- 1Earth Island Institute, Berkeley, CA, United States
- 2Wildlife Ecologist, South Lake Tahoe, CA, United States
In mixed-conifer forests inhabited by California spotted owls, land managers hypothesize that without human intervention natural conifer regeneration will take many decades or longer to begin within interior areas of large high-severity fire patches, due to long distances from live tree seed sources. As a result, widespread post-fire logging, followed by sprayed application of herbicides and planting of conifer seedlings, are used to create tree plantations. These are activities routinely conducted in spotted owl territories following fires, despite current data that indicate this approach has adverse impacts on spotted owl occupancy. Land managers acknowledge such impacts, but continue these forest management practices, assuming they are a necessary harm, one that is warranted to ensure the later return of mature conifer forests used by spotted owls for nesting and roosting. However, few data have been gathered to test this hypothesis. At 5 years post-fire, we surveyed field plots on a grid within large high-severity fire patches in spotted owl habitat within the Rim fire of 2013 in the Sierra Nevada, California. In our analysis the percentage of plots lacking conifer regeneration decreased significantly with larger plot sizes, a finding contrary to previous studies which assumed vast “deforested” areas in wildland fires, a bias created by small plot size. We found higher conifer regeneration closer to live-tree edges, but we consistently found natural post-fire conifer regeneration at all distances into interior spaces of large high-severity fire patches, including >300 m from the nearest live trees. Distance from live-tree edges did not affect pine dominance in post-fire regeneration. The post-fire natural conifer regeneration reported in our results suggests that the adverse effects of current post-fire management in spotted owl habitat are not necessary practices that can be justified.
Introduction
In ponderosa pine (Pinus ponderosa) and mixed-conifer forests inhabited by California spotted owls (Strix occidentalis occidentalis) in the Sierra Nevada mountains, historical fire regimes were characterized by a mix of fire severities, comprised primarily of low/moderate-severity effects but also including substantial occurrence of high-severity fire (Mallek et al., 2013; Baker, 2014; Odion et al., 2014; Doerr and Santín, 2016; Baker and Hanson, 2017), in which most or all live trees become snags (Miller and Thode, 2007). However, while it is clear from numerous lines of evidence that large high-severity fire patches (hundreds of ha in size and larger) occurred historically in relatively frequent-fire forest types like ponderosa pine and mixed-conifer in the Sierra Nevada (Leiberg, 1902; Baker, 2014; DellaSala and Hanson, 2019), debate remains about the historical frequency of occurrence of large patches of high-severity fire in these forests and concern exists about the response of forest ecosystems to some large fires that have occurred in recent years (Stephens et al., 2013; Hessburg et al., 2016; Stevens et al., 2016). Similar issues and concerns have been expressed globally, in forests and other ecosystems. This includes forests of Southeastern Australia, boreal forests in Canada and Russia, and fynbos ecosystems in Africa (Kelly et al., 2020; Wintle et al., 2020).
In the ponderosa pine and mixed-conifer forests occupied by spotted owls in California, it is widely hypothesized by land managers that these forest types are not resilient to large high-severity fire patches. Conifer species in these forest types are post-fire seeders (Pausas and Keeley, 2014), and some hypothesize that interior areas of such patches will take many decades or longer for natural conifer regeneration to begin, due to long distances from surviving conifer seed sources in any direction, and that conifer regeneration that does occur will disfavor pine species that have already declined in some areas, while fir and cedar species have increased in places (USFS, 2004, 2016). Other research has reported substantial and heterogeneous natural post-fire conifer regeneration in large high-severity fire patches, even hundreds of meters away from surviving trees, due to a combination of wind dispersal of seeds and dispersal by native birds and small mammals (Owen et al., 2017; Hanson, 2018).
Some previous research has reported vast “deforested” interior areas in large high-severity fire patches, based on the proportion of plots lacking post-fire conifer regeneration (Collins and Roller, 2013; Welch et al., 2016). However, these studies used very small plot sizes (e.g., 0.001–0.006 ha), and it is not known whether the proportions of plots reported to have no conifer seedlings/saplings was influenced by plot size.
Spotted owls inhabit areas burned in wildland fires (Lee and Bond, 2015; Lee, 2018) and actively forage in the snag forest habitat created by high-severity fire patches (Bond et al., 2009, 2013), and post-fire logging of even a seemingly minor portion of such habitat is associated with substantial loss of spotted owl occupancy (Hanson et al., 2018). Nevertheless, extensive post-fire logging, herbicide spraying, and creation of tree plantations is being conducted by land managers in California spotted owl habitat based in significant part on the hypothesis that such activities are warranted and are a necessity to reestablish and ensure future recovery of mature conifer forests used by spotted owls for nesting and roosting (USFS, 2004, 2016). Such post-fire management typically affects over one-third of the conifer forests that experience high-severity fire (Odion and Hanson, 2013), but can affect the majority of high-severity fire areas in some large individual fires (DellaSala et al., 2017).
