- 1Department of Biology, Faculty of Natural Sciences, Shumen University, Shumen, Bulgaria
- 2Department of Integrative Zoology, Vienna University, Vienna, Austria
- 3Faculty of Natural and Agricultural Sciences, Ovidius University of Constanţa, Constanţa, Romania
- 4Asociatia Chelonia Romania, Bucharest, Romania
- 5CEDMOG Research Center, Ovidius University of Constanţa, Constanţa, Romania
The study of the feeding mechanisms in vertebrates requires an integrative approach since the feeding event consists of a chain of behaviors. In the present study we investigated the food uptake behavior in different ontogenetic stages in the Danube crested newt (Triturus dobrogicus). We focused on the coordination in the kinematics of the elements of the locomotor and the feeding systems at the transition between the approach of the newt to the prey and the food uptake start. In the feeding strategy of the larvae of T. dobrogicus, the phase of food search is replaced by an initial “food detection phase.” In both larvae and adult specimens, the animals approached the food to a close distance by a precise positioning of the snout besides the food item. The larvae were able to reach food items offered at over 80° relative to the longitudinal midline of the head. When the food was offered at a large distance or laterally, the food uptake was either not successful or the coordination chain at the transition between food approach and food uptake was interrupted. In young larvae we detected an abrupt change in the activity of the locomotor system and the feeding system. The larvae approached the food by tail undulation and after reaching the final position of attack, no further activity of the locomotor apparatus was detectable. The larvae used a pure form of inertial suction to ingest food. In pre-metamorphic larvae and adults we registered an integrated activation of the locomotor apparatus (both limbs and tail) and the feeding apparatus during prey capture in the form of compensatory suction. The drastic change in the feeding mode of the pre-metamorphotic larvae and the adults compared to the younger larvae in T. dobrogicus may indicate the evolutionary development of a defined relation in the activity of the locomotor system and the control of the feeding apparatus. We propose that in newts, the interaction between the control execution in both systems switched from successive (body movement – feeding) into integrated (body movement – body movement and feeding) during the ontogeny. The main trigger for such a switch (at least in T. dobrogicus) is the formation of functional limbs during the late larval development.
Introduction
In vertebrates, the movements of the cranial and post-cranial element during the feeding events can be integrated to different levels (Montuelle and Kane, 2019). In many vertebrate groups, the kinematics of the feeding apparatus during the initial phases of the feeding process (see Schwenk, 2000) is well documented by using high-speed videography and cineradiography (for an overview see Bels and Whishaw, 2019). Schwenk and Rubega (2005) define the feeding stages which follow after the reaching of a distance that allows for successful food uptake, as discrete stages: (i) the capture/subjugation (present in snakes, some birds and mammals), (ii) the food ingestion, (iii) the intraoral food transport, (iv) the food processing (sometimes simultaneously occurring with the transport), and (v) the swallowing. The stages of capture/subjugation (when present) and ingestion are regarded as the initial stages of the feeding process sensu stricto. Montuelle and Kane (2019) define two behavioral phases which precede the prey capture – the “searching” and the “approaching.” The activity of the sensors and the locomotion system during searching and approaching, allows the predator to recognize and access the prey before the initial stages of the feeding process. The activity of the locomotion system during the searching phase may be absent in some “sit and wait” predators.
Such a theoretical framework allows for an exact description of the food uptake mode and behavior in most ectothermic vertebrates, where often the capture/subjugation stage is integrated within the ingestion phase. However, in some species a discrete capture/subjugation feeding stage can be recognized. Very specific pre-ingestion phase is for example the forming of the so-called “hydrodynamic tongue” in mudskippers (Michel et al., 2015). Some specialized fish species may immobilize their prey by using electric current (Catania, 2014, 2019; Mendes-Júnior et al., 2020), or may use their rostrums to confuse, hurt or kill prey before ingestion (Wisner, 1958; Scott and Tibbo, 1968; Stillwell and Kohler, 1985; Shimose et al., 2006). The “scooping” behavior, typical for some frog species like Xenopus leviae (Carreño and Nishikawa, 2010), Pipa pipa (O’Reilly et al., 2002; Carreño and Nishikawa, 2010; Cundall et al., 2017), and Pelophylax ridibundus (Yordanova et al., 2017) involves the forelimbs in prey capture. In this feeding behavior, the limbs are used as a barrier that prohibits the prey from leaving the oropharynx of the predator (the limbs are not involved directly in prey capture and the capture itself is based on the use of hydrodynamic forces).
