- 1Department of Biology, University of Kentucky, Lexington, KY, United States
- 2College of Veterinary Medicine, North Carolina State University, Raleigh, NC, United States
- 3Department of Entomology, Michigan State University, East Lansing, MI, United States
- 4Department of Osteopathic Medical Specialties, Michigan State University, East Lansing, MI, United States
- 5Ecology, Evolutionary Biology, and Behavior Program, Michigan State University, East Lansing, MI, United States
Sociality has independently arisen in several wood-dwelling insect lineages, yet little is understood about how the properties of decaying logs have favored the evolution of cooperative social groups. Here we evaluate the current literature on wood-dwelling social insects to identify the structural, nutritional, and microbial properties of decaying logs that have led to the repeated evolution of social behavior. Wood-tissue is structural resilient, and thus provided an enclosed, defensible nest site for early wood-feeding insect groups. This structural stability enabled the long-term persistence of family groups, and was likely a key feature in the transition toward more complex eusocial societies. The resilient structure and relatively poor nutritional quality of wood also likely provided a stable environment for the evolution of complex mutualisms with prokaryote and fungal symbionts to digest this resource. Parental care likely evolved as a means to protect the valuable nest site and ensure adequate nutrition for offspring in this environment by allowing parents to both provision and transfer microbial symbionts to offspring. Pathogenic microbes are also abundant in nests constructed in wood-tissue, and social adaptations such as allogrooming and nest maintenance may have evolved in response to microbial invaders. In general, the dynamic relationships between insects, microbes, and the wood-tissue that they inhabit was a critical component in the evolution of sociality in this habitat.
Introduction
Of William D. Hamilton’s many notable contributions to evolutionary biology, his most significant work was his formalized theory of kin selection (Hamilton, 1964). This theory provided an explanation for the evolution of altruism that was consistent with individual selection by demonstrating that helpers could produce copies of their own alleles by assisting non-descendent kin (Hamilton, 1964). In addition to investigating the genetic processes of altruistic evolution, William D. Hamilton was also fascinated by the ecological conditions that facilitated the evolution of complex insect societies. In his lesser known 1978 paper, “Funeral Feasts: Evolution and Diversity Under Bark,” Hamilton noted the peculiar diversity of social insects inhabiting decaying logs (Hamilton, 1978). He recognized that while close relatedness was essential to the evolution of worker altruism, these early social groups were more likely to arise in certain habitats. He suggested that decaying logs, due to their protective, long lasting properties, provided the ideal environment for the early evolution of highly altruistic eusocial societies, such as those of ants and termites.
Since Hamilton’s observations nearly 40 years ago, much has been revealed about the intricacies of insect sociality under bark. New social wood-dwelling insects have been discovered (Kent and Simpson, 1992), fascinating details of different insect societies have been described (Biedermann and Taborsky, 2011; Smith et al., 2018), and the mechanisms driving the evolution of sociality in these environments are continuing to be explored (Thorne and Traniello, 2003; Inward et al., 2007; Korb et al., 2012; Nuotclà et al., 2019). We draw upon this growing literature to investigate the factors that have contributed to the diversity of insect societies inhabiting decaying logs within the context of William D. Hamilton’s pioneering work.
Family and Group Formation in Decaying Logs
The evolution of complex sociality can be broken into three major phases: the onset of group formation (initially through extended parental care), maintenance of social groups, and the subsequent transition to complex, obligate social-living (Bourke, 2011; Korb and Heinze, 2016). Family groups consisting of parents and offspring are considered a critical prerequisite for the transition to more complex eusocial societies, and are generally more common in social log-dwelling lineages than aggregations of unrelated individuals (Table 1; Kent and Simpson, 1992; Inward et al., 2007; Nalepa et al., 2008; Biedermann and Taborsky, 2011; Suzuki, 2013; but see Zorapterans, Mashimo et al., 2014). Indeed, the two instances of eusociality that have arisen in wood-dwelling insect lineages evolved from ancestral family groups (ambrosia beetles, Smith et al., 2018; termites, Klass et al., 2008). Identifying the properties of the decaying log habitat that favored the evolution and maintenance of parent-offspring groups is thus key to understanding the prevalence of sociality in log-dwelling insects.
