- 1Vermont Center for Ecostudies, Norwich, VT, United States
- 2Department of Terrestrial Ecology, Norwegian Institute for Nature Research, Trondheim, Norway
Integrated models of the ecology of migratory species require tracking of individual migratory organisms throughout the annual cycle. Here, we report the first information on the movement patterns of nine Upland Sandpipers (Bartramia longicauda) that were captured at breeding sites in Kansas and Massachusetts, and tracked with GPS and PTT tags to non-breeding sites in South America. Upland Sandpipers were extreme migrants that regularly made non-stop flights that were >5,000 km in length and lasted up to 7 days. Sandpipers traveled up to 20,000 km per year in their annual movements. Our project resulted in a series of new discoveries. Sandpipers regularly crossed major ecological barriers during migration, which included long oceanic flights, high elevation mountains, and tropical forests. Migrating birds used known stopover sites in the central flyway of North America and eastern slope of the Andes in South America, and a subset of birds wintered in core non-breeding sites in the Pampas ecoregion of Uruguay and Argentina. We documented new staging sites at canefields in the mountain valleys of Colombia, grasslands in the Llanos of Venezuela, and at airports along the Atlantic Coast of the US. Unexpectedly, some sandpipers spent the non-breeding season on river islands in the Amazon basin, and pastures in the Cerrado ecoregion of Brazil; areas not previously known to host overwintering Upland Sandpipers. Like many other migratory birds in the Western Hemisphere, Upland Sandpipers had elliptical migration routes within the Southern Hemisphere, moved among separate activity areas during the non-breeding season, migrated faster during northbound than southbound migration, and spent more time at non-breeding than breeding sites. Collectively, the birds used sites across much of northern South America as a broad front migrant. Overall, the migratory patterns of Upland Sandpipers were more similar to migratory landbirds than to shorebirds that typically stage at wetlands and coastal estuaries. Upland Sandpipers should be buffered against habitat loss and degradation at local sites within their migratory range, but it may be difficult to protect specific sites or broad landscapes that would be needed to conserve a high percentage of the global population.
Introduction
Long distance migration between breeding and non-breeding areas is a common life history strategy for animals that live in seasonal environments, and understanding the biology of migratory movements is central to four core areas of ecology. In functional ecology, mechanistic questions include study of the morphological and physiological adaptations for long distance movement, the navigation systems used to travel through unfamiliar environments, and the timing of migration in relation to reproduction, feather molt, organ regulation, and other events in the annual cycle (Piersma and Gill, 1998; Hedenström, 2008; Alfaro et al., 2018). In population biology, the goals are to understand the role of food, predation, and climate as limiting factors, and how carryover effects and density-dependence may act to regulate population numbers (Newton, 2006; Rakhimberdiev et al., 2015; Watts et al., 2019). In evolutionary ecology, the adaptive significance of migration is considered in terms of tradeoffs between the demographic costs and benefits of movement, and the role of past events and phylogenetic history in shaping migratory routes (Ruegg and Smith, 2002; Alerstam et al., 2003). In conservation biology, increasing concerns about population declines among migratory species have increased interest in identifying stages of the annual cycle and sites where conservation actions can be targeted, and understanding patterns of migratory connectivity that link spatially structured populations at different stages of the annual cycle (Vickery et al., 1999; Fraser et al., 2012; Jahn et al., 2017; Pearce-Higgins et al., 2017; Cohen et al., 2018). Integration of knowledge across these four key areas has become the basis for development of full-annual-cycle (FAC) models (Hostetler et al., 2015; Marra et al., 2015).
Integrated models of the ecology of migratory species require tracking of individual small-bodied animals across continental-scale distances. New technologies including geolocators, GPS tags, satellite transmitters, and other miniaturized tracking devices have provided a wealth of new movement data (McKinnon and Love, 2018; Sergio et al., 2018; Hofman et al., 2019). The emerging information has shown that migration systems are shaped by species characteristics such as phylogeny, body size, and social systems, as well as environmental features such as the distribution of critical habitats and ecological barriers to migration.
Among migratory birds, shorebirds are remarkable examples of long-distance migrants that often travel up to 20,000–40,000 km in the course of their annual cycle (Battley et al., 2012; Lanctot et al., 2016; Conklin et al., 2017). Migration strategies are often closely linked to body size due to physiological relationships between fuel stores and flight efficiency that determine maximum flight distance (Warnock, 2010). Among migratory shorebirds, small-bodied species can make short “hops” of 1,000–5,000 km (Western Sandpiper Calidris mauri; Iverson et al., 1996; Semipalmated Sandpiper C. pusilla; Brown et al., 2017), mid-sized species may “skip” up to 5,000–8,000 km (Great Knot C. tenuirostris Lisovski et al., 2016; Red Knot C. canutus; Niles et al., 2010), whereas large-bodied species can make longer “jumps” of up to 7,000–11,000 km (Ruddy Turnstones Arenaria interpres; Minton et al., 2011; Bar-tailed Godwits Limosa lapponica; Battley et al., 2012; Hudsonian Godwits L. haemastica, Senner et al., 2014). Space use and movements of migratory shorebirds are also linked to variation in mating systems and social behavior. Socially monogamous and polyandrous species often show strong fidelity to breeding sites (Weiser et al., 2017; van Bemmelen et al., 2019), whereas promiscuous species can be more vagile with low site fidelity and wide-ranging movements during the breeding season (Lanctot et al., 2016; Kempenaers and Valcu, 2017).
The migratory shorebirds that breed or stage in native grasslands of North America include both short-distance migrants that remain on the continent (Page et al., 2014; Pierce et al., 2017; Ruthrauff et al., 2019), and long-distance migrants that travel to South America (Blanco and López-Lanús, 2008; Penner et al., 2015; Jahn et al., 2017). In the Western Hemisphere, intercontinental shorebird migrants must cross major ecological barriers including the water barriers of the Gulf of Mexico and Caribbean Sea, high elevation terrain in the Andes mountains, and unsuitable habitats including the vast tropical forests of the Amazon Basin (Bayly et al., 2018). In this study, we used new tracking technologies to conduct one of the first investigations of the individual, year-round movements of Upland Sandpipers (Bartramia longicauda). Upland Sandpipers are long distance migrants that use temperate grasslands on both the breeding grounds in North America (Bowen and Kruse, 1993; Garvey et al., 2013; Sandercock et al., 2015) and non-breeding grounds in southeast South America (White, 1988; Blanco and López-Lanús, 2008; Alfaro et al., 2015, 2018). Little is known about migratory connectivity of this species because banding effort has been low and band recoveries are rare (Garber et al., 1997; Houston et al., 1999), and previous satellite tracking has provided information on southbound migration for a single bird (Grosselet et al., 2019). Based on specimen and natural history records, migratory routes are thought to include corridors through the Great Plains and Atlantic Coast, stopover sites in central America, and southern routes east of the Andes (Blanco and López-Lanús, 2008; Houston et al., 2011), as well as newly discovered sites along the Pacific coast of northern Chile (Medrano et al., 2018).
Population numbers of Upland Sandpipers are stable within their core range in the Great Plains of US and Canada, but are declining at the edge of their distribution in the Upper Midwest and New England (Osborne and Peterson, 1984; Houston, 1999; Vickery et al., 2010; Andres et al., 2012). The Upland Sandpiper is an area-sensitive species that requires large tracts of native grasslands with heterogeneous vegetative structure (Vickery et al., 1994; Sandercock et al., 2015). Threats on the breeding grounds include habitat loss due to expansion of rowcrop agriculture and afforestation in New England (Vickery et al., 1999; Foster et al., 2002), and habitat degradation due to changes in rangeland management in the Great Plains (Sandercock et al., 2015; Hill and Renfrew, 2019a). Threats on the non-breeding grounds include intensification of livestock grazing and loss of grasslands to rowcrops (Blanco and López-Lanús, 2008; Jahn et al., 2017). Threats during migration include exposure to agricultural chemicals and legal harvest (Strum et al., 2010; Pérez-Arteaga et al., 2019), and regular mortality events have been reported at high elevation lakes in the central Andes of Ecuador (Vickery et al., 2010). Conservation planning for Upland Sandpiper has been difficult because migratory strategies, key habitats, and migratory connectivity have been unknown (Vickery et al., 2010; Jahn et al., 2017).
We fitted adult Upland Sandpipers with satellite tags on their breeding grounds in Kansas and Massachusetts. Our objectives were to use new tracking technologies to collect basic data on the migratory routes, timing, and movement behavior of individual birds. A number of shorebirds in the Western Hemisphere migrate along north-south routes (Myers et al., 1990; Page et al., 2014), sometimes with westerly routes in spring and easterly routes in autumn (Senner et al., 2014; Brown et al., 2017). We predicted that Upland Sandpipers from Kansas and Massachusetts would migrate elliptically along separate routes in the midcontinent and Atlantic Coast but converge in the non-breeding range in Uruguay and northern Argentina. Population studies have indicated that the breeding period in Kansas lasted 3.0 mos from late April to mid-July, and the non-breeding season in Uruguay lasted 3.5 mos from mid-November to late February (Sandercock et al., 2015; Alfaro et al., 2018). We predicted that sandpipers would be stationary during these two stages but would be in transit at other times of year. Upland Sandpipers are a mid-sized shorebird with a body mass averaging 140–160 g, and we predicted that they might be able to make long “skips” of 5,000–8,000 km. The species is socially monogamous with a mate defense mating system, semi-colonial nesting, and biparental incubation (Bowen and Kruse, 1993; Casey et al., 2011). Color-banded adults have annual return rates of 38.1% (n = 189 birds, Mong and Sandercock, 2007). We predicted that adult sandpipers would show site fidelity to breeding areas.
Methods
Study Sites and Field Methods
Our field sites included three US military installations and a natural preserve located in the western and eastern parts of the continental range of Upland Sandpipers. Our two field sites in Kansas included Fort Riley (39.207°N, −96.824°W) and the Konza Prairie Biological Station (Konza; 39.100°N, −96.608°W). Field sites in Massachusetts included Joint Base Cape Cod (Cape Cod; 41.658°N, −70.521°W) and Westover Air Reserve Base (Westover; 42.199°N, −72.542°W). The habitat at our Kansas field sites was tallgrass prairie dominated by warm-season grasses with a mixture of forbs. The field sites were used for military training or ecological research. The adjoining lands in Kansas were private rangelands managed with prescribed fires in spring and used for cattle grazing, and were also suitable habitat for sandpipers (Sandercock et al., 2015). Our field sites in Massachusetts were active airfields with air strips surrounded by open fields dominated by cool-season grasses with a short sward during our field work (<30 cm). At Cape Cod, we also captured sandpipers in grassland habitats at a covered landfill that was also on the base and 0.8 km from the airfield. Landscapes surrounding our field sites in Massachusetts had small patches of grasslands embedded in a matrix of suburban housing, golf courses, and forested areas, and were less suitable for sandpipers.
We captured Upland Sandpipers during April and May 2016. We searched for roosting birds at night by driving slowly (~5 km h−1) along dirt tracks in the prairie or on the edge of airport runways. Roosting sandpipers were located with handheld spotlights, and then approached on foot and captured with long-handled dip nets. We recorded body mass and morphometrics at capture and considered birds to be females if they were >160 g in body mass or if we could detect the presence of an egg by palpitating the abdomen. We attached tracking tags to sandpipers with leg loop harnesses constructed from elastic cord (1 mm, Stretch Magic, Pepperell Crafts, Massachusetts, US). Harnesses were individually fit to each bird by adjusting the leg loops around the upper thigh so that the tag was positioned above the pelvis with a whip antenna extending out over the tail (Figure 1). Harnesses were individually adjusted for a relaxed but secure fit and were secured with one double-overhand knot that was coated with a thin film of Loctite superglue (Henkel Corporation, Connecticut, US). Our harness design has little effect on behavior or seasonal survival of sandpipers but may reduce annual return rates (Mong and Sandercock, 2007; Smith et al., 2017). Individual sandpipers were identified by a unique Bird ID: a two-digit code with the breeding location (e.g., FR = Fort Riley and WO = Westover), a three-letter code representing the tag type (PTT vs. GPS), and a unique two-digit identifier (Table 1).
