Controlled-Release Dispenser and Dry Trap Developments for Drosophila suzukii Detection
- 1Invasive Insect Biocontrol and Behavior Laboratory, Beltsville Agricultural Research Center-West, USDA-ARS, Beltsville, MD, United States
- 2Department of Biological Sciences, Towson University, Towson, MD, United States
The spotted wing drosophila (SWD), Drosophila suzukii, is an exotic vinegar fly originating from South-East Asia and has invaded many American and European countries. This devastating pest has caused severe damage on soft-skinned fruit crops, which has resulted in large economic losses. Although many D. suzukii detection devices have been developed and used by growers/farmers in orchards, most of them did not meet their expectation. More simple, convenient, and efficient detection tools are still urgently needed so that growers/farmers can make timely management decision. In our previous study a quinary blend, containing acetoin, as a long-range, and ethyl octanoate, as a short-range attractant for SWD, had been identified. In this study, a controlled-release polyethylene dispenser containing five identified attractants: acetoin, ethyl octanoate, ethyl acetate, phenethyl alcohol, and acetic acid, was tested in laboratory conditions for release rates, as well as in a blueberry field and an adjacent wooded area for trapping activity. Release of the most D. suzukii attractants from the polyethylene dispenser was constant. In the laboratory, release rates of ethyl acetate (0.3 g/day over 14 days), phenethyl alcohol (0.0054 g/day over 36 days), and acetic acid (0.13 g/day over 36 days) followed zero order kinetics. Acetoin and ethyl octanoate were blended together and had a varied release rate. In the field, dry and liquid traps baited with the optimized controlled-release dispenser were evaluated and compared with liquid traps baited with apple cider vinegar (ACV). During blueberry harvest season in 2018, the liquid traps containing drowning solution baited with the optimized controlled-release dispenser caught both male and female D. suzukii 2 weeks earlier and had significantly higher selectivity than that of ACV traps in the field. Additionally, in 2019 field tests, dry traps baited with the controlled-release dispenser demonstrated earlier detection compared to the ACV traps. Due to the simplicity of the dry trap design and easy processing, it has great potential to be an efficient and convenient D. suzukii detection tool for growers/farmers.
Introduction
The infestation of a variety of commercial fruits by the highly invasive fruit fly, spotted wing drosophila (SWD) Drosophila suzukii Matsumura (Diptera: Drosophilidae), recently detected in North America (Goodhue et al., 2011; Hauser, 2011; Lee et al., 2011; Walsh et al., 2011), South America (Depra et al., 2014; Andreazza et al., 2016; Lavagnino et al., 2018), and Europe (Calabria et al., 2012; Cini et al., 2014; Poyet et al., 2014; Aabanowska and Wojciech, 2015; Briem et al., 2015; Mazzetto et al., 2015; Radonjic and Hrncic, 2015; Arno et al., 2016; Hamby et al., 2016; Briem et al., 2018) is the cause of great concern because of its economic impact (Goodhue et al., 2011; Walsh et al., 2011; Cini et al., 2012; Farnsworth et al., 2017). To combat D. suzukii, many preventive studies, such as attractant identification (Cha et al., 2012; Landolt et al., 2012; Kleiber et al., 2014; Abraham et al., 2015; Cha et al., 2015; Mazzetto et al., 2016; Feng et al., 2018; Jaffe et al., 2018; Zhang and Feng, 2019), trap comparison (Lee et al., 2012; Lee et al., 2013; Iglesias et al., 2014; Renkema et al., 2014; Kirkpatrick et al., 2016), management methodology (Cuthbertson et al., 2014; Hampton et al., 2014; Rice et al., 2017; Yousef et al., 2018), and biological control approach (Gabarra et al., 2015; Woltz et al., 2015; Dancau et al., 2017; Girod et al., 2018; Matsuura et al., 2018; Renkema and Cuthbertson, 2018; Wolf et al., 2018), have been conducted to reduce post-infestation measures such as pesticide usage (Klick et al., 2016; Wallingford et al., 2016; Knapp et al., 2019). Among all of these efforts, development of a more efficient, reliable, and convenient detection tool for D. suzukii population in orchards/farms is essential for growers/farmers to make decisions on timely applications of control interventions.
The liquid trap, consisting of a bottle/cup baited with liquid such as apple cider vinegar (ACV), wine, other vinegars or yeast solution, is the most common and popular D. suzukii detection device currently used in the fields by growers/farmers and researchers (Cha et al., 2012; Landolt et al., 2012; Belien et al., 2014; Hamby et al., 2014; Iglesias et al., 2014; Kirkpatrick et al., 2017; Lethmayer and Egartner, 2017; Briem et al., 2018). However, the bulk liquid bait (or drowning solution) needs to be carried into the field and trap contents must be taken to a laboratory for processing, which is labor intensive and inefficient (Kirkpatrick et al., 2018). In addition, the life time of a bait (or lure) is typically short and need to be replaced frequently. Moreover, identifying and counting massive amounts of flies caught in liquid traps is time consuming.
Although some SWD attractive compounds have been identified from fermentation volatiles and synthetic versions of these chemicals have been added into the aqueous drowning solution or partially released from vials by diffusion (Cha et al., 2012, 2015; Landolt et al., 2012), measurement and control of the release rates of the compounds within lures are difficult. Additionally, in order to increase trap efficiency, a long-lasting attractive source with a release rate closely simulating that of the host is also required (Murali-Baskaran et al., 2018).
The most common semiochemical release devices typically do not maintain a constant release rate, and instead it usually decreases during the season exhibiting first-order kinetics (Weatherston et al., 1985; McDonough et al., 1992; Bradley et al., 1995; Mayer and Mitchell, 1998; Heuskin et al., 2011). From an insect pest management standpoint, it is desirable to have a device that could release the semiochemical at a constant rate (Zhang, 2005). Such constant release devices with zero-order kinetics based on glass capillaries (Weatherston et al., 1985), wax-based formulations (Atterholt et al., 1999), and polyethylene vials/sachets (Pajares et al., 2010) have been tested in the laboratory and/or applied in the field for some insect pest control. Therefore, a dispenser, that is capable of sustainably releasing D. suzukii volatile attractive compounds to the surrounding area, at a constant rate, and over a long period of time needs to be developed in a SWD integrated pest management program. The current research is aimed at developing a simple, convenient, and efficient D. suzukii detection tool by evaluating (1) type of dispenser (controlled-release dispenser vs. uncontrolled-release dispenser) and (2) type of trap (dry trap vs. liquid trap) to facilitate accurate pest management measures in IPM program. Early detection of D. suzukii population and species selectivity are two major performance criterions for this study.
Materials and Methods
Chemicals
Volatile chemicals used in this research are commercially available and purchased from Sigma-Aldrich (St. Louis, MO, United States): acetoin (AT, 3-hydroxy-2-butanone), 99%, CAS 512-86-0; ethyl octanoate (EO), 99+ %, CAS 106-32-1; acetic acid (AA), 99.7+ %, CAS 64-19-7; phenethyl alcohol (PE), 99+ %, CAS 60-12-8; and ethyl acetate (EA), solvent grade, CAS 141-78-6.
Controlled-Release Dispensers With Dry Traps
SWD controlled-release dispensers were made of clear polyethylene tubing rolls (2′′ width; 2, 3, 4, and 6 Mil thicknesses; ULINE, Pleasant Prairie, WI, United States). The white polyester felt strip (1′′ width, 1/8′′ thickness, Grainger, Lake Forest, IL, United States) was used as a carrier or reservoir of the volatile chemicals. The FoodSaver heat sealer (Sunbeam Products Inc, Boca Raton, FL, United States) was employed to seal the polyethylene tubing. To prepare dispensers, the polyethylene tubing was first cut into 80 mm length pieces and heat sealed at one end (using the heat sealer) to create a bag. Slice(s) of felt strip was (were) inserted into the bag, and the desired amount of volatile chemical was loaded into it. The top of the bag was then heat sealed to form a sachet, leaving space above the seal to punch holes into the sachet to allow hanging within a trap. SWD controlled-release dispensers consisted of four sachets, differentiated by the number of holes punched into the space above the top seal of the polyethylene sachets and chemicals (EA, 1 hole; PE, 2 holes; AA, 3 holes, AT/EO, 4 holes). For the EA sachet, 5 mL ethyl acetate were loaded onto 2 slices of felt strips (40 mm length). For the PE sachet, 1 mL phenethyl alcohol was loaded on 1 slice of felt strip (40 mm length). For the AA sachet, 5 mL acetic acid were loaded on 2 slices of felt strips (40 mm length). For the AT/EO sachet, 1 mL and 2 mL of a mixture of acetoin and ethyl octanoate (1:1 ratio) were loaded on 1 slice of felt strip (30 mm length), respectively. After polyethylene sachet preparation, they were kept in a refrigerator until field use. Release rates of each sachet with all four different kinds of thicknesses polyethylene (2, 3, 4, and 6 Mil) were individually measured in laboratory conditions.
For the field trials, the EA sachet was made using polyethylene tubing with a thickness of 6 Mil for lure longevity, and the PE and AA sachets were made using polyethylene tubing of 2 Mil thickness for maximum active material releasing. The sachets of AT/EO were made into each of the 2, 3, 4, and 6 Mil polyethylene tubing with 1 ml and 2 ml loadings. For placement into the field, one of each of the sachets (EA, PE, AA, and AT/EO) were tied together by a paper clip (Figure 1A) and secured onto the non-sticky side of a dry trap (single sided 16 × 4.75′′ window bug catchers, Alpha Scents, Inc., West Linn, OR, United States) with a twist-tie (Figure 1B, one small hole was punched on each side of dry trap). Dry sticky traps without lure were placed within the field to serve as blank controls.
Uncontrolled-Release Dispensers With Liquid Traps
Commercially available Victor yellow jacket & flying insect traps (Great Lakes IPM Inc., Vestaburg, MI, United States) were filled with ∼300 mL tap water containing a surfactant (Seventh generationTM natural dish liquid - Free & Clear, Shoppers Food and Pharmacy, College Park, MD, United States 1.1 ml/L) as a drowning solution were used as liquid traps (Feng et al., 2018; Zhang and Feng, 2019). For uncontrolled release dispenser preparation, 1 mL AT:EO blend (1:1) was loaded onto a cotton ball contained within a polypropylene flex micro-centrifuge tube (1.5 mL Eppendorf micro-centrifuge tube, VWR International, Radnor, PA, United States) and the lid of the tube was then closed. In the field, the micro-centrifuge tube lure was opened and the lid was snapped into one of four openings on the cap of the Victor® trap, leaving the three remaining openings to serve as entrances for attracted insects (Feng et al., 2018; Zhang and Feng, 2019). The remaining components (AA, EA, and PE) were added directly to the soapy water drowning solution within the Victor traps at the following volumes: 20 mL 3:1 AA/EA and 1 mL PE before placement into the field. Even though some more complex SWD liquid baits and traps are commercially available on the market, due to the availability and ease of purchase, Victor traps filled with 300 mL ACV (Essential Everyday®, 5% acidity, Shoppers Food and Pharmacy, College Park, MD, United States) were used as SWD standard trap and bait for activity comparison.
Controlled-Release Dispensers With Liquid Traps
The four different sachets, containing the EA, PE, AA, and AT/EO described in the previous dry trap section, were tied together using a paper clip (Figure 1A) and attached to one of the openings in the lid of the Victor® trap (Feng et al., 2018; Zhang and Feng, 2019) so that they were suspended inside of the liquid trap above drowning solution.
Laboratory Release Rate Measurement
A release rate study of SWD attractant sachets made of polyethylene tubing with different thicknesses was conducted in a laboratory fume hood (temperature: 20–25°C, face velocity: 129 feet/min) by measuring the remaining residues for 36 days. All controlled-release sachets (five replicates) were suspended on hooks in fume hood individually and each sachet was weighted by an Ohaus GA110 analytical balance (Pine Brook, NJ, United States) every 24- or 72-h (weekend) and amount of residue of was recorded. Mean accumulated weight loss of each sachet was calculated.
