- 1J.F. Blumenbach Institute of Zoology and Anthropology, University of Göttingen, Göttingen, Germany
- 2Institute of Biology of Komi Scientific Centre of the Ural Branch of the Russian Academy of Sciences, Syktyvkar, Russia
- 3Department of Soil Sciences and Land Resources, Institut Pertanian Bogor, Bogor, Indonesia
- 4Centre of Biodiversity and Sustainable Land Use, Göttingen, Germany
Focusing on nematodes and their well-developed indices of community, ecosystem structure and function, we investigated the effects of the conversion of rainforest into rubber and oil palm plantations in Sumatra, Indonesia. Land use did not affect the total abundance of litter- and soil-dwelling nematodes, neither in riparian nor in well-drained sites. However, the rainforest nematode community differed from communities in plantations, with differences in litter being more pronounced compared to soil. In litter, fungivores and nematodes with short generation time (c-p2) increased in monoculture plantations, while that of bacterivores, herbivores, and nematodes with longer generation time and higher sensitivity to disturbances (c-p3) decreased. This indicates higher environmental pressure on nematodes in monoculture plantations than in rainforest. In soil of monoculture plantations, bacterivores, and c-p3 nematodes decreased while herbivores increased. This suggests that the damage of plants by nematodes in oil palm plantations exceeds that in rainforest. Overall, nematode functional diversity indices suggest that the stability of the decomposer community is higher in rainforest compared to monoculture plantations. Importantly, functional diversity indices were much more meaningful than nematode abundance. Further, changes with land use manifested more in litter than in soil, reflecting that nematode communities in soil are buffered against changes in land use and associated environmental conditions. Therefore, to fully assess changes in the structure and functioning of decomposer systems with changes in land use, the litter layer, which often receives little attention, requires more careful consideration.
Introduction
The conversion of lowland rainforest into oil palm and rubber monoculture plantations in Sumatra started in 1911 and has been intensified with the transmigration program implemented by the Indonesian government in the late 1970s (Fitzherbert et al., 2008; Laumonier et al., 2010). Such agricultural expansion and land-use intensification provoke losses in biodiversity and pose challenges for ecosystem functioning (Hooper et al., 2012; Newbold et al., 2015). For Sumatra, it has been shown that the conversion of rainforest into monoculture plantations results in losses in plant and animal diversity (Clough et al., 2016; Drescher et al., 2016), and alters the composition of soil microorganisms (Schneider et al., 2015; Krashevska et al., 2016; Berkelmann et al., 2018). Due to biodiversity loss, the functioning of the system and its stability may be at risk (Barnes et al., 2014; Clough et al., 2016; Drescher et al., 2016). However, changes in biodiversity and ecosystem functioning related to the conversion of tropical lowland forests into agricultural production systems have been little studied. Especially periodically flooded riparian areas with reduced agricultural output, but presumably high conservation value, received little attention (Naiman et al., 1993; Paoletti et al., 2018).
In general, plantations have been found to be colonized by less than half the number of animal species present in primary or secondary rainforests (Fitzherbert et al., 2008), but the decline in the number of species with the conversion of rainforest into plantations varies among the organisms studied (Clough et al., 2016). Soil organisms that colonize plantations comprise functionally diverse communities that at least in part are adapted to changes in land use. However, there is the risk of losing less adapted but functionally important species, which may result in a decrease in the functionality and stability of decomposer systems of plantations. For example, the balanced abundance of groups of high and low trophic levels of testate amoebae in rainforests has shifted toward the dominance of low trophic level communities in plantations (Krashevska et al., 2016). In addition, it has been shown that invertebrate predators are strongly influenced by changes in land use, as evidenced by the loss of species, as well as biomass and energy flux (Barnes et al., 2014; Potapov et al., 2019). These changes are also associated with shifts in the prey spectrum of predators (Klarner et al., 2017). Thus, for understanding the functioning of converted ecosystems knowledge on both changes in biodiversity with land-use change as well as changes in the functional role of microbial and animal communities is needed. Yet, gaining this knowledge is hampered by limited information on the functional role of many animal taxa in soil.
The belowground system harbors an immense diversity of prokaryotes and eukaryotes, providing a range of ecosystem services (Wall and Six, 2015). One of the dominant and functionally important groups are nematodes, which colonize virtually any soil in large numbers. Nematodes significantly contribute to nutrient cycling by grazing on soil microorganisms, thereby releasing nutrients and stimulating plant growth (Ferris, 2010a). In addition, it is known that nematodes are sensitive to changes in agricultural practices and cropping systems, and therefore are used widely as indicators of soil health (Ferris and Tuomisto, 2015). For nematodes, various indices have been developed to judge the state and extent of ecosystem disturbance (Ferris and Bongers, 2009; Ferris, 2010b). However, little is known on the response of nematodes to the conversion of rainforest into agricultural production systems, such as oil palm or rubber plantations (Xiao et al., 2014; Carron et al., 2015). This is unfortunate as nematode communities can provide important information on how land-use changes affect the functioning of converted ecosystems (Bongers and Bongers, 1998). Since nematodes comprise a wide range of trophic groups, such studies, for example, can provide information on the distribution of economically important plant-parasitic species (Nicol et al., 2011). Further, changes in the proportion of bacterial and fungal feeding nematodes, which differ in metabolic rate and life cycles, provide insight into changes in energy flux and nutrient cycling due to conversion of rainforest (De Ruiter et al., 1993; Ferris et al., 2004). In addition, it is known that predatory and omnivorous nematodes are more sensitive to disturbance than, for example, bacterivores, which typically recover faster from perturbations (Wang and Hooks, 2011). This may provide information on other ecosystem functions, such as top-down control of e.g., plant-parasitic nematodes and, therefore, on plant health (Khan and Kim, 2007). Furthermore, based on functional indices it is possible to predict structural characteristics as well as the channeling of energy through food webs (Bongers, 1990; Ferris and Bongers, 2009; Ferris, 2010b).
