- 1Center for the Study of Social Insects, São Paulo State University (UNESP), Campus Rio Claro, Rio Claro, Brazil
- 2Comparative Plant and Fungal Biology, Royal Botanic Gardens, Kew, London, United Kingdom
Leaf-cutting ants employ diverse behavioral strategies for promoting the growth of fungal cultivars in a structure known as fungus garden. As a nutritionally rich resource for the ants, the fungal crop is threatened by microbial antagonists and pathogens. Strategies for protecting the garden against harmful microbes have been described in detail, although the process of microbial threat recognition is not fully understood. Here, we review the literature on leaf-cutting ants’ social immunity traits, in search of possibilities by which workers recognize harmful microbes in their system. Based on current data, we suggest mechanisms regarding (1) chemical recognition, where discrimination could be related to chemical cues from the antagonistic microbe or semiochemicals released by the fungus garden during harmful interactions, or (2) through associative learning when workers would connect the microbe cues with a damage in the fungus garden, developing a “colony-level memory” toward this threat. We also discuss evidence supporting ant–fungus communication as key for maintaining the health of the fungus garden, as well as experimental setups for future evaluation of threat detection and recognition by leaf-cutting ants.
Introduction
Social behavior evolved in several lineages of insects, ranging from diverse to complex levels of organization (Toth and Rehan, 2017). Across this continuum, some social insects achieved a major transition point of no return, where queen and workers are a lifetime morphological differentiated caste in a superorganismal level of hierarchy (Wheeler, 1911; Boomsma and Gawne, 2018). Social evolution is influenced by several environmental factors, including interactions between insect societies and microbes (Boomsma et al., 2005; Biedermann and Rohlfs, 2017; Toth and Rehan, 2017). By living in dense aggregations of genetically similar individuals, social insects have increased risks of infectious diseases spreading in their colonies (Schmid-Hempel, 1998, 2017; Naug and Camazine, 2002; Cremer et al., 2007, 2018; Rosengaus et al., 2011; Boomsma et al., 2014; Loreto et al., 2014; Stroeymeyt et al., 2014; Meunier, 2015; Cremer, 2019). Selective forces between social insects and pathogenic organisms have modulated defensive strategies, adaptations in physiological traits, behavior, and social organization (Pie et al., 2004; Fernández-Marín et al., 2006; Cremer et al., 2007, 2018; Ugelvig and Cremer, 2007; Yanagawa and Shimizu, 2007; Stow and Beattie, 2008; Walker and Hughes, 2009; Wilson-Rich et al., 2009; Yanagawa et al., 2011, 2012; Konrad et al., 2012, 2018; Kamhi and Traniello, 2013; Stroeymeyt et al., 2014; Liu et al., 2015, 2019; Quevillon et al., 2015; Malagocka et al., 2019), such as communication (Rosengaus et al., 1999) and caste specialization (Hughes et al., 2003; Brown et al., 2006; Griffiths and Hughes, 2010; Abramowski et al., 2011). Because these defensive adaptations involve the cooperation of the individuals for a colony-level response, they are collectively described as social immunity (Cremer et al., 2007). In this context, group members collaborate to avoid, control, or eliminate pathogens, thus acting as parts of an immune system (Cremer and Sixt, 2009; Cremer, 2019).
Social traits are also strongly influenced by interactions between social insects and beneficial microbes, either for defensive or nutritional symbiosis (Biedermann and Rohlfs, 2017). Fungal cultivation by social insects is a remarkable example of an insect–microbe association impacting social behavior (Mueller et al., 2005). The fungus-growing lifestyle independently evolved in the ants of the subtribe Attina (Hymenoptera: Formicidae: Myrmicinae; Mueller et al., 2001), termites in the subfamily Macrotermitinae (Isoptera: Termitidae; Aanen et al., 2002), and the subsocial beetles in the subfamilies Scolytinae and Platypodinae (Coleoptera: Curculionidae; Farrell et al., 2001; Hulcr and Stelinski, 2017). Because of their dependence on the fungal crop for nutritional resources, fungus-growing insects present a series of adaptations for fungal cultivation, maintenance, propagation, and protection (Mueller et al., 2005). Defending the fungal cultivar through chemical and behavioral responses is fundamental to the evolutionary success of the insect–fungal symbiosis, as the crop is a nutritionally valuable resource susceptible to microbial competitors and pathogens (Bass and Cherret, 1996; Currie et al., 1999a; Mueller et al., 2005; Morelos-Juárez et al., 2010; Visser et al., 2011; Um et al., 2013; Beemelmaans et al., 2017; Biedermann and Rohlfs, 2017). Thus, traits of social immunity in fungus-growing systems could have evolved targeting both insect hosts and the fungal crops.
The complex microbial environment of leaf-cutting ants, the most derived clade in the subtribe Attina, provides an interesting perspective for investigating how the responses to both harmful and beneficial microbes could have influenced ants’ social immunity (Biedermann and Rohlfs, 2017). Leaf-cutting ants have an obligate association with the basidiomycete species Leucoagaricus gongylophorus (Leucocoprini: Agaricales: Agaricaceae), on which all larvae and most of the adult ants feed (Mueller et al., 2005; Schultz and Brady, 2008; De Fine Licht et al., 2013). The maintenance of fungus gardens involves continuous substrate incorporation, which depends on specific behaviors for foraging and processing fresh leaves and flowers (Quinlan and Cherrett, 1977; Hölldobler and Wilson, 1990; Diniz and Bueno, 2010). However, foraging activities bring into the fungus garden several microorganisms along with the plant biomass (Fisher et al., 1996; Rodrigues et al., 2008; Van Bael et al., 2009). Also, mated queens may bring microorganisms during colony foundation on their integuments and in the piece of fungus gardens they carry (Poulsen et al., 2005; Pagnocca et al., 2012; Andersen et al., 2013, 2015; Meirelles et al., 2016). Regardless how they are introduced, once inside the colony, microbes may engage in distinct interactions with the fungal crop, as antagonists (Currie et al., 1999a; Rodrigues et al., 2008) or as mutualists (Poulsen et al., 2005). Ant workers can detect intruders and employ diverse physiological and behavioral strategies to protect the fungal crop (Currie and Stuart, 2001; Poulsen et al., 2002; Fernández-Marín et al., 2006; Abramowski et al., 2011; Gerstner et al., 2011; Rocha et al., 2014, 2017; Tranter et al., 2015; Nilssøn-Moller et al., 2018). It is reasonable to consider that ants may recognize and discriminate beneficial microorganisms from those detrimental to the fungus garden. However, the mechanisms by which leaf-cutting ants carry out these processes are poorly understood.
Here we review the literature for investigating the influence of the leaf-cutting ants’ microbial environment on their hygienic behavior. We first present the microbial environment where leaf-cutting ants live and the social immunity traits that evolved to protect the fungal culture and the ants from pathogens in general. Then, we propose two mechanisms by which ants could recognize distinct microbes and apply such defenses: (1) by responding to chemicals or semiochemicals released by microbes and the fungus crop indicating the presence of harmful interactions and (2) by associative learning and memorization derived from recurrent infection events. Through these scenarios, we aim to discuss the potential contribution of the fungal crop to the leaf-cutting ant’s social immunity.
The Microbial Environment of Leaf-Cutting Ants
All ant lineages in the subtribe Attina cultivate fungus for food, although both the fungal symbiont and the strategies for cultivation vary throughout these fungus-growing systems (Mueller et al., 1998, 2017; Schultz and Brady, 2008; Diniz and Bueno, 2010; Henrik et al., 2014). Attine ants in the genera Atta and Acromyrmex practice higher leaf-cutting fungiculture cultivating L. gongylophorus, a truly domesticated fungal symbiont that seems unable to support a free-living existence (Schultz and Brady, 2008; De Fine Licht et al., 2013; Nygaard et al., 2016; Mueller et al., 2017). The fungal crop is vertically transmitted when the foundress ant queen leaves her original colony carrying a mycelium pellet inside the infrabuccal pocket, which forms the initial crop inoculum (Mueller et al., 2001). The fungal symbiont evolved several adaptations to the symbiotic lifestyle, including swollen hyphal tips (i.e., gongylidia) that provide carbohydrates, amino acids, and enzymes to the ants (Quinlan and Cherrett, 1979; Schultz and Brady, 2008; Mikheyev et al., 2010; De Fine Licht et al., 2013). Leaf-cutting ants nourish the crop using fresh leaves as substrate, ultimately creating a structure known as the fungus garden (Figure 1), which is kept within underground chambers for most attine ant species (Hölldobler and Wilson, 1990; Mueller et al., 2001). The lignocellulolytic capacity of the fungus garden has been fundamental for supporting the mutualism (De Fine Licht et al., 2013; Khadempour et al., 2016; Vigueras et al., 2017), allowing the enzymatic conversion of massive amounts of fresh leaves into nutrients available to the queen, larvae, and most of the ant workers (Hölldobler and Wilson, 1990; Costa et al., 2008; De Fine Licht et al., 2013).
