- 1State Key Laboratory of Vegetation and Environmental Change, Institute of Botany, Chinese Academy of Sciences, Beijing, China
- 2College of Resources and Environment, University of Chinese Academy of Sciences, Beijing, China
Plant performance is commonly temperature-dependent so that this performance could vary with climate warming. Seeds are among the most important propagules of plants, and seed traits strongly influence plant invasion success. Therefore, understanding seed traits under climate warming is useful for predicting invasion risks. To this end, we conducted a warming experiment with an infrared radiator and examined the effects of 5 years warming (approximately 2°C above ambient) on the seed quality and subsequent germination of Solidago canadensis from North America, where it is native (24 native populations), and from China, where it is invasive (29 invasive populations). Temperature regimes (i.e., ambient vs. warming) interacted with population sources (i.e., native vs. invasive) to significantly influence seed germination, but not thousand-seed mass. Warming significantly advanced the seed germination timing of native S. canadensis populations and increased their seed germination rate; warming did not influence the germination timing but decreased the germination rate of invasive S. canadensis populations. Across two temperature regimes combined, 24 native S. canadensis populations had smaller seeds, later germination timing, and lower germination rate than 29 invasive S. canadensis populations. These findings suggest that climate warming could facilitate the seed germination of native but not invasive populations. Our data also highlight that invasive populations might be more successful than native populations due to better seed quality and faster and higher seed germination.
Introduction
Plant invasions are complex processes (Theoharides and Dukes, 2007; Catford et al., 2009; Lockwood et al., 2013) so that dozens of hypotheses (e.g., ideal weed and novel weapons) have been put forth to explain invasion success (Catford et al., 2009; Jeschke, 2014). Successful invasion needs sufficient propagules, which can reflect the idiosyncratic nature of invasions (Lockwood et al., 2005). To date, two invasion hypotheses (i.e., propagule pressure and global competition) primarily based on propagules have been proposed (Catford et al., 2009). Seeds, stolons, and rhizomes are the most important propagules of plants (Harper, 2010) and thus play an extremely crucial role in the invasion processes (Lockwood et al., 2005). Overall, the relative importance of seeds, stolons, and rhizomes varies among different plant invaders (Wang et al., 2016) and/or with invasion stages within the same plant invader (Theoharides and Dukes, 2007). Actually, clonal plant invaders account for a high proportion of plant invaders, particularly in grasslands (Wang et al., 2016) and the mortality of established genets is a rare event and clonal growth plays a crucial role in spatial spread after invader establishment (Szymura and Szymura, 2016). Additionally, the relative role of different propagules depends on manners through which a given taxon is introduced from its native range into new ranges.
Here, we focused on seeds for the following reasons. First, seeds are among the fundamental progagules of higher plants and commonly determine the success of their early establishment (Harper, 2010). Second, the seedlings of plant invaders usually occupy disturbed sites and seed dispersal is important for their long-distance expansion (Lockwood et al., 2013). Of seed traits, seed quality and germination, to a larger extent, determine the recruitment of seedlings and dynamics of plant populations (Fenner and Thompson, 2006; Harper, 2010). Similarly, for plant invaders, seed quality and germination largely influence their success, and ultimately shape their capacity to exclude native plants (Valliere et al., 2019).
An abundant literature shows that seed quality and germination are unavoidably influenced by a wide range of ecological factors (Harper and Benton, 1966; Baskin and Baskin, 1998; Guillemin and Chauvel, 2011; Valliere et al., 2019; Wang and He, 2019). One of these, air temperature has long been recognized as an important factor influencing seed quality and germination (Baker, 1965; Baskin and Baskin, 1998; Fenner and Thompson, 2006). Climate warming is occurring rapidly and strongly affects plants (IPCC, 2015). This is because plant performance is highly temperature-dependent (Crawley, 1997). Since climate warming can influence plant invasions (Bradley et al., 2010), this has particular relevance to seed quality and seed germination (Aragn-Gastlum et al., 2018). For example, recent studies have shown that climate warming may strongly influence seed quality and seed germination (Aragn-Gastlum et al., 2018; Li et al., 2018) and plant mortality and phenology during the invasion process (Peng et al., 2018; He and He, 2020). Plant populations from native and non-native ranges perform differentially, including reproductive output, seedling recruitment, individual density, and competitive ability (Lockwood et al., 2013). Thus, studying climate warming effects on the seed traits of native and invasive populations could be useful for understanding invasion success in the context of global warming from a biogeographic perspective.
Here, we concentrated on one scientific question: how climate warming influences the seed quality and subsequent germination of native and invasive Solidago canadensis populations. Solidago canadensis was selected as a focal species, because it is among seriously noxious invaders in China (Dong et al., 2015) and sensitive to climate warming (Peng et al., 2018, 2019). The central hypothesis was that climate warming may influence the seed quality and subsequent germination of native S. canadensis populations stronger than those of invasive counterparts, because native populations of Centaurea maculosa and S. canadensis are more sensitive to climate warming than invasive populations (He et al., 2012; Peng et al., 2019). The secondary hypothesis was that invasive S. canadensis populations may have larger seeds and higher germination than native populations, because the former can produce more biomass than the latter (He et al., 2012; Peng et al., 2019). If this is true, it could help to partially explain the differences in seedling recruitment and individual density between native and invasive populations. To test both hypotheses, we collected seeds from our long-term climate warming experiment to determine thousand-seed mass, and then performed a germination experiment that compared the legacy effects of climate warming on the seed germination of S. canadensis from its native range of North America and invaded range of China.