We investigated natural post-fire conifer regeneration in high-severity fire areas within spotted owl habitat by surveying plots on a grid within large high-severity fire patches in the Rim fire of 2013 within ponderosa pine and mixed-conifer forests inhabited by California spotted owls (Lee and Bond, 2015). We hypothesized that, in areas of pre-existing (pre-fire) forest in such high-severity fire patches: (a) small field plot size creates a bias toward reporting higher proportions of plots lacking conifer regeneration; (b) natural post-fire conifer regeneration would be denser closer to live-tree edges but would occur at all distances from live, surviving conifers; and (c) natural post-fire conifer regeneration would be more pine dominated farther from live-tree edges.
Materials and Methods
Our study area was a 1,766-ha subset of large high-severity fire patches in the ~104,000-ha Rim fire of 2013 in the central Sierra Nevada. A substantial portion of the Rim fire occurred in non-conifer vegetation. Most (69%) of the conifer forest portion was comprised of low/moderate-severity fire, and portions of the high-severity fire component had been post-fire logged prior to our study (Hanson, 2018). Within the high-severity fire areas (Miller and Thode, 2007) in ponderosa pine and mixed-conifer forests in the Rim fire, some were in the form of smaller high-severity fire patches (<50 ha), or were near the low/moderate-severity edges of large high-severity fire patches. In 2018, the U.S. Forest Service mapped a 1,766-ha subset of large high-severity fire patches (hundreds of ha up to >2000 ha) in the Rim fire on the Stanislaus National Forest (USFS, 2018) where the Forest Service and partner agencies were prioritizing post-fire logging and artificial tree planting (Figure 1) on the basis that these specific areas were the most severely burned and were generally farthest from live trees. Thus, all sampling occurred in large high-severity fire patches. Such areas were considered to have “little to no” potential for natural conifer regeneration (HCD (California Department of Housing and Community Development), 2017; HUD (U.S. Department of Housing and Urban Development), 2018). Within this 1,766-ha area, our survey efforts were limited to the ~two-thirds that had not been post-fire logged.
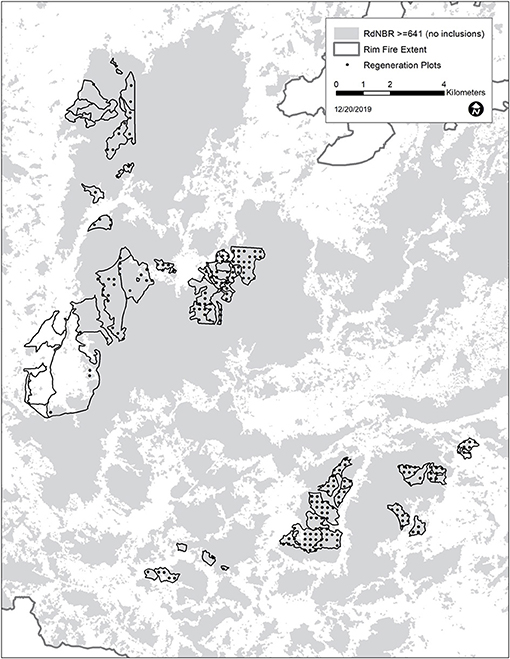
Figure 1. Study area map of the high-severity fire areas (Relative differenced Normalized Burn Ratio [RdNBR] values > 641; Miller and Thode, 2007) and field plots surveyed, in 2018, for this study in the Rim fire.
Forests surveyed within our study area ranged from 900 to 1525 m in elevation, and have mean annual precipitation of 102 cm, but can range from 80 to 172 cm (Kane et al., 2015). Forests primarily consist of ponderosa pine (Pinus ponderosa), Jeffrey pine (P. jeffreyi), sugar pine (P. lambertiana), white fir (Abies concolor), incense-cedar (Calocedrus decurrens), Douglas-fir (Pseudotsuga menziesii), and California black oak (Quercus kelloggii), with shrubs mainly consisting of mountain whitethorn (Ceanothus cordulatus), deer brush (C. integerrimus), and greenleaf manzanita (Arctostaphylos patula) (Hanson, 2018).
