- 1Department of Paleontology, American Museum of Natural History, New York, NY, United States
- 2Department of Anatomy, College of Osteopathic Medicine, New York Institute of Technology, College of Osteopathic Medicine, Old Westbury, NY, United States
- 3Department of Pediatrics, Alfred I. DuPont Hospital for Children, Wilmington, DE, United States
The hypsodont crown of Equus and of other hypsodont ungulates has two functions: It has an extra crown in the alveolus which erupts and becomes a functional crown that enables the horse to live longer and feed on abrasive foods and grit. The second functional aspect is that the crown, while it is in the alveolus, acts as a root to support high stress during mastication. In general, roots do not increase in size during evolution when the tooth crown increases. Delayed development of the true root is a heterochrony phenomenon and is possibly dynamically interactive with the forces applied on the crown. Thus, when the crown becomes worn, as in old age, the mastication forces acting on it are very strong. This is an interesting phenomenon and reinforces our hypothesis of the second functional difference that the young tooth's crown embedded in the alveolus acts as a supporting root. The Equus hypsodont tooth has been represented by a class I lever. That is, the fulcrum is in the middle: the effort is applied on one side of the fulcrum and the resistance (or load) on the other side, for example, as in a crowbar. As an individual Equus ages, the alveolar tooth height decreases. Data display an exponential increase in force generated as tooth height decreases. The elongation and closure of the root is delayed until the crown is almost entirely worn. When the crown becomes worn, the mastication forces acting on it are very strong. This is an interesting phenomenon and reinforces our hypothesis that the young tooth's crown, embedded in the alveolus, acts as a supporting root. This discovery is based on the observation that fossil ungulates most commonly die at an early age, leaving a substantial amount of crown unused. The unused crown is not likely a reserve tooth crown for a season of hardship because it is rare to find examples of such hardships in the fossil record.
Introduction
Ungulate (hoofed) mammals have often evolved tall tooth crowns. The tall-crowned teeth have been termed hypsodont teeth (hypso; meaning tall) (Van Valen, 1960). Hypsodonty is a common specialization in many herbivorous mammals and it is found in artiodactyls, perissodactyls, suids, proboscideans, rodents, South American ungulates, and other groups (Janis, 1988; Williams and Kay, 2001; Mihlbachler and Solounias, 2006; Damuth and Janis, 2011). It has evolved independently from a low crowned tooth termed brachydont (brachy; meaning short). Among these, Equus is one of the most hypsodont ungulates known (Stirton, 1947; Simpson, 1951). In Equus and in all other species with hypsodont teeth, a major part of the crown is embedded in the alveoli which erupts slowly to replace the active crown that has become worn. Hypsodonty has been studied extensively (see Damuth and Janis, 2011; Madden, 2015 for reviews). Our study is not meant to be a review of hypsodonty. The equid hypsodonty is commonly envisioned as an adaptation for grazing. Grasses are abrasive to teeth as they contain more phytoliths than other plants. Phytoliths are intracellular silica inorganic particles, which are thought to abrade teeth. Hence, equids and other ungulates that feed on grass and other abrasive vegetation wear down their enamel crowns more and faster (Strömberg, 2006; Janis, 2008). Mihlbachler et al. (2011) have shown that in the evolution of Equidae, hypsodonty correlates with the onset and further adaptation to grazing. Semprebon et al. (2016) show that Pleistocene grazing in Equus is not the only factor but grit plays a role as well (Sneva et al., 1983; Madden, 2015). Equid grazers need an extra crown, which is exactly what a hypsodont tooth can provide. That extra crown is situated in the alveoli. In contrast, browsers feed on softer vegetation than grazers and the entire tooth is exposed in the oral cavity. Only the roots are positioned in the alveoli. Browsers wear down about 0.9 microns of enamel per day. Grazers wear about 8.1 microns of enamel per day and hence possess more crown than browsers (Solounias et al., 1994; from Table 1). Recently, many researchers tend to think that it is grit that is the main cause of tooth wear (see Damuth and Janis, 2011 and Madden, 2015 for reviews). What is interesting, however, is that grit should wear down the teeth of browsers as well; a topic avoided. Pronghorns, goats and camels are hypsodont browsers, but the majority of browsers are brachydont. Many problems on how teeth actually become worn down remain to be solved.
Numerous articles and books have been written specifically about the evolution of equids (e.g., Matthew, 1926; Stirton, 1947; Simpson, 1951; Rensberger et al., 1984; MacFadden, 1994; Strömberg, 2006; Mihlbachler et al., 2011; Evans and Janis, 2008). If one considers broader studies on ungulates, including equids, an additional 20 pivotal studies could be included. The evolution of horses over the past 55 million years is clearly reflected in the changes of their teeth and diet (Mihlbachler et al., 2011; Semprebon et al., 2016). The present study does not question that hypsodonty relates to the abrasive grass diet or exposure to exogenous grit. It certainly does. An additional function, however, is put forward for the unerupted tooth crown. As vegetation contacts the tooth crown in the oral cavity, major stress is generated on that crown. It is proposed that Equus requires additional roots to take on excessive masticatory stress. The hypothesis is that the active exposed crown cannot be supported by the regular roots alone. Genetically, roots in mammals have a conservative morphology and apparently cannot increase in number or size. The new hypothesis is that the unerupted crown of Equus functions as a root. We propose that two functional regimes are present: (1) an extra crown for an abrasive diet (2) a root to support the stress. Our new hypothesis is similar to the alveolar hypothesis discussing eruption (Fortelius, 1985). The proposed term for this type of root is a plioroot. Plio means more in Greek; more root-additional root. It is also a pun as many of the hypsodonts evolve during the Pliocene which is a time of notable increase of grasslands and of hypsodont ungulates. In our study we treat the forces of mastication, tall teeth and the alveolus, and exposed functioning enamel as a first-class lever system. Archimedes, the ancient Greek polymath, referred to a first-class lever in his famous quote “Give me one firm spot on which to rest (a fulcrum) and I will move the Earth.”