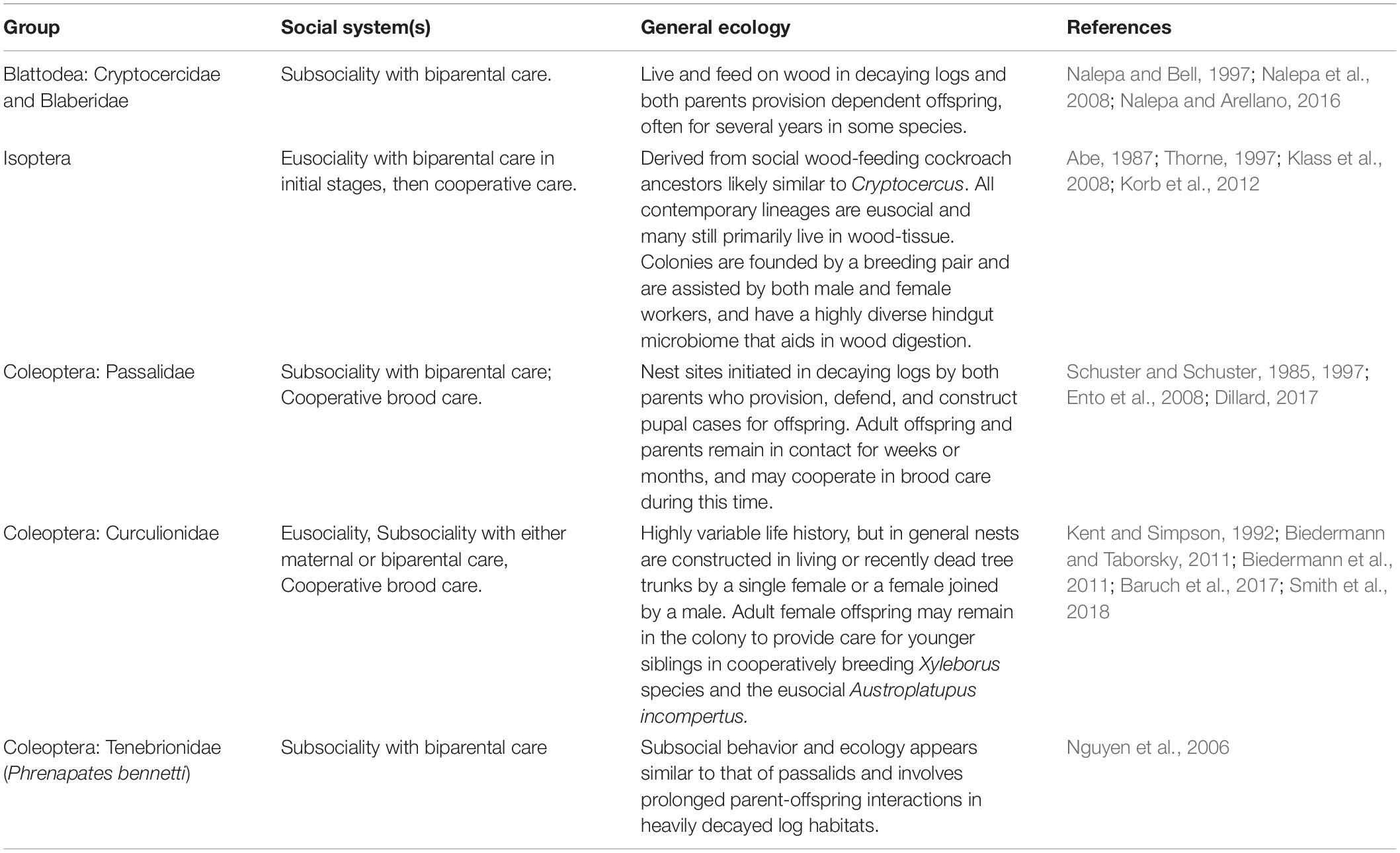
Table 1. Summary of the ecology and life history of the social and gregarious wood-dwelling insects.
The selective pressures that favor the transition to cooperative societies from simple family groups in any environment can generally be classified as either benefits associated with staying in the natal nest or costs associated with dispersal (Koenig et al., 1992; Emlen, 1994). The benefits of remaining in family groups in log-dwelling lineages can generally be classified as structural, nutritional, or microbial benefits that the log resource provides to offspring that delay dispersal from the nest (Table 2 and Figure 1). Logs provide a safe, sheltered, food-abundant nesting resource for retained offspring, allowing for prolonged parent-offspring interactions that set the stage for the subsequent evolution of more complex social behaviors. Dispersal costs can also be extremely high in wood-dwelling insects, drastically reducing the likelihood of independent establishment for those that disperse from the natal nest. Competition for log resources is fierce and some termite species experience a <1% success rate in establishing a new nest site following dispersal (Chouvenc, 2019). Below we discuss these specific attributes of wood environments that have shaped sociality in log-dwelling lineages in more detail.
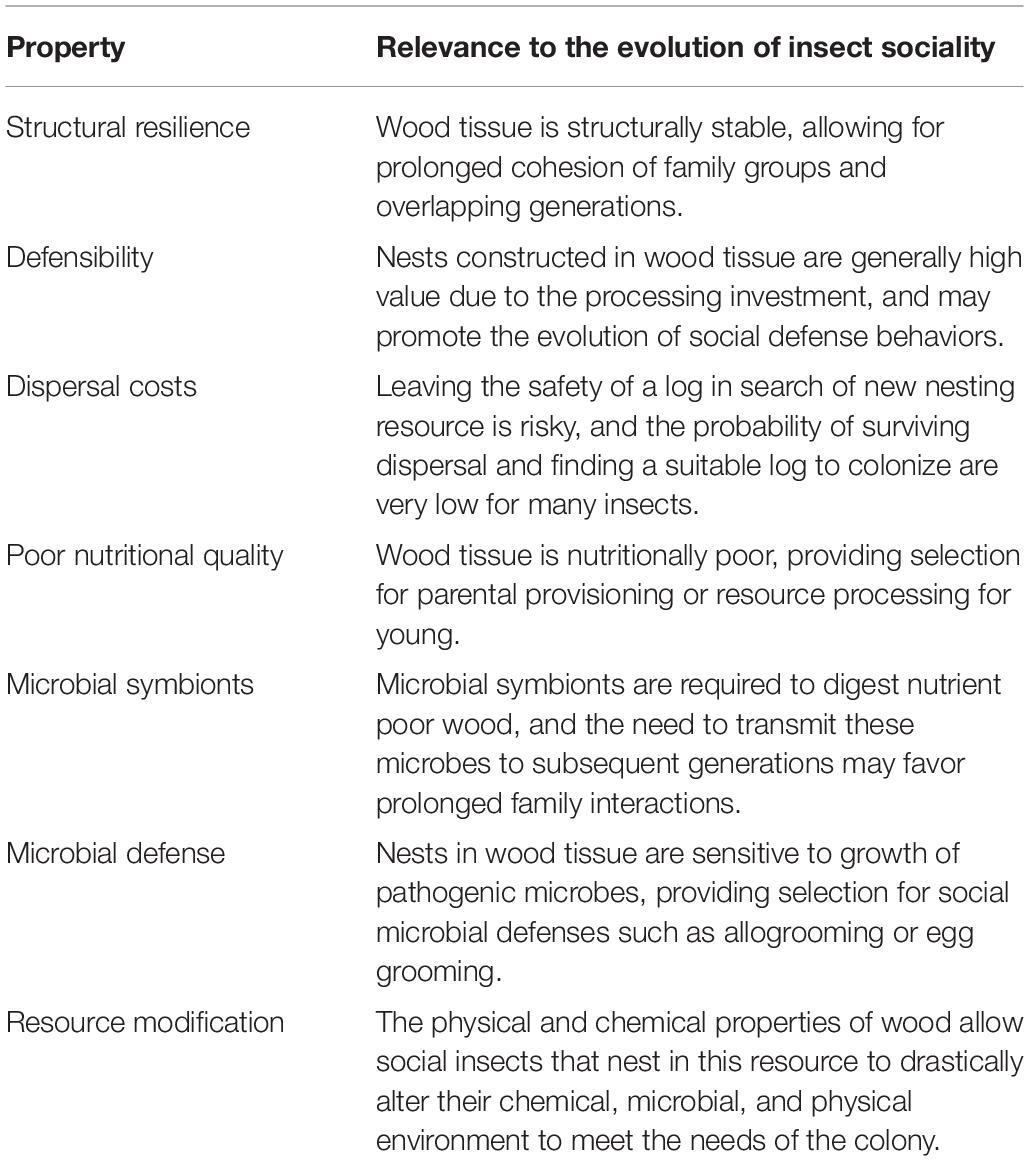
Table 2. Properties of wood tissue that may have facilitated the evolution of parental care and cooperative societies in wood-dwelling insects.