Within the 1,766-ha study area, we surveyed for post-fire conifer regeneration in 2018, at 5 years post-fire, in plots on a grid spaced by 200 m (Figure 1), using plot location coordinates provided to us by the U.S. Forest Service from 2015 surveys that the agency conducted (USFS, 2016). The Forest Service did not gather data at a minor portion of plot locations on this 200-m grid, so we added plots at those locations. Areas that had been post-fire logged in 2014–2015 had been mapped by the U.S. Forest Service and we excluded plots from these areas. At each plot location, we recorded the total number of conifer seedlings and saplings within a 5.1-m radius of the plot center, as well as the species group of each seedling/sapling (i.e., fir/cedar or pine). Since our objective was to study post-fire conifer regeneration in conifer forests that were mature at the time of the Rim fire, based on our physical inspections of the plots, we excluded plots confounded by post-fire logging (given that post-fire logging kills most natural conifer regeneration; USFS, 2016), plots with no pre-fire conifers due to past (decades ago) logging damage (old logging roads, landings, and skid trails), and plots in immature tree plantations from clearcut logging prior to the Rim fire. We also excluded natural non-conifer cover types, such as rock outcroppings and water bodies. If we found no conifer regeneration within a 5.1-m radius of plot centers that we surveyed, we then recorded the number and species group (fir/cedar or pine) of conifer regeneration present within a 10-m radius. If no conifer regeneration was present within a 10-m radius, we recorded conifer regeneration amount and species group within a 20-m radius, and so on up to a 30-m radius. From a given plot center-point location, each plot radius (5.1, 10, 20, and 30 m) from that center point was sampled once.
To determine whether plot size (increasing radii) affects the proportion of plots with conifer regeneration, we ran a generalized linear mixed model in R, using a binomial error distribution, with plot area as the independent variable (fixed effect) and conifer regeneration (presence/absence) as the response variable. To address variance stemming from the semi-repeated nature of the increasing plot sizes at any given plot center point, in the model we included plot location as a random effect blocking factor. We used package lme4 (Bates et al., 2015) in R (R Core Team, 2020). We also conducted a pairwise test of comparisons of each combination of plot size, using a Tukey post-hoc test, with p-value adjustment for multiple comparisons (function glht, multcomp package for R) (Torsten et al., 2008).
In the Forest Service's 2015 data at the plot locations 2 years post-fire, the agency recorded the distance to the nearest live conifer that survived the Rim fire. With these data (and our own data where Forest Service data were missing, as discussed above), we analyzed the effect of distance from the nearest surviving conifer on the density (stems/ha) of natural conifer regeneration, from data in our 5.1-m radius plots, using a Kruskal–Wallis test (Rosner, 2000) to assess whether categories of increasing distance (<50, 51–150, 151–300, and >300 m from the nearest surviving conifer) affected levels of conifer regeneration.
Last, we used a Chi-square test for trends in binomial proportions (Rosner, 2000) to determine whether the proportion of plots dominated by pine (>50% pine), vs. fir/cedar, within a 5.1-m radius of plot centers increased with greater distance from surviving conifers. Since the sample size was smaller for this analysis, given that some plots did not have regeneration within the 5.1-m plot radius, we used two distance categories: <151 m from surviving conifers and >151 m from surviving conifers.
Results
Of the 169 plots that were visited, we surveyed a total of 102 plots in pre-fire forested areas within high-severity fire patches in the Rim fire. A total of 14 of the 169 initial plots did not meet our study criteria because they were located on rock outcroppings or meadows, or in streams, and were not forested at the time of the Rim fire due to natural landscape conditions. An additional 53 plots of the initial 169 did not meet our study criteria because, while they were historically forested, conifers had not grown in these locations for approximately one to four decades prior to the Rim fire, due to soil damage associated with past logging activities, such as logging roads, skid trails, landings, and logging slash pile burn sites. Thus, 34% of the historically forested landscape (53 out of 155 plots) did not support conifer tree cover at the time the Rim fire occurred due to past logging impacts.
There was a significant effect of increasing plot size on the proportion of plots lacking natural conifer regeneration (Table 1), with lower proportions of plots lacking conifer regeneration in larger plot sizes (Figure 2). Post-hoc pairwise comparisons between each combination of plot sizes revealed that there were significant differences between each plot radius, with fewer and fewer plots lacking conifer regeneration as plots increased in size (Table 2). For plots <151 m from surviving conifers, the proportion of plots lacking natural post-fire conifer regeneration was 0.25, 0.11, 0.05, and 0.04 for plots with a 5.1-m (82 m2), 10-m (314 m2), 20-m (1,256 m2), and 30-m radius (2,826 m2), respectively. For plots 151–300 m from surviving conifers, the proportion of plots lacking conifer regeneration, from smallest to largest plot size, was 0.32, 0.11, 0.00, and 0.00, and, for plots >300 m from surviving conifers, the proportion of plots lacking conifer regeneration was 0.50, 0.18, 0.07, and 0.07 as plot size increased.