This study was inspired by the observation that in most species, death occurs well before the entire tooth is used (by NS). So why make so much more enamel if the species in question is not going to need it for mastication? The plioroot hypothesis explains these observations.
Recent studies have addressed hypsodonty and emphasized how it would form as a response to stress from feeding affecting the growth zone. Renvoisé and Michon (2014) explained in a different way that a hypsodont tooth is merely an expansion of the same framework of a brachydont tooth, by prolonging the growth zone between the root and enamel. It is an acceleration of that boundary which results in a heterochrony of the enamel. That extra growth will result in a wall (the tall crown). This wall of enamel-band was one of the types of hypsodonty in von Koeningswald's categories (von Koeningswald, 2011). Heterochrony results in that acceleration while the size and shape remain in their ancestral relationship (Gould, 1977). Renvoisé and Michon (2014) also thought of the stress during mastication which would create a feedback loop affecting heterochronic growth at the boundary of the enamel with the root. Witzel et al. (2018) pointed out that the formation of enamel occurs after the tooth has erupted and, hence its function. Thus, function relates and can affect growth. Growth of enamel may relate to longevity.
Materials and Methods
Specimens of Equus were examined in the mammalogy and the paleontology collections of the American Museum of Natural History (AMNH), the University of Colorado Museum in Boulder (UCM), and the Natural History Museum of Basel (NHMBa). Specimens of Equus sp. from Alaska, Hyracotherium spp., Mesohippus bairdii, Merychippus insignis and Protohippus primus, and Equus sp. were examined to estimate root sizes. We had access to four domesticated Equus caballus specimens of a known age in the laboratory of NS.
A small number of specimens were used to support the development of this new theory, at this initial qualitative stage. For these, root, crown height, as well as occlusal lengths and widths were measured with digital calipers (Figure 1). Figure 1 shows the excavated maxilla and mandible of Equus.
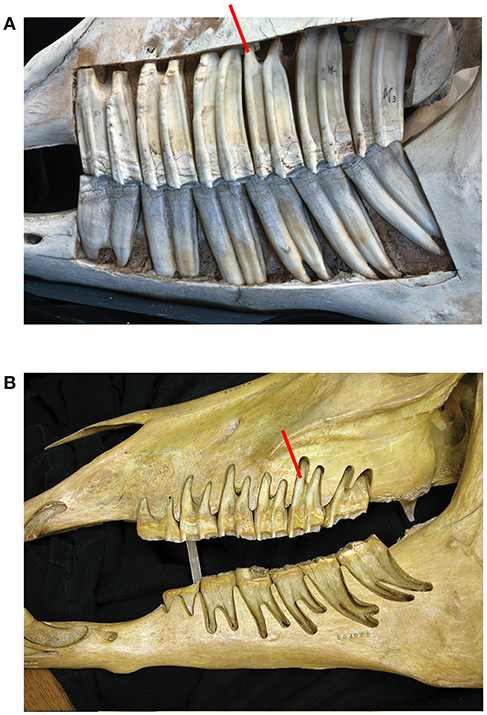
Figure 1. (A) In Equus caballus NHMBa 28, extreme hypsodonty is observed; all six teeth (three premolars and three molars) are approximately equally hypsodont. The maxilla and mandible have been cut open to show the crown contained in the alveoli. The active crown is the exposed part. Red square shows the roots which are short and open-ended. (B) An old Equus individual AMNH 204019. The enamel crowns have been almost totally worn out. In this specimen, the roots are longer than in younger horses and closed. Root increase is most likely an epigenetic response to the reduction of the height of the crown which transmits increased forces. The pressures of mastication change and that induces the roots to grow longer.
By examination of the dentition, the specimens we scored either as a younger adult or as very old. NS asked the question: was the crown completely exposed in the oral cavity or was there more enamel deep in the alveoli? If the crown is completely exposed, the roots should be visible where they contact the enamel. Visual comparison between younger and older teeth of the same species facilitated the scoring and the data collection. In hypsodont species, when the roots were visible, the individuals were older, as most of the crown was worn down. When scoring the age of individuals it is critical to observe the base of the crown and the roots. Using this methodology, we counted the number of young ungulate adults and very old individuals from 16 localities at the AMNH and UCM (Table 2). The exploratory sample of taxa were: dromomerycids, bovids, equids, and camelids.
A few root lengths and crown dimensions of some species were recorded (Table 1) at the AMNH. To measure root lengths dentitions were used where the roots were exposed due to the brakeage of the fossil.
Four domesticated Equus caballus skulls and teeth were sectioned with a carpenter's band saw in the lab (young samples included: a 7 year old male NS 10, 5 year old NS 295; old samples included: an 16 year old male NS 102, and an 18 year old whose sex could not be identified as the canines had been removed NS 104). These specimens were donated from the Bolton Equine Center. Open maxillae and mandibles exposed horse dentitions and the internal anatomy of the alveoli (Table 1).
The longitudinally sectioned molar was hand painted with physical paints to help isolate it in Image J for quantification (Figure 2). In order to calculate enamel thickness and cementum we used Image J (Fiji) that quantified the tissues in photographs as pixels and calculated the square mm (specimen of Figure 2).