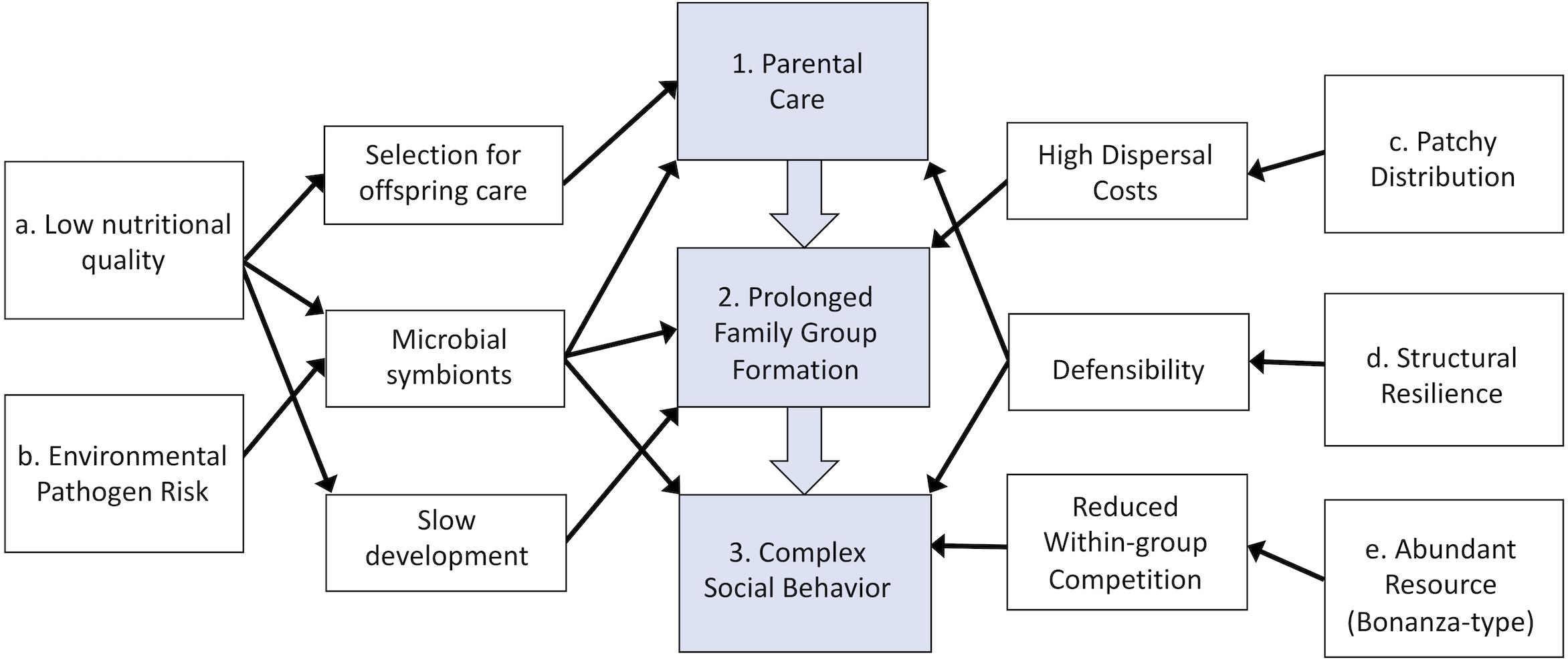
Figure 1. Conceptual diagram of the factors associated with log-habitats that have shaped the evolution of parental care and the transition to more complex family and social groups. The physical properties of the log habitat (boxes a–e) favored the sequential evolution of complex family-based social groups from ancestors with simple parental care (boxes 1–3) by generally favoring prolonged family cohesion and reducing dispersal and independent establishment success.
Structural Resilience of Nest Sites Constructed in Wood Tissue
Wood tissue is a stable, long-lasting resource that provides both food and protection for nests constructed within. When used as a food source, decaying logs serve as plentiful bonanza resources, reducing competition among group members and favoring family group formation by limiting within-group conflict (Korb and Heinze, 2016). Wood-tissue, particularly large tree trunks, degrades slowly and is structurally resilient, allowing insect families to persist for several generations before the resource is depleted (Nalepa and Bell, 1997; Thorne, 1997; Korb et al., 2012). Both wood-dwelling passalid beetles and ambrosia beetles form families in which the social group and the nest persist well after offspring mature into adults (Schuster and Schuster, 1997; Biedermann and Taborsky, 2011). In both systems, young adults have been observed helping to care for younger siblings, highlighting the importance of prolonged family cohesion in the evolution of cooperation (Schuster and Schuster, 1985; Biedermann and Taborsky, 2011).