This study aims at inspecting how changes in land use of tropical lowland ecosystems affect belowground animal communities and the functioning of these ecosystems. Using soil nematodes as model group, we investigated changes in nematode abundance, community composition and functional diversity with the conversion of rainforest into major agricultural production systems, such as rubber and oil palm plantations in Sumatra, Indonesia, in a straightforward experimental design. To study changes in functional diversity we used the following indices: (1) trophic groups of nematodes, i.e., bacterivores, fungivores, herbivores, omnivores, and predators (Yeates et al., 1993), and (2) life strategies assigning all taxa to one of five classes of colonizer-persisters depending on their r- and K-characteristics (Bongers and Bongers, 1998; Ferris, 2010a). Further, faunal profiles were studied to identify nematode successional trends, i.e., whether nematode communities are basal, enriched or structured and stable (Ferris and Bongers, 2009). In earlier studies, we found that belowground taxa in litter and soil respond differently to changes in land use in tropical lowland ecosystems (Krashevska et al., 2015, 2016). Therefore, in this study, we analyzed litter and soil nematodes separately. We included well-drained and riparian areas to cover the heterogeneity of our study sites. As riparian sites are often characterized by high plant and animal diversity, whereas agricultural production is compromised, these habitats may serve as targets for conservation efforts (Maisonneuve and Rioux, 2001; Young-Mathews et al., 2010; Paoletti et al., 2018). Also, we included environmental variables related to land-use change, such as the amount of litter, carbon-to-nitrogen ratio, pH, water content, bacterial and fungal markers to improve understanding of driving forces of changes in nematode communities with land-use change. We hypothesized that (1) changes in land use detrimentally affect soil nematode abundance, with the effect being more pronounced in riparian compared to well-drained sites; (2) land-use change alters nematode community composition, with the changes being most pronounced in riparian compared to well-drained sites; (3) the impact of land-use change varies among trophic groups of nematodes being most pronounced in omnivores and predators; (4) the conversion of rainforest into plantations is associated with an increase in the number of nematodes with short generation time and high resistance to harsh environmental conditions. Specifically, we expected the conversion of rainforest into plantations to be associated with a decrease in system stability, and this being reflected in nematode faunal profiles. In general, we expected that the impact of land-use changes will be more pronounced in litter than in soil, regardless of whether the number of nematodes, the composition of communities or parameters of the functional diversity of nematodes is used.
Materials and Methods
Study Sites and Sampling
The sampling sites were located in the tropical lowlands of Jambi Province in Sumatra, Indonesia. Two landscapes were studied, Bukit Duabelas (2° 0′ 57″ S, 102° 45′ 12″ E) and Harapan (1° 55′ 40″ S, 103° 15′ 33″ E). At each landscape secondary lowland rainforest and two typical land-use systems, i.e., rubber plantations and oil palm plantations, were selected. Each land-use system was replicated four times in each landscape resulting in 24 plots (2 landscape × 3 land-use types × 4 replicates). In addition, in the Harapan landscape riparian sites of each of the three land-use systems were investigated with four replicates each resulting in a total of 36 sites. The non-riparian (well-drained) sites were on well-drained soils, whereas the riparian sites were flooded during the rainy season approximately from October until February. The plots were 50 × 50 m. More details on the design of the study are given in Drescher et al. (2016) and Paoletti et al. (2018).
Litter (L/F horizon) and upper mineral soil samples (Ah horizon, to a depth of 50 mm) were taken in October/November 2016 using a corer of a diameter of 50 mm. In order to account for small scale spatial variation five cores were taken from a subplot of 5 × 5 m randomly placed in the plot; the samples were pooled and homogenized. From litter samples seeds, twigs, roots and coarse woody debris were removed by hand. From soil samples, roots and stones (>5 mm) were removed by consecutive sieving through 10 and 5 mm mesh.
Nematodes were extracted from subsamples of 10 g litter and 25 g soil using modified Baermann funnels (Ruess, 1995). After extracting for 48 h, the nematodes were transferred into 50 mL Falcon tubes and fixed in 4% hot formalin. From each sample, at least 100 individuals were identified, if possible to genus level, using a Leica DM4000 B inverted microscope (Table 1). In three cases it was not possible to assign nematodes to any genus, therefore, they were assigned to Cephalobidae comb., Tylenchidae comb., and Rhabditidae comb. If the total number of nematodes in the sample was <100, all nematodes were identified. For identification, the keys of Jairajpuri and Ahmad (1992), Bongers (1994) and Brzeski (1998) were used. The abundance of nematodes was calculated as individuals per 100 g dry weight of litter, respectively soil. Nematodes were assigned to five trophic groups (bacterivores, fungivores, herbivores, omnivores, predators) according to Yeates et al. (1993) (see Table 1). Filenchus was ascribed to fungivores (Okada and Kadota, 2003; Christensen et al., 2007; Buchan et al., 2013); the other genera of Tylenchidae were considered herbivores. Individuals that could not be assigned to any trophic group were grouped as unidentified. The calculation of ecological indices followed Ferris and Bongers (2009). The genera of nematodes were categorized into colonizer-persister classes (Table 1), ranging from c-p1 to c-p5, for extreme r- to extreme K-strategists (Bongers, 1990; Ferris, 2010a, http://nemaplex.ucdavis.edu). Further ecological indices were calculated, including the Structure Index (SI), reflecting the connectedness of the nematode community, the Enrichment Index (EI), reflecting soil enrichment. The faunal profile was analyzed (based on EI and SI) for reflecting the nematode successional trends (for details see Ferris and Bongers, 2009; Ferris, 2010b, http://nemaplex.ucdavis.edu; see Supplementary Tables 1, 2). Only indices significantly varying between land-use systems or between well-drained and riparian sites are shown in the result section; for mean values of indices, SD and F-values (see Tables 2, 3).