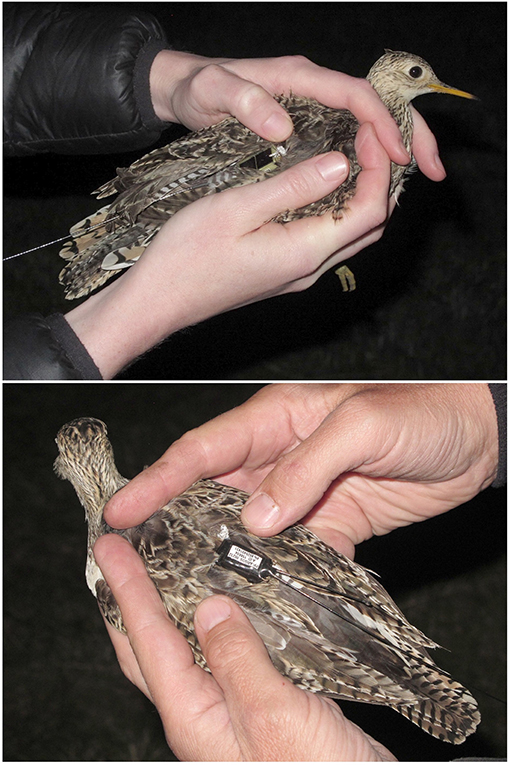
Figure 1. Upland Sandpipers with tracking tags attached with an elastic leg-loop harness; solar-powered Argos Platform Transmitter Terminals (5 g PTT, Microwave Telemetry, USA, Top) and battery-powered PinPoint Argos-GPS tags (4 g, Lotek Wireless, Canada, Bottom). The two birds were captured at Konza Prairie, Kansas on the night of 4 May 2016 (KO-PTT-69, Top; KO-GPS-02, Bottom).
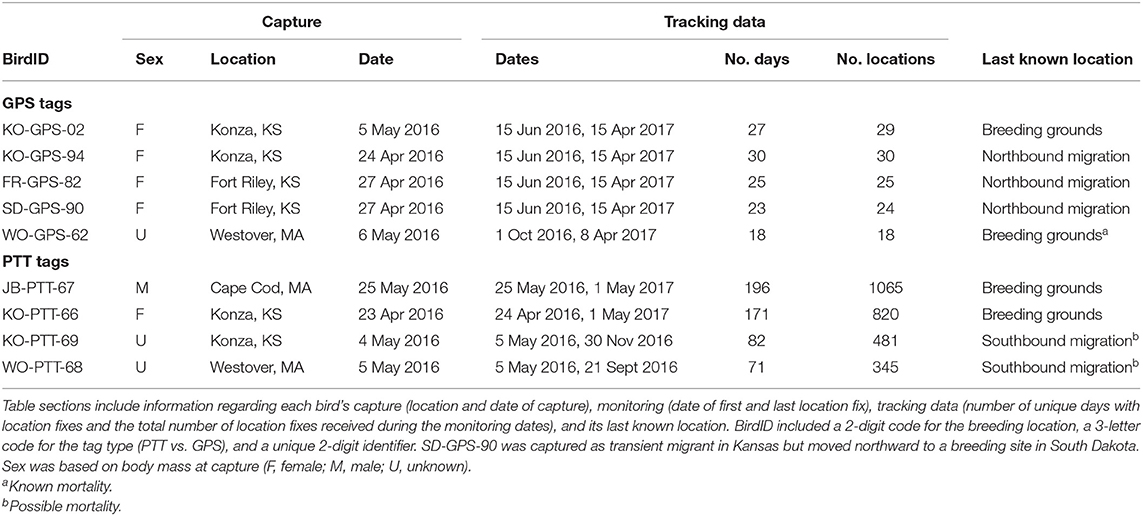
Table 1. Summary of movement data and status of Upland Sandpipers monitored with PinPoint GPS Argos tags (GPS tags), and solar-powered Argos Platform Transmitter Terminals (PTT tags) from 24 April 2016 until 1 May 2017.
Tracking Tags and Movement Data
We used two types of tracking tags to investigate the migratory movements of Upland Sandpipers: 4-g battery-powered PinPoint GPS Argos tags (GPS tags, Lotek Wireless, Canada), and 5-g solar-powered Argos Platform Transmitter Terminals (PTT tags, Microwave Telemetry, Inc., Maryland, US). The GPS tags were less expensive than the PTT tags (ca. US$1,200 vs. $4,500), but PTT tags can provide real time movement data with more locations. We opted to use a combination of GPS and PTT tags to obtain good quality movement data for a representative sample of birds, but our study was not designed to directly compare tag performance. Mass and dimensions were similar for both types of tags. GPS tags were 2.5 L × 1.4 W × 0.7 H cm and had an 18-cm whip antenna, which was reinforced at the base to guard against bird-inflicted damage. Solar PTT tags were 2.5 L × 1.5 L × 0.8 W cm wide with a 22 cm whip antenna. The average body mass of sandpipers that received GPS tags was 171.7 ± 34.3 (SD) grams (range = 135 to 229 g, n = 11), whereas the average body mass of birds that received PPTs was 175.5 ± 15.6 (SD) grams (range = 162 to 196 g, n = 4). The tracking devices with the elastic harness were <3% of the bird's body mass at the time of capture. All of the Upland Sandpiper movement data recorded by the GPS and PTT tags were archived at Movebank (www.movebank.org; Hill and Renfrew, 2019b), an open online database for animal tracking data (Wikelski and Kays, 2018).
GPS Tags
We deployed PinPoint GPS Argos tags on 11 Upland Sandpipers between 24 April and 25 May 2016. Six birds were captured in Kansas (Fort Riley: n = 2; Konza: n = 4), and five birds were captured in Massachusetts (Cape Cod: n = 1; Westover: n = 4). The GPS tags had an expected battery life of just under 1 year, and a memory capacity for storage of 30 locations from GPS fixes with an expected accuracy of ~10 m. Movements and habitat use of Upland Sandpipers have been studied on the breeding grounds (Mong and Sandercock, 2007; Sandercock et al., 2015). Here, we were mainly interested in collecting movement data outside of the breeding season, and we programmed the GPS tags to start collecting locations ~2 months after deployment, on 15 July 2016, and to continue for a 9-month period until 15 April 2017. We set the check frequency for biweekly fixes during the expected stationary periods of July to August and December to January, and for weekly fixes during the expected migratory periods of September to November and February to April. All GPS location fixes were programmed to occur during midday at 12:00 UTC, and were sequentially added to the tag memory. To recover information from the GPS tags, we set a pre-programmed date of 15 April 2017 to automatically upload all stored data on the tags to the Argos satellite system. We anticipated that some sandpipers would still be migrating northward in April 2017, but we expected battery life to expire by 1 May 2017 (Lotek Wireless, pers. comm.). Movement data were then downloaded from the satellite system and sent to us via email. Successful recovery of movement data required the GPS tag to be functional, and its battery operational through 15 April 2017. Movement data could not be recovered if a tag malfunctioned or was damaged in the 12-month period before the scheduled upload date.
PTT Tags
We deployed solar Argos Platform Transmitter Terminal tags on four Upland Sandpipers between 23 April and 25 May 2016. Two birds were captured in Kansas (Konza: n = 2), and two birds were captured in Massachusetts (one each at Cape Cod and Westover). We were able to monitor several birds for multiple years with PTT tags, but here we limit our analysis to the first year of tracking data collected from April 2016 through April 2017. PTT transmitters are monitored by the Argos satellite system, which is operated by Collecte Localisation Satellites (CLS). Transmitter locations are calculated via the Doppler effect based on the frequency shift in the signal from the transmitter that is received by the Argos satellites (Lopez et al., 2013). Thus, the solar PTT tags can produce multiple daily locations for multiple years but the accuracy and precision of locations can vary depending on environmental conditions. The Argos system assigns location estimates from PTT tags to seven different location quality classes based on a Kalman filtering algorithm (LC 3 to LC B). Some location estimates can be too imprecise to receive any error estimate, but the assigned error radii usually range from <0.25 to >1.5 km (Douglas et al., 2012). Validation trials with stationary PTT tags at known locations have suggested that the error estimates from CLS may be too optimistic, and in one study >75% of the true locations were not contained within the estimated error polygon (Douglas et al., 2012; Boyd and Brightsmith, 2013).
Location information from the PTT tags was passed directly from the Argos satellite system to the Movebank system. We used two integrated tools in Movebank to improve the quality of our data from the PTT tags and our subsequent data products. First, we applied the Douglas Argos-filter (DAF) algorithm to our data, which removed low-quality locations from our dataset. The DAF filter identifies problematic locations and movement rates by examining distances, velocities, and turning angles within clusters of sequential locations. Application of the DAF filter may reduce the number of location estimates for subsequent analysis, but the overall accuracy of the remaining data is increased by 50–90% (Douglas et al., 2012). We opted to use the “best hybrid” method of the DAF algorithm in Movebank which was developed for filtering avian movement data. We used the default settings with the exception of two parameters that we adjusted following the recommendations of Douglas et al. (2012). We set the maximum sustainable movement rate over several hours (MINRATE) to 145 km h−1, and we set the maximum redundant setting (MAXREDUN) for filtering data to 5 km during the stationary periods of breeding and non-breeding periods, and to 15 km for the two migratory periods (D. Douglas, pers. comm.). The MAXREDUN setting retains near-consecutive locations within those distance thresholds to ensure independent error estimates. Setting MAXREDUN to 15 km results in large error estimates which are acceptable when analyzing continental scale movements (Douglas et al., 2012). Second, we used less optimistic error estimates for locations rather than those provided by CLS with our PTT data (D. Douglas, pers. comm.). For each Argos Doppler location class (LC) we assumed the following error radii: LC 3 = 0.46 km, LC 2 = 0.91 km, LC 1 = 1.81 km, LC 0 = 6.66 km, LC A = 1.59 km, and LC B = 1.95 km. Data assigned to the location class of LC Z or “invalid location” were not used in our analyses.
Statistical Analysis
The GPS and PTT tags differed in the quantity and quality of movement data: GPS tags recorded a single location every 1–2 weeks with high accuracy, whereas the solar PTT tags generally recorded multiple locations per day with relatively lower accuracy. Thus, the movement data from the GPS and PTT tags required different analytical methods. For both types of tags, we examined patterns of habitat use by examining bird locations in relation to geographic features identified in aerial photographs from Google Earth. Timing and speed of migratory movements were difficult to determine from GPS tags due to low frequency of location fixes. Thus, we used the median of dates that bounded the onset of a seasonal or behavioral change such as the initiation of southbound migration. It was easier to determine the onset and duration of seasonal movements with daily fixes from the PTT tags. For birds with PTT tags, we calculated travel rate (km day−1) as the total migration distance divided by the number of days that a bird was moving. Also, we measured ground speed (km h−1) for long flight segments over open water when birds were likely to be in continuous flight. We were able to detect only long stopover events for birds with GPS tags because locations were recorded every 1–2 weeks. For birds with PTT tags, we defined migratory stopover events as local movements within an area of a 50-km radius that lasted more than 24 h. To calculate total migration distance, we first calculated a centroid for stopover events for birds with PTT tags to make our estimates comparable to birds with GPS tags. We then used the distance function in the “move” package for Program R to estimate the length of flight segments and migration distances for migratory paths during northbound and southbound migration (Kranstauber et al., 2018; R Core Team, 2018).
The daily locations from the PTT tags allowed for additional seasonal analyses of movements and space use of migratory Upland Sandpipers. We used dynamic Brownian bridge movement models (dBBMM) to analyze the detailed information available from the PTT tags (Kranstauber et al., 2012). The dBBMM model has at least two advantages: it controls for temporal autocorrelation among sequential locations that are collected a short time apart, and it tests for behavioral changes within a movement path associated with turning radius and length of movements. For example, star-shaped movements from a central point might indicate a roosting site, short movements with a high rate of turning might indicate residence at a staging site, and long unidirectional movements are expected with migration. The dBBMM model is especially appropriate for analyzing location data that are collected at frequent (<1 h apart) but irregular intervals, which was the case for our data from the PTT tags (Kranstauber et al., 2012; Walter and Fischer, 2018).
We filtered the movement tracks for Upland Sandpipers with the DAF algorithm in Movebank to screen implausible locations (Douglas et al., 2012), and then analyzed the movement data with the brownian.bridge.dyn function in the “move” package of program R. For each individual sandpiper, we analyzed the movement data separately for the four stages of the annual cycle: breeding in North America, southbound movements during autumn migration, non-breeding in South America, and northbound movements during spring migration. Long gaps between consecutive locations can result in large movement variances and create problems for model convergence with dBBMMs. Thus, we excluded gaps of >3 days from the variance calculation using the burst function of the “move” package (B. Kranstauber; pers. comm.). We used a margin of 11 and a window size of 25 locations to obtain stable estimates of the Brownian motion variance. During the breeding and non-breeding periods, we used dBBMMs to calculate 50% (core areas) using small grid cells of 0.001 km2, and calculated area of the utilization distributions using ArcGIS (ESRI, 2018). For the migratory periods, we calculated 99% utilization distributions to characterize movement paths with larger grid cells of 0.01 km2. The dBBMMs explicitly incorporate location uncertainty into the estimation of the utilization distributions, which is a better approach than treating the location estimates as if they were recorded without error (Kranstauber et al., 2012).