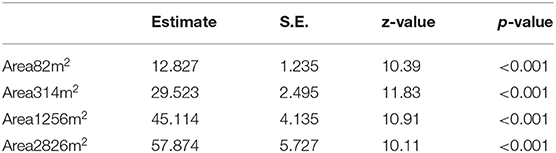
Table 1. Generalized mixed-effects model results for the proportion of plots with conifer regeneration as plot area (m2) increases.
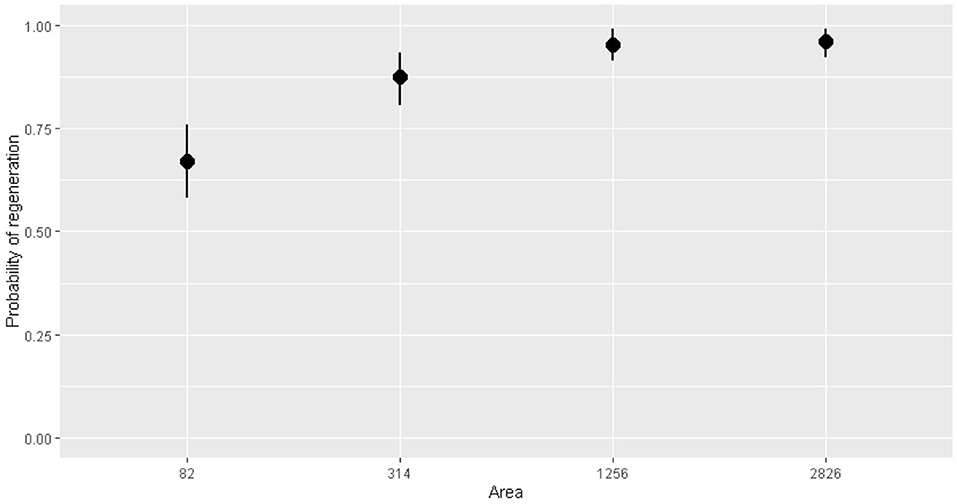
Figure 2. From the raw data, the proportion of plots with natural post-fire conifer regeneration in large high-severity fire patches increases with increasing plot area (m2), resulting in only a small proportion of plots lacking conifer regeneration in the larger plots.
There was a significant effect of distance from surviving trees on the density of conifer regeneration, with denser regeneration closer to live trees and more open regeneration at farther distances from surviving trees (H = 18.29, df = 3, p < 0.001). We found mean natural regeneration of 1,953 stems/ha (SD = 2,529), 389 stems/ha (SD = 530), 514 stems/ha (SD = 1,050), and 256 stems/ha (SD = 362) at distances of <50 m (n = 36), 51-150 m (n = 19), 151-300 m (n = 19), and >300 m (n = 28) from the nearest live, surviving conifers, respectively.
There was no effect of distance from the nearest surviving conifers on the proportion of plots dominated by pine regeneration (χ2 = 0.002, df = 1, p = 0.964). A total of 78% of plots <151 m from surviving conifers were dominated by pine regeneration, and 77% of plots >151 m from surviving conifers were dominated by pine regeneration. For plots >300 m from the nearest live, surviving conifers, the result was the same: 77% of such plots were dominated by pine regeneration.
Discussion
Our results indicate that ponderosa pine and mixed-conifer forests inhabited by California spotted owls are experiencing substantial natural conifer regeneration after large, high-severity wildland fires, contrary to the assumptions underlying current prevailing land management policies dominated by extensive post-fire clearcutting and tree plantation establishment in spotted owl territories.
Plot size was important regarding the proportion of plots containing conifer regeneration in interior areas of large high-severity fire patches, similar to the results of Dunn et al. (2020). Our results indicate that use of small plot sizes creates a bias toward reporting high proportions of plots lacking conifer regeneration in high-severity fire patches. In other words, small plot sizes create a bias against “detecting” conifer regeneration when it is present. For example, in plots >300 m from the nearest live tree, increasing plot size from a 5.1-m radius to a 20-m radius reduced the percentage of plots without conifer regeneration from 50 to only 7%. Use of larger plots, or plot clusters, has become common in studies measuring natural conifer regeneration in high-severity burn forests (Donato et al., 2009; Crotteau et al., 2013; Owen et al., 2017; Downing et al., 2019; Dunn et al., 2020) and is a sampling methodology that offsets potential error associated with the natural variability and heterogeneity of forested landscapes.
