- Sustainable Farms, Fenner School of Environment and Society, The Australian National University, Canberra, ACT, Australia
Millions of hectares of land worldwide is in urgent need of restoration. However, many past landscape and ecosystem restoration efforts are likely to have been less than effective. Some of the reasons for these problems include a lack of monitoring, or being subjected to poor quality monitoring practices, or that the restoration efforts are too often not linked with ecological concepts and/or theory. These deficiencies make it difficult to: (1) identify general principles for broader application beyond site-specific insights, (2) quantify success, and (3) identify general ways to improve restoration programs. In this paper, I outline some personal perspectives on how these deficiencies might be addressed using insights from two decades of work on restoration in the temperate woodlands of south-eastern Australia. I argue problems associated with a lack of monitoring and limited connection to ecological theory need to be rectified if future landscape and ecosystem restoration programs are to be more successful than those in the past and are to deliver better ecological returns on investment. This should help enhance learning and boost the potential for continuous improvement in restoration efforts.
Introduction
The 10 year period from 2019 has been declared the decade of ecosystem restoration (UN 2019) and this must include extensive restoration of degraded landscapes. This is a monumental task given the billions of hectares of degraded forest and agricultural land, and riparian areas globally (Gibbs and Salmon, 2015; Intergovernmental Science-policy Platform on Biodiversity and Ecosystem Services (IPBES), 2019). Some workers have estimated that the cost of remediating degraded land globally could exceed $US12 trillion (R. Crouzeilles et al., unpublished data) or approximately two-thirds of the GDP of the United States. However, many past restoration efforts have been less ecologically effective and less cost effective than they might otherwise have been if good objective setting, planning, monitoring and adaptive management had been in place (Bernhardt et al., 2005; Kleijn et al., 2006; Hajkowicz, 2009; Fuentes-Montemayor et al., 2011; Batary et al., 2015; Nilsson et al., 2015).
Here, I argue that ecosystem and landscape restoration will under-deliver unless more restoration programs are better linked to the existing body of ecological concepts and theory (Hobbs and Norton, 1996; Hobbs and Harris, 2001; Temperton et al., 2004; Lamb, 2011; Perring et al., 2015; Suding et al., 2015). This can help better identify the key ecological processes associated with the patterns of biotic response that are observed. It is also can help promote learning across restoration programs and enhance the ability to predict the outcomes of management interventions in new settings. In addition, many restoration programs globally have suffered from a lack of robust long-term monitoring. These include (among many others) riparian restoration programs in the United States (Bernhardt et al., 2005), France (Morandi et al., 2014), and Scandinavia (Nilsson et al., 2015), as well as restoration programs to revegetate native woodland cover (Hajkowicz, 2009) and tackle secondary salinity in agricultural Australia (Pannell and Roberts, 2010). Without robust monitoring, it is impossible to determine what actions were (and were not) effective and the reasons for these outcomes (Lindenmayer and Likens, 2018).
In this paper, I provide some personal perspectives on the need to link restoration programs with ecological concepts and theory and rigorously assess their effectiveness through robust, well designed monitoring programs. My perspectives are based on a detailed assessment of the monitoring literature (reviewed in Lindenmayer and Likens, 2018; see also Legge et al., 2018) together with more than 20 years of practical experience in large-scale restoration programs in the temperate woodlands of south-eastern Australia (Lindenmayer et al., 2018c). These woodlands are among the most heavily cleared and altered biomes on earth (Fischer et al., 2009), with literally billions of trees having been cleared to establish croplands and pastures for domestic livestock production (Walker et al., 1993). Such changes have resulted in widespread land degradation, soil erosion, secondary salinity, and biodiversity loss (Benson, 2008; Lindenmayer et al., 2010a). Many hundreds of millions of dollars have been invested in woodland replanting programs over the past 20–30 years in an effort to tackle these problems (Hajkowicz, 2009; Pannell and Roberts, 2010).
Better Links to Ecological Concepts and Theory
Different authors use different terminology and emphases to define ecological theory. However, it can be broadly considered to be a system of conceptual constructs including axioms or assumptions, hypotheses, and concepts (Levin, 2009). The simplest form of an ecological theory would comprise a testable hypothesis, and allied assumptions and concepts (Driscoll and Lindenmayer, 2012). A range of seminal articles and books have discussed the importance of ecological theory in guiding landscape and ecosystem restoration programs (e.g., Hobbs and Norton, 1996; Hobbs and Harris, 2001; Temperton et al., 2004; Lamb, 2011; Perring et al., 2015; Suding et al., 2015). However, my experience in working in temperate woodland restoration is that many programs are focused on “getting trees in the ground” and are undertaken with limited or no reference to ecological concepts and theory. I argue that this can restrict learning to individual case studies and make it difficult to identify more general insights and principles from across a suite of studies and which can be applied more broadly (Driscoll and Lindenmayer, 2012; Tscharntke et al., 2012). In many respects, this was one of the key reasons why journals such as Restoration Ecology were instigated (R. Hobbs, personal communication).
Connection to ecological concepts and theory has many important advantages (reviewed by Pickett et al., 1994; Driscoll and Lindenmayer, 2012). First, consideration of ecological theory will often necessitate the careful definition and subsequent rigorous use of key terms which can be important in the execution of restoration programs. For example, the term habitat is often used liberally to mean vegetation cover, when a more precise application should be as a species-specific entity (Hall et al., 1997). Such a distinction matters because simply restoring the native vegetation cover in an area may not mean that it provides habitat for a given species. Rather, the provisioning conditions that would serve as habitat for a target species may require the maintenance or development of particular site attributes that are needed for it to persist (Morrison et al., 2006) [such as a particular food plant species for a butterfly (Wilson et al., 1997; Schultz, 2002) or rock features for a reptile species (Webb and Shine, 2000)]. In other cases, better understanding and definition of the concept and term habitat in a restoration context may highlight that restoration efforts for a given species may compromise outcomes for a different species with different habitat requirements (e.g., see Fedriani et al., 2017). Another example of the need to consider key definitions that underpin ecological theory is the use of the term landscape. Like the term habitat, what constitutes a landscape is species-dependent and scale-dependent and therefore may vary markedly between organisms (Wiens et al., 1997). Moreover, different organisms may perceive an area differently from the way it is perceived by humans (Manning et al., 2004). This matters in a restoration context because the scale of actions such as establishing a replanting will likely vary substantially if the aim is to restore populations of a plant versus those of a beetle or wide-ranging bird or mammal (McAlpine et al., 2016). Similarly, particular species that are unable to coexist at a given spatial scale (e.g., locally) may do so at a larger scale (Fedriani et al., 2017) meaning that targeted restoration and conservation actions may need to accommodate the needs of different species at different scales.