Field Test
Field tests were conducted in a blueberry field and nearby wooded area at Butler’s Orchard (22222 Davis Mill Rd, Germantown, MD, United States) during the summers of 2018 and 2019. On Jun 13, 2018, liquid traps baited with controlled-release and uncontrolled-release dispensers were hung from the branches of mixed variety (Duke, Spartan, and Blueray) highbush blueberry bushes, deployed in six rows skipping one row in between each treatment row (ca. x m). Each row received one of each of the treatments. Six replications were tested for each treatment (N = 6). Placements of traps were randomized weekly in each row and were placed at least 5 m apart from other traps. Blank control liquid traps consisted of a Victor® trap filled with only the tap-water/soap drowning solution (because the blank control liquid traps caught almost nothing, they were not included in 2018 data analysis). On May 14, 2019, dry trap (baited with controlled-release dispenser), blank control dry trap (without dispenser), and ACV liquid traps were set up in the same field and wooded area as previously descripted (N = 5 for each treatment). Because the blank control liquid traps caught almost nothing in 2018 field season, they were omitted in these field tests. After a dry trap was hung in the field, the paper layer was torn off to expose the sticky layer. All traps were collected every 7 days. The contents of the liquid traps were emptied into separate containers and returned to the laboratory for identification. Dry traps were collected and the lures were removed and the traps were folded in half with the sticky side in taking care to not press the trap together fully so that the catch could be counted within the laboratory. Liquid traps were then refilled and the dry trap lures were placed onto new dry traps. Uncontrolled-release dispensers were replaced weekly. Controlled-release dispensers were changed every other week.
Trap Catch Counting
Captures from traps were sorted into the following categories: SWD (D. suzukii – male and female), African fig fly (Zaprionus indianus), other drosopila spp., big flies (house flies, crane flies, etc.), and other arthropods. Male SWD were identified by the characteristic spot near the tip of each wing, while females were identified by the large-serrated ovipositor present at the posterior portion of the abdomen. Mean (±SE) selectivity per trap for weeks 1–4, and 8 during blueberry harvest for 2018. Trap catches from liquid traps were strained using a simple handheld strainer made from mosquito netting. The strained individuals were then placed into a Petri dish and separated and counted under Olympus SZ60 and SZ61 microscopes (Waltham, MA, United States). Microscope stages were illuminated by Lexco iLED Series LED light sources (Bothell, WA, United States). Catches containing greater than four milliliters of strained individuals were approximated by counting two milliliters, and then multiplying the counts by the calculated factor. Dry sticky trap catches were visually counted under the same microscopes as described above. Due to the difficulty in discerning the female serrated ovipositor (degradation occurred), counts for female SWD were approximated by calculating the percentage of females caught in the liquid traps and then using that percentage to adjust the total counts from the sticky trap.
Statistical Analyses
For laboratory release rate study, mean accumulated weight loss of each formulation was plotted against time (day). The data were fitted with logarithmic trendline and kinetic equations and R2 values were obtained (Microsoft Office Excel 2016). For field work, weekly capture data and of each of the six treatment replicates were summed to calculate the attractiveness (# D. suzukii caught) and selectivity (% D. suzukii/total catch) of the lures. Average catch per trap was additionally calculated. Means of catch results were compared using a One-way ANOVA followed by a Tukey’s post-test to determine significance (α = 0.5). Trap data were square-root transformed to more closely conform to the assumptions of normalcy. Selectivity results were arcsine-square-root transformed to achieve normalcy and the transformed data were then analyzed using a One-way ANOVA with a Tukey’s post-test. All data presented is in the untransformed state.
Results
Laboratory Release Rate
In laboratory conditions, releases of EA (5 mL loading) from polyethylene sachets were constant (2 Mil, ∼1.09 g/day; 3 Mil, ∼0.49 g/day; 4 Mil, ∼0.41 g/day; 6 Mil, 0.30 g/day) over 4 – 14 days (Figure 2A). Similarly, releases of PE (1 mL loading) from polyethylene sachets were constant (2 Mil, ∼0.0054 g/day; 3 Mil, ∼0.0030 g/day; 4 Mil ∼0.0025 g/day; 6 Mil, ∼0.0023g/day) over 36 days (Figure 2B); and releases of AA (5 mL loading) from polyethylene sachets were constant (2 Mil, ∼0.13 g/day; 3 Mil, ∼0.068 g/day; 4 Mil ∼0.056 g/day; 6 Mil, ∼0.051g/day) over 36 days (Figure 2C). The curve pattern of release rate for these compounds from all four sachets with different polyethylene film thickness conformed pretty well to zero-order kinetic model. However, release of AT/EO blend (AT: EO = 1: 1) is more complicated. Releases of AT/EO blend (1 mL loading) from polyethylene sachets followed first-order kinetics (Figure 2D), as from the micro-centrifuge tube dispenser (Feng et al., 2018), while releases of AT/EO blend (2 mL loading) from polyethylene sachets were depended on polyethylene film thickness (Figure 2E). With 2 Mil thickness, the blend was diffused following first-order kinetics (R2 = 0.9273). Amount released from sachet was not constant and varied from 0.12 g/day to 0.024 g/day over 36 days. However, release rate curves were much close to straight lines when film thickness was increased. AT/EO blend was diffused approaching zero-order kinetics from 3 Mil (R2 = 0.9589 zero-order vs. R2 = 0.9165 first-order), 4 Mil (R2 = 0.9743 zero-order vs. R2 = 0.8985 first-order), and 6 Mil (R2 = 0.9785 zero-order vs. R2 = 0.8944 first-order) thickness polyethylene sachets (3 Mil ∼0.040 g/day; 4 Mil ∼0.033 g/day; 6 Mil, ∼0.031g/day) over 30 days (Figure 2E). Release rates of different D. suzukii volatile attractive components from polyethylene sachets with different film thickness under laboratory conditions are summarized in Table 1.
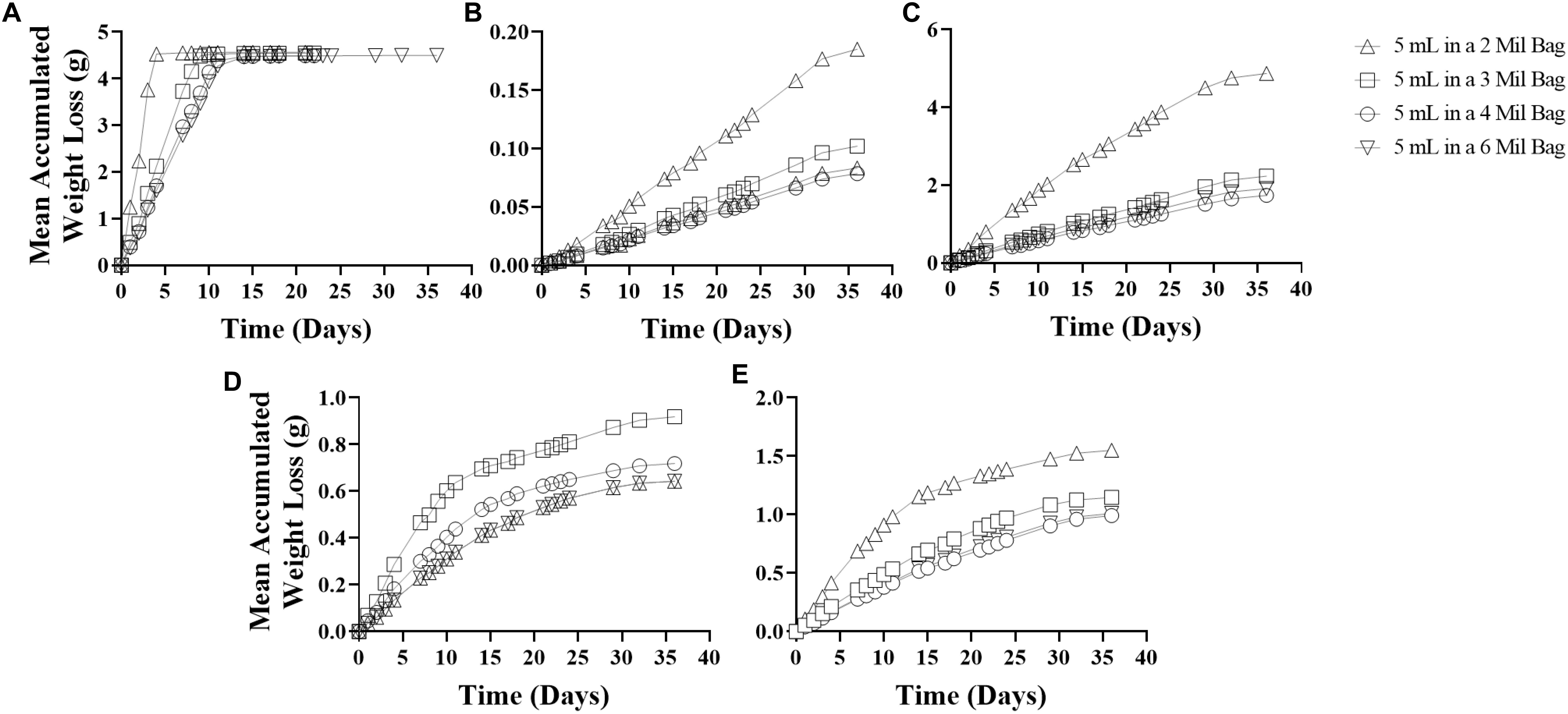
Figure 2. Release rates of different compounds from sachets with different polyethylene film thickness in laboratory conditions for a period of 36 days. (A) ethyl acetate (EA); (B) phenethyl alcohol (PE); (C) acetic acid (AA); (D) acetoin/ethyl octanoate (AT/EO) (1:1, 1 mL); (E) acetoin/ethyl octanoate (AT/EO) (1:1, 2 mL).
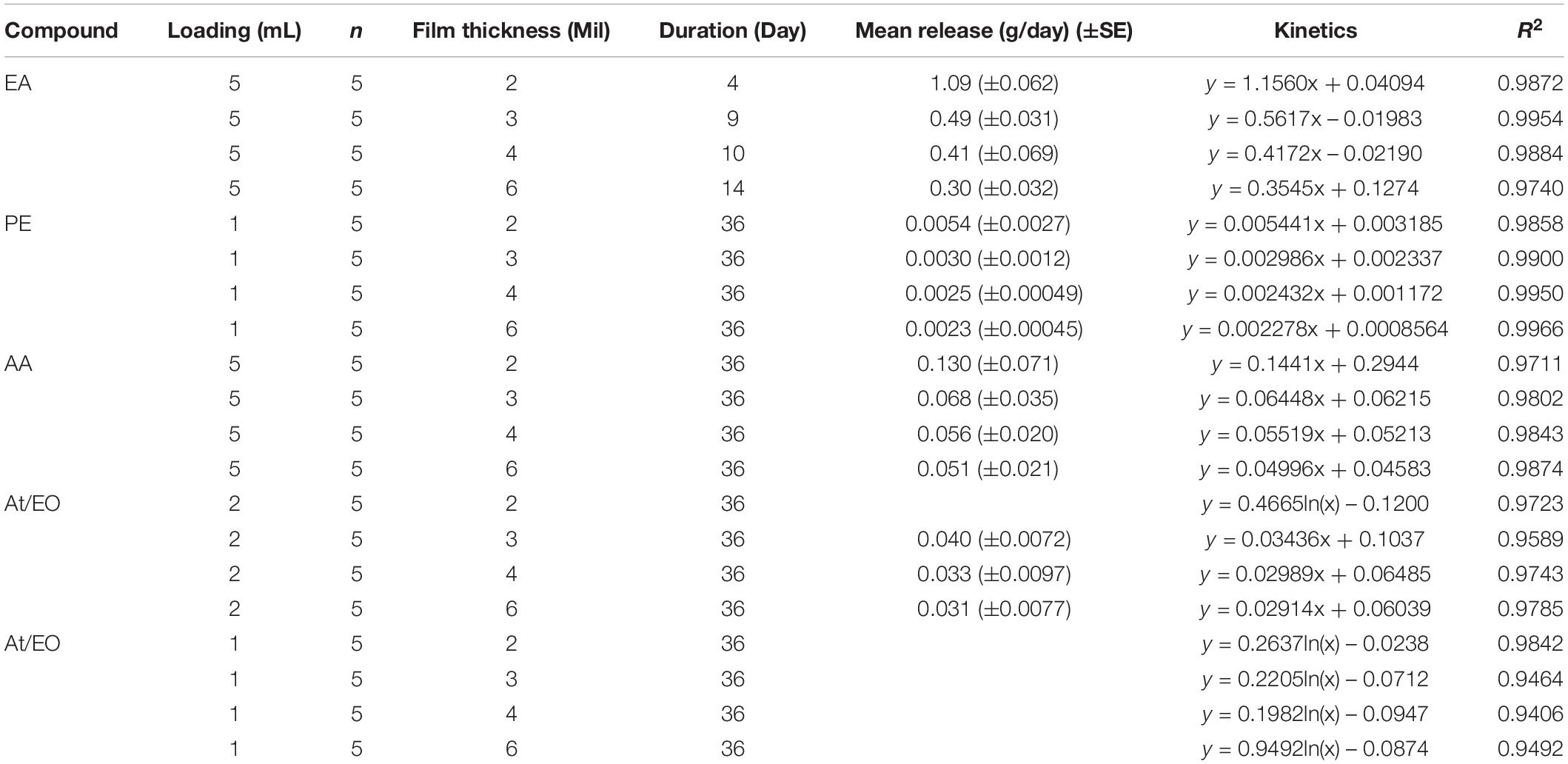
Table 1. Release rates of D. suzukii volatile attractive components from polyethylene sachets with different film thickness in a fume hood under laboratory conditions for a period of 36 days.