Data Analysis
Variations in nematode abundance and indices with land use, and between riparian and well-drained sites, were analyzed using linear models (lm) with the explanatory variables land-use system (rainforest = F, oil palm plantations = O, rubber plantations = R) and riparian sites (well-drained and riparian; Harapan landscape only), and landscape included as block. All analyses were done using R software version 3.5.1 (R Core Team, 2018). Data handling and transformation was done with the packages vegan (Oksanen et al., 2017) and car (Fox et al., 2012). Graphics were plotted in R using the package ggplot2 (Wickham, 2009). Prior to the analyses, relative abundances of nematode groups, expressed as percentages of total per plot, were arcsine-square root transformed. Normality and homoscedasticity of model residuals were tested using Shapiro–Wilk and Bartlett tests; if significant, data were log-transformed. When assumptions were not met after transformation, effects of land-use system and riparian sites were assessed using non-parametric tests (Kruskal–Wallis rank sum test for effects of land-use system and Wilcoxon test for differences between riparian and well-drained sites). Differences between pairs of land-use systems were inspected by pairwise test for multiple comparisons of mean rank sums (function post hoc.kruskal.nemenyi.test of the PMCMR package; Pohlert, 2014). Nematode community composition in litter and soil (based on genera) was analyzed by discriminant function analysis (DFA). The community composition of litter nematodes differed from that in soil (Wilks Lambda = 0.42; F2,64 = 43.2***). Therefore, the litter and soil layer were analyzed separately. DFA was carried out using STATISTICA 13.0. Squared Mahalanobis distances (MD2) between group centroids (rainforest, oil palm and rubber plantations) and the reliability of sample classification were determined. All means, SD, F- and p-values for the effect of land-use system, and for differences between riparian and well-drained sites are shown in Tables 2, 3 for litter and soil, respectively. Levels of significance in text and figures are indicated as not significant (ns) (p > 0.05), * (p < 0.05), ** (p < 0.01), and *** (p < 0.001). Principal components analysis (PCA) was used to analyse the response of trophic groups and environmental variables at the plot level. The following environmental variables were included in the PCA: amount of litter (g per cm2), carbon-to-nitrogen ratio, pH value, water content, Gram-positive and Gram-negative bacterial phospholipid fatty acids (PLFAs), fungal PLFA (18:2ω6,9), relative marker of arbuscular mycorrhizal fungal neutral lipid (16:1ω5) (Frostegård et al., 2011; Ngosong et al., 2012; Krashevska, unpublished data; see Supplementary Table 3). PCA was carried out in CANOCO 5.04 (Ter Braak and Šmilauer, 2012). The graphic representation of faunal profiles was based on Ferris and Bongers (2009).
Results
Variations in Nematode Abundance and Community Composition With Land-Use Changes, and Between Riparian and Well-Drained Sites
In litter and in soil, the total abundance of nematodes differed neither between land-use systems nor riparian and well-drained sites (Tables 2, 3). However, the community composition of nematodes in litter varied with land-use change (Wilks Lambda = 0.23, F2,60 = 8.35***). The community composition in rainforest differed from that in rubber (MD2 = 14.5, F4,30 = 19.8***) and oil palm plantations (MD2 = 5.4, F4,30 = 7.42***); further, the community composition in rubber plantations differed from that in oil palm plantations (MD2 = 14.5, F4,30 = 3.8*). By contrast, the community composition in riparian sites was similar to that in well-drained sites (F4,20 = 0.34ns). Similar to litter, the community composition of nematodes in soil varied with land-use change, although differences were only marginally significant (Wilks Lambda = 0.57, F8,50 = 2.04, p = 0.06). As indicated by Mahalanobis distances, the nematode community in rainforest differed from that in rubber plantations (MD2 = 3.00, F4,25 = 3.30*). The community composition of nematodes in soil of riparian sites was similar to that of well-drained sites (F4,26 = 1.17ns).
Indices of the Functional Diversity of Nematodes
In general, in litter 46.8% of total nematodes comprised fungivores, followed by 35.3% bacterivores, 7.53% herbivores, 5.12% unidentified, 4.99% omnivores, and only 0.26% predators. The relative abundance of fungivores was lower in rainforest and higher in rubber and oil palm plantations (Figure 1; Table 2). By contrast, the relative abundance of bacterivores and herbivores was higher in rainforest and lower in oil palm and rubber plantations. The relative abundance of predators, omnivores and unidentified was similar in each of the land-use systems (Table 2).
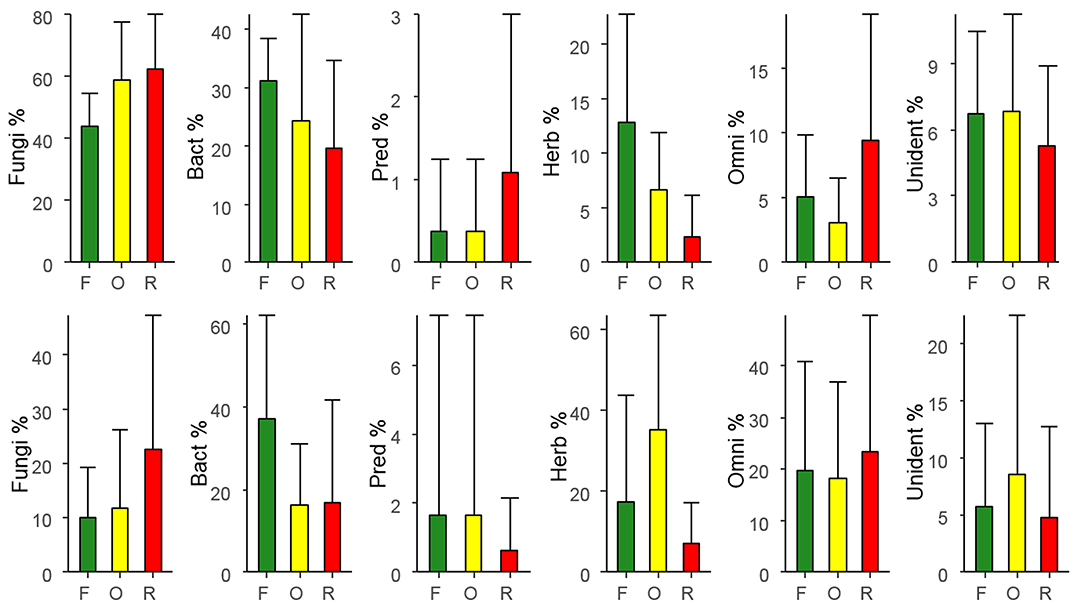
Figure 1. Variations in relative abundance of trophic groups of nematodes (percentages of total) with land use in litter (top row) and in soil (bottom row), means ± SD; F, Rainforest; O, oil palm plantation; R, rubber plantation; Fungi, fungivores; Bact, bacterivores; Pred, predators; Herb, herbivores; Omni, omnivores; Unident, unidentified.
In general, in soil 31.2% of the total community was represented by herbivores, followed by bacterivores (26.7%), omnivores (20.0%), fungivores (15.5%), unidentified (5.77%), and predators with only 0.77%. In soil, the relative abundance of bacterivores was higher in rainforest and lower in rubber and oil palm plantations (Figure 1; Table 3). By contrast, the relative abundance of herbivores was high in oil palm plantations and lower in rainforest and rubber plantations. The relative abundance of fungivores, predators, omnivores and unidentified was similar in all land-use systems (Table 3).
