- 1Department of Poultry Science, University of Georgia, Athens, GA, United States
- 2Warnell School of Forestry and Natural Resources, University of Georgia, Athens, GA, United States
As humans continue to infringe on natural habitats, more animals are exposed to urbanization and its associated challenges. It is still unclear, however, whether the movement of animals into urban habitats negatively influences the health and/or survival of those animals, however those animals often experience shifts in resource availability, diet composition, and exposure to stimuli that are new and potentially stressful. Recently, white ibises (Eudocimus albus) have become increasingly common in urban habitats where they forage in close proximity to humans and even interact with them, collecting food handouts. We hypothesized that foraging in urban habitats would negatively impact measures of health, impair innate immunity, trigger elevated concentrations of corticosterone, and depress physiological responses to stressors in white ibises. We found that plasma from birds captured from urban sites had higher bactericidal capacity against Escherichia coli than those captured in natural sites. Additionally, adults captured in urban habitats had a significantly lower baseline corticosterone concentrations during the post-breeding season, and corticosterone responses to a handling challenge were lower for birds captured from urban sites during year 2 of the study. These results indicate that exposure to urban habitats impacts ibis health, though in the opposite direction of what was predicted.
Introduction
The ability of a species to adapt to, and subsequently succeed in, urban environments is an area of study of increasing importance as urbanization continues to transform natural habitats. Central to survival in a changing environment is the ability to recognize and aptly respond to novel situations or factors within a short period of time and without adverse effects on health (Bonier, 2012). The effects of urbanization are often species-specific, making some species better-equipped for urban habituation than others (Bonier et al., 2007; Evans et al., 2011). Supplemental resources in urban habitats provide wildlife with opportunity, however moving to urban habitats often means exposure to new sets of challenges that wildlife must acclimate or even adapt to if they are to exploit the resources provided there (Lepczyk et al., 2004; Vincze et al., 2016; Bradley and Altizer, 2007); this is particularly relevant when discussing species of conservation concern, and those whose natural habitats are disappearing.
Historically, it was assumed that urban environments were suboptimal for most species when compared with their native habitats. Frequent exposure to new stimuli [e.g., humans, lights, anthropogenic noise, etc. (McKinney, 2006)] or inadequate nutritional quantity or quality (Becker et al., 2015) can act as challenges to wildlife. In vertebrates, environmental challenges are often transduced into physiological signals through the hypothalamic-pituitary-adrenal axis, which functions to shift energy balance to critical physiological functions needed during the challenge (Sapolsky et al., 2000). In birds, the glucocorticoid corticosterone is the main adrenal hormone that responds during such a challenge, and there are some studies showing that exposure to urban environments may result in higher concentrations of corticosterone in birds (Zhang et al., 2011; Russ et al., 2015). However, many studies have shown no relationship between living in urban environments and circulating corticosterone concentrations (reviewed in Bonier, 2012 and Injaian et al., 2020) and still other studies indicate that individuals in urban environments may instead have lower concentrations of circulating corticosterone (Schoech et al., 2004; French et al., 2008). These studies call into question whether living in an urban environment is, in fact, challenging to animals. Indeed, many species have adapted to and are still abundant in urban environments.
Also of concern are the potential effects that urban exposure may have on the immune system. Urban environments often bring with them novel pathogens and higher population densities, which can facilitate higher rates of pathogen transmission and parasites (Delgado-Valez and French, 2012; Becker et al., 2015). Novel dietary components consumed in urban environments may be limited in critical nutrients or overall caloric availability (Becker et al., 2015), or may even be contaminated (Milner et al., 2014). This could have dramatic effects particularly for aspects of the immune response that are energetically costly or require specific nutrients. For example, T-lymphocytes require specific protein levels and types to function properly (Lochmiller et al., 1993; Murray et al., 2016), and a meta-analysis of birds revealed that an immune response comes with a significant elevation in energy consumption (Hasselquist and Nilsson, 2012). Finally, elevations of glucocorticoids have well-documented suppressive effects on immunological responses (Kleist et al., 2018; Padgett and Glaser, 2003), an important factor if urban environments do, in fact, provoke an adrenal response. There are some studies in which exposure to urban habitats depressed immune responses in birds (Zylberberg et al., 2013; Bailly et al., 2016). Still other studies show no difference in immune responses between urban and rural animals (Iglesias-Carrasco et al., 2017; Amdekar et al., 2018), and in some studies, immune responses are larger in urban individuals (Audet et al., 2015; Møller, 2009). Once again, it is unclear whether exposure to urban environments is truly challenging to wildlife.
To better understand how exposure to urban habitats influences physiology in wildlife, we measured how a measure of innate immune function, plasma bactericidal capacity against both Escherichia coli and Salmonella paratyphi B., varies in American White Ibises by the habitat (urban vs. natural) in which the birds were captured. This measure of immune function is influenced by challenging conditions such as captivity, handling, and restraint (Matson et al., 2006; Buehler et al., 2008; Merrill et al., 2012; Gao et al., 2017). We also measured baseline corticosterone and corticosterone elevations in response to handling to assess whether urban ibis show higher baseline concentrations of this hormone and whether they exhibit differences in their abilities to respond to a psychological challenge. A depressed ability to produce corticosterone in response to a challenge may indicate a downregulation of the hypothalamic-pituitary-adrenal axis in response to chronic physiologically challenge (Sapolsky et al., 2000), or alternatively, a heightened response could indicate a heightened state of psychological reactivity (Pusch et al., 2018). We hypothesized that, if foraging in urban environments and consuming anthropogenic resources is harmful to ibises, ibises captured in urban environments would have dampened plasma bactericidal capacity, higher levels of baseline corticosterone, and differences in the way they respond to the psychological stress of handling.
Materials and Methods
Study Species
American white ibises (Eudocimus albus) are wading birds commonly found in wetlands along the coast of the southeastern United States. While they continue to breed in mixed-flock rookeries in freshwater wetlands, white ibises have recently become frequent foragers in urban habitats throughout South Florida (Boyle et al., 2014). Florida is one of the most rapidly urbanizing landscapes in the United States, and anthropogenic development, contamination, and draining have degraded the wetlands (Chimney and Goforth, 2001; Dorn et al., 2011). Likely in response to wetland degradation in Florida, ibis nesting numbers have declined significantly over the past 80 years in the state of Florida, and they are now listed as a Species of Concern (Crozier and Gawlik, 2003; Frederick et al., 2009). White ibis naturally prey on aquatic invertebrates, fish, and insects (Kushlan, 1986; Bancroft et al., 2002). As a result of recent changes to wetland ecosystems, water levels are often suboptimal for natural foraging and natural prey abundance for white ibis fluctuates more than it has naturally in the past. Ibis are now commonly found foraging throughout urban parks, zoos, and residential neighborhoods (Dorn et al., 2011). While they can maintain their natural foraging behavior and consume terrestrial invertebrates and aquatic organisms in these habitats, portions of their diet come directly from human handouts (e.g., park goers tossing bread) or landfills (Murray et al., 2018). The provisioned resources ibis consume vary in carbohydrate and protein content from their natural diets and these diets may have implications, both beneficial and detrimental, on their physiology and the habitat types that this nomadic species chooses to inhabit (Kidd, 2018; Murray et al., 2018).
Previous studies have shown varying effects of urbanization and provisioned diets on the health and ecology of the American white ibis. Murray et al. (2018) found a negative relationship between urbanization and body condition, likely a result of shifts in dietary quality, as birds captured from more urbanized sites consumed diets higher in urban resources; Hernandez et al. (2016) reported a higher prevalence of Salmonella spp. in ibis captured in landscapes affected by urbanization and Curry (2017) showed that the degree of urbanization at sites where ibis were captured was positively related to fecal glucocorticoid concentrations. These results indicate ibis health may respond negatively to urbanization. However, ibis captured in more urban habitats had fewer ectoparasites (Murray et al., 2018), indicating potential tradeoffs in ibis physiology when foraging in urban areas. In fact, reliable access to supplemental resources (or other urban-associated benefits) may allow urban ibis to commit more time for other activities, such as preening (i.e., to remove ectoparasites).
Study Area
To assess how urban resource provisioning affects ibis health, plasma bactericidal capacity and corticosterone concentrations, we captured ibises at 18 sites within Palm Beach County (PBC), Florida, a highly developed county with portions of the Everglades wetland ecosystem located immediately to the west where white ibis are commonly found. Ten sites were classified as urban and 8 as natural; Classifications were made based on impervious land cover and ibis behavior, such that urban sites were those where birds were seen being fed or consuming provisioned resources and were habituated enough to human presence to be approached within 30 ft, while natural sites contained birds that would flush an area if a human came within 30 ft. Urban sites included several public parks, a zoo, a landfill, and a residential cul-de-sac. Natural sites consisted of either constructed, restored, or undisturbed wetlands. Details of the sites are shown in Figure 1.