Field Tests
Results from the field traps show that there is not a significant difference in attractiveness or selectivity between the any of the controlled-release dispensers (AT/EO blend) using different polyethylene sachet with different thickness in the blueberry field using liquid traps during the first 8-weeks of trapping (Figures 3A,B). The average weekly catch of SWD for the each of the controlled-release dispensers within the blueberry field are as follows: 1 mL AT/EO and 2 Mil polyethylene sachet = 189.6 ± 136.4, 1 mL AT/EO and 3 Mil polyethylene sachet = 128.9 ± 88.5, 1mL AT/EO and 4 Mil polyethylene sachet = 197.1 ± 163.4, 2 mL AT/EO and 2 Mil polyethylene sachet = 336.6 ± 286.1, 2 mL AT/EO and 3 Mil polyethylene sachet = 250.8 ± 213, and 2 mL AT/EO and 4 Mil polyethylene sachet = 220.9 ± 163.4. The average selectivity for each of the dispensers is as follows: 1 mL AT/EO in 2 Mil polyethylene sachet = 0.51 ± 0.11, 1 mL AT/EO in 3 Mil polyethylene sachet = 0.45 ± 0.09, 1 mL AT/EO in 4 Mil polyethylene sachet = 0.42 ± 0.11, 2 mL AT/EO in 2 Mil polyethylene sachet = 0.49 ± 0.11, 2 mL AT/EO in 3 Mil polyethylene sachet = 0.48 ± 0.11, with 2 mL AT/EO in 4 Mil polyethylene sachet = 0.46 ± 0.09.
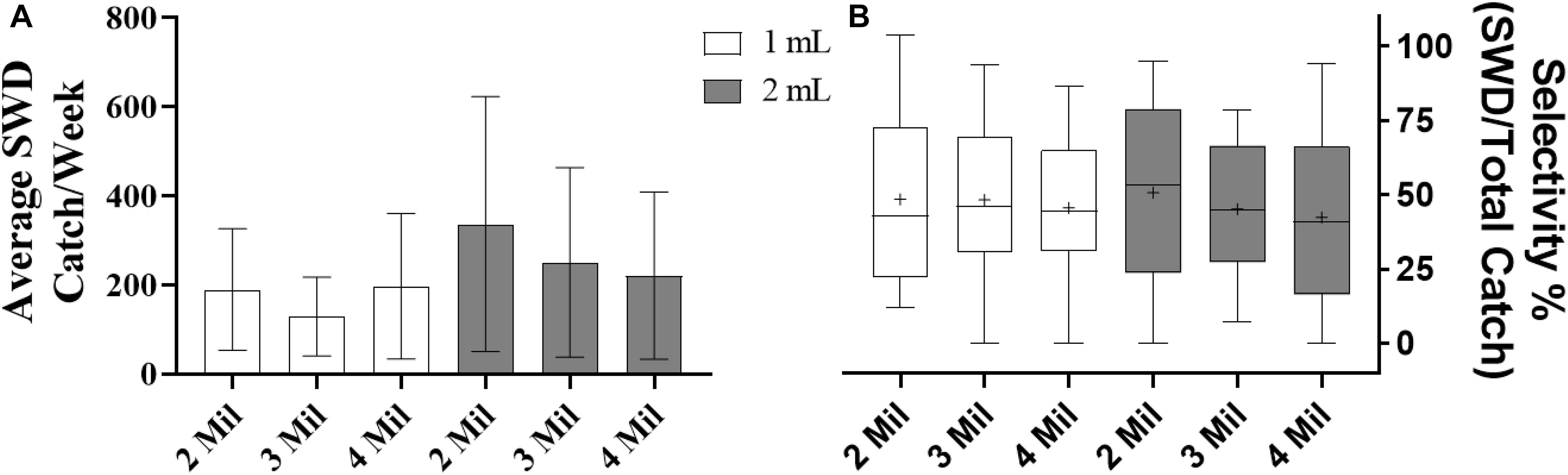
Figure 3. Average weekly SWD catch and selectivity over the first 8 weeks of trapping period during 2018 by liquid trap baited with different controlled-release dispensers (AT/EO blend). (A) Average catch in blueberry field (N = 48, df = 5, 42, F = 0.064, P = 0.9971); (B) selectivity within blueberry field (N = 47, df = 5, 41, F = 0.081, P = 0.9949). Means separated by One-way ANOVA with a Tukey’s posttest. Error bars represent standard error of the mean. The boxes enclose the lower and upper quartiles, the solid line represents the median, the plus represents the mean, and the whiskers represent the minimum and maximum value. The values 1 and 2 mL represent the amount of AT/EO blend loaded and the values 2 Mil, 3 Mil, and 4 Mil represent polyethylene thickness.
The first catch of an SWD, in 2018, varied between the lures and formulations (Table 2). For the uncontrolled release dispenser (U), two female SWD were caught in the blueberry field and five females were caught in the wooded area during the week of June 20, 2018. Eight males were found in these formulation traps during the second week of collecting (June 27, 2018) in the wooded area, and then a week later (July 3, 2018) nine males were found within traps from the blueberry field. Traps utilizing the liquid trap baited with controlled-release dispenser (C) caught two males and females within the blueberry field during the first week of trapping (June 20). They also caught 2 females within the wooded area during the same trapping time. Males were not caught in the wooded area until the third trapping week (July 3). The standard apple cider vinegar traps (A) performed well within the wooded area catching six males and 167 females during the first week of trapping, however detection within the blueberry field did not initially occur until the third trapping (July 3) week where two males were caught and then one female SWD was found in the traps during the fourth trapping week of July 7, 2018.
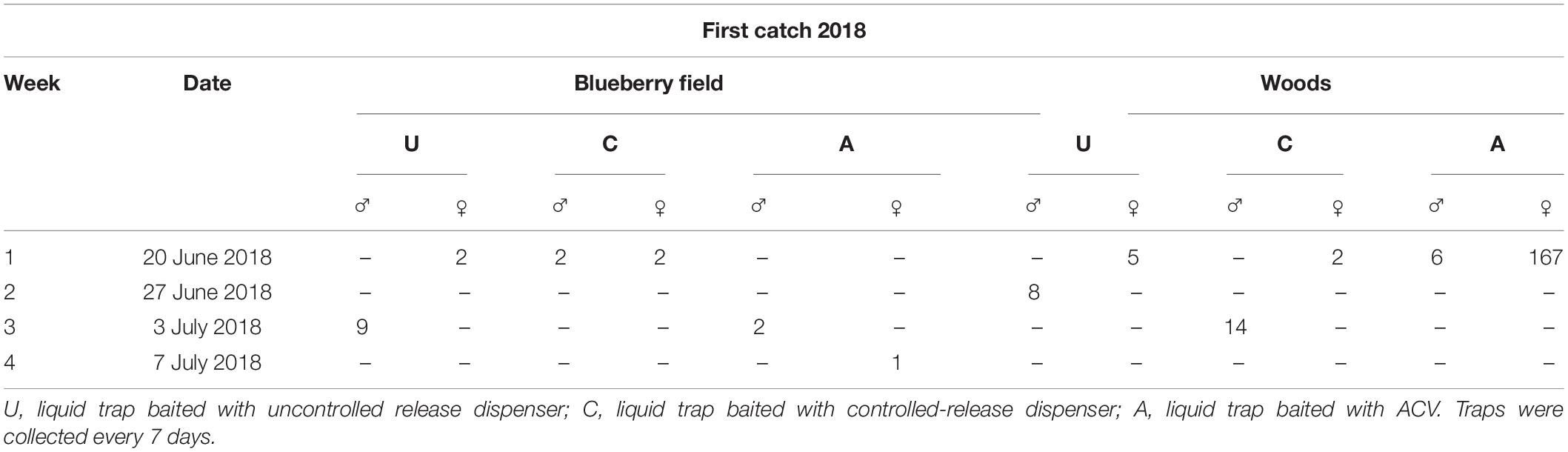
Table 2. Week, date, and number of males and females of first catch of D. suzukii by different trapping systems within a blueberry field and a nearby wooded area in 2018.
For the first 8 weeks of trapping (harvest) within the blueberry field, in 2018, the mean catch per trap of male and female D. suzukii for the A, U and C traps were not significantly different (Table 3). A traps caught on average 17.7 ± 6.7 females and 18.9 ± 8.3 males, U traps caught 9.2 ± 3 females and 14.8 ± 5.1 males, and C traps caught 19.2 ± 6.4 females and 37 ± 14.7 males. The average non-target Drosophila spp. catch per trap for each of the types of lures were also not significant. A traps caught on average 45.2 ± 8.8 non-target drosphilids, while U and C traps caught 34.5 ± 4.9 and 35.2 ± 5.9 respectively. Both U and C traps caught significantly lower number of large flies over this trapping period with both traps catching and average of 0.02 ± 0.02 large flies, while A caught 5.2 ± 1.3 large flies. The U and C traps also differed statistically from A in the average number of other arthropods (lepidotera, coleopteran, arachnids, etc.) caught per trap. U and C traps caught 6.5 ± 0.76 and 10.6 ± 0.95 other arthropods per trap respectively, while A traps caught 24.5 ± 4.5 per trap. The average SWD female and male catches per trap within the wooded area were additionally not significant. A traps caught on average 14.4 ± 3.6 females and 23.6 ± 9.6 males, U traps caught 10.4 ± 2 females and 24.1 ± 6.1 males, and C traps caught 10.1 ± 2.2 females and 30.8 ± 8.6 males. The average catches for non-target drosophila were significantly different for each of the traps. A traps caught on average 355.9 ± 49.9 non-target drosophila, while U and C traps caught 519.8 ± 67.2 and 179.6 ± 19.9 non-target drosophila respectively. Both U and C trap average catches for large flies, 0.06 ± 0.04 and 0.02 ± 0.02 respectively, were significantly lower than the average catch for A traps 4.3 ± 0.7. The average catches of other arthropods were not significantly different between the traps. A, U, and C traps caught on average 70.8 ± 13.3, 49.9 ± 5.1, and 60.9 ± 6.9 other arthropod individuals per trap respectively.