The hydrodynamics of the aquatic food ingestion in lower tetrapods are influenced by several factors (see Heiss et al., 2018). Some frogs like X. laevis and P. pipa (as adults) rely on suction feeding (Carreño and Nishikawa, 2010; Cundall et al., 2017). Due to the lack of gill slits, the frogs which can feed underwater use bidirectional water suction mode (see Lauder and Shaffer, 1986). The adult anurans are restricted in their ability to engulf large volumes of water in a suction cycle and suction feeding demand specialization of the feeding apparatus. A unique specialization was described in P. pipa, where the ability to suction feed impacted the whole body plan and even had led to the reorganization of some muscle insertions (Cundall et al., 2017). The metamorphosed urodelans also rely on bidirectional suction feeding (e.g., Lauder and Reilly, 1988; Deban and Wake, 2000; Deban et al., 2001; Deban, 2003). To date, a significant delay in the hyoid retraction start relative to the gape opening start was reported only for cryptobranchids (Reilly and Lauder, 1992; Heiss et al., 2013b). In the Chinese giant salamander (Andrias davidianus), the suction forces are generated initially and predominantly by the displacement of the upper and the lower jaw (Heiss et al., 2013b). In contrast – the under-pressure within the buccal cavity in most salamanders is generated by a rapid retraction of the hyo-lingual complex prior to peak gape (Lauder and Shaffer, 1986; Lauder and Reilly, 1994). Several studies were published on the aquatic feeding behavior and kinematic in adult newts (Heiss et al., 2013a, 2015, 2016, 2017, 2018, 2019; Lukanov et al., 2016; Schwarz et al., 2020a,b,c), but data on the food uptake in newt larvae are still scarce especially in the early larval stages. The current knowledge on the prey capture behavior in urodelans is biased toward investigations on late larval stages and adult specimens (see Lauder and Reilly, 1988; Lauder and Shaffer, 1988; Schwarz et al., 2020a,b,c).
The feeding fitness of the larvae is of a crucial importance for the viability of fishes and amphibians. The feeding success rate of the larvae depends on the proper execution both of the approach to the food and the execution of the food uptake (Sanderson and Kupferberg, 1999). In the present study we investigate the food uptake behavior of the Danube crested newt (Triturus dobrogicus) during different ontogenetic stages. We focus on the locomotor kinematics of both larvae and adults during the approach phases and the timing of the execution of the final approach and the food uptake. We then describe the integration of the motor control of the locomotor and the feeding apparatuses and their functional implications.
Materials and Methods
The Danube Crested Newt has a restricted distribution area, being present in floodplains of large rivers from eastern Austria to Danube Delta (Fahrbach and Gerlach, 2018). The species is characterized by its long body and short limbs. The Danube Crested Newt is the most water related species and has the longest larval stage among Triturus species (Furtula et al., 2009).
On 14.02.2020, we captured nine adult males and eight adult females of T. dobrogicus from a temporary pond located in southeast Romania, part of Danube Delta Biosphere Reserve (Grindul Lupilor, 44°37′15.83″N, 28°48′24.92″E). Immediately after capture, the adults were transported to the laboratory for “Functional Morphology” at “Konstantin Preslavsky” University of Shumen, Bulgaria.
We randomly assigned three females and three males per aquarium (50 cm × 33 cm × 20 cm), except for one aquarium where only two females were housed with three males. The aquaria were filled with 30 L of aged tap water and provided with plastic strips used for oviposition. We renewed the plastic strips every two days and collected the eggs. All the eggs were considered with the date when they were deposited. Half of the water volume was changed every 3 days.