A second key reason why ecological concepts and theory is critical in restoration programs is that it can help identify the underlying ecological mechanisms giving rise to observed patterns (such as which species and sets of species can colonize restored sites) (Temperton et al., 2004; Suding, 2011). Identification of mechanistic processes can help better predict the outcomes of restoration programs in new areas (Perring et al., 2015; Suding et al., 2015; Palmer et al., 2016). On this basis, I suggest that better connection between ecological concepts and theory with the applied practice of restoration will help advance the discipline of restoration both more rapidly and more widely. As an example, the vertical structure hypothesis (MacArthur, 1964) in which more niches and therefore species occur in more vertically heterogeneous vegetation was used to guide studies that sought to better understand the response of arboreal marsupials to stand conditions in wet forests (Lindenmayer et al., 1991). The resulting mechanistic understanding, helped guide the design of a monitoring program for birds in replanted temperate woodlands and which underscored the importance of establishing restored areas around large old paddock trees to create multi-aged stand conditions to boost bird biodiversity (Lindenmayer et al., 2010b).
Table 1 shows a small subset of ecological concepts and theories that have broad relevance to restoration ecology and were used to guide a range of studies of restoration programs in the temperate woodlands of south-eastern Australia. Importantly, the use of theory proved to be instructive in reshaping new phases of restoration programs, including in areas where they had not previously been applied (Lindenmayer et al., 2013). For example, threshold theory suggests there should be critical breakpoints in vegetation cover below which losses of biodiversity will be extremely rapid and many species will not be able to persist (Andren, 1994; Radford et al., 2005; Haslem et al., 2015). A nominal value of 30% native vegetation cover in a landscape was suggested to be a key threshold or breakpoint (e.g., McIntyre et al., 2002). On this basis, some management agencies in southern Australia considered that targeted revegetation programs would be most appropriate in landscapes where levels of native vegetation cover exceed 30%. My research team was asked by vegetation managers to explore relationships between species richness and the occurrence of individual bird species in relation to total amounts of native vegetation to uncover evidence of thresholds. Threshold theory was therefore used in guiding subsequent empirical analyses and, surprisingly, the null hypothesis (an absence of threshold or breakpoint relationships) was upheld both for overall bird species richness and for the occurrence of a suite of individual bird species (Cunningham et al., 2014a, b). Importantly, the response curve for measures such as species richness was a distinctive asymptotic shape, suggesting that the fastest gains in species richness were for increases in vegetation amount at low levels of native vegetation cover (Cunningham et al., 2014a, b). This result, based on testing threshold theory, has re-shaped vegetation restoration incentive schemes, with funding for revegetated projects from local natural resource management agencies now no longer confined to landscapes with already high levels of native vegetation cover.
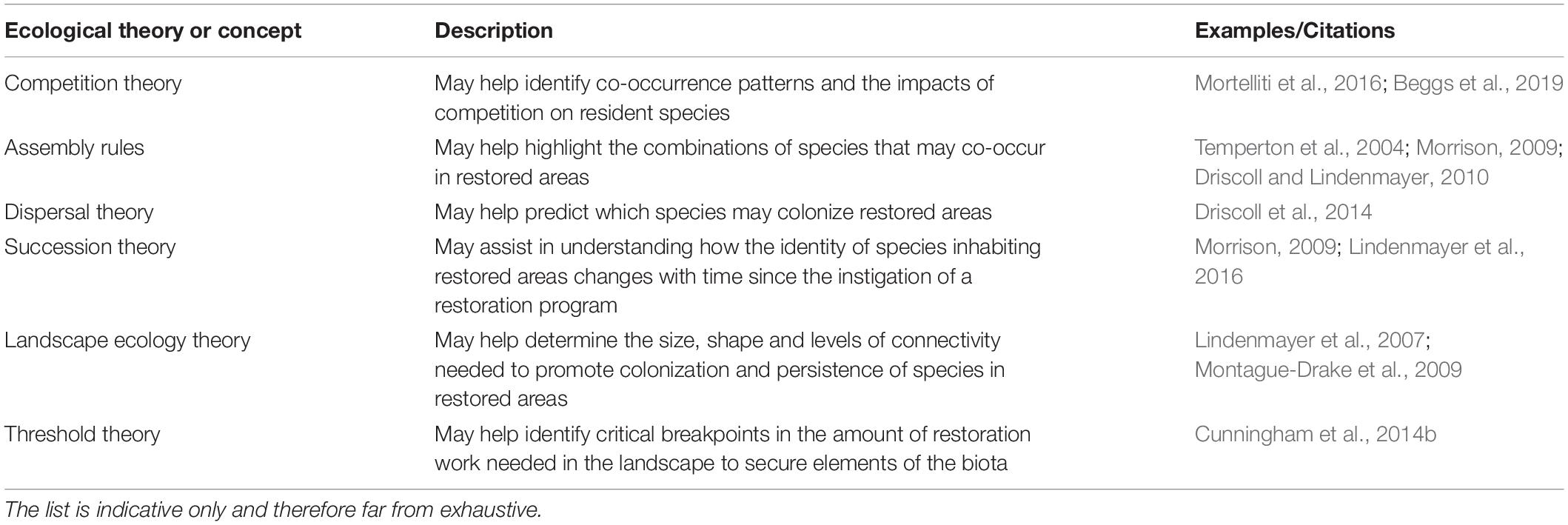
Table 1. Some areas of ecological theory and concepts with broad relevance to restoration management and employed in long-term restoration studies in the temperate woodlands of south-eastern Australia.