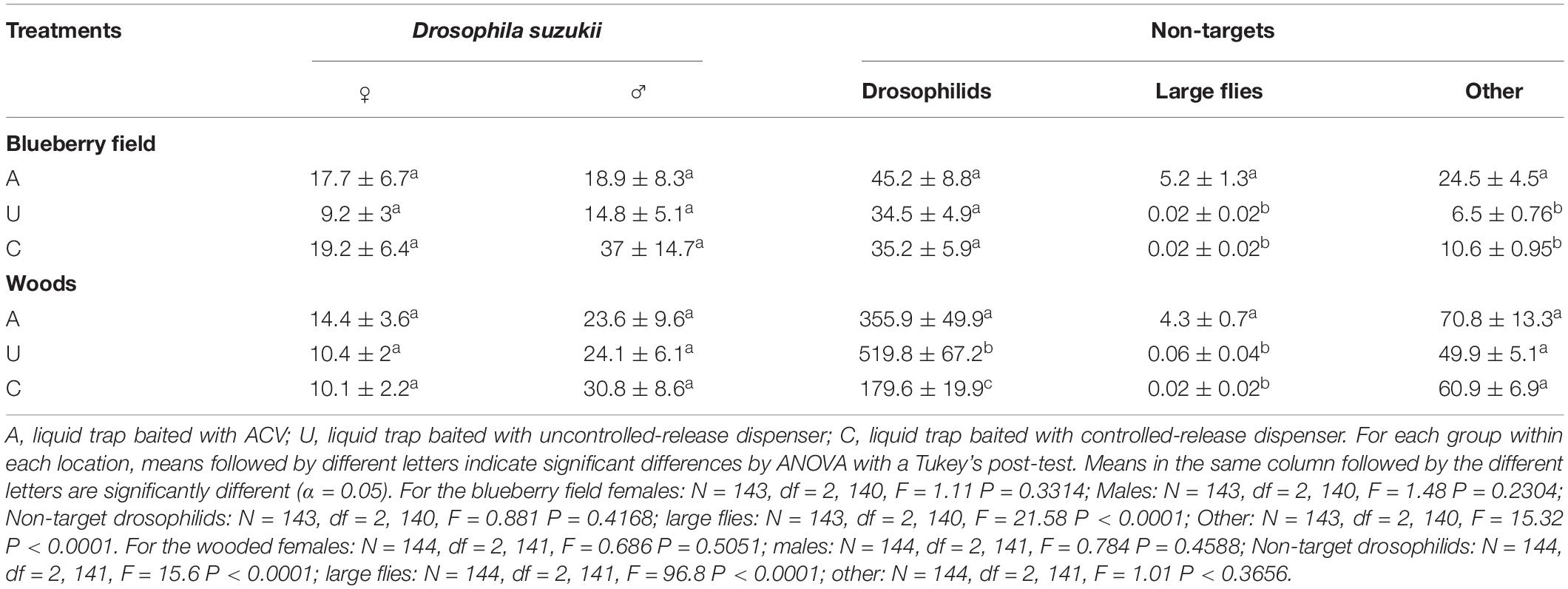
Table 3. Mean (±SE) catch (per trap) of female, male, other drosophilids, large flies, and other non-target arthropods through blueberry harvest period (first 8 weeks of trapping) of 2018.
The average percent selectivity per trap (SWD catch/total catch) for each week of harvest in 2018 can be seen in Table 4. Selectivity generally increased for traps, within the blueberry field, over the course of the 8 week harvest period. For week one average selectivity for A traps was 0, significantly lower than the selectivity of both U and C traps that were 0.36 ± 0.23% and 1.1 ± 0.71% respectively. Week two again had A traps with an average selectivity per trap of 0, with U traps increasing slightly to 0.49 ± 0.49%, and C traps increasing to 7.5 ± 3.7%. Week three saw A traps have ca. 0.34 ± 0.34% selectivity, and this was not significantly different from U and C traps with average selectivity per trap of 7.4 ± 4.3% and 3.7 ± 1.5. The selectivity of A traps increased slightly in the fourth week of trapping with an average selectivity of 0.48 ± 0.48% per trap. This was not significantly different from the selectivity found within U traps 4.3 ± 2.6%. A selectivity was significantly lower than the selectivity found within C traps, 7.6 ± 2.5%, however C trap selectivity was not significantly different from the U trap selectivity. Week five saw increases in selectivity across all types of traps. Selectivity for A, U, and C traps were not significantly different with averages of 5.3 ± 2.2%, 26.7 ± 9.3%, and 23.8 ± 1.5% respectively. Week six saw selectivity within A traps decrease ca. 7-fold to 0.76 ± 0.76%, while both the U and C traps saw slight increases in selectivity to 31.7 ± 2.7% and 39.1 ± 8.6% respectively. Week seven A trap selectivity increased to 7.6 ± 3.5%, while U trap selectivity decreased to 20.3 ± 5.4%, and C trap selectivity decreased slightly to 38.1 ± 6%. By week eight A selectivity had increased to 59.8 ± 1.49%, U trap selectivity increased to 63.4 ± 4.2%, and C trap selectivity increased to 71.4 ± 4.4%. Similarly, the selectivity within the wooded area increased over the 8-week trapping period with noticeable decreases in week six and seven, and then increasing again in week eight. For week one average selectivity per trap for each type of trap was low. A traps had an average selectivity per trap of 3 ± 0.59%, and U and C traps were significantly lower than this with values of 0.08 ± 0.03% and 0.09 ± 0.06% respectively. Week two A trap selectivity diminished to 0.22 ± 0.16%, while both U and C trap selectivity increased to 0.24 ± 0.07% and 0.1 ± 0.07% respectively. Week three again showed an increase in selectivity across the traps. A trap selectivity was 0.62 ± 0.32%, and while U and C trap selectivity was only slightly higher, 2.1 ± 0.52% and 1.5 ± 0.79% respectively, these differences were not significant. In week four A trap selectivity increased by ca. 12-fold to 7.4 ± 0.75%. U trap selectivity increased by ca. 1.9-fold to 3.9 ± 1.2%, and C trap selectivity increased by ca. 8.5-fold to 21.7 ± 2.6%. Week five again saw selectivity increases across the traps with A selectivity increasing to 8.2 ± 1.5%, U trap selectivity increasing to 13.2 ± 2.3%, and C trap selectivity increasing to 20.4 ± 4.1%. Week six however saw a decrease in selectivity across the traps. A selectivity fell ca. 1.8-fold to 4.5 ± 0.81%, U trap selectivity fell ca. 2-fold to 6.6 ± 1.7%, and C trap selectivity fell ca. 4-fold to 5.1 ± 1.8%. Week seven showed a further decrease in selectivity for A and U traps. A trap selectivity decreased ca. 2.4-fold to 1.9 ± 0.36%, and U trap selectivity dropped slightly to 5 ± 0.91%. C trap selectivity conversely increased by slightly to 8.3 ± 1.4%. During the final week the selectivity of each type of trap increased greatly. A trap selectivity increased ca. 20.8-fold to 39.7 ± 7.2%, U trap selectivity increased ca. 6-fold to 29.8 ± 2.4%, and C trap selectivity increased ca. 6.2-fold to 51.2 ± 6.1%.
During the first week of trapping in 2019, three males were caught by dry sticky traps using the SWD dispenser in the blueberry field (Table 5). The following week a single male was caught by dry traps within the wooded area. The first female catch occurred during the week of June 12 in both the blueberry and wooded area in ACV traps that caught 2 and 29 individuals respectively. The following week of June 19 the ACV traps in the blueberry field and wooded area had their first male SWD catches with 15 and 21 individuals. A single female was identified on a dry trap during the week of July 10 in the wooded area. No females were identified from dry traps within the blueberry field over the entire course of trapping.
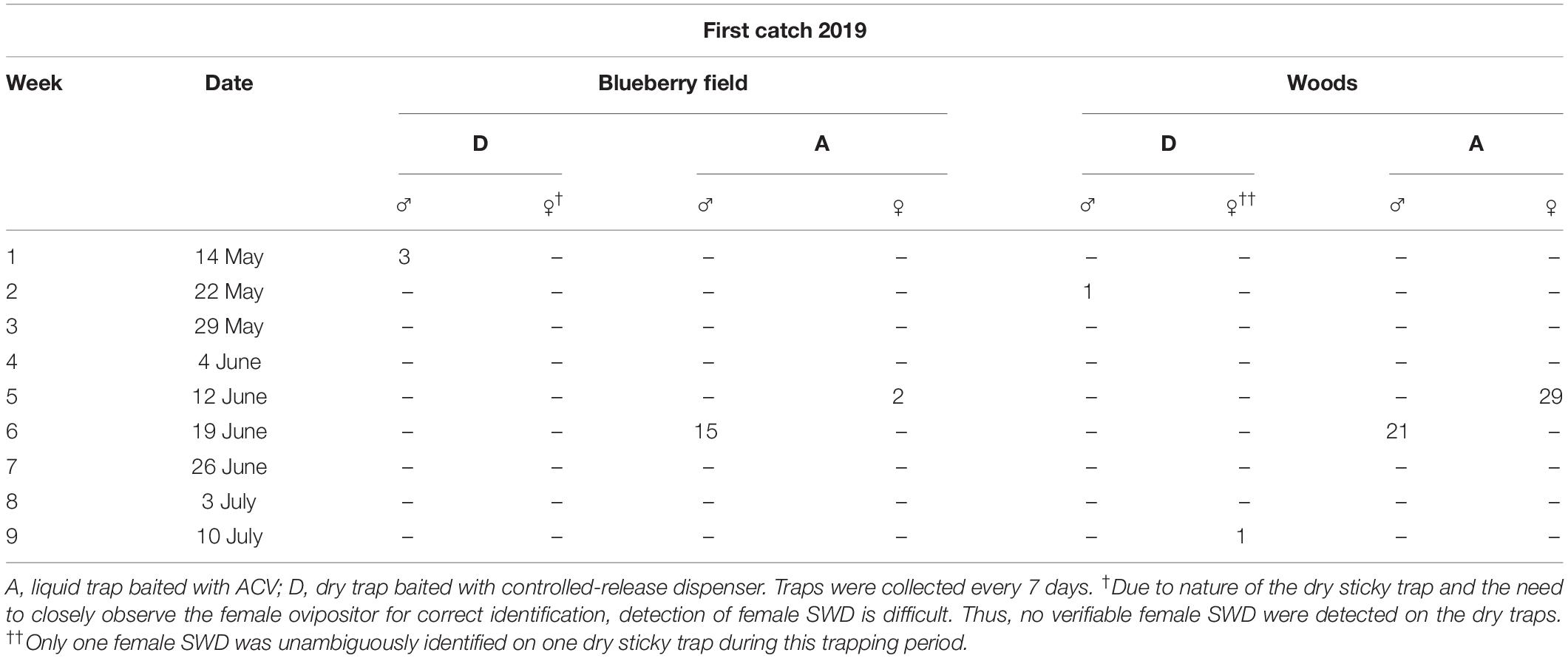
Table 5. Week, date, and number of males and females of first catch of D. suzukii by different trapping systems within a blueberry field and a nearby wooded area at beginning of trapping season in 2019.
The mean catch for 8 weeks of trapping during the harvest period in 2019 within the blueberry field and the nearby wooded area is shown in Table 6. A traps, within the blueberry field caught on average 36.7 ± 11.9 SWD females and 28.8 ± 10 males per trap, while D traps caught 0 SWD females and 6.1 ± 1.6 males per trap and C traps caught 26.3 ± 6.2 females and 27.5 ± 8. A traps within the wooded area caught on average 163.8 ± 34.4 female SWD and 223.9 ± 46.9 male SWD, D traps caught 0.03 ± 0.03 female SWD and 18.4 ± 3.3 male SWD on average, and C traps caught 86.6 ± 16.1 and 162.7 ± 22.1 females and males respectively. A traps within both the blueberry field and wooded area caught significantly more non-target Drosophila spp., 165.6 ± 26.8 and 1322 ± 212 respectively, than D traps, 83.4 ± 17.3 and 214.1 ± 4.8 respectively, and C traps, 54.7 ± 10.7 and 171.9 ± 17.6 respectively. C traps caught significantly fewer large flies than both A traps and D traps within the blueberry field. A traps caught 14.6 ± 3.8 while D traps caught 20.4 ± 5.7, conversely C traps caught on average only 0.47 ± 0.24 large flies. Within the wooded area A traps caught significantly more large flies, 17.1 ± 4.8, than both D traps, 5 ± 1.1 and C traps 0.4 ± 0.21. There was no significant difference in the average number of other arthropods that was caught by A and D traps within the blueberry field, however C traps caught significantly fewer other arthropods. The average number of other arthropods caught in A traps in the blueberry field was 111.2 ± 13.3, with D traps catching 96.4 ± 13.5, and C traps catching 27.5 ± 3.5. The wooded area A traps caught 131.8 ± 12.5 compared to 104.3 ± 11.4 caught by D traps, and 89.9 ± 20.4 caught in C traps. A single female SWD was unambiguously identified on one dry sticky trap in the wooded area during this trapping period (Table 6). It is likely that this female SWD had been recently caught (it was not completely desiccated) and identification was performed easily as the ovipositor was protruding out from the abdomen.