Results
Performance of Tracking Tags
We deployed tracking tags on 15 Upland Sandpipers in Spring 2016 and recovered movement data from 5 of 11 GPS tags (45%) and all four solar PTT tags (100%, Table 1). Four of 5 successful GPS tags (80%) worked as programmed and recorded movement data for a 10-month period from 15 June 2016 until 15 April 2017. For unknown reasons, one GPS tag only recorded movement data for a 6.2-month period that started on the non-breeding grounds on 1 Oct 2016 and continued until 8 April 2017 (WO-GPS-62). Six of 11 GPS tags (55%) provided no movement data. Two of 4 PTT tags provided a full year of movement data (KO-PTT-66 and JB-PTT-67). The other two tags (50%) provided data for 4.6–6.8 mos, and then stopped transmitting data during southbound migration in 2016 (KO-PTT-69 and WO-PTT-68). The PTT tags required constant recharging of the battery from the solar panels and it was not uncommon for the tags to suspend reporting, but then restart again after a hiatus of 2–3 days. Overall, our analyses of seasonal variation in migratory movements and space use were based on nine Upland Sandpipers, where the GPS tags provided 18–25 locations over 190–305 days of monitoring and the PTT tags recorded locations on 71 to 196 unique days during 140–373 days of monitoring (Table 1).
Mortality and Annual Survival
We documented one known mortality event among our 15 tagged birds. A sandpiper that was marked with a GPS tag on 6 May 2016 at Westover ARB was recovered dead a year later on 17 May 2017 on an airport runway and 0.89 km from the original capture location (WO-GPS-62). While cause of death was unknown, a collision with an aircraft is plausible, as the bird had successfully carried the tag and harness for an entire annual cycle. The GPS tag was recovered from the carcass but showed no signs of damage. The GPS tag apparently malfunctioned because the movement track was incomplete: the first locations were recorded during the non-breeding period when the bird was in Mato Grosso province in Brazil, but the last locations were recorded during northbound migration from Vichada province in Colombia. The GPS tag failed to record movements between North and South America during either migration period.
We were unable to determine the fate of tagged birds if the GPS tags failed to upload data as scheduled or if the PTT tags stopped transmitting in <12 months. In both situations, missing data could have been due to harness failure, damage to the tracking tag or the external antenna, tag malfunction, or death of the bird. Six of the 11 GPS tags provided no data, but we had expected some attrition since the tags were programmed to upload data 1 year after deployment on 15 April 2017. Two birds with PTT tags disappeared during southbound migration before or after long distance water crossings. One bird from Kansas was last recorded near Victoria, Texas on 30 Nov 2016 close to the Gulf of Mexico (KO-PTT-69), whereas a second bird from Massachusetts was last recorded south of Calabozo, Venezuela on 21 Sept 2016 after a successful flight across the Caribbean Sea (WO-PTT-68). If we assumed that all losses were due to mortality, the minimum annual survival rates were 36.4% for GPS tags (4 of 11) and 50% for PTT tags (50%, 2 of 4). A pooled survival rate of 40.0% (6 of 15) for birds with GPS and PTT tags in this study was not significantly different from annual return rates of Upland Sandpipers marked with VHF radio tags (20.9%, 18 of 86, Fisher's Exact test: P = 0.18) or with color bands only (38.1%, 72 of 189, P = 1) from our long-term population study at Konza Prairie Biological Station in Kansas (Mong and Sandercock, 2007).
Breeding Season
We obtained information on breeding season movements for 8 of 9 sandpipers because one GPS tag did not start recording locations until the bird had reached the non-breeding grounds (WO-GPS-62). One sandpiper captured at Fort Riley, Kansas on 27 April 2016 turned out to be a migratory transient (SD-GPS-90). The first locations from the GPS tag on 15 June and 17 July indicated that this bird had continued moving north after it was tagged, and spent the breeding season near Hosmer, South Dakota. The other 7 of 8 sandpipers remained on breeding home ranges near their capture sites in Kansas and Massachusetts from late April until late summer. The minimum duration of the breeding season from first capture until southbound migration averaged 81 days (range = 57–121 days) or 22% of the annual cycle (range = 16–33%, n = 8 birds). Patterns of space use during the breeding season were estimated for the four birds with PTT tags (Table 1). Space use and home range size differed between birds in open tallgrass prairie in Kansas and the grassland remnants in Massachusetts (Figure 2). Two sandpipers in Kansas had multiple activity centers and large home ranges with a 50% core area of 49 and 64 km2 (KO-PTT-66 and KO-PTT-69). In contrast, the two birds in Massachusetts had a single activity center and smaller home ranges with a 50% core area of 22 and 23 km2 (JB-PTT-67 and WO-PTT-68).
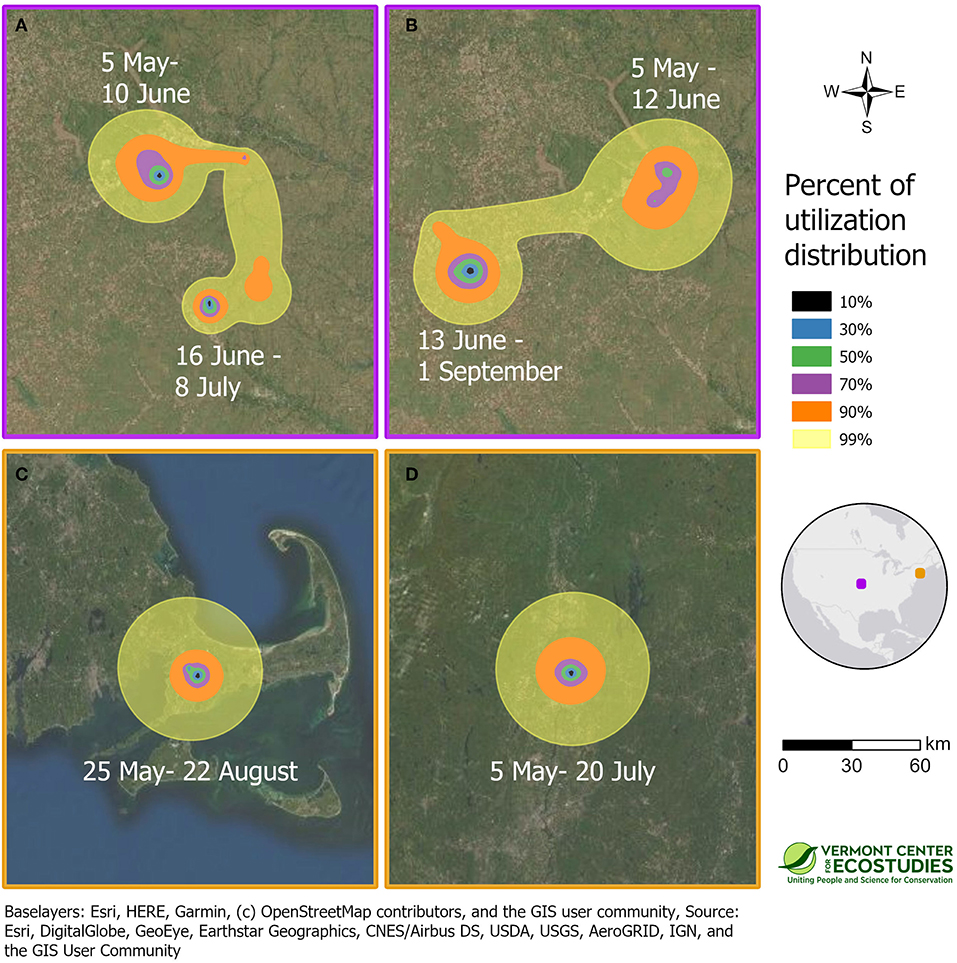
Figure 2. Space use on the breeding grounds during the summer of 2016 for four sandpipers marked with PTT transmitters at tallgrass prairie sites in Kansas [KO-PTT-66 (A) and KO-PTT-69 (B)], and prairie remnants in Massachusetts [JB-PTT-67 (C) and WO-PTT-68 (D)]. Approximate 99% utilization distributions indicate probability of use for each sandpiper throughout the breeding period, and were calculated using dynamic Brownian bridge movement models. The border color of a panel indicates its location on the inset map, and all panels are shown at the same spatial scale.
Southbound Migration
We obtained complete routes for southbound migration for six sandpipers: four birds with GPS tags and two birds with PTT tags (Figure 3). The three birds from Kansas and the lone sandpiper that spent the breeding season in South Dakota, followed a narrow inland corridor in the Great Plains to the Texas coast, and then made long distance flights across the Gulf of Mexico and Central America to staging sites in the Andes of Colombia and Ecuador. The two birds from Massachusetts made shorter regional movements along the Atlantic coast and then made long flights over the Caribbean Sea to inland sites in northeast Venezuela. Sandpipers followed one of two migration routes upon arrival in South America: three birds crossed the Amazon basin to reach non-breeding sites in central Brazil, and three birds continued down the eastern slopes of the Andes with stopover sites in Bolivia, Brazil (Mato Grosso do Sul), and Paraguay en route to non-breeding sites in Uruguay and northern Argentina.
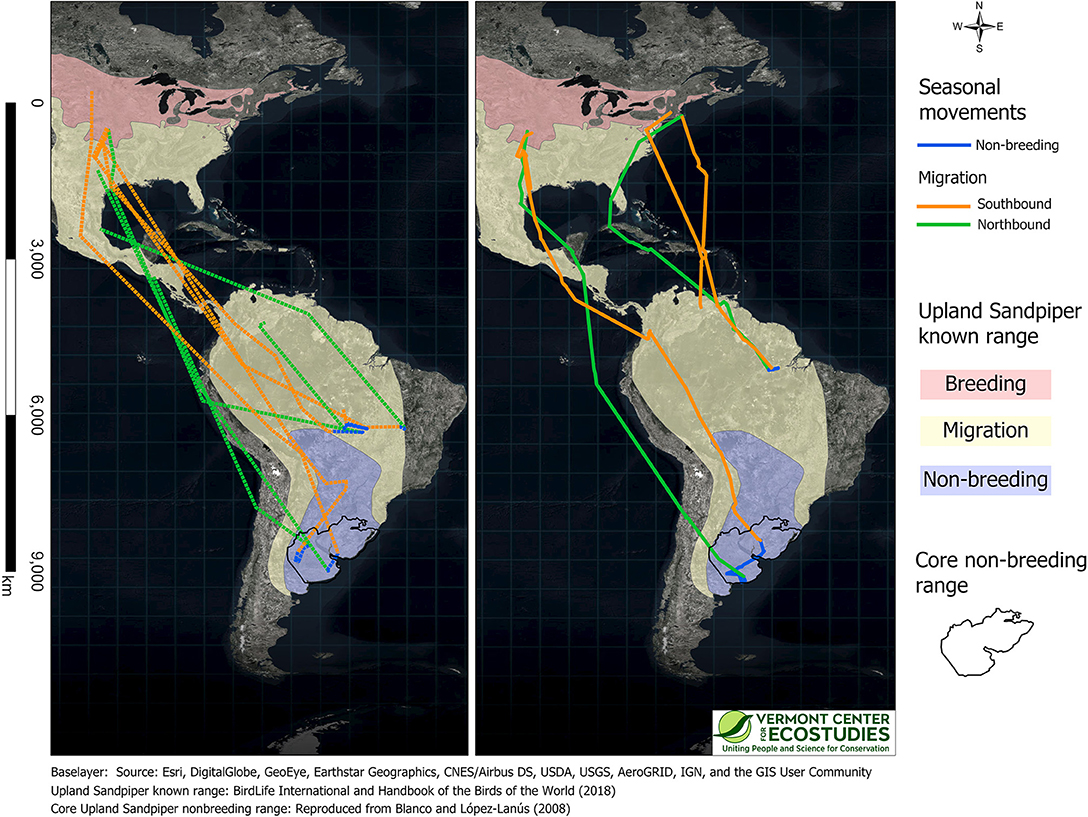
Figure 3. Annual movement paths for nine Upland Sandpipers captured in Kansas or Massachusetts and tracked with GPS (Left, dashed lines, n = 5 birds) or PTT tags (Right, solid lines, n = 4 birds). We recorded complete tracks for six birds (4 GPS, 2 PTT), only northbound migration for one bird with a GPS tag (WO-GPS-62) and only southbound migration for two birds with PTT tags (KO-PTT-69 and WO-PTT-68). Movement paths were drawn by connecting consecutive locations with orthodromes, and include southbound migration (Aug-Nov, orange), winter movements (Dec-Feb, blue), and northbound migration (Mar-May, green). Colored semi-transparent polygons are the known breeding, migration, and non-breeding range of Upland Sandpiper (BirdLife International Handbook of the Birds of the World, 2018), and are reproduced with permission here. The core non-breeding range (black polygon, both panels) represents the area with the highest densities of Upland Sandpipers on the non-breeding grounds (reproduced with permission from Blanco and López-Lanús, 2008).