Although ecological theory and concepts have an important role in informing and guiding restoration programs (e.g., Hobbs and Norton, 1996; Hobbs and Harris, 2001; Temperton et al., 2004; Lamb, 2011; Perring et al., 2015; Suding et al., 2015), it also has limitations. Some researchers suggest that poorly developed theory has led to a lack of repeatability and even false discoveries in some disciplines (Andren, 1994; Smaldino, 2019) – and this problem may apply to some areas of restoration ecology. Another limitation is the challenge in developing theory when linking restoration ecology to other disciplines such as economics. For example, our work in temperate woodlands has begun exploring the development of new models for sustainable financing to promote restoration actions on farms. The aim here is to facilitate large-scale restoration programs (that can sometimes be costly) but without creating massive financial burdens on government or individual property owners (Chapman and Lindenmayer, 2019). Unfortunately, there is currently no cogent body of cross-disciplinary theory to guide such kinds of intersecting finance and ecological research, with the development of innovative financing approaches for promoting degraded agricultural land restoration sourced from the higher education sector in this case (Chapman and Lindenmayer, 2019). One possible opportunity in this regard is to use the System of Economic and Environmental Accounting (SEEA) developed by the United Nations (2012) to quantify the economic value of natural assets in a coherent way. However, links between biodiversity accounting and economics and restoration in such a framework currently remain elusive (Keith et al., 2017).
The Critical Need to Improve Monitoring
Ecological monitoring is the only way to judge the ecological success (or otherwise) of restoration programs. However, for monitoring programs to be effective, they must be well designed and implemented, ideally with the same rigor as high quality research programs. Notably, restoration efforts such as animal reintroduction programs have shown the array of benefits than can arise from them being implemented as well designed experiments (Caughley and Gunn, 1996; Sheean et al., 2011).
Several key inter-related characteristics underpin well designed monitoring programs. These include (after Lindenmayer and Likens, 2018):
1. A well formulated and well articulated set of objectives. This sets the benchmark against which to develop key ecological questions and design subsequent monitoring programs.
2. A well-developed set of key ecological questions that are of management relevance and which can be answered through the monitoring program.
3. A simple, but insightful conceptual model of the ecosystem (or other entity) that is being monitored, so that appropriate questions can be posed.
4. A robust experimental design with sufficient replication of sites to ensure enough statistical power to detect change and answer key questions.
5. Control sites where restoration actions are not implemented to provide contrast to those places where management interventions (e.g., tree planting, weed removal, or riparian area repair) have taken place.
6. Trigger points for management action if problems are encountered such as, for example, a major decline in the population of a species targeted for restoration.
7. Appropriate data management, analyses of data, and reporting of outcomes.
The key objectives of a restoration program need to be well conceived and transparently articulated at the outset. That is, the ecological (as well as social) goals of a restoration program need to be made clear to all relevant stakeholders including policy makers, researchers, practitioners and others. This is a fundamentally important step that allows the results of restoration to be evaluated against objectives. However, it is surprising how often this critical step is ignored or ill-considered. Only when objectives are well developed is it feasible to frame good questions to ask and, subsequently, design and implement a robust restoration program. Hence, monitoring should not be a post-restoration activity that is unconnected to objective setting and related planning and implementation.
Appropriately designed monitoring will often need to be conducted with reference to what entails success for a given restoration program (Morandi et al., 2014; Nilsson et al., 2015). Determining, a priori, what constitutes success demands (as outlined above) setting objectives for an effective restoration program, including identifying benchmarks against which management interventions can be assessed (Gibbons et al., 2010). Success also may need to be assessed through seeking answers to key questions that underpin restoration programs. For example: Did a native bird species of conservation concern recolonize areas after they had been revegetated? Did native plant species recover in places where invasive plants were removed? Did the water quality of streams improve once riparian zones were remediated? There is considerable value in efforts to communicate successes in restoration programs as determined through robust monitoring (e.g., Springer, 2018). This is because it can provide learnings about what has and has not worked. Communication of success (as determined from robust monitoring) also can provide inspiration for others seeking to instigate restoration programs (Garnett et al., 2018).
As outlined above, the robust design of monitoring programs for restoration (see Gellie et al., 2018) will often have to include control sites where management interventions are not established for comparison with those areas where they have been implemented. The notion of monitoring areas where restoration has not occurred can be challenging for some management agencies and restoration practitioners, as they may perceive it to be unnecessary and a waste of money. Scientists need to work harder to better explain to managers why areas where there has been no management intervention can be an important part of monitoring for restoration programs (Lindenmayer and Likens, 2018), and the basis for building a body of information to guide evidence-based management (sensu Sutherland et al., 2004).
A further valuable attribute of a robust monitoring program includes documenting not only the responses of, for example, native plants and animals to restoration actions (McAlpine et al., 2016), but also the management inputs associated with restoration programs (such as the costs of buying and planting trees, erecting fences, ongoing weed control, and other management activities). Such input data can be useful for determining return on investment and hence identifying which actions recover or conserve the most biodiversity for a given amount of funding.
Effective monitoring programs will often need to be long-term, potentially spanning several decades or longer (Nilsson et al., 2015). This is particularly critical as the recovery of ecosystems or populations of particular species may take a prolonged period, especially if past levels of human disturbance and environmental modification have been substantial (Majer et al., 2013; Palmer et al., 2016). In the case of the restoration programs in the temperate woodlands, the value of replanted areas as refugia for birds (including species of conservation concern) during droughts only became apparent after prolonged monitoring spanning more than a decade (Lindenmayer et al., 2018b).