There is a body of research expressing concern about lack of natural post-fire conifer regeneration in high-severity fire patches, with a special emphasis placed on large high-severity patches (Savage and Mast, 2005; Collins and Roller, 2013; Welch et al., 2016; Stevens-Rumann et al., 2018). Such findings must be considered seriously and carefully evaluated. One common denominator among these studies is very small plot size. While plot size would not be expected to affect mean values, where there is adequate sampling, our findings suggest it influences the proportion of plots reported to have no conifer regeneration—a key metric reported in recent studies (Collins and Roller, 2013; Welch et al., 2016). Other concerns regarding obstacles to forest regrowth have been made by researchers who have hypothesized that hotter and drier future conditions, driven by climate change, could create additional challenges for natural post-fire conifer regeneration within recurrent high-severity fire patches in some areas (Tepley et al., 2017). Future research will be needed to assess this.
Our results indicate that using small plots and reporting the proportion of these plots without conifer regeneration, or the median values (which will often be zero when plots are very small), has the potential to create a misleading impression that post-fire conifer regeneration in high-severity fire patches occurs almost exclusively near live-tree edges and is nearly absent far from surviving conifers—an assumption that is driving and misdirecting current forest management to perform widespread post-fire clearcutting and artificial tree planting in spotted owl territories (USFS, 2014, 2016).
Moreover, this effect may be exacerbated by the fact that some post-fire conifer regeneration studies fail to exclude natural non-conifer locations, such as rock outcroppings and meadows (there were 14 such plots that we excluded from our analysis), or adequately characterize pre-fire baseline forested conditions at locations where conifers have not grown for decades due to soil damage from past logging activities, such as in logging roads, landings, and logging slash pile burn sites (Elliot et al., 1996). There were 53 such plots that we excluded from our analysis.
Another factor that is driving post-fire logging and planting policies in spotted owl habitat is adherence by land managers to older industrial forestry “stocking” standards, such as the U.S. Forest Service's 1989 Silvicultural Forest Handbook, which contains a standard for planting dense tree plantations with 494 seedlings/ha after clearcutting (USFS, 1989). Though there is no requirement that such industrial forestry standards be applied to post-fire environments, especially if such post-fire habitats are not subjected to clearcut logging, land managers have been consistently applying this standard to wildland fires for decades. Many scientists, including U.S. Forest Service scientists, have recently recommended that land managers embrace ecological heterogeneity in post-fire environments—an approach that accepts both dense and open forest regeneration as beneficial (Welch et al., 2016; North et al., 2019)—but incorporation of these recommendations into management decisions has thus far been minimal.
Post-fire conifer regeneration in the Rim fire was highest near live-tree edges, but we found substantial post-fire conifer regeneration at all distances from surviving conifers. Even in locations farthest into the interior of large high-severity fire patches, >300 m from the nearest surviving conifer, density of natural post-fire conifer regeneration was 256 stems/ha, which is in the mid-range of recently-articulated values associated with successful post-fire conifer regeneration (North et al., 2019).
We did not find any effect of distance from surviving conifers on the proportion of conifer regeneration comprised by pine species, contrary to Hanson (2018), and our results contradict the hypothesis adopted by USFS (USFS, 2004, 2016) suggesting conifer regeneration that occurs in large high-severity fire patches will be dominated by fir/cedar species. In plots >300 m from surviving conifers, over three-quarters had post-fire regeneration that was pine-dominated. Our results support the North et al. (2019) conclusion, based on a synthesis of recent scientific findings about post-fire conifer regeneration, recommending that land managers consider high-severity fire areas within 200 m of live trees to be capable of natural regeneration, and consequently not in need of human intervention. Our findings indicate that forests inhabited by spotted owls do not require assistance for recovery and are capable to naturally regenerate quite well at much farther distances from live trees than previously suggested.
Future choices made by land managers regarding post-fire habitats, and emerging science about post-fire succession, will be consequential for spotted owls. Despite ongoing methodological differences between researchers, there is broad agreement that current post-fire management—characterized by clearcutting of snag forest habitat, spraying of herbicides to eliminate native shrubs, and creation of tree plantations—is harmful to declining spotted owl populations (Hanson et al., 2018; Jones et al., 2018; Lee, 2018; Peery et al., 2019). Moreover, research indicates that such post-fire clearcutting and creation of tree plantations may often lead to higher future fire severity (Thompson et al., 2007; Bradley et al., 2016).
Our results indicate that well-documented harm to spotted owl occupancy from current post-fire management practices is not a necessary impact to which the owls must be subjected in order to restore or recover forests following large, intense wildland fires, and that forest types primarily selected by spotted owls naturally regenerate after such fires. We believe the current evidence warrants a reevaluation of past and ongoing post-fire management in spotted owl habitat.
Data Availability Statement
The original contributions presented in the study are included in the article/supplementary materials, further inquiries can be directed to the corresponding author/s.