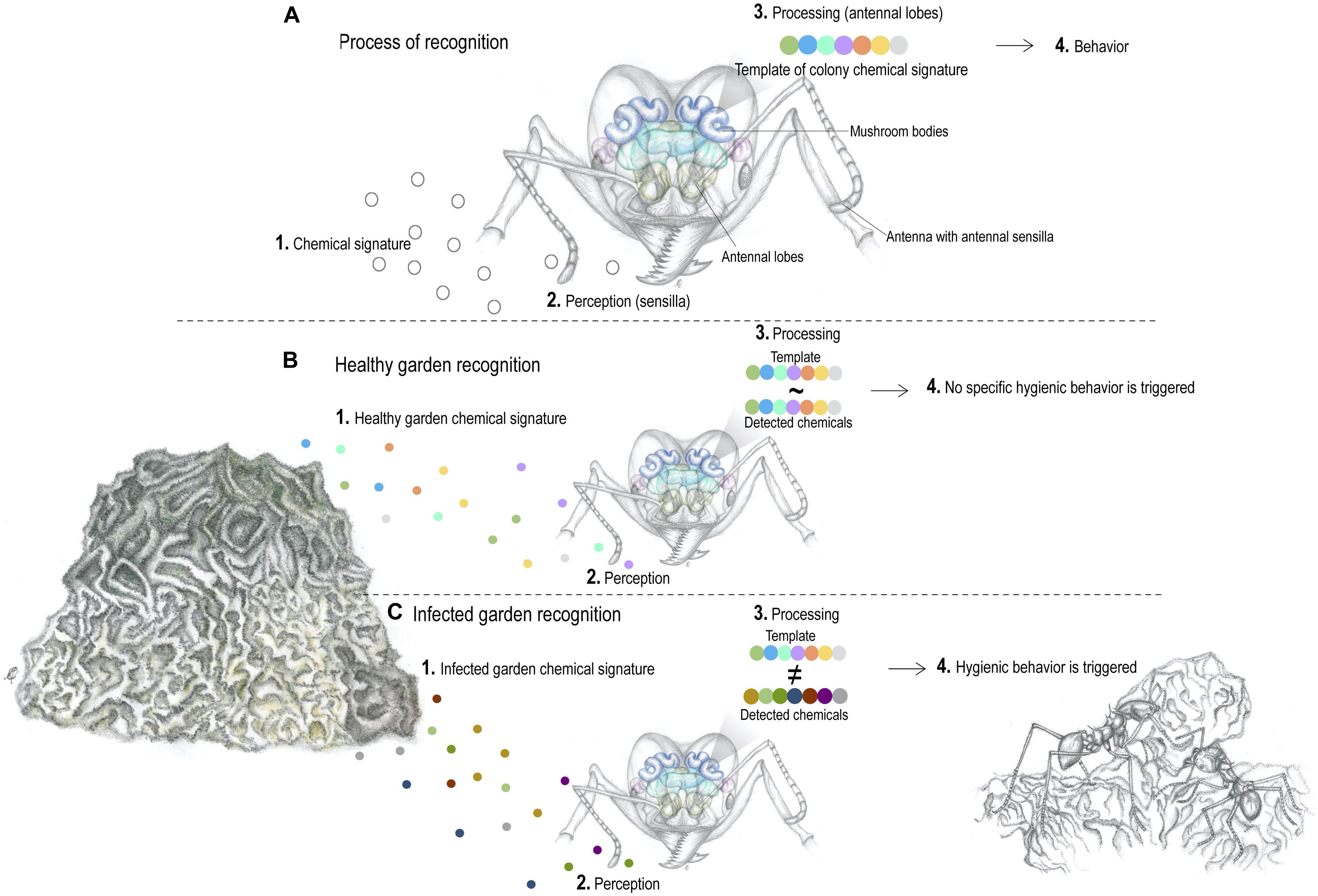
Figure 1. Proposed mechanism for detecting harmful interactions with microbes. (A) In a generalized process of recognition, (1) the chemical signature of the detected organism (that could include the garden, nestmates, non-nestmates, larvae, and microbes) is (2) perceived by workers’ antennal sensilla. (3) In the antennal lobes, the detected label is processed by comparison to the template of colony odor, a neural pattern that workers have stored (as memory) in the mushroom bodies. Depending on the label-template differences, (4) hygienic behaviors may or may not be triggered. (B) (1) A healthy garden chemical signature is (2) perceived by the antennal sensilla and (3) processed as similar to the memorized template, (4) not triggering hygienic behaviors. (C) (1) Harmful interactions may result in a particular chemical signature, (2) which is perceived by the antennal sensilla and (3) processed in the antennal lobe as different from the template, (4) triggering specific hygienic behavior for removing the pathogen and/or the infected area. Pencil drawings by Mariana O. Barcoto: ant head was adapted from a photograph by Casey Richart (http://bit.ly/2OZ6Oyf); ant brain was adapted from Mizunami et al. (2010); composition of ant head and brain was inspired on Bos and d’Ettorre (2012); and the two ants on the fungus garden were adapted from a photograph by Don Parsons (http://bit.ly/3bKgAOi).
Fungus gardens are nutritionally rich environments (Martin et al., 1969; Huang et al., 2014), harboring a wide diversity of microorganisms including bacteria, yeasts, and filamentous fungi (Currie, 2001a; Rodrigues et al., 2005, 2008, 2011; Sen et al., 2009; Scott et al., 2010; Suen et al., 2010; Aylward et al., 2013). These microbes may access the fungus garden in different ways, such as via the foraged plant material and from the belowground surroundings. Endophytic fungi (fungi for which part of their life cycle takes place within plant tissue) were thought to interact with the fungus gardens as neutral transients (Poulsen and Currie, 2006). However, some authors suggest that these fungi are potential antagonists (nutritional competitors or pathogens) of the fungal crops (Van Bael et al., 2009, 2012; Mighell and Van Bael, 2016). Besides the presence of endophytes, soil-borne fungi in the genera Fusarium, Syncephalastrum, Trichoderma, and Cunninghamella were isolated from fungus gardens of Atta sexdens and Acromyrmex species (Rodrigues et al., 2005, 2008). Bacteria and yeasts also contribute to the complex and diverse microbiota of the fungus gardens (Craven et al., 1970; Carreiro et al., 1997, 2004; Rodrigues et al., 2009; Scott et al., 2010; Kellner et al., 2015).
Although the functional capacity is undefined for most of the microorganisms found in the ant fungus-growing system, some microbes are considered symbionts (Currie et al., 1999a; Pinto-Tomás et al., 2009; Sen et al., 2009; Suen et al., 2010; Aylward et al., 2013). For instance, fungi in the genus Escovopsis (Ascomycota: Hypocreales) are considered specialized antagonists of the fungus garden (Currie et al., 1999a) and are reported to negatively impact colony health (Currie, 2001b). Tripartite coevolution between the ants, the cultivated fungi and Escovopsis species are supported by patterns of phylogenetic congruence (Currie et al., 2003; Gerardo et al., 2006b). Thus, harmful potential of Escovopsis possibly has regulated the leaf-cutting ants’ defenses on an evolutionary scale. Indeed, ant workers employ physiological and behavioral strategies when the garden is infected by Escovopsis conidia (Currie and Stuart, 2001; Abramowski et al., 2011; Nilssøn-Moller et al., 2018).
Actinobacteria in the genus Pseudonocardia and in other genera play a role in the attine ants’ defensive strategies (Currie et al., 1999b; Mueller et al., 2008; Poulsen et al., 2010; Li et al., 2018). Antimicrobial compounds produced by Actinobacteria protect workers and the fungus garden from infection and dispersal of pathogenic microbes, including Escovopsis (Currie et al., 1999b, 2003; Oh et al., 2009; Sen et al., 2009; Mattoso et al., 2012). For several attine ant species, these bacteria are maintained in cuticular structures (e.g., tubercles, tubercles within crypts) on the ant’s exoskeleton, nourished by glandular secretions (Currie et al., 2006). Cuticular structures that house Actinobacteria and where these bacteria are located on the ant integument vary per ant genus (Li et al., 2018). Evidence supports the association between Actinobacteria and attine ants evolved close to the origin of fungus-farming by ants, even though this mutualistic symbiosis has been lost multiple times over the evolutionary time (Currie et al., 2006; Li et al., 2018). While Acromyrmex species host abundant Pseudonocardia layers on their integuments, these bacteria are found in low frequency (or even absent) on the integument of Atta species (Currie et al., 2006; Marsh et al., 2013; Li et al., 2018). This could suggest that Atta species have replaced the use of Actinobacteria defenses by alternative mechanisms, including the application of glandular chemical compounds and intricate behavioral strategies to physically remove pathogens (Currie and Stuart, 2001; Fernández-Marín et al., 2009; Yek et al., 2012). However, the coevolution of Pseudonocardia with the ants and Escovopsis is debated, and our knowledge is still limited on how the diversity of these bacteria is distributed on individual ants as well as within colonies (Mueller et al., 2008, 2010; Andersen et al., 2013).
Defensive Strategies in Leaf-Cutting Ant Societies
Managing disease outbreaks is a central aspect of the ant–fungal symbiosis (Currie and Stuart, 2001; Hart et al., 2002). Besides the antimicrobial compounds produced by Actinobacteria (Currie et al., 1999b; Oh et al., 2009; Sen et al., 2009), the fungal crop potentially controls pathogen growth. The fungal cultivar of Apterostigma auriculatus was reported to inhibit the in vitro growth of Escovopsis (Gerardo et al., 2006a), and the fungal cultivar of Atta colombica was able to inhibit the growth of several endophytic fungi, including Glomerella cingulata (Van Bael et al., 2009). This inhibition could involve compounds with antimicrobial properties, as observed for the cultivar of Cyphomyrmex ants, which produces lepiochlorin (Hervey and Nair, 1979) and diketopiperazines (Wang et al., 1999). An additional defensive barrier could be constituted by cultivar-secreted laccases (De Fine Licht et al., 2013), detoxifying secondary metabolites produced by antimicrobial-producing antagonists (Divya and Sadasivan, 2016).
Beyond antimicrobial barriers from the fungus garden and associated symbionts, multiple hygienic behaviors represent a key part of attine ants’ social immunity for avoiding the spread of diseases in the colony (Currie and Stuart, 2001; Fernández-Marín et al., 2013). Ant workers monitor the foraged substrate, the fungus garden, brood, and nestmates for disease traits, employing diverse strategies to deal with infections (Currie and Stuart, 2001; Poulsen et al., 2002; Fernández-Marín et al., 2006, 2013; Little et al., 2006; Rocha et al., 2014). Some of these strategies are hygienic behaviors commonly performed by insects, such as grooming contaminated body areas. Ants employ grooming by rubbing one or more legs at different parts of their bodies, thus targeting themselves (self-grooming). Besides, social insects can groom each other (allogrooming) removing contaminants from body areas difficult to access by self-grooming (Schmid-Hempel, 1998; Morelos-Juárez et al., 2010; Fernández-Marín et al., 2013; Zhukovskaya et al., 2013) or from the immature castes. The grooming behavior, common to nestmates inside the colony, may be more frequent for those ants returning from foraging (Richard and Errard, 2009; Morelos-Juárez et al., 2010). For instance, Acromyrmex subterraneus foragers spend more time on self-grooming than non-foragers, presumably due to their recurrent contact with microbial contaminants (Richard and Errard, 2009). Spatial avoidance of both contaminated environments and sick nestmates may also reduce the risk of infection (Stroeymeyt et al., 2014; Quevillon et al., 2015; Tranter et al., 2015). Microbial infections are additionally controlled through antimicrobial secretions from workers’ metapleural glands (Fernández-Marín et al., 2006, 2015), a complex glandular structure exclusive to ants (Yek and Mueller, 2011). Workers use characteristic movements of their forelegs in the metapleural gland opening, transferring gland secretions to contaminated areas (Fernández-Marín et al., 2006, 2013).
Prophylactic behavior during the selection and preparation of plant substrate is equally important to prevent (or decrease) infection risks (Quinlan and Cherrett, 1977; Mangone and Currie, 2007; Van Bael et al., 2012; Rocha et al., 2017). Insects tend to avoid foraging sites and food that is contaminated by parasites or pathogens (de Roode and Lefèvre, 2012), as reported for leaf-cutting ants (Acromyrmex echinatior, Tranter et al., 2015; A. sexdens, Rocha et al., 2017) and for fungus-growing termites (Macrotermes natalensis, Bodawatta et al., 2019). Thus, choosing and preparing plant substrates for the fungus garden may be fundamental to avoid the introduction of alien microbes. This is also true for endophytes, because leaf-cutting ants spend more time processing leaves with high endophyte loads than those with a low abundance. The presence of endophytes may also influence ants’ foraging preferences, as they tend to collect leaf material containing a low abundance of endophytes (Cobletz and Bael, 2013). For instance, workers avoid plant substrates enriched with Trichoderma species (Rocha et al., 2014, 2017), a recurrent endophytic fungus and potential antagonist of the fungal cultivar (Ortiz and Orduz, 2000; Silva et al., 2006). Besides the surveillance of what is entering the colony, it is also important to control what is being thrown away. Waste management by leaf-cutting ants is an important task to prevent the access of already removed microbes and reinfection with contaminated material (Bot et al., 2001a). Old or infected pieces of fungus garden, dead brood, corpses, and even dried or unsuitable leaves are carried away to underground dumps (Autuori, 1947; Hart and Ratnieks, 2001) or disposed above the soil far away from the colony in some leaf-cutting ant species (Weber, 1972). Waste workers do not access garden chambers, preventing the introduction of microbes of the refuse material in the fungus garden (Bot et al., 2001a).