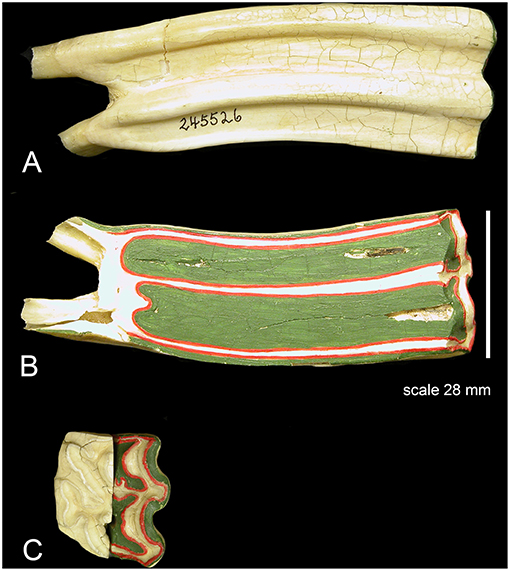
Figure 2. An upper second molar of Equus caballus AMNH 245526 sectioned longitudinally and hand-painted. Colors: red is enamel, green cementum, white is dentine. The section is through the central cavities which are filled with cementum. The cementum is notably thick, and it surrounds the entire tooth. The enamel is thin. (A) The external aspect there the tooth is covered by cementum including the roots where it is thinner coating and hard to observe. (B) The inner aspect; note that the majority of the section is composed of cementum. The enamel is thin. (C) Occlusal view; half of the tooth is painted, and the other half is natural.
Mechanics
The study shows that hypsodonty reduces the force required for mastication. The mechanical interpretation of a tall crown can be studied for upper molar two as a two-dimensional structure; a simplified model. Therefore, the following findings apply to all hypsodont teeth, including the molars and premolars of Equus. The results are shown in the following two Figures 3, 4.
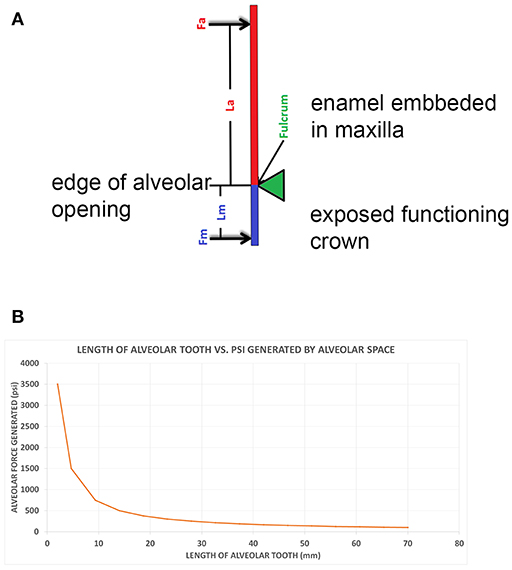
Figure 3. (A) A class I lever representation of a hypsodont tooth. The black bar is the exposed functioning enamel crown. The black arrow is the transverse force not a vertical force. The green shows the fulcrum point as the opening of the alveolar space (again transverse) and the red line is the plioroot deep into the maxilla. In developing this model, the tooth is tilted horizontally. Fm is the force of mastication (estimated). Lm is the length of the exposed tooth to mastication (measured) in the oral cavity. La is the length of the crown embedded in the alveolus (measured). Fa is the force of the alveolar tooth needed to stabilize the tooth during mastication (calculated). The fulcrum represents the interface between the exposed tooth and alveolar tooth and is the oral opening of the alveolus. Blue indicates the exposed tooth and red indicates the hidden alveolar portion of the tooth. (B) Applying Equation 3 with constant values Lm = 14, Fm = 500lbsFm = 500lbs, and knowing that the alveolar length of the tooth (Lα) for a horse at age three is 70 mm and age 18 is 2 mm, we can plot the alveolar force Fα vs. the alveolar length Lα, shown in Figure 2.
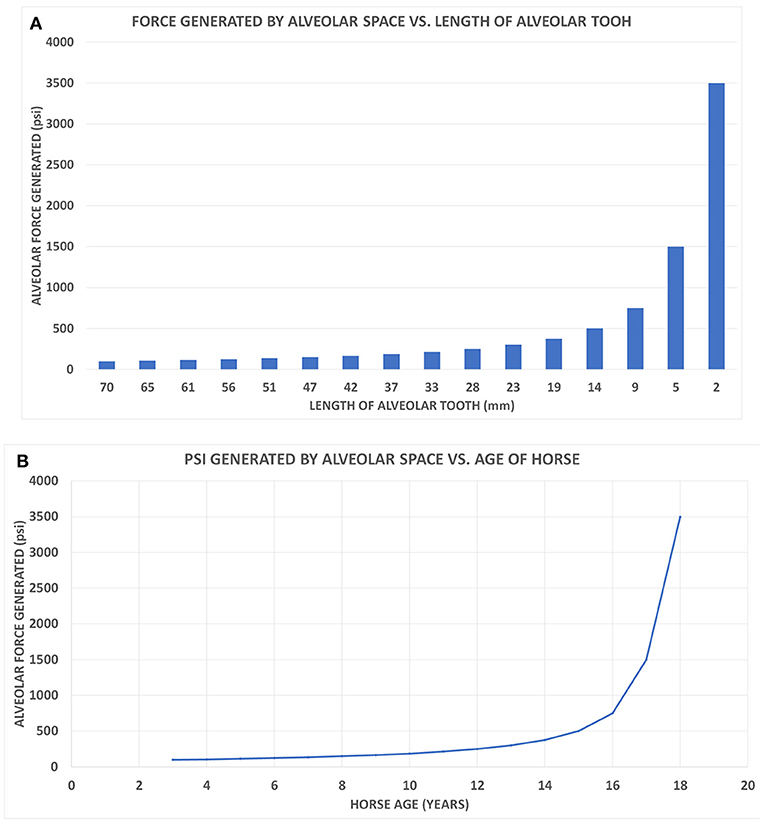
Figure 4. (A) The alveolar force Fα vs. the alveolar length Lα shown on a bar chart to correspond with the age of the horse when read from left to right. (B) The graph displays the force generated by the alveolar space (Fα) vs. the age of the horse.