Author Contributions
CH and TC were involved in all aspects of this study. CH was relatively more involved in the design and writing of the study, while TC was relatively more involved in the field data collection, but both authors were substantially involved in all stages. All authors contributed to the article and approved the submitted version.
Conflict of Interest
The authors declare that the research was conducted in the absence of any commercial or financial relationships that could be construed as a potential conflict of interest.
Acknowledgments
We thank the two reviewers for helping to improve this manuscript through their thoughtful comments and suggestions. We thank Tim Sinnott for the Geographic Information System (GIS) work on this study. We also thank Christy Sherr and Maya Khosla for assistance in field plot data collection, and extend a very special thanks to our late friend and colleague, Dennis Odion, for his assistance in collection of field data for this study. Dennis Odion, his professional contributions, and lifelong scientific expertise will be greatly missed in the ecological community. Finally, we thank Environment Now foundation for providing funding to conduct this study.
References
Baker, W. L. (2014). Historical forest structure and fire in Sierran mixed-conifer forests reconstructed from General Land Office survey data. Ecosphere 5:79. doi: 10.1890/ES14-00046.1
Baker, W. L., and Hanson, C. T. (2017). Improving the use of early timber inventories in reconstructing historical dry forests and fire in the western United States. Ecosphere 8:e01935. doi: 10.1002/ecs2.1935
Bates, D., Maechler, M., Bolker, B., and Walker, S. (2015). Fitting linear mixed-effects models using lme4. J. Stat. Software 67, 1–48. doi: 10.18637/jss.v067.i01
Bond, M. L., Lee, D. E., Siegel, R. B., and Tingley, M. W. (2013). Diet and home-range size of California spotted owls in a burned forest. Birds 44, 114–126. Available online at: https://archive.westernfieldornithologists.org/archive/V44/WB-Bond-44(2).pdf
Bond, M. L., Lee, D. E., Siegel, R. B., and Ward, J. P. Jr. (2009). Habitat use and selection by California spotted owls in a postfire landscape. J. Wildlife Manage. 73, 1116–1124. doi: 10.2193/2008-248
Bradley, C. M., Hanson, C. T., and DellaSala, D. A. (2016). Does increased forest protection correspond to higher fire severity in frequent-fire forests of the western USA? Ecosphere 7:e01492. doi: 10.1002/ecs2.1492
Collins, B. M., and Roller, G. B. (2013). Early forest dynamics in stand- replacing fire patches in the northern Sierra Nevada, California, USA. Landscape Ecol. 28, 1801–1813. doi: 10.1007/s10980-013-9923-8
Crotteau, J. S., Varner, J. S. I. I. I., and Ritchie, M. W. (2013). Post-fire regeneration across a fire severity gradient in the southern Cascades. For. Ecol. Manage. 287, 103–112. doi: 10.1016/j.foreco.2012.09.022
DellaSala, D. A., and Hanson, C. T. (2019). Are wildland fires increasing large patches of complex early seral forest habitat? Diversity 11:157. doi: 10.3390/d11090157
DellaSala, D. A., Hutto, R. L., Hanson, C. T., Bond, M. L., Ingalsbee, T., Odion, D., and Baker, W. L. (2017). Accomodating mixed-severity fire to restore and maintain ecosystem integrity with a focus on the Sierra Nevada of California, USA. Fire Ecol. 13, 148–171. doi: 10.4996/fireecology.130248173
Doerr, S. H., and Santín, C. (2016). Global trends in wildfire and its impacts: perceptions versus realities in a changing world. Phil. Transac. Royal Soc. B 371:20150345. doi: 10.1098/rstb.2015.0345
Donato, D. C., Fontaine, J. B., Robinson, W. D., Kauffman, J. B., and Law, B. E. (2009). Vegetation response to a short interval between high-severity wildfires in a mixed-evergreen forest. J. Ecol. 19, 142–154. doi: 10.1111/j.1365-2745.2008.01456.x
Downing, W. M., Krawchuk, M. A., Meigs, G. W., Haire, S. L., Coop, J. D., Walker, R. B., et al. (2019). Influence of fire refugia spatial pattern on post-fire forest recovery in Oregon's Blue Mountains. Landscape Ecol. 34, 771–792. doi: 10.1007/s10980-019-00802-1
Dunn, C. J., Johnston, J. D., Reilly, M. J., Bailey, J. D., and Miller, R. A. (2020). How does tree regeneration respond to mixed-severity fire in the western Oregon Cascades, USA? Ecosphere 11:e03003. doi: 10.1002/ecs2.3003
Elliot, W. J., Page-Dumroese, D., and Robichaud, P. R. (1996). The Effects of Forest Management on Erosion and Soil Productivity. Symposium on Soil Quality and Erosion Interaction. Keystone, CO (1996).