Considering the central role of the fungal crop for fungus-growing insects, it is reasonable to consider that individual and group-level mechanisms may have evolved to avoid disease outbreaks in the fungus garden, comprising an important trait of their social immunity. Leaf-cutting ants combine diverse chemical and behavioral mechanisms to protect the fungus gardens from infective pathogens. Chemical defenses involve ants applying secretions of their metapleural and labial glands, known for exhibiting fungistatic, fungicidal, and bacteriostatic activity, to prevent the growth of entomopathogenic microbes (Graystock and Hughes, 2011). Gland secretions are also applied to the fungus garden surface, inhibiting the development of recurrent antagonistic microbes (Ortius-Lechner et al., 2000; Bot et al., 2002; Poulsen et al., 2002; Fernández-Marín et al., 2003, 2006, 2015). When facing contaminations on the fungus garden, leaf-cutting workers may use behaviors such as grooming the garden by “licking” possibly contaminated areas (Currie and Stuart, 2001). They can also transplant a healthy piece of fungus garden to an infected area (known as fungus-planting behavior; Fernández-Marín et al., 2013). Depending on the extent of the contaminated area, ants may employ weeding, a multiple-step behavior performed as an effort to restrain an established garden infection (Currie and Stuart, 2001; Barcoto et al., 2017; Nilssøn-Moller et al., 2018). During weeding, minima workers chew the edges of contaminated garden fragments, holding and pulling until the fragment is detached, ultimately being carried to the waste chamber (Currie and Stuart, 2001). It is worth to note that the majority of these behaviors are observed in experimental fungal infections, especially against fungal contaminants that normally are found in this environment, including Escovopsis (Currie and Stuart, 2001; Fernández-Marín et al., 2006; Barcoto et al., 2017; Nilssøn-Moller et al., 2018; Bonadies et al., 2019).
Fine-tuned mechanisms for detecting and recognizing microbial threats to the fungus garden may be an important part of social immunity, modulating defensive strategies that allow an early avoidance and reduce the cost of infection (Cremer et al., 2007; Meunier, 2015; Tranter et al., 2015). As discriminating mutualistic microbes from antagonistic ones might be a recurrent task in a fungicultural system, an efficient recognition process may be required for the ants to decide which mechanism of their social immunity is the most suitable for a specific situation (Cremer et al., 2007). In leaf-cutting ants, workers present specific responses toward harmful microbes, preferentially removing from the colony those that could cause damage (Currie and Stuart, 2001; Mighell and Van Bael, 2016). Although ant workers are reported to detect infections threatening the colony (Currie and Stuart, 2001; Abramowski et al., 2011; Gerstner et al., 2011; Mighell and Van Bael, 2016; Rocha et al., 2014, 2017; Tranter et al., 2015), the specific mechanism behind the recognition of distinct microbes remains unclear. In this context, we pose the following questions: (1) How are the processes of detection and recognition of microbial threats triggered and executed? (2) Does the fungus garden influence these processes? In the following sections, we discuss possible scenarios that could explain how ants recognize and discriminate microbes that are harmful to the fungus garden.
Discriminating Between Microbes
Through the Chemical Profiles of the Fungus Garden and Alien Microbes
Each fungus-growing ant colony has a particular odor (Jaffé and Villegas, 1985; Hernández et al., 2006; Richard et al., 2007a; Nehring et al., 2011). As the chemical blends from the garden have a higher diversity of compounds than the chemical blends of workers and brood, the fungal crop possibly influences the colony odor (Bot et al., 2001b; Richard et al., 2007a, b). Ants probably recognize these chemical cues and discriminate between their resident fungal cultivar and that of sympatric colonies (Bot et al., 2001b; Viana et al., 2001; Poulsen and Boomsma, 2005). Fungal crops of closely related ant species (e.g., Ac. octospinosus and Ac. echinatior) produce a similar set of compounds but in different concentrations, suggesting that the ants’ process of recognition may be fine-tuned to qualitative and quantitative differences in the fungal chemical profile (Bot et al., 2001b; Viana et al., 2001; Richard et al., 2007a; Valadares et al., 2015). Also, the discrimination of volatile organic compounds (VOCs) seems to be used by insects to recognize and select their mutualistic fungus strain (Bot et al., 2001b; Viana et al., 2001; Mueller et al., 2004; Richard et al., 2007a), as demonstrated for some Macrotermitidae species that collect fungal spores from the environment every new generation (Biedermann and Kaltenpoth, 2014). The termites’ fine-tuned ability to localize and recognize their mutualistic fungus is probably guided by specific odors (Biedermann and Kaltenpoth, 2014). Nevertheless, fungus-growing termites can distinguish scent profiles from their mutualistic and that from invasive fungus, rejecting the weedy fungus after recognition (Katariya et al., 2017).
Considering the diverse VOCs produced by microbes (Schulz and Dickschat, 2007; Feofilova et al., 2012; Morath et al., 2012; Davis et al., 2013; Schulz-Bohm et al., 2017) that could act as signaling molecules for insects (Rohlfs et al., 2005; Davis et al., 2013), we inquire whether leaf-cutting ants may distinguish between alien microbes and their mutualistic fungus by recognizing VOCs or chemical cues. The ants could detect volatile compounds or surface chemicals of invasive microbes, discriminating a chemical signature that does not match that of their colony, then triggering hygienic responses (Figure 1). Therefore, what has been reported as “specific removal” or “specific hygienic responses” (Currie and Stuart, 2001; Tranter et al., 2015; Mighell and Van Bael, 2016) could be related not only to the threat level of an alien microbe in the fungus garden but also to their distinct chemical profile. Future assays offering only “scents” from different microorganisms to leaf-cutting ant colonies could unveil if detection only depends on VOCs, or whether the presence of physical structures (e.g., spores, mycelia, or bacteria cells) is also required. Hence, the quantification of avoidance or repellence for each bait could clarify the potential of recognition. Also, electroantennogram assays are plausible to compare responses from workers’ antennae (receptor and action potentials) regarding the presence of different microbe species, seeking for species-specific odor detection. In cases where the microbe has coevolved with the leaf-cutting ants’ fungiculture, like the genus Escovopsis (Currie et al., 2003; Gerardo et al., 2006b), research on the detection based on chemical profiles must be taken with caution. The recognition process of such microorganisms could be a result of genetically determined neurophysiological mechanisms that trigger a cascade of physiological reactions in ant workers, resulting in immediate actions to remove it. Therefore, comparative studies toward different strains of Escovopsis species and microbes that did not coevolve within the system will help to understand patterns in overall gene expression (transcriptome) during the ants’ responses.
Through Fungus Garden Semiochemicals
Leaf-cutting ant workers are capable of recognizing changes in the physiological conditions of the fungal cultivar (Ridley et al., 1996; North et al., 1999; Herz et al., 2008). When incorporating a substrate unsuitable for the cultivar (e.g., toxic leaves and baits containing fungicide), workers avoid foraging for this substrate for several weeks, even if it is not harmful to the ants themselves (Ridley et al., 1996; North et al., 1997, 1999; Herz et al., 2008; Thiele et al., 2014). Because workers cease to forage for the harmful substrate after recognizing the damage in the fungus garden, the avoidance comprises a phenomenon known as delayed rejection (Herz et al., 2008; Saverschek et al., 2010; Saverschek and Roces, 2011; Arenas and Roces, 2016a, b, 2017). The delayed avoidance of particular plant substrates suggests that the response is influenced by the fungus garden (Ridley et al., 1996; Herz et al., 2008). Such modulation can be explained by chemical compounds produced during harmful interactions, which may be recognized by ant workers, thus acting as semiochemicals (chemicals that convey a message from one organism to another; Knapp et al., 1990; Ridley et al., 1996; North et al., 1999; Green and Kooij, 2018).
We speculate that a similar mechanism could be involved in the ants’ recognition of harmful microbes, triggering a generalist response by the colony. Negative interactions between the fungal cultivar and antagonistic microbes could be communicated to ant workers via detectable modifications on the chemical profile of the fungus garden, acting as semiochemicals (Green and Kooij, 2018). During cultivar–pathogen interactions, defensive metabolites or incompatibility compounds produced by the cultivar (Poulsen and Boomsma, 2005; Gerardo et al., 2006a), derived products of hyphae breakdown (North et al., 1999), and even responses to metabolites released by the pathogen (Dhodary et al., 2018; Heine et al., 2018) could shift the fungus-garden chemical profile. These alterations would be processed in the antennal lobes by comparing the detected blend to the colony template memorized by the ant. By differing from the colony template, the chemical from fungus gardens’ infected portions would trigger hygienic behaviors (Figure 1). As above, discrimination of microbes would happen when semiochemicals are released from negative interactions. Mechanisms by which the fungal crop signalizes harmful interactions, as well as the compounds involved in this process, remain unclear (Green and Kooij, 2018). Analyzing metabolites produced by both “infected” and “uninfected” cultivars may reveal context-dependent molecules, which can be tested for having a direct influence on the ant’s behavior (e.g., a semiochemical role). A whole branch of research could be derived from investigating the evolution of ant–fungus communication and its influence on social immunity.
Through Associative Learning
Leaf-cutting ant workers learn to differentiate between suitable and unsuitable leaf substrates mainly through the olfactory system, associating the fungal crop response to the chemical profile of the foraged substrate (Herz et al., 2008; Saverschek et al., 2010). Chemical information characterizing the unsuitable substrate is stored in the ants’ brain as “olfactory memory,” coding a long-term memory that will be retrieved once the same detrimental plant is collected (Herz et al., 2008; Saverschek et al., 2010; Saverschek and Roces, 2011; Falibene et al., 2015). Learning from olfactory experience and formation of associative memories involve structural remodeling of brain centers for sensory integration and association, such as the mushroom bodies (Galizia and Rössler, 2010; Falibene et al., 2015). When leaf-cutting ants learn how to differentiate between substrates according to the suitability to the fungal crop, the development of long-term associative memories is correlated to transient modifications in the density of synaptic complexes in the mushroom bodies (Falibene et al., 2015). Similarly, chemical signals from the infected garden could be detected by olfactory neuron sensors in the ants’ antennal sensilla, present throughout the ants’ antennae, and ultimately reaching the olfactory glomeruli in the antennal lobe, where the information is processed (Kleineidam et al., 2005; Galizia and Szyszka, 2008; Galizia and Rössler, 2010; Carey and Carlson, 2011). Developing long-term memories associating with the odor of an infection as a threat for the garden health could involve transferring olfactory information from the antennal lobe to the mushroom bodies, where it would promote a reorganization of associative networks (Galizia and Rössler, 2010; Falibene et al., 2015). Thus, we suggest that olfactory associative learning, which comprises the cognitive ability to connect different stimuli and predict relationships between them (Giurfa, 2007; Leadbeater and Chittka, 2007; Dickinson, 2012), could be related to the recognition of harmful microbes.
We postulate that ant workers would learn and memorize the chemical profile of harmful microorganisms, associating it with the response of the fungal crop (Figure 2, step A4). Groups of tending workers that associate chemical cues with detrimental interactions would compose a “colony temporary memory.” Hence, in subsequent contacts with a known pathogen, this mechanism would provide a faster response in grooming contaminated plant debris and foraging workers to prevent pathogens from entering the colony (Figure 2, step B1). If the microbe reaches the fungus garden, additional workers could be recruited to the infected area either by chemically interacting via antennation or by releasing alarm pheromones (Gerstner et al., 2011) from “memory workers” (Figure 2, step B2). Alternatively, in cases in which microbes never had caused negative outcomes before, the ants perhaps are only able to detect its chemical cues. Thus, strategies for preventing the infection would be more generalized (e.g., applying antimicrobials secreted from metapleural glands and microbe removal through fungus grooming).