Structural stability also ensures that the nest site remains intact even after the death of a parent, providing offspring that remain in the nest the opportunity to inherit the breeding resource. For instance, competition between colonies for nesting resources in Microcerotermes papuanas, an extant termite species that forms colonies in a single log resource (“one-piece” nesting; Abe, 1987), often results in the death of one or more primary reproductive individuals in the colony (Thorne and Traniello, 2003). Young workers in these colonies can molt into reproductive adults and begin to reproduce in the nest via parthenogenesis to fill this breeding vacancy (Roisin, 1990; Fougeyrollas et al., 2017). Indeed, the likelihood of inheriting a breeding position within the natal colony is actually higher than that of successfully dispersing and establishing a nest in a new log for some lower termite species (Korb and Schneider, 2007; Korb and Heinze, 2016).
Nesting in log resources also provided early social lineages with valuable, defensible nest sites, facilitating the evolution of eusociality via a “fortress defender” route (Queller and Strassmann, 1998). In contrast to eusocial “life insurers” in which workers specialized into a foraging caste, fortress defenders, such as deadwood termites, favored soldier castes to defend the nest site against competitors (Queller and Strassmann, 1998). Low establishment success, high competition, and high value of the log resource resulting from its function as both food and shelter makes this habitat particularly amenable to the evolution of fortress defense eusociality.
Sociality and Symbionts: Adaptations to Poor Nutritive Quality of Wood
Although wood is abundant and long-lasting in large tree trunks, most of the nutrients contained in wood tissue are not accessible to the digestive systems of animals (but see Tokuda et al., 2004), and feeding on this resource could select for a variety of social adaptations to overcome this limitation. Wood is generally nitrogen-poor and difficult to digest due to its high cellulose and lignin content (Tallamy and Wood, 1986). Generally, feeding on resources that are low quality can result in slower development of young, ultimately increasing the amount of time during which offspring are dependent on parental care (Beehler, 1985; Strahl, 1988; Nalepa and Bell, 1997; Nalepa and Arellano, 2016). In some wood-feeding taxa, juveniles that do not possess the enzymes or symbionts necessary to digest wood depend on parents to process the wood resource for them (King and Fashing, 2007; Mishima et al., 2016). Parental care in these systems may thus primarily function as a means to provide offspring with adequate nutrition. The evolution of both parents assisting in brood care (which has occurred in passalids, cockroaches, and termites) may have arisen to meet the high nutritional needs of the brood, with increasing parental care setting the stage for the co-evolution of even more dependent (altricial) young (Nalepa et al., 2008). The nutritional deficiencies of wood may therefore have been a major determinant of the subsequent evolution of complex cooperation by initiating the evolution of extreme offspring dependency through parent-offspring feedback selection (Nalepa et al., 2001, 2008). Increased offspring altriciality likely then increased the benefits of offspring care, providing selection for the evolution of even more complex social traits, including cooperative breeding and eventually eusociality.
Symbiont Transmission Between Parents and Offspring
To subsist on the generally nutrient-poor wood tissue, wood-feeding insects have evolved complex symbiotic relationships with bacteria and fungi that allow them to extract nutrients from these largely indigestible resources (Nalepa et al., 2001; Suh et al., 2003; Biedermann et al., 2009; Mishima et al., 2016). Although these microbial symbionts allow their insect partners to thrive in the nutrient-poor wood tissue environment, maintaining the integrity of the microbial communities and transmitting them to subsequent generations can be challenging.
Intraspecific coprophagy (feces ingestion) and anal trophallaxis were key adaptations allowing for the transfer of beneficial microbial communities from parents to offspring. In the Cryptocercus cockroaches and termites, the need to share symbionts between parents and offspring was likely a major driver of prolonged parental care (Nalepa et al., 2001). Juveniles are fed microbe-rich secretions produced by their parents via anal trophallaxis to initiate and maintain their own hindgut community of wood-digesting microbial symbionts (Thorne, 1997; Klass et al., 2008). This mode of direct transfer rendered these microbes dependent on their hosts, and contributed to the strong mutualism observed between host and symbiont observed in both cockroaches and termites (Nalepa et al., 2001; Nalepa, 2017). Passalids, in contrast, share symbionts with offspring via ingestion of feces (coprophagy) and processed wood in the nest (Suh et al., 2003; Mishima et al., 2016). Phrenapates bennetti, a tenebrionid that strongly resembles passalids in morphology, life history, and subsocial behavior, also uses similar xylose fermenting yeasts (Nguyen et al., 2006). Although little is known about the social behavior of Phrenapates, given their convergent evolution with the passalids it is possible that they transfer symbiotic yeasts from parents to young via coprophagy as well, with subsociality potentially arising as a consequence.
Other wood-dwelling lineages, including the Xyleborine and Platypodine ambrosia beetles, feed on fungus that they cultivate in tree trunks rather than on the wood tissue itself (Kent and Simpson, 1992; Biedermann and Taborsky, 2011). Xyleborine ambrosia beetle females disperse as young adults to initiate their own colonies, and solve the transmission problem by carrying “starter” cultures from their natal nest that they store in either the gut or fungal storage organs called mycangia prior to dispersal (Batra, 1963; Biedermann et al., 2009; Seibold et al., 2019). As with the other microbial-dependent, wood-feeding insects, this form of symbiont transmission requires prolonged interaction between parents and offspring in the natal nest.