Materials and Methods
Experimental Design
Solidago canadensis, native to North America, is a worldwide invasive forb, and has now invaded Europe, Asia, Australia, and New Zealand (Weber, 2001; Abhilasha et al., 2008). Solidago canadensis was introduced into Europe in the seventeenth century (Weber, 2001). This invader was introduced into China in 1913, has occupied several provinces in southeastern China, and is expanding rapidly (Dong et al., 2006, 2015). Overall, S. canadensis usually appears along roadsides, abandoned agricultural lands, and other disturbed habitats (Dong et al., 2015). In its native range (i.e., North America), the inflorescence of S. canadensis is evident in late July or early August (Werner et al., 1980). However, in its invaded range (i.e., China), it does not start flowering until September (Peng et al., 2018, 2019). Solidago canadensis is a perennial forb with large clonal colonies, can grow up to 4 m, and its flowers are self-sterile and seeds are wind-dispersed (Abhilasha et al., 2008; Dong et al., 2015). In our experiment, the seeds of S. canadensis were field-collected from multiple populations across multiple sites (Table 1), regardless of native or invaded ranges. Specifically, we located five sampling sites per range and collected seeds from six mature individuals per site spaced at least 10 m apart. The seeds from a particular individual were put into an envelope. Meanwhile, we also collected the seeds of common native plants from the both ranges of S. canadensis. These collections allowed us to create synthetic plant communities.
Our long-term experiment, which encompassed (1) climate warming, (2) nitrogen deposition, (3) community types, (4) species origin, and (5) population sources, began in June 2012. At the beginning of the experiment, we set up 256 mesocosms including dozens of plant assemblages from seed (i.e., monocultures of S. canadensis and mixtures consisting of S. canadensis and four native plant species from North America or China). The conditions of mesocosms and their photographs have been described previously (Peng et al., 2018). As described above, each mesocosm was either a monoculture or a mixture. Accordingly, there were about 20 S. canadensis populations per manipulation initially. The long-term experiment has been described previously (Peng et al., 2018, 2019). Briefly, our long-term experiment was a factorial design and included five factors (see above), each with two levels. All the warming mesocosms were continuously heated with a MSR-2420 infrared radiator (Kalglo Electronics, Bethlehem, PA, United States) that was suspended 1.5–2.0 m above the soil surface, roughly increasing air temperatures by 2°C (Peng et al., 2018, 2019). This increase in air temperature (i.e., approximately 2°C) was chosen according to the range (1.5–3.0°C) projected by IPCC (2015) and the future goal by the Paris Agreement. For each unwarmed mesocosm, one “dummy” heater with the same shape and size as the infrared radiator was hanged to simulate the shading effect of the infrared radiator. Non-stop warming has been lasting since June 2012. The long-term temperature changes under the heated and unheated conditions have been reported in our recent paper (Peng et al., 2018).
To address the effects of climate warming and population sources on seed quality and seed germination, we collected the seeds from those mesocosms consisting of different climates (i.e., ambient vs. warming) and population sources (i.e., native vs. invaded) in November 2017. In this seed experiment, due to the lack of seeds in some mesocosms the number of available populations was 12, 12, 15, and 14 for native populations grown under the ambient, native populations grown under warming, invasive populations grown under the ambient, invasive populations grown under warming, respectively. Accordingly, there were 53 populations (12 + 12 + 15 + 14) in total. The populations from North America were referred to as native populations and the populations from China were referred to as invasive populations. All the seeds collected in November 2017 were stored in a refrigerator (−4°C).
We conducted the seed germination experiment in April 2018, roughly corresponding to the beginning of a growing season. Prior to germination experiment, we first measured seed mass. To determine seed mass, we randomly selected 100 seeds from each population and weighed their air-dried mass, and such a measurement was replicated five times. Thousand-seed mass was calculated as: hundred-seed mass × 10. To explore the effects of experimental manipulations on subsequent seed germination, we performed a germination experiment in a naturally lit greenhouse at the Chengdu University of Technology (a naïve environment), in which the air temperature and relative humidity ranged 15–25°C and 60–80%. Specifically, seeds from each population were placed in petri dishes filled with filter paper (i.e., 30 seeds per petri dish). Each population had five replicates of 30 seeds, totaling 265 petri dishes (53 populations × 5 replicates per population). All petri dishes were watered as needed to maintain adequate moisture. Seed germination, defined as the radicle emergence, was checked daily and then germinated seeds with radicle were removed from petri dishes.
Data Analyses
We selected thousand-seed mass as an indicator of seed quality. To describe seed germination, we determined the following metrics as described in our recent study (Wang and He, 2019): (1) the first germinating time (a proxy of germinating onset), (2) half germinating time (a proxy of germinating speed), and (3) germination rate (a proxy of germinating potential). The first germinating time was defined as the number of days when the first germination occurred. The half germinating time was defined as the number of days when 50% of the total number of germinated seeds occurred. The germination rate was calculated as: the number of germinated seeds/the initial number of seeds × 100%.
The normality of data and homogeneity of variance were tested using the Shapiro-Wilk test and Bartlett’s test, respectively. We fitted a linear mixed effect model using the NLME package with the “lme” function (Pinheiro et al., 2017) to examine the effects of temperature regimes (i.e., ambient vs. warming) and population sources (i.e., native vs. invasive) on thousand-seed mass, first germinating time, half germinating time, and germination rate, in which plant populations were treated as a random term. All the analyses were performed using R v.4.0.2 (R Core Team, 2020).
Results
Across two temperature regimes, native populations produced smaller seeds [0.0411 ± 0.0015 g, (means ± 1 SE)] than invasive populations (0.0491 ± 0.0010 g) (Table 2: source effect; Figure 1). Across two population sources, seed mass substantially decreased from 0.0496 ± 0.0011 g under the ambient to 0.0412 ± 0.0014 g under the warming (Table 2: temperature effect; Figure 1). Population sources and temperature regimes had no interaction on seed mass (Table 2: source × temperature effect; Figure 1). Thus, warming decreased seed mass independent of population sources (P = 0.336; Figure 1).