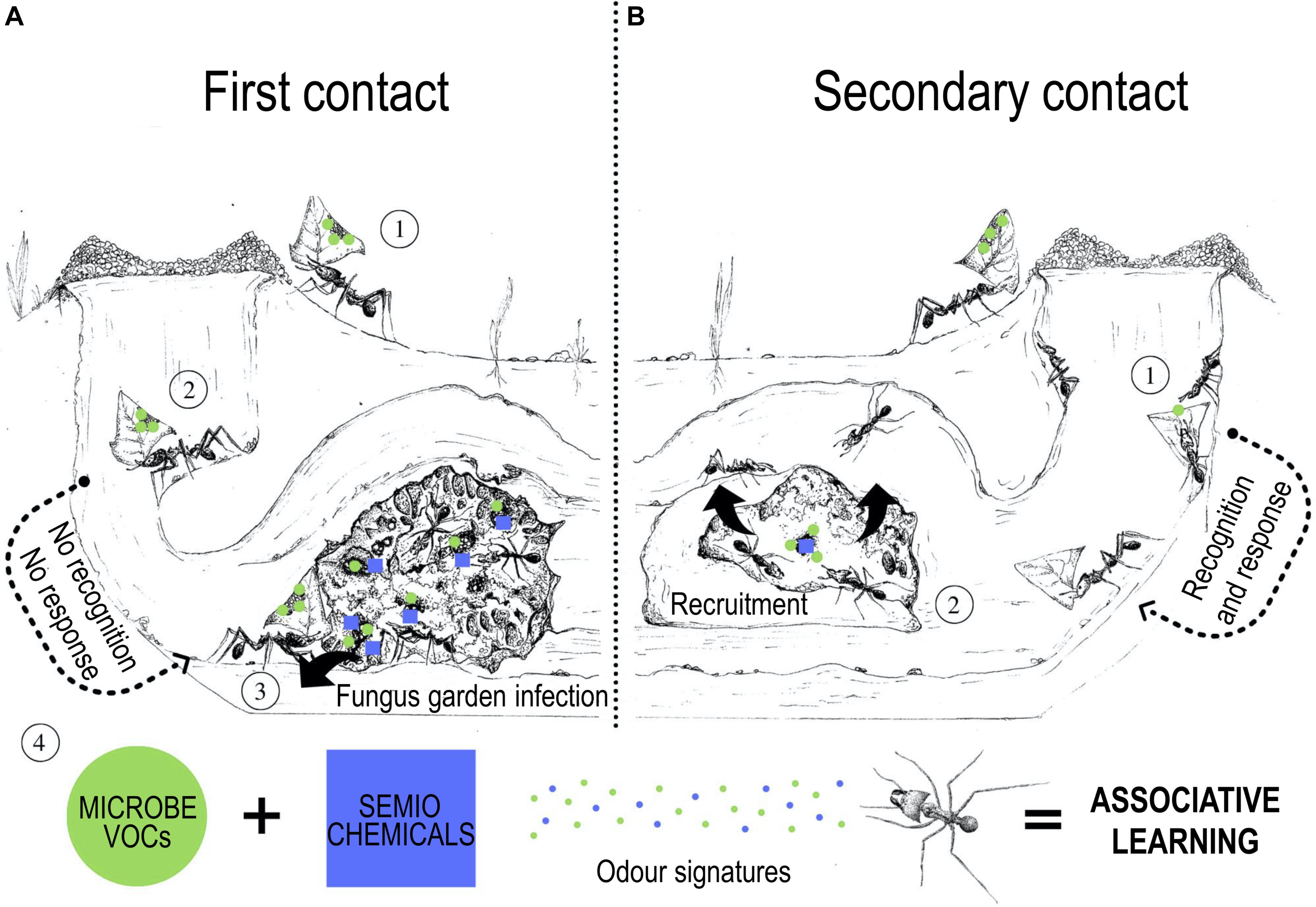
Figure 2. Associative learning and the “colony temporary memory.” (A) First contact: (1) a forager worker carries inside the colony a microbe on a leaf and/or on its integument. When workers have not experienced previous contact with this microbe, it may not be recognized by workers. Generalized hygienic behaviors may be employed for removing the microbe. If these mechanisms fail, (2) the microbe reaches the fungus garden and potentially harms the fungal cultivar (3). The “damaged area” of the fungus garden would signalize alterations to the tending workers. Recruited workers would interact and experience this feedback, linking it to specific chemical cues (volatile organic compounds - VOCs) of the harmful microbe through associative learning (4). By memorizing the pathogen’s cues, these workers are capable to recognize this microbe in future encounters, associating its presence with damage to the fungus garden. (B) Secondary contacts: (1) in future contacts, workers would be able to recognize and remove the microbe from plant debris or even from themselves, preventing it from reaching the fungus garden. (2) In cases where the same harmful microbe reaches the fungal crop, workers who learned from previous contacts would recruit other nestmates (naive workers) for cleaning and removal, increasing the number of experienced ants to recognize this microbe as an antagonist. Therefore, over time and exposure to different antagonist species, the colony would acquire their own “immune memory.” This colony-memory would be temporary, lasting during the lifespan of these workers, therefore putatively reprocessed during the lifetime of the colony. Illustration by Beatriz Garcia Gonçalves.
For further investigation of this hypothesis, studies involving the structural alterations of the mushroom bodies (Falibene et al., 2015), in experimental setups where the fungus garden is threatened by pathogens, could answer questions on neurological activities and expressions during defensive responses in an individual-level perspective. Also, genomic and transcriptomic tools on active workers defending the fungus garden, or assays where the ants are exposed only to the pathogen, could fill a gap in our knowledge about physiological and genetic traits involved in their social immunity. For a colony-level perspective, responses could be verified through repeated inoculation of antagonistic microbes in the fungus garden, seeking evidence for learning processes, colony-memory to recognize the same pathogen or even “immunization” (Traniello et al., 2002; Ugelvig and Cremer, 2007; Walker and Hughes, 2009; Konrad et al., 2012).
Conclusion
Ant–fungal communication and ants’ ability to detect and recognize pathogens have played a key role in the success of the fungus-growing ants’ symbiosis. Future research should address the ant–garden communication and defensive strategies across the attine ant lineages, investigating the evolutionary history of these mechanisms. Also, it remains unclear whether the defensive responses target specific pathogens genera or species and whether the hygienic behaviors and frequency of responses would vary accordingly. In an attempt to address such gaps, here we discussed the possible role of associative learning (to experience which microbes could be harmful to the fungus garden) and how chemicals could lead to microbe-specific recognition. The proposed mechanisms can be considered frameworks to build experiments to understand how ants defend fungus gardens against harmful microbes. However, we cannot predict how costly or beneficial each of these mechanisms would be at both the individual and society levels. Nevertheless, addressing possibilities regarding learning due to recurrent infection to increase the survival and fitness of the colony will open new areas in social immunity knowledge. As pointed out in this review, we have only just begun to understand how social immunity evolved in leaf-cutting ants, and there is still a long way to go before we can form a full picture of the process from encountering a microbe to applying defenses.
Author Contributions
AG and MB conceptualized the hypothesis and reviewed the literature. AG, MB, PK, OB, and AR thoroughly discussed the hypothesis, wrote and reviewed the draft versions of the manuscript. MB conceived Figure 1. AG, MB, PK, and AR wrote the final manuscript. All authors approved the final version of the manuscript.
Funding
AR received funding from FAPESP (Fundação de Amparo à Pesquisa do Estado de São Paulo), grants # 2019/03746-0 and #2012/25299-6. AG received a scholarship from FAPESP (# 2019/03087-6). PK received funding from CAPES-PrInt (grant #88887.468939/2019-00).
Conflict of Interest
The authors declare that the research was conducted in the absence of any commercial or financial relationships that could be construed as a potential conflict of interest.
Acknowledgments
We express our gratitude to Irina Jiménez Gómez, Karina Bueno de Oliveira, Maria Jesus Sutta Martiarena, Quimi Vidaurre Montoya, Rodolfo Bizarria Jr., and Laurence Marianne Vincianne Culot for collaborating with thoughts and discussions during the construction of this manuscript. We would like to thank Casey Richart, John Longino, and Don Parsons for permission to adapt the photos cited in the legend of Figure 1 and to Beatriz Garcia Gonçalves for illustrating Figure 2. Finally, we are grateful to the two reviewers for their constructive criticism and input in this manuscript.
References
Aanen, D. K., Eggleton, P., Rouland-Lefèvre, C., Guldberg-Frøslev, T., Rosendahl, S., and Boomsma, J. J. (2002). The evolution of fungus-growing termites and their mutualistic fungal symbionts. Proc. Natl. Acad. Sci. U.S.A. 99, 14887–14892. doi: 10.1073/pnas.222313099
Abramowski, D., Currie, C. R., and Poulsen, M. (2011). Caste specialization in behavioral defenses against fungus garden parasites in Acromyrmex octospinosus leaf-cutting ants. Insect. Soc. 58, 65–75. doi: 10.1007/s00040-010-0117-y
Andersen, S. B., Hansen, L. H., Sapountzis, P., Sørensen, S. J., and Boomsma, J. J. (2013). Specificity and stability of the Acromyrmex-Pseudonocardia symbiosis. Mol. Ecol. 22, 4307–4321. doi: 10.1111/mec.12380
Andersen, S. B., Yek, S. H., Nash, D. R., and Boomsma, J. J. (2015). Interaction specificity between leaf-cutting ants and vertically transmitted Pseudonocardia bacteria. BMC Evol. Biol. 15:27. doi: 10.1186/s12862-015-0308-2
Arenas, A., and Roces, F. (2016a). Learning through the waste: olfactory cues from the colony refuse influence plant preferences in foraging leaf-cutting ants. J. Exp. Biol. 219, 2490–2496. doi: 10.1242/jeb.139568
Arenas, A., and Roces, F. (2016b). Gardeners and midden workers in leaf-cutting ants learn to avoid plants unsuitable for the fungus at their worksites. Anim. Behav. 115, 167–174. doi: 10.1016/j.anbehav.2016.03.016
Arenas, A., and Roces, F. (2017). Avoidance of plants unsuitable for the symbiotic fungus in leaf-cutting ants: learning can take place entirely at the colony dump. PLoS One 12:1–16. doi: 10.1371/journal.pone.0171388
Autuori, M. (1947). Contribuição para o conhecimento da saúva (Atta spp. IV). O sauveiro depois da primeira revoada (Atta sexdens rubropilosa Forel, 1908). Arq. Inst. Biol. 18, 39–70.