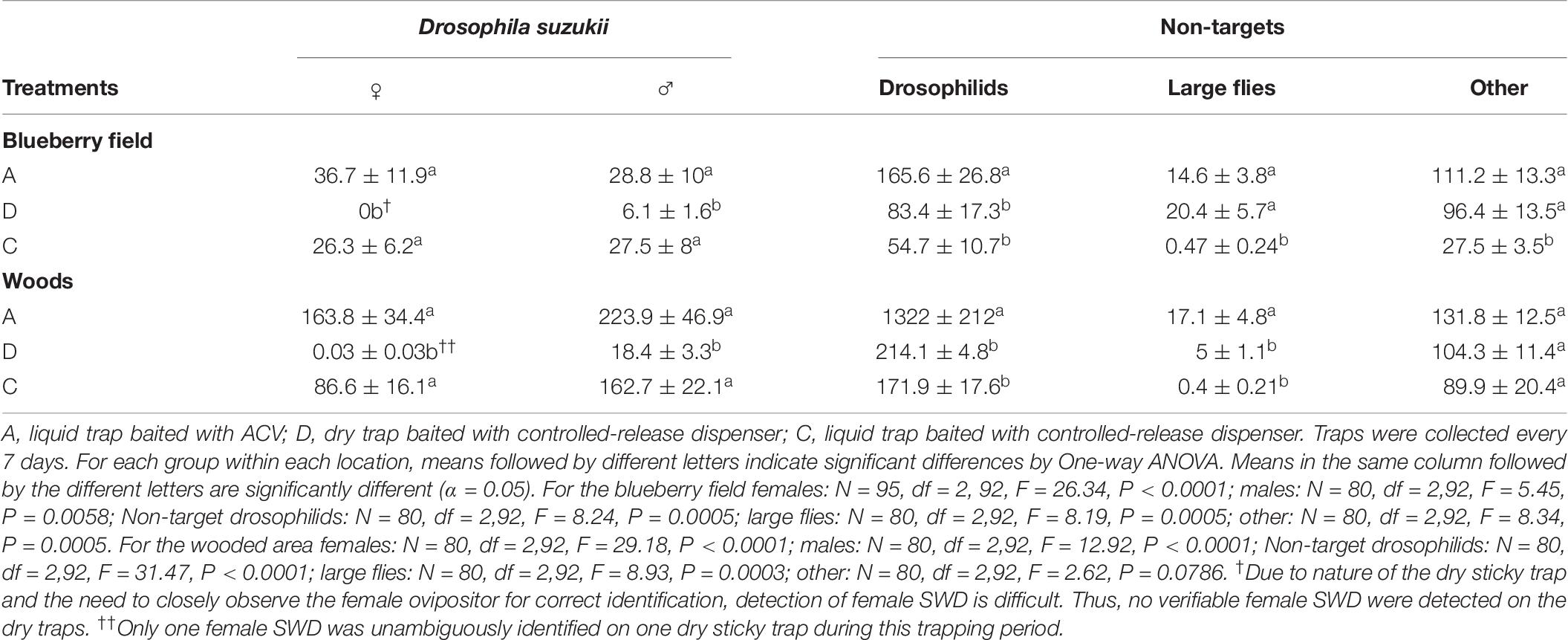
Table 6. Mean (±SE) catch (per trap) of female, male, other drosophilids, large flies, and other non-target arthropods over the course of 8-weeks (6/4 to 7/24) during blueberry harvest in 2019.
For the period of the blueberry harvest in 2019 the average percent selectivity of the traps is shown in Table 7. For the first 2 weeks into harvest all traps within the blueberry filed and wooded area caught no SWD. Selectivity increased in the third week for both the A traps and D traps in the blueberry field to 0.72 ± 0.21 and 0.18 ± 0.18 respectively. The traps in the wooded area additionally saw an increase in selectivity with A traps showing an average selectivity of 0.17 ± 0.09, and D traps showing an average selectivity of 0.05 ± 0.05. During the fourth week of harvest liquid traps containing the same bag formulation as the D sticky traps were added to the field and wooded area. During this trapping week selectivity, within the blueberry field, for the A traps increased slightly to 1.7 ± 0.26, while the selectivity of the D traps increased ca. 173-fold to 31.2 ± 13.8. The liquid C traps started the fourth week with an average selectivity of 31.4 ± 5.3, however, this value was not significantly different from either the A or D trap selectivity values. Selectivity within the wooded area for the fourth week also increased. A trap selectivity increased ca. 26-fold to 4.4 ± 1.5, D trap selectivity increased ca. 320-fold to 16 ± 10, and the C trap selectivity was shown to be 33.7 ± 3.2. C trap selectivity was significantly different from A trap selectivity but not D trap selectivity. Average selectivity for A traps in the blueberry filed increased slightly to 3.6 ± 1.4, while both D and C traps saw selectivity drop to 12.2 ± 3.3 and 19.9 ± 0.31 respectively in the fifth week of trapping. A similar trend was seen in the wooded area with A trap selectivity increasing to 11.2 ± 2.8, while D and C trap selectivity decreased to 3 ± 1 and 16.2 ± 3.7. Selectivity for all traps in both the blueberry field and wooded area increased during the sixth week of trapping. Average selectivity for A traps within the blueberry field increased 9-fold to 32.8 ± 7.3, while D and C traps increased ca. 2.7 and 2.8-fold to 33 ± 15 and 55 ± 6.1 respectively. Traps within the wooded area saw an increase in A trap selectivity to 17.8 ± 2.3, while D and C trap selectivity increased to 22.6 ± 4.4 and 47.6 ± 0.97. During the seventh week of trapping during harvest selectivity once again dropped within the blueberry field. Interestingly for each type of trap selectivity dropped ca. 3-fold to 10.5 ± 2.1, 11.6 ± 2.4, and 17.7 ± 5.7 for A, D, and C traps respectively. In contrast the wooded area trap selectivity did not decrease, instead it increased. Selectivity for A traps approximately doubled to 39.7 ± 4.5, and D and C trap selectivity increased by about 1.3-fold to 30.2 ± 2 and 64.7 ± 3.7 respectively. In the final week of trapping during the harvest period A trap selectivity within the blueberry field slightly decreased to 9.8 ± 2.9, D trap selectivity increased slightly to 12 ± 3.4, and C trap selectivity increased ca. 2.7-fold to 47.5 ± 2.7. The selectivity A traps within the wooded area slightly increased to 42.5 ± 4.5, while D trap selectivity slightly decreased to 29.5 ± 5.6. C trap selectivity increased to its highest value of 72.5 ± 1.6.
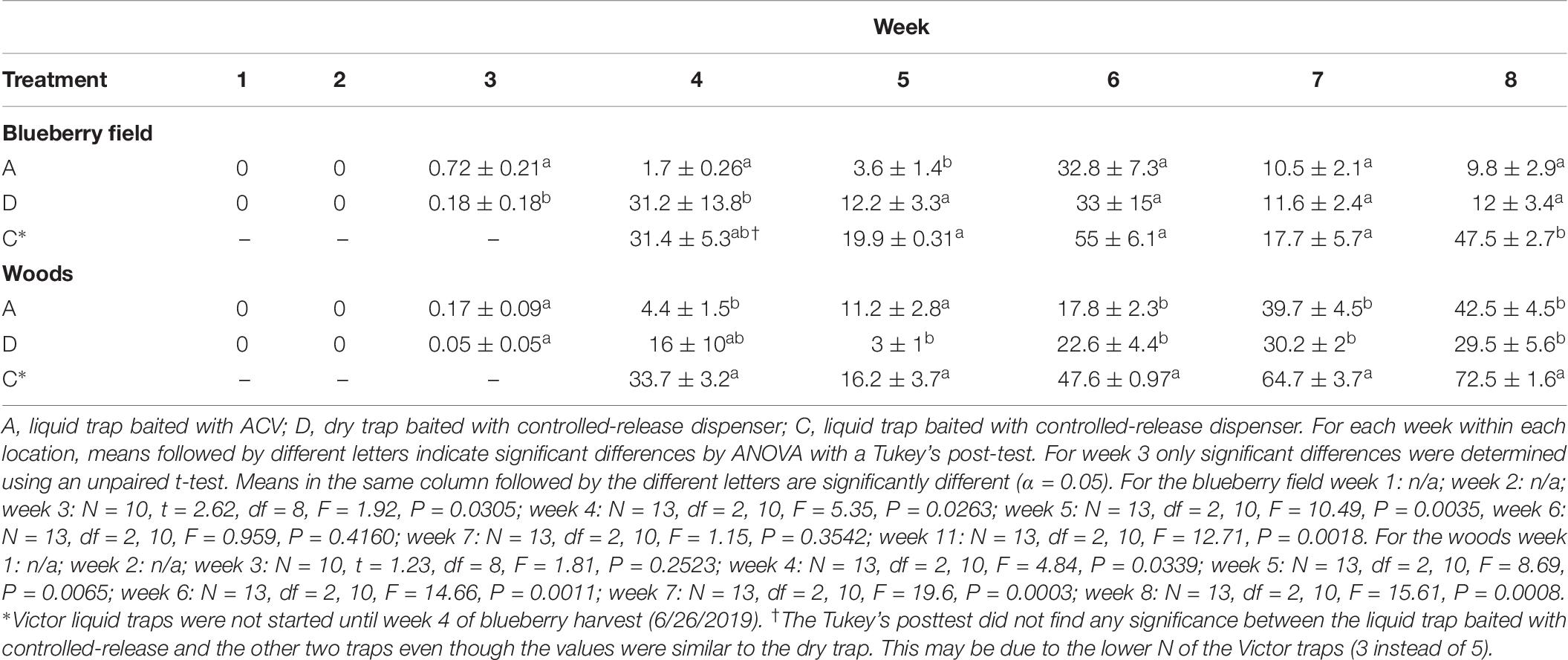
Table 7. Mean (±SE) percent selectivity per trap for weeks 1–8 (6/4 to 7/24), during blueberry harvest for 2019.
Discussion
Although some commercial dispensers, that can release volatile chemicals with constant rates have been developed (McDonough et al., 1992; Cross et al., 2006; Hebert et al., 2007; Evenden and Gries, 2010), the most common semiochemical release devices used by researchers, e.g., rubber septa (Zhang et al., 2013), laminates flakes (Kehat et al., 1983), microcapsules (Light and Beck, 2010), polyethylene vials (Zhang et al., 2008), PVC-resins (Cork et al., 2008), sepiolite-based tablets (Femenia-Ferrer et al., 2007), wax-base granules (Behle et al., 2008), and plastic ropes (Mayer and Mitchell, 1998), are first-order matrices that release with an initial burst and then decline gradually over time. Dispensers with zero-order kinetics are perceived to be beneficial in many situations but are more difficult to achieve in the laboratory. We have demonstrated that the controlled-release sachets are easily prepared from polyethylene tubing and polyester felt strip, in which the polyethylene film functioned as a volatile releasing surface and felt served as a carrier or reservoir for the volatile chemicals. For most of the compounds, the polyethylene controlled-release sachets diffused significantly large amounts of chemical volatiles into the environment over a certain time at constant rates (zero-kinetics). Additionally, release rates of chemical could be altered by changing the thickness of the polyethylene tubing. For example, when three synergistic volatile components, ethyl acetate, acetic acid and phenethyl alcohol, were added directly into the drowning solution in the liquid trap baited with the AT/EO uncontrolled release dispenser, determining how long the three chemicals will last, as they are released into the surroundings, is difficult in either lab or field conditions. However, the ethyl acetate sachet with 5 mL loading lasts at least for 2 weeks using 6 Mil film thickness of polyethylene tubing at ∼0.3 g/day rate in laboratory conditions. It is the same for phenethyl alcohol (1 mL loading) and acetic acid (5 mL loading), releases could be controlled at ∼0.0054 g/day to ∼0.0023 g/day rates and ∼0.13 g/day to ∼0.051 g/day rates, respectively, over 36 days by using 2, 3, 4, or 6 Mil thickness of polyethylene sachets.
In contrast, the polyethylene controlled-release sachets did not always release the AT/EO blend at constant rates (zero-kinetics). Release of this blend with 1 mL loading followed first-order kinetics regardless of the film thickness. However, it diffused at close to constant rates from 3, 4, and 6 Mil thickness of polyethylene sachets with 2 mL loading (zero-order release). This indicates that the release kinetics, of the controlled-release polyethylene sachet, not only relied on the film thickness and chemical volatility at the same physical conditions, e.g., temperature, wind velocity and dispenser surface area, but it also depended on amount of content loading.