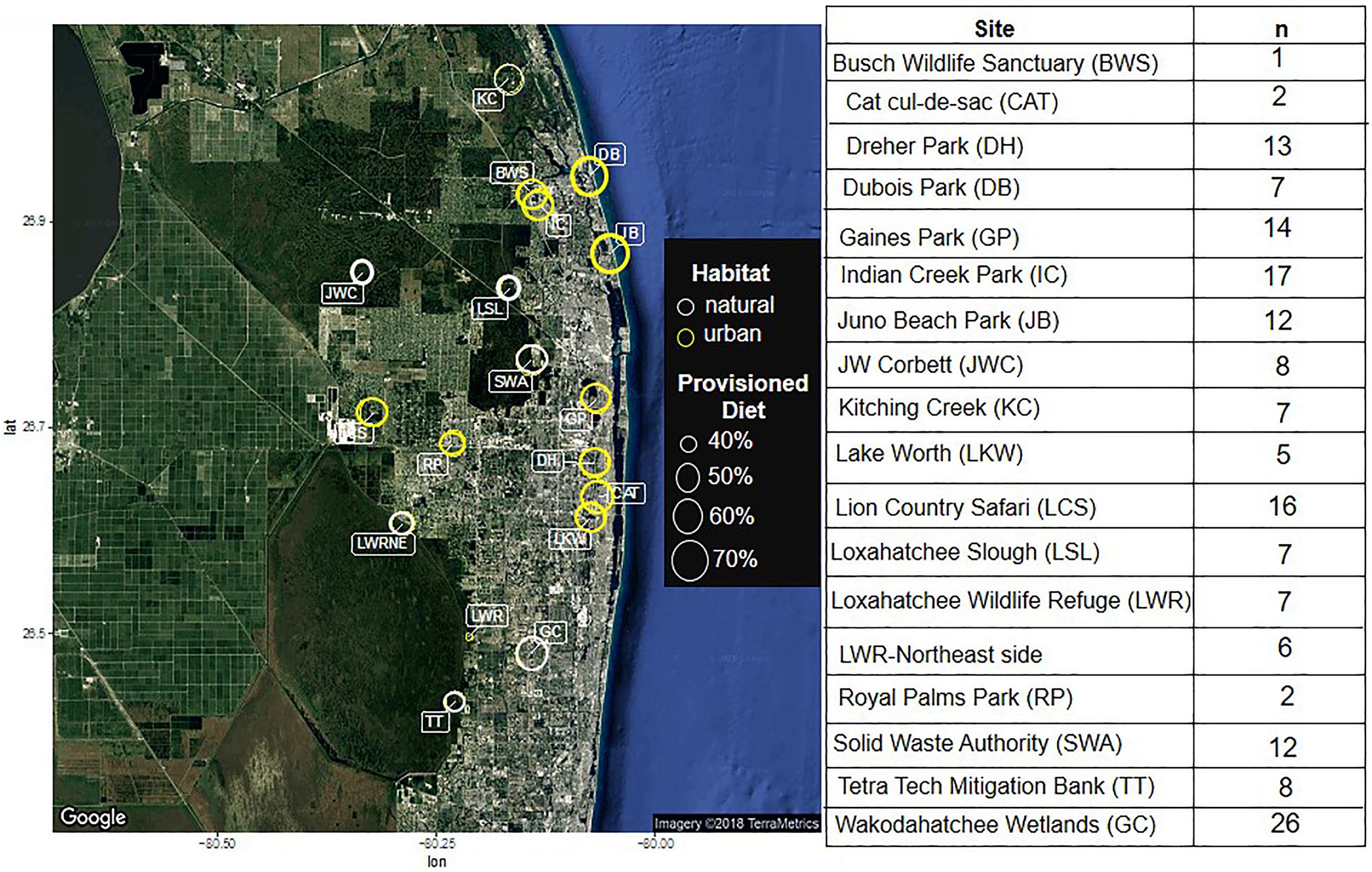
Figure 1. A diagram of the sampling locations, with urban environments circled in yellow and natural environments circled in white. The size of the circle indicates the proportion of the diet that contains anthropogenic sources, as indicated by radioisotope analyses conducted in Murray et al. (2018). The table contains the number of animals captured at each site.
Capture and Sample Collection
We captured ibises during 6 field seasons from 2015 to 2017; these were categorized based on by weather fluctuations and ibis life history as pre-breeding (February and March), breeding (June and July), and post-breeding (October and November) seasons (Heath and Frederick, 2003). Ibis were either captured using nylon leg lassos or a flip-nets (urban sites) or ibis decoys and mist nets (natural sites) (Heath and Frederick, 2003; Hernandez et al., 2016). To obtain a baseline blood sample, birds were physically restrained immediately after capture (while still in net or lasso) for blood collection from the medial metatarsal vein within 3 min of capture to avoid the confounds of handling stress, because Romero and Reed (2005) showed that birds can begin mounting a stress response even prior to 3 min after the beginning of handling in some cases. Thereafter, birds were held in well-ventilated pillowcases until we collected biological samples, mass, and morphometric measurements. Blood was then collected at two additional time points (15 min post-capture and 30 min post-capture) from either the metatarsal or jugular vein to assess stress responses provoked by handling of birds; it has been shown in many avian species that this is an adequate amount of time to induce a stress response resulting in an increased concentration of corticosterone in the blood (Romero and Romero, 2002).
Blood was transferred to heparinized tubes and immediately stored in a cooler with frozen gel packs. Blood samples were centrifuged within 2 h of collection for 10 min at 3,500 × g to obtain the plasma for our analyses. We then aliquoted 80 uL from time points A and B into individual cryovials for use in bactericidal assays and 80 uL from time points A, B, and C into individual cryovials for use in corticosterone analyses. Aliquots were stored in liquid nitrogen and at the end of a field season transferred to a −80°C freezer for subsequent analyses, which were conducted within 6 months of collection.
For the measure of general health, body condition was calculated as the residuals of the regression of mass to tarsus length. All methods used were approved by the University of Georgia Institutional Animal Care and Use Committee (AUP# A2016 11-019-Y3-A3).
Bactericidal Assays
We used bactericidal assays following Matson et al. (2006) with minor modifications to measure in vitro bactericidal capacity of plasma using an assay that gives a proportion of colony-forming units (CFU) that have been killed by the plasma compared to a control without plasma. Quantification of bactericidal capacity was performed using both Escherichia coli from a working culture following manufacturer’s instructions (E. coli ATCC 8739 pellets, Microbiologics, St. Cloud, MN, United States) and S. paratyphi B, a strain that was isolated from white ibises and the habitats where they forage. Aliquots of E. coli and S. paratyphi were made from dilutions of each bacteria with a mixture of 80% culture broth (Sigma-Aldrich Inc., St. Louis, MO, United States) and 20% glycerol (VWR International, Radnor, PA, United States) at a dilution which resulted in ∼600 CFUs/10 μL when plated and incubated at 37°C. Ibis plasma was diluted with CO2-independent media (Life Technologies, Carlsbad, CA, United States) enriched with 4 mM L-glutamine (VWR International). For use in E. coli assays, plasma dilutions were optimized to achieve an average of ∼30–80% killing. The plasma dilutions needed to achieve this varied substantially between seasons and years, ranging from 1:70 in 2015 to 1:10 in 2017. The variation in plasma dilutions is likely due to the variation in bactericidal capacity by season and year; there were no obvious changes in storage conditions or assay methods that explained this variation. For all S. paratyphi assays, we used a 1:2 (60 μL plasma + 60 μL media mixture) plasma dilution; no variation in dilution was necessary. 120 μL of each plasma dilution was then separately combined with 40 μL of each bacterial aliquot (from pre-made aliquots) and left to incubate at room temperature for 30 min. Controls contained only bacteria and media. 50 μL of all plasma-bacteria combinations and controls were plated onto tryptic soy agar plates (VRW International) in duplicate, and all plates were incubated at 37°C for 16h, after which the number of growing colonies were counted. The average of the 2 plates per sample, including the controls, was taken; the bactericidal capacity for each sample was then calculated as:
(mean control colonies-mean sample colonies)/mean control colonies
Bactericidal capacity was primarily assessed using time point A plasma, however, some A samples did not contain enough plasma for both Salmonella and E. coli assays, so B results were reported for E. coli in those cases. In a separate preliminary analysis, we tested whether bactericidal capacity against E. coli was similar between A and B time points by comparing 10 sets of samples using a simple regression analysis. For 9 of 10, samples between the two time points were highly correlated (R2 = 0.90, p < 0.0001). Only 1 was substantially different between the two (11% killing versus 99%) and we believe this was due to sampling error. Thus we felt comfortable using B samples when A samples were not present. In total, we assessed 89 urban and 83 natural ibises for bactericidal ability against E. coli and 55 urban and 50 natural ibises for bactericidal ability against S. paratyphi.
Because the scales of plasma dilutions needed to achieve bacterial killing within a measurable range varied widely among seasons and years for our assays involving E. coli, we needed to standardize the data to allow true comparisons of bactericidal capacity. To allow for integration of all plasma dilutions used in E. coli bactericidal assays (1:70, 1:25, 1:10) into one analysis, we converted initial E. coli bactericidal values to a uniform scale by multiplying the proportion of colonies killed from each sample by the dilution factor used over the maximum dilution factor used (70) (equation below). This transformed bactericidal values from assays using a 1:25 or 1:10 dilution to a value calculated on a 1:70 scale (e.g., how many colonies the sample would kill using a 1:70 dilution).