Aylward, F. O., Burnum-Johnson, K. E., Tringe, S. G., Teiling, C., Tremmel, D. M., Moeller, J. A., et al. (2013). Leucoagaricus gongylophorus produces diverse enzymes for the degradation of recalcitrant plant polymers in leaf-cutter ant fungus gardens. Appl. Env. Microbiol. 79, 3770–3778. doi: 10.1128/AEM.03833-12
Barcoto, M. O., Pedrosa, F., Bueno, O. C., and Rodrigues, A. (2017). Pathogenic nature of Syncephalastrum in Atta sexdens rubropilosa fungus gardens. Pest Manag. Sci. 73, 999–1009. doi: 10.1002/ps.4416
Bass, M., and Cherret, J. M. (1996). Leaf-cutting ants (Formicidae, Attini) prune their fungus to increase and direct its productivity. Funct. Ecol. 10, 55–61. doi: 10.2307/2390262
Beemelmaans, C., Ramadhar, T. R., Kim, K. H., Klassen, J. L., Cao, S., Wyche, T. P., et al. (2017). Macrotermycins A–D, glycosylated macrolactams from a termite-associated Amycolatopsis sp. M39. Org. Lett. 19, 1000–1003. doi: 10.1021/acs.orglett.6b03831
Biedermann, P. H., and Kaltenpoth, M. (2014). New synthesis: the chemistry of partner choice in insect-microbe mutualisms. J. Chem. Ecol. 40, 99–99. doi: 10.1007/s10886-014-0382-8
Biedermann, P. H., and Rohlfs, M. (2017). Evolutionary feedbacks between insect sociality and microbial management. Curr. Opin. Insec. Sci. 22, 92–100. doi: 10.1016/j.cois.2017.06.003
Bodawatta, K., Poulsen, M., and Bos, N. (2019). Foraging Macrotermes natalensis fungus-growing termites avoid a mycopathogen but not an entomopathogen. Insects 10:185. doi: 10.3390/insects10070185
Bonadies, E., Wcislo, W. T., Gálvez, D., Hughes, W. O., and Fernández-Marín, H. (2019). Hygiene defense behaviors used by a fungus-growing ant depend on the fungal pathogen stages. Insects 10:130. doi: 10.3390/insects10050130
Boomsma, J. J., and Gawne, R. (2018). Superorganismality and caste differentiation as points of no return: how the major evolutionary transitions were lost in translation. Biol. Rev. Camb. Philos. Soc. 93, 28–54. doi: 10.1111/brv.12330
Boomsma, J. J., Jensen, A. B., Meyling, N. V., and Eilenberg, J. (2014). Evolutionary interaction networks of insect pathogenic fungi. Annu. Rev. Entomol. 59, 467–485. doi: 10.1146/annurev-ento-011613-162054
Boomsma, J. J., Schimid-Hempel, P., and Hughes, W. O. H. (2005). “Life histories and parasite pressure across the major groups of social insects,” in Insect Evolutionary Ecology, eds M. D. E. Fellowes, G. Holloway, and J. Rolff (Wallingford: CABI Publishing), 139–173.
Bos, N., and d’Ettorre, P. (2012). Recognition of social identity in ants. Front. Psychol. 3:83. doi: 10.3389/fpsyg.2012.00083
Bot, A. N. M., Currie, C. R., Hart, A. G., and Boomsma, J. J. (2001a). Waste management in leaf-cutting ants. Ethol. Ecol. Evol. 13, 225–237. doi: 10.1080/08927014.2001.9522772
Bot, A. N. M., Rehner, S. A., and Boomsma, J. J. (2001b). Partial incompatibility between ants and symbiotic fungi in two sympatric species of Acromyrmex leaf-cutting ants. Evolution 10, 1980–1991. doi: 10.1111/j.0014-3820.2001.tb01315.x
Bot, A. N. M., Ortius-Lechner, D., Finster, K., Maile, R., and Boomsma, J. J. (2002). Variable sensitivity of fungi and bacteria to compounds produced by the metapleural glands of leaf-cutting ants. Insect. Soc. 49, 363–370. doi: 10.1007/PL00012660
Brown, M. J., Bot, A. N. M., and Hart, A. G. (2006). Mortality rates and division of labor in the leaf-cutting ant Atta colombica. J. Insec. Sci. 6, 1–8. doi: 10.1673/2006_06_18.1
Carey, A. F., and Carlson, J. R. (2011). Insect olfaction from model systems to disease control. Proc. Natl. Acad. Sci. U.S.A. 108, 12987–12995. doi: 10.1073/pnas.1103472108
Carreiro, S. C., Pagnocca, F. C., Bacci, M., Lachance, M. A., Bueno, O. C., Hebling, M. J. A., et al. (2004). Sympodiomyces attinorum sp. nov., a yeast species associated with nests of the leaf-cutting ant Atta sexdens. Int. J. Syst. Evol. Microbiol. 54, 1891–1894. doi: 10.1099/ijs.0.63200-0
Carreiro, S. C., Pagnocca, F. C., Bueno, O. C., Bacci, M. Jr., and Hebling, M. J. A. (1997). Yeasts associated with nests of the leaf-cutter ant Atta sexdens rubropilosa Forel, 1908. Antonie Van Leeuwenhoek 71, 243–248. doi: 10.1023/A:1000182108648
Cobletz, K. E., and Bael, S. A. V. (2013). Field colonies of leaf-cutting ants select plant materials containing low abundances of endophytic fungi. Ecosphere 4:art66. doi: 10.1890/ES13-00012.1
Costa, A. N., Vasconcelos, H. L., Vieira-Neto, E. H. M., and Bruna, E. M. (2008). Do herbivores exert top-down effects in Neotropical savannas? Estimates of biomass consumption by leaf-cutter ants. J. Veg. Sci. 19, 849–854. doi: 10.3170/2008-8-18461
Craven, S. E., Dix, M. W., and Michaels, G. E. (1970). Attine fungus gardens contains yeasts. Science 169, 184–186. doi: 10.1126/science.169.3941.184
Cremer, S. (2019). Social immunity in insects. Curr. Biol. 29, R458–R463. doi: 10.1016/j.cub.2019.03.035
Cremer, S., Armitage, S. A. O., and Schmid-Hempel, P. (2007). Social immunity. Curr. Biol. 17, 693–702. doi: 10.1016/j.cub.2007.06.008
Cremer, S., Pull, C. D., and Fürst, A. (2018). Social immunity: emergence and evolution of colony-level disease protection. Annu. Rev. Entomol. 63, 105–123. doi: 10.1146/annurev-ento-020117-043110
Cremer, S., and Sixt, M. (2009). Analogies in the evolution of individual and social immunity. Philos. Trans. Roy. Soc. B 364, 129–142. doi: 10.1098/rstb.2008.0166
Currie, C. R. (2001a). A community of ants, fungi, and bacteria: a multilateral approach to studying symbiosis. Annu. Rev. Microbiol. 55, 357–380. doi: 10.1146/annurev.micro.55.1.357
Currie, C. R. (2001b). Prevalence and impact of a virulent parasite on a tripartite mutualism. Oecologia 128, 99–106. doi: 10.1007/s004420100630
Currie, C. R., Mueller, U. G., and Malloch, D. (1999a). The agricultural pathology of ant fungus gardens. Proc. Natl. Acad. Sci. U.S.A. 96, 7998–8002. doi: 10.1073/pnas.96.14.7998
Currie, C. R., Scott, J. A., and Summerbell, R. C. (1999b). Fungus-growing ants use antibiotic-producing bacteria to control garden parasites. Nature 398, 701–705. doi: 10.1038/19519
Currie, C. R., Poulsen, M., Mendenhall, J., Boomsma, J. J., and Billen, J. (2006). Coevolved crypts and exocrine glands support mutualistic bacteria in fungus-growing ants. Science 311, 81–83. doi: 10.1126/science.1119744
Currie, C. R., and Stuart, A. E. (2001). Weeding and grooming of pathogens in agriculture by ants. Proc. R. Soc. B 268, 1033–1039. doi: 10.1098/rspb.2001.1605
Currie, C. R., Wong, B., Stuart, A. E., Schultz, T. R., Rehner, S. A., Mueller, U. G., et al. (2003). Ancient tripartite coevolution in the attine ant-microbe symbiosis. Science 299, 386–388. doi: 10.1126/science.1078155
Davis, T. S., Crippen, T. L., Hofstetter, R. W., and Tomberlin, J. K. (2013). Microbial volatile emissions as insect semiochemicals. J. Chem. Ecol. 39, 840–859. doi: 10.1007/s10886-013-0306-z
De Fine Licht, H. H., Schiøtt, M., Rogowska-Wrzesinska, A., Nygaard, S., Roepstorff, P., and Boomsma, J. J. (2013). Laccase detoxification mediates the nutritional alliance between leaf-cutting ants and fungus-garden symbionts. Proc. Natl. Acad. Sci. U.S.A. 110, 583–587. doi: 10.1073/pnas.1212709110
de Roode, J. C., and Lefèvre, T. (2012). Behavioral immunity in insects. Insects 3, 789–820. doi: 10.3390/insects3030789
Dhodary, B., Schilg, M., Wirth, R., and Spiteller, D. S. (2018). Secondary metabolites from Escovopsis weberi and their role in attacking the garden fungus of leaf-cutting ants. Chemistry 24, 4445–4452. doi: 10.1002/chem.201706071
Dickinson, A. (2012). Associative learning and animal cognition. Philos. Trans. R. Soc. Lond. B Biol. Sci. 367, 2733–2742. doi: 10.1098/rstb.2012.0220
Diniz, E. A., and Bueno, O. C. (2010). Evolution of substrate preparation behaviors for cultivation of symbiotic fungus in attine ants (Hymenoptera: Formicidae). J. Insec. Behav. 23, 205–214. doi: 10.1007/s10905-010-9207-y
Divya, L., and Sadasivan, C. (2016). Trichoderma viride laccase plays a crucial role in defense mechanism against antagonistic organisms. Front. Microbiol. 7:741. doi: 10.3389/fmicb.2016.00741
Falibene, A., Roces, F., and Rössler, W. (2015). Long-term avoidance memory formation is associated with a transient increase in mushroom body synaptic complexes in leaf-cutting ants. Front. Behav. Neurosci. 9:84. doi: 10.3389/fnbeh.2015.00084
Farrell, B. D., Sequeira, A. S., O’Meara, B. C., Normark, B. B., Chung, J. H., and Jordal, B. H. (2001). The evolution of agriculture in beetles (Curculionidae: Scolytinae and Platypodinae). Evolution 55, 2011–2027. doi: 10.1111/j.0014-3820.2001.tb01318.x
Feofilova, E. P., Ivashechkin, A. A., Alekhin, A. I., and Sergeeva, Y. E. (2012). Fungal spores: dormancy, germination, chemical composition, and role in biotechnology. Appl. Biochem. Microbiol. 48, 1–11. doi: 10.1134/S0003683812010048
Fernández-Marín, H., Bruner, G., Gomez, E. B., Nash, D. R., Boomsma, J. J., and Wcislo, W. T. (2013). Dynamic disease management in Trachymyrmex fungus-growing ants (Attini: Formicidae). Am. Nat. 181, 571–582. doi: 10.1086/669664
Fernández-Marín, H., Nash, D. R., Higginbotham, S., Estrada, C., van Zweden, J. S., d’Ettorre, P., et al. (2015). Functional role of phenylacetic acid from metapleural gland secretions in controlling fungal pathogens in evolutionarily derived leaf-cutting ants. Proc. R. Soc. B. 282:1807. doi: 10.1098/rspb.2015.0212
Fernández-Marín, H., Zimmerman, J. K., Nash, D. R., Boomsma, J. J., and Wcislo, W. T. (2009). Reduced biological control and enhanced chemical pest management in the evolution of fungus farming in ants. Proc. R. Soc. B 276, 2263–2269. doi: 10.1098/rspb.2009.0184
Fernández-Marín, H., Zimmerman, J. K., Rehner, S. A., and Wcislo, W. T. (2006). Active use of the metapleural glands by ants in controlling fungal infection. Proc. R. Soc. B. 273, 1689–1695. doi: 10.1098/rspb.2006.3492