Upland Sandpipers traveled long distances during southbound migration and the total length of routes averaged 8,793 km (range = 5,410–10,675 km, n = 6 birds, Table 2). Individual variation included a 2-fold difference in distance and a 3-fold difference in duration. The shortest migration was a sandpiper from Massachusetts that wintered in northern Pará province, Brazil, and this bird traveled 5,410 km over 36 days (JB-PTT-67). One of the longest southbound migration routes recorded was a sandpiper tagged in Kansas that traveled 10,040 km over 123 days to a non-breeding site in northwest Uruguay (mean stopover duration = 8 days, range = 1 to 38 days, n = 13 stopover events; KO-PTT-66). The length of migration segments between consecutive stopover events averaged 1,057 km (range = 20–3,758 km, n = 20 segments). The longest non-stop flights occurred over water for two sandpipers from the breeding population in Massachusetts. One bird completed a long-distance flight from Cape Cod to a site west of El Tigre, Venezuela that lasted up to 5 days and included a 3,758 km non-stop flight (JB-PTT-67; Figure 4). A second bird flew 3,432 km from Baltimore, Maryland to a site north of Calabozo, Venezuela in the Llanos grasslands (WO-PTT-68). We also recorded two instances of reverse migration with relatively short flights back to the north (138 km and 290 km) that occurred during southbound migration of two birds through Oklahoma (KO-PTT-66 and KO-PTT-69). Both cases occurred at midday and at approximately the same time in early August.
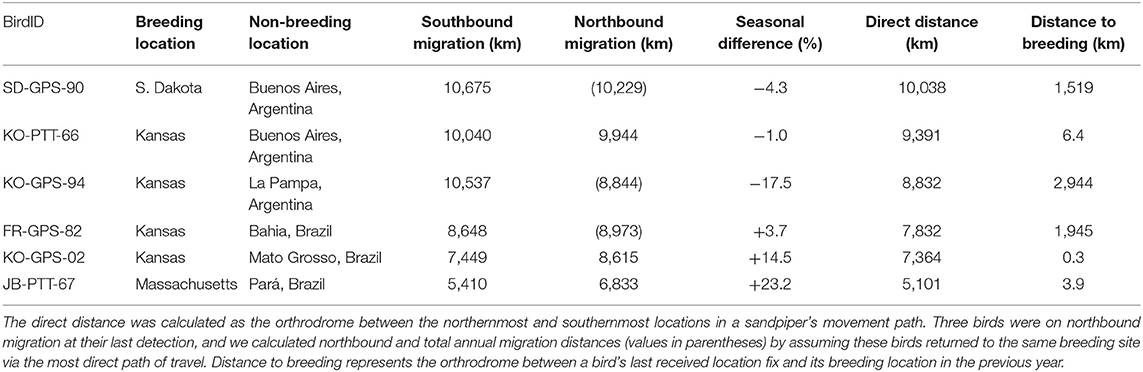
Table 2. Summary of migration distances throughout the annual cycle for six Upland Sandpipers from 24 April 2016 to 1 May 2017, including the southbound and northbound migration distances, and the difference (%) between the two migration routes.
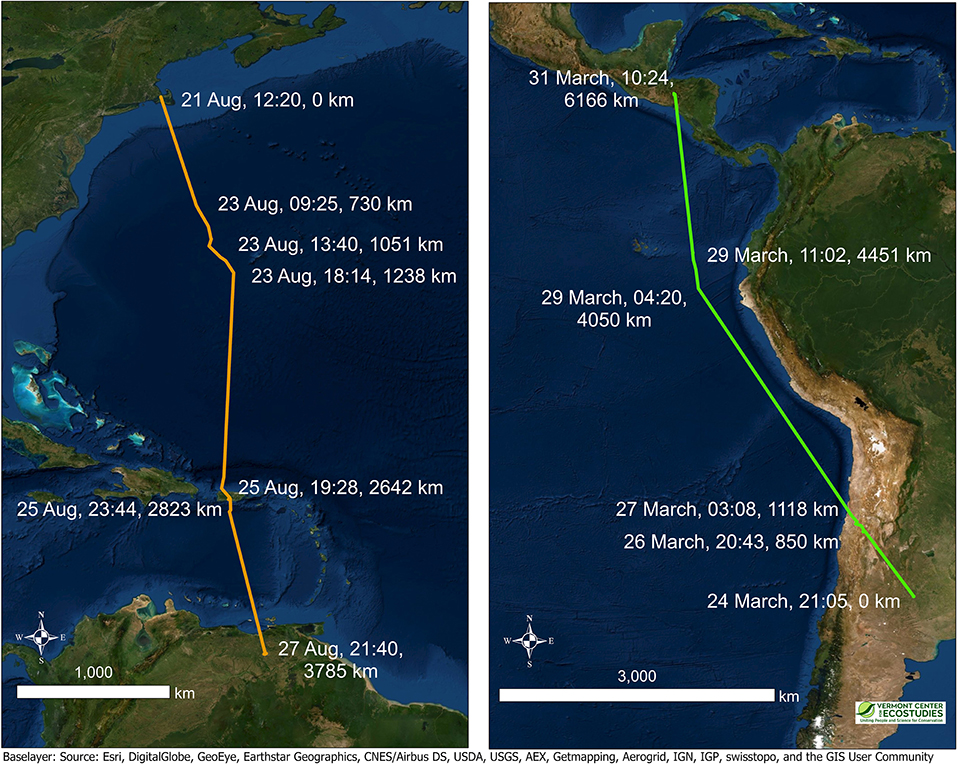
Figure 4. Two examples of migratory segments illustrating non-stop flights over long distances for multiple days by Upland Sandpipers with PTT tags, 2016–2017. Extreme migratory movements included a southbound flight of 3,785 km in a 6-day period over the Atlantic Ocean from Massachusetts to Venezuela (Left, JB-PTT-67), and a northbound flight of 6,166 km in a 7-day period across the Andes and along the Pacific coast from Argentina to Honduras (Right, KO-PTT-66). Colored lines connect consecutive locations and highlight the longest suspected non-stop segments of the migratory flights for the two birds. Annotated values for a subset of fixes in the figure include the date, time (in UTC), and the cumulative distance traveled (km). The exact times of departure and arrival were not known precisely, but we provide timestamps for fixes immediately preceding and following the non-stop flight segments. Note the difference in spatial scales between the two panels.
We recorded the onset of migration at the end of the breeding season for seven sandpipers, and all birds initiated migration in the 2-month period between 1 July and 1 September. The total duration of southbound migration averaged 99 days (range = 36–146 days) or 26% of the annual cycle (range = 10–40%, n = 6 birds). Migration flights were usually initiated around dusk with ground speeds that ranged between 33 and 61 km h−1, including an average flight speed of 40 km h−1 during 3,476 kilometers of overwater flight (n = 4 overwater migration segments). The average rate of travel was 556 km per day for the six sandpipers where tracking tags recorded their entire southbound migration route. Stopover events averaged 12 days (range = 1–54 days, n = 15 stopover events) among birds with PTT tags. The longest staging event in North America was for a sandpiper that flew 484 km from Westover, Massachusetts to Baltimore Washington Airport, Maryland, where it spent 54 days (20 July to 12 September) before continuing on a 4-day non-stop flight of 3,441 km to Venezuela (WO-PTT-68). Long staging visits after arriving in South America were also common. One sandpiper from Kansas staged for 41 days (6 September to 16 October) near Cali, Colombia before traveling >4,500 km over a 21-day period to a non-breeding site in Uruguay (KO-PTT-66). Similarly, a bird from Massachusetts staged for 28 days (27 August to 23 September) in northern Venezuela before moving another 1,614 km over a 5-day period to a site in northern Brazil (JB-PTT-67).
Non-breeding Season
We collected movement data from seven sandpipers at their non-breeding sites in South America. The three general areas where non-breeding birds were located included: northern Brazil, central Brazil, and Uruguay/Argentina. Of four sandpipers that wintered in Brazil, one bird overwintered on islands within the Amazon River at the mouth of the Tapajós River in the northern state of Pará (JB-PTT-67), and three birds overwintered further south in the Cerrado ecoregion at the southeastern edge of the Amazon Basin in the states of Mato Grosso and Bahia (FR-GPS-82, KO-PTT-69, WO-GPS-62). The remaining three birds overwintered in the Pampas ecoregion of Uruguay and a 3-province region of northern Argentina (Buenos Aires, La Pampa, and Córdoba, SD-GPS-90, KO-PTT-66), including one bird that also used overwintering sites in southern Brazil (KO-GPS-94). Two sandpipers that were captured at sites only 27 km apart in Kansas overwintered ca. 2,600 km apart from each other in central Brazil (KO-GPS-82) and Uruguay (KO-PTT-66, Figure 3). On the other hand, one sandpiper from Kansas overwintered at a site in central Brazil (KO-GPS-02) that was <100 km from a sandpiper from the Massachusetts breeding population (WO-GPS-62).
Migratory sandpipers completed southbound migration in the 2-month period between late September and late November, and then spent an average of 147 days (range = 116–167 days) or 39% (range = 32–46%, n = 7 birds) of their annual cycle at the non-breeding grounds. Upland Sandpipers were not stationary during the winter, and all seven birds used multiple discrete areas on the non-breeding grounds. In one case, a sandpiper tagged with a GPS tag in Kansas spent 35 days in Mato Grosso do Sul, Brazil (8 October to 15 November), and then moved 1,459 km to spend another 105 days at sites southwest of Rosario, Argentina (1 December to 15 March, KO-GPS-94). Local movements were observed among birds with GPS tags wintering in central Brazil. One bird from Kansas used four activity centers that were 20–100 km apart over 169 days (15 October to 1 April, FR-GPS-82), and a bird from Massachusetts moved among five activity centers that were 30 to 400 km apart over a 152-day period (15 October to 15 March, WO-GPS-62). We were able to examine space use during the non-breeding season for two sandpipers tracked with PTT tags. A bird from Massachusetts that wintered in the Amazon had three activity centers with a 50% area of 47 km2 (JB-PTT-67), and a bird from Kansas that overwintered in the Rio de la Plata Basin of Uruguay and Argentina had multiple activity centers with a 50% utilization distribution of 118 km2 (KO-PTT-66, Figure 5). Non-breeding birds used a variety of open lands that included both natural and agricultural habitats. Wintering sites in northern Brazil were islands in the Amazon River with short vegetation affected by seasonal flooding. Stopover and non-breeding sites in Venezuela and Brazil were open cropfields where forests had been cleared for agriculture. Non-breeding sites in Uruguay and Argentina were mainly open grassland habitats in native rangelands used for livestock grazing.
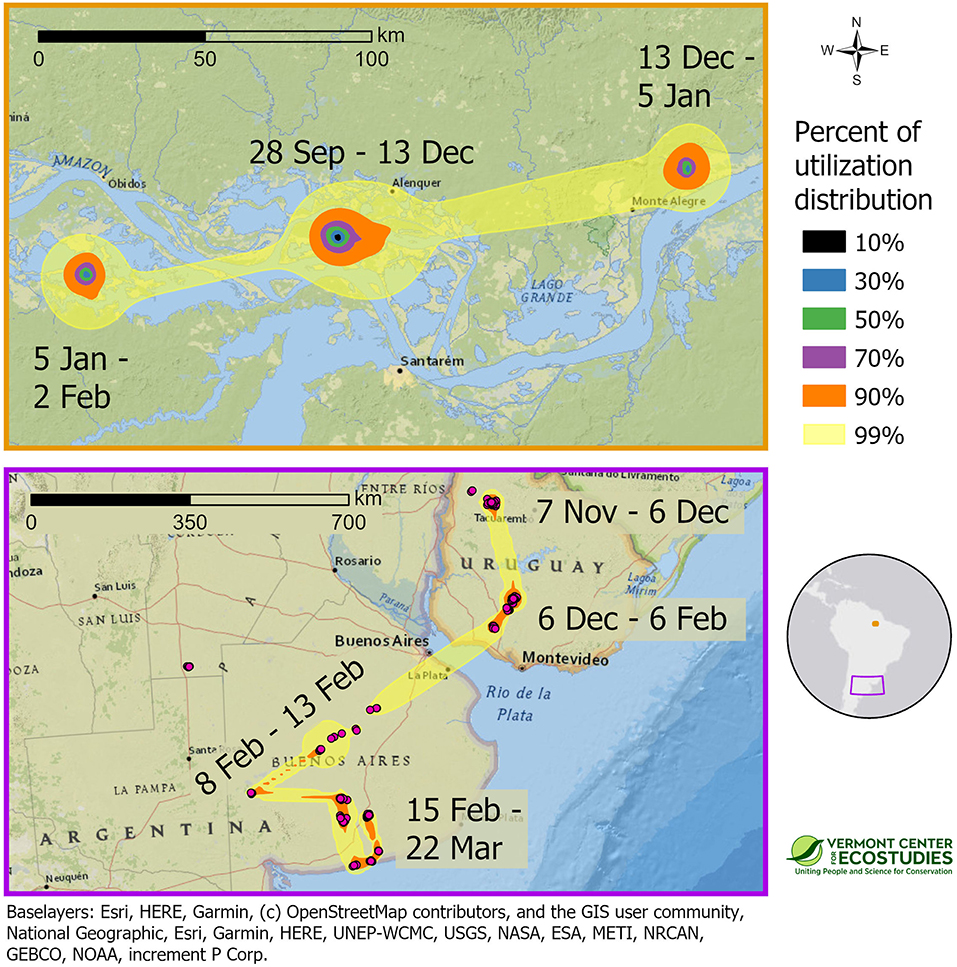
Figure 5. Space use of two Upland Sandpipers, tracked with PTT transmitters, at non-breeding sites in South America during the winter of 2016–2017. One sandpiper from Massachusetts wintered on a network of islands along the Amazon River in Brazil (WO-PTT-68, Top), whereas one sandpiper from Kansas overwintered in Uruguay and northern Argentina (KO-PTT-66, Bottom). Approximate 99% utilization distributions indicate probability of use for each sandpiper during the non-breeding period, and were calculated using dynamic Brownian bridge movement models. Note that scale bars differ between the two panels. Border color of the panels indicates their location on the inset map. Note the difference in scales between the two panels.