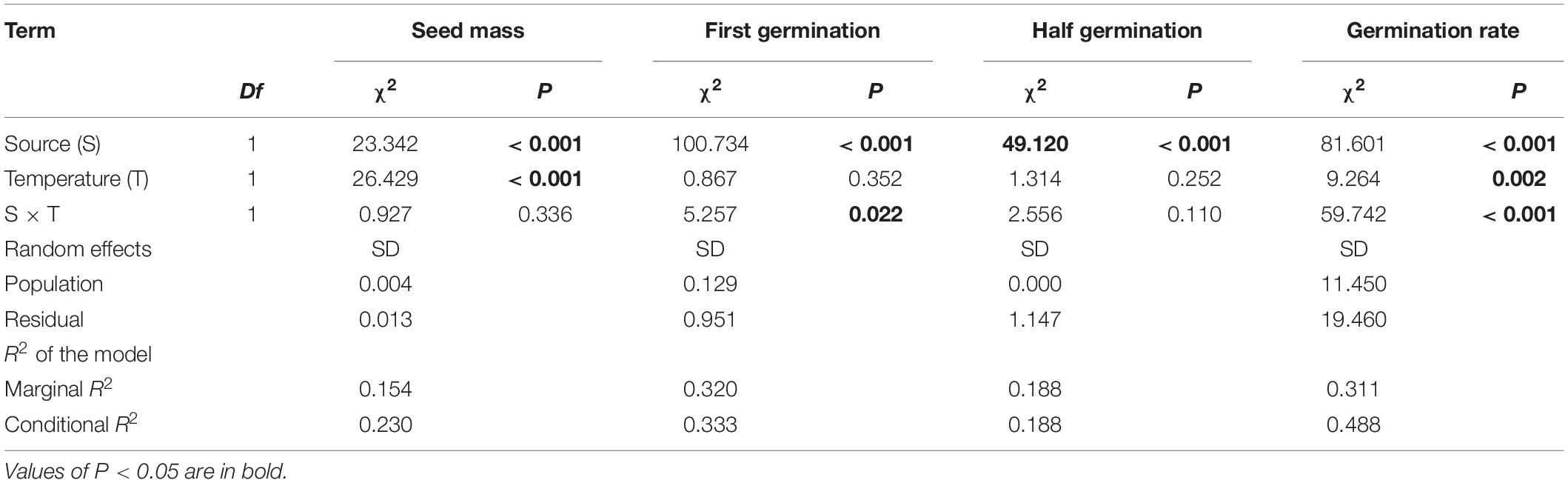
Table 2. Effects of population sources (source) and temperature regimes (temperature), both of which were treated as fixed factors, on the thousand-seed mass (seed mass), first germinating time (first germination), half germinating time (half germination), and germination rate.
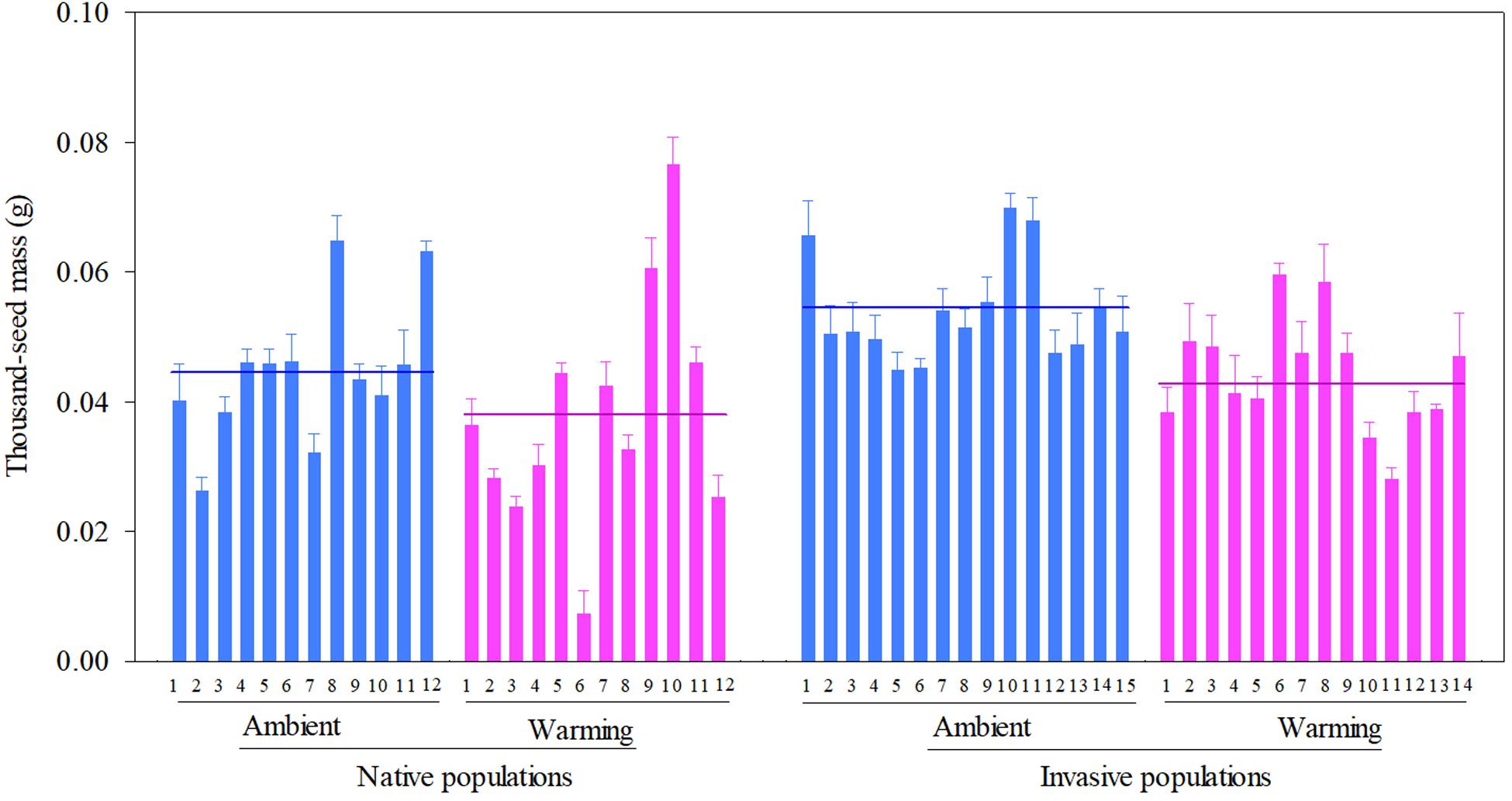
Figure 1. Effects of population sources and temperature regimes on the thousand-seed mass of Solidago canadensis populations. Data are means + 1 SE (n = 5). The line indicates the average value of the thousand-seed mass for populations grown under a given temperature regime. Detailed statistical analyses are presented in Table 2.
