 229–235. doi: 10.1016/j.ecolind.2017.10.047
Gabor, C. R., Zabierek, K. C., Kim, D. S., Alberici Da Barbiano, L., Mondelli, M. J., Bendik, N. F., et al. (2016). A non-invasive water-borne assay of stress hormones in aquatic salamanders. Copeia 2016, 172–181. doi: 10.1643/OT-14-207
Giesy, J. P., Dobson, S., and Solomon, K. R. (2000). “Ecotoxicological risk assessment for roundup® herbicide,” in Reviews of Environmental Contamination and Toxicology: Continuation of Residue Reviews, ed G. W. Ware. (New York, NY: Springer New York), 35–120. doi: 10.1007/978-1-4612-1156-3_2
Glennemeier, K. A., and Denver, R. J. (2002). Small changes in whole-body corticosterone content affect larval Rana pipiens fitness components. General Compa. Endocrinol. 127, 16–25. doi: 10.1016/S0016-6480(02)00015-1
Gosner, K. (1960). A simplified table for staging anuran embryos and larvae with notes on identification. Herpetologica 16, 183–190.
Hayes, T., Falso, P., Gallipeau, S., and Stice, M. (2010). The cause of global amphibian declines: a developmental endocrinologist's perspective. J. Exp. Biol. 213, 921–933. doi: 10.1242/jeb.040865
Hayes, T. B., Case, P., Chui, S., Chung, D., Haeffele, C., Haston, K., et al. (2006). Pesticide mixtures, endocrine disruption, and amphibian declines: Are we underestimating the impact? Environ. Health Perspect. 114, 40–50. doi: 10.1289/ehp.8051
Hossie, T. J., Ferland-Raymond, B., Burness, G., and Murray, D. L. (2010). Morphological and behavioural responses of frog tadpolesto perceived predation risk: a possible role for corticosterone mediation? Ecoscience 17, 100–108. doi: 10.2980/17-1-3312
Lurling, M., and Scheffer, M. (2007). Info-disruption: pollution and the transfer of chemical information between organisms. Trends Ecol. Evol. 22, 374–379. doi: 10.1016/j.tree.2007.04.002
McEwen, B. S., and Wingfield, J. C. (2003). The concept of allostasis in biology and biomedicine. Hormones Behav. 43, 2–15. doi: 10.1016/S0018-506X(02)00024-7
McMahon, T. A., Boughton, R. K., Martin, L. B., and Rohr, J. R. (2017). Exposure to the herbicide atrazine non-linearly affects tadpole corticosterone levels. J. Herpetol. 51, 270–273. doi: 10.1670/16-126
Middlemis Maher, J., Werner, E. E., and Denver, R. J. (2013). Stress hormones mediate predator-induced phenotypic plasticity in amphibian tadpoles. Proc. Royal Soc. Biol. Sci. Series B 280:20123075. doi: 10.1098/rspb.2012.3075
Miko, Z., Ujszegi, J., Gal, Z., and Hettyey, A. (2017). Effects of a glyphosate-based herbicide and predation threat on the behaviour of agile frog tadpoles. Ecotoxicol Environ. Saf. 140, 96–102. doi: 10.1016/j.ecoenv.2017.02.032
Moore, H., Chivers, D. P., and Ferrari, M. C. O. (2015). Sub-lethal effects of Roundup (TM) on tadpole anti-predator responses. Ecotoxicol. Environ. Saf. 111, 281–285. doi: 10.1016/j.ecoenv.2014.10.014
Relyea, R. A. (2012). New effects of Roundup on amphibians: Predators reduce herbicide mortality; herbicides induce antipredator morphology. Ecological Applications 22, 634–647. doi: 10.1890/11-0189.1
Relyea, R. A. (2018). The interactive effects of predator stress, predation, and the herbicide Roundup. Ecosphere 9:e02476. doi: 10.1002/ecs2.2476
Rohr, J. R., Raffel, T. R., Halstead, N. T., Mcmahon, T. A., Johnson, S. A., Boughton, R. K., et al. (2013). Early-life exposure to a herbicide has enduring effects on pathogen-induced mortality. Proc. Royal Soc. Biol. Sci. Series B 280:20131502. doi: 10.1098/rspb.2013.1502
Romero, L. M. (2004). Physiological stress in ecology: lessons from biomedical research. Trends Ecol. Evol. 19, 249–255. doi: 10.1016/j.tree.2004.03.008
Scott, A. P., and Ellis, T. (2007). Measurement of fish steroids in water - a review. General Comp. Endocrinol. 153, 392–400. doi: 10.1016/j.ygcen.2006.11.006
Solomon, K., and Thompson, D. (2003). Ecological risk assessment for aquatic organisms from over-water uses of glyphosate. J. Toxicol. Environ. Health Part B 6, 289–324. doi: 10.1080/10937400306468
Stuart, S. N., Chanson, J. S., Cox, N. A., Young, B. E., Rodrigues, A. S. L., Fischman, D. L., et al. (2004). Status and trends of amphibian declines and extinctions worldwide. Science 306, 1783–1786. doi: 10.1126/science.1103538
Tierney, K. B., Ross, P. S., Jarrard, H. E., Delaney, K. R., and Kennedy, C. J. (2006). Changes in juvenile coho salmon electro-olfactogram during and after short-term exposure to current-use pesticides. Environ. Toxicol. Chem. 25, 2809–2817. doi: 10.1897/05-629R1.1
Van Buskirk, J., McCollum, S. A., and Werner, E. E. (1997). Natural selection for environmentally induced phenotypes in tadpoles. Evolution 51, 1983–1992. doi: 10.2307/2411018
Keywords: amphibians, ecotoxicology, glyphosate, conservation physiology, stress, water-borne hormones
Citation: Gabor CR, Perkins HR, Heitmann AT, Forsburg ZR and Aspbury AS (2019) Roundup™ With Corticosterone Functions as an Infodisruptor to Antipredator Response in Tadpoles. Front. Ecol. Evol. 7:114. doi: 10.3389/fevo.2019.00114
Received: 31 December 2018; Accepted: 21 March 2019;
Published: 11 April 2019.
Edited by:
Shannon J. McCauley, University of Toronto Mississauga, CanadaReviewed by:
Jessica Hua, Binghamton University, United StatesTravis Eli Wilcoxen, Millikin University, United States
Copyright © 2019 Gabor, Perkins, Heitmann, Forsburg and Aspbury. This is an open-access article distributed under the terms of the Creative Commons Attribution License (CC BY). The use, distribution or reproduction in other forums is permitted, provided the original author(s) and the copyright owner(s) are credited and that the original publication in this journal is cited, in accordance with accepted academic practice. No use, distribution or reproduction is permitted which does not comply with these terms.
*Correspondence: Caitlin R. Gabor, gabor@txstate.edu