Northbound Migration
We obtained routes for northbound migration for six Upland Sandpipers, including four birds with GPS tags and two birds with PTT tags. The total length of estimated northbound migration routes averaged 8,906 km (range = 6,833–10,229 km, n = 6 birds, Table 2). All six birds had elliptical migration routes in South America and in five cases, the paths for northbound movements were west of their southbound migration routes (Figure 3). The westerly routes used during northbound migration were similar in length to the easterly routes used during southbound migration (mean percent difference = +3.1%, range = −4.3 to +23.2%, n = 6 birds). Three birds from Kansas that wintered in the Pampas ecoregion of Uruguay and Argentina moved northwest and crossed the Andes to the southern edge of the Atacama desert of Chile before continuing north over the Pacific Ocean on the west coast of South America (KO-GPS-94, SD-GPS-90, KO-PTT-66). Another bird from Kansas that wintered in Mato Grosso, Brazil flew west and crossed the Andes of central Peru and then turned north after reaching the Pacific coast (KO-GPS-02). All four birds followed the Pacific Coast and made continuous non-stop flights until making landfall in Central America or the southern Great Plains. One bird from Kansas that wintered in Bahia, Brazil had an elliptical migration pattern where the northbound migration route followed a more easterly path to stopover sites in Venezuela and Mexico (FR-GPS-82). Last, a bird from Massachusetts that wintered in Brazil retraced her migratory path to Venezuela, but then used a westerly route where she flew 2,591 km over a 4-day period (9 to 12 April) and stopped over in Cuba for 9 days, then flew 1,686 km and stopped over at an airfield near Blackstone, Virginia for 4 days (24 to 27 April), and then completed the final 791 km back to Cape Cod in 1 day (JB-PTT-67).
Long-distance movements of >5,000 km were a common feature of northbound migration for Upland Sandpipers (Figures 3, 4). The longest recorded flight was for a bird from Kansas that departed the non-breeding grounds in eastern Argentina on 24 March, was detected in flight at night over Chile on 27 March, again off the coast of Ecuador on the morning of 29 March, and continued northward until it reached Honduras on 30 March; a non-stop flight of 7,581 km in 7 days (KO-PTT-66). The flight segment over northern Chile included a 5-h overnight flight that passed 125 km southwest of Ojos del Salado where the mountainous terrain had an average elevation of 3,703 m (range = 2,070–4,792 m, n = 8 locations). Two other sandpipers from Kansas made long-distance movements during the same time period: one bird traveled 5,952 km from Argentina to El Salvador in the week of 19 March to 1 April (KO-GPS-94), and a second bird traveled 4,475 km from the coast of Peru to Texas in the week of 23 March to 1 April (KO-GPS-02).
Upland Sandpipers started migrating northward over a 2-month period between 3 February and 4 April (mean = 17 March, n = 7 birds). The routes used during northbound and southbound migration were similar in length, but birds migrating north completed their movements in half the time, with an average duration of 47 days (range = 23–84 days) or 13% of the annual cycle (6–23%, n = 3 birds). Flight speeds for overwater flight segments averaged 62 km h−1 (range = 60 to 64 km h−1 over 875 km, n = 2 segments). Tagged sandpipers traveled 683 km per day with an average non-stop flight segment of 1,615 km (range = 84–7,581 km, n = 11 segments). Stopover events during northbound migration were similar to southbound migration and typically lasted 11 days (range = 2–28 days, n = 9 events).
Annual Movements and Breeding Site Fidelity
We were able to evaluate the complete annual cycle for six sandpipers with tracking tags (Table 2). The four stages of the annual cycle differed in duration: northbound migration was shorter than southbound migration, and the non-breeding season was longer than the breeding season (Figure 6). Two birds from Kansas (KO-GPS-02, KO-PTT-66) and one bird from Massachusetts (JP-PTT-67) successfully completed migration and returned to the breeding grounds. All three birds showed strong breeding site fidelity and the locations of the tagged birds after 305–373 days of monitoring were <7 km from their previous breeding locations in 2016. A fourth bird with a malfunctioning GPS tag had an incomplete track but this individual also showed site fidelity because it was recovered dead 0.89 km from the original banding site (WO-GPS-62). The remaining three birds with GPS tags were still migrating north when their movement data was uploaded on 15 April; the last known locations on northbound migration were 1,519–2,944 km south of the breeding grounds near San Vincent, El Salvador (KO-GPS-94), Ciudad Madero, Mexico (FR-GPS-82), and Dallas, Texas (SD-GPS-90). We assumed that these three birds returned to the same breeding site for calculation of the round-trip distances traveled during the annual cycle. Total migration distance averaged 17,700 km (n = 6 birds), including a round trip of 20,904 km for a sandpiper that bred in South Dakota, a median of 18,526 km for four birds from Kansas (range = 16,064–19,984 km), and 12,467 km for a bird from Massachusetts (Table 2).
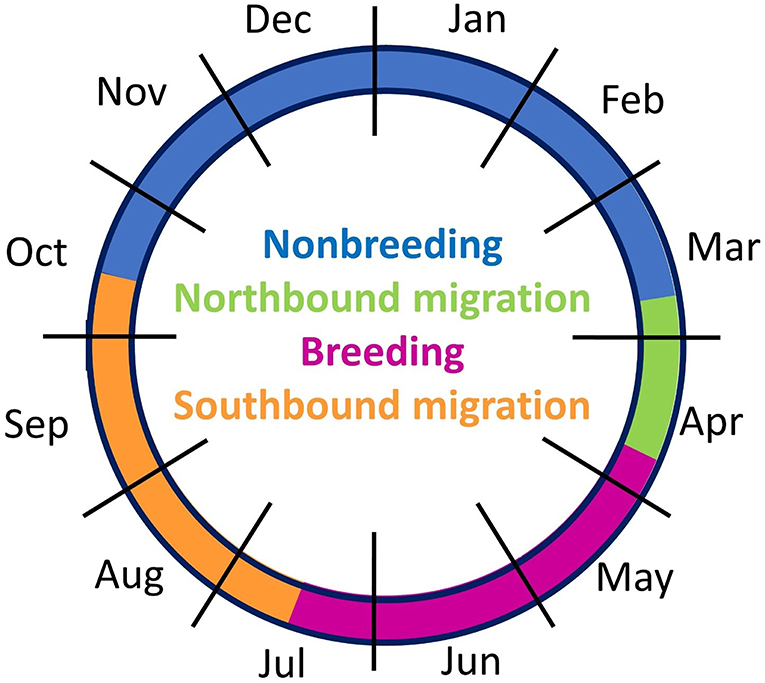
Figure 6. Annual cycle based on movement tracking of nine migratory Upland Sandpipers in the Western Hemisphere, May 2016 to May 2017. The four periods of the annual cycle were based on the median dates of transition between consecutive stages.
Discussion
In our field study, we collected the first complete migratory tracks of individual Upland Sandpipers during their entire annual cycle in the Western Hemisphere. The scope of our project was relatively limited, as a 1-year study with movement data from nine birds captured in two separate breeding populations. Nevertheless, our data provide new insights into the migratory strategies, routes and sites, and the phenological timing of Upland Sandpipers. Our project resulted in three new discoveries. First, Upland Sandpipers were extreme migrants that can travel long distances >20,000 km during their annual cycle. Individual birds used long non-stop flights that were >5,000 km and lasted 5–7 days to cross major ecological barriers. Second, birds from two disparate breeding populations wintered across large areas of South America. We confirmed use of known stopover and non-breeding sites in Uruguay and Argentina, but we also identified unexpected staging sites in Colombia and non-breeding sites in two different areas of Brazil. Last, the migration patterns included several phenomena that have been reported in other migratory birds but were not previously known for Upland Sandpipers, including staging events that lasted up to a month, frequent movements during the non-breeding season, elliptical migration within South America with different northbound vs. southbound routes, and strong fidelity to breeding sites.
Tag Performance and Effects of Tracking Tags
Our project joins previous work in demonstrating that miniature 1-to 5-g GPS and PTT tags can be successfully used to track relatively small-bodied birds throughout their annual cycle (McKinnon and Love, 2018), including Purple Martins (52 g, Progne subis, Fraser et al., 2017), Common Nighthawks (>70 g, Chordeiles minor; Ng et al., 2018), Common Cuckoos (102 g, Cuculus canorus, Vega et al., 2016), and Upland Sandpipers (170 g, this study). The solar-powered Argos PTT tags were more expensive but provided higher resolution movement data with fewer tag malfunctions than the PinPoint GPS Argos tags. Successful completion of long distance migration and carrying tags for multiple years suggests that our tags and harness design had relatively little effect on the movements or demographic performance of Upland Sandpipers (Mong and Sandercock, 2007; this study). Recent analyses have shown that tracking tags attached with elastic leg harnesses usually have little effect on behavior or reproductive output, but may have weak effects on the annual survival rates of small-bodied birds (Weiser et al., 2016; Smith et al., 2017; Brlík et al., 2019). Long-distance flights seem to be a risky part of migration because two sandpipers with PTT tags disappeared around a transoceanic flight, similar to mortality patterns of Whimbrel (Numenius phaeopus) during flights across the Caribbean basin (Watts et al., 2019) and Black-tailed Godwits (Limosa limosa) crossing the Sahara Desert (Loonstra et al., 2019). Despite these losses, annual return rates of sandpipers tagged with GPS and PTT transmitters were comparable to birds marked with color bands only (Mong and Sandercock, 2007). Further tests of the potential impacts of tracking tags awaits future developments in tag miniaturization and improved attachment methods (Wikelski et al., 2007).
Extreme Migration
Upland Sandpipers were known to be long distance migrants based on the wide separation of their breeding and non-breeding ranges in temperate grasslands in the Northern and Southern Hemisphere (Blanco and López-Lanús, 2008; Houston et al., 2011). With our field study, we provide the first data on how individual Upland Sandpipers complete their long migratory movements between temperate grasslands on different continents. Open water, forest habitats, and mountain ranges were not ecological barriers for migrating sandpipers because individual birds made non-stop flights across the Caribbean Sea, Amazon basin, and Andes mountains, and also moved long distances along the Pacific coast.
Our data revealed that Upland Sandpipers were capable of long non-stop flights of up to 5 days and 3,758 km during southbound migration, and up to 7 days and 7,581 km during northbound migration. Individual birds traveled long distances during their annual movements with total migration distances ranging from 12,467 to 20,904 km. While these migratory movements are remarkable, a growing body of evidence suggests that extreme flights are relatively common among migratory shorebirds. Conklin et al. (2017) reviewed evidence for long-jump movements among migratory birds and compiled data showing that 19 other species of shorebirds are capable of making extreme non-stop flights >5,000 km in length and round trip migrations of >20,000 km. The most extreme migrants tend to be large-bodied shorebirds that breed in the arctic or subarctic regions but make long oceanic flights of >10,000 km to reach non-breeding sites in the Southern Hemisphere for total migration distances >30,000 km, including Red Knots (Tomkovich et al., 2013), Bar-tailed Godwits (Battley et al., 2012), and Hudsonian Godwits (Senner et al., 2014).
In this study, we tracked sandpipers that were captured in two breeding populations in their core range in the continental USA. However, a separate disjunct population of Upland Sandpipers breeds in Alaska and the Yukon (Buss, 1951; Houston et al., 2011), and as far north as Ivvavik National Park (69.2°N, Miller et al., 2015), which is roughly 4,400 km north of our field sites in Kansas. The migratory ecology of the boreal populations of Upland Sandpipers remains unknown, but has the potential to be among the longest routes used by migratory shorebirds.
Migratory Routes
Movement tracks of migrating Upland Sandpipers confirmed use of sites that have been identified as important, but also led to discovery of some previously unknown staging and non-breeding sites. Birds that bred in Kansas and South Dakota used a relatively narrow corridor in the Great Plains during southbound migration (Houston et al., 2011), and birds from Massachusetts used grassland habitats at airfields along the Atlantic coast for both breeding and staging (Garber et al., 1997). Newly discovered staging sites used during southbound migration that were not previously known included canefields in mountain valleys of Colombia and the Llanos grasslands of central Venezuela. One of our tagged birds (SD-GPS-90) moved through the high elevation sites near Ozogoche Lagoon in Ecuador where mass mortality events have been reported for this species (Vickery et al., 2010). Grosselet et al. (2019) recently reported a similar path for an Upland Sandpiper tagged in Mexico which also crossed the Andes in northern Ecuador, and continued south along the eastern side of the Peruvian Andes until the signal was eventually lost. Three of the birds that we tracked wintered at sites in the expected non-breeding distribution in the Pampas ecoregion of Uruguay and Argentina (Blanco and López-Lanús, 2008; Alfaro et al., 2018). However, our tracking data showed that four other Upland Sandpipers wintered in two different areas of Brazil, far north of the main non-breeding range, including grassland habitats in the Cerrado ecoregion, and more unexpectedly, river islands in the Amazon basin. Small numbers of Upland Sandpipers were thought to spend the non-breeding season in northern South America (Haverschmidt, 1966; Houston et al., 1999; Blanco and López-Lanús, 2008), and our tracking data have confirmed this prediction. Finally during northbound migration, three tagged birds crossed the Andes through northern Chile, and near sites where Upland Sandpipers have recently been reported as nocturnal migrants (Medrano et al., 2018).