There can be considerable costs associated with ensuring that restoration programs are underpinned by a rigorous experimental design and ongoing sampling. A general rule of thumb is that approximately 10% of the overall cost of restoration programs should be dedicated to monitoring (Lindenmayer and Likens, 2018). Providing adequate resources for effective monitoring will require a change in attitude among many management agencies. This is because monitoring is often the last activity funded and the first one cut when budgets come under pressure. A key issue here is that the initial costing for restoration programs must include a reasonable budget for well-designed and appropriately implemented ongoing and robust monitoring. This should include some budget provision for addressing aspects of restoration programs that are found to be failing and in need of modification to set them on a better trajectory for success. A further form of cost is time. That is, it can take time to design and then fully implement a robust monitoring program. However, taking slightly longer to frame a project, especially one where there are complex issues to resolve, can lead to better outcomes of lasting value (Lindenmayer and Likens, 2018).
Finally, a key part of monitoring restoration programs must be for monitoring to include at least some component of on-the-ground activities. That is, monitoring must go beyond, purely “desk-based” assessments like remote sensing. This is important for several reasons. First, work in the field provides scientists with a key source of insight and inspiration around the ecological reasons for the patterns they have been recorded. Second, some groups of organisms (e.g., reptiles) are very difficult to monitor remotely. Third, some on-the-ground monitoring demands interactions with restoration practitioners such as farmers who are revegetating parts of their properties. This can promote knowledge exchange and facilitate rapid adoption of improved management practices (that are revealed from the results of monitoring). This has occurred in revegetation programs in Australian temperate woodlands, where science-manager partnerships have fostered rapid changes in on-the-ground practices such as increasing the width of linear plantings between grazing paddocks (Lindenmayer et al., 2013).
Despite the importance of monitoring, it is remarkable how often it is not part of restoration programs. Indeed, many assessments of major environmental programs around the world that include restoration activities lament the paucity of robust “outcomes monitoring” (e.g., Bernhardt et al., 2005; Kleijn et al., 2006; Hajkowicz, 2009; Robins and Kanowski, 2011; Morandi et al., 2014). Outcomes monitoring can be broadly defined as monitoring to determine where a given kind of management intervention has resulted in an improvement in a particular biotic target (such as the increase of a target population of a species). Where outcomes monitoring has been conducted, it has often been done poorly, making it difficult to determine if a restoration program has been effective (reviewed by Lindenmayer and Likens, 2018). In other cases where monitoring is conducted, it is often what can be termed “activities monitoring.” That is, an appraisal focused on what actions were done (e.g., whether a fence was constructed or trees were planted), but the impacts of the actions, such the recovery of a threatened species or the eradication of an invasive plant, were not quantified (Hajkowicz, 2009).
Avoid Excessive Risk Aversion
The vast majority of restoration programs are motivated by a strong desire to succeed but sometimes also an even greater sense of aversion to failure. Projects that fail are seen by some to be a threat to the integrity and competence of management agencies. Yet, high levels of risk aversion can limit learning and thwart opportunities to improve future programs; indeed, more may be learned from failures than success (Redford and Taber, 2000). Notably, research from other fields shows that success is often preceded by repeated failures (e.g., see Yin et al., 2019). Of course, some organizations may anticipate that monitoring may produce results that are uncomfortable, and therefore elect to avoid monitoring altogether. This both precludes learning and increases the risk of unwanted ecological surprises (Lindenmayer et al., 2010c). Many examples from natural resource management also show that ignorance also can be very expensive and opportunities for restoration are limited, such as when environmental problems that have developed may be irreversible (e.g., when populations of species go extinct or ecosystems collapse, see Steffen et al., 2015). Many of the initiatives of the Society for Ecological Restoration discuss issues associated with baselines and benchmarks and what is a reasonable basis for determining success in restoration programs.
Two examples from relatively recent experiences in Australian temperate woodland restoration highlight the problems that can arise from excessive risk aversion. The first involves the Noisy Miner (Manorina melanocephala), which is a hyper-aggressive Australian honeyeater bird. The species excludes a wide range of smaller bird species from patches of remnant native woodland through harassment and interference competition (Beggs et al., 2019). The negative impacts of the Noisy Miner occur in millions of hectares of agricultural land and affect many species of woodland birds including several of major conservation concern (Maron et al., 2013). The Noisy Miner is listed by the Australian Government as a Key Threatening Process1. Culling has been proposed as a way to mitigate the problem and restore native woodland bird assemblages (Mortelliti et al., 2016). However, a carefully designed, randomized and replicated experiment revealed that culling programs for the Noisy Miner may not work – patches where birds are repeatedly removed are rapidly recolonized (Beggs et al., 2019). Moreover, culling was found to be cost prohibitive over large areas (Beggs et al., 2019). Although the experimental cull failed, allied research showed that Noisy Miners can be excluded from areas by establishing densely spaced tree replantings as part of large-scale revegetation programs (Lindenmayer et al., 2010b), as well as by creating a dense understorey of shrubs under an overstorey of old growth woodland (Lindenmayer et al., 2018a). Many of these insights have been derived from long-term monitoring and they have shown what works and what does not in terms of guiding effective approaches for controlling the Noisy Miner and, in turn, restoration programs in Australian temperate woodlands. Notably, some agencies continue to employ culling programs because relatively large sums of money have already been dedicated to them, and the notion of large “sunk costs” and program ineffectiveness may seem to be too detrimental in terms of damage to institutional reputations.