The ordinations underline that nematode trophic groups differed between land-use systems (Figures 2A,B). In litter, axis 1 of the PCA of trophic groups separated oil palm plantations from rainforest, whereas axis 2 separated rubber plantations from rainforest and oil palm plantations (Figure 2A). High amounts of litter in rainforest were associated with herbivorous nematodes, whereas bacterivorous nematodes were associated with Gram-positive PLFA markers, both being high in rainforest and oil palm plantations. Fungivorous nematodes were associated with high amounts of fungal PLFA and AMF NLFA markers in rubber plantations. The position of omnivorous and predatory nematodes reflects that their abundance positively correlated with high water content and high litter C-to-N ratio.
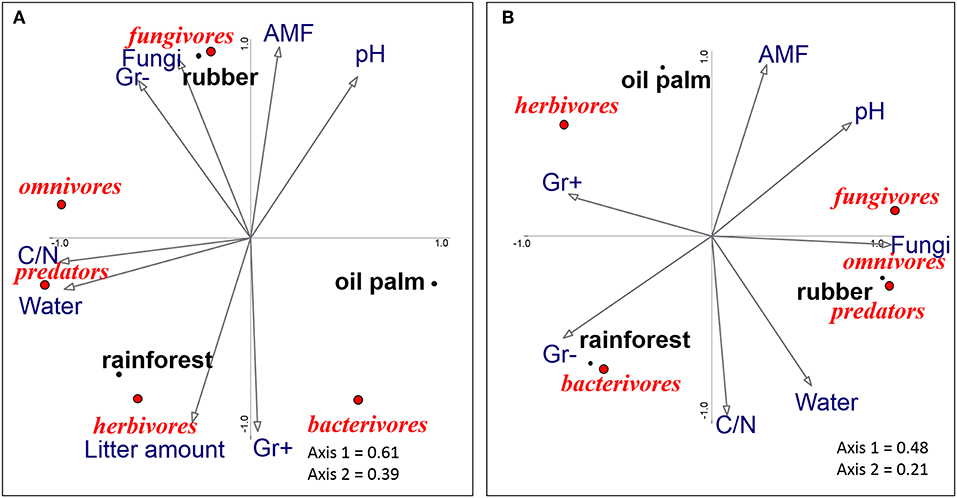
Figure 2. Principal components analysis of nematode trophic groups (fungivores, omnivores, predators, herbivores, and bacterivores) in litter (A) and soil (B) as related to environmental variables (arrows) of three land-use systems (rainforest, rubber, and oil palm plantations). The position of trophic groups is represented by centroids; n = 12. C/N, carbon-to-nitrogen ratio, pH, pH-value; Water, water content; Gr+ and G–, Gram-positive and Gram-negative bacterial phospholipid fatty acids (PLFAs); Fungi, fungal PLFA (18:2ω6,9); AMF, relative marker of arbuscular mycorrhizal fungal neutral lipid (NLFA, 16:1ω5); for the environmental data, see Supplementary Table 3.
In soil, axis 1 of the PCA separated rainforest from rubber plantations and axis 2 separated oil palm plantations from rainforest and rubber plantations (Figure 2B). Oil palm plantations were associated with herbivorous nematodes, whereas rubber plantations were associated with a high amount of the fungal phospholipid fatty acid marker and fungivorous nematodes. Furthermore, the position of omnivorous, predatory and fungivorous nematodes reflects that their abundances are positively correlated. Bacterivorous nematodes were associated with rainforest and with high amounts of Gram-negative bacterial PLFA markers.
In general, in litter the most abundant nematode class was c-p2 (72.0%), followed by c-p1 (17.4%), c-p4 (6.77%), c-p3 (3.48%), and c-p5 (0.33%). In litter, only c-p2 and c-p3 class nematodes varied with land-use system (Table 2). The relative abundance of c-p2 class nematodes was low in rainforest, higher in oil palm plantations and highest in rubber plantations. By contrast, the relative abundance of c-p3 class nematodes was low in rubber plantations, higher in oil palm plantations and highest in rainforest.
In soil, the most abundant nematode class was c-p2 (59.4%), followed by c-p4 (28.3%), c-p3 (8.64%), c-p1 (2.47%), and c-p5 (1.22%). Only c-p3 class nematodes varied significantly with land-use system (Table 3). The relative abundance of c-p3 class nematodes decreased from rainforest to oil palm plantations to rubber plantations.
In litter, the nematode faunal profile placed nematodes from rainforest in Quadrat C (resource-limited and structured) and B (enriched and structured), whereas those from oil palm and rubber plantations in Quadrat A (enriched but unstructured) with the exception of rubber plantations of the Bukit Duabelas landscape (Figure 3A). In soil, the nematode faunal profile placed nematodes from all land-use systems in Quadrat C (resource-limited and structured; Figure 3B).
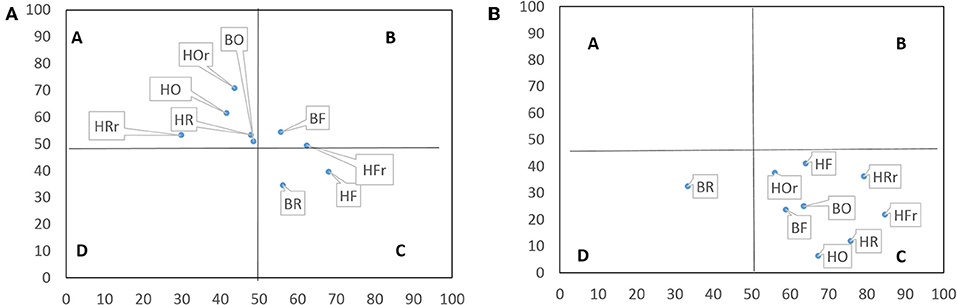
Figure 3. Nematode faunal profile in litter (A) and soil (B). The vertical axis represents enrichment and the horizontal axis structure. Quadrats refer to the faunal profile A, B, C, and D (Ferris and Bongers, 2009); F, rainforest; R, rubber; O, oil palm plantation; r, riparian; H, Harapan; B, Bukit Duabelas, n = 4.
Discussion
Nematode Abundance and Community Composition
In contrast to our first hypothesis, the abundance of nematodes did not vary significantly with land-use systems, or between riparian and well-drained sites. This is in line with the study of Young-Mathews et al. (2010), which also found no differences in nematode abundance between major land-use systems in the Mediterranean region. Further, it is consistent with the lack of land-use effect on the abundance of protists studied at our field sites (Schulz et al., 2019). Thus, nematode abundance based on units dry weight of litter and soil should not be considered as a valuable indicator of land-use change (Yeates and Bongers, 1999).