To avoid overcrowding, we housed the eggs in eight plastic aquaria (25 cm × 16 cm × 15 cm), with no more than 14 eggs in each. The aquaria were filled with 6L aged tap water and half of the volume was refreshed weekly. The measured water physico-chemical parameters were constant during the experiment period (mean values: pH: 7.98; dissolved oxygen: 8.36 mg/l; conductivity: 395 μS). Ambient temperature was maintained constant at 20°C ± 1°C and the animals were kept under natural photoperiod throughout the experiment. We checked for eggs that were not developing and removed them from aquaria to avoid fungal infections. After hatching, we moved the freshly hatched larvae to the experimental boxes. These aquaria were filled with 3 L of aged tap water, 1/3 of the water from the aquaria was refreshed with aged tap water every 4 days. When the active feeding started after the yolk resorption, the larvae were fed ad libitum with Cladocera (Daphnia sp., Moina sp.) and Copepoda (Cyclops sp.). At the later larval stages (after 42), we supplemented the food with a mixture of dry low-fat meat (beef liver and beef heart) every day. Metamorphosed animals were housed until the end of the experiment when all the juveniles together with the adults were released at the point of capture.
We started the feeding kinematics investigation at larval stage 42 (according to the developmental stages of Bernabò and Brunelli, 2019). Before the recordings we housed the specimens with no food for 24 h, then we moved the larvae individually in the experimental aquaria where they were filmed. The larvae of T. dobrogicus, are strictly carnivorous (Stojanov et al., 2011) and feed only on moving prey. To simulate movements of the prey, we used same sized pellets of beef liver-heart mixture. Since larvae of the Pacific giant salamander (genus Dicamptodon) were reported to react to larger prey offered at longer distances (Parker, 1994), to exclude the effect of prey size we used calibrated food pellets.
The particles were allowed to drop from the water surfaces to the bottom of the experimental water basin, aimed to sink directly in front of the larvae snout. During sinking, the food pellets usually deviated from the target, landing at the bottom at different distances from the predator and at different angles relative to the longitudinal axis of the larval head (see Figure 1). Each feeding event (successful or not) was recorded in order to assess the effects of the recorded variables on the final feeding success. The performance of the experiments was complicated due to the problems related to the food offering. However, we were able to record a total of 78 films in larval stages 42 to 50 (from stage 42 – 4 films from 2 specimens; stage 43 – 16 films from 5 specimens; stage 44 – 2 films from 1 specimen; stage 46 – 23 films from 5 specimens; stage 47 – 2 films from 2 specimens; stage 48 – 9 films from 2 specimens; stage 49 – 6 films from 3 specimens, stage 50 – 7 films from 2 specimens).