Across two temperature regimes, the first germinating time was later in native populations (2.54 ± 0.15 days) than in invasive populations (1.24 ± 0.05 days) (Table 2: source effect; Figure 2). Temperature regimes did not influence the first germination across two population sources (Table 2: temperature effect; Figure 2). Importantly, population sources interacted with temperature regimes to influence the first germinating time (Table 2: source × temperature effect): warming advanced the first germinating time of native populations from 2.82 ± 0.31 days under the ambient to 2.33 ± 0.09 days under the warming, but did not influence this time of invasive populations (P = 0.022; Figure 2).
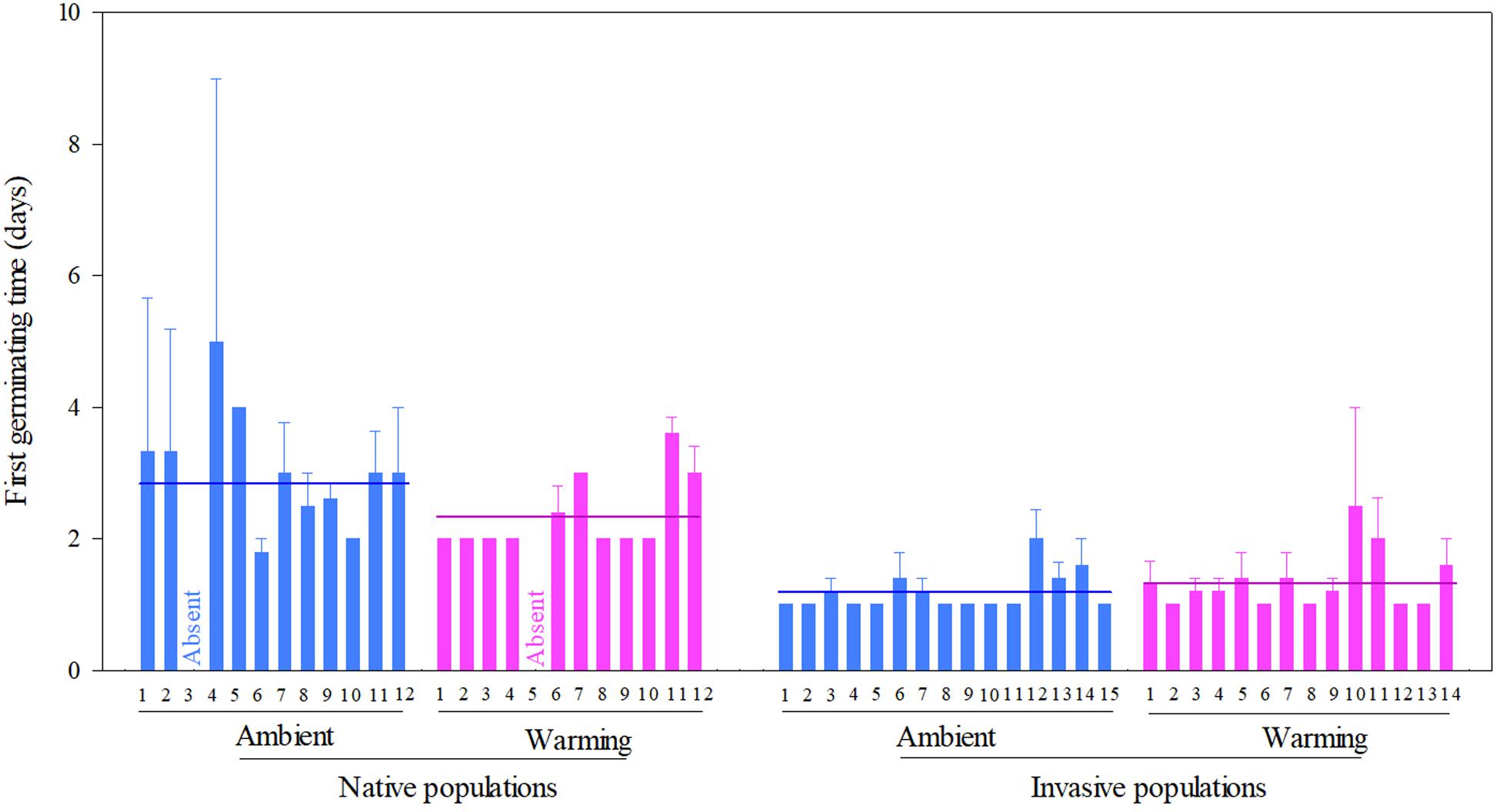
Figure 2. Effects of population sources and temperature regimes on the first germinating time of Solidago canadensis populations. Data are means + 1 SE (n = 5). The line indicates the average value of the first germinating time for populations grown under a given temperature regime. Detailed statistical analyses are presented in Table 2.
Across two temperature regimes, the half germinating time was later in native populations (2.81 ± 0.15 days) than in invasive populations (1.71 ± 0.08 days) (Table 2: source effect; Figure 3). Temperature regimes did not influence the half germination across two population sources (Table 2: temperature effect; Figure 3). Population sources and temperature regimes exhibited a weak interaction on the half germination, although this role was not significant statistically (Table 2: source × temperature effect). For example, warming slightly decreased the half germinating time of native populations but failed to influence this time of invasive populations (P = 0.110; Figure 3).