During the 2018 and 2019 harvest trapping season, several tests were conducted to determine whether the lure synergists (AA, EA, and PE) and attractants (AT/EO) (Feng et al., 2018) could be improved through the alteration of the lure dispenser (sachet vs. centrifuge tube) and the type of trap used (sticky trap vs. liquid bait trap). Results show that there was no significant difference between the two lure dispensers in average catch of any of the measured groups (Tab. 3) within the blueberry field. However, there was a significant reduction in non-target drosophilid catch in the controlled-release lure traps placed within the wooded area, with prior research addressing the need for this type of reduction in non-target catch (Kirkpatrick et al., 2017). In addition to altering the type of dispenser tested (controlled- vs. uncontrolled-release) the thickness of the sachets used to contain the attractants, AT/EO, were varied. This was done to test whether the release rates of the attractant compounds had any measurable effect on trap efficacy and to extend the duration of the lure. Our data indicate that there were no significant differences found in the average weekly catch of SWD or in the selectivity between the traps utilizing the variously sized sachets (Figure 3).
Dependent upon the goal of the grower/user of the trap system both efficacy and selectivity may be important factors for consideration. A trapping system with high efficacy could be utilized to trap out or mass trap the target pest (Landolt et al., 2012). Our data has shown that efficacy is dependent on trap type (Figures 4, 5). Within the blueberry field, during the 2019 8-week harvest trapping period the controlled released lure attached to the sticky trap caught the lowest average SWD per trap compared to the liquid based traps containing either ACV or the controlled release lure. It should be noted that the sticky trap average value was not significantly different from the value for the controlled release rate liquid trap, though this may be due to lower replicate numbers for the liquid based trap. Achieving high selectivity is important to not only avoid harming the beneficial insect population in a trapping area but also to avoid several other factors that can reduce the efficiency of trap processing. A large proportion of non-target species can complicate target pest identification resulting in increased labor to correctly identify the target (Adams et al., 1989) as well as causing reduced catch from increased decomposing biomass (Weber and Ferro, 1991). Previous work has shown the selectivity of the uncontrolled-release 5-component lure to be ca. 47% (Feng et al., 2018). Here we have shown that average weekly selectivity, for controlled-release 5-component lures, ranges from ca. 41 – 51% (Figure 3), an improvement over the selectivity of yeast bait lures and commercial baits that have been shown to have selectivity ranging from ca. 6–40% (Burrack et al., 2015; Kirkpatrick et al., 2017; Tonina et al., 2018)in raspberries and blueberries. Additionally, selectivity tends to increase over the 8-week trapping period for both years in both the blueberry field and wooded area (Tables 4, 7). This could be due to a decrease in available resources, i.e., blueberries as the harvest period is ending, thus the lures have an increase in attraction.
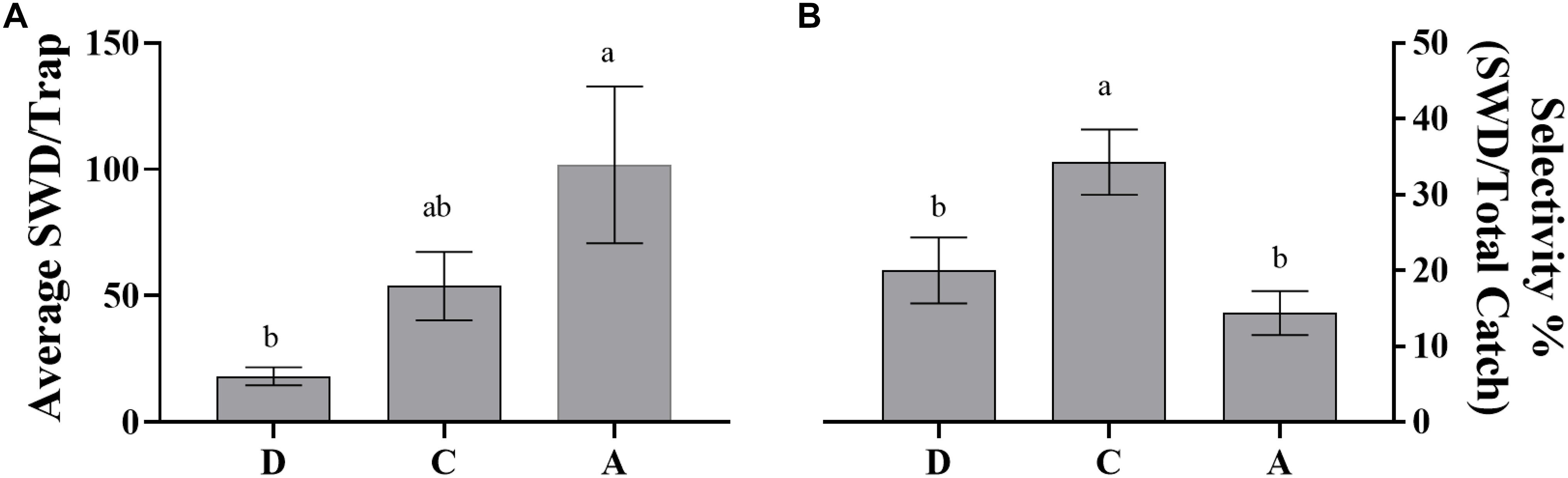
Figure 4. Average SWD catch per trap and overall selectivity over 8 weeks of trapping period during blueberry harvest season in blueberry field in 2019 (6/4 to 7/24) by dry and liquid traps baited with controlled-release dispenser and ACV. (A) Average catch in blueberry field (N = 65, df = 2, 62 F = 7.018, P = 0.0018); (B) selectivity within blueberry field (N = 65, df = 2,62, F = 6.63, P = 0.0025). Means separated by One-way ANOVA with a Tukey’s posttest. Error bars represent standard error. Different letters above bars indicate significant differences at α = 0.05. D: dry trap baited with controlled-release dispenser; dry catch data was adjusted by control dry trap catch; dry trap female SWD catch was estimated through determination of% female catch within liquid trap baited with controlled-release dispenser; C: liquid trap baited with controlled-release dispenser; A: liquid trap baited with ACV. Bigger font for each figure label, small cap letters above error bars. The female SWD catch per trap was estimated by taking the percent average female SWD catch within the liquid trap baited with the SWD controlled-release dispenser.
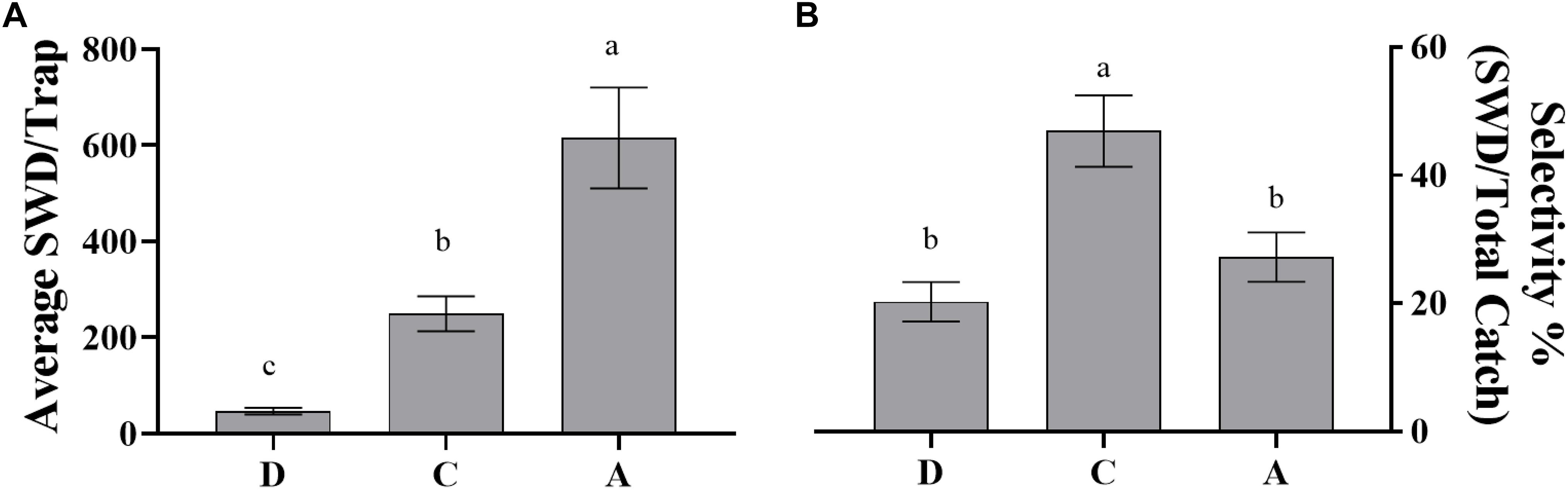
Figure 5. Average SWD catch per trap and overall selectivity over 8 weeks of trapping during blueberry harvest season in wooded area in 2019 (6/4 to 7/24) by dry and liquid traps baited with controlled-release dispenser and ACV. (A) Average catch in blueberry field (N = 65, df = 2, 62, F = 22.59, P < 0.0001); (B) selectivity within blueberry field (N = 65, df = 2, 62, F = 9.686, P = 0.0002). Means separated by One-way ANOVA with a Tukey’s posttest. Error bars represent standard error. Different letters above bars indicate significant differences at α = 0.05. D: dry trap baited with controlled-release dispenser; dry catch data was adjusted by control dry trap catch; dry trap female SWD catch was estimated through determination of % female catch within liquid trap baited with controlled-release dispenser; C: liquid trap baited with controlled-release dispenser, A: liquid trap baited with ACV. The female SWD catch per trap was estimated by taking the percent average female SWD catch within the liquid trap baited with the SWD controlled-release dispenser.
Early detection is another important benchmark for lures as it allows for SWD control to be implemented as soon as possible. Currently, control is implemented at first detection of a single individual (Iglesias et al., 2014), thus development of a lure-trapping system that can provide significant early detection capabilities will benefit growers by allowing them to respond to the detected pest before significant pest incursion has occurred. Both uncontrolled and controlled release dispensers caught SWD females 2 weeks earlier than the standard ACV lure within the blueberry field. The controlled release dispenser also caught SWD males at the same time (Table 2). Interestingly, all three lure types caught SWD females during the first week of trapping within the wooded area. The ACV trap was the only trap type to catch SWD males during this time. The uncontrolled and controlled release traps had their first male catches 1 and 2 weeks after respectively. The quantity that was caught during this time was also greater within the wooded area than what was caught within the blueberry field. This could be due to the density of individuals being greater within the wooded area at the beginning of the harvest season (Tait et al., 2019). Ohrn and Dreves (2011) reported that initial catches of SWD within the monitored field were low while traps in adjacent trees surrounding the field initially caught more and that overtime the trap catches within the monitored field grew as SWD moved in. In the 2019 harvest season the controlled-release lure attached to a sticky trap performed similarly in providing early detection compared to a liquid trap baited with ACV (Table 5). Male SWD were detected 5 weeks earlier than detection by the ACV trap in the blueberry field and 4 weeks earlier in the wooded area. It should be noted that the 2019 trapping study was started 5 weeks earlier than the previous year to test how early SWD could be detected within the blueberry field. Additionally, as can be seen in Table 5, no female SWD were detected for sticky traps in either location. This is likely due to the rapid degradation of the specimens that occurs once an individual is caught (Iglesias et al., 2014). We observed degradation of all specimens on sticky traps, however, the male SWD identifying wing spots were easily visible compared to the need to view the serrated ovipositor for female identification.
In this study, we have demonstrated that the controlled-release dispenser, which was capable of sustainably releasing D. suzukii volatile attractive compounds to the environment at a constant release rate (zero-order kinetics), could be achieved relatively easy and convenient in the laboratory. Application of liquid traps baited with the controlled-release dispenser that can early detect both male and female D. suzukii and exhibit significantly higher selectivity in blueberry growing seasons will assist farmers/growers in timely implementations of action plans for SWD control interventions. Typically, only the male D. suzukii is easily identifiable from dry trap catches, however, a single female SWD had been decidedly identified on a dry sticky trap over the first 8-week trapping period, indicating that it is possible to identify female SWD if the dry trap catch is checked in a timely manner (e.g., on a daily basis). The fact that dry traps require low maintenance and are easily processed, especially when compared to the liquid trap baited with the controlled-release dispenser, indicating that dry traps make improvement of early detection of SWD achievable. These advantages prove that this is an efficient, reliable, and convenient detection tool, which is needed within IPM programs, and will facilitate successful management of D. suzukii in soft-skinned fruit crop orchards/farms.
Data Availability Statement
The datasets generated for this study are available on request to the corresponding author.