Interestingly, not all insects that breed in wood that rely on symbionts to aid in digestion live socially. Females of many stag beetle species, for instance, do not remain with their offspring after oviposition, although they appear to inoculate the oviposition site with xylose-fermenting yeasts from a fungal storage structure (mycangium) before departing to transmit the symbionts to their young (Tanahashi et al., 2009, 2010). Stag beetles only consume decaying wood as larvae, however, and this difference in feeding modes between adult and neonates may partly explain how they have adapted to transmit endosymbionts to young without prolonged parent-offspring associations (Tanahashi et al., 2009, 2010). In contrast, all of the social wood-dwelling insects that feed on wood or microbes cultivated in wood do so as both adults and larvae (Nalepa and Bell, 1997; Schuster and Schuster, 1997; Thorne, 1997; Biedermann et al., 2011). Remaining in the log nest to feed as adults may have been an important pre-requisite in the evolution of parental care as well as more complex social behaviors. Further research comparing the ecological, physiological, and microbial differences between wood-feeding insects that transmit symbionts to offspring socially to those that can do so without prolonged parent-offspring contact may reveal valuable insight into the predisposing factors that have promoted sociality in certain wood-feeding insect groups.
Microbial Defense Against Pathogens in Social Groups
Although wood tissue is amenable to the growth and maintenance of beneficial microbes, these environments also provide suitable habitat for growth of harmful or pathogenic microbes. These challenges might be exacerbated for lineages that facilitate the growth of beneficial microbes in the nest site (Nuotclà et al., 2019). Many wood-feeding insects have evolved social defenses against these deleterious microbes such as corpse management (López-Riquelme and Fanjul-Moles, 2013; Sun and Zhou, 2013; Sun et al., 2018), allogrooming (Rosengaus et al., 1998; Wilson-Rich et al., 2009; Meunier, 2015), and chemical defenses (Biedermann and Rohlfs, 2017). While many of these social adaptations most likely arose after the onset of group-living, they suggest ways that living in resources like wood could provide feedback selection on incipient social groups, reinforcing and elaborating social traits. Female ambrosia beetles of the species Xyleborinus saxesenii, for instance, delay dispersal for longer periods of time when their nests have been infected with Aspergillus spores (Nuotclà et al., 2019). Infection of ambrosia beetle nests with Aspergillus also leads to greater expression of allogrooming and corpse maintenance behavior, providing evidence that social behavior can be enhanced when microbial defense is needed. The gut symbionts of dampwood termites (Zootermopsis nevadensis) also have been shown to have anti-pathogen properties. In addition to their digestive function, the intestinal microorganisms of these dampwood termites increase intestinal acetate which has inhibitory effects on the opportunistic pathogen Serratia marcescens (Inagaki and Matsuura, 2018).
Dynamic Structure of Decomposing Log Resources
Feeding within decaying logs either on the wood tissue itself or on the microbes cultivated in the nest site results in complex, dynamic resource properties. First, by boring into the wood, insects increase both the surface area and physical heterogeneity of the resource, allowing wood-degrading microbes (i.e., bacteria, protists, and fungi) to more readily colonize the resource (Ulyshen, 2016). Many insects alter the wood via physical processing, chemical additives, and inoculation of beneficial microbes to either enhance the quality of the wood (external rumination; Suh et al., 2003) or to cultivate food resources (fungal farming; Batra, 1963; Biedermann et al., 2009). Insect tunneling also leads to better aeration and fragmentation of the wood, improving habitat quality for both the insects and microbes (Ulyshen, 2016). This fragmentation, however, may reduce the structural integrity of the resource over time, causing the wood to collapse or the bark to slough off. Log degradation from colonization, which can be rapid when colonized by large or efficient social insects such as the passalids or termites, respectively, may thus reduce the total lifespan of the colony resource. Each log likely has a carrying capacity that varies not only with the abiotic conditions of the surrounding habitat, but also the use and transformation by the inhabiting insects through activities such as tunneling, enhancing, and cultivating the resource. This eventual degradation of the log resource by wood-feeding insects is perhaps one reason why the most complex termite societies evolved following the transition from log-nesting to inhabiting more permanent, self-constructed nest sites (Korb et al., 2012).
Social Insect and Microbial Community Ecology in Decaying Logs
Microbial communities of logs and other large and recalcitrant wood debris are complex and not well understood (see review by Johnston et al., 2016). However, they are known to be mediated by organisms that have evolved to either consume the organic matter directly or use it as a relatively stable habitat (e.g., compared to carrion or leaf litter) for breeding or nest establishment (Ulyshen, 2016). Habitat stability has been proposed to provide the circumstances for positive evolutionary feedback between insect sociality and microbial community management (Biedermann and Rohlfs, 2017). However, as wood becomes more fragmented either through abiotic damage (e.g., wind, damage by falling, and rain) and insect tunneling activities (e.g., ambrosia beetles), the area available for microbial colonization increases (Ulyshen, 2016), suggesting that the importance of microbes to wood inhabiting insects becomes increasingly relevant during later decomposition. For many wood feeding insects, such as some Passalidae, those microbes likely make up a considerable portion of their nutritional needs (Castillo and Reyes-Castillo, 2009; Filipiak, 2018). Thus, the microbial communities and how they change during the long course of wood debris decomposition are thought to play at least a nutritional role in the ecology of wood feeding insects. There is also evidence of additional relationships between microbes and wood feeding insects, especially within the context of co-evolution of endosymbionts (Suh and Blackwell, 2005; Nardi et al., 2006; Warnecke et al., 2007; Ulyshen, 2016) and fungal ectosymbiosis (Krivosheina, 1991; Mueller et al., 2005; Biedermann and Vega, 2020).
Conclusion and Future Directions
Some of the most complex, cooperative insect societies have arisen from ancestors that lived and fed in the wood tissue of large trees (Kent and Simpson, 1992; Klass et al., 2008). William D. Hamilton largely attributed this occurrence to the structural longevity of wood tissue, and the defensibility of nest sites constructed within (Hamilton, 1978). Newer discoveries reveal a critical role of both symbiotic and pathogenic microbes in the evolution and maintenance of parental care and sociality in wood-dwelling lineages (Biedermann and Rohlfs, 2017; Nuotclà et al., 2019). Parental care and other forms of sociality afforded these insects the means to provide adequate nutrition of young through provisioning and symbiont transfer and to defend their nest sites from biological threats (microbial and otherwise). The structural longevity of large tree trunks likely allowed these small family groups to persist for multiple generations, facilitating the transition from subsociality to eusociality in some lineages.