The Equus hypsodont tooth is represented by a class I lever. That is, the fulcrum is in the middle: the effort is applied on one side of the fulcrum and the resistance (or load) on the other side, for example, as in a seesaw, a crowbar or a pair of scissors. The effort is in the oral cavity and the resistance is in the crown situated in the alveolus. The tall tooth is embedded in the alveolus and the force of chewing is on one side; in the oral cavity. This tooth leans on the side of the alveolus which acts like a supporting fulcrum. The force of mastication, Fm, was estimated while the length of exposed tooth to mastication, Lm, and the length of the crown, La, were measured. Fa is the force of the alveolar tooth needed to stabilize the tooth during mastication (calculated). The fulcrum helps demonstrate the transition between the exposed and alveolar tooth crown as well as the part of the crown embedded in the alveolus. Mastication occurs at the exposed portion of the tooth. The alveolar portion of the tooth stabilizes the exposed portion of the tooth during mastication. This static system assumes no movement of the tooth during mastication therefore indicating all forces will equate to zero in equilibrium. The fulcrum placement is at the location where the exposed tooth meets the alveolar tooth (gum line). The model in Figure 3A is based on the measured values for Equus which are shown in Table 1.
In Figure 3A the black bar is the exposed functioning enamel crown. The black arrow is the transverse force not a vertical force. The green shows the fulcrum point as the opening of the alveolar space (again transverse) and the red line is the plioroot deep into the maxilla. We rotated the model to make it as in an upper molar. Now all of our calculations are based on a horizontal movement. This is a simplified version as there is a vertical force applied as well. However, this is a simple introductory model. A more complex model needs to be developed where both vertical, transverse, and torque forces are explored.
The transverse vector of mastication is estimated and applied as a perpendicular force to the tooth (Fm). Applying class I lever mechanics to Figure 3A, Equation 1 is applied to the system. Two instances of torque results in this condition: Torque due to mastication which equals the product of the mastication force and the length of the tooth (Fm × Lm) in a counterclockwise direction. The second torque vector is the product of the force of the alveolar space force and the length of the alveolar tooth (Fα × Lα) in a clockwise direction. Due to the hypsodont tooth not moving during mastication (static system), these opposite torque values are therefore equal, creating Equation 2 below. Solving for Fα (the only unknown value) in Equations (2, 3) is generated.
The change in forces is exponential. The dramatic increase in force generated is observed especially when the length of the alveolar tooth crown decreases.
Brief Anatomy of the Equus Molars
In Equus, the enamel crowns are embedded in the maxilla and the mandible (Figure 1A). All six teeth (three premolars and three molars) are approximately equally hypsodont. The Equus M2 selected for this study is representative of the other molars and premolars (Figure 2). The outer walls of the enamel are relatively vertical and almost parallel to one another. This gives the tooth a uniform thickness and a columnar shape.
A substantial part of the crown in the Equus M2 is enclosed in the alveolar bone of the mandible and the maxilla (Figure 1A). Only a small part of the crown is exposed in the oral cavity: the active tooth crown. As the animal feeds, the active crown is worn-down by the ingested vegetation and or exogenous grit. An additional crown slowly emerges from the alveolar space to replace the worn crown. In older individuals, albeit rare in the wild and prior to death, very little if any crown remains in the alveoli (Figure 1B).
The tooth root of any mammal is encased externally in a thin coating of cementum. In Equus this coating also extends outside the enamel surface where it is thick (Figure 2). The cementum is a distinct tissue but histologically similar to the dentine (White, 1959; Peyer, 1968). The periodontal ligaments cannot attach to enamel directly. Thus, the cementum adheres to the enamel and provides a surface for the periodontal ligaments. Each alveolar space is lined internally by a thin layer of bone (the lamina dura) (Peyer, 1968; Fortelius, 1985; Williams, 1995 Gray's Anatomy; text figure 12.50). The periodontal ligaments bind the cementum to the lamina dura. The crowns of brachydont teeth are not in the alveoli and hence they have a thin layer of cementum only around the true roots.
The crown of the alveolus constantly erupts and progressively becomes the new active crown while the tooth wears with age. As the tooth emerges from the alveolar space, the empty alveolar space is gradually filled with spongy trabecular bone. The various structures of the hypsodont crown such as styles, ribs, and the vertical wall of the cementum/enamel are also inside the alveolar bone and contact the lamina dura. The lamina dura follows the shape of the three buccal styles of Equus teeth. These structures prevent rotation of the tooth and are clearly used in support during mastication. In older teeth where the very base of the crown does not have these styles, the tooth often rotates and is out of alignment. Worn down teeth create problems in horses (Kirkland et al., 1996; Dacre et al., 2008; Kopke et al., 2012).
Results
This study uses a qualitative approach to develop a new theory. Hence, we have not used a statistical analysis to quantify our results. Hyracotherium and Mesohippus are brachydont taxa. Protohippus and Merychippus are mesodont while Equus is hypsodont. The geological age of the taxa is as follows: Hyracotherium (55–50 Ma); Mesohippus (40–30 Ma); Protohippus (15–7 Ma); Merychippus (23–14 Ma); Equus 1-present).
Size of Roots
Table 1 shows five representative species of equids. In Equus where the teeth are hypsodont, the roots similar in length to the roots of brachydont teeth. The number of specimens examined is small and the calculations are an approximation. Thus, the roots of Hyracotherium are even longer than those of Equus (Table 1). These preliminary results show the roots in evolution do not increase in size as hypsodonty increases; the roots rather decrease. The roots decrease when compared to the crown. Examination of a few roots provided the following findings: The Hyracotherium leporinum have a ratio of (crown height over root length) 1.77, crown/root. In Mesohippus bairdi the ratio is slightly decreased (1.30). That is, the crowns are more hypsodont than in Hyracotherium. In Merychippus insignis, the hypsodonty is evident as the ratio drops to 0.62. Protohippus primus has long roots and the ratio is 0.47. In the Pleistocene Equus sp. from Alaska, the height of the crown height is as much as 80 mm (Table 1) and the ratio to root is only 0.06.