Fernández-Marín, H., Zimmermann, J. K., and Wcislo, W. T. (2003). Nest-founding in Acromyrmex octospinosus (Hymenoptera, Formicidae, Attini): demography and putative prophylactic behaviors. Insect. Soc. 50, 304–308. doi: 10.1007/s00040-003-0687-z
Fisher, P. J., Stradling, D. J., Sutton, B. C., Petrin, A. N. D. L. E., Lane, B., and Two, S. (1996). Microfungi in the fungus gardens of the leaf-cutting ant Atta cephalotes: a preliminary study. Mycol. Res. 100, 541–546. doi: 10.1016/S0953-7562(96)80006-2
Galizia, C. G., and Rössler, W. (2010). Parallel olfactory systems in insects: anatomy and function. Ann. Rev. Entomol. 55, 399–420. doi: 10.1146/annurev-ento-112408-085442
Galizia, C. G., and Szyszka, P. (2008). Olfactory coding in the insect brain: molecular receptive ranges, spatial and temporal coding. Entomol. Exp. Appl. 128, 81–92. doi: 10.1111/j.1570-7458.2007.00661.x
Gerardo, N. M., Jacobs, S. R., Currie, C. R., and Mueller, U. G. (2006a). Ancient host-pathogen associations maintained by specificity of chemotaxis and antibiosis. PLoS Biol. 4:e235. doi: 10.1371/journal.pbio.0040235
Gerardo, N. M., Mueller, U. G., and Currie, C. R. (2006b). Complex host-pathogen coevolution in the Apterostigma fungus-growing ant-microbe symbiosis. BMC Evol. Biol. 6:88. doi: 10.1186/1471-2148-6-88
Gerstner, A. T., Poulsen, M., and Currie, C. R. (2011). Recruitment of minor workers for defense against a specialized parasite of Atta leaf-cutting ant fungus gardens. Ethol. Ecol. Evol. 23, 61–75. doi: 10.1080/03949370.2010.529828
Giurfa, M. (2007). Behavioral and neural analysis of associative learning in the honeybee: a taste from thematic well. J. Comp. Physiol. A Neuroethology. Sens. Neural. Behav. Physiol. 193, 801–824. doi: 10.1007/s00359-007-0235-9
Graystock, P., and Hughes, W. O. H. (2011). Disease resistance in a weaver ant, Polyrhachis dives, and the role of antibiotic-producing glands. Behav. Ecol. Soc. 65, 2319–2327. doi: 10.1007/s00265-011-1242-y
Green, P. W. C., and Kooij, P. W. (2018). The role of chemical signaling in maintenance of the fungus garden by leaf-cutting ants. Chemoecology 28, 101–107. doi: 10.1007/s00049-018-0260-x
Griffiths, H. M., and Hughes, W. O. H. (2010). Hitchhiking and the removal of microbial contaminants by the leaf-cutting ant Atta colombica. Ecol. Entomol. 35, 529–537. doi: 10.1111/j.1365-2311.2010.01212.x
Hart, A. G., Bot, A., and Brown, M. J. (2002). A colony-level response to disease control in leaf-cutter ant. Naturwissenschaften 89, 275–277. doi: 10.1007/s00114-002-0316-0
Hart, A. G., and Ratnieks, F. L. W. (2001). Task partitioning, division of labour and nest compartmentalisation collectively isolate hazardous waste in the leafcutting ant Atta cephalotes. Acta Etholog. 49, 387–392.
Heine, D., Homes, N. A., Worsley, S. F., Santos, A. C. A., Innocent, T. M., Scherlach, K., et al. (2018). Chemical warfare between leafcutter ant symbionts and a co-evolved pathogen. Nat. Commun. 9:2208. doi: 10.1038/s41467-018-04520-1
Henrik, H., Boomsma, J. J., and Tunlid, A. (2014). Symbiotic adaptations in the fungal cultivar of leaf-cutting ants. Nat. Commun. 5:5675. doi: 10.1038/ncomms6675
Hernández, J. V., Goitia, W., Osio, A., Cabrera, A., Lopez, H., Sainz, C., et al. (2006). Leaf-cutter ant species (Hymenoptera: Atta) differ in the types of cues used to differentiate between self and others. Anim. Behav. 71, 945–952. doi: 10.1016/j.anbehav.2005.09.004
Hervey, A., and Nair, M. S. R. (1979). Antibiotic metabolite of a fungus cultivated by gardening ants. Mycologia 71, 1064–1066.
Herz, H., Hölldobler, B., and Roces, F. (2008). Delayed rejection in a leaf-cutting ant after foraging on plants unsuitable for the symbiotic fungus. Behav. Ecol. 19, 575–582. doi: 10.1093/beheco/arn016
Huang, E. L., Aylward, F. O., Kim, Y.-M., Webb-Robertson, B.-J. M., Nicora, C. D., et al. (2014). The fungus gardens of leaf-cutter ants undergo a distinct physiological transition during biomass degradation. Envir. Microbiol. Rep. 6, 389–395. doi: 10.1111/1758-2229.12163
Hughes, W. O. H., Summer, S., Van Borm, S., and Boomsma, J. J. (2003). Worker caste polymorphism has a genetic basis in Acromyrmex leaf-cutting ants. Proc. Natl. Acad. Sci. U. S. A. 100, 9394–9397. doi: 10.1073/pnas.1633701100
Hulcr, J., and Stelinski, L. L. (2017). The ambrosia symbiosis: from evolutionary ecology to practical management. Ann. Rev. Entomo. 62, 285–303. doi: 10.1146/annurev-ento-031616-035105
Jaffé, K., and Villegas, G. (1985). On the communication systems of the fungus-growing ant Trachymyrmex urichi. Insectes Soc. 32, 257–274. doi: 10.1007/BF02224915
Kamhi, J. F., and Traniello, J. F. (2013). Biogenic amines and collective organization in a superorganism: neuromodulation of social behavior in ants. Brain Behav. Evolut. 82, 220–236. doi: 10.1159/000356091
Katariya, L., Ramesh, P. B., Gopalappa, T., Desireddy, S., Bessière, J., and Borges, R. M. (2017). Fungus-farming termites selectively bury weedy fungi that smell different from crop fungi. J. Chem. Ecol. 43, 986–995. doi: 10.1007/s10886-017-0902-4
Kellner, K., Ishak, H. D., Linksvayer, T. A., and Mueller, U. G. (2015). Bacterial community composition and diversity in an ancestral ant fungus symbiosis. FEMS Micro. Ecol. 91:fiv073. doi: 10.1093/femsec/fiv073
Khadempour, L., Burnum-Johnson, K. E., Baker, E. S., Nicora, C. D., Webb-Robertson, B. M., White, R. A. III, et al. (2016). The fungal cultivar of leaf-cutter ants produces specific enzymes in response to different plant substrates. Mol. Ecol. 25, 5795–5805. doi: 10.1111/mec.13872
Kleineidam, C. J., Obermayer, M., Halbich, W., and Rössler, W. (2005). A macroglomerulus in the antennal lobe of leaf-cutting ant workers and its possible functional significance. Chem. Senses 30, 383–392. doi: 10.1093/chemse/bji033
Knapp, J. J., Howse, P. E., and Kermarrec, A. (1990). “Factors controlling foraging patterns in the leaf-cutting ant Acromyrmex octospinosus (Reich),” in Applied Myrmecology: a World Perspective, eds R. K. Vander Meer, K. Jaffe, and A. Cedeno (Boulder, CO: Westview Press), 382–409.
Konrad, M., Pull, C. D., Metzler, S., Seif, K., Nederlinger, E., Grasse, A. V., et al. (2018). Ants avoid superinfections by performing risk-adjusted sanitary care. Proc. Natl. Acad. Sci. U.S.A. 115, 2782–2787. doi: 10.1073/pnas.1713501115
Konrad, M., Vyleta, M. L., Theis, F. J., Stock, M., Tragust, S., Klatt, M., et al. (2012). Social transfer of pathogenic fungus promotes active immunization in ant colonies. PLoS Biol. 10:e1001300. doi: 10.1371/journal.pbio.1001300
Leadbeater, E., and Chittka, L. (2007). Social learning in insects – from miniature brains to consensus building. Curr. Biol. 17, R703–R713. doi: 10.1016/j.cub.2007.06.012
Li, H., Sosa-Calvo, J., Horn, H. A., Pupo, M. P., Clardy, J., Rabeling, C., et al. (2018). Convergent evolution of complex structures for ant–bacterial defensive symbiosis in fungus-farming ants. Proc. Natl. Acad. Sci. U.S.A. 115, 10720–10725. doi: 10.1073/pnas.1809332115
Little, A. E. F., Murakami, T., Mueller, U. G., and Currie, C. R. (2006). Defending against parasites: fungus-growing ants combine specialized behaviours and microbial symbionts to protect their fungus gardens. Biol. Lett. 2, 12–16. doi: 10.1098/rsbl.2005.0371
Liu, L., Li, G., Sun, P., Lei, C., and Huang, Q. (2015). Experimental verification and molecular basis of active immunization against fungal pathogens in termites. Sci. Rep. 5:15106. doi: 10.1038/srep15106
Liu, L., Zhao, X.-Y., Tang, Q.-B., Lei, C. L., and Huang, Q. Y. (2019). The mechanisms of social immunity against fungal infections in eusocial insects. Toxins 11:244. doi: 10.3390/toxins11050244
Loreto, R. G., Elliot, S. L., Freitas, M. L. R., Pereira, T. M., and Hughes, D. P. (2014). Long-term disease dynamics for a specialized parasite of ant societies: a field study. PLoS One 9:e103516. doi: 10.1371/journal.pone.0103516
Malagocka, J., Eilenberg, J., and Jensen, A. B. (2019). Social immunity behaviour among ants infected by specialist and generalist fungi. Curr. Opin. Insec. Sci. 33, 99–104. doi: 10.1016/j.cois.2019.05.001
Mangone, D. M., and Currie, C. R. (2007). Garden substrate preparation behaviours in fungus-growing ants. Can. Entomol. 139, 841–849. doi: 10.4039/n06-105
Marsh, S. E., Poulsen, M., Gorosito, N. B., Pinto-Tomás, A. A., Masiulionis, V. E., and Currie, C. R. (2013). Association between Pseudonocardia symbionts and Atta leaf-cutting ants suggested by improved isolation methods. Int. Microbiol. 16, 17–25. doi: 10.2436/20.1501.01.176
Martin, M. M., Macconnell, J. G., and Gale, G. R. (1969). The chemical basis for the attine ant-fungus symbiosis. Absence of antibiotics. Ann. Entomol. Soc. Am. 62, 386–388. doi: 10.1093/aesa/62.2.386
Mattoso, T. C., Moreira, D. D. O., and Samuels, R. I. (2012). Symbiotic bacteria on the cuticle of the leaf-cutting ant Acromyrmex subterraneus subterraneus protect workers from attack by entomopathogenic fungi. Biol. Lett. 8, 461–464. doi: 10.1098/rsbl.2011.0963
Meirelles, L. A., McFrederick, Q. S., Rodrigues, A., Mantovani, J. D., Rodovalho, C. M., Ferreira, H., et al. (2016). Bacterial microbiomes from vertically transmitted fungal inocula of the leaf-cutting ant Atta texana. Environ. Microbiol. Rep. 8, 630–640. doi: 10.1111/1758-2229.12415
Meunier, J. (2015). Social immunity and the evolution of group living in insects. Philos. Trans. R. Soc. B. 370:20140102. doi: 10.1098/rstb.2014.0102
Mighell, K., and Van Bael, S. A. (2016). Selective elimination of microfungi in leaf-cutting ant gardens. Fungal Ecol. 24, 15–20. doi: 10.1016/j.funeco.2016.08.009
Mikheyev, A. S., Mueller, U. G., and Abbot, P. (2010). Comparative dating of attine ant and lepiotaceous cultivar phylogenies reveals coevolutionary synchrony and discord. Am. Nat. 175, E126–E133. doi: 10.1086/652472