In our study, the abundance of nematodes, with the total average values in litter of 5,459 and in soil of 99 individuals per 100 g of dry matter was generally low. These low numbers are in line with the recent global-scale study by van den Hoogen et al. (2019), documenting overall low abundance of nematodes in tropical regions compared to high latitude regions with high soil organic carbon stocks, supporting high food availability due to high microbial biomass. However, the low abundance of nematodes in the different land-use systems in our study are likely to be due to different mechanisms. In rainforest, the low nematode abundance likely is related to fast litter decomposition (Krashevska et al., 2018), whereas in monoculture plantations it rather is due to low residue input and high C loss due to erosion (Guillaume et al., 2015). Since nematodes comprise very different functional groups contributing differently to total nematode abundance, changes in nematode community composition need to be considered for understanding their response to land-use changes.
The community composition of nematodes in litter of rainforest differed significantly from that in rubber and oil palm plantations, supporting our second hypothesis. However, differences were less pronounced in soil suggesting that litter nematode communities are more sensitive to changes in land use than those in soil. This is in line with recent studies showing that the response of prokaryotic and eukaryotic microorganisms to changes in land use in litter exceed that in soil (Krashevska et al., 2015, 2016). These results highlight that to fully understand consequences of land-use change for soil biota the litter layer needs closer attention.
Similar to the abundance of nematodes, the community composition of nematodes did not significantly vary between well-drained and riparian sites, neither in litter nor in soil indicating that nematodes are insensitive to prolonged inundation. However, further studies considering seasonal dynamics are needed to further investigate the sensitivity of nematodes to flooding in tropical ecosystems.
Nematode Functional Diversity
In contrast to our third hypothesis, omnivorous and predatory nematodes did not respond to changes in land use suggesting that they are rather insensitive to changes in land use contrasting results of previous studies (Wang and Hooks, 2011). However, fungivores, bacterivores and herbivores responded to changes in land use. In litter, the relative abundance of fungivores increased from rainforest to monoculture plantations. The higher abundance of fungivores in plantations correlated with higher concentrations of markers of saprotrophic fungi and AMF in the litter layer suggesting that the higher abundance is based on higher food availability in plantations. We did not find earlier reports on changes in fungivore nematodes in litter due to changes in land use. However, contrasting the pattern in litter, fungivore nematodes in soil have been shown to decrease with the conversion of forests into agricultural systems, presumably due to fertilizer application (Ettema et al., 1998, 1999; Neher et al., 2005). While fungivore nematodes in litter increased with the conversion of rainforest into plantations, herbivore and bacterivore nematodes decreased. The decrease in herbivore nematodes from rainforest to plantations was related to the decrease in the thickness of the organic layer as indicated by principal components analysis. The decrease in bacterivore nematodes with the conversion of rainforest into plantations may have been due to the reduction of certain bacterial groups, especially in rubber plantations. Rubber plants produce salicylic acid to control microbial pathogens (Kästner et al., 2014; Deenamo et al., 2018) and this may reduce certain prey species of bacterivores. However, in general, the abundance and diversity of bacteria benefitted from the conversion of rainforest into plantations, presumably due to fertilizer application and liming (Ettema et al., 1999; Schneider et al., 2015). The decline in bacterivore nematodes, therefore, is difficult to explain, however nematode—bacteria interactions are known to be complex (Murfin et al., 2012), and high relative abundance of bacterivore nematodes in rainforest may also be due to favorable abiotic conditions for reproduction rather than the availability of bacterial prey.
In soil, only the abundance of bacterivore and herbivore nematodes changed with the conversion of rainforest into plantations. As in litter, the decrease in bacterivore nematodes in soil of plantations was unexpected, as bacteria usually benefited from converting rainforest into plantations (Schneider et al., 2015; see above). Notably, in oil palm plantations bacterivore nematodes have been found to be abundant suggesting that they may also benefit from fertilizer application (Carron et al., 2015). In contrast to litter, herbivore nematodes were the dominant trophic group in soil of oil palm plantations presumably reflecting increased feeding on roots. Indeed, host species identity plays a major role in determining the density of herbivore nematodes in soil (De Deyn et al., 2004). Higher relative abundance of herbivores in oil palm than in rubber plantations indicates high sensitivity of oil palms to nematode infections; low relative abundance of herbivore nematodes in rubber plantations might be due to defense compounds in rubber or to mycorrhizal fungi suppressing nematode infection and reproduction (de la Peña et al., 2006). Overall, the data suggest that resource availability and/or environmental conditions in soil of oil palm plantations favor herbivore nematodes. Further, the results reflect the typically higher plant damage caused by nematodes in monoculture plantations compared to systems rich in plant species, such as rainforest (van der Putten et al., 2006).
Generally, ca. 90% of all nematodes in litter were represented by taxa with short generation time and high reproduction rates (c-p1 and c-p2), suggesting that litter species are exposed to harsher environmental conditions compared to species in soil. Supporting this conclusion, in soil nematodes with long generation time and low resistance to desiccation (e.g., c-p3 and c-p4) became abundant (Bongers, 1990; Ferris, 2010b). However, only c-p2 and c-p3 nematodes significantly responded to changes in land use and the response was more pronounced in litter than in soil. Despite the fact that only two colonizer-persister classes were impacted by land use, the findings support our fourth hypothesis. In litter, opportunistic c-p2 nematodes with short generation time and relatively high reproduction rates increased with the conversion of rainforest into plantations, whereas persister c-p3 nematodes with longer generation time and higher sensitivity to disturbances decreased, indicating more stressful environmental conditions in plantations (Bongers and Ferris, 1999). Similar to the litter, in soil c-p3 group nematodes decreased with the conversion of rainforest into plantations, again reflecting increased environmental harshness in plantations (Bongers, 1990; Bongers and Ferris, 1999). In litter, the nematode profile indicated that the communities in oil palm and rubber plantations are enriched but unstructured, whereas in rainforest they are resource-limited and structured or enriched and structured. By contrast, in soil the nematode profile indicated that the nematode communities of each of the studied land-use systems are resource-limited and structured.
Overall, changes in nematode community composition and functional diversity indices with changes in land use were much more informative than data on nematode abundance per unit litter or soil, which were similar in each of the studied land-use systems. Functional diversity indices of nematodes indicated that the decomposer community in rainforest is more stable compared to that in monocultures. Further, higher abundance of herbivore nematodes in oil palm plantations likely reflects the increased susceptibility of monoculture plantations to root-feeding nematode infections. The results highlight that plantation management practices should target to increase the stability and herbivore pest resistance of monoculture plantations in particular in oil palm. This might be achieved by both reducing fertilizer and pesticide application (Darras et al., 2019) and by increasing structural complexity in plantations (Zemp et al., 2019). Notably, changes in land use are manifested more intensively in litter than in soil, indicating that nematode communities in soil are protected from changes in land use and associated environmental changes. This highlights that to fully appreciate changes in the structure and functioning of decomposer systems with changes in land use, the litter layer, which often receives little attention, needs more close consideration.