Open Roots vs. Closed Roots
The adult Equus teeth display short roots that are open-ended implying potential for growth of these roots (Table 1; Figures 2A,B). The roots of old individuals are longer and closed. In domesticated cases, it is more common to find old individuals. In our collection, there are two old Equus caballus specimens where the crowns are almost completely worn off (Table 1). The same is true in AMNH 204019, which is an old specimen of Equus caballus (Figure 1B). In these three, the roots are elongated and not open-ended. This implies the elongated roots have completed their growth. The ratio of these old specimens of Equus caballus for crown height to root length is 0.79 and 0.44, respectively. These ratios are different from those of younger adult individuals where that ratio is 0.06 (Table 1).
It Is Rare to Find Wild Older Individuals in Fossils in Museums
It was possible to evaluate roughly the age of individuals and separate the adults from the old based on the stage of tooth eruption and tooth wear. In brachydont teeth, the entire crown is exposed, and the base of the roots is also exposed. Thus, in brachydont teeth, the roots can be seen at the opening of the alveoli at the base of the crowns but the same is not true in young hypsodont teeth. In these, the roots are very deep in the alveoli and can be seen only when the crown is heavily worn. Using this evaluation procedure, we examined 15 localities with ungulates. The species were: dromomerycids, bovids, cemelids, and equids and reveal a clear pattern. Namely, the majority of the examined specimens are young adults. The very old individuals were few to none (Table 2).
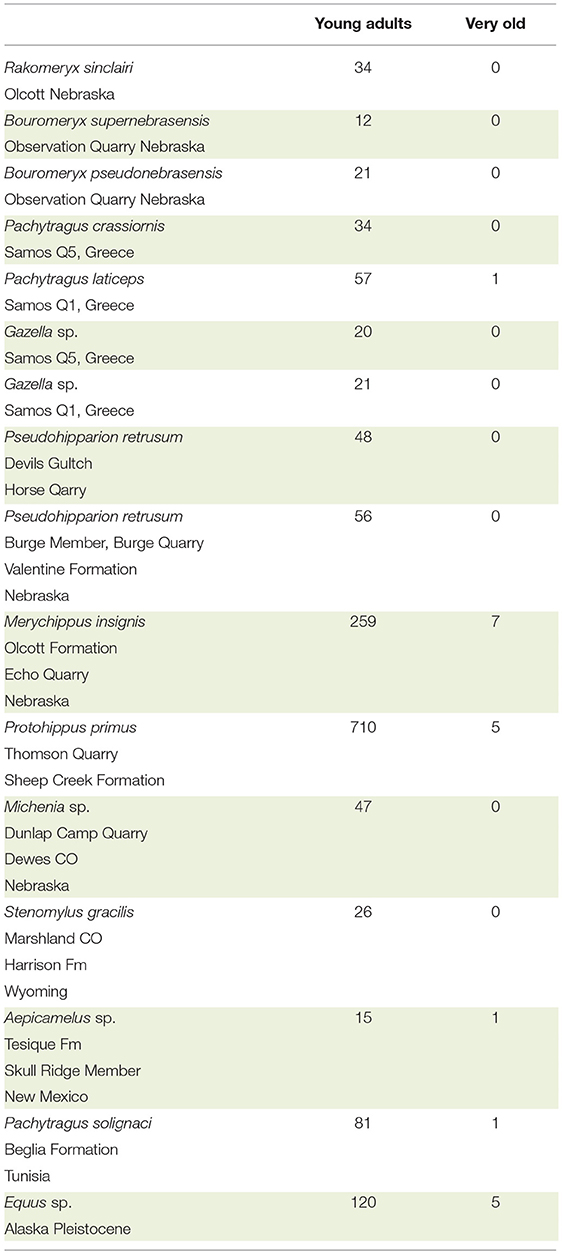
Table 2. Brief survey of number of young adults vs. very old for selected taxa of Dromomerycidae, Bovidae, Equidae, and Camelidae from the AMNH.
The Longitudinal Section Through the Middle of a Second Upper Molar of Equus
The central cavities are the dominant feature in this longitudinal cut and are filled with cementum (green). The dentine (white) is developed by the roots and along the enamel walls. The enamel (red) is not a dominant feature is this view. Using the surface of this longitudinal cut and the colors the areas in square millimeters of each color and hence material is: cementum: 953.369, enamel: 205.668, and dentine: 389.783 mm2.
Mechanics
Another way of evaluating the interaction between force and tooth height is seen in Figure 4A. This bar graph (Figure 4A) allows the changes in tooth height to also parallel the hypsodont's lifespan as traveling from left to right on the x-axis. As an Equus ages, the alveolar tooth length decreases, which is why the graph follows time. Observing the force of the alveolar space compared to the length of the tooth, at 9 mm of alveolar length, there is a drastic increase in the force generated. This exponential trend continues for the last two points of 5 and 2 mm, respectively. Essentially this model indicates that as the tooth length approaches zero millimeters in length, the force of the alveolar space will approach infinity. It can be mathematically understood by having La (alveolar length) in Equation (3) approach zero, therefore resulting in Fa having a limit of infinity. This graph displays an exponential increase in force generated as tooth length decreases. This is similar to Figure 3B, where the force exponentially increased with decrease in alveolar tooth height. Using the same y-axis force values from Figure 4A, a plot shows the alveolar force vs. the age of the hypsodont tooth as seen in Figure 4B. It is observed that the alveolar tooth height and the horse's age are inversely related following the same exponential trends of force as seen in Figures 3B, 4A. Figure 4B shows the dramatic change in the force as the embedded crown is worn off which occurs in our model of wear after age 16.