Mizunami, A., Yamagata, N., and Nishino, H. (2010). Alarm pheromone processing in the ant brain: an evolutionary perspective. Front. Behav. Neurosci. 4:28. doi: 10.3389/fnbeh.2010.00028
Morath, S. U., Hung, R., and Bennett, J. W. (2012). Fungal volatile organic compounds: a review with emphasis on their biotechnological potential. Fungal Biol. Rev. 26, 73–83. doi: 10.1016/j.fbr.2012.07.001
Morelos-Juárez, C., Walker, T. N., Lopes, J. F. S., and Hughes, O. H. W. (2010). Ant farmers practice proactive personal hygiene to protect their fungus crop. Curr. Biol. 13, 553–554. doi: 10.1016/j.cub.2010.04.047
Mueller, U. C., Gerardo, N. M., Aanen, D. K., Six, D. L., and Schultz, T. R. (2005). The evolution of agriculture in insects. Ann. Rev. Ecol. Evol. Syst. 36, 563–595. doi: 10.1146/annurev.ecolsys.36.102003.152626
Mueller, U. G., Dash, D., Rabeling, C., and Rodrigues, A. (2008). Coevolution between attine ants and actinomycete bacteria: a reevaluation. Evolution 62, 2894–2912. doi: 10.1111/j.1558-5646.2008.00501.x
Mueller, U. G., Ishak, H., Lee, J. C., Sen, R., and Gutell, R. R. (2010). Placement of attine ant-associated Pseudonocardia in a global Pseudonocardia phylogeny (Pseudonocardiaceae, Actinomycetales): a test of two symbiont-association models. Antonie Van Leeuwenhoek 98, 195–212. doi: 10.1007/s10482-010-9427-3
Mueller, U. G., Ishak, H. D., Bruschi, S. M., Smith, C. C., Herman, J. J., Solomon, S. E., et al. (2017). Biogeography of mutualistic fungi cultivated by leafcutter ants. Mol. Ecol. 26, 6921–6937. doi: 10.1111/mec.14431
Mueller, U. G., Poulin, J., and Adams, R. M. M. (2004). Symbiont choice in a fungus-growing ant (Attini. Formicidae). Behav. Ecol. 15, 357–364. doi: 10.1093/beheco/arh020
Mueller, U. G., Rehner, S. A., and Schultz, T. R. (1998). The evolution of agriculture in ants. Science 281, 2034–2038. doi: 10.1126/science.281.5385.2034
Mueller, U. G., Schultz, T. R., Currie, C. R., Adams, R. M., and Malloch, D. (2001). The origin of the attine ant-fungus mutualism. Q. Rev. Biol. 76, 169–197. doi: 10.1086/393867
Naug, D., and Camazine, S. (2002). The role of colony organization on pathogen transmission in social insects. J. Theor. Biol. 214, 427–439. doi: 10.1006/jtbi.2001.2524
Nehring, V., Evison, S. E. F., Santorelli, L. A., d’Ettorre, P., and Hughes, W. O. H. (2011). Kin-informative recognition cues in ants. Proc. R. Soc. B 278, 1942–1948. doi: 10.1098/rspb.2010.2295
Nilssøn-Moller, S., Poulsen, M., and Innocent, T. M. (2018). A visual guide for studying behavioral defenses to pathogen attacks in leaf-cutting ants. J. Vis. Exp. 140:e58420. doi: 10.3791/58420
North, R. D., Jackson, C. W., and Howse, P. E. (1997). Evolutionary aspects of ant-fungus interactions in leaf-cutting ants. Trends Ecol. Evol. 12, 386–389. doi: 10.1016/S0169-5347(97)87381-8
North, R. D., Jackson, C. W., and Howse, P. E. (1999). Communication between the fungus garden and workers of the leaf-cutting ant, Atta sexdens rubropilosa, regarding choice of substrate for the fungus. Physiol. Entomol. 24, 127–133. doi: 10.1046/j.1365-3032.1999.00122.x
Nygaard, S., Hu, H., Li, C., Schiøtt, M., Chen, Z., Yang, Z., et al. (2016). Reciprocal genomic evolution in the ant–fungus agricultural symbiosis. Nat. Commun. 7:12233. doi: 10.1038/ncomms12233
Oh, D. C., Poulsen, M., Currie, C. R., and Clardy, J. (2009). Dentigerumycin: a bacterial mediator of an ant-fungus symbiosis. Nat. Chem. Biol. 5, 391–393. doi: 10.1038/nchembio.159
Ortius-Lechner, D., Maile, R., Morgan, E. D., and Boomsma, J. J. (2000). Metaplural gland secretion of the leaf-cutter ant Acromyrmex octospinosus: new compounds and their functional significance. Jour. Chem. Ecol. 26, 1667–1683. doi: 10.1023/A:1005543030518
Ortiz, A., and Orduz, S. (2000). In vitro evaluation of Trichoderma and Gliocladium antagonism against the symbiotic fungus of the leaf-cutting ant Atta cephalotes. Mycopathologia 150, 53–60.
Pagnocca, F. C., Masiulionis, V. E., and Rodrigues, A. (2012). Specialized fungal parasites and opportunistic fungi in gardens of attine ants. Psyche 2012:905109. doi: 10.1155/2012/905109
Pie, M. R., Rosengaus, R. B., and Traniello, J. F. A. (2004). Nest architecture, activity, pattern, worker density and the dynamics of disease transmission in social insects. J. Theor. Biol. 226, 45–51. doi: 10.1016/j.jtbi.2003.08.002
Pinto-Tomás, A. A., Anderson, M. A., Suen, G., Stevenson, D. M., Chu, F. S., Cleland, W. W., et al. (2009). Symbiotic nitrogen fixation in the fungus gardens of leaf-cutting. Science 326, 1120–1123. doi: 10.1126/science.1173036
Poulsen, M., and Boomsma, J. J. (2005). Mutualistic fungi control crop diversity in fungus-growing ants. Science 307, 741–744. doi: 10.1126/science.1106688
Poulsen, M., Bot, A. N. M., Nielsen, M. G., and Boomsma, J. J. (2002). Experimental evidence for the costs and hygienic significance of the antibiotic metapleural gland secretion in leaf-cutting ants. Behav. Ecol. Sociobiol. 52, 151–157. doi: 10.1007/s00265-002-0489-8
Poulsen, M., Cafaro, M., Boomsma, J. J., and Currie, C. R. (2005). Specificity of the mutualistic association between actinomycete bacteria and two sympatric species of Acromyrmex leaf-cutter ants. Mol. Ecol. 14, 3597–3604. doi: 10.1111/j.1365-294X.2005.02695.x
Poulsen, M., Cafaro, M. J., Erhardt, D. P., Little, A. E., Gerardo, N. M., Tebbets, B., et al. (2010). Variation in Pseudonocardia antibiotic defence helps govern parasite-induced morbidity in Acromyrmex leaf-cutting ants. Environ. Microbiol. Rep. 2, 534–540. doi: 10.1111/j.1758-2229.2009.00098.x
Poulsen, M., and Currie, C. R. (2006). “Complexity of insect-fungal associations: exploring the influence of microorganisms on the attine ant-fungus symbiosis,” in Insect Symbiosis, Vol. II, eds K. Bourtzis and T. Miller (Boca Raton, FL: CRC Press).
Quevillon, L. E., Hanks, E. M., Bansal, S., and Hughes, D. P. (2015). Social, spatial, and temporal organization in a complex insect society. Sci. Rep. 5, 1–11. doi: 10.1038/srep13393
Quinlan, R. J., and Cherrett, J. M. (1977). Role of substrate preparation in symbiosis between leaf-cutting ant Acromyrmex octospinosus (Reich) and its food fungus. Ecol. Entomol 2, 161–170. doi: 10.1111/j.1365-2311.1977.tb00877.x
Quinlan, R. J., and Cherrett, J. M. (1979). Role of fungus in the diet of the leaf-cutting ant Atta cephalotes (L). Ecol. Entomol. 4, 151–160. doi: 10.1111/j.1365-2311.1979.tb00570.x
Richard, F. J., and Errard, C. (2009). Hygienic behavior, liquid-foraging, and trophallaxis in the leaf-cutting ants, Acromyrmex subterraneus and Acromyrmex octospinosus. J. Insec. Sci. 9, 1–9. doi: 10.1673/031.009.6301
Richard, F. J., Poulsen, M., Drijfhout, F., Jones, G., and Boomsma, J. J. (2007a). Specificity in chemical profiles of workers, brood and mutualistic fungi in Atta, Acromyrmex, and Sericomyrmex fungus-growing ants. J. Chem. Ecol. 33, 2281–2292. doi: 10.1007/s10886-007-9385-z
Richard, F. J., Poulsen, M., Hefetz, A., Errard, C., Nash, D. R., and Boomsma, J. J. (2007b). The origin of the chemical profiles of fungal symbionts and their significance for nestmate recognition in Acromyrmex leaf-cutting ants. Behav. Ecol. Sociobiol. 61, 1637–1649. doi: 10.1007/s00265-007-0395-1
Ridley, P., Howse, P. E., and Jackson, C. W. (1996). Control of the behaviour of leaf-cutting ants by their ‘symbiotic’ fungus. Experientia 52, 631–635. doi: 10.1007/BF01969745
Rocha, S. L., Evans, H. C., Jorge, V. L., Cardoso, L. A. O., Pereira, F. S. T., Rocha, F. B., et al. (2017). Recognition of endophytic Trichoderma species by leaf-cutting ants and their potential in a Trojan-horse management strategy. R. Soc. Open Sci. 4:160628. doi: 10.1098/rsos.160628
Rocha, S. L., Jorge, V. L., Della Lucia, T. M. C., Barreto, R. W., Evans, H. C., and Elliot, S. L. (2014). Quality control by leaf-cutting ants: evidence from communities of endophytic fungi in foraged and rejected vegetation. Art. Plant Int. 8, 485–493. doi: 10.1007/s11829-014-9329-9
Rodrigues, A., Bacci, M. Jr., Mueller, U. G., Ortiz, A., and Pagnocca, F. C. (2008). Microfungal “weeds” in the leafcutter ant symbiosis. Micro. Ecol. 56, 604–614. doi: 10.1007/s00248-008-9380-0
Rodrigues, A., Cable, R. N., Mueller, U. G., Bacci, M. Jr., and Pagnocca, F. C. (2009). Antagonistic interactions between garden yeasts and microfungal garden pathogens of leaf-cutting ants. Antonie Van Leeuwenhoek 96, 331–342. doi: 10.1007/s10482-009-9350-7
Rodrigues, A., Mueller, U. G., Ishak, H. D., Bacci, M. Jr., and Pagnocca, F. C. (2011). Ecology of microfungal communities in gardens of fungus-growing ants (Hymenoptera: Formicidae): a year-long survey of three species of attine ants in Central Texas. FEMS Microbiol. Ecol. 78, 244–255. doi: 10.1111/j.1574-6941.2011.01152.x
Rodrigues, A., Pagnocca, F. C., Bacci, M. JR., Hebling, M. J. A., and Bueno, O. C. (2005). Variability of non-mutualistic fungi associated with Atta sexdens rubropilosa nests. Folia Microbiol. 50, 421–425. doi: 10.1007/BF02931424
Rohlfs, M., Obmann, B., and Petersen, R. (2005). Competition with filamentous fungi and its implication for a gregarious lifestyle in insects living on ephemeral resources. Ecol. Entomol. 30, 556–563. doi: 10.1111/j.0307-6946.2005.00722.x
Rosengaus, R. B., Jordan, C., Lefebvre, M. L., and Traniello, J. F. A. (1999). Pathogen alarm behavior in a termite: a new form of communication in social insects. Naturwissenschaften 86, 544–548. doi: 10.1007/s001140050672
Rosengaus, R. B., Traniello, J. F. A., and Bulmer, M. S. (2011). “Ecology, behavior and evolution of disease resistance in termites,” in Biology of Termites: A Modern Synthesis, eds D. E. Bignell, Y. Roisin, and N. Lo (New York: Springer), 165–191.