Migratory routes of Upland Sandpipers generally followed a northwest-southeast axis with birds from Kansas using more westerly routes than birds from Massachusetts. Migration along a north-south axis is common among migratory shorebirds in the Western Hemisphere, including both short (Page et al., 2014) and long-distance migrants (Johnson et al., 2016; Brown et al., 2017). A majority of Upland Sandpipers also had elliptical or loop migration with a clockwise pattern within South America where northbound routes were more westerly than southbound routes. For example, the individual bird with the greatest difference in routes had a direct flight over the Caribbean Sea during southbound migration but then used a westerly route and stopped over in Cuba during northbound migration (JB-PTT-67). Elliptical migration has been previously reported for other shorebirds that use eastern flyways to travel to South America (Myers et al., 1990; Senner et al., 2014; Brown et al., 2017; Johnson et al., 2018). The movement pattern may be related to predictable seasonal dynamics of the atmospheric conditions over the Gulf of Mexico and Caribbean Sea, with migratory birds taking advantage of favorable tailwinds (La Sorte et al., 2014; Bayly et al., 2018).
Many shorebirds that migrate long distances show a high degree of structure in their migratory routes, with a majority of a population using key staging sites at inland wetlands along continental flyways (Myers et al., 1987; Senner et al., 2014), coastal estuaries such as Chesapeake and Delaware Bays on the Atlantic Coast (Baker et al., 2004; Johnson et al., 2016), or the Yellow Sea region of eastern China (Battley et al., 2012; Studds et al., 2017). In contrast, the diversity of migration tracks among our tagged birds suggests a pattern of weak migratory connectivity. Birds from two breeding populations were broad front migrants without shared staging sites, and individual movement tracks covered a large area of northern South America. Our analysis was based on a relatively small sample of birds and it is possible that adding more tracks and additional populations would allow identification of migratory network nodes for different breeding and non-breeding populations (Knight et al., 2018), and quantitative analyses of the patterns of migratory connectivity (Cohen et al., 2018). Overall, the migratory patterns of Upland Sandpipers appear to be more similar to migratory landbirds, where migratory connectivity is often fairly weak (Renfrew et al., 2013; Finch et al., 2017; Hill and Renfrew, 2019a).
Reliance on a relatively small number of staging sites increases population vulnerability for migratory shorebirds, but it offers opportunities to target conservation actions. Alternatively, weak migratory connectivity may buffer local breeding populations against loss or degradation of habitat elsewhere in their migratory range. Low densities over a wide distribution make it more difficult to implement conservation measures at specific sites (Vickery et al., 2010; Pearce-Higgins et al., 2017), with two possible exceptions. First, our field sites in Kansas are part of the Flint Hills ecoregion, which has been designated as a Landscape of Hemispheric Importance under the Western Hemisphere Shorebird Reserve Network (WHSRN) based on its importance to Buff-Breasted Sandpipers, Upland Sandpipers, and American Golden-Plovers (Penner et al., 2015). Second, space use and movement tracks indicated that airfields provide critical habitat for breeding and staging sites for migratory Upland Sandpipers (Osborne and Peterson, 1984; Garber et al., 1997; this study). Thus, conservation of eastern populations of Upland Sandpipers would benefit from protection of appropriate habitat within airfields and other remaining patches of native grasslands along the Atlantic coast.
Time-Budgets During the Annual Cycle
Northbound migration (13%) was a shorter period than southbound migration (26%), and Upland Sandpipers spent less time at the breeding (22%) than the non-breeding grounds (39%). Thus, the duration of northbound migration was relatively short because the average ground speed and distances traveled were greater for Upland Sandpipers during northbound (ca. 62 km h−1 and 683 km per day) compared to southbound migration (40 km h−1 and 556 km per day). Our estimates of movement rates were comparable to non-stop oceanic flights on southbound migration for an Upland Sandpiper tagged in Mexico (40 km h−1, Grosselet et al., 2019). Faster northbound migration and similar ground speeds have also been reported in Pacific Golden-Plovers Pluvialis fulva (northbound vs. southbound: 63 and 58 km h−1; Johnson et al., 2011), two subspecies of Bar-tailed Godwits (L.l. baueri: 59–63 and 53 km h−1, L.l. menzbieri: 60–76 and 53–58 km h−1, Battley et al., 2012), Ruddy Turnstones (48–79 and 30–40 km h−1, Minton et al., 2011), and Great Knots (24–92 and 13–74 km h−1; Lisovski et al., 2016). Seasonal differences in migration speed may be related to reproductive advantages of early arrival at the breeding grounds (Weiser et al., 2018; Morrison et al., 2019), or to predation risk during southbound migration (Ydenberg and Hope, 2019). The potential carry-over effects from linkages of events at different stages of the annual cycle have been studied in some shorebirds (Barshep et al., 2011; Senner et al., 2014; Carneiro et al., 2019), but await further investigation in Upland Sandpipers.
One of the main advantages of GPS and PTT tags is that they allow constant monitoring of individuals in space and time, and can record forays that cannot be detected with ground-based telemetry systems (McKinnon and Love, 2018). Our previous estimates of home range size for birds breeding in Kansas were based on VHF radio tags (8.4 km2, Sandercock et al., 2015) and our new estimates based on improved tracking technologies were about seven times larger (49–64 km2, this study). The main difference between estimates was due to our discovery that sandpipers in Kansas had multiple activity centers during the breeding season that were ca. 40–60 km apart. Multiple activity centers might have been due to renesting after clutch failure, foraging to prepare for migration, or flocking with other birds that had completed nesting. Estimates of home range size were smaller for the two birds breeding in Massachusetts, presumably because other available habitat was extremely limited in the surrounding landscapes. Large space requirements help to explain why Upland Sandpipers and other grassland birds are area-sensitive species that are less likely to occur in small grassland fragments (Vickery et al., 1994).
The non-breeding season is sometimes described as a stationary period, and migratory shorebirds that use coastal wetlands are often sedentary during the non-breeding season (Battley et al., 2012; Senner et al., 2014). In contrast, our tracking data revealed that within-season movements were common during the non-breeding season for Upland Sandpipers. Birds did not settle in a single location, but rather moved among consecutive activity centers that were 20–400 km apart before eventually departing on northbound migration. Movement among separate activity areas during the non-breeding season has also been reported for Buff-breasted Sandpipers and Bobolinks Dolichonyx oryzivorus wintering in grassland habitats in South America (Renfrew et al., 2013; Lanctot et al., 2016), Red-necked Phalaropes (Phalaropus lobatus) wintering in the Arabian Sea (van Bemmelen et al., 2019), and a diversity of tropical songbirds (Stutchbury et al., 2016; Bayly et al., 2018; McKinnon and Love, 2018). Migratory birds may be mobile during the non-breeding season because they are tracking ephemeral food resources (Jahn et al., 2010), which for Upland Sandpipers would primarily be grasshoppers and other arthropods (Alfaro et al., 2015).
Our new tracking data suggest that Upland Sandpipers are a highly vagile species because individual birds had multiple activity centers during both the breeding and non-breeding seasons, and the diversity of migratory tracks suggests that they are broad front migrants. Despite this suite of traits, individual sandpipers also demonstrated remarkable homing skills with strong fidelity to breeding sites. Four birds returned to breeding sites that were <6.5 km from their locations in the previous year, despite traveling up to 20,000 km during their annual migration. Upland Sandpipers nest in loose colonies among birds that are genetically related, and both females and males share incubation duties (Casey et al., 2011). Thus, strong breeding fidelity may enhance reproductive success for a long-distance migrant if an experienced sandpiper is able to breed near relatives, repair quickly with a former partner, or nest at a familiar site where they were successful in a previous year.
Future Research
Our project demonstrates that new tracking technologies can provide unexpected insights into the migratory ecology of small-bodied birds, and opens new lines of enquiry for future research. The migratory tracks from a small number of Upland Sandpipers were highly variable and more work is needed to clarify the importance of the new non-breeding areas that we have discovered in Brazil. More tracking data for birds from other breeding populations are needed to better understand migratory connectivity and the population structure of the species within in the continental range vs. boreal populations in Alaska and the Yukon (Buss, 1951; Miller et al., 2015). Our analyses were based on adults only, and tracking of juveniles is needed to understand the ontogeny of migration (Rotics et al., 2016; Vega et al., 2016). Moreover, we tracked birds for a single year and multiple tracks from the same set of individuals are needed to investigate repeatability in migratory behavior (Carneiro et al., 2019; Ruthrauff et al., 2019). Understanding potential carryover effects will require better integration of movement data with additional information on local environmental conditions, habitat use, and the timing of feather molt, fat deposition, and other costly activities (Barshep et al., 2011; Senner et al., 2014; McKinnon and Love, 2018). Similarly, parameterization of full-annual-cycle models requires better methods for determining causes of mortality from different types of tag failure (Sergio et al., 2018; Loonstra et al., 2019; Watts et al., 2019). Upland Sandpipers were capable of extreme migratory movements across oceans and mountainous terrain, and new types of tracking tags with accelerometers and altimeters will provide information on their physiological capacity during sustained flights. Finally, the sandpipers demonstrated a remarkable ability to return to the same breeding sites despite traveling long distances within the Western Hemisphere, and the sensory systems and environmental cues used for navigation will be an important area for future work.
Data Availability Statement
The datasets for migratory movements of tagged birds have been archived at Movebank (www.movebank.org) as a project of the Vermont Center for Ecostudies: Upland Sandpiper Annual Life Cycle Ecology (Movebank ID: 176770813; Hill and Renfrew, 2019b).
Ethics Statement
Our research protocols followed recommended procedures in the Guidelines to the Use of Wild Birds in Research of the Ornithological Council. We conducted our project under government permits for scientific research, including federal and state permits for live capture and tagging of wild birds, and access permits for working at facilities of the US Department of Defense and Konza Prairie Biological Station.
Author Contributions
RR conceived and designed the project. JH and RR directed and executed the project. JH coordinated data management with Movebank. JH and BS conducted statistical analyses of the movement tracks. BS led the writing of the manuscript. All authors contributed to the fieldwork, edits, and approved the final version.
Funding
Funding for this research project was provided by the Legacy Resource Management Program of the US Department of Defense (Project 15-764).
Conflict of Interest
The authors declare that the research was conducted in the absence of any commercial or financial relationships that could be construed as a potential conflict of interest.
Acknowledgments
We thank the biological field technicians and crew leaders for their hard work, and the biologists and staff of the Fire Desks, Air Traffic Control Towers, and Range Controls that supported our research and kept field workers safe while working at Fort Riley (KS), Konza Prairie (KS), Camp Grafton (ND), Fort McCoy (WI), Camp Ripley (MN), Joint Base Cape Cod (MA), Westover Air Reserve Base (MA), and Naval Air Station Patuxent River (MD). Thanks to Asociación Calidris and Jim Giocomo for their assistance in locating sandpipers, and Sarah Davidson at Movebank and David Douglas at USGS for project guidance and generous use of their time. Thank you to Daniel Blanco, Mark Balman, and BirdLife International permission to reproduce the Upland Sandpiper range maps. Two referees provided helpful comments that improved the quality and content of our manuscript. The ESRI Conservation GIS Program provided software.