Transformed bactericidal capacity = proportion of colonies killed x (X∗/70)
∗ X = dilution factor used in bactericidal assay (either 70, 25, or 10)
This calculation assumes a linear relationship between plasma dilution and bactericidal capacity, which is what we have generally seen in previous validation experiments.
Plasma Corticosterone Analyses
Plasma corticosterone was measured at the three time points (A, B, and C = 0 min, 15 min and 30 min after capture) using an enzyme-linked immunosorbent assay (ELISA; Corticosterone ELISA Kit, Enzo Life Sciences) (Herring et al., 2011). We followed kit instructions for a small sample volume of serum/plasma, resulting in a 1:40 dilution of each plasma sample. Time point A was regarded as each individual’s baseline. Because the highest corticosterone concentration was found at Time point B for some birds and Time point C for others, the peak corticosterone response was then calculated as the baseline subtracted from the highest corticosterone concentration (either time point B sample or time point C sample). We only used individuals for which all three time points were available to calculate peak corticosterone responses. This resulted in a total of 160 observations of baseline corticosterone (80 urban, 80 natural) and 148 (76 urban, 72 natural) observations of peak corticosterone responses. Inter-assay variation for these kits did not exceed 8%.
Statistical Analyses
To ensure that variables met model assumptions, we arcsin transformed bactericidal values and log transformed corticosterone concentrations for analyses. To explore whether the habitat in which birds were captured was related to body condition, differences in plasma bactericidal capacity, baseline corticosterone concentrations, and the maximum corticosterone elevations in response to handling, we used separate general linear models for each dependent variable. We included habitat (urban or natural), sex (male or female), season of capture (pre-breeding, breeding, or post-breeding), and year of capture (1 or 2), as well as the interactions between habitat and each of these variables as predictor variables. Prior to fitting the model, we checked for multicollinearity and excluded any highly correlated variables. To further tease out interaction effects, Fisher’s PLSD post hoc tests were used. In addition, to test whether birds captured in the two habitats differed in the timing of their corticosterone peaks, we used a logistic regression, assigning birds a code of “1” when their highest concentration of corticosterone occurred at timepoint B and “2” when it occurred at timepoint C. Finally, we conducted simple regression analyses to test for relationships among the dependent variables. All analyses were done using JMP® Pro, Version 15. SAS Institute Inc., Cary, NC, 1989–2019.
Results
Influences of Habitat on Plasma Bactericidal Capacity
We captured 170 ibises at 18 sites. For overall ibis health, we found that body condition was not different between birds captured in urban versus natural environments, but was related to sex, age, and season of capture (F1,165 = 5.60, p < 0.0001, Table 1). Post hoc analyses indicated that body condition was highest in males (p < 0.0001) and adult birds (p = 0.0056), and was higher in the pre-breeding season (Feb-Mar) compared to breeding (p < 0.0001) and post-breeding (p = 0.0013) seasons.
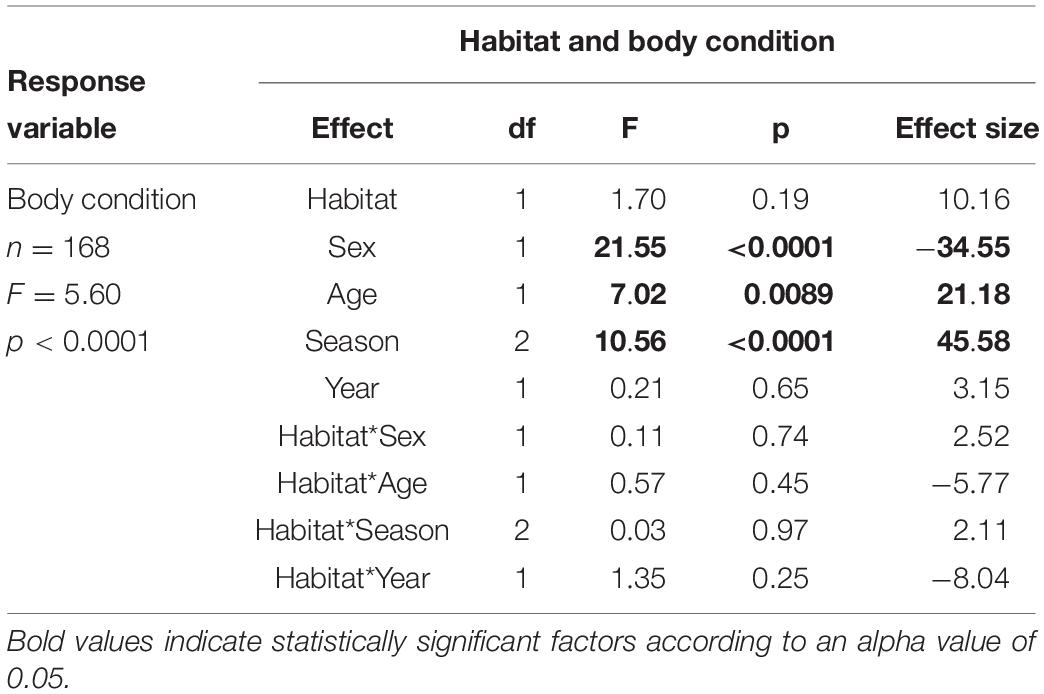
Table 1. Results of model testing whether body condition relates to habitat (urban or natural) in which a bird is captured, sex, age, season, and year of capture.
We then explored whether being found in an urban habitat influenced plasma bactericidal capacity, and whether any effects varied with season and year during which samples were collected, and the age of the birds. Our model analyzing effects on plasma bactericidal capacity against E. coli indicated that the ability of birds to kill E. coli differed significantly based on the habitat in which the birds were captured; birds captured in urban habitats showed significantly better abilities to kill E. coli compared with birds captured in more natural habitats (F1,171 = 29.67, p < 0.01) (Table 2 and Figure 2). This was only true for plasma bactericidal capacity against E. coli, however.
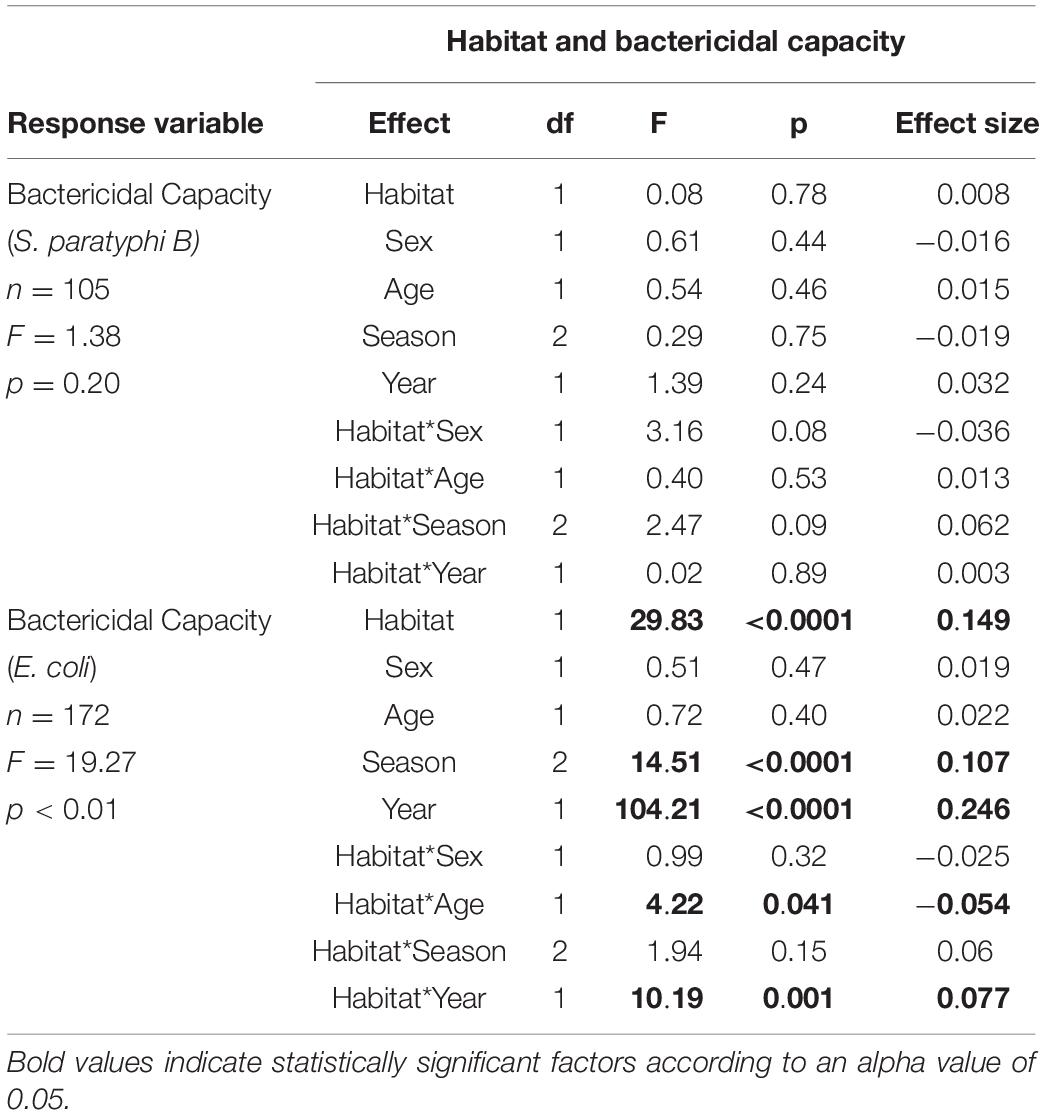
Table 2. Results of model testing whether the ability of blood plasma to prevent the growth of E. coli and S. paratyphi relates to habitat (urban or natural) in which a bird is captured, sex, age, season, and year of capture.