Author Contributions
AZ and NL conceived the research idea. AZ performed the laboratory release rate study. NL and JS conducted field bioassay. NL and AZ carried out the data analysis. All authors contributed to the writing and reviewing of the manuscript.
Disclaimer
The findings and conclusions in this preliminary publication have not been formally disseminated by the U.S. Department of Agriculture and should not be construed to represent any Agency determination or policy. Mention of trade names or commercial products in this publication is solely for the purpose of providing specific information and does not imply recommendation or endorsement by the USDA. USDA is an equal opportunity provider and employer.
Conflict of Interest
The authors declare that the research was conducted in the absence of any commercial or financial relationships that could be construed as a potential conflict of interest.
Acknowledgments
The authors greatly acknowledge Mr. Ben Butler, assistant farm manager of Butler’s Orchard, for wiliness to use of their property and valuable help during this study. Thanks also go to Mahalet Nega, Filadelfo Guzman, and Ugochukwu Okeke, Invasive Insect Behavior and Biocontrol Laboratory, USDA, ARS, for assistance in lab and field work.
References
Aabanowska, B. H., and Wojciech, P. (2015). The spotted wing drosophila Drosophila suzukii (Matsumura, 1931) monitoring and first records In Poland. J. Hort Res. 30, 49–57. doi: 10.2478/johr-2015-0020
Abraham, J., Zhang, A., Angeli, S., Abubeker, S., Michel, C., Feng, Y., et al. (2015). Behavioral and antennal responses of spotted wing drosophila, Drosophila suzukii, to volatiles from fruit extracts. Environ. Entomol. 44, 356–367. doi: 10.1093/ee/nvv013
Adams, R. G., Murray, K. D., and Los, L. M. (1989). Effectiveness and selectivity of sex pheromone lures and traps for monitoring fall armyworm (Lepidoptera: Noctuidae) adults in Connecticut sweet corn. J. Econ. Entomol. 82, 285–290. doi: 10.1093/jee/82.1.285
Andreazza, F., Baronio, C. A., Botton, M., Valgas, R. A., Ritschel, P. S., Maia, J. D., et al. (2016). Susceptibility of grape vine genotypes by the infestation of Drosophila suzukii (Diptera: Drosophilidae). Pesqui Agropecu Bras 51, 599–606.
Arno, J., Sola, M., Riudavets, J., and Gabarra, R. (2016). Population dynamics, non-crop hosts, and fruit susceptibility of Drosophila suzukii in Northeast Spain. J. Pest Sci. 89, 713–723. doi: 10.1007/s10340-016-0774-3
Atterholt, C. A., Delwiche, M. J., Rice, R. E., and Krochta, J. M. (1999). Controlled release of insect sex pheromones from paraffin wax and emulsions. J. Control Release 57, 233–247. doi: 10.1016/s0168-3659(98)00119-9
Behle, R. W., Cosse, A. A., Dunlap, C., Fisher, J., and Koppenhoffer, A. M. (2008). Developing wax-based granule formulations for mating disruption of oriental beetles (Coleoptera: Scarabaeidae) in turfgrass. J. Econ. Entomol. 101, 1856–1863. doi: 10.1603/0022-0493-101.6.1856
Belien, T., Thys, T., Fassotte, C., Walrant, C., Tomme, M., Bolen, M., et al. (2014). Population dynamics of Drosophila Suzukii (Diptera: Drosophilidae) in Belgium in 2013. Commun. Agric. Appl. Biol. Sci. 79, 169–175.
Bradley, S. J., Suckling, D. M., McNaughon, K. G., Wearing, C. H., and Karg, G. (1995). A temperature-dependent model for predicting release rates of pheromone from a polyethylene tubing dispenser. J. Chem. Ecol. 21, 745–760. doi: 10.1007/bf02033458
Briem, F., Breuer, M., Koppler, K., and Vogt, H. (2015). Phenology and occurrence of spotted wing drosophila in Germany and case studies for its control in berry crops. IOBC/WPRS Bull. 109, 233–237.
Briem, F., Dominic, A. R., Golla, B., Hoffmann, C., Englert, C., Herz, A., et al. (2018). Explorative data analysis of Drosophila suzukii trap catches from a seven-year monitoring program in Southwest Germany. Insects 9:E125. doi: 10.3390/insects9040125
Burrack, H. J., Asplen, M., Bahder, L., Collins, J., Drummond, F. A., Guedot, C., et al. (2015). Multistate comparison of attractants for monitoring Drosophila suzukii (Diptera: Drosophilidae) in blueberries and cranberries. Environ. Entomol. 44, 704–712. doi: 10.1093/ee/nvv022
Calabria, G., Maca, J., Bachli, G., Serra, L., and Pascual, M. (2012). First records of the potential pest species Drosophila suzukii (Diptera: Drosophilidae) in Europe. J. Appl. Entomol. 136, 139–147. doi: 10.1111/j.1439-0418.2010.01583.x
Cha, D., Adams, T., Rogg, H., and Landolt, P. (2012). Identification and field evaluation of fermentation volatiles from wine and vinegar that mediate attraction of spotted wing drosophila, Drosophila suzukii. J. Chem. Ecol. 38, 1419–1431. doi: 10.1007/s10886-012-0196-5
Cha, D. H., Hesler, S. P., Park, S., Adams, T. B., Zack, R. S., Rogg, H., et al. (2015). Simpler is better: fewer non-target insects trapped with a four-component chemical lure vs. a chemically more complex food-type bait for Drosophila suzukii. Entomol. Exp. Appl. 154, 251–260. doi: 10.1111/eea.12276
Cini, A., Anfora, G., Escudero-Colomar, L. A., Grassi, A., Santosuosso, U., Seljak, G., et al. (2014). Tracking the invasion of the alien fruit pest Drosophila suzukii in Europe. J. Pest Sci. 87, 559–566. doi: 10.1007/s10340-014-0617-z
Cini, A., Ioriatti, C., and Anfora, G. (2012). A review of the invasion of Drosophila suzukii in Europe and a draft research agenda for integrated pest management. Bull. Insectol. 65, 149–160.
Cork, A., De Souza, K., Hall, D. R., Jones, O. T., Casagrande, E., Krishnaiah, K., et al. (2008). Development of PVC-resin-controlled release formulation for pheromones and use in mating disruption of yellow rice stem borer, Scirpophaga incertulas. Crop Protect. 27, 248–255. doi: 10.1016/j.cropro.2007.05.011
Cross, J. V., Hesketh, H., Jay, C. N., Hall, D. R., Innocenzi, P. J., Farman, D. I., et al. (2006). Exploiting the aggregation pheromone of strawberry blossom weevil Anthonomus rubi Herbst (Coleoptera: Curculionidae). 1. Development of lure and trap. Crop Protect. 25, 144–154. doi: 10.1016/j.cropro.2005.04.002
Cuthbertson, A. G., Collins, D. A., Blackburn, L. F., Audsley, N., and Bell, H. A. (2014). Preliminary screening of potential control products against Drosophila suzukii. Insects 5, 488–498. doi: 10.3390/insects5020488
Dancau, T., Stemberger, T. L. M., Clarke, P., and Gillespie, D. R. (2017). Can competition be superior to parasitism for biological control? The case of spotted wing Drosophila (Drosophila suzukii), Drosophila melanogaster and Pachycrepoideus vindemmiae. Biocontrol. Sci. Technol. 27, 3–16. doi: 10.1080/09583157.2016.1241982
Depra, M., Poppe, J. L., Schmitz, H. J., De Toni, D. C., and Valente, V. L. S. (2014). The first records of the invasive pest Drosophila suzukii in the South American continent. J. Pest Sci. 87, 379–383. doi: 10.1007/s10340-014-0591-5
Evenden, M. L., and Gries, R. (2010). Assessment of commercially available pheromone lures for monitoring diamondback moth (Lepidoptera: Plutellidae) in Canola. J. Econ. Entomol. 103, 654–661. doi: 10.1603/ec09339
Farnsworth, D., Hamby, K. A., Bolda, M., Goodhue, R. E., Williams, J. C., and Zalom, F. G. (2017). Economic analysis of revenue losses and control costs associated with the spotted wing drosophila, Drosophila suzukii (Matsumura), in the California raspberry industry. Pest Manage. Sci. 73, 1083–1090. doi: 10.1002/ps.4497
Femenia-Ferrer, B., Bosch, D., Moya, P., Avilla, J., Primo, J., Cross, J., et al. (2007). Field assays of new biodegradable controlled-release pheromone dispensers for mating disruption of Cydia pomonella (L.). Bull. Oilb/Srop 30, 107–114.
Feng, Y., Bruton, R., Park, A., and Zhang, A. (2018). Identification of attractive blend for spotted wing drosophila, Drosophila suzukii, from apple juice. J. Pest. Sci. 91, 1251–1267. doi: 10.1007/s10340-018-1006-9
Gabarra, R., Riudavets, J., Rodriguez, G. A., Pujade-Villar, J., and Arno, J. (2015). Prospects for the biological control of Drosophila suzukii. BioControl 60, 331–339. doi: 10.1007/s10526-014-9646-z
Girod, P., Lierhmann, O., Urvois, T., Kenis, M., Haye, T., and Turlings, T. C. J. (2018). Host specificity of Asian parasitoids for potential classical biological control of Drosophila suzukii. J. Pest Sci. 91, 1241–1250. doi: 10.1007/s10340-018-1003-z
Goodhue, R. E., Farnsworth, D., Williams, J. C., Bolda, M., and Zalom, F. G. (2011). Spotted wing drosophila infestation of California strawberries and raspberries: economic analysis of potential revenue losses and control costs. Pest Manage. Sci. 67, 1396–1402. doi: 10.1002/ps.2259
Hamby, K. A., Bellamy, D. E., Chiu, J. C., Lee, J. C., Walton, V. M., York, R. M., et al. (2016). Biotic and abiotic factors impacting development, behavior, phenology, and reproductive biology of Drosophila suzukii. J. Pest. Sci. 89, 605–619. doi: 10.1007/s10340-016-0756-5
Hamby, K. A., Bolda, M. P., Sheehan, M. E., and Zalom, F. G. (2014). Seasonal monitoring for Drosophila suzukii (Diptera: Drosophilidae) in California commercial raspberries. Environ. Entomol. 43, 1008–1018. doi: 10.1603/en13245
Hampton, E., Koski, C., Barsoian, O., Faubert, H., Cowles, R. S., and Alm, S. R. (2014). Use of early ripening cultivars to avoid infestation and mass trapping to manage Drosophila suzukii (Diptera: Drosophilidae) in Vaccinium corymbosum (Ericales: Ericaceae). J. Econ. Entomol. 107, 1849–1857. doi: 10.1603/ec14232
Hauser, M. (2011). A historic account of the invasion of Drosophila suzukii (Matsumura) (Diptera: Drosophilidae) in the continental United States, with remarks on their identification. Pest Manage. Sci. 67, 1352–1357. doi: 10.1002/ps.2265
Hebert, V. R., Tomaszewska, E., Brunner, J. F., Jones, V. P., Doerr, M., and Scopus, C. (2007). Evaluating the pheromone release rate characteristics of commercial mating disruption devices. ACS Symp. Ser. 947, 144–157. doi: 10.1021/bk-2007-0947.ch010
Heuskin, S., Verheggen, F. J., Haubruge, E., Wathelet, J. P., and Lognay, G. (2011). The use of semiochemical slow-release devices in integrated pest management strategies. Biotechnol. Agron. Soc. Environ. 15, 459–470.