Discussion
A significant aspect in evaluating the proposed plioroot theory is that we do not yet have the true phylogenetic lineage for the evolution of Equus. The taxa listed represent an approximation to the real evolution which is not studied here and is not known. The taxa are simply used to make the point.
Roots do not appear to increase in size during evolution when the tooth crown increases. In fact, the roots of Equus are smaller than those of Hyracotherium in actual size. The difference in size of the two genera is great (9 kg for Hyracotherium vs. 380–1,000 kg for Equus (Radinsky, 1978; Bongianni, 1987; Edwards, 1994). We found that during evolution, the roots decrease in size as the tooth crown and body size increases. This may be due to the plioroot hypothesis, in which the crown in the alveolus acts as a root. Hence, the true roots alone are incapable of supporting the tooth. We observed no evolutionary diversification or enlargement of the true roots to support the extra crowns as teeth become more hypsodont.
The true roots of Equus are small and open-ended and contain live tissues for the possibility of growth. When a horse is old there is no more crown in the alveolus (Gregory, 1957, text figure 21.45) (Figure 1B). The forces of mastication tremendously affect the small remaining old tooth in several ways. One way is the root response. That is, the true roots develop more and they elongate and close at the tips. These longer roots support better the remaining feeble crown. Thus, elongation and closure of the root is delayed until the crown is almost entirely worn. Delayed development of the true roots is a heterochrony phenomenon and it is possibly dynamically interactive with the forces applied on the crown. When the crown is worn, the mastication forces acting on it are very strong. This is interesting and reinforces our hypothesis that the young tooth's crown embedded in the alveolus acts as a supporting root.
As previously stated, in old individuals, prior to death, very little, if any crown remains in the alveoli (Gregory, 1957; Rooney et al., 1977; Loweder and Mueller, 1998; Pence, 2002). It is known that when an individual runs out of crown it will die from starvation. In wild Equus, individuals often die well-before their entire enamel crown is used up. This observation is true for Equus and for other ungulates. Many samples have shown the absence of numerous old individuals in the fossil record. There are clearly exceptions to this observation but in general, it is true. In the Alaska Equus sp. sample, we found 120 adults and five old individuals. In domesticated species of Equus, it is possible for an individual to live up to the point where little or no enamel remains (Loweder and Mueller, 1998; Pence, 2002). The data suggest that individuals die long before they become old. Hence, they die with a substantial amount of tooth crowns unused (Table 2). The excess crown in the alveoli of fossil populations can be explained with our theory of it acting as the plioroot. Naturally, there are other reasons for the early death of extinct individuals. Two of these are predation and catastrophic events. In general, however the plioroot hypothesis cannot be ruled out.
A longitudinal section of the upper M2 is revealing. The cementum dominates and the enamel is less than what would expect. The enamel unsupported by the cementum would fracture. The cementum functions to provide support, and as it wears differently it enhances the relief which is useful during mastication. Thus, in equid evolution it is not the enamel that becomes taller alone, but that along with a thick cementum layer. As it is, the enamel wears and forms cutting edges which are constantly supported by the cementum and the dentine (Rensberger et al., 1984; Fortelius, 1985).
The hypothesis that the excess crown in the alveoli can be explained as two functions: by acting as a reservoir for wear in the oral cavity and as a root. This observation applies to Equus and it can be extended to all other hypsodont ungulates. DeMiguel et al. (2015) discussed the interplay of increased tooth crown height and chewing efficiency in Cervidae. In their study, they showed how selenodont teeth respond to become more hypsodont with an increased force of stress. What these authors found is that stresses are more concentrated in small areas (where the mastication loading occurs) as the teeth are more hypsodont. Accordingly, hypsodonty is interesting as an adaptation because it enables selenodont teeth to adopt a mechanically improved design that increases the pressure whilst shearing foods, which is highly advantageous when feeding on mechanically resistant, tough, and fibrous foods.
From Merychippus to Equus, the primary dental change is an increased degree of hypsodonty which follows increasing grazing (Matthew, 1926; Simpson, 1951; Stebbins, 1981; MacFadden, 1994; Strömberg, 2002; Mihlbachler et al., 2011) and/or exposure to exogenous grit (Gregory, 1957; Rensberger et al., 1984; Semprebon et al., 2016). The hypsodont tooth is interesting as an adaptation and has been a leading force in the evolution of Equidae (Damuth and Janis, 2011; Mihlbachler et al., 2011; Strömberg, 2011; Semprebon et al., 2016).
The three buccal styles described earlier inhibit the rotation of the tooth during mastication. The mastication rotation would be strongest in the direction of the buccal side as the mastication is slightly oblique (Fortelius, 1985, Figure 25) and buccal. The styles of the upper molars play the role of anchors. The usefulness of these styles becomes evident in older individuals where teeth and the molars often rotate as the styles are not present to prevent this.
The results of modeling the fulcrum and the forces show that the crown in the alveolus acts to function as a root. Hence, enlargement of the true roots is not necessary.
During mastication, the two different stresses are exerted on the teeth in various combinations. One extreme is that of transverse forces; another is that of vertical forces. In general, the Equus true roots appear to be conservative in shape and size and are small. The various ribs and styles as well as the vertical walls of the enamel clearly act as anchors supporting the active crown, preventing it from rotating and mal-occluding. For more vertical mastication modes, the true roots probably can sufficiently support the crown. In transverse forces as in cutting vegetation and grazing, the true roots cannot be of much support. In these conditions, hypsodonty evolves roots out of the additional crowns; the plioroots. These are hypotheses that need further testing.
Applying the laws of mechanics and torque to a two-dimensional class I lever representation of a hypsodont tooth, there appears to be a direct correlation between the force generated by the alveolar space and the length of the alveolar crown. Seen in Figures 3B, 4, as the alveolar part of the crown becomes shorter due to wear, there is a linear increase in the force needed to maintain the tooth's stability and hence effectiveness. During the final years of the crown's life there is an exponential increase in the alveolar force due to the diminishing alveolar tooth length. This relates to Equation (1) when applying to a class I lever model. Assuming mastication is a constant force, the torque needed to counterbalance the system is based on the force generated and distance (Equation 1). If the alveolar tooth is continuously decreasing in height, the force will increase due to the inverse relationship.