Despite this developing framework, much still remains to be understood about the nuanced interactions between social insects and their microbial and physical environment. Not all wood-dwelling insects have evolved social behavior to better exploit wood-tissue, and identifying the fundamental similarities and differences between the non-social and social wood-dwelling species may provide insight into the factors critical to the evolution of sociality in wood (Tanahashi et al., 2009, 2010). For instance, the microbial communities employed by different species to aid in wood digestion may differ drastically between social and non-social species, and the properties of these microbial symbionts may have been a critical factor determining whether or not a lineage became social. Fundamental differences in life history may also distinguish social from solitary wood-feeding insects, including adult feeding behavior. Further investigations into the coevolution between microbes and the behavior of their insect hosts will likely yield tremendous insight into the ways that microbes have contributed to the evolution of insect societies.
Author Contributions
JD and MB both contributed to the writing and editing of this manuscript.
Funding
MB was funded, in part, by the College of Agriculture and Natural Resources, AgBioResearch, and the Department of Entomology at Michigan State University.
Conflict of Interest
The authors declare that the research was conducted in the absence of any commercial or financial relationships that could be construed as a potential conflict of interest.
Acknowledgments
We thank Christine Nalepa for her thoughtful comments on this review.
References
Abe, T. (1987). “Evolution of life types in termites,” in Evolution and Coadaptation in Biotic Communities, eds S. Kawano, J. H. Connell, and T. Hidaka (Tokyo: University of Tokyo press), 125–148.
Baruch, O., Mendel, Z., Scharf, I., and Harari, A. R. (2017). Mating system, mate choice and parental care in a bark beetle. Bull. Entomol. Res. 107, 611–619. doi: 10.1017/S0007485317000311
Batra, L. (1963). Ecology of Ambrosia fungi and their dissemination by Beetles. Trans. Kansas Acad. Sci. 66, 213–236.
Beehler, B. (1985). Adaptive significance of monogamy in the trumpet manucode, Manucodia keraudrenii (Aves: Paradisaeidae). Ornithol. Monogr. 37, 83–99.
Biedermann, P. H., and Rohlfs, M. (2017). Evolutionary feedbacks between insect sociality and microbial management. Curr. Opin. Insect Sci. 22, 92–100. doi: 10.1016/j.cois.2017.06.003
Biedermann, P. H. W., Klepzig, K. D., and Taborsky, M. (2009). Fungus cultivation by Ambrosia Beetles: behavior and laboratory breeding success in three Xyleborine species. Environ. Entomol. 38, 1096–1105. doi: 10.1603/022.038.0417
Biedermann, P. H. W., Klepzig, K. D., and Taborsky, M. (2011). Costs of delayed dispersal and alloparental care in the fungus-cultivating ambrosia beetle Xyleborus affinis Eichhoff (Scolytinae: Curculionidae). Behav. Ecol. Sociobiol. 65, 1753–1761. doi: 10.1007/s00265-011-1183-5
Biedermann, P. H. W., and Taborsky, M. (2011). Larval helpers and age polyethism in ambrosia beetles. Proc. Natl. Acad. Sci. U.S.A. 108, 17064–17069. doi: 10.1073/pnas.1107758108
Biedermann, P. H. W., and Vega, F. E. (2020). Ecology and evolution of insect–fungus mutualisms. Annu. Rev. Entomol. 65, 431–455. doi: 10.1146/annurev-ento-011019-024910
Castillo, M. L., and Reyes-Castillo, P. (2009). Passalidae, insects which live in decaying logs. Trop. Biol. Conserv. Manage. VII, 112–133.
Chouvenc, T. (2019). The relative importance of queen and king initial weights in termite colony foundation success. Insectes Soc. 66, 177–184. doi: 10.1007/s00040-019-00690-3
Dillard, J. (2017). High rates of extra-pair paternity in a socially monogamous beetle with biparental care. Ecol. Entomol. 42, 1–10. doi: 10.1111/een.12346
Emlen, S. T. (1994). Benefits, constrainsts and the evolution of the family. Trends Ecol. Evol. 9, 282–285. doi: 10.1016/0169-5347(94)90030-2
Ento, K., Araya, K., and Kudo, S. (2008). Trophic egg provisioning in a passalid beetle (Coleoptera). Eur. J. Entomol. 5759, 99–104. doi: 10.14411/eje.2008.014
Filipiak, M. (2018). “Nutrient dynamics in decomposing dead wood in the context of wood eater requirements: the ecological stoichiometry of saproxylophagous insects,” in Saproxylic Insects, ed. M. Ulyshen (Cham: Springer), 429–469. doi: 10.1007/978-3-319-75937-1_13
Fougeyrollas, R., Křivánek, J., Roy, V., Dolejšová, K., Frechault, S., Roisin, Y., et al. (2017). Asexual queen succession mediates an accelerated colony life cycle in the termite Silvestritermes minutus. Mol. Ecol. 26, 3295–3308. doi: 10.1111/mec.14095
Hamilton, W. D. (1964). The genetical evolution of social behaviour. I. J. Theor. Biol. 7, 1–16. doi: 10.1016/0022-5193(64)90038-4
Hamilton, W. D. (1978). “Evolution and diversity under bark,” in Diversity of Insect Faunas. Symposia of the Royal Entomological Society of London No. 9, eds L. A. Mound and N. Waloff (Oxford: Blackwell Scientific), 154–175.
Inagaki, T., and Matsuura, K. (2018). Extended mutualism between termites and gut microbes: nutritional symbionts contribute to nest hygiene. Sci. Nat. 105:52. doi: 10.1007/s00114-018-1580-y
Inward, D. J. G., Vogler, A. P., and Eggleton, P. (2007). A comprehensive phylogenetic analysis of termites (Isoptera) illuminates key aspects of their evolutionary biology. Mol. Phylogenet. Evol. 44, 953–967. doi: 10.1016/j.ympev.2007.05.014
Johnston, S. R., Boddy, L., and Weightman, A. J. (2016). Bacteria in decomposing wood and their interactions with wood-decay fungi. FEMS Microbiol. Ecol. 92, 1–12.