References
Alerstam, T., Hedenström, A., and Åkesson, S. (2003). Long-distance migration: evolution and determinants. Oikos 103, 247–260. doi: 10.1034/j.1600-0706.2003.12559.x
Alfaro, M., Sandercock, B. K., Liguori, L., and Arim, M. (2015). The diet of Upland Sandpipers (Bartramia longicauda) in managed farmland in their Neotropical non-breeding grounds. Ornitol. Neotrop. 26, 337–347. Available online at: http://journals.sfu.ca/ornneo/index.php/ornneo/article/view/69
Alfaro, M., Sandercock, B. K., Liguori, L., and Arim, M. (2018). Body condition and feather molt of a migratory shorebird during the non-breeding season. J. Avian Biol. 49:e01480. doi: 10.1111/jav.01480
Andres, B. A., Smith, P. A., Morrison, R. G., Gratto-Trevor, C. L., Brown, S. C., and Friis, C. A. (2012). Population estimates of North American shorebirds, 2012. Wader Study Group Bull. 119, 178–194. Available online at: https://www.waderstudygroup.org/article/1967/
Baker, A. J., González, P. M., Piersma, T., Niles, L. J., De Lima Serrano Do Nascimento, I., Atkinson, P. W., et al. (2004). Rapid population decline in Red Knots: fitness consequences of decreased refuelling rates and late arrival in Delaware Bay. Proc. R. Soc. Lond. B 271, 875–882. doi: 10.1098/rspb.2003.2663
Barshep, Y., Minton, C., Underhill, L. G., and Remisiewicz, M. (2011). The primary moult of Curlew Sandpipers Calidris ferruginea in North-Western Australia shifts according to breeding success. Ardea 99, 43–51. doi: 10.5253/078.099.0106
Battley, P. F., Warnock, N., Tibbitts, T. L., Gill, R. E., Piersma, T., Hassell, C. J., et al. (2012). Contrasting extreme long-distance migration patterns in Bar-tailed Godwits Limosa lapponica. J. Avian Biol. 43, 21–32. doi: 10.1111/j.1600-048X.2011.05473.x
Bayly, N. J., Rosenberg, K. V., Easton, W. E., Gomez, C., Carlisle, J., Ewert, D. N., et al. (2018). Major stopover regions and migratory bottlenecks for Nearctic-Neotropical landbirds within the Neotropics: a review. Bird Conserv. Int. 28, 1–26. doi: 10.1017/S0959270917000296
BirdLife International Handbook of the Birds of the World (2018). Bird Species Distribution Maps of the World. Version 2018.1. Available online at: http://datazone.birdlife.org/species/requestdis (accessed September 25, 2019).
Blanco, D. E., and López-Lanús, B. (2008). Non-breeding distribution and conservation of the Upland Sandpiper (Bartramia longicauda) in South America. Ornitol. Neotrop. 19, 613–621. Available online at: https://sora.unm.edu/node/133519
Bowen, B. S., and Kruse, A. D. (1993). Effects of grazing on nesting by Upland Sandpipers in southcentral North Dakota. J. Wildl. Manag. 57, 291–301. doi: 10.2307/3809426
Boyd, J. D., and Brightsmith, D. J. (2013). Error properties of Argos satellite telemetry locations using least squares and Kalman filtering. PLoS ONE 8:e63051. doi: 10.1371/journal.pone.0063051
Brlík, V., Koleček, J., Burgess, M., Hahn, S., Humple, D., Krist, M., et al. (2019). Weak effects of geolocators on small birds: a meta-analysis controlled for phylogeny and publication bias. J. Anim. Ecol. doi: 10.1111/1365-2656.12962
Brown, S., Gratto-Trevor, C., Porter, R., Weiser, E. L., Mizrahi, D., Bentzen, R., et al. (2017). Migratory connectivity of Semipalmated Sandpipers and implications for conservation. Condor 119, 207–224. doi: 10.1650/CONDOR-16-55.1
Buss, I. O. (1951). The Upland Plover in southwestern Yukon Territory. Arctic 4, 204–213. doi: 10.14430/arctic3945
Carneiro, C., Gunnarsson, T. G., and Alves, J. A. (2019). Why are Whimbrels not advancing their arrival dates into Iceland? Exploring seasonal and sex-specific variation in consistency of individual timing during the annual cycle. Front. Ecol. Evol. 7:248. doi: 10.3389/fevo.2019.00248
Casey, A. E., Sandercock, B. K., and Wisely, S. M. (2011). Genetic parentage and local population structure in the socially monogamous Upland Sandpiper. Condor 113, 119–128. doi: 10.1525/cond.2011.100100
Cohen, E. B., Hostetler, J. A., Hallworth, M. T., Rushing, C. S., Sillett, T. S., and Marra, P. P. (2018). Quantifying the strength of migratory connectivity. Methods Ecol. Evol. 9, 513–524. doi: 10.1111/2041-210X.12916
Conklin, J. R., Senner, N. R., Battley, P. F., and Piersma, T. (2017). Extreme migration and the individual quality spectrum. J. Avian Biol. 48, 19–36. doi: 10.1111/jav.01316
Douglas, D. C., Weinzierl, R., C., Davidson, S., Kays, R., Wikelski, M., et al. (2012). Moderating Argos location errors in animal tracking data. Methods Ecol. Evol. 3, 999–1007. doi: 10.1111/j.2041-210X.2012.00245.x
Finch, T., Butler, S. J., Franco, A. M., and Cresswell, W. (2017). Low migratory connectivity is common in long-distance migrant birds. J. Anim. Ecol. 86, 662–673. doi: 10.1111/1365-2656.12635
Foster, D. R., Motzkin, G., Bernardos, D., and Cardoza, J. (2002). Wildlife dynamics in the changing New England landscape. J. Biogeogr. 29, 1337–1357. doi: 10.1046/j.1365-2699.2002.00759.x
Fraser, K. C., Shave, A., Savage, A., Ritchie, A., Bell, K., Siegrist, J., et al. (2017). Determining fine-scale migratory connectivity and habitat selection for a migratory songbird by using new GPS technology. J. Avian Biol. 48, 339–345. doi: 10.1111/jav.01091
Fraser, K. C., Stutchbury, B. J. M., Silverio, C., Kramer, P. M., Barrow, J., Newstead, D., et al. (2012). Continent-wide tracking to determine migratory connectivity and tropical habitat associations of a declining aerial insectivore. Proc. R. Soc. Lond. B Biol. Sci. 279, 4901–4906. doi: 10.1098/rspb.2012.2207
Garber, S. D., Chevalier, S., and Cohen, J. R. (1997). Twenty-eight year study of Upland Sandpiper breeding population in New York. North Am. Bird Bander 22, 109–113.
Garvey, M. E., Nol, E., Howerter, D. W., and Armstrong, L. M. (2013). A spatial analysis of factors affecting nesting success of shorebirds in the Canadian Prairies. Condor 115, 58–66. doi: 10.1525/cond.2012.110146
Grosselet, M. O., Ruiz, G. J., and Zuniga, F. M. (2019). First migratory route of an Upland Sandpiper tracked with satellite transmitter during fall migration. Wader Study 126, 125–128. doi: 10.18194/ws.00154
Haverschmidt, F. (1966). The migration and wintering of the Upland Plover in Surinam. Wilson Bull. 78, 319–320.
Hedenström, A. (2008). Adaptations to migration in birds: behavioural strategies, morphology and scaling effects. Philos. Trans. R. Soc. B 363, 287–299. doi: 10.1098/rstb.2007.2140
Hill, J. M., and Renfrew, R. B. (2019a). Migratory patterns and connectivity of two North American grassland bird species. Ecol. Evol. 9, 680–692. doi: 10.1002/ece3.4795
Hill, J. M., and Renfrew, R. B. (2019b). Data from: migration patterns of Upland Sandpipers in the Western Hemisphere. Movebank Data Reposit. doi: 10.5441/001/1.3pt25757
Hofman, M. P. G., Hayward, M. W., Heim, M., Marchand, P., Rolandsen, C. M., Mattisson, J., et al. (2019). Right on track? Performance of satellite telemetry in terrestrial wildlife research. PLoS ONE 14:e0216223. doi: 10.1371/journal.pone.0216223
Hostetler, J. A., Sillett, T. S., and Marra, P. P. (2015). Full-annual-cycle population models for migratory birds. Auk 132, 433–449. doi: 10.1642/AUK-14-211.1
Houston, C. S. (1999). Decline in Upland Sandpiper populations: history and interpretations. Blue Jay 57, 136–142.
Houston, C. S., Drury, S. C., and Holroyd, G. (1999). Upland Sandpiper banding in North America. North Am. Bird Bander 24, 1–2.
Houston, C. S., Jackson, C., and Bowen, D. E. Jr. (2011). Upland Sandpiper (Bartramia longicauda), ver. 2. Ithaca, NY: Cornell Lab of Ornithology.
Iverson, G. C., Warnock, S. E., Butler, R. W., Bishop, M. A., and Warnock, N. (1996). Spring migration of Western Sandpipers along the Pacific Coast of North America: a telemetry study. Condor 98, 10–21. doi: 10.2307/1369502
Jahn, A. E., Bejarano, V., Cueto, V. R., Di Giacomo, A. S., and Fontana, C. S. (2017). Movement ecology research to advance conservation of South America's grassland migratory birds. Perspect. Ecol. Conserv. 15, 209–215. doi: 10.1016/j.pecon.2017.07.003
Jahn, A. E., Levey, D. J., Hostetler, J. A., and Mamani, A. M. (2010). Determinants of partial bird migration in the Amazon Basin. J. Anim. Ecol. 79, 983–992. doi: 10.1111/j.1365-2656.2010.01713.x
Johnson, A. S., Perz, J., Nol, E., and Senner, N. R. (2016). Dichotomous strategies? The migration of Whimbrels breeding in the eastern Canadian sub-Arctic. J. Field Ornithol. 87, 371–383. doi: 10.1111/jofo.12173
Johnson, O. W., Connors, P. G., and Pyle, P. (2018). American Golden-Plover Pluvialis dominica, ver. 3.0. Ithaca, NY: Cornell Lab of Ornithology. doi: 10.2173/bna.amgplo.03
Johnson, O. W., Fielding, L., Fox, J. W., Gold, R. S., Goodwill, R. H., and Johnson, P. M. (2011). Tracking the migrations of Pacific Golden-Plovers (Pluvialis fulva) between Hawaii and Alaska: new insight on flight performance, breeding ground destinations, and nesting from birds carrying light level geolocators. Wader Study Group Bull. 118, 26–31. Available online at: https://www.waderstudygroup.org/article/1755/
Kempenaers, B., and Valcu, M. (2017). Breeding site sampling across the Arctic by individual males of a polygynous shorebird. Nature 541, 528–531. doi: 10.1038/nature20813
Knight, S. M., Bradley, D. W., Clark, R. G., Gow, E. A., Belisle, M., Berzins, L. L., et al. (2018). Constructing and evaluating a continent-wide migratory songbird network across the annual cycle. Ecol. Monogr. 88, 445–460. doi: 10.1002/ecm.1298
Kranstauber, B., Kays, R., Lapoint, S. D., Wikelski, M., and Safi, K. (2012). A dynamic Brownian bridge movement model to estimate utilization distributions for heterogeneous animal movement. J. Anim. Ecol. 81, 738–746. doi: 10.1111/j.1365-2656.2012.01955.x
Kranstauber, B., Smolla, M., and Scharf, A. K. (2018). Move: Visualizing and Analyzing Animal Track Data. R package version 3.1.0. Available online at: https://CRAN.R-project.org/package=move (accessed March 15, 2019).
La Sorte, F. A., Fink, D., Hochachka, W. M., Farnsworth, A., Rodewald, A. D., Rosenberg, K. V., et al. (2014). The role of atmospheric conditions in the seasonal dynamics of North American migration flyways. J. Biogeogr. 41, 1685–1696. doi: 10.1111/jbi.12328
Lanctot, R. B., Yezerinac, S., Aldabe, J., Bosi De Almeida, J., Castresana, G., Brown, S., et al. (2016). Light-level geolocation reveals migration patterns of the Buff-breasted Sandpiper. Wader Study 123, 29–43. doi: 10.18194/ws.00032
Lisovski, S., Gosbell, K., Hassell, C., and Minton, C. (2016). Tracking the full annual-cycle of the Great Knot Calidris tenuirostris, a long-distance migratory shorebird of the east Asian-Australasian flyway. Wader Study 123, 177–189. doi: 10.18194/ws.00048
Loonstra, A. H. J., Verhoeven, M. A., Senner, N. R., Both, C., and Piersma, T. (2019). Adverse wind conditions during northward Sahara crossings increase the in-flight mortality of Black-tailed Godwits. Ecol. Lett. 22, 2060–2066. doi: 10.1111/ele.13387
Lopez, R., Malardé, J.-P., Royer, F., and Gaspar, P. (2013). Improving Argos Doppler location using multiple-model Kalman filtering. IEEE Trans. Geosci. Remote Sens. 52, 4744–4755. doi: 10.1109/TGRS.2013.2284293
Marra, P. P., Cohen, E. B., Loss, S. R., Rutter, J. E., and Tonra, C. M. (2015). A call for full annual cycle research in animal ecology. Biol. Lett. 11:20150552. doi: 10.1098/rsbl.2015.0552
McKinnon, E. A., and Love, O. P. (2018). Ten years tracking the migrations of small landbirds: lessons learned in the golden age of bio-logging. Auk 135, 834–856. doi: 10.1642/AUK-17-202.1
Medrano, F., Barros, R., Peredo, R., and De Groote, F. (2018). Extension of the northward migratory route of the Upland Sandpiper (Bartramia longicauda) to the western slope of the Andes. Wilson J. Ornithol. 130, 805–809. doi: 10.1676/17-075.1
Miller, V., Nol, E., Nguyen, L. P., and Turner, D. M. (2015). Habitat selection and nest success of the Upland Sandpiper (Bartramia longicauda) in Ivvavik National Park, Yukon, Canada. Can. Field Natural. 128, 341–349. doi: 10.22621/cfn.v128i4.1627
Minton, C., Gosbell, K., Johns, P., Christie, M., Klaassen, M., Hassell, C., et al. (2011). Geolocator studies on Ruddy Turnstones Arenaria interpres and Greater Sandplovers Charadrius leschenaultii in the East Asian-Australasia Flyway reveal widely different migration strategies. Wader Study Group Bull. 118, 87–96. Available online at: https://www.waderstudygroup.org/article/2046/
Mong, T. W., and Sandercock, B. K. (2007). Optimizing radio retention and minimizing radio impacts in a field study of Upland Sandpipers. J. Wildl. Manag. 71, 971–980. doi: 10.2193/2005-775
Morrison, C. A., Alves, J. A., Gunnarsson, T. G., Þórisson, B., and Gill, J.A. (2019). Why do earlier-arriving migratory birds have better breeding success? Ecol. Evol. 9, 8856–8864. doi: 10.1002/ece3.5441
Myers, J., Morrison, R., Antas, P., Harrington, B., Lovejoy, T., Sallaberry, M., et al. (1987). Conservation strategy for migratory species. Am. Sci. 75, 19–26. doi: 10.2307/3179457
Myers, J., Sallaberry, A., M., Ortiz, E., Castro, G., Gordon, L., Maron, J., et al. (1990). Migration routes of New World Sanderlings (Calidris alba). Auk 107, 172–180.