Data Availability Statement
All datasets generated for this study are included in the article/Supplementary Material.
Author Contributions
VK and SS conceived and designed the study. Soil sampling and nematode extraction performed by VK. VK and AK analyzed the samples. VK prepared and analyzed the data. SS, VK, and RW contributed the reagents, material, and analysis tools. RW contributed the field logistical support. VK wrote the first draft of the manuscript. All authors contributed to editing the paper.
Funding
This study was financed by the Deutsche Forschungsgemeinschaft (DFG) in the framework of the collaborative German—Indonesian research project CRC990.
Conflict of Interest
The authors declare that the research was conducted in the absence of any commercial or financial relationships that could be construed as a potential conflict of interest.
Acknowledgments
We thank the following persons and organizations for granting us access to and use of their properties: village leaders, local plot owners, PT Humusindo, PT REKI, PT Perkebunan Nusantara VI, Bukit Duabelas National Park. We thank the many colleagues and helpers for their support in the field and assistance in the laboratory. We acknowledge support by the German Research Foundation and the Open Access Publication Funds of the Göttingen University.
Supplementary Material
The Supplementary Material for this article can be found online at: https://www.frontiersin.org/articles/10.3389/fevo.2019.00487/full#supplementary-material
References
Barnes, A. D., Jochum, M., Mumme, S., Haneda, N. F., Farajallah, A., Widarto, T. H., et al. (2014). Consequences of tropical land use for multitrophic biodiversity and ecosystem functioning. Nat. Commun. 5:5351. doi: 10.1038/ncomms6351
Berkelmann, D., Schneider, D., Engelhaupt, M., Heinemann, M., Christel, S., Wijayanti, M., et al. (2018). How rainforest conversion to agricultural systems in sumatra (Indonesia) affects active soil bacterial communities. Front. Microbiol. 9, 1–13. doi: 10.3389/fmicb.2018.02381
Bongers, T. (1990). The maturity index: an ecological measure of environmental disturbance based on nematode species composition. Oecologia 83, 14–19. doi: 10.1007/BF00324627
Bongers, T. (1994). De Nematoden van Nederland. Een Identificatietabel voor de in Nederland Aangetroffenen Zoetwater- en Bodembewonende Nematoden. Utrecht : Koninklijke Nederlandse Natuurhistorische Vereniging, John Wiley & Sons, Ltd
Bongers, T., and Bongers, M. (1998). Functional diversity of nematodes. Appl. Soil Ecol. 10, 239–251. doi: 10.1016/S0929-1393(98)00123-1
Bongers, T., and Ferris, H. (1999). Nematode community structure as a bioindicator in environmental monitoring. Trends Ecol. Evol. 14, 224–228. doi: 10.1016/S0169-5347(98)01583-3
Brzeski, M. W. (1998). Nematodes of Tylenchina in Poland and Temperate Europe. Warszawa: Muzeum i Instytutu Zoologii, Polska Akademia Nauk (MiIZ PAN).
Buchan, D., Gebremikael, M. T., Ameloot, N., Sleutel, S., and De Neve, S. (2013). The effect of free-living nematodes on nitrogen mineralisation in undisturbed and disturbed soil cores. Soil Biol. Biochem. 60, 142–155. doi: 10.1016/j.soilbio.2013.01.022
Carron, M. P., Auriac, Q., Snoeck, D., Villenave, C., Blanchart, E., Ribeyre, F., et al. (2015). Spatial heterogeneity of soil quality around mature oil palms receiving mineral fertilization. Eur. J. Soil Biol. 66, 24–31. doi: 10.1016/j.ejsobi.2014.11.005
Christensen, S., Alphei, J., Vestergård, M., and Vestergaard, P. (2007). Nematode migration and nutrient diffusion between vetch and barley material in soil. Soil Biol. Biochem. 39, 1410–1417. doi: 10.1016/j.soilbio.2006.11.026
Clough, Y., Krishna, V. V., Corre, M. D., Darras, K., Denmead, L. H., Meijide, A., et al. (2016). Land-use choices follow profitability at the expense of ecological functions in Indonesian smallholder landscapes. Nat. Commun. 7:13137. doi: 10.1038/ncomms13137
Darras, K., Corre, M., Formaglio, G., Tjoa, A., Potapov, A., Brambach, F., et al. (2019). Reducing fertilizer and avoiding herbicides in oil palm plantations—ecological and economic valuations. Front. For. Glob. Chang. 2:65. doi: 10.3389/ffgc.2019.00065
De Deyn, G. B., Raaijmakers, C. E., van Ruijven, J., Berendse, F., and van der Putten, W. H. (2004). Plant species identity and diversity effects on different trophic levels of nematodes in the soil food web. Oikos 106, 576–586. doi: 10.1111/j.0030-1299.2004.13265.x
de la Peña, E., Echeverría, S. R., van der Putten, W. H., Freitas, H., and Moens, M. (2006). Mechanism of control of root-feeding nematodes by mycorrhizal fungi in the dune grass Ammophila arenaria. New Phytol. 169, 829–840. doi: 10.1111/j.1469-8137.2005.01602.x
De Ruiter, P. C., Van Veen, J. A., Moore, J. C., Brussaard, L., and Hunt, H. W. (1993). Calculation of nitrogen mineralization in soil food webs. Plant Soil 157, 263–273. doi: 10.1007/BF00011055
Deenamo, N., Kuyyogsuy, A., Khompatara, K., Chanwun, T., Ekchaweng, K., and Churngchow, N. (2018). Salicylic acid induces resistance in rubber tree against Phytophthora palmivora. Int. J. Mol. Sci. 19:1883. doi: 10.3390/ijms19071883
Drescher, J., Rembold, K., Allen, K., Beckschäfer, P., Buchori, D., Clough, Y., et al. (2016). Ecological and socio-economic functions across tropical land use systems after rainforest conversion. Philos. Trans. R. Soc. B Biol. Sci. doi: 10.1098/rstb.2015.0275
Ettema, C. H., Coleman, D. C., Vellidis, G., Lowrance, R., and Rathbun, S. L. (1998). Spatiotemporal distributions of bacterivorous nematodes and soil resources in a restored riparian wetland. Ecology 79, 2721–2734. doi: 10.1890/0012-9658(1998)079<2721:SDOBNA>2.0.CO;2
Ettema, C. H., Lowrance, R., and Coleman, D. C. (1999). Riparian soil response to surface nitrogen input: the indicator potential of free-living soil nematode populations. Soil Biol. Biochem. 31, 1625–1638. doi: 10.1016/S0038-0717(99)00072-3
Ferris, H. (2010a). Contribution of nematodes to the structure and function of the soil food web. J. Nematol. 42, 63–67.