Representing a single hypsodont tooth as a two-dimensional class I lever it is determined that the force needed to maintain the tooth during mastication linearly increases for approximately the first 12 years of the hypsodont tooth's life before exponentially increasing for the remainder of its life (Figures 3B, 4). The Equus tooth is much more stable when the alveolar crown is longer, and hence more hypsodont. This provides a longer moment arm allowing the alveolar force to be drastically less during mastication. The presence of the alveolar portion of the tooth crown not only delivers the tooth vital material throughout its life but also likely provides essential mechanical support as indicated from the proposed model. Therefore, the extra enamel is likely there for two reasons, support and availability for eruption and ultimate wear in the oral cavity. The former is new to science, while the latter has been known and studied (Fortelius and Solounias, 2000).
We believe the abrupt increase in force for each bite shown reflects in nature why there is no large gradation of brachydont to hypsodont teeth in various species. The three types of teeth that exist (brachydont, hypsodont, mesodont) do not exist in a continuum. Rather they can be sorted into limited, albeit three, distinct categories (e.g., Fortelius and Solounias, 2000; Solounias and Semprebon, 2002; Semprebon et al., 2016). This observation needs to be studied further.
Is the extra tooth of hypsodont animals a reserve for hardships? If that were so, there would be fossil localities where old individuals have been sampled due to hardships upon these populations. However, there are no such samples of ungulates known to us in the fossil record. Hardship cases are not cited by Damuth and Janis (2011) as they surveyed all the reasons of hypsodonty.
Concluding Remarks
The physical fact that the crowns of hypsodont ungulates are embedded in the jaws and maxillae makes the concept of the crown acting as a necessary reinforcement to the root highly probable. Mechanically, it would seem to be impossible for such crowns not to act as roots, and we have proven this to be true for Equus.
The high transverse forces encountered with grit, abrasive vegetation or with high attrition were probably the selection for Equus hypsodonty and for other ungulate hypsodonty in its dual function; an extra crown to wear and an extra root. Equus and other species needed extra crowns for grit, abrasive, and attractive properties of foods. Species also had to evolve a type of root to endure the strong stresses during mastication. The available true roots were not designed for this and apparently, as in so many other examples, nature used what was adjacent and available; namely the crown. Selection favored the increase of crown height. An enamel crown is a formidably strong and an important support structure as a root. Add to this embedded crown the styles and the ribs, and you make a strong peg which also resists rotation.
The evolution of cementum external to enamel was needed to enable the periodontal ligaments to bind with the lamina dura of the alveolus. The same part of the crown can be used as a root in the alveolus or for wear in the oral cavity.
Author Contributions
NS conceived the idea for this original research paper and collected the data at the museums for Tables 1, 2 while putting together the figures and text. IB worked on the text and tables and plots. ZC developed the physics of the root hypothesis. MD worked at the museum collecting data and worked on the text.
Conflict of Interest Statement
The authors declare that the research was conducted in the absence of any commercial or financial relationships that could be construed as a potential conflict of interest.
Acknowledgments
We sincerely thank the Paleontology and Mammalogy Departments of the American Museum of Natural History (New York), for access to specimens. We thank Loic Costeur in the Naturshitorishes Museum in Basel. Peter Robison and Jacob Veldhuisen at the University of Colorado Museum in Boulder. We thank Perry Habecker and Ralph Steimer for equine material, at the New Bolton Center of Pennsylvania. Special thanks to Ray Bernor, Matthew Mihlbachler, and Gina Semprebon. We thank Henry Galiano, Akinobe Watanabe and two reviewers. We thank the Anatomy Department at the New York Institute of Technology College of Osteopathic Medicine. The NYIT COM has supported the publication costs.
References
Dacre, I. E., Kempson, S., and Dixon, P. M. (2008). Pathological studies of cheek teeth apical infections in the horse: 1. Normal entodontic anatomy and dental structure of equine cheek teeth. Vet. J. 178, 311–320. doi: 10.1016/j.tvjl.2008.09.025
Damuth, J., and Janis, C. M. (2011). On the relationship between hyspodonty and feeding ecology in ungulate mammals, and its utility in paleoecology. Biol. Rev. 86, 733–758. doi: 10.1111/j.1469-185X.2011.00176.x
DeMiguel, D., Azanza, B., Cegoñino, J., Ruiz, I., and Morales, J. (2015). The interplay between increased tooth crown-height and chewing efficiency, and implications for Cervidae evolution. Lethaia 49, 117–129. doi: 10.1111/let.12139
Fortelius, M. (1985). Ungulate cheek teeth: developmental, functional, and evolutionary interrelations. Acta Zool. Fenn. 180, 1–76.
Fortelius, M., and Solounias, N. (2000). Functional characterization of ungulate molars using the abrasion-attrition wear gradient: a new method for reconstructing paleodiets. Am. Mus. Novi. 3301, 1–36.
Gould, S. J. (1977). Ontogeny and Phylogeny. Cambridge, MA: President and Fellows of Harvard College.
Janis, C. (1988). “An estimation of tooth volume and hypsodonty indices in ungulate mammals, and the correlation of these factors with dietary preference,” in Teeth Revisited: Proceedings of the VIIth International Symposium on Dental Morphology, Mémoires du Muséum National d'Histoire Naturelle, (série C), eds Russell, D. E., Santoro, J. P., and Sigogneau-Russell, D. (Paris: Muséum national d'Histoire naturelle), 367–387.
Janis, C. (2008). “An evolutionary history of browsing and grazing ungulates,” in The Ecology of Browsing and Grazing (Berlin; Heidelberg: Springer), 21–45.