Newton, I. (2006). Can conditions experienced during migration limit the population levels of birds? J. Ornithol. 147, 146–166. doi: 10.1007/s10336-006-0058-4
Ng, J. W., Knight, E. C., Scarpignato, A. L., Harrison, A. L., Bayne, E. M., and Marra, P. P. (2018). First full annual cycle tracking of a declining aerial insectivorous bird, the Common Nighthawk (Chordeiles minor), identifies migration routes, nonbreeding habitat, and breeding site fidelity. Can. J. Zool. 96, 869–875. doi: 10.1139/cjz-2017-0098
Niles, L. J., Burger, J., Porter, R. R., Dey, A. D., Minton, C. D., González, P. M., et al. (2010). First results using light level geolocators to track Red Knots in the Western Hemisphere show rapid and long intercontinental flights and new details of migration pathways. Wader Study Group Bull. 117, 123–130. Available online at: https://www.waderstudygroup.org/article/2213/
Osborne, D. R., and Peterson, A. T. (1984). Decline of the Upland Sandpiper (Bartramia longicauda) in Ohio: an endangered species. Ohio J. Sci. 84, 8–10.
Page, G. W., Warnock, N., Tibbitts, T. L., Jorgensen, D., Hartman, C. A., and Stenzel, L. E. (2014). Annual migratory patterns of Long-billed Curlews in the American West. Condor 116, 50–61. doi: 10.1650/CONDOR-12-185-R2.1
Pearce-Higgins, J. W., Brown, D. J., Douglas, D. J. T., Alves, J. A., Bellio, M., Bocher, P., et al. (2017). A global threats overview for Numeniini populations: synthesising expert knowledge for a group of declining migratory birds. Bird Conserv. Int. 27, 6–34. doi: 10.1017/S0959270916000678
Penner, R., Andres, B., Lyons, J., and Young, E. (2015). Spring surveys (2011–2014) for American Golden-Plovers (Pluvialis dominica), Upland Sandpipers (Bartramia longicauda), and Buff-breasted Sandpipers (Calidris subruficollis) in the Flint Hills. Kansas Ornithol. Soc. Bull. 66, 37–52.
Pérez-Arteaga, A., Martínez-Chávez, C. C., and Salgado-Ortiz, J. (2019). Body condition of the Upland Sandpiper (Bartramia longicauda) en route through central Mexico. Ornitol. Neotrop. 30, 73–78. Available online at: http://journals.sfu.ca/ornneo/index.php/ornneo/article/view/419
Pierce, A. K., Dinsmore, S. J., Jorgensen, D., and Wunder, M. B. (2017). Migration routes and timing of Mountain Plovers revealed by geolocators. J. Field Ornithol. 88, 30–38. doi: 10.1111/jofo.12184
Piersma, T., and Gill, R. E. (1998). Guts don't fly: small digestive organs in obese Bar-Tailed Godwits. Auk 115, 196–203. doi: 10.2307/4089124
R Core Team (2018). R: A Language and Environment for Statistical Computing. Vienna: R Foundation for Statistical Computing. Available online at: http://www.r-project.org (accessed March 15, 2019).
Rakhimberdiev, E., Van Den Hout, P. J., Brugge, M., Spaans, B., and Piersma, T. (2015). Seasonal mortality and sequential density dependence in a migratory bird. J. Avian Biol. 46, 332–341. doi: 10.1111/jav.00701
Renfrew, R. B., Kim, D., Perlut, N., Smith, J., Fox, J., and Marra, P. P. (2013). Phenological matching across hemispheres in a long-distance migratory bird. Divers. Distrib. 19, 1008–1019. doi: 10.1111/ddi.12080
Rotics, S., Kaatz, M., Resheff, Y. S., Turjeman, S. F., Zurell, D., Sapir, N., et al. (2016). The challenges of the first migration: movement and behaviour of juvenile vs. adult White Storks with insights regarding juvenile mortality. J. Anim. Ecol. 85, 938–947. doi: 10.1111/1365-2656.12525
Ruegg, K. C., and Smith, T. B. (2002). Not as the crow flies: a historical explanation for circuitous migration in Swainson's Thrush (Catharus ustulatus). Proc. R. Soc. B Biol. Sci. 269, 1375–1381. doi: 10.1098/rspb.2002.2032
Ruthrauff, D. R., Tibbitts, T. L., and Gill, R. E. Jr. (2019). Flexible timing of annual movements across consistently used sites by Marbled Godwits breeding in Alaska. Auk Ornithol. Adv. 136, 1–11. doi: 10.1093/auk/uky007
Sandercock, B. K., Alfaro-Barrios, M., Casey, A. E., Johnson, T. N., Mong, T. W., Odom, K. J., et al. (2015). Effects of grazing and prescribed fire on resource selection and nest survival of Upland Sandpipers in an experimental landscape. Landsc. Ecol. 30, 325–337. doi: 10.1007/s10980-014-0133-9
Senner, N. R., Hochachka, W. M., Fox, J. W., and Afanasyev, V. (2014). An exception to the rule: carry-over effects do not accumulate in a long-distance migratory bird. PLoS ONE 9:e86588. doi: 10.1371/journal.pone.0086588
Sergio, F., Tanferna, A., Blas, J., Blanco, G., Hiraldo, F., and Bieber, C. (2018). Reliable methods for identifying animal deaths in GPS- and satellite-tracking data: review, testing, and calibration. J. Appl. Ecol. 56, 562–572. doi: 10.1111/1365-2664.13294
Smith, K. W., Trevis, B. E., and Reed, M. (2017). The effects of leg-loop harnesses and geolocators on the diurnal activity patterns of Green Sandpipers Tringa ochropus in winter. Ring. Migr. 32, 104–109. doi: 10.1080/03078698.2017.1437886
Strum, K. M., Hooper, M. J., Johnson, K. A., Lanctot, R. B., Zaccagnini, M. E., and Sandercock, B. K. (2010). Exposure of nonbreeding migratory shorebirds to cholinesterase-inhibiting contaminants in the Western Hemisphere. Condor 112, 15–28. doi: 10.1525/cond.2010.090026
Studds, C. E., Kendall, B. E., Murray, N. J., Wilson, H. B., Rogers, D. I., Clemens, R. S., et al. (2017). Rapid population decline in migratory shorebirds relying on Yellow Sea tidal mudflats as stopover sites. Nat. Commun. 8:14895. doi: 10.1038/ncomms14895
Stutchbury, B. J., Siddiqui, R., Applegate, K., Hvenegaard, G. T., Mammenga, P., Mickle, N., et al. (2016). Ecological causes and consequences of intratropical migration in temperate-breeding migratory birds. Am. Nat. 1, S28–S40. doi: 10.1086/687531
Tomkovich, P. S., Porter, R. R., Loktionov, E. Y., and Niles, L. J. (2013). Pathways and staging areas of Red Knots Calidris canutus rogersi breeding in southern Chukotka, Far Eastern Russia. Wader Study Group Bull. 120, 181–193. Available online at: https://www.waderstudygroup.org/article/1292/
van Bemmelen, R. S. A., Kolbeinsson, Y., Ramos, R., Gilg, O., Alves, J. A., Smith, M., et al. (2019). A migratory divide among Red-necked Phalaropes in the Western Palearctic reveals contrasting migration and wintering movement strategies. Front. Ecol. Evol. 7:86. doi: 10.3389/fevo.2019.00086
Vega, M. L., Willemoes, M., Thomson, R. L., Tolvanen, J., Rutila, J., Samas, P., et al. (2016). First-time migration in juvenile Common Cuckoos documented by satellite tracking. PLoS ONE 11:e0168940. doi: 10.1371/journal.pone.0168940
Vickery, P., Tubaro, P., Cardosa Da Silva, J., Peterjohn, B., Herkert, J., and Cavalcanti, R. (1999). Conservation of grassland birds in the Western Hemisphere. Stud. Avian Biol. 19, 2–26.
Vickery, P. D., Blanco, D. E., and López-Lanús, B. (2010). Conservation Plan for the Upland Sandpiper (Bartramia longicauda), ver. 1.1. Manomet, MA: Manomet Center for Conservation Sciences.
Vickery, P. D., Hunter, M. L. Jr., and Melvin, S. M. (1994). Effects of habitat area on the distribution of grassland birds in Maine. Conserv. Biol. 8, 1087–1097. doi: 10.1046/j.1523-1739.1994.08041087.x
Walter, W. D., and Fischer, J. W. (2018). Manual of Applied Spatial Ecology. State College, PA: Walter Applied Spatial Ecology Lab, Pennsylvania State University.
Warnock, N. (2010). Stopping vs. staging: the difference between a hop and a jump. J. Avian Biol. 41, 621–626. doi: 10.1111/j.1600-048X.2010.05155.x
Watts, B. D., Smith, F. M., Hamilton, D. J., Keyes, T., Paquet, J., Pirie-Dominix, L., et al. (2019). Seasonal variation in mortality rates for Whimbrels (Numenius phaeopus) using the Western Atlantic Flyway. Condor Ornithol. Appl. 121, 1–13. doi: 10.1093/condor/duy001
Weiser, E. L., Brown, S. C., Lanctot, R. B., Gates, H. R., Abraham, K. F., Bentzen, R. L., et al. (2018). Life-history tradeoffs revealed by seasonal declines in reproductive traits of Arctic-breeding shorebirds. J. Avian Biol. 49:jav-01531. doi: 10.1111/jav.01531
Weiser, E. L., Lanctot, R. B., Brown, S. C., Alves, J. A., Battley, P. F., Bentzen, R., et al. (2016). Effects of geolocators on hatching success, return rates, breeding movements, and change in body mass in 16 species of Arctic-breeding shorebirds. Mov. Ecol. 4:12. doi: 10.1186/s40462-016-0077-6
Weiser, E. L., Lanctot, R. B., Brown, S. C., Gates, H. R., Bentzen, R. L., Bêty, J., et al. (2017). Environmental and ecological conditions at arctic breeding sites have limited effects on true survival rates of adult shorebirds. Auk 135, 29–43. doi: 10.1642/AUK-17-107.1
White, R. P. (1988). Wintering grounds and migration patterns of the Upland Sandpiper. Am. Birds 42, 1247–1253.
Wikelski, M., and Kays, R. (2018). Movebank: Archive, Analysis and Sharing of Animal Movement Data. Max Planck Institute for Ornithology. Available online at: www.movebank.org (accessed May 20, 2019).
Wikelski, M., Kays, R. W., Kasdin, N. J., Thorup, K., Smith, J. A., and Swenson, G. W. Jr. (2007). Going wild: what a global small-animal tracking system could do for experimental biologists. J. Exp. Biol. 210, 181–186. doi: 10.1242/jeb.02629
Keywords: Bartramia longicauda, elliptical migration, full annual cycle, long-distance migration, seasonal, space use, transoceanic flight
Citation: Hill JM, Sandercock BK and Renfrew RB (2019) Migration Patterns of Upland Sandpipers in the Western Hemisphere. Front. Ecol. Evol. 7:426. doi: 10.3389/fevo.2019.00426
Received: 01 August 2019; Accepted: 21 October 2019;
Published: 19 November 2019.
Edited by:
Shannon J. McCauley, University of Toronto Mississauga, CanadaReviewed by:
Celina Baines, University of Toronto Mississauga, CanadaKevin C. Fraser, University of Manitoba, Canada
Copyright © 2019 Hill, Sandercock and Renfrew. This is an open-access article distributed under the terms of the Creative Commons Attribution License (CC BY). The use, distribution or reproduction in other forums is permitted, provided the original author(s) and the copyright owner(s) are credited and that the original publication in this journal is cited, in accordance with accepted academic practice. No use, distribution or reproduction is permitted which does not comply with these terms.
*Correspondence: Jason M. Hill, amhpbGxAdnRlY29zdHVkaWVzLm9yZw==