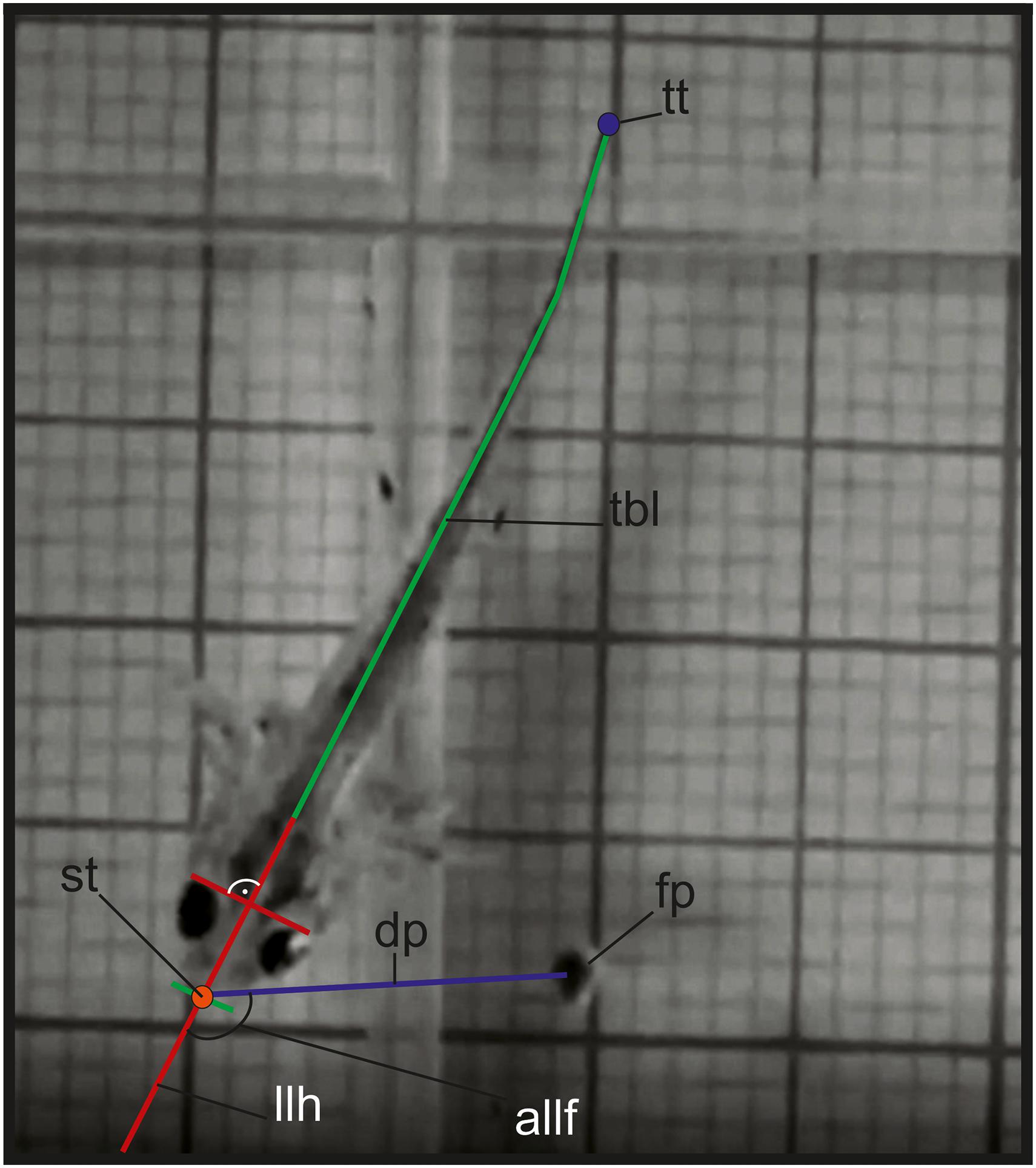
Figure 1. Variables measured in the kinematic analysis in larval feeding: allf, angle between the longitudinal axis of the head and the prey; dp, distance between the tip of the snout and the center of mass of the food item; fp, food particle; llh, longitudinal axis of the head; tbl, total body length; st, snout tip; tt, tail tip.
We refer further in the text to the larvae from stages before 50 as “young larvae.” We noticed that when the larvae entered the pre-metamorphosis stages (around stage 50), the animals started to swim and maneuver very actively. The food was attacked by the predator almost at the water surface – not allowing the particle to drop. This activity hampered us in gathering information concerning the feeding behavior in a comparable way we collected it for the “young larvae” (we produced only seven films from dorsal view). For kinematical comparison we documented three feeding events in our largest larva from stage 50 – we filmed that animal in lateral view when food (domestic fly) was offered at the water surface. We also documented 19 feeding events in two females and two male adult Danube crested newts. The adults were filmed laterally, which allowed for detailed observations of the kinematics of the elements of the feeding apparatus during prey capture.
For high-speed video documentation we used a “Sony RX-III” video system (Sony corporation, Minato, Tokyo, Japan). The films were performed with 1,000 fps (set at “quality mode”: 1136 × 384 p) and for illumination we used two “Ledlenser T-16” (LEDLENSER GmbH & Co. KG, Solingen, North Rhine-Westphalian, Germany) light sources. The videos were imported in Movavi Video Editor v20.1.0 Plus for video processing and frame extraction. The images were digitized by the use of “SIMI-MatchiX” (SIMI Reality Motion Systems, Unterschleißheim, Germany). For larvae we extracted the following variables: A, angle between the food particle center of mass and the longitudinal axis of the larvae head (in degrees); D, prey distance (cm); S, speed of the larvae to the prey during the approach stage (cm/s); TL, Length of the larvae (cm); TT, length of the trajectory of the tail tip (cm); ST, max. speed of the movement of the tail tip during the approach stage (cm/s); SDi, distance traveled by the snout tip during the inertial suction (cm); SS, speed of the displacement of the snout tip during the inertial suction (cm/s) – see Table 1. For the adults we collected data concerning: La, start of limbs before gape opening (s); Dag, delay of limb adduction end to start gape opening (s); Dla, duration of limb adduction (s) – see Table 3 and Figure 2.