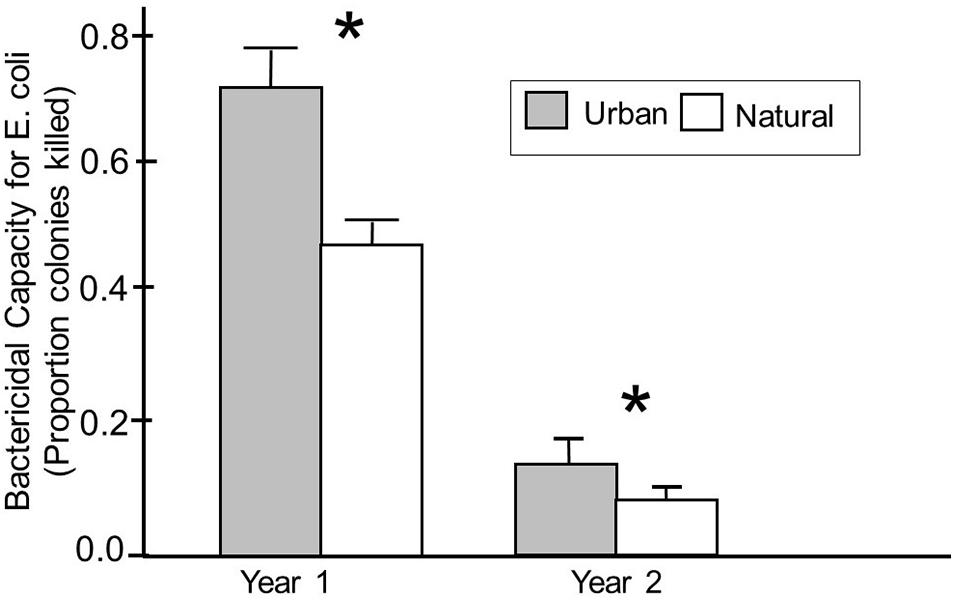
Figure 2. Bactericidal capacity against E. coli in plasma from birds captured in urban and natural habitats in years 1 and 2 of the study. Habitats were classified based on behavior of birds at that site, particularly the likelihood that a bird would be habituated to humans at that site. Numbers inside each box indicate sample sizes, and an asterisk indicates a significant difference in bactericidal capacity between urban and natural birds based on Fishers PLSD analyses.
A significant habitat year interaction indicates that this effect was more pronounced during year 1 than year 2. In fact, birds captured in year 1 showed significantly higher capacities to kill E. coli compared to birds captured from year 2 (F1,171 = 101.95, p < 0.01) (Table 2). This difference was so dramatic that we needed to use plasma that was 7 times more dilute in year 1 compared to samples run in year 2 (see Figure 2). Plasma bactericidal capacity against S. paratyphi also differed between years, but the difference was not as pronounced. The final model for S. paratyphi indicated no significant differences in the abilities of birds from urban versus natural habitats to kill S. paratyphi (F1,104 = 0.09, p = 0.76) (Table 3).
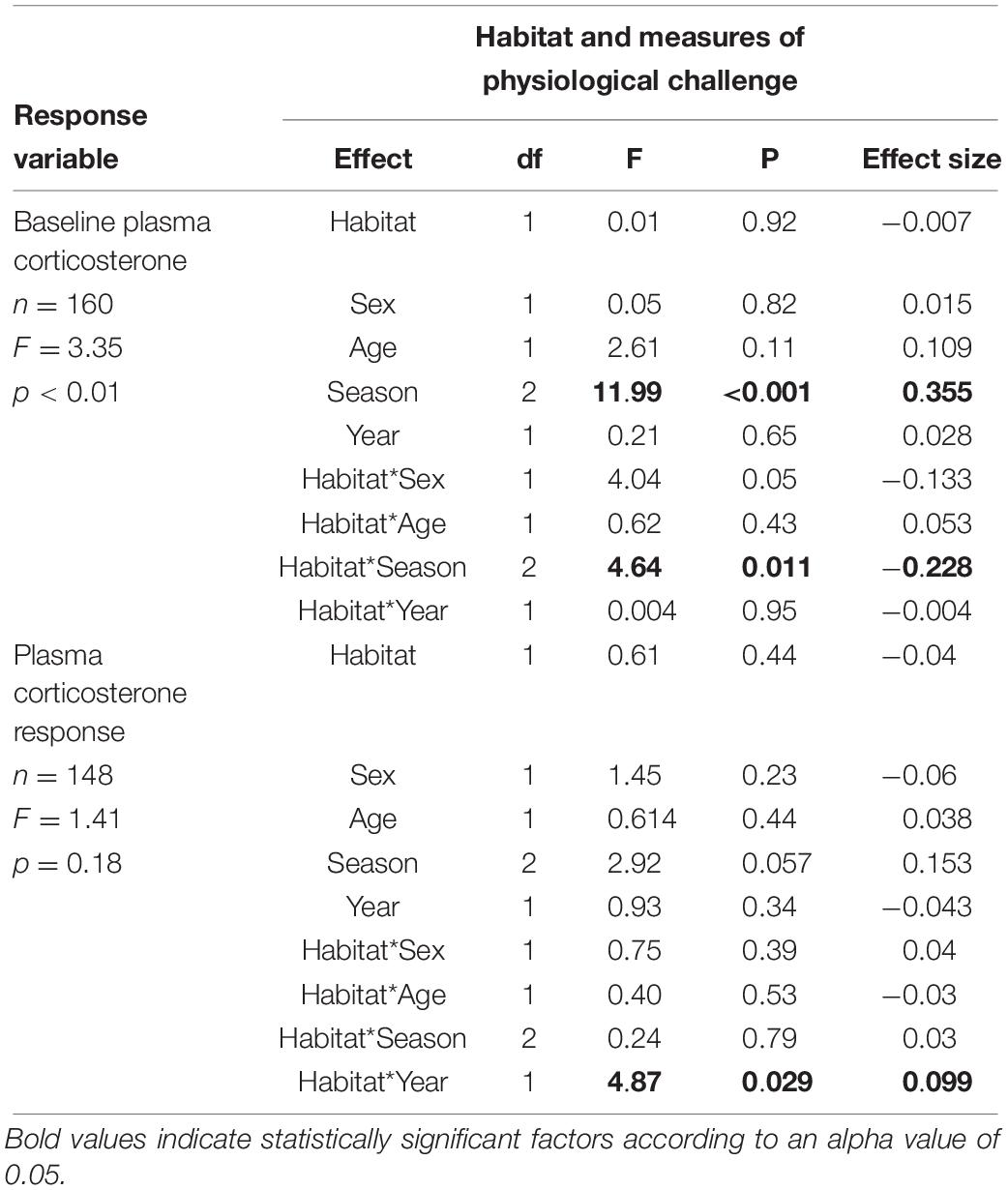
Table 3. Final model testing the influences of habitat baseline concentrations of corticosterone, and changes in concentrations of circulating corticosterone in response to the stress of handling.
We also found that plasma bactericidal capacity varied substantially based on the season of capture. The ability of birds to kill E. coli was significantly lower in the breeding season (June and July) compared to the other two seasons (F2,170 = 14.36, p < 0.01) (Table 1 and Figure 3), while there was no seasonal variation in the ability to kill S. paratyphi (F2,146 = 0.09, p = 0.51).