Hanson, C. T. (2018) Landscape heterogeneity following high-severity fire in California's forests. Wildlife Soc. Bull. 42, 264–271. doi: 10.1002/wsb.871.
Hanson, C. T., Bond, M. L., and Lee, D. E. (2018) Effects of post-fire logging on California spotted owl occupancy. Nat. Cons. 24, 93–105. doi: 10.3897/natureconservation.24.20538
HCD (California Department of Housing and Community Development) (2017). Record of Decision, Rim fire reforestation activities funded by CDBG-NDR Forest and Watershed Health Program (FWHP) (Sacramento, CA).
Hessburg, P. F., Spies, T. A., Perry, D. A., Skinner, C. N., Taylor, A. H., and Brown, P. M. (2016) Tamm Review: Management of mixed-severity fire regime forests in Oregon, Washington, northern California. For. Ecol. Manage. 366, 221–250. doi: 10.1016/j.foreco.2016.01.034.
HUD (U.S. Department of Housing and Urban Development) (2018). State of California Rim Fire Recovery and Reforestation Projects, Authority to Use Grant Funds (HUD form 7015.16). HUD San Francisco Regional Office, Region IX, San Francisco, CA.
Jones, G. M., Gutiérrez, R. J., Kramer, H. A., Tempel, D. J., Berigan, W. J., Whitmore, S. A., et al. (2018). Assessing the quality of fire refugia for wildlife habitat. Nat. Cons. 37, 31–51. doi: 10.1016/j.foreco.2020.118868
Kane, V. R., Cansler, C. A., Povak, N. A., Kane, J. T., McGaughey, R. J., Lutz, J. A., Churchill, D. J., and North, M. P. (2015). Mixed severity fire effects within the Rim fire: relative importance of local climate, fire weather, topography, and forest structure. For. Ecol. Manage. 358, 62–79. doi: 10.1016/j.foreco.2015.09.001
Kelly, L. T., Giljohann, K. M., Duane, A., Aquilué, N., Archibald, S., Batllori, E., et al. (2020). Fire and biodiversity in the Anthropocene. Science 370:eabb0355. doi: 10.1126/science.abb0355
Lee, D. E. (2018). Spotted Owls and forest fire: a systematic review and meta-analysis of the evidence. Ecosphere 9:e02354. doi: 10.1002/ecs2.2354
Lee, D. E., and Bond, M. L. (2015). Occupancy of California spotted owl sites following a large fire in the Sierra Nevada. Condor 117, 228–236. doi: 10.1650/CONDOR-14-155.1
Leiberg, J. B. (1902). Forest Conditions in the Northern Sierra Nevada, California. USDI Geological Survey, Professional Paper No. 8. U.S. Government Printing Office, Washington, D.C., USA. doi: 10.3133/pp8
Mallek, C., Safford, H., Viers, J., and Miller, J. (2013). Modern departures in fire severity and area vary by forest type, Sierra Nevada and southern Cascades, USA. Ecosphere 4:153. doi: 10.1890/ES13-00217.1
Miller, J. D., and Thode, A. E. (2007). Quantifying burn severity in a heterogeneous landscape with a relative version of the delta Normalized Burn Ratio (dNBR). Remote Sens. Envir. 109, 66–80. doi: 10.1016/j.rse.2006.12.006
North, M. P., Stevens, J. T., Greene, D. F., Coppoletta, M., Knapp, E. E., Latimer, A. M., et al. (2019). TAMM review: reforestation for resilience in dry western U.S. forests. For. Ecol. Manage. 432, 209–224. doi: 10.1016/j.foreco.2018.09.007
Odion, D. C., and Hanson, C. T. (2013). Projecting impacts of fire management on a biodiversity indicator in the Sierra Nevada and Cascades, USA: the Black-backed Woodpecker. Open For. Sci. J. 6, 14–23. doi: 10.2174/1874398620130508001
Odion, D. C., Hanson, C. T., Arsenault, A., Baker, W. L., DellaSala, D. A., Hutto, R. L., et al. (2014). Examining historical and current mixed-severity fire regimes in ponderosa pine and mixed-conifer forests of western North America. PLoS ONE 9:e87852. doi: 10.1371/journal.pone.0087852
Owen, S. M., Sieg, C. H., Sánchez Meador, A. J., Ful,é, P. Z., Inigueza, J. M., Baggett, L. S., Fornwalt, P. J., and Battaglia, M. A. (2017). Ponderosa pine regeneration in high-severity burn patches. Forest Ecol. Manage. 405, 134–149. doi: 10.1016/j.foreco.2017.09.005
Pausas, J. G., and Keeley, J. E. (2014). Evolutionary ecology of resprouting and seeding in fire-prone ecosystems. N. Phytol. 204, 55–65. doi: 10.1111/nph.12921
Peery, M. Z., Jones, G. M., Gutiérrez, R. J., Redpath, S. M., Franklin, A. B., and Simberloff, D. (2019) The conundrum of agenda-driven science in conservation. Front. Ecol. Envir. 17, 80–82. doi: 10.1002/fee.2006.