Iglesias, L. E., Nyoike, T. W., and Liburd, O. E. (2014). Effect of trap design, bait type, and age on captures of Drosophila suzukii (Diptera: Drosophilidae) in berry crops. J. Econ. Entomol. 107, 1508–1518. doi: 10.1603/ec13538
Jaffe, B. D., Avanesyan, A., Bal, H. K., Feng, Y., Grant, J., Grieshop, M. J., et al. (2018). Multistate comparison of attractants and the impact of fruit development stage on trapping Drosophila suzukii (Diptera: Drosophilidae) in raspberry and blueberry. Environ. Entomol. 47, 935–945. doi: 10.1093/ee/nvy052
Kehat, M., Dunkelblum, E., and Gothilf, S. (1983). Mating disruption of the cotton leafworm, Spodoptera littoralis (Lepidoptera: Noctuidae), by release of sex pheromone from widely separated Hercon-laminated dispensers. Environ. Entomol. 12, 1265–1269. doi: 10.1093/ee/12.4.1265
Kirkpatrick, D. M., Gut, L. J., and Miller, J. R. (2018). Development of a novel dry, sticky trap design incorporating visual cues for Drosophila suzukii (Diptera: Drosophilidae). J. Econ. Entomol. 111, 1775–1779. doi: 10.1093/jee/toy097
Kirkpatrick, D. M., McGhee, P. S., Gut, L. J., and Miller, J. R. (2017). Improving monitoring tools for spotted wing drosophila, Drosophila suzukii. Entomol. Exp. Appl. 164, 87–93. doi: 10.1111/eea.12602
Kirkpatrick, D. M., McGhee, P. S., Hermann, S. L., Gut, L. J., and Miller, J. R. (2016). Alightment of spotted wing drosophila (diptera: Drosophilidae) on odorless disks varying in color. Environ. Entomol. 45, 185–191. doi: 10.1093/ee/nvv155
Kleiber, J. R., Unelius, C. R., Suckling, D. M., Lee, J. C., Qian, M. C., and Bruck, D. J. (2014). Attractiveness of fermentation and related products to spotted wing Drosophila (Diptera: Drosophilidae). Environ. Entomol. 43, 439–447. doi: 10.1603/en13224
Klick, J., Yang, W. Q., Lee, J. C., and Bruck, D. J. (2016). Reduced spray programs for Drosophila suzukii management in berry crops. Int. J. Pest Manage. 62, 368–377. doi: 10.1080/09670874.2016.1222105
Knapp, L., Mazzi, D., and Finger, R. (2019). Management strategies against Drosophila suzukii: insights into Swiss grape growers choices. Pest Manage. Sci. 75, 2820–2829. doi: 10.1002/ps.5397
Landolt, P. J., Adams, T., and Rogg, H. (2012). Trapping spotted wing drosophila, Drosophila suzukii (Matsumura) (Diptera: Drosophilidae), with combinations of vinegar and wine, and acetic acid and ethanol. J. Appl. Entomol. 136, 148–154. doi: 10.1111/j.1439-0418.2011.01646.x
Lavagnino, N. J., Diaz, B. M., Cichon, L., De La Vega, G. J., Garrido, S. A., Lago, J. D., et al. (2018). New records of the invasive pest Drosophila suzukii (Matsumura) (Diptera: Drosophilidae) in the South American continent. Rev. Soc. Entomol. Argent. 77, 27–31. doi: 10.25085/rsea.770105
Lee, J. C., Bruck, D. J., Curry, H., Edwards, D., Haviland, D. R., Van Steenwyk, R. A., et al. (2011). The susceptibility of small fruits and cherries to the spotted-wing drosophila, Drosophila suzukii. Pest Manage. Sci. 67, 1358–1367. doi: 10.1002/ps.2225
Lee, J. C., Burrack, H. J., Barrantes, L. D., Beers, E. H., Dreves, A. J., Hamby, K. A., et al. (2012). Evaluation of monitoring traps for Drosophila suzukii (Diptera: Drosophilidae) in North America. J. Econ. Entomol. 105, 1350–1357. doi: 10.1603/ec12132
Lee, J. C., Shearer, P. W., Barrantes, L. D., Walsh, D. B., Beers, E. H., Burrack, H. J., et al. (2013). Trap designs for monitoring Drosophila suzukii (Diptera: Drosophilidae). Environ. Entomol. 42, 1348–1355.
Lethmayer, C., and Egartner, A. (2017). Enhancement of Drosophila suzukii trapping. IOBC/WPRS Bull. 123, 143–149.
Light, D. M., and Beck, J. J. (2010). Characterization of microencapsulated pear ester, (2E,4Z)-ethyl-2,4-decadienoate, a kairomonal spray adjuvant against neonate codling moth larvae. J. Agric. Food Chem. 58, 7838–7845. doi: 10.1021/jf101167p
Matsuura, A., Mitsui, H., and Kimura, M. T. (2018). A preliminary study on distributions and oviposition sites of Drosophila suzukii (Diptera: Drosophilidae) and its parasitoids on wild cherry tree in Tokyo, central Japan. Appl. Entomol. Zool. 53, 47–53. doi: 10.1007/s13355-017-0527-7
Mayer, M. S., and Mitchell, E. R. (1998). Rapid measure of sex pheromone emission from plastic rope dispensers: example of utility in sexual communication disruption of the diamondback moth, Plutella xylostella. Phytoparasitica 26, 117–125. doi: 10.1007/bf02980678
Mazzetto, F., Gonella, E., Pontini, M., Alma, A., Crotti, E., Vacchini, V., et al. (2016). Olfactory attraction of Drosophila suzukii by symbiotic acetic acid bacteria. J. Pest Sci. 89, 783–792. doi: 10.1007/s10340-016-0754-7
Mazzetto, F., Pansa, M. G., Ingegno, B. L., Tavella, L., and Alma, A. (2015). Monitoring of the exotic fly Drosophila suzukii in stone, pome and soft fruit orchards in NW Italy. J. Asia-Pacif Entomol. 18, 321–329. doi: 10.1016/j.aspen.2015.04.001
McDonough, L. M., Aller, W. C., and Knight, A. L. (1992). Performance characteristics of a commercial controlled-release dispenser of sex pheromone for control of codling moth (Cydia pomonella) by mating disruption. J. Chem. Ecol. 18, 2177–2189. doi: 10.1007/bf00984945
Murali-Baskaran, R. K., Sharma, K. C., Kaushal, P., Kumar, J., Parthiban, P., Senthil-Nathan, S., et al. (2018). Role of kairomone in biological control of crop pests – A review. Physiol. Mol. Plant Pathol. 101, 3–15.
Ohrn, A., and Dreves, A. J. (2011). “Using landscape ecology to inform spotted wing drosophila management practices, in Proceedings of the Research Reports 71st Annual Pacific Northwest insect management conference, January 9th and 10th Hilton Hotel, Portland Oregon.
Pajares, J. A., Alvarez, G., Ibeas, F., Gallego, D., Hall, D. R., and Farman, D. I. (2010). Identification and field activity of a male-produced aggregation pheromone in the pine sawyer beetle, Monochamus galloprovincialis. J. Chem. Ecol. 36, 570–583. doi: 10.1007/s10886-010-9791-5
Poyet, M., Eslin, P., Heraude, M., Le Roux, V., Prevost, G., Gibert, P., et al. (2014). Invasive host for invasive pest: when the Asiatic cherry fly (Drosophila suzukii) meets the American black cherry (Prunus serotina) in Europe. Agric. For. Entomol. 16, 251–259. doi: 10.1111/afe.12052
Radonjic, S., and Hrncic, S. (2015). First record of spotted wing drosophila Drosophila suzukii (Diptera: Drosophilidae) in Montenegro. Pesticides Phytomed. 30, 35–40. doi: 10.2298/pif1501035r
Renkema, J. M., Buitenhuis, R., and Hallett, R. H. (2014). Optimizing trap design and trapping protocols for Drosophila suzukii (Diptera: Drosophilidae). J. Econ. Entomol. 107, 2107–2118. doi: 10.1603/ec14254
Renkema, J. M., and Cuthbertson, A. G. S. (2018). Impact of multiple natural enemies on immature Drosophila suzukii in strawberries and blueberries. BioControl 63, 719–728. doi: 10.1007/s10526-018-9874-8
Rice, K. B., Short, B. D., and Leskey, T. C. (2017). Development of an attract-and-kill strategy for Drosophila suzukii (Diptera: Drosophilidae): evaluation of attracticidal spheres under laboratory and field conditions. J. Econ. Entomol. 110, 535–542. doi: 10.1093/jee/tow319
Tait, G., Cabianca, A., Grassi, A., Pfab, F., Oppedisano, T., Puppato, S., et al. (2019). Drosophila suzukii daily dispersal between distinctly different habitats. Entomol. Gen. doi: 10.1127/entomologia/2019/0876
Tonina, L., Mori, N., Giomi, F., Grassi, A., Gottardello, A., Anfora, G., et al. (2018). Comparison of attractants for monitoring Drosophila suzukii in sweet cherry orchards in Italy. J. Appl. Entomol. 142, 18–25. doi: 10.1111/jen.12416
Wallingford, A. K., Connelly, H. L., Brind’Amour, G. D., Boucher, M. T., Mafra-Neto, A., and Loeb, G. M. (2016). Field evaluation of an oviposition deterrent for management of spotted-wing drosophila, Drosophila suzukii, and potential nontarget effects. J. Econ. Entomol. 109, 1779–1784. doi: 10.1093/jee/tow116
Walsh, D. B., Bolda, M. P., Goodhue, R. E., Dreves, A. J., Lee, J., Bruck, D. J., et al. (2011). Drosophila suzukii (Diptera: Drosophilidae): invasive pest of ripening soft fruit expanding its geographic range and damage potential. J. Integr. Pest Manage. 2, G1–G7.
Weatherston, I., Miller, D., and Dohse, L. (1985). Capillaries as controlled release devices for insect pheromones and other volatile substances-A reevaluation - Part I. Kinetics and development of predictive model for glass capillaries. J. Chem. Ecol. 11, 953–965. doi: 10.1007/bf01020666
Weber, D. C., and Ferro, D. N. (1991). Nontarget noctuids complicate integrated pest management monitoring of sweet corn with pheromone traps in Massachusetts. J. Econ. Entomol. 84, 1364–1369. doi: 10.1093/jee/84.4.1364
Wolf, S., Romeis, J., Collatz, J., Zeisler, C., Sint, D., and Traugott, M. (2018). A simple and cost-effective molecular method to track predation on Drosophila suzukii in the field. J. Pest Sci. 91, 927–935. doi: 10.1007/s10340-017-0948-7
Woltz, J. M., Donahue, K. M., Bruck, D. J., and Lee, J. C. (2015). Efficacy of commercially available predators, nematodes and fungal entomopathogens for augmentative control of Drosophila suzukii. J. Appl. Entomol. 139, 759–770. doi: 10.1111/jen.12200
Yousef, M., Aranda-Valera, E., and Quesada-Moraga, E. (2018). Lure-and-infect and lure-and-kill devices based on Metarhizium brunneum for spotted wing drosophila control. J. Pest Sci. 91, 227–235. doi: 10.1007/s10340-017-0874-8
Zhang, A. (2005). “Laboratory evaluation of semiochemical dispensers: a theoretical chemical kinetic approach to the emission rate of disparlure active ingredient,” in Proceedings, 16th US Department of Agriculture interagency Research forum on Gypsy Moth and Other Invasive Species 2005. Annapolis, MD; 2005 January 18-21, Gen. Tech. Rep. NE-337, ed. K. W. Gottschalk (Newtown Square, PA: U.S. Department of Agriculture, Forest Service, Northeastern Research Station).
Zhang, A., and Feng, Y. (2019). Methods of attracting Drosophila suzukii using acetoin blend. Patent Number US 2019/002319 A1. Jan. 24.
Zhang, A., Kuang, L. F., Maisin, N., Karumuru, B., Hall, D. R., Virdiana, I., et al. (2008). Activity evaluation of cocoa pod borer sex pheromone in cacao fields. Environ. Entomol. 37, 719–724. doi: 10.1603/0046-225x(2008)37
Keywords: spotted wing drosophila, host plant volatiles, kairomone, formulation, release rate, pest management
Citation: Larson NR, Strickland J, Shields VDC and Zhang A (2020) Controlled-Release Dispenser and Dry Trap Developments for Drosophila suzukii Detection. Front. Ecol. Evol. 8:45. doi: 10.3389/fevo.2020.00045
Received: 05 December 2019; Accepted: 12 February 2020;
Published: 06 March 2020.
Edited by:
Sebastien Lebreton, Aix-Marseille Université, FranceReviewed by:
Sergio Angeli, Free University of Bozen-Bolzano, ItalyGianfranco Anfora, Fondazione Edmund Mach, Italy
Copyright © 2020 Larson, Strickland, Shields and Zhang. This is an open-access article distributed under the terms of the Creative Commons Attribution License (CC BY). The use, distribution or reproduction in other forums is permitted, provided the original author(s) and the copyright owner(s) are credited and that the original publication in this journal is cited, in accordance with accepted academic practice. No use, distribution or reproduction is permitted which does not comply with these terms.
*Correspondence: Aijun Zhang, aijun.zhang@ars.usda.gov