Kent, D., and Simpson, J. (1992). Eusociality in the Beetle Austroplatypus incompertus (Coleoptera: Curculiomdae). Naturwissenschaften 87, 86–87.
King, A., and Fashing, N. (2007). Infanticidal behavior in the subsocial Beetle Odontotaenius disjunctus (Illiger) (Coleoptera: Passalidae). J. Insect Behav. 20, 527–536. doi: 10.1007/s10905-007-9094-z
Klass, K. D., Nalepa, C., and Lo, N. (2008). Wood-feeding cockroaches as models for termite evolution (Insecta: Dictyoptera): Cryptocercus vs. Parasphaeria boleiriana. Mol. Phylogenet. Evol. 46, 809–817. doi: 10.1016/j.ympev.2007.11.028
Koenig, W. D., Pitelka, F. A., Carmen, W. J., Mumme, R. L., and Stanback, M. T. (1992). The evolution of delayed dispersal in cooperative breeders. Q. Rev. Biol. 67, 111–150. doi: 10.1086/417552
Korb, J., Buschmann, M., Schafberg, S., Liebig, J., and Bagnères, A.-G. (2012). Brood care and social evolution in termites. Proc. Biol. Sci. 279, 2662–2671. doi: 10.1098/rspb.2011.2639
Korb, J., and Heinze, J. (2016). Major hurdles for the evolution of sociality. Annu. Rev. Entomol. 61, 297–316. doi: 10.1146/annurev-ento-010715-023711
Korb, J., and Schneider, K. (2007). Does kin structure explain the occurrence of workers in a lower termite? Evol. Ecol. 21, 817–828. doi: 10.1007/s10682-006-9153-5
Krivosheina, N. P. (1991). “Relations between wood-inhabiting insects and fungi,” in Forest Insect Guilds: Patterns of Interaction with Host Trees; 1989 August 13-17; Abakan, Siberia, U.S.S.R. Gen. Tech. Rep. NE-153, eds Y. N. Baranchikov, W. J. Mattson, F. P. Hain, and T. L. Payne (Radnor, PA: U.S. Department of Agriculture, Forest Service, Northeastern Forest Experiment Station), 335–346.
López-Riquelme, G. O., and Fanjul-Moles, M. L. (2013). The funeral ways of social insects. Social strategies for corpse disposal. Trends Entomol. 9, 71–128.
Mashimo, Y., Matsumura, Y., Machida, R., Dallai, R., Gottardo, M., Yoshizawa, K., et al. (2014). 100 years Zoraptera – a phantom in insect evolution and the history of its investigation. Insect Syst. Evol. 45, 371–393. doi: 10.1163/1876312x-45012110
Meunier, J. (2015). Social immunity and the evolution of group living in insects. Philos. Trans. R. Soc. Lond. B Biol. Sci. 370, 19–21. doi: 10.1098/rstb.2014.0102
Mishima, T., Wada, N., Iwata, R., Anzai, H., Hosoya, T., and Araya, K. (2016). Super-protective child-rearing by japanese bess beetles, Cylindrocaulus patalis: adults provide their larvae with chewed and predigested wood. Insects 7:18. doi: 10.3390/insects7020018
Mueller, U. G., Gerardo, N. M., Aanen, D. K., Six, D. L., and Schultz, T. R. (2005). The evolution of agriculture in insects. Annu. Rev. Ecol. Evol. Syst. 36, 563–595.
Nalepa, C. (2017). What kills the hindgut flagellates of lower termites during the host molting cycle? Microorganisms 5:82. doi: 10.3390/microorganisms5040082
Nalepa, C., and Bell, W. (1997). “Postovulation parental investment and parental care in cockroaches,” in The Evolution of Social Behavior in Insects and Arachnids, eds J. C. Choe and B. Crespi (Cambridge: Cambridge University Press), 26–51. doi: 10.1017/cbo9780511721953.004
Nalepa, C. A., and Arellano, C. (2016). Parental social environment alters development of nutritionally independent nymphs in Cryptocercus punctulatus (Dictyoptera: Cryptocercidae). Behav. Ecol. Sociobiol. 70, 881–887. doi: 10.1007/s00265-016-2110-6
Nalepa, C. A., Bignell, D. E., and Bandi, C. (2001). Detritivory, coprophagy, and the evolution of digestive mutualisms in Dictyoptera. Insectes Soc. 48, 194–201. doi: 10.1007/pl00001767
Nalepa, C. A., Maekawa, K., Shimada, K., Saito, Y., Arellano, C., and Matsumoto, T. (2008). Altricial development in subsocial wood-feeding cockroaches. Zoolog. Sci. 25, 1190–1198. doi: 10.2108/zsj.25.1190
Nardi, J. B., Bee, C. M., Miller, L. A., Nguyen, N. H., Suh, S.-O., and Blackwell, M. (2006). Communities of microbes that inhabit the changing hindgut landscape of a subsocial beetle. Arthropod Struct. Dev. 35, 57–68. doi: 10.1016/j.asd.2005.06.003
Nguyen, N. H., Suh, S. O., Marshall, C. J., and Blackwell, M. (2006). Morphological and ecological similarities: wood-boring beetles associated with novel xylose-fermenting yeasts, Spathaspora passalidarum gen. sp. nov. and Candida jeffriesii sp. nov. Mycol. Res. 110, 1232–1241.