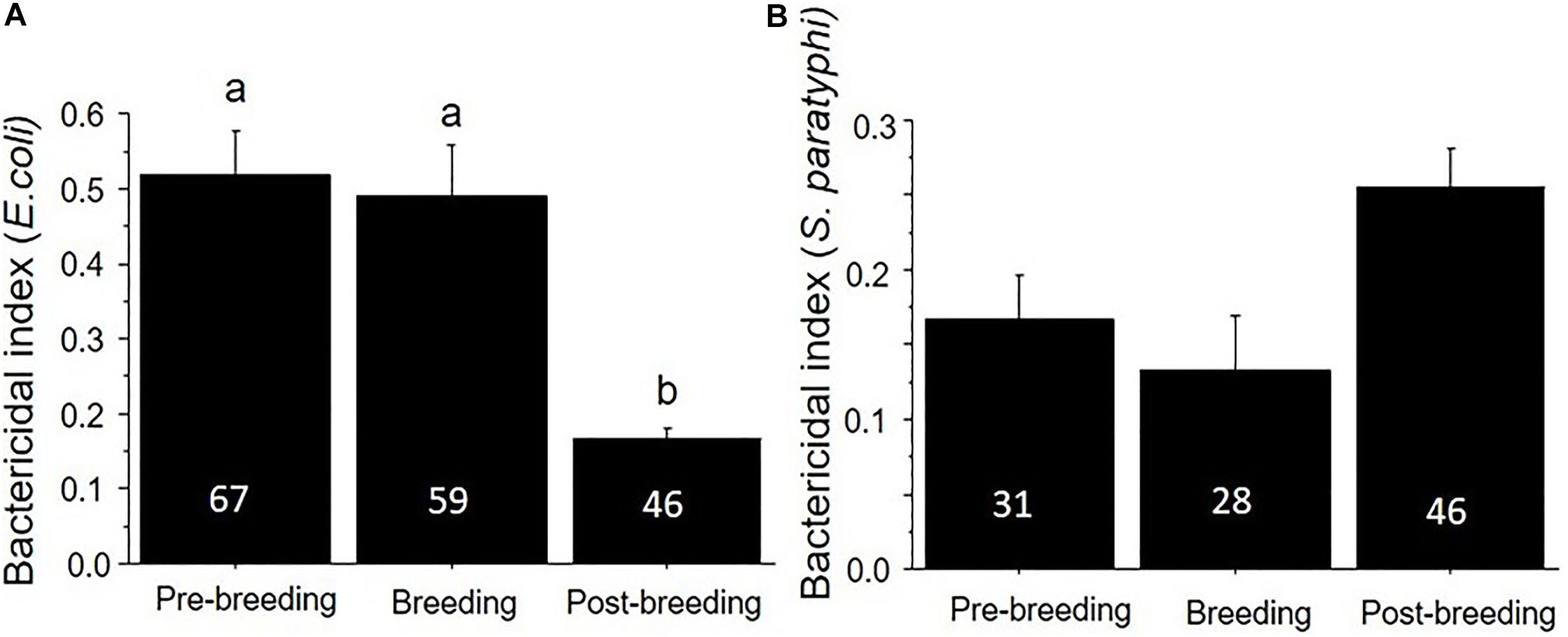
Figure 3. Bactericidal capacity (mean ± SE) against (A) E. coli and (B) S. paratyphi in plasma collected from birds in the pre-breeding, breeding, and post-breeding seasons. Numbers in the bars indicate sample sizes and different letters above bars indicate significant differences. Urban and natural populations were combined in these figures.
Finally, we also found an effect of habitat on the relationship of how plasma bactericidal capacity against E. coli related to age. In natural environments, juveniles had significantly higher bactericidal capacities than adults (p = 0.04), but there was no significant differences between the age groups in urban birds (p = 0.21) (Figure 4). Plasma bactericidal capacity was numerically greater in urban birds compared to natural birds for both age groups, indicating a greater difference between natural and urban birds in the adult group compared to the juveniles.
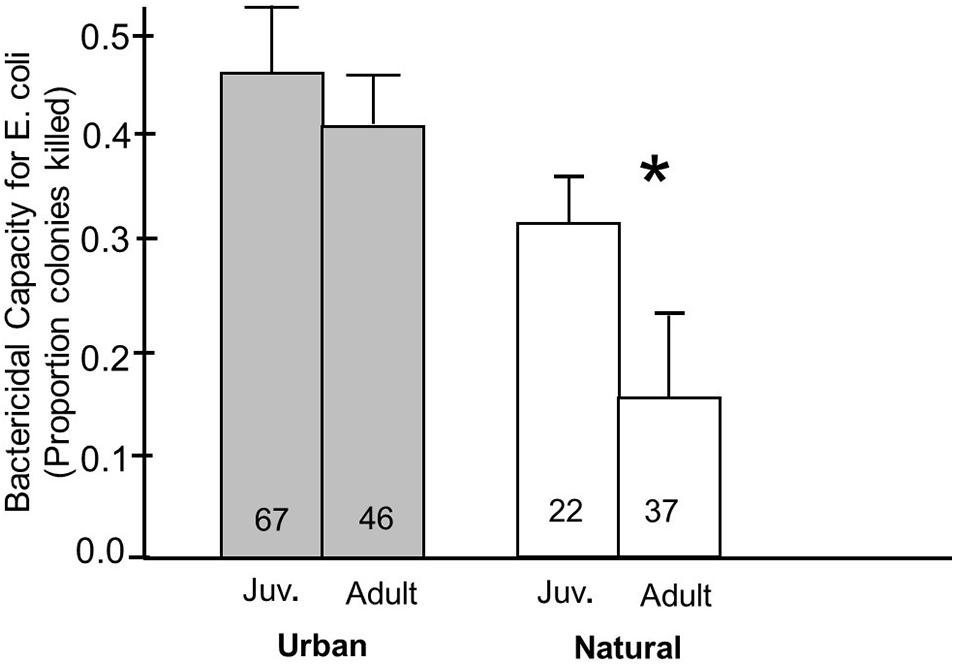
Figure 4. Bactericidal capacity against E. coli in plasma from juvenile and adult birds breeding in urban and natural habitats. A Fisher’s PLSD post hoc analysis was used to determine whether breeding in an urban or natural site influenced the differences in bactericidal capacity in juvenile and adult birds. In natural environments, juvenile birds had significantly higher bactericidal capacity against E. coli than natural birds. This relationship was not present in urban environments. Numbers in the bars indicate sample sizes and an asterisk above indicates a significant difference between juvenile and adult birds.
Baseline Corticosterone
We next explored whether being found in an urban habitat influenced baseline corticosterone concentrations, and whether any effects varied with season and year during which samples were collected, as well as with the age of the birds. There was no significant effect of habitat on baseline corticosterone overall (F1,159 = 0.01, p = 0.92) (Table 2). There was, however, a significant interaction between habitat and season (F2,158 = 4.64, p = 0.01) (Table 2). When examining the relationships between habitat and baseline corticosterone in individual seasons using post hoc analyses, the direction of the patterns differed between seasons; in the post-breeding season (October and November), urban birds had significantly lower concentrations of corticosterone in circulation compared to natural birds (p = 0.03), while there was no difference between urban and natural birds in the other two seasons (breeding: p = 0.96, pre-breeding: p = 0.13) (Figure 5).
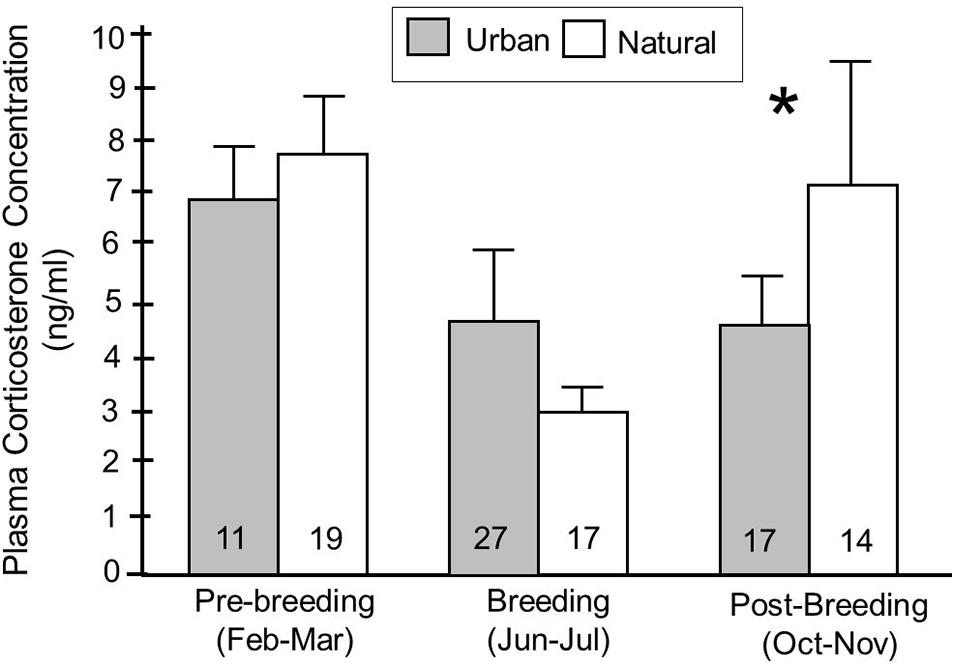
Figure 5. Baseline corticosterone concentrations (means ± SE) in birds captured in urban and natural habitats, split according to whether birds were captured in the pre-breeding (February-March), breeding (June-July), or post-breeding (October-November) seasons. Fisher’s PLSD post hoc analyses were used to examine whether corticosterone concentrations were significantly different between habitats in each of the seasons. Habitats were classified based on behavior of birds at that site, particularly the likelihood that a bird exhibited evidence of habituation to humans at that site. Numbers in the bars indicate sample sizes and an asterisk indicates that values were significantly different between urban and natural habitats.