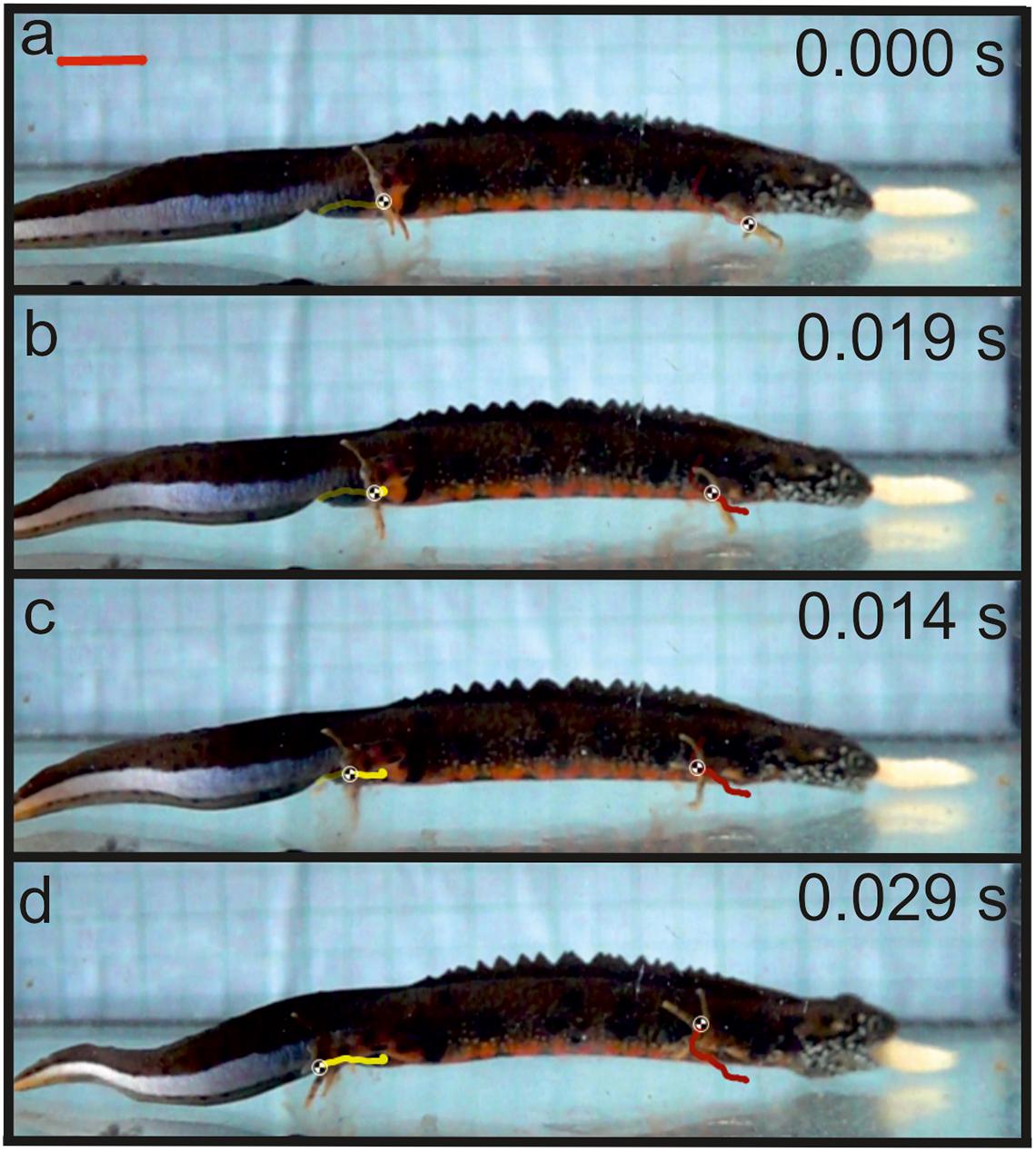
Figure 2. Prey capture event in an adult T. dobrogicus. (a) Start of the limb adduction; (b) start of gape opening; (c) start of hyoid depression; (d) end of limb adduction; the red line indicates the trajectory of the metapodium of the fore-limb and the yellow line indicates the trajectory of the metapodium of the hind-limb.