A second example of risk aversion involves the use of biodiversity offsets (sensu Maron et al., 2015). Specifically, attempts were made to offset the impacts of large scale clearing of large old trees to widen a major highway in the temperate woodland belt of southern New South Wales. The offset was the installation of more than 500 nest boxes. The nest box program was designed to reduce the impacts of road widening on a suite of cavity-tree dependent threatened vertebrates (Lindenmayer et al., 2017). However, 4 years of monitoring showed that the nest box program largely failed, with few instances of occupancy by target threatened species and a number of instances of nest boxes being used by invasive animals (Lindenmayer et al., 2017). A key learning from the work was that a likely better investment would have been to revegetate some areas of semi-cleared woodland. This would have been a management intervention with better and longer-lasting outcomes for biodiversity, although there would have been a prolonged lag before such areas provided suitable nesting sites for cavity-using threatened species (Lindenmayer et al., 2017). The result has provided some clear lessons for management but the agency responsible for the nest box program has been somewhat reticent to acknowledge there were problems and it failed to view the results as a way to improve future management.
One way to avoid excessive risk aversion is to facilitate a change of culture within resource management agencies and ensure there are well developed approaches for learning from failures and, conversely, avoiding repeatedly making the same mistakes. Indeed, reintroduction biology – a sub-discipline of restoration ecology – has a well developed framework for rapid learning from failures and mistakes (e.g., Ewen et al., 2011; Robinson et al., 2019). Another approach to tackling problems with risk aversion is to provide ways to better document what activities did not work. The journal Restoration Ecology has a special section termed “setbacks and surprises” for documenting where the outcomes of projects were not as initially anticipated2.
Concluding Comments
Large parts of the world’s land surface are in urgent need of restoration (Intergovernmental Science-policy Platform on Biodiversity and Ecosystem Services (IPBES), 2019). Significant numbers of restoration programs have been instigated around the world (e.g., see Crouzeilles et al., 2016; Palmer et al., 2016). However, many are likely to have been less effective than they might have been. It is critical that future restoration programs are as cost-effective and ecologically effective as possible. This is because of the current mismatch between the spatial scale of the restoration challenge and the limited funding available to tackle the problem, and the extent of ongoing biodiversity loss if efforts to reverse land degradation are inadequate.
Three inter-related strategies are needed to improve restoration programs, particularly as they relate to better learning and opportunities to improve the basis for evidence-based management. These are more attention to, and greater funding for, monitoring; a better acceptance of risks associated with on-ground restoration works; and a strengthened connection between applied actions and ecological concepts and theory.
Author Contributions
DL conceived and wrote the manuscript.
Funding
The Sustainable Farms project’s major funders are the Ian Potter Foundation, the Vincent Fairfax Family Foundation, the Department of Agriculture and Water Resources, and the Australian National University.
Conflict of Interest
The author declares that the research was conducted in the absence of any commercial or financial relationships that could be construed as a potential conflict of interest.
Acknowledgments
The work reported here is part of the Sustainable Farms project at the Australian National University. Key members of that project include Michelle Young, Mason Crane, Daniel Florance, Clare Crane, David Smith, Martin Westgate, and Wade Blanchard. I thank Michael Morrison for encouraging me to write this article and providing several rounds of insightful feedback that improved earlier versions of the manuscript. I am grateful to Richard Hobbs for many interesting discussions about restoration ecology over many years.
Footnotes
- ^ http://www.environment.gov.au/biodiversity/threatened/key-threatening-processes/overabundant-noisy-miners
- ^ https://onlinelibrary.wiley.com/page/journal/1526100x/homepage/forauthors.html
References
Andren, H. (1994). Effects of habitat fragmentation on birds and mammals in landscapes with different proportions of suitable habitat: a review. Oikos 71, 355–366.
Batary, P., Dicks, L. V., Kleijn, D., and Sutherland, W. J. (2015). The role of agri-environment schemes in conservation and environmental management. Conserv. Biol. 29, 1006–1016. doi: 10.1111/cobi.12536
Beggs, R., Tulloch, A. I. T., Pierson, J., Blanchard, W., Crane, M., and Lindenmayer, D. B. (2019). Patch-scale culls of an overabundant bird defeated by immediate recolonization. Ecol. Appl. 29:e01846. doi: 10.1002/eap.1846
Benson, J. S. (2008). New South Wales vegetation classification and assessment: part 2 Plant communities of the NSW South-western Slopes bioregion and update of the NSW Western Plains plant communities, version 2 of the NSWVCA database. Cunninghamia 10, 599–673.
Bernhardt, E. S., Palmer, M. A., and Allan, J. D. (2005). Synthesizing US river restoration projects. Science 308, 636–637. doi: 10.1126/science.1109769
Caughley, G. C., and Gunn, A. (1996). Conservation Biology in Theory and Practice. Cambridge, MA: Blackwell Science.
Chapman, B., and Lindenmayer, D. B. (2019). A novel approach to the sustainable financing of the global restoration of degraded agricultural land. Environ. Res. Lett. 14:124084. doi: 10.1088/1748-9326/ab5deb
Crouzeilles, R., Curran, M., Ferreira, M. S., Lindenmayer, D. B., Grelle, C. E. V., and Rey Benayas, J. M. (2016). A global meta-analysis on the ecological drivers of forest restoration success. Nat. Commun. 7:11666. doi: 10.1038/ncomms11666
Cunningham, R. B., Lindenmayer, D. B., Barton, P., Ikin, K., Crane, M., Michael, D., et al. (2014a). Cross-sectional and temporal relationships between bird occupancy and vegetation cover at multiple spatial scales. Ecol. Appl. 24, 1275–1288. doi: 10.1890/13-0872.1
Cunningham, R. B., Lindenmayer, D. B., Crane, M., Michael, D. R., Barton, P. S., Gibbons, P., et al. (2014b). The law of diminishing returns: woodland birds respond to native vegetation cover at multiple spatial scales and over time. Divers. Distrib. 20, 59–71. doi: 10.1111/ddi.12145
Driscoll, D., and Lindenmayer, D. B. (2012). Framework to improve the application of theory in ecology and conservation. Ecol. Monogr. 82, 129–147. doi: 10.1890/11-0916.1
Driscoll, D. A., Banks, S. C., Barton, P. S., Ikin, K., Lentini, P., Lindenmayer, D. B., et al. (2014). The trajectory of dispersal research in conservation biology: systematic review. PLoS One 9:e95053. doi: 10.1371/journal.pone.0095053
Driscoll, D. A., and Lindenmayer, D. B. (2010). Assembly rules are rare in SE Australian bird communities, but sometimes apply in fragmented agricultural landscapes. Ecography 33, 854–865. doi: 10.1111/j.1600-0587.2010.06094.x
Ewen, J. G., Armstrong, D. P., Parker, K. A., and Seddon, P. J. (eds). (2011). Reintroduction Biology: Integrating Science and Management. Oxford: Wiley-Blackwell.