R Core Team (2020). R: A language and environment for statistical computing. R Foundation for Statistical Computing, Vienna, Austria. http://www.R-project.org/
Rosner, A. (2000). Fundamentals of biostatistics. Fifth edition. Duxbury, Pacific Grove, California, USA.
Savage, M., and Mast, J. N. (2005). How resilient are southwestern ponderosa pine forests after crown fires? Can. J. For. Res. 35, 967–977. doi: 10.1139/x05-028
Stephens, S. L., Agee, J. K., Fulé, P. Z., North, M. P., Romme, W. H., Swetnam, T. W., et al. (2013). Managing forests and fire in changing climates. Science 342, 41–42. doi: 10.1126/science.1240294
Stevens, J. T., Safford, H. D., North, M. P., Fried, J. S., Gray, A. N., Brown, P. M., et al. (2016). Average stand age from forest inventory plots does not describe historical fire regimes in ponderosa pine and mixed-conifer forests of western North America. PLoS ONE 11:e0147688. doi: 10.1371/journal.pone.0147688
Stevens-Rumann, C., Kemp, K. B., Higuera, P. E., Harvey, B. J., Rother, M. T., Donato, D. C., et al. (2018). Evidence for declining forest resilience to wildfires under climate change. Ecol. Lett. 21, 243–252. doi: 10.1111/ele.12889
Tepley, A. J., Thompson, J. R., Epstein, H. E., Anderson-Teixeira, K. J., and Anderson-Teixeira, K. J. (2017) Vulnerability to forest loss through altered postfire recovery dynamics in a warming climate in the Klamath Mountains. Glob. Chang. Biol. 23, 4117–4132. doi: 10.1111/gcb.13704
Thompson, J. R., Spies, T. A., and Ganio, L. M. (2007). Reburn severity in managed and unmanaged vegetation in a large wildfire. Proc. Natl. Acad. Sci. U.S.A. 104, 10743–10748. doi: 10.1073/pnas.0700229104
Torsten, H., Bretz, F., and Westfall, P. (2008). Simultaneous inference in general parametric models. Biometr. J. 50, 346–363. doi: 10.1002/bimj.200810425
USFS (1989). Silvicultural Forest Handbook R5. USDA Forest Service Pacific Southwest Region, San Francisco, California, USA.
USFS (2004). Sierra Nevada Forest Plan Amendment, Final Environmental Impact Statement and Record of Decision. U.S. Forest Service, Pacific Southwest Region, Vallejo, California, USA.
USFS (2014). Rim fire recovery final environmental impact statement. U.S. Forest Service, Stanislaus National Forest. Sonora, California, USA.
USFS (2016). Rim fire reforestation final environmental impact statement. U.S. Forest Service, Stanislaus National Forest. Sonora, California, USA.
USFS (2018). Stanislaus National Forest NDRC [National Disaster Resilience Competition] Grant Work and Hazard Tree Removal Map. Stanislaus National Forest Supervisor's Office, Sonora, CA.
Welch, K. R., Safford, H. D., and Young, T. P. (2016). Predicting conifer establishment post wildfire in mixed conifer forests of the North American Mediterranean-climate zone. Ecosphere 7:e01609. doi: 10.1002/ecs2.1609
Keywords: spotted owl, fire severity, forest, postfire logging, forest regrowth
Citation: Hanson CT and Chi TY (2021) Impacts of Postfire Management Are Unjustified in Spotted Owl Habitat. Front. Ecol. Evol. 9:596282. doi: 10.3389/fevo.2021.596282
Received: 18 August 2020; Accepted: 11 January 2021;
Published: 02 February 2021.
Edited by:
Jose Marcelo Domingues Torezan, State University of Londrina, BrazilReviewed by:
Carl R. Gosper, Conservation and Attractions (DBCA), AustraliaViorel Dan Popescu, Ohio University, United States
Copyright © 2021 Hanson and Chi. This is an open-access article distributed under the terms of the Creative Commons Attribution License (CC BY). The use, distribution or reproduction in other forums is permitted, provided the original author(s) and the copyright owner(s) are credited and that the original publication in this journal is cited, in accordance with accepted academic practice. No use, distribution or reproduction is permitted which does not comply with these terms.
*Correspondence: Chad T. Hanson, Y3RoYW5zb24xQGdtYWlsLmNvbQ==