Ferris, H. (2010b). Form and function: metabolic footprints of nematodes in the soil food web. Eur. J. Soil Biol. 46, 97–104. doi: 10.1016/j.ejsobi.2010.01.003
Ferris, H., and Bongers, T. (2009). “Indices developed specifically for analysis of nematode assemblages,” in Nematodes as Environmental Indicators, eds M. J. Wilson and T. Kakouli-Duarte (Wallingford: CABI), 124–145. doi: 10.1079/9781845933852.0124
Ferris, H., and Tuomisto, H. (2015). Unearthing the role of biological diversity in soil health. Soil Biol. Biochem. 85, 101–109. doi: 10.1016/j.soilbio.2015.02.037
Ferris, H., Venette, R., and Scow, K. (2004). Soil management to enhance bacterivore and fungivore nematode populations and their nitrogen mineralisation function. Appl. Soil Ecol. 25, 19–35. doi: 10.1016/j.apsoil.2003.07.001
Fitzherbert, E. B., Struebig, M. J., Morel, A., Danielsen, F., Brühl, C. A., Donald, P. F., et al. (2008). How will oil palm expansion affect biodiversity? Trends Ecol. Evol. 23, 538–545. doi: 10.1016/j.tree.2008.06.012
Fox, J., Weisberg, S., Adler, D., Bates, D., Baud-Bovy, G., Ellison, S., et al. (2012). Package ‘Car'. Vienna: R Foundation for Statistical Computing.
Frostegård, Å., Tunlid, A., and Bååth, E. (2011). Use and misuse of PLFA measurements in soils. Soil Biol. Biochem. 43, 1621–1625. doi: 10.1016/j.soilbio.2010.11.021
Guillaume, T., Damris, M., and Kuzyakov, Y. (2015). Losses of soil carbon by converting tropical forest to plantations: erosion and decomposition estimated by δ 13 C. Glob. Chang. Biol. 21, 3548–3560. doi: 10.1111/gcb.12907
Hooper, D. U., Adair, E. C., Cardinale, B. J., Byrnes, J. E. K., Hungate, B. A., Matulich, K. L., et al. (2012). A global synthesis reveals biodiversity loss as a major driver of ecosystem change. Nature 486:105. doi: 10.1038/nature11118
Jairajpuri, M. S., and Ahmad, W. (1992). Dorylaimida: Free-Living, Predaceous and Plant-Parasitic Nematodes. Leiden: E.J. Brill.
Kästner, J., von Knorre, D., Himanshu, H., Erb, M., Baldwin, I. T., and Meldau, S. (2014). Salicylic acid, a plant defense hormone, is specifically secreted by a molluscan herbivore. PLoS ONE 9:e86500. doi: 10.1371/journal.pone.0086500
Khan, Z., and Kim, Y. H. (2007). A review on the role of predatory soil nematodes in the biological control of plant parasitic nematodes. Appl. Soil Ecol. 35, 370–379. doi: 10.1016/j.apsoil.2006.07.007
Klarner, B., Winkelmann, H., Krashevska, V., Maraun, M., Widyastuti, R., and Scheu, S. (2017). Trophic niches, diversity and community composition of invertebrate top predators (Chilopoda) as affected by conversion of tropical lowland rainforest in Sumatra (Indonesia). PLoS ONE 12:e0180915. doi: 10.1371/journal.pone.0180915
Krashevska, V., Klarner, B., Widyastuti, R., Maraun, M., and Scheu, S. (2015). Impact of tropical lowland rainforest conversion into rubber and oil palm plantations on soil microbial communities. Biol. Fertil. Soils 51, 697–705. doi: 10.1007/s00374-015-1021-4
Krashevska, V., Klarner, B., Widyastuti, R., Maraun, M., and Scheu, S. (2016). Changes in structure and functioning of protist (testate amoebae) communities due to conversion of lowland rainforest into rubber and oil palm plantations. PLoS ONE 11:e0160179. doi: 10.1371/journal.pone.0160179
Krashevska, V., Malysheva, E., Klarner, B., Mazei, Y., Maraun, M., Widyastuti, R., et al. (2018). Micro-decomposer communities and decomposition processes in tropical lowlands as affected by land use and litter type. Oecologia 187, 255–266. doi: 10.1007/s00442-018-4103-9
Laumonier, Y., Uryu, Y., Stüwe, M., Budiman, A., Setiabudi, B., and Hadian, O. (2010). Eco-floristic sectors and deforestation threats in Sumatra: identifying new conservation area network priorities for ecosystem-based land use planning. Biodivers. Conserv. 19, 1153–1174. doi: 10.1007/s10531-010-9784-2
Maisonneuve, C., and Rioux, S. (2001). Importance of riparian habitats for small mammal and herpetofaunal communities in agricultural landscapes of southern Québec. Agric. Ecosyst. Environ. 83, 165–175. doi: 10.1016/S0167-8809(00)00259-0
Murfin, K. E., Dillman, A. R., Foster, J. M., Bulgheresi, S., Slatko, B. E., Sternberg, P. W., et al. (2012). Nematode-bacterium symbioses—cooperation and conflict revealed in the “Omics” age. Biol. Bull. 223, 85–102. doi: 10.1086/BBLv223n1p85
Naiman, R. J., Decamps, H., and Pollock, M. (1993). The role of riparian corridors in maintaining regional biodiversity. Ecol. Appl. 3, 209–212. doi: 10.2307/1941822
Neher, D. A., Wu, J., Barbercheck, M. E., and Anas, O. (2005). Ecosystem type affects interpretation of soil nematode community measures. Appl. Soil Ecol. 30, 47–64. doi: 10.1016/j.apsoil.2005.01.002
Newbold, T., Hudson, L. N., Hill, S. L. L., Contu, S., Lysenko, I., Senior, R. A., et al. (2015). Global effects of land use on local terrestrial biodiversity. Nature 520, 45–50. doi: 10.1038/nature14324
Ngosong, C., Gabriel, E., and Ruess, L. (2012). Use of the signature fatty acid 16:1ω5 as a tool to determine the distribution of arbuscular mycorrhizal fungi in soil. J. Lipids 2012, 1–8. doi: 10.1155/2012/236807
Nicol, J. M., Turner, S. J., Coyne, D. L., Nijs, L., den Hockland, S., and Maafi, Z. T. (2011). “Current nematode threats to world agriculture,” in Genomics and Molecular Genetics of Plant-Nematode Interactions (Dordrecht: Springer Netherlands), 21–43. doi: 10.1007/978-94-007-0434-3_2
Okada, H., and Kadota, I. (2003). Host status of 10 fungal isolates for two nematode species, Filenchus misellus and Aphelenchus avenae. Soil Biol. Biochem. 35, 1601–1607. doi: 10.1016/j.soilbio.2003.08.004
Oksanen, J., Blanchet, F., Guillaume Friendly, M., Kindt, R., Legendre, P., McGlinn, D., et al. (2017). Vegan: Community Ecology Package. Available online at: https://cran.r-project.org/package=vegan
Paoletti, A., Darras, K., Jayanto, H., Grass, I., Kusrini, M., and Tscharntke, T. (2018). Amphibian and reptile communities of upland and riparian sites across Indonesian oil palm, rubber and forest. Glob. Ecol. Conserv. 16:e00492. doi: 10.1016/j.gecco.2018.e00492
Pohlert, T. (2014). The Pairwise Multiple Comparison of Mean Ranks Package (PMCMR). Compr. R Arch. Netw. Available online at: https://cran.csiro.au/web/packages/PMCMRplus/index.html
Potapov, A. M., Klarner, B., Sandmann, D., Widyastuti, R., and Scheu, S. (2019). Linking size spectrum, energy flux and trophic multifunctionality in soil food webs of tropical land-use systems. J. Anim. Ecol. doi: 10.1111/1365-2656.13027. [Epub ahead of print].