Fedriani, J. M., Garcıa, L. V., Sanchez, M. E., Calderon, J., and Ramo, C. (2017). Long-term impact of protected colonial birds on a jeopardized cork oak population: conservation bias leads to restoration failure. J. Appl. Ecol. 54, 450–458. doi: 10.1111/1365-2664.12672
Fischer, J., Stott, J., Zerger, A., Warren, G., Sherren, K., and Forrester, R. I. (2009). Reversing a tree regeneration crisis in an endangered ecoregion. Proc. Natl. Acad. Sci. U.S.A. 106, 10386–10391. doi: 10.1073/pnas.0900110106
Fuentes-Montemayor, E., Goulson, D., and Park, K. J. (2011). Pipistrelle bats and their prey do not benefit from four widely applied agri-environment management prescriptions. Biol. Conserv. 144, 2233–2246. doi: 10.1016/j.biocon.2011.05.015
Garnett, S., Latch, P., Lindenmayer, D. B., and Woinarski, J. (2018). Recovering Australian Threatened Species. A Book of Hope. Melbourne: CSIRO Publishing.
Gellie, N., Breed, M. F., Mortimer, P. E., Harrison, R. D., Xu, J., and Lowe, A. J. (2018). Networked and embedded scientific experiments will improve restoration outcomes. Front. Ecol. Environ. 16, 288–294. doi: 10.1002/fee.1810
Gibbons, P., Briggs, S. V., Murphy, D. Y., Lindenmayer, D. B., McElhinny, C., and Brookhouse, M. (2010). Benchmark stem densities for forests and woodlands in south-eastern Australia under conditions of relatively little modification by humans since European settlement. For. Ecol. Manage. 260, 2125–2133. doi: 10.1016/j.foreco.2010.09.003
Gibbs, H., and Salmon, J. M. (2015). Mapping the world’s degraded lands. Appl. Geogr. 57, 12–21. doi: 10.1016/j.apgeog.2014.11.024
Hajkowicz, S. (2009). The evolution of Australia’s natural resource management programs: towards improved targeting and evaluation of investments. Land Use Policy 26, 471–478. doi: 10.1016/j.landusepol.2008.06.004
Hall, L. S., Krausman, P. A., and Morrison, M. L. (1997). The habitat concept and a plea for the use of standard terminology. Wildl. Soc. Bull. 25, 173–182.
Haslem, A., Nimmo, D. G., Radford, J. Q., and Bennett, A. F. (2015). Landscape properties mediate the homogenization of bird assemblages during climatic extremes. Ecology 96, 3165–3174. doi: 10.1890/14-2447.1
Hobbs, R., and Harris, J. A. (2001). Restoration ecology: repairing the Earth’s damaged ecosystems in the new millennium. Restor. Ecol. 9, 239–246. doi: 10.1046/j.1526-100x.2001.009002239.x
Hobbs, R., and Norton, D. A. (1996). Towards a conceptual framework for restoration ecology. Restor. Ecol. 4, 93–100. doi: 10.1111/brv.12470
Intergovernmental Science-policy Platform on Biodiversity and Ecosystem Services (IPBES) (2019). IPBES Global Assessment Summary for Policymakers. New York, NY: United Nations.
Keith, H., Vardon, M., Stein, J. A. R., Stein, J. L., and Lindenmayer, D. B. (2017). Ecosystem accounts define explicit and spatial trade-offs for managing natural resources. Nat. Ecol. Evol. 1, 1683–1692. doi: 10.1038/s41559-017-0309-1
Kleijn, D., Baquero, R. A., Clough, Y., Diaz, M., De Estaban, J., Fernandez, F., et al. (2006). Mixed biodiversity benefits of agri-environment schemes in five European countries. Ecol. Lett. 9, 243–254. doi: 10.1111/j.1461-0248.2005.00869.x
Lamb, D. (2011). Regreening the Bare Hills: Tropical Forest Regeneration in the Asia-Pacific Region. Dordrecht: Springer.
Legge, S. M., Lindenmayer, D. B., Robinson, N. M., Scheele, B. C., Southwell, D. M., and Wintle, B. A. (eds). (2018). Monitoring Threatened Species and Ecological Communities. Melbourne: CSIRO Publishing.
Levin, S. A. (ed.) (2009). The Princeton Guide to Ecology. Princeton, NJ: Princeton University Press.
Lindenmayer, D. B., Bennett, A. F., and Hobbs, R. J. (eds). (2010a). Temperate Woodland Conservation and Management. Melbourne: CSIRO Publishing.