Each variable was checked for normality using Shapiro-Wilk test. Next we used a Generalized Linear Model (GLM) to check the effect of our variables on the larval success in feeding. For all tests, differences were significant at p < 0.05. We performed all of the statistical analyses using RStudio Version 1.3.1093© 2009-2020 RStudio, PBC.
Results
The relations between the position of the food and the distance to the snout and the feeding success is presented in Tables 1, 2 and Figure 3. A binomial logistic regression performed by using Generalized Linear Models (GLMs) assessed the effects of: (a) A, angle between the center of mass of the food item to longitudinal axis of the larvae head; (b) D, food item distance (cm); (c) S, speed of the larvae during approach (cm/s); (d) TL, Length of the larvae body (cm); (e) TT, trajectory of the tail tip (cm); (f) ST, speed of the movement of the tail tip (cm/s); (g) SDi, inertial suction distance (cm) and (h) SS, speed of the inertial suction displacement of the snout tip (cm/s) (Table 2 and Figure 3). The logistic regression model was statistically significant in case of D (Wald = −2.32, p = 0.02) and ST. The larvae fed successfully with decreasing of the distance to the food item. In case of successful feeding, a higher ST indicates a lower probability of feeding.
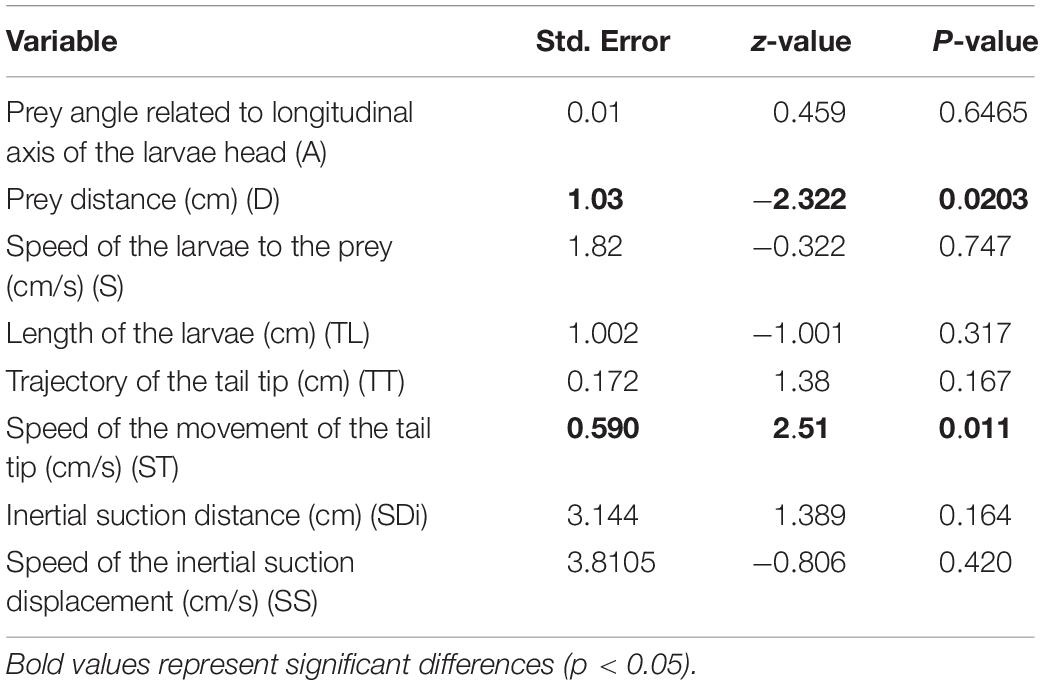
Table 2. Binomial regression coefficients of the following parameters related to success of feeding.