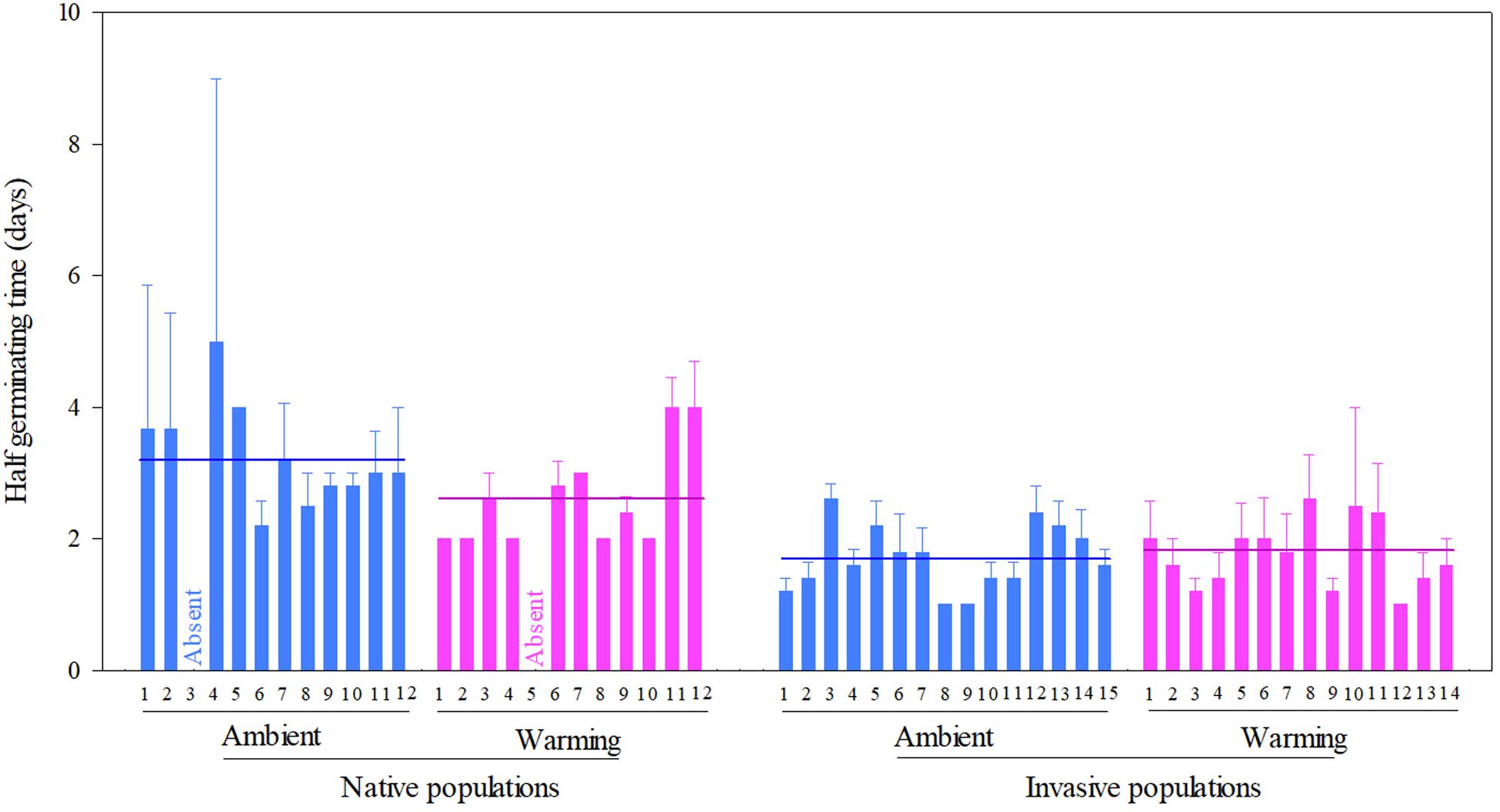
Figure 3. Effects of population sources and temperature regimes on the half germinating time of Solidago canadensis populations. Data are means + 1 SE (n = 5). The line indicates the average value of the half germinating time for populations grown under a given temperature regime. Detailed statistical analyses are presented in Table 2.
Across two temperature regimes, the germination rate was lower in native populations (21.3 ± 2.3%) than in invasive populations (43.3 ± 2.0%) (Table 2: source effect; Figure 4). The germination rate decreased from 37.1 ± 2.4% under the ambient to 29.5 ± 2.2% under the warming (Table 2: temperature effect; Figure 4). More importantly, population sources and temperature regimes jointly influenced germination rate (Table 2: source × temperature effect): warming increased the germination rate of native populations from 14.89 ± 2.88% under the ambient to 27.78 ± 3.54% under the warming, but decreased this rate of invasive populations from 54.89 ± 2.13% under the ambient to 30.91 ± 2.66% under the warming (P < 0.001; Figure 4).