Nuotclà, J. A., Biedermann, P. H., and Taborsky, M. (2019). Pathogen defence is a potential driver of social evolution in ambrosia beetles. Proc. R. Soc. B 286:20192332. doi: 10.1098/rspb.2019.2332
Queller, D., and Strassmann, J. (1998). Kin selection and social insects. Bioscience 48, 165–175. doi: 10.2307/1313262
Roisin, Y. (1990). Queen replacement in the termite. Entomol. Exp. Appl. 56, 83–90. doi: 10.1111/j.1570-7458.1990.tb01383.x
Rosengaus, R. B., Maxmen, A. B., Coates, L. E., and Traniello, J. F. A. (1998). Disease resistance: a benefit of sociality in the dampwood termite Zootermopsis angusticollis (Isoptera: Termopsidae). Behav. Ecol. Sociobiol. 44, 125–134. doi: 10.1007/s002650050523
Schuster, J., and Schuster, L. (1985). Social behavior in passalid beetles (Coleoptera: Passalidae): cooperative brood care. Florida Entomol. 68, 266–272.
Schuster, J. C., and Schuster, L. B. (1997). “The evolution of social behavior in Passalidae (Coleoptera),” in The Evolution of Social Behavior in Insects and Arachnids, eds J. C. Choe and B. Crespi (Cambridge: Cambridge University Press), 260–269. doi: 10.1017/cbo9780511721953.013
Seibold, S., Müller, J., Baldrian, P., Cadotte, M. W., Štursová, M., Biedermann, P. H. W., et al. (2019). Fungi associated with beetles dispersing from dead wood – let’s take the beetle bus! Fungal Ecol. 39, 100–108. doi: 10.1016/j.funeco.2018.11.016
Smith, S. M., Kent, D. S., Boomsma, J. J., and Stow, A. J. (2018). Monogamous sperm storage and permanent worker sterility in a long-lived ambrosia beetle. Nat. Ecol. Evol. 2, 1009–1018. doi: 10.1038/s41559-018-0533-3
Strahl, S. (1988). The social organization and behaviour of the Hoatzin Opisthocomus hoazin in central venezuela. IBIS 130, 483–502. doi: 10.1111/j.1474-919x.1988.tb02714.x
Suh, S., and Blackwell, M. (2005). The beetle gut as a habitat for new species of yeasts. Insect Fungal Assoc. Ecol. Evol. 109, 244–256.
Suh, S.-O., Marshall, C. J., Mchugh, J. V., and Blackwell, M. (2003). Wood ingestion by passalid beetles in the presence of xylose-fermenting gut yeasts. Mol. Ecol. 12, 3137–3145. doi: 10.1046/j.1365-294x.2003.01973.x
Sun, Q., Haynes, K. F., and Zhou, X. (2018). Managing the risks and rewards of death in eusocial insects. Philos. Trans. R. Soc. Lond. B Biol. Sci. 373:20170258. doi: 10.1098/rstb.2017.0258
Sun, Q., and Zhou, X. (2013). Corpse management in social insects. Int. J. Biol. Sci. 9, 313–321. doi: 10.7150/ijbs.5781
Suzuki, S. (2013). Biparental care in insects: paternal care, life history, and the function of the nest. J. Insect Sci. 13:131. doi: 10.1673/031.013.13101
Tallamy, D. W., and Wood, T. K. (1986). Convergence patterns in subsocial insects. Annu. Rev. Entomol. 31, 369–390. doi: 10.1146/annurev.en.31.010186.002101
Tanahashi, M., Kubota, K., Matsushita, N., and Togashi, K. (2010). Discovery of mycangia and the associated xylose-fermenting yeasts in stag beetles (Coleoptera: Lucanidae). Naturwissenschaften 97, 311–317. doi: 10.1007/s00114-009-0643-5
Tanahashi, M., Matsushita, N., and Togashi, K. (2009). Are stag beetles fungivorous? J. Insect Physiol. 55, 983–988. doi: 10.1016/j.jinsphys.2009.07.002
Thorne, B. L. (1997). Evolution of eusociality in termites. Annu. Rev. Ecol. Syst. 28, 27–54. doi: 10.1146/annurev.ecolsys.28.1.27
Thorne, B. L., and Traniello, J. F. A. (2003). Comparative social biology of basal taxa of ants and termites. Annu. Rev. Entomol. 48, 283–306.
Tokuda, G., Lo, N., Watanabe, H., Arakawa, G., Matsumotos, T., and Noda, H. (2004). Major alteration of the expression site of endogenous cellulases in members of an apical termite lineage. Mol. Ecol. 13, 3219–3238.
Ulyshen, M. D. (2016). Wood decomposition as influenced by invertebrates. Biol. Rev. 91, 70–85. doi: 10.1111/brv.12158
Warnecke, F., Luginbühl, P., Ivanova, N., Ghassemian, M., Richardson, T. H., Stege, J. T., et al. (2007). Metagenomic and functional analysis of hindgut microbiota of a wood-feeding higher termite. Nature 450, 560–565.
Keywords: social evolution, social insects, log decomposition, saproxylic, microbial community, symbionts
Citation: Dillard J and Benbow ME (2020) From Symbionts to Societies: How Wood Resources Have Shaped Insect Sociality. Front. Ecol. Evol. 8:173. doi: 10.3389/fevo.2020.00173
Received: 28 December 2019; Accepted: 15 May 2020;
Published: 05 June 2020.
Edited by:
Peter H. W. Biedermann, Julius Maximilian University of Würzburg, GermanyReviewed by:
Christine Nalepa, North Carolina State University, United StatesBarbara Milutinovic, Institute of Science and Technology Austria (IST Austria), Austria
Copyright © 2020 Dillard and Benbow. This is an open-access article distributed under the terms of the Creative Commons Attribution License (CC BY). The use, distribution or reproduction in other forums is permitted, provided the original author(s) and the copyright owner(s) are credited and that the original publication in this journal is cited, in accordance with accepted academic practice. No use, distribution or reproduction is permitted which does not comply with these terms.
*Correspondence: Jacqueline Dillard, anJkbGlsYXJAbmNzdS5lZHU=; anJkaWxsYXJAbmNzdS5lZHU=