Lindenmayer, D. B., Blanchard, W., Crane, M., Michael, D., and Florance, D. (2018a). Size or quality. What matters in vegetation restoration for bird biodiversity in endangered temperate woodlands? Austral Ecol. 43, 798–806. doi: 10.1111/aec.12622
Lindenmayer, D. B., Crane, M., Evans, M. C., Maron, M., Gibbons, P., Bekessy, S., et al. (2017). The anatomy of a failed offset. Biol. Conserv. 210, 286–292. doi: 10.1016/j.biocon.2017.04.022
Lindenmayer, D. B., Cunningham, R., Crane, M., Michael, D., and Montague-Drake, R. (2007). Farmland bird responses to intersecting replanted areas. Landsc. Ecol. 22, 1555–1562. doi: 10.1007/s10980-007-9156-9
Lindenmayer, D. B., Cunningham, R. B., Tanton, M. T., Nix, H. A., and Smith, A. P. (1991). The conservation of arboreal marsupials in the montane ash forests of the Central Highlands of Victoria, South-East Australia: III. The habitat requirements of Leadbeaters possum Gymnobelideus leadbeateri and models of the diversity and abundance of arboreal marsupials. Biol. Conserv. 56, 295–315. doi: 10.1016/0006-3207(91)90063-f
Lindenmayer, D. B., Knight, E. J., Crane, M. J., Montague-Drake, R., Michael, D. R., and MacGregor, C. I. (2010b). What makes an effective restoration planting for woodland birds? Biol. Conserv. 143, 289–301. doi: 10.1016/j.biocon.2009.10.010
Lindenmayer, D. B., Lane, P., Crane, M., Florance, D., Foster, C. N., Ikin, K., et al. (2018b). Weather effects on birds of different size are mediated by long-term climate and vegetation type in endangered temperate woodlands. Glob. Chang. Biol. 25, 675–685. doi: 10.1111/gcb.14524
Lindenmayer, D. B., Lane, P. W., Barton, P. S., Crane, M., Ikin, K., Michael, D. R., et al. (2016). Long-term bird colonization and turnover in restored woodlands. Biodivers. Conserv. 25, 1587–1603. doi: 10.1007/s10531-016-1140-8
Lindenmayer, D., and Likens, G. E. (2018). Effective Ecological Monitoring. Melbourne: CSIRO Publishing.
Lindenmayer, D. B., Likens, G. E., Krebs, C. J., and Hobbs, R. J. (2010c). Improved probability of detection of ecological “surprises”. Proc. Natl. Acad. Sci. U.S.A. 107, 21957–21962. doi: 10.1073/pnas.1015696107
Lindenmayer, D. B., Michael, D. R., Crane, M., Florance, D., and Burns, E. (2018c). Restoring Farm Woodlands for Wildlife. Melbourne: CSIRO Publishing.
Lindenmayer, D. B., Willinck, E., Crane, M., Michael, D., Okada, S., Cumming, C., et al. (2013). Murray Catchment habitat restoration: lessons from landscape-level research and monitoring. Ecol. Manag. Restor. 14, 80–92. doi: 10.1111/emr.12051
MacArthur, R. H. (1964). Environmental factors affecting bird species diversity. Am. Nat. 98, 387–397. doi: 10.1086/282334
Majer, J. D., Heterick, B., Gohr, T., Hughes, E., Mounsher, L., and Grigg, A. (2013). Is thirty-seven years sufficient for full return of the ant biota following restoration? Ecol. Process. 2:19.
Manning, A. D., Lindenmayer, D. B., and Nix, H. A. (2004). Continua and Umwelt: novel perspectives on viewing landscapes. Oikos 104, 621–628. doi: 10.1111/j.0030-1299.2004.12813.x
Maron, M., Gordon, A., Possingham, H. P., Mackey, B. G., and Watson, J. (2015). Stop misuse of biodiversity offsets. Nature 523, 401–403. doi: 10.1038/523401a
Maron, M., Grey, M. J., Catterall, C. P., Major, R. E., Oliver, D. L., Clarke, M. F., et al. (2013). Avifaunal disarray due to a single despotic species. Divers. Distrib. 19, 1468–1479. doi: 10.1111/ddi.12128
McAlpine, C., Catterall, C. P., Mac Nally, R., Lindenmayer, D. B., Reid, L., Holl, K. D., et al. (2016). Integrating plant and animal-based perspectives for more effective restoration of biodiversity. Front. Ecol. Environ. 14, 37–45. doi: 10.1002/16-0108.1
McIntyre, S., McIvor, J. G., and Heard, K.M. (eds.) (2002). Managing and Conserving Grassy Woodlands. Melbourne: CSIRO Publishing.
Montague-Drake, R. M., Lindenmayer, D. B., and Cunningham, R. B. (2009). Factors affecting site occupancy by woodland bird species of conservation concern. Biol. Conserv. 142, 2896–2903. doi: 10.1016/j.biocon.2009.07.009
Morandi, B., Piégay, H., Lamouroux, N., and Vaudor, L. (2014). How is success or failure in river restoration projects evaluated? Feedback from French restoration projects. J. Environ. Manag. 137, 178–188. doi: 10.1016/j.jenvman.2014.02.010
Morrison, M. (2009). Restoring Wildlife. Ecological Concepts and Practical Applications. Washington, DC: Island Press.
Morrison, M. L., Marcot, B. G., and Mannan, R. W. (2006). Wildlife-Habitat Relationships. Concepts and Applications. Washington, DC: Island Press.
Mortelliti, A., Ikin, K., Tulloch, A. I. T., Cunningham, R., Stein, J. A. R., Michael, D., et al. (2016). Surviving with a resident despot: do revegetated patches act as refuges from the effects of the noisy miner (Manorina melanocephala) in a highly fragmented landscape? Divers. Distrib. 22, 770–782. doi: 10.1111/ddi.12444
Nilsson, C., Polvi, L. E., Gardeström, J., Hasselquist, E., Lind, L., and Sarneel, J. M. (2015). Riparian and in-stream restoration of boreal streams and rivers: success or failure? Ecohydrology 8, 753–764. doi: 10.1002/eco.1480
Palmer, M. A., Zedler, J. B., and Falk, D.A. (eds). (2016). Foundations of Restoration Ecology. Washington, DC: Island Press.