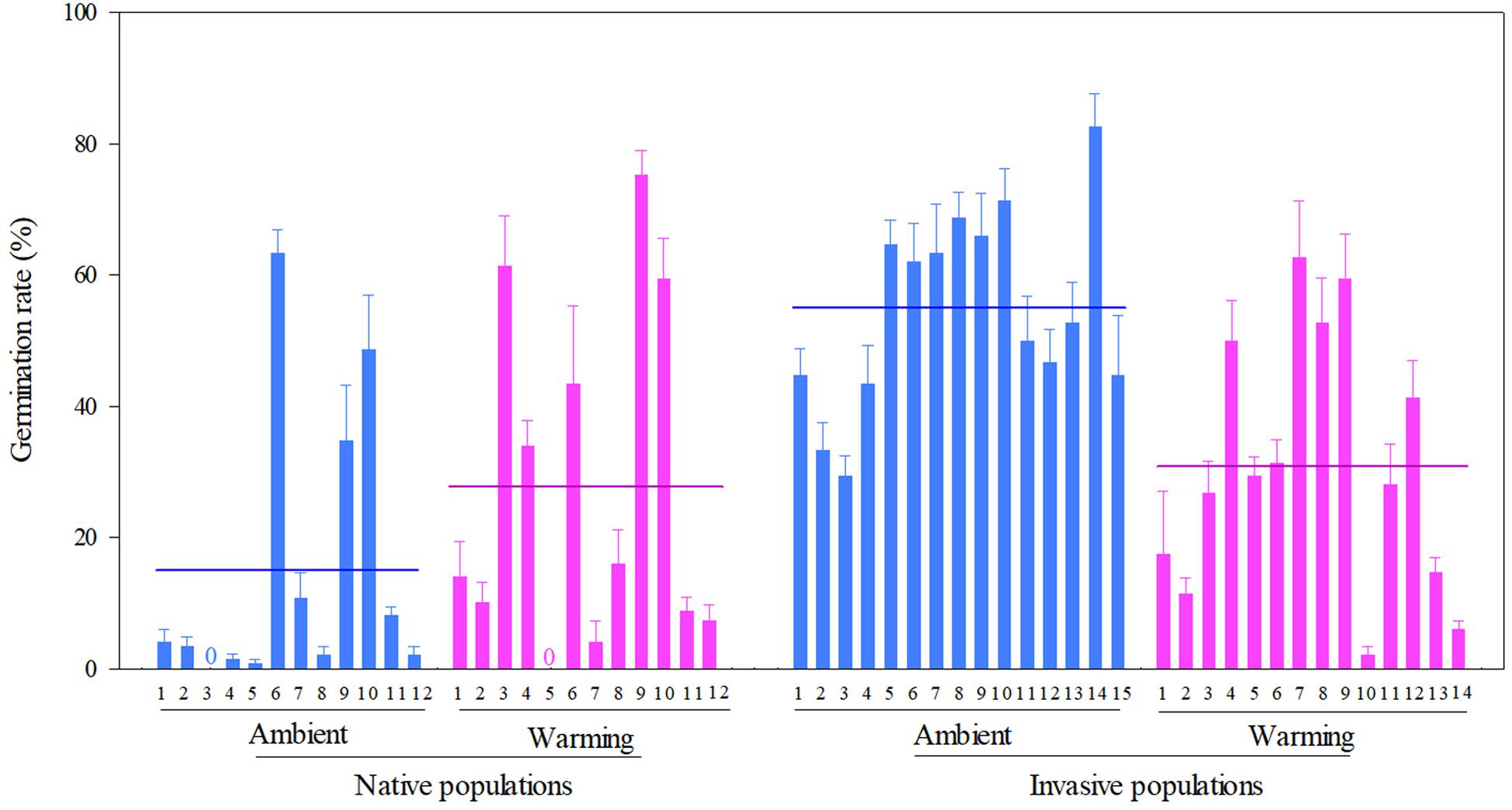
Figure 4. Effects of population sources and temperature regimes on the germination rate of Solidago canadensis populations. Data are means + 1 SE (n = 5). The line indicates the average value of the germination rate for populations grown under a given temperature regime. Detailed statistical analyses are presented in Table 2.
Discussion
The first key finding of our study was that 5-year continued warming (increasing air temperatures by approximately 2°C), as an environmental cue, yielded more positive effects on the seed germination of S. canadensis from its native but not introduced range. In our recent study, Peng et al. (2019) found that native S. canadensis populations were more sensitive to climate warming and nitrogen deposition than invasive populations in terms of leaf traits, ramet growth, and phenological sequences. It is noteworthy that warming effects were not directional: warming decreased the seed mass of native populations but enhanced their seed germination. Similar phenomena have been detected in our previous studies. For example, the competitive ability of C. maculosa populations from an invaded range was greater than that of C. maculosa populations from a native range under the ambient, but this competitive ability followed the opposite direction under the warming (He et al., 2012). Thus, our finding partly supports the hypothesis of climate-of-origin (Drake et al., 2015) and our central hypothesis.
The pattern that native S. canadensis populations benefited more from climate warming than invasive S. canadensis populations might be linked to climate-of-provenance (Drake et al., 2015). For example, the growth of two widely distributed Eucalyptus species originating in a cooler climate (i.e., temperate climate) was enhanced due to climate warming through physiological acclimation, whereas the opposite was the case for Eucalyptus species originating in a warmer climate (i.e., tropical climate) (Drake et al., 2015). Similarly, S. canadensis is native to temperate North America (Werner et al., 1980), where air temperature is lower compared to invaded subtropical areas (Table 1). Thus, the same rising temperature may yield contrasting thermal consequences for plant populations and seeds in temperate and subtropical climates (Fenner and Thompson, 2006). On the other hand, the experience of populations might also matter in response to climate warming. Evidence is accumulating that the experience of plants strongly influences their responses to the surrounding environments (Kohl and Dearing, 2012; Sun and He, 2018). Since native and invasive S. canadensis populations grew in different climatic conditions (Table 1), they had contrasting experience, thereby influencing their sensitivity to climate warming.
Our findings may add significantly to plant invasions under climate warming. First, climate warming might differentially influence the invasion risk of native vs. invasive populations, in line with our previous study (He et al., 2012). Specificlly, climate warming could increase the invasion risk of native populations via earlier germination and higher germination rates, but decrease the risk of invasive populations through suppressing their seed germination rates. Second, our findings, from a propagule perspective, provide evidence for the hypothesis that climate warming could facilitate plant invasion (Bradley et al., 2010). For example, climate warming might increase seed numbers, due to seed size/number tradeoffs (Baskin and Baskin, 1998), ultimately enhancing seedling recruitments.