Saverschek, N., Herz, H., Wagner, M., and Roces, F. (2010). Avoiding plants unsuitable for the symbiotic fungus: learning and long-term memory in leaf-cutting ants. Anim. Behav. 79, 689–698. doi: 10.1016/j.anbehav.2009.12.021
Saverschek, N., and Roces, F. (2011). Foraging leafcutter ants: olfactory memory underlies delayed avoidance of plants unsuitable for the symbiotic fungus. Anim. Behav. 82, 453–458. doi: 10.1016/j.anbehav.2011.05.015
Schmid-Hempel, P. (2017). Parasites and their social hosts. Trends Parasitol. 33, 453–462. doi: 10.1016/J.PT.2017.01.003
Schultz, T. R., and Brady, S. G. (2008). Major evolutionary transitions in ant agriculture. Proc. Natl. Acad. Sci. U.S.A. 105, 5435–5440. doi: 10.1073/pnas.0711024105
Schulz, S., and Dickschat, J. S. (2007). Bacterial volatiles: the smell of small organisms. Nat. Prod. Rep. 24, 814–842. doi: 10.1039/b507392h
Schulz-Bohm, K., Martín-Sánchez, L., and Garbeva, P. (2017). Microbial volatiles: small molecules with an important role in intra- and inter-kingdom interactions. Front. Microbiol. 8:2484. doi: 10.3389/fmicb.2017.02484
Scott, J. J., Budsberg, K. J., Suen, G., Wixon, D. L., Balser, T. C., and Currie, C. R. (2010). Microbial community structure of leaf-cutter ant fungus gardens and refuse dumps. PLoS One 5:e9922. doi: 10.1371/journal.pone.0009922
Sen, R., Ishak, H. D., Estrada, D., Dowd, S. E., Hong, E., and Mueller, U. G. (2009). Generalized antifungal activity and 454-quantification of Pseudonocardia and Amycolatopsis bacteria in nests of fungus-growing ants. Proc. Natl. Acad. Sci. U. S. A. 106, 17805–17810. doi: 10.1073/pnas.0904827106
Silva, A., Rodrigues, A., Bacci, J. R. M., Pagnocca, F. C., and Bueno, O. C. (2006). Susceptibility of ant-cultivated fungus Leucoagaricus gongylophorus (Agaricales: Basidiomycota) towards microfungi. Mycopathologia 132, 115–119. doi: 10.1007/s11046-006-0037-6
Stow, A., and Beattie, A. (2008). Chemical and genetic defenses against disease in insect societies. Brain Behav. Immun. 22, 1009–1013. doi: 10.1016/j.bbi.2008.03.008
Stroeymeyt, N., Casillas-Pérez, B., and Cremer, S. (2014). Organizational immunity in social insects. Curr. Opin. Insect Sci. 5, 1–15. doi: 10.1016/j.cois.2014.09.001
Suen, G., Teiling, C., Li, L., Holt, C., Abouheif, E., Bornberg-Bauer, E., et al. (2010). The genome sequence of the leaf-cutter ant Atta cephalotes reveals insights into its obligate symbiotic lifestyle. PLoS Genetics 7:e1002007. doi: 10.1371/journal.pgen.1002007
Thiele, T., Kost, C., Roces, F., and Wirth, R. (2014). Foraging leaf-cutting ants learn to reject Vitis vinifera ssp. vinifera plants that emit herbivore-induced volatiles. J. Chem. Ecol. 40, 617–620. doi: 10.1007/s10886-014-0460-y
Toth, A. L., and Rehan, S. M. (2017). Molecular evolution of insect sociality: an eco-evo-devo perspective. Ann. Rev. Entomol. 62, 419–442. doi: 10.1146/annurev-ento-031616-035601
Traniello, J. F. A., Rosengaus, R. B., and Savoie, K. (2002). The development of immunity in a social insect: evidence for the group facilitation of disease resistance. Proc. Natl. Acad. Sci. U.S.A. 99, 6838–6842. doi: 10.1073/pnas.102176599
Tranter, C., Lefèvre, L., Evison, S. E. F., and Hughes, W. O. H. (2015). Threat detection: contextual recognition and response to parasites by ants. Behav. Ecol. 26, 396–405. doi: 10.1093/beheco/aru203
Ugelvig, L. V., and Cremer, S. (2007). Social prophylaxis: group interaction promotes collective immunity in ant colonies. Curr. Biol. 17, 1967–1971. doi: 10.1016/j.cub.2007.10.029
Um, S., Fraimout, A., Sapountzis, P., Oh, D. C., and Poulsen, M. (2013). The fungus-growing termite Macrotermes natalensis harbors bacillaene-producing Bacillus sp. that inhibit potentially antagonistic fungi. Sci. Rep. 3:3250. doi: 10.1038/srep03250
Valadares, L., Nascimento, D., and Nascimento, F. (2015). Foliar substrate affects cuticular hydrocarbon profiles and intraspecific aggression in the leafcutter ant Atta sexdens. Insects 6, 141–151. doi: 10.3390/insects6010141
Van Bael, S. A., Fernández-Marín, H., Valencia, M. C., Rojas, E. I., Wcislo, W. T., and Herre, E. A. (2009). Two fungal symbioses collide: endophytic fungi are not welcome in leaf-cutting ant gardens. Proc. Biol. Sci. 276, 2419–2426. doi: 10.1098/rspb.2009.0196
Van Bael, S. A., Seid, M., and Wcislo, W. (2012). Endophytic fungi increase the processing rate of leaves by leaf-cutting ants (Atta). Ecol. Entomol. 37, 318–321. doi: 10.1111/j.1365-2311.2012.01364.x
Viana, A. M., Frézard, A., Malosse, C., Della Lucia, T. M., Errard, C., and Lenoir, A. (2001). Colonial recognition of fungus in the fungus-growing ant Acromyrmex subterraneus subterraneus (Hymenoptera: Formicidae). Chemoecology 11, 29–36. doi: 10.1007/PL00001829
Vigueras, G., Paredes-Hernández, D., Revah, S., Valenzuela, J., Olivares-Hernández, R., and Le Borgne, S. (2017). Growth and enzymatic activity of Leucoagaricus gongylophorus, a mutualistic fungus isolated from the leaf-cutting ant Atta mexicana, on cellulose and lignocellulosic biomass. Lett. Appl. Microbiol. 65, 173–181. doi: 10.1111/lam.12759
Visser, A. A., Kooij, P. W., Debets, A. J. M., Kuyper, T. W., and Aanen, D. K. (2011). Pseudoxylaria as stowaway of the fungus-growing termite nest: interaction asymmetry between Pseudoxylaria, Termitomyces and free-living relatives. Fungal Ecol. 4, 322–332. doi: 10.1016/j.funeco.2011.05.003
Walker, N. T., and Hughes, W. O. H. (2009). Adaptative social immunity in leaf-cutting ants. Biol. Lett. 5, 446–448. doi: 10.1098/rsbl.2009.0107
Wang, Y., Mueller, U. G., and Clardy, J. C. (1999). Antifungal diketopiperazines from the symbiotic fungus of the fungus-growing ant Cyphomyrmex minutus. J. Chem. Ecol. 25, 935–941. doi: 10.1023/A:1020861221126
Weber, N. A. (1972). The fungus-culturing behavior of ants. Am. Zool. 12, 577–587. doi: 10.1016/j.jinsphys.2018.05.007
Wilson-Rich, N., Spivak, M., Fefferman, N. H., and Starks, P. T. (2009). Genetic, individual, and group facilitation of disease resistance in insect societies. Ann. Rev. Entomol. 54, 405–423. doi: 10.1146/annurev.ento.53.103106.093301
Yanagawa, A., Fujiwara-Tsujii, N., Akino, T., Yoshimura, T., Yanagawa, T., and Shimizu, S. (2011). Musty odor of entomopathogens enhances disease-prevention behaviors in the termite Coptotermes formosanus. J. Invertebr. Pathol. 108, 1–6. doi: 10.1016/j.jip.2011.06.001
Yanagawa, A., Fujiwara-Tsujii, N., Akino, T., Yoshimura, T., Yanagawa, T., and Shimizu, S. (2012). Odor aversion and pathogen-removal efficiency in grooming behavior of the termite Coptotermes formosanus. PLoS One 7:e47412. doi: 10.1371/journal.pone.0047412
Yanagawa, A., and Shimizu, S. (2007). Resistance of the termite Coptotermes formosanus Shiraki to Metarhizium anisopliae due to grooming. Bio. Control 52, 75–85. doi: 10.1007/s10526-006-9020-x
Yek, S. H., Boomsma, J. J., and Poulsen, M. (2012). Towards a better understanding of the evolution of specialized parasites of fungus-growing ant crops. Psyche 2012, 1–10. doi: 10.1155/2012/239392
Yek, S. H., and Mueller, U. G. (2011). The metapleural gland of ants. Biol. Rev. 86, 774–791. doi: 10.1111/j.1469-185X.2010.00170.x
Keywords: attine ants, social immunity, behavioral immunity, communication, diseases
Citation: Goes AC, Barcoto MO, Kooij PW, Bueno OC and Rodrigues A (2020) How Do Leaf-Cutting Ants Recognize Antagonistic Microbes in Their Fungal Crops? Front. Ecol. Evol. 8:95. doi: 10.3389/fevo.2020.00095
Received: 29 November 2019; Accepted: 24 March 2020;
Published: 05 May 2020.
Edited by:
Marko Rohlfs, University of Bremen, GermanyReviewed by:
Nick Bos, University of Helsinki, FinlandSandra Breum Andersen, New York University, United States
Copyright © 2020 Goes, Barcoto, Kooij, Bueno and Rodrigues. This is an open-access article distributed under the terms of the Creative Commons Attribution License (CC BY). The use, distribution or reproduction in other forums is permitted, provided the original author(s) and the copyright owner(s) are credited and that the original publication in this journal is cited, in accordance with accepted academic practice. No use, distribution or reproduction is permitted which does not comply with these terms.
*Correspondence: Andre Rodrigues, YW5kcmVyQHJjLnVuZXNwLmJy