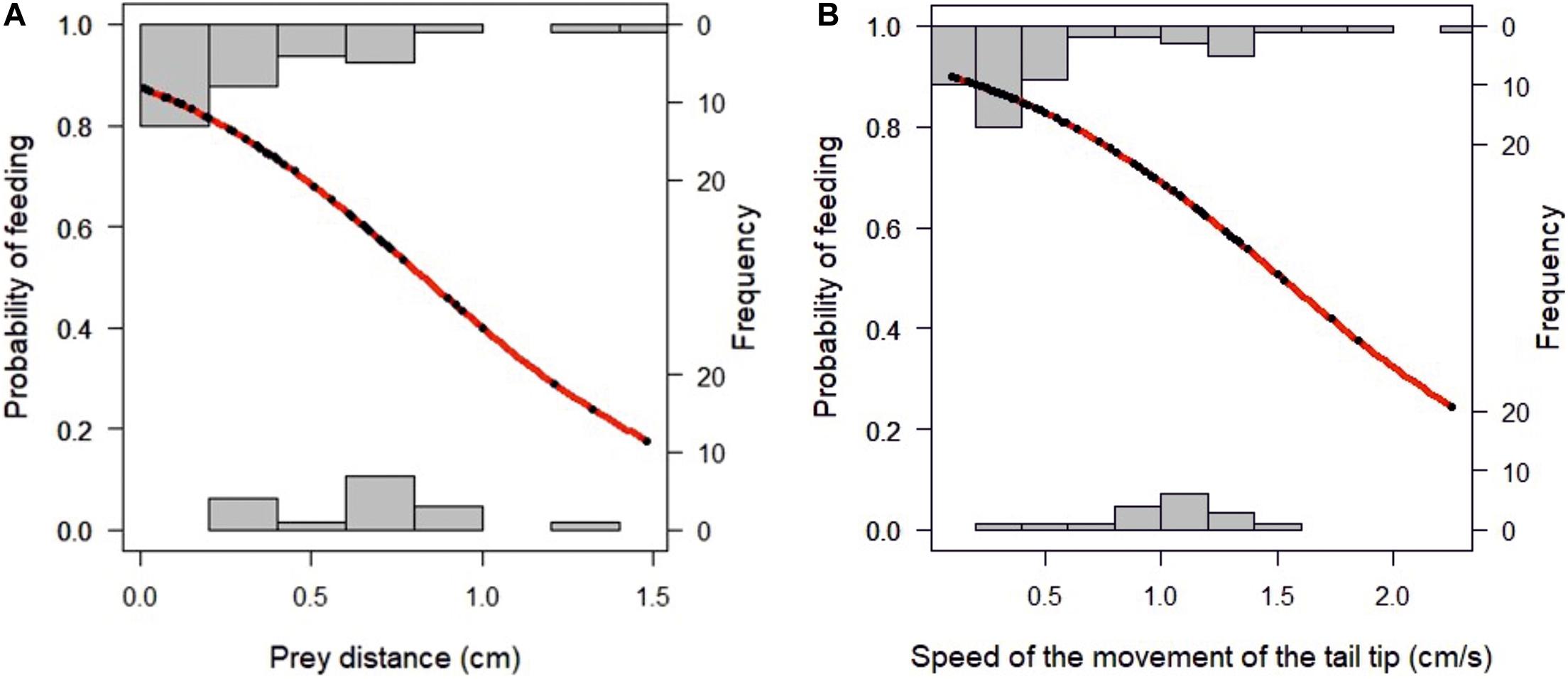
Figure 3. (A) Effects of prey distance on probability of successful feeding; (B) effect of speed on probability of successful feeding.
Our experiments demonstrated that the initial distance between newt and prey had a significant effect on the feeding behavior in the larvae of T. dobrogicus. When the food particle landed on the bottom of the aquarium at relatively long distance (but less than 74% of the total body length), the larvae actively swam toward the item, adjusting the head position and correcting the angle of alignment of the head and the food. In the young larvae, the locomotion was performed only by tail undulation. No involvement of the limbs was detected as the larvae approached the food in a way that the tip of the snout was positioned very close to the food, almost touching it. In the next phase, the food pellet was captured by suction feeding (Figure 4). In case the pellet sank at a distance of less than 6% of the larval body length, it was immediately attacked by the larvae without any activity of the locomotor system (Figure 5).