A second key finding of our study was that invasive S. canadensis populations had larger seeds and faster and higher seed germination than native counterparts, thereby supporting our second hypothesis. This biogeographic difference could be attributable partly to rapid evolution (Maron et al., 2004; Buswell et al., 2011) and lagging adaptation (Wilczek et al., 2014). The native range of S. canadensis is relatively unfavorable, whereas its invaded range is relatively favorable (Table 1). Thus, such a difference in site favorability might drive the rapid evolution of invasive S. canadensis populations in southern China. This evolution can be supported by recent studies by Peng et al. (2018, 2019), who found that invasive S. canadensis populations grew faster than native S. canadensis populations. This growth difference could favor invasive populations to produce larger seeds. Since seed mass is commonly correlated to germination timing and rate (Wang and He, 2019), larger seeds tend to take shorter to germinate and have a higher germination rate. On the other hand, native populations might exhibit lagging adaptation under a warming climate (Wilczek et al., 2014), because non-native subtropical areas might be relatively naïve for them.
Seed quality and seed germination are among the most important characteristics of plant sexual propagules (Harper, 2010), and thus contribute to invasion success (Baker, 1965; Alpert et al., 2000; Richardson and Pyšek, 2006; Lockwood et al., 2013). The findings from this experiment and our previous experiments (Peng et al., 2018, 2019) suggest that invasive populations may be more successful than native populations due to rapid evolution. Accordingly, invasive populations seem to have greater risks than native populations. Additionally, our findings might help us to understand why S. canadensis is mainly restricted to local patches in its native range (Werner et al., 1980) and widespread in invaded southern China (Dong et al., 2015).
In conclusion, we suggest that climate warming as an environmental cue may have stronger effects on seed germination in plant populations from native but not invaded ranges. In other words, native populations might be more sensitive to climate warming than invasive populations in terms of germination. Across two climates combined, invasive populations may be more successful than native populations, as the former had better seed quality and faster and higher seed germination. Our results indicate that: network studies on seed traits across different climatic zones are imperative for understanding plant invasions under climate warming, and future experiments including the seeds of native and invasive plants will be useful for further assessing invasion risks under global change.
Data Availability Statement
The datasets presented in this article are not readily available because the work is part of our project. Requests to access the datasets should be directed to W-MH, V2VpbWluZ2hlQGliY2FzLmFjLmNu.
Author Contributions
X-HZ and W-MH conceived and designed the study, analyzed the data, and wrote the manuscript. X-HZ performed the experiment. Both authors contributed to the article and approved the submitted version.
Funding
This work was supported by the National Natural Science Foundation of China (31971552).
Conflict of Interest
The authors declare that the research was conducted in the absence of any commercial or financial relationships that could be construed as a potential conflict of interest.
References
Abhilasha, D., Quintana, N., Vivanco, J., and Joshi, J. (2008). Do allelopathic compounds in invasive Solidago canadnesis s.l. restrain the native European flora? J. Ecol. 96, 993–1001. doi: 10.1111/j.1365-2745.2008.01413.x
Alpert, P., Bone, E., and Holzapfel, C. (2000). Invasiveness, invasibility and the role of environmental stress in the spread of non-native plants. Perspect. Plant Ecol. 3, 52–66. doi: 10.1078/1433-8319-00004
Aragn-Gastlum, J., Flores, J., Jurado, E., Ramrez-Tobas, H. M., Robles-Daz, E., Rodas-Ortiz, J. P., et al. (2018). Potential impact of global warming on seed bank, dormancy and germination of three succulent species from the Chihuahuan Desert. Seed Sci. Res. 28, 312–318. doi: 10.1017/s0960258518000302
Baker, H. G. (1965). “Characteristics and modes of origin of weeds,” in The Genetics of Colonizing Species, eds H. G. Baker and G. L. Stebbins (San Diego: Academic Press), 147–168.
Baskin, C. C., and Baskin, J. M. (1998). Seeds: Ecology, Biogeography, and Evolution of Dormancy and Germination. San Diego: Academic Press.
Bradley, B. A., Blumenthal, D. M., Wilcove, D. S., and Ziska, L. H. (2010). Predicting plant invasions in an era of global change. Trends Ecol. Evol. 25, 310–318. doi: 10.1016/j.tree.2009.12.003
Buswell, J. M., Moles, A. T., and Hartley, S. (2011). Is rapid evolution common in introduced plant species? J. Ecol. 99, 214–224. doi: 10.1111/j.1365-2745.2010.01759.x
Catford, J. A., Jansson, R., and Nilsson, C. (2009). Reducing redundancy in invasion ecology by integrating hypotheses into a single theoretical framework. Divers. Distrib. 15, 22–40. doi: 10.1111/j.1472-4642.2008.00521.x
Dong, M., Lu, J., Zhang, W., Chen, J., and Li, B. (2006). Canada goldenrod (Solidago canadensis): an invasive alien weed rapidly spreading in China. Acta Phytotaxon. Sin. 44, 72–85. doi: 10.1360/aps050068
Dong, L. J., Yu, H. W., and He, W. M. (2015). What determines positive, neutral, and negative impacts of Solidago canadensis invasion on native plant species richness? Sci. Rep. 5:16804. doi: 10.1038/srep16804
Drake, J. E., Aspinwall, M. J., Pfautsch, S., Rymer, P. D., Reich, P. B., Smith, R. A., et al. (2015). The capacity to cope with climate warming declines from temperate to tropic latitudes in two widely distributed Eucalyptus species. Glob. Change Biol. 21, 459–472. doi: 10.1111/gcb.12729
Guillemin, J. P., and Chauvel, B. (2011). Effects of the seed weight and burial depth on the seed behavior of common ragweed (Ambrosia artemisiifolia). Weed Biol. Manag. 11, 217–223. doi: 10.1111/j.1445-6664.2011.00423.x
Harper, J. L., and Benton, R. A. (1966). The behaviour of seeds in soil: The germination of seeds on the surface of a water supplying substrate. J. Ecol. 54, 151–166. doi: 10.2307/2257664
He, Z. S., and He, W. M. (2020). Asymmetric climate warming does not benefit plant invaders more than natives. Sci. Total Environ. 742:140624. doi: 10.1016/j.scitotenv.2020.140624
He, W. M., Li, J. J., and Peng, P. H. (2012). Simulated warming differentially affects the growth and competitive ability of Centaurea maculosa populations from home and introduced ranges. PLoS One 7:e31170. doi: 10.1371/journal.pone.0031170
Jeschke, J. M. (2014). General hypotheses in invasion ecology. Divers. Distrib. 20, 1229–1234. doi: 10.1111/ddi.12258
Kohl, K., and Dearing, M. D. (2012). Experience matters: prior exposure to plant toxins enhances diversity of gut microbes in herbivores. Ecol. Lett. 15, 1008–1015. doi: 10.1111/j.1461-0248.2012.01822.x
Li, J., Ren, L., Bai, Y., Lecain, D., Blumenthal, D., and Morgan, J. (2018). Seed traits and germination of native grasses and invasive forbs are largely insensitive to parental temperature and CO2 concentration. Seed Sci. Res. 28, 303–311. doi: 10.1017/s0960258518000314
Lockwood, J. L., Cassey, P., and Blackburn, T. (2005). The role of propagule pressure in explaining species invasions. Trends Ecol. Evol. 20, 223–228. doi: 10.1016/j.tree.2005.02.004
Lockwood, J. L., Hoopes, M. F., and Marchetti, M. P. (2013). Invasion Ecology. New York, NY: John Wiley and Sons.