Kirkland, K. D., Baker, G. J., Manfra, M. S., Eurell, J. A., and Losonsky, J. M. A. (1996). Effects of aging on the endodontic system, reserve crown, and roots of equine mandibular cheek teeth. Am. J. Vet. Res. 57, 31–38.
Kopke, S., Angrisani, N., and Staszyk, C. (2012). The dental cavities of equine check teeth: three-dimensional reconstructions based on high resolution micro-computed tomography. BMC Vet. Res. 8:173. doi: 10.1186/1746-6148-8-173
Loweder, M. Q., and Mueller, E. P. O. (1998). Dental disease in geriatric horses. Dental disease veterinary clinics of North America. Equine Pract. 14, 365–380.
MacFadden, B. J. (1994). Fossil Horses: Systematics, Paleobiology, and Evolution of the Family Equidae. Cambridge University Press.
Mihlbachler, M. C., Rivals, F., Solounias, N., and Semprebon, G. M. (2011). Dietary change and evolution of horses in North America. Science 331, 1178–1181. doi: 10.1126/science.1196166
Mihlbachler, M. C., and Solounias, N. (2006). Coevolution of tooth crown height and diet in oreodonts (Merycoidontidae, Artiodactyla) examined with phylogenetically independent contrasts. J. Mammal. Evol. 13, 11–36.
Pence, P. (2002). Equine Dentistry: A Practical Guide. New York, NY: Lippincott Williams and Wilkins.
Rensberger, J. M., Forstén, A.-M., and Fortelius, M. (1984). Functional evolution of the cheek tooth patter and chewing direction in Tertiary horses. Paleobiology 10, 439–452.
Renvoisé, E., and Michon, F. (2014). An evo-devo perspective on ever-growing teeth in mammals and dental stem cell maintenance. Front. Physiol. 28:324. doi: 10.3389/fphys.2014.00324
Rooney, J. R., Sack, W. O., Habel, R. E., and Sadler, L. L. (1977). Guide to the Dissection of the Horse Rooney's Guide to the Dissection of the Horse (No. Sirsi) i9780960115211).
Semprebon, G. M., Rivals, F., Solounias, N., and Hulbert, R. C. (2016). Paleodietary reconstruction of fossil horses from the Eocene through Pleistocene of North America. Palaeogeogr. Palaeoclimatol. Palaeoecol. 442, 110–127. doi: 10.1016/j.palaeo.2015.11.004
Simpson, G. G. (1951). Horses: The Story of the Horse Family in the Modern World and Through Sixty Million Years of Evolution. Oxford: Oxford University Press.
Sneva, F. A., Mayland, H. F., and Vavra, M. (1983). Soil ingestion by ungulates grazing a sagebrush-bunchgrass range in eastern Oregon. Oregon Agricultural Experiment Station Special Report. Corvallis, OR:Oregon State University, 182, 1–48.
Solounias, N., Fortelius, M., and Freeman, P. (1994). Molar wear rates in ruminants: a new approach. Annal. Zool. Fenn. 31, 219–227.
Solounias, N., and Semprebon, G. M. (2002). Advances in the Reconstruction of Ungulate Ecomorphology and Application to Early Fossil Equids. American Museum of Natural History Novitates Number 3366, 1–49.
Strömberg, C. A. E. (2002). The origin and spread of grass-dominated ecosystems in the Late Tertiary of North America: preliminary results concerning the evolution of hypsodonty. Palaeogeogr. Palaeoclimatol. Palaeoecol. 177, 59–75. doi: 10.1016/S0031-0182(01)00352-2
Strömberg, C. A. E. (2006). Evolution of hypsodonty in equids: testing a hypothesis of adaptation. Paleobiology 32, 236–258. doi: 10.1666/0094-8373(2006)32[236:EOHIET]2.0.CO;2
Strömberg, C. A. E. (2011). Evolution of grasses and grassland ecosystems. Ann. Rev. Earth Planet. Sci. 39, 517–544. doi: 10.1146/annurev-earth-040809-152402
von Koeningswald, W. (2011). Diversity of hypsodont teeth in mammalian dentitions—construction and classification. Palaeontographica 294, 63–94. doi: 10.1127/pala/294/2011/63
White, T. E. (1959). The Endocrine Glands and Evolution, No. 3: Os Cementrum, Hypsodonty and Diet. Contributions to the Museum of Paleontology. The University of Michigan 13, 211–265.
Williams, S. H., and Kay, R. F. (2001). A comparative test of adaptive explanations of hypsodonty in ungulates and rodents. J. Mammal Evol. 8, 207–229. doi: 10.1023/A:1012231829141
Keywords: Equus, hypsodont, roots, evolution of hypsodonty, crowns as roots
Citation: Solounias N, Danowitz M, Buttar I and Coopee Z (2019) Hypsodont Crowns as Additional Roots: A New Explanation for Hypsodonty. Front. Ecol. Evol. 7:135. doi: 10.3389/fevo.2019.00135
Received: 30 January 2019; Accepted: 05 April 2019;
Published: 03 May 2019.
Edited by:
Gina Marie Semprebon, Bay Path University, United StatesReviewed by:
Daniel DeMiguel, Fundacion Agencia Aragonesa para la Investigacion y el Desarrollo, SpainDanielle Fraser, Canadian Museum of Nature, Canada
Copyright © 2019 Solounias, Danowitz, Buttar and Coopee. This is an open-access article distributed under the terms of the Creative Commons Attribution License (CC BY). The use, distribution or reproduction in other forums is permitted, provided the original author(s) and the copyright owner(s) are credited and that the original publication in this journal is cited, in accordance with accepted academic practice. No use, distribution or reproduction is permitted which does not comply with these terms.
*Correspondence: Nikos Solounias, bnNvbG91bmlAbnlpdC5lZHU=