Pannell, D. J., and Roberts, A. M. (2010). Australia’s national action plan for salinity and water quality: a retrospective assessment. Aust. J. Agric. Resour. Econ. 54, 437–456. doi: 10.1111/j.1467-8489.2010.00504.x
Perring, M. P., Standish, R. J., Price, J. N., Craig, M. D., Erickson, T. E., Ruthrof, K. X., et al. (2015). Advances in restoration ecology: rising to the challenges of the coming decades. Ecosphere 6, 1–25.
Pickett, S. T. A., Kolasa, J., and Jones, C. G. (1994). Ecological Understanding. San Diego, CA: Academic Press.
Radford, J. Q., Bennett, A. F., and Cheers, G. J. (2005). Landscape-level thresholds of habitat cover for woodland-dependent birds. Biol. Conserv. 124, 317–337. doi: 10.1016/j.biocon.2005.01.039
Redford, K., and Taber, A. (2000). Writing the wrongs: developing a safe-fail culture in conservation. Conserv. Biol. 14, 1567–1568. doi: 10.1046/j.1523-1739.2000.01461.x
Robins, L., and Kanowski, P. (2011). Crying for our Country: eight ways in which “Caring for our Country” has undermined Australia’s regional model for natural resource management. Aust. J. Environ. Manag. 18, 88–108. doi: 10.1080/14486563.2011.566158
Robinson, N., Brewster, R., Dexter, N., MacGregor, C., Lindenmayer, D. B., Maple, D., et al. (2019). Be nimble with threat mitigation: lessons learned from the reintroduction of an endangered species. Restor. Ecol. 28, 29–38. doi: 10.1111/rec.13028
Schultz, C. B. (2002). Restoring resources for an endangered butterfly. J. Appl. Ecol. 38, 1007–1018.
Sheean, V. A., Manning, A. D., and Lindenmayer, D. B. (2011). An assessment of scientific approaches towards species relocations in Australia. Austral Ecol. 37, 204–215. doi: 10.1111/j.1442-9993.2011.02264.x
Smaldino, P. (2019). Better methods can’t make up for mediocre theory. Nature 575:9. doi: 10.1038/d41586-019-03350-5
Springer, K. (2018). “Eradication of invasive species on Macquarie Island to restore the natural ecosystem,” in Recovering Australian Threatened Species, eds S. T. Garnett, P. Latch, D. B. Lindenmayer, and J. Woinarski (Melbourne: CSIRO Publishing), 13–22.
Steffen, W., Richardson, K., Rockström, J., Cornell, S., Fetzer, I., Bennett, E. M., et al. (2015). Planetary boundaries: guiding human development on a changing planet. Science 347, 736–745.
Suding, K., Higgs, E., Palmer, M., Callicott, J. B., Anderson, C. B., Baker, M., et al. (2015). Committing to ecological restoration. Science 348, 638–640.
Suding, K. N. (2011). Toward an era of restoration in ecology: successes, failures and opportunities ahead. Annu. Rev. Ecol. Evol. Syst. 42, 465–487. doi: 10.1146/annurev-ecolsys-102710-145115
Sutherland, W. J., Pullin, A. S., Dolman, P. M., and Knight, T. M. (2004). The need for evidence-based conservation. Trends Ecol. Evol. 19, 305–308. doi: 10.1016/j.tree.2004.03.018
Temperton, V. M., Hobbs, R. J., Nuttle, T., and Halle, S. (eds). (2004). Assembly Rules and Restoration Ecology Bridging the Gap Between Theory and Practice. Washington, DC: Island Press.
Tscharntke, T., Tylianakis, J. M., Rand, T. A., Didham, R. K., Fahrig, L., Batary, P., et al. (2012). Landscape moderation of biodiversity patterns and processes - eight hypotheses. Biol. Rev. 87, 661–685. doi: 10.1111/j.1469-185X.2011.00216.x
United Nations (2012). System of Environmental-Economic Accounting Central Framework. New York, NY: United Nations.
Walker, J., Bullen, F., and Williams, B. G. (1993). Ecohydrological changes in the Murray-Darling Basin. I. The number of trees cleared over two centuries. J. Appl. Ecol. 30, 265–273.
Webb, J., and Shine, R. (2000). Paving the way for habitat restoration: can artificial rocks restore degraded habitats of endangered reptiles? Biol. Conserv. 92, 93–99. doi: 10.1016/s0006-3207(99)00056-7
Wiens, J. A., Schooley, R. L., and Weekes, R. D. (1997). Patchy landscapes and animal movements: do beetles percolate? Oikos 78, 257–264.
Wilson, M. V., Hammond, P. C., and Schultz, C. B. (1997). “The interdependence of native plants and Fender’s blue butterfly,” in Conservation and Management of Native Plants and Fungi, eds T. N. Kaye, A. Liston, R. M. Love, D. L. Luoma, R. J. Meinke, and M. V. Wilson (Corvallis, OR: Native Plant Society of Oregon), 83–87.
Keywords: community engagement, biodiversity recovery, revegetation programs, biodiversity conservation, temperate eucalypt woodlands, south-eastern Australia
Citation: Lindenmayer D (2020) Improving Restoration Programs Through Greater Connection With Ecological Theory and Better Monitoring. Front. Ecol. Evol. 8:50. doi: 10.3389/fevo.2020.00050
Received: 20 August 2019; Accepted: 19 February 2020;
Published: 05 March 2020.
Edited by:
David Jack Coates, Department of Biodiversity, Conservation and Attractions (DBCA), AustraliaReviewed by:
Jim Harris, Cranfield University, United KingdomMichael Perring, Ghent University, Belgium
Copyright © 2020 Lindenmayer. This is an open-access article distributed under the terms of the Creative Commons Attribution License (CC BY). The use, distribution or reproduction in other forums is permitted, provided the original author(s) and the copyright owner(s) are credited and that the original publication in this journal is cited, in accordance with accepted academic practice. No use, distribution or reproduction is permitted which does not comply with these terms.
*Correspondence: David Lindenmayer, RGF2aWQuTGluZGVubWF5ZXJAYW51LmVkdS5hdQ==