Birds captured in urban habitats show a different seasonal profile in baseline corticosterone concentrations compared to natural birds; natural birds have low levels of corticosterone in the breeding season (June and July) compared to all other seasons (F2,77 = 11.23, p < 0.01) while urban birds have low corticosterone concentrations during both the breeding and post-breeding (October and November) seasons (F2,77 = 3.25, p = 0.05) (Figure 5).
Stress-Induced Corticosterone Responses
When examining the corticosterone response to handling stress (i.e., the change in corticosterone from baseline to maximum levels during handling), we found a significant habitat by year interaction (F1,147 = 4.18, p = 0.04); post hoc analyses showed that the peak corticosterone response was significantly higher in the natural habitat compared to the urban habitat, but only during the second year of the study (p = 0.01). Finally, a logistic regression analysis showed that birds from the two habitats did not differ in terms of whether their peak corticosterone responses occurred at 15 or 30 min after capture (χ2 = 0.99, p = 0.32).
Discussion
In this study, we tested whether foraging in urban habitats influences measures of health, physiological challenge, and immunity in white ibises. We predicted that foraging in urban habits would lead to indications of impaired health, including lower body condition, decreased ability to kill bacteria in vitro, higher concentrations of corticosterone, and depressed physiological responses to a handling stress. Instead, we found the opposite. Plasma from urban ibises was more capable of preventing growth of one bacterial species. Ibises from urban habitats also had lower concentrations of corticosterone in circulation compared to natural ibises during the post-breeding season, and had lower responsiveness to stress than natural ibises during year 2 of the study. Overall, these results indicate that foraging in urban habitats may not be as physiologically challenging as was initially predicted.
While our results did not match our predictions, they do match results of studies in some other species. For example, in adult tree swallows (Tachycineta bicolor), birds living in areas with a high level of agricultural development had higher levels of plasma bactericidal capacity, the same measure employed in the current study (Schmitt et al., 2017). Barbados bullfinches (Loxigilla barbadensis) captured in urban environments had larger T-cell mediated immune responses to phytohemagglutinin compared to rural birds (Audet et al., 2015). Further, in a study of 39 avian species found in urban environments, urban birds generally had larger bursa of Fabricius, the organ responsible for producing B-lymphocytes in young birds (Møller, 2009). Garamszegi et al. (2007) suggested that animals exhibiting higher levels of feed innovation tend to have larger bursa of Fabricius as a result of parasite-mediated selection. This would indicate that exposure to novel food brings with it an exposure to novel pathogens, and as a result, selection for a more robust set of immune responses. If this is in fact the case, it is possible that we are seeing selection for a more robust innate immune response, particularly the ability to kill bacteria in the bloodstream.
It is important to note, however, that we measured a completely different aspect of the immune system that is generally unrelated to bursal size. The bactericidal capacity of plasma is a constitutive response that is largely dependent on complement activity using proteins that are already in circulation (Merchant et al., 2003). Studies suggest that this measure of immunity is negatively influences by stress (Matson et al., 2006; Buehler et al., 2008; Merrill et al., 2012; Gao et al., 2017), is positively correlated to the presence of ectoparasites in another species (Girard et al., 2011), and may also be heritable (Stambaugh et al., 2011). These studies add promise to the idea that bactericidal capacity may indicate health and could be a target of selection in ibises. However, to our knowledge, there are no studies examining the energetic cost, if any, of constitutive bactericidal capacity, and we still need to know more about how this measure relates to the ability of an animal to battle disease.
If, in fact, white ibises are undergoing selection for a more potent bactericidal capacity, it is unclear why the bactericidal response to E. coli was different between the two habitats, but the response to S. paratyphi was not. While measuring bactericidal capacity in plasma against E. coli tends to be the standard, we tested it against S. paratyphi because previous studies suggest that ibises are carriers of Salmonella to natural environments and this strain, in particular, is a pathogenic strain of Salmonella that is found at higher loads in ibises captured in urban parks (Phalen et al., 2010; Hernandez et al., 2016). It was surprising to see such a low response to this bacterial species, and the reason behind the difference in patterns of bactericidal capacity against the two species remains unclear. However, Tieleman et al. (2010) also showed that bactericidal capacity differs against different pathogens. The process of bacterial killing via complement is complex; complement can mark the bacteria for destruction by phagocytes or bind itself to the bacteria and activate proteases that kill the bacteria (Blom et al., 2009). As a result, the mechanisms of defense against E. coli and S. paratyphi could differ, and the availability of cell types and/or functional components needed to carry out the bactericidal activity for each bacterial type could be differentially influenced by environmental variables. Thus, it’s possible that we are seeing selection on an aspect of the immune system that is particularly suited to combat invasion by E. coli and other species that provoke a similar response. We must also consider that these pathogens tend to infect animals via an oral or fecal route, invading the digestive system. Immunity in the gut can differ from levels of immunity in plasma, so the plasma assay that we used here may not accurately show how well the ibis can combat one or both of these pathogens. In future work, it is important to test additional aspects of the immune system in urban and rural ibises.
Our baseline plasma corticosterone values (mean ± SE = 5.92 ng/mL ± 0.45) were comparable to previously recorded values in white ibis (Herring et al., 2011) and there are also studies to support our finding that urban ibises had lower corticosterone concentrations during part of the year. Urban tree lizards (Urosaurus ornatus) had lower concentrations of corticosterone and lower heterophil:lymphocyte ratios (French et al., 2008), which is often used as a measure of long-term physiological challenge, compared to natural counterparts. Similarly, urban Florida scrub jays had lower concentrations of corticosterone in circulation than woodland jays (Schoech et al., 2004). There is even evidence that urban animals may process stressful stimuli differently than natural counterparts; urban blackbirds have different single nucleotide polymorphisms for genes that are associated with anxiety-related traits (Müller et al., 2013). Our finding that natural birds had higher stress responses than urban birds supports the idea that these birds are more anxious or psychologically reactive (Pusch et al., 2018), though it is unclear why we only saw this pattern in year 2 of our study and not in year 1. More work is needed to further understand whether urban ibises process stressful stimuli differently than those in natural environments, and what environmental conditions may influence those processes.
It is unclear why the relationship we found between habitat and baseline corticosterone concentrations was only present in the post-breeding season (October-November). In birds captured in natural habitats, corticosterone concentrations increased significantly after the breeding season, but in urban birds, concentrations remained low through the post-breeding season. This seasonal pattern is not uncommon in birds; glucocorticoids tend to decrease during breeding and rise when birds are not breeding (Romero, 2002). Other studies have also shown that the relationship between corticosterone concentrations in urban and natural birds varies seasonally. For example, Fokidis et al. (2009) found that urban northern mockingbirds (Mimus polyglottos) had lower baseline corticosterone levels than birds in natural habitats during the non-breeding season, but this effect disappeared during breeding and post-breeding/molting seasons. So while there was seasonal variation in both species, our pattern does not match that seen in the northern mockingbirds.
It is likely that the life history of white ibises during the post-breeding season drives the habitat-related patterns of baseline corticosterone that we saw. Using stable isotope of urban ibis feces, Murray et al. (2018) showed that urban ibises took in more anthropogenic food during the post-breeding season than any other season. Further, our ibises experienced a significant drop in body condition between our measure during the breeding season (June-July) and our post-breeding measure (October-November). Perhaps the consumption of anthropogenic food alleviates challenges associated with foraging and decreases corticosterone concentrations as a result. Indeed, work in multiple avian species shows elevated corticosterone concentrations when food resources are limited (e.g., Kitaysky et al., 1999; Doody et al., 2008). More studies must be done to tease out the relationships between resource availability and corticosterone concentrations in urban and natural environments.
We are still left with a question of why plasma bactericidal capacity and that habitat differences in the corticosterone responses to handling stress differed substantially between the 2 years of the study. While it is possible that the yearly effects in plasma bactericidal capacity were due to the age of the bacteria used, we think this is unlikely because the aliquots of bacteria that were used for these assays remained frozen at −80°C until use, and we were able to use the same bacterial dilution to produce the needed 200 colonies through the duration of the experiment. In a study in house martins (Delichon urbicum), nestlings reared after a period of high rainfall amounts had significantly larger T-cell mediated swelling responses to phytohemagglutinin (Christe et al., 2001), so it is possible that ability to mount responses to E. coli and corticosterone could be dependent upon energetic reserves and the resources available. However, body condition was similar between years, and while there was some variation in precipitation between the study years, this variation wasn’t very large (52 inches in 2016 and 2016 versus 58 inches in 2017) (Florida Climate Center, 2020). Thus, it is unlikely that rain-related resources drove the differences that we saw in plasma bactericidal capacity between years. More work needs to be done to determine how both bactericidal capacity and corticosterone responses vary by time and season, and how external variables may influence these measures.