Maron, J. L., Vilà, M., Bommarco, R., Elmendorf, S., and Beardsley, P. (2004). Rapid evolution of an invasive plant. Ecol. Monogr. 74, 261–280. doi: 10.1890/03-4027
Peng, Y., Yang, J. X., Zhou, X. H., Peng, P. H., Li, J. J., and He, W. M. (2018). Warming delays the phonological sequences of an autumn-flowering invader. Ecol. Evol. 8, 6299–6307. doi: 10.1002/ece3.4177
Peng, Y., Yang, J. X., Zhou, X. H., Peng, P. H., Li, J. J., Zhang, S. M., et al. (2019). An invasive population of Solidago canadensis is less sensitive to warming and nitrogen-addition than its native population in an invaded range. Biol. Invasions 21, 151–162. doi: 10.1007/s10530-018-1812-2
Pinheiro, J., Bates, D., DebRoy, S., Sarkar, D., and R Core Team. (2017). nlme: Linear and Nonlinear Mixed Effects Models. R package version 3. 1–131.
R Core Team. (2020). R: A language and environment for statistical computing. Vienna: R Foundation for Statistical Computing.
Richardson, D. M., and Pyšek, P. (2006). Plant invasions: merging the concepts of species invasiveness and community invasibility. Progr. Phys. Geogr. 30, 409–431. doi: 10.1191/0309133306pp490pr
Sun, Z. K., and He, W. M. (2018). Invasive Solidago canadensis versus its new and old neighbors: their competitive tolerance depends on soil microbial guilds. Flora 248, 43–47. doi: 10.1016/j.flora.2018.08.0115
Szymura, M., and Szymura, T. H. (2016). Historical contingency and spatial processes rather than ecological niche differentiation explain the distribution of invasive goldenrods (Solidago and Euthamia). Plant Ecol. 217, 565–582. doi: 10.1007/s11258-016-0601-1
Theoharides, K. A., and Dukes, J. S. (2007). Plant invasion across space and time: factors affecting nonindigenous species success during four stages of invasion. N. Phytol. 176, 256–273. doi: 10.1111/j.1496-8137.2007.02207.x
Valliere, J. M., Escobedo, E. B., Bucciarelli, G. M., Sharifi, M. R., and Rundel, P. W. (2019). Invasive annuals respond more negatively to drought than native species. N. Phytol. 223, 1647–1656. doi: 10.1111/nph.15865
Wang, N., Li, W., Zhou, B., and Yan, X. (2016). Invasiveness, clonal form and geographical origin of invasive clonal plant species in China. Biodivers. Sci. 24, 12–22. doi: 10.17520/biods.2015190
Wang, Z. X., and He, W. M. (2019). Effects of soil-burial depths on the relationship between seed mass and seed emergence. Chin. J. Plant Ecol. 43, 899–908. doi: 10.17521/cjpe.2019.0154
Weber, E. (2001). Current and potential ranges of three exotic goldenrods (Solidago) in Europe. Conserv. Biol. 15, 122–128. doi: 10.1111/j.1523-1739.2001.99424.x
Werner, P. A., Bradbury, I. K., and Gross, R. S. (1980). The biology of Canadian weeds. 45. Solidago canadensis L. Can. J. Plant Sci. 60, 1393–1409. doi: 10.4141/cjps80-194
Keywords: climate warming, germination rate, germinating time, plant invasion, thousand-seed mass
Citation: Zhou X-H and He W-M (2020) Climate Warming Facilitates Seed Germination in Native but Not Invasive Solidago canadensis Populations. Front. Ecol. Evol. 8:595214. doi: 10.3389/fevo.2020.595214
Received: 15 August 2020; Accepted: 11 November 2020;
Published: 27 November 2020.
Edited by:
Bin Zhu, University of Hartford, United StatesReviewed by:
Magdalena Szymura, Wrocław University of Environmental and Life Sciences, PolandCongyan Wang, Jiangsu University, China
Copyright © 2020 Zhou and He. This is an open-access article distributed under the terms of the Creative Commons Attribution License (CC BY). The use, distribution or reproduction in other forums is permitted, provided the original author(s) and the copyright owner(s) are credited and that the original publication in this journal is cited, in accordance with accepted academic practice. No use, distribution or reproduction is permitted which does not comply with these terms.
*Correspondence: Wei-Ming He, d2VpbWluZ2hlQGliY2FzLmFjLmNu