R Core Team (2018). R: A Language and Environment for Statistical Computing. Available online at: https://www.r-project.org/
Ruess, L. (1995). Studies on the nematode fauna of an acid forest soil: spatial distribution and extraction. Nematologica 41, 229–239. doi: 10.1163/003925995X00198
Schneider, D., Engelhaupt, M., Allen, K., Kurniawan, S., Krashevska, V., Heinemann, M., et al. (2015). Impact of lowland rainforest transformation on diversity and composition of soil prokaryotic communities in sumatra (Indonesia). Front. Microbiol. 6:1339. doi: 10.3389/fmicb.2015.01339
Schulz, G., Schneider, D., Brinkmann, N., Edy, N., Daniel, R., Polle, A., et al. (2019). Changes in trophic groups of protists with conversion of rainforest into rubber and oil palm plantations. Front. Microbiol. 10:240. doi: 10.3389/fmicb.2019.00240
Ter Braak, C. J. F., and Šmilauer, P. (2012). Canoco Reference Manual and User's Guide: Software for Ordination, Version 5.0. Ithaca, NY: Microcomputer Power.
van den Hoogen, J., Geisen, S., Routh, D., Ferris, H., Traunspurger, W., Wardle, D. A., et al. (2019). Soil nematode abundance and functional group composition at a global scale. Nature 572, 194–198. doi: 10.1038/s41586-019-1418-6
van der Putten, W. H., Cook, R., Costa, S., Davies, K. G., Fargette, M., Freitas, H., et al. (2006). Nematode interactions in nature: models for sustainable control of nematode pests of crop plants? Adv. Agron. 89, 227–260. doi: 10.1016/S0065-2113(05)89005-4
Wall, D. H., and Six, J. (2015). Give soils their due. Science. 347, 695–695. doi: 10.1126/science.aaa8493
Wang, K.-H., and Hooks, C. R. R. (2011). “Chapter 4: Managing soil health and soil health bioindicators through the use of cover crops and other sustainable practices,” in MD Organic Vegetable Growers, ed G. E. Brust, 1–18.
Wickham, H. (2009). ggplot2: Elegant Graphics for Data Analysis. New York, NY: Springer-Verlag. doi: 10.1007/978-0-387-98141-3
Xiao, H. F., Tian, Y. H., Zhou, H. P., Ai, X. S., Yang, X. D., and Schaefer, D. A. (2014). Intensive rubber cultivation degrades soil nematode communities in Xishuangbanna, southwest China. Soil Biol. Biochem. 76, 161–169. doi: 10.1016/j.soilbio.2014.05.012
Yeates, G. W., and Bongers, T. (1999). Nematode diversity in agroecosystems. Agri. Ecosyst. Environ. 74, 113–135. doi: 10.1016/B978-0-444-50019-9.50010-8
Yeates, G. W., Bongers, T., Goede, R. G. M., de Freckman, D. W., and Georgieva, S. S. (1993). Feeding habits in soil nematode families and genera—an outline for soil ecologists. J. Nematol. 25, 315–331.
Young-Mathews, A., Culman, S. W., Sánchez-Moreno, S., Toby O'Geen, A., Ferris, H., Hollander, A. D., et al. (2010). Plant-soil biodiversity relationships and nutrient retention in agricultural riparian zones of the Sacramento Valley, California. Agrofor. Syst. 80, 41–60. doi: 10.1007/s10457-010-9332-9
Keywords: microfauna, trophic groups, land use, monoculture, lowland rainforest, riparian area
Citation: Krashevska V, Kudrin AA, Widyastuti R and Scheu S (2019) Changes in Nematode Communities and Functional Diversity With the Conversion of Rainforest Into Rubber and Oil Palm Plantations. Front. Ecol. Evol. 7:487. doi: 10.3389/fevo.2019.00487
Received: 10 September 2019; Accepted: 29 November 2019;
Published: 13 December 2019.
Edited by:
Charles K. Lee, University of Waikato, New ZealandReviewed by:
Manqiang Liu, Nanjing Agricultural University, ChinaMarek Renčo, Institute of Parasitology, Slovak Academy of Sciences (SAS), Slovakia
Copyright © 2019 Krashevska, Kudrin, Widyastuti and Scheu. This is an open-access article distributed under the terms of the Creative Commons Attribution License (CC BY). The use, distribution or reproduction in other forums is permitted, provided the original author(s) and the copyright owner(s) are credited and that the original publication in this journal is cited, in accordance with accepted academic practice. No use, distribution or reproduction is permitted which does not comply with these terms.
*Correspondence: Valentyna Krashevska, dmtyYXNoZUBnd2RnLmRl