In conclusion, American white ibis physiology may be affected by exposure to urban habitats, but in the opposite direction than was predicted. In addition, the innate bactericidal response to bacteria, the daily production of corticosterone, and the corticosterone responses to challenges are all dependent not only upon habitat, but also upon environmental variables such as weather challenges, food availability, social interactions, and pathogen challenges that differ between seasons and between years. More work needs to be done to test what environmental variables most potently influence immunity and adrenal physiology in white ibises, and how urbanization interacts with those variables to shape ibis acclimation, selection and ultimately survival in these novel environments.
Data Availability Statement
The raw data supporting the conclusions of this article will be made available by the authors, without undue reservation.
Ethics Statement
The animal study was reviewed and approved by the University of Georgia Institutional Animal Care and Use Committee AUP#A2016 11-019-Y3-A3.
Author Contributions
KN, SH, and MM designed the studies, assisted with data collection, and helped with writing and revision of the manuscript. CC was the chief graduate student in charge of executing the data collection and analyzing the data. TE, CW, and NK collected and analyzed data for the experiment. All authors contributed to the article and approved the submitted version.
Funding
This work was funded through the National Science Foundation EEID grant no. DEB-1518611.
Conflict of Interest
The authors declare that the research was conducted in the absence of any commercial or financial relationships that could be construed as a potential conflict of interest.
Acknowledgments
We thank S. Altizer, R. Hall, J. Heppenstall, and E. Lipp for their valuable input on the interpretation of these results.
References
Amdekar, M. S., Kakkar, A., and Thaker, M. (2018). Measures of health provide insights into the coping strategies of urban lizards. Front. Ecol. Evol. 6:128.
Audet, J. N., Ducatez, S., and Lefebvre, L. (2015). The town bird and the country bird: problem solving and immunocompetence vary with urbanization. Behav. Ecol. 27, 637–644. doi: 10.1093/beheco/arv201
Bailly, J., Scheifler, R., Belvalette, M., Garnier, S., Boissier, E., Clément-Demange, V. A., et al. (2016). Negative impact of urban habitat on immunity in the great tit Parus major. Oecologia 182, 1053–1062. doi: 10.1007/s00442-016-3730-2
Bancroft, G. T., Gawlik, D. E., and Rutchey, K. (2002). Distribution of Wading Birds Relative to Vegetation and Water Depths in the Northern Everglades of Florida. USA. Waterbirds 25, 265–277. doi: 10.1675/1524-4695(2002)025[0265:dowbrt]2.0.co;2
Becker, D. J., Streicker, D. G., Altizer, S., and Lafferty, K. (2015). Linking anthropogenic resources to wildlife–pathogen dynamics: a review and meta−analysis. Ecol. Lett. 18, 483–495. doi: 10.1111/ele.12428
Blom, A. M., Hallström, T., and Riesbeck, K. (2009). Complement evasion strategies of pathogens—acquisition of inhibitors and beyond. Mol. Immunol. 46, 2808–2817. doi: 10.1016/j.molimm.2009.04.025
Bonier, F. (2012). Review: Hormones in the city: Endocrine ecology of urban birds. Horm. Behav. 61, 763–772. doi: 10.1016/j.yhbeh.2012.03.016
Bonier, F., Martin, P. R., and Wingfield, J. C. (2007). Urban birds have broader environmental tolerance. Biol. Lett. 3, 670–673. doi: 10.1098/rsbl.2007.0349
Boyle, R. A., Dorn, N. J., and Cook, M. I. (2014). Importance of Crayfish Prey to Nesting White Ibis (Eudocimus albus). Waterbirds 37, 19–29. doi: 10.1675/063.037.0105
Bradley, C. A., and Altizer, S. (2007). Urbanization and the ecology of wildlife diseases. Trends Ecol. Evol. 22, 95–102. doi: 10.1016/j.tree.2006.11.001
Buehler, D. M., Piersma, T., and Tieleman, B. I. (2008). Captive and free-living red knots Calidris canutus exhibit differences in non-induced immunity that suggest different immune strategies in different environments. J. Avian. Biol. 39, 560–566. doi: 10.1111/j.0908-8857.2008.04408.x
Chimney, M. J., and Goforth, G. (2001). Environmental impacts to the Everglades ecosystem: a historical perspective and restoration strategies. Water Sci. Technol. 44, 93–100. doi: 10.2166/wst.2001.0814
Christe, P., De Lope, F., González, G., Saino, N., and Møller, A. P. (2001). The influence of environmental conditions on immune responses, morphology and recapture probability of nestling house martins (Delichon urbica). Oecologia 126, 333–338. doi: 10.1007/s004420000527
Crozier, G. E., and Gawlik, D. E. (2003). Wading Bird Nesting Effort as an Index to Wetland Ecosystem Integrity. Waterbirds 26, 303–324. doi: 10.1675/1524-4695(2003)026[0303:wbneaa]2.0.co;2
Curry, E. S. (2017). Public motivations for resource provisioning and physiological impacts on white ibis (Eudocimus albus) in urban South Florida. (PhD Dissertation) Athens, GA: University of Georgia.
Delgado-Valez, C. A., and French, K. (2012). Parasite–bird interactions in urban areas: Current evidence and emerging questions. Landscape Urb. Plan. 105, 5–14. doi: 10.1016/j.landurbplan.2011.12.019
Doody, L. M., Wilhelm, S. I., McKay, D. W., Walsh, C. J., and Storey, A. E. (2008). The effects of variable foraging conditions on common murre (Uria aalge) corticosterone concentrations and parental provisioning. Horm. Behav. 53, 140–148. doi: 10.1016/j.yhbeh.2007.09.009
Dorn, N. J., Cook, M. I., Herring, G., Boyle, R. A., Nelson, J., and Gawlik, D. E. (2011). Aquatic prey switching and urban foraging by the White Ibis Eudocimus albus are determined by wetland hydrological conditions. Ibis 153, 323–335. doi: 10.1111/j.1474-919x.2011.01101.x
Evans, K. L., Chamberlain, D. E., Hatchwell, B. J., Gregory, R. D., and Gaston, K. J. (2011). What makes an urban bird? Glob. Change Biol. 17, 32–44. doi: 10.1111/j.1365-2486.2010.02247.x
Florida Climate Center (2020). Available online at https://climatecenter.fsu.edu/products-services/data/statewide-averages/precipitation (accessed June 24, 2020).
Fokidis, H. B., Orchinik, M., and Deviche, P. (2009). Corticosterone and corticosteroid binding globulin in birds: Relation to urbanization in a desert city. Gen. Comp. Endocrinol. 160, 259–270. doi: 10.1016/j.ygcen.2008.12.005
Frederick, P. C., Gawlik, D. E., Ogden, J. C., Cook, M. I., and Lusk, M. (2009). The White Ibis and Wood Stork as indicators for restoration of the everglades ecosystem. Ecol. Indic. 9, S83–S95.
French, S. S., Fokidis, H. B., and Moore, M. C. (2008). Variation in stress and innate immunity in the tree lizard (Urosaurus ornatus) across an urban–rural gradient. J. Compar. Physiol. B 178, 997–1005. doi: 10.1007/s00360-008-0290-8
Gao, S., Sanchez, C., and Deviche, P. J. (2017). Corticosterone rapidly suppresses innate immune activity in the house sparrow (Passer domesticus). J. Exp. Biol. 220, 322–327. doi: 10.1242/jeb.144378
Garamszegi, L. Z., Erritzøe, J., and Møller, A. P. (2007). Feeding innovations and parasitism in birds. Biol. J. Linn. Soc. 90, 441–455. doi: 10.1111/j.1095-8312.2007.00733.x
Girard, J., Goldberg, T. L., and Hamer, G. L. (2011). Field investigation of innate immunity in passerine birds in suburban Chicago. Illinois, USA. J. Wildlife Dis. 47, 603–611. doi: 10.7589/0090-3558-47.3.603
Hasselquist, D., and Nilsson, J. Å (2012). Physiological mechanisms mediating costs of immune responses: what can we learn from studies of birds? Animal Behav. 83, 1303–1312. doi: 10.1016/j.anbehav.2012.03.025
Heath, J. A., and Frederick, P. C. (2003). Trapping White Ibises with rocket nets and mist nets in the Florida Everglades. J. Field Ornithol. 74, 187–192. doi: 10.1648/0273-8570-74.2.187
Hernandez, S. M., Welch, C. N., Peters, V. E., Lipp, E. K., Curry, S., Yabsley, M. J., et al. (2016). Urbanized White Ibises (Eudocimus albus) as Carriers of Salmonella enterica of Significance to Public Health and Wildlife. PLoS One 11:e0164402. doi: 10.1371/journal.pone.0164402
Herring, G., Cook, M. I., Gawlik, D. E., and Call, E. M. (2011). Food availability is expressed through physiological stress indicators in nestling white ibis: a food supplementation experiment. Funct. Ecol. 25, 682–690. doi: 10.1111/j.1365-2435.2010.01792.x
Iglesias-Carrasco, M., Martín, J., and Cabido, C. (2017). Urban habitats can affect body size and body condition but not immune response in amphibians. Urb. Ecosyst. 20, 1331–1338. doi: 10.1007/s11252-017-0685-y
Injaian, A. S., Francis, C. D., Ouyang, J. Q., Dominoni, D. M., Donald, J. W., Fuxjager, M. J., et al. (2020). Baseline and Stress-Induced Corticosterone Levels Across Birds and Reptiles Do Not Reflect Urbanication Levels. Conserv. Physiol. 8:1.
Kidd, A. D. (2018). Movement Ecology of the American White Ibis (Eudocimus albus) across an Urbanization Gradient. (Masters of Science Thesis) Athens, GA: University of Georgia.
Kitaysky, A. S., Wingfield, J. C., and Piatt, J. F. (1999). Dynamics of food availability, body condition and physiological stress response in breeding black−legged kittiwakes. Funct. Ecol. 13, 577–584. doi: 10.1046/j.1365-2435.1999.00352.x
Kleist, N. J., Guralnick, R. P., Cruz, A., Lowry, C. A., and Francis, C. D. (2018). Chronic anthropogenic noise disrupts glucocorticoid signaling and has multiple effects on fitness in an avian community. Proc. Natl. Acad. Sci. 115, E648–E657.
Kushlan, J. A. (1986). Responses of Wading Birds to Seasonally Fluctuating Water Levels: Strategies and Their Limits. Col. Waterbirds 9, 155–162. doi: 10.2307/1521208
Lepczyk, C. A., Mertig, A. G., and Liu, J. (2004). Landowners and cat predation across rural-to-urban landscapes. Biol. Conserv. 115, 191–201. doi: 10.1016/s0006-3207(03)00107-1
Lochmiller, R. L., Vestey, M. R., and Boren, J. C. (1993). Relationship between Protein Nutritional Status and Immunocompetence in Northern Bobwhite Chicks. Auk 110, 503–510. doi: 10.2307/4088414
Matson, K. D., Irene Tieleman, B., and Klasing, Kirk, C. (2006). Capture Stress and the Bactericidal Competence of Blood and Plasma in Five Species of Tropical Birds. Physiol. Biochem. Zool. 79, 556–564. doi: 10.1086/501057
McKinney, M. L. (2006). Urbanization as a major cause of biotic homogenization. Biol. Conserv. 127, 247–260. doi: 10.1016/j.biocon.2005.09.005
Merchant, M. E., Roche, C., Elsey, R. M., and Prudhomme, J. (2003). Antibacterial properties of.serum from the American alligator (Alligator mississippiensis). Compar. Biochem. Physiol. B Biochem. Mole. Biol. 136, 505–513.
Merrill, L., Angelier, F., O’Loghlen, A. L., Rothstein, S. I., and Wingfield, J. C. (2012). Sex-specific variation in brown-headed cowbird immunity following acute stress: a mechanistic approach. Oecologia 170, 25–38. doi: 10.1007/s00442-012-2281-4
Milner, J. M., Beest, F. M. V., Schmidt, K. T., Brook, R. K., and Storaas, T. (2014). To feed or not to feed? Evidence of the intended and unintended effects of feeding wild ungulates. J. Wildlife Manage. 78, 1322–1334. doi: 10.1002/jwmg.798
Møller, A. P. (2009). Successful city dwellers: a comparative study of the ecological characteristics of urban birds in the Western Palearctic. Oecologia 159, 849–858. doi: 10.1007/s00442-008-1259-8
Müller, J. C., Partecke, J., Hatchwell, B. J., Gaston, K. J., and Evans, K. L. (2013). Candidate gene polymorphisms for behavioural adaptations during urbanization in blackbirds. Mole. Ecol. 22, 3629–3637. doi: 10.1111/mec.12288
Murray, M. H., Becker, D. J., Hall, R. J., and Hernandez, S. M. (2016). Wildlife health and supplemental feeding: A review and management recommendations. Biol. Conserv. 204, 163–174. doi: 10.1016/j.biocon.2016.10.034
Murray, M. H., Kidd, A. D., Curry, S. E., Hepinstall-Cymerman, J., Yabsley, M. J., Adams, H. C., et al. (2018). From wetland specialist to hand-fed generalist: shifts in diet and condition with provisioning for a recently urbanized wading bird. Philos. Transac. R. Soc. B Biol. Sci. 373:20170100. doi: 10.1098/rstb.2017.0100
Padgett, D. A., and Glaser, R. (2003). How stress influences the immune response. Trends in Immunol. 24, 444–448. doi: 10.1016/s1471-4906(03)00173-x
Phalen, D. N., Drew, M. L., Simpson, B., Roset, K., Dubose, K., and Mora, M. (2010). Salmonella enterica subsp. enterica in cattle egret (Bubulcus ibis) chicks from central Texas: prevalence, serotypes, pathogenicity, and epizootic potential. J. Wildlife Dis. 46, 379–389. doi: 10.7589/0090-3558-46.2.379
Pusch, E. A., Bentz, A. B., Becker, D. J., and Navara, K. J. (2018). Behavioral phenotype predicts physiological responses to chronic stress in proactive and reactive birds. Gen. Compar. Endocrinol. 255, 71–77. doi: 10.1016/j.ygcen.2017.10.008
Romero, L. M. (2002). Seasonal changes in plasma glucocorticoid concentrations in free-living vertebrates. Gen. Compar. Endocrinol. 128, 1–24. doi: 10.1016/s0016-6480(02)00064-3
Romero, L. M., and Reed, J. M. (2005). Collecting baseline corticosterone samples in the field: is under 3 min good enough? Compar. Biochem. Physiol. A Mole. Int. Physiol. 140, 73–79. doi: 10.1016/j.cbpb.2004.11.004
Romero, L. M., and Romero, R. C. (2002). Corticosterone responses in wild birds: The importance of rapid initial sampling. Condor 104, 129–135. doi: 10.1650/0010-5422(2002)104[0129:criwbt]2.0.co;2
Russ, A., Reitemeier, S., Weissmann, A., Gottschalk, J., Einspanier, A., and Klenke, R. (2015). Seasonal and urban effects on the endocrinology of a wild passerine. Ecol. Evol. 5, 5698–5710. doi: 10.1002/ece3.1820
Sapolsky, R. M., Romero, L. M., and Munck, A. U. (2000). How do glucocorticoids influence stress responses? Integrating permissive, suppressive, stimulatory, and preparative actions. End. Rev. 21, 55–89. doi: 10.1210/er.21.1.55
Schmitt, C., Garant, D., Bélisle, M., and Pelletier, F. (2017). Agricultural intensification is linked to constitutive innate immune function in a wild bird population. Physiol. Biochem. Zool. 90, 201–209. doi: 10.1086/689679
Schoech, S. J., Bowman, R., and Reynolds, S. J. (2004). Food supplementation and possible mechanisms underlying early breeding in the Florida Scrub-Jay (Aphelocoma coerulescens). Horm. Behav. 46, 565–573. doi: 10.1016/j.yhbeh.2004.06.005
Stambaugh, T., Houdek, B. J., Lombardo, M. P., Thorpe, P. A., and Caldwell Hahn, D. (2011). Innate immune response development in nestling tree swallows. Wilson J. Ornithol. 123, 779–787. doi: 10.1676/10-197.1
Tieleman, B. I., Croese, E., Helm, B., and Versteegh, M. A. (2010). Repeatability and individual correlates of microbicidal capacity of bird blood. Compar. Biochem. Physiol. A Mole. Int. Physiol. 156, 537–540. doi: 10.1016/j.cbpa.2010.04.011
Vincze, E., Papp, S., Preiszner, B., Seress, G., Bokony, V., and Liker, A. (2016). Habituation to human disturbance is faster in urban than rural house sparrows. Behav. Ecol. 27, 1304–1313. doi: 10.1093/beheco/arw047
Zhang, S., Lei, F., Liu, S., Li, D., Chen, C., and Wang, P. (2011). Variation in baseline corticosterone levels of Tree Sparrow (Passer montanus) populations along an urban gradient in Beijing, China. J. Ornithol. 152, 801–806. doi: 10.1007/s10336-011-0663-8
Keywords: urbanization, corticosterone, Salmonella, stress, microbicidal, immune, glucocorticoid, bird
Citation: Cummings CR, Khan NY, Murray MM, Ellison T, Welch CN, Hernandez SM and Navara KJ (2020) Foraging in Urban Environments Increases Bactericidal Capacity in Plasma and Decreases Corticosterone Concentrations in White Ibises. Front. Ecol. Evol. 8:575980. doi: 10.3389/fevo.2020.575980
Received: 24 June 2020; Accepted: 20 November 2020;
Published: 14 December 2020.
Edited by:
Darryl Jones, Griffith University, AustraliaReviewed by:
Travis Eli Wilcoxen, Millikin University, United StatesKimberly Lynn Maute, University of Wollongong, Australia
Copyright © 2020 Cummings, Khan, Murray, Ellison, Welch, Hernandez and Navara. This is an open-access article distributed under the terms of the Creative Commons Attribution License (CC BY). The use, distribution or reproduction in other forums is permitted, provided the original author(s) and the copyright owner(s) are credited and that the original publication in this journal is cited, in accordance with accepted academic practice. No use, distribution or reproduction is permitted which does not comply with these terms.
*Correspondence: Kristen J. Navara, a25hdmFyYUB1Z2EuZWR1