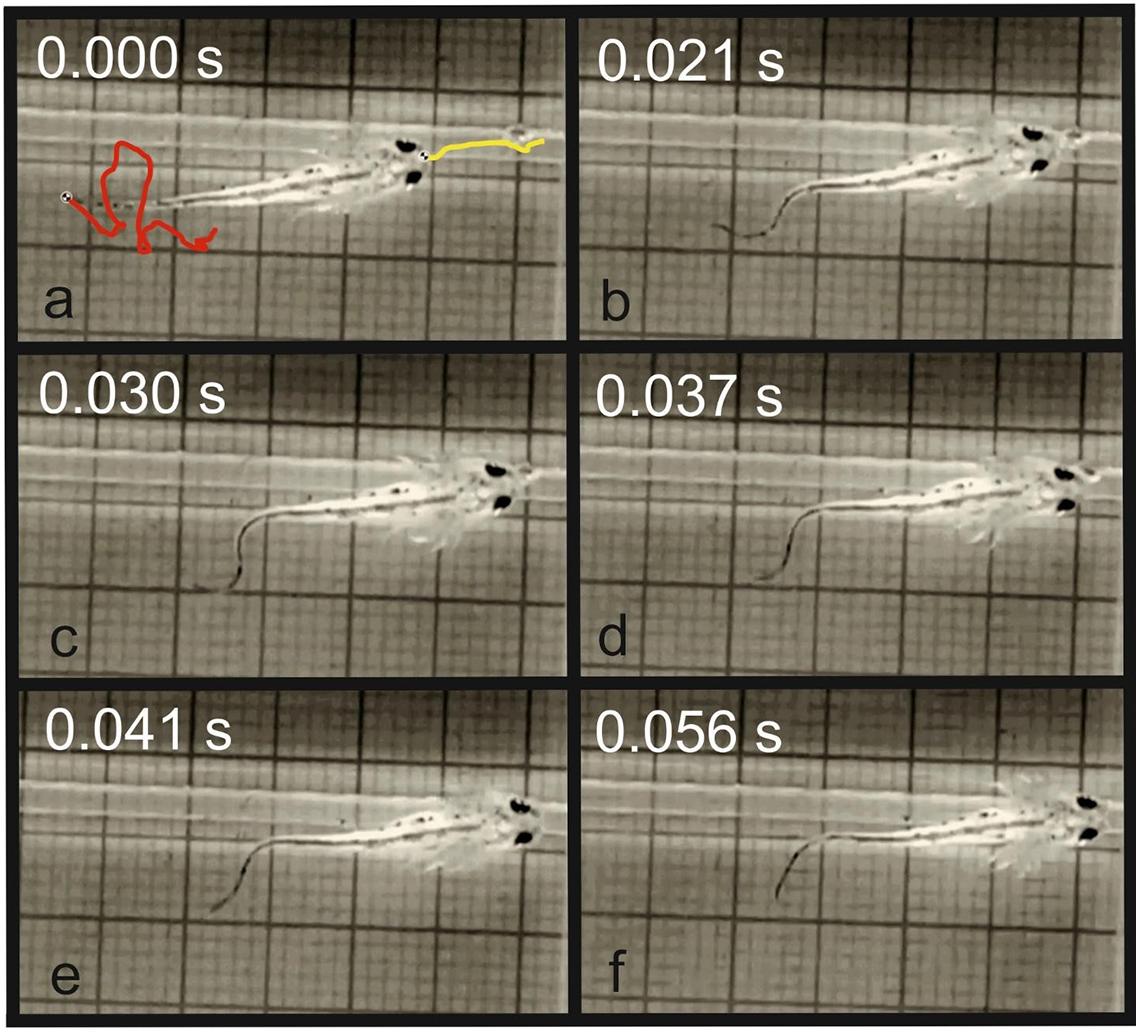
Figure 4. Prey capture in T. dobrogicus larvae. (a) Start of the approach phase (the yellow line represents the trajectory of the tip of the snout and the red line represents the trajectory of the tail tip); (b) end of the approach phase; (c) stationary position of the predator; (d) start of the suction feeding; (e) suction of the food item; (f) end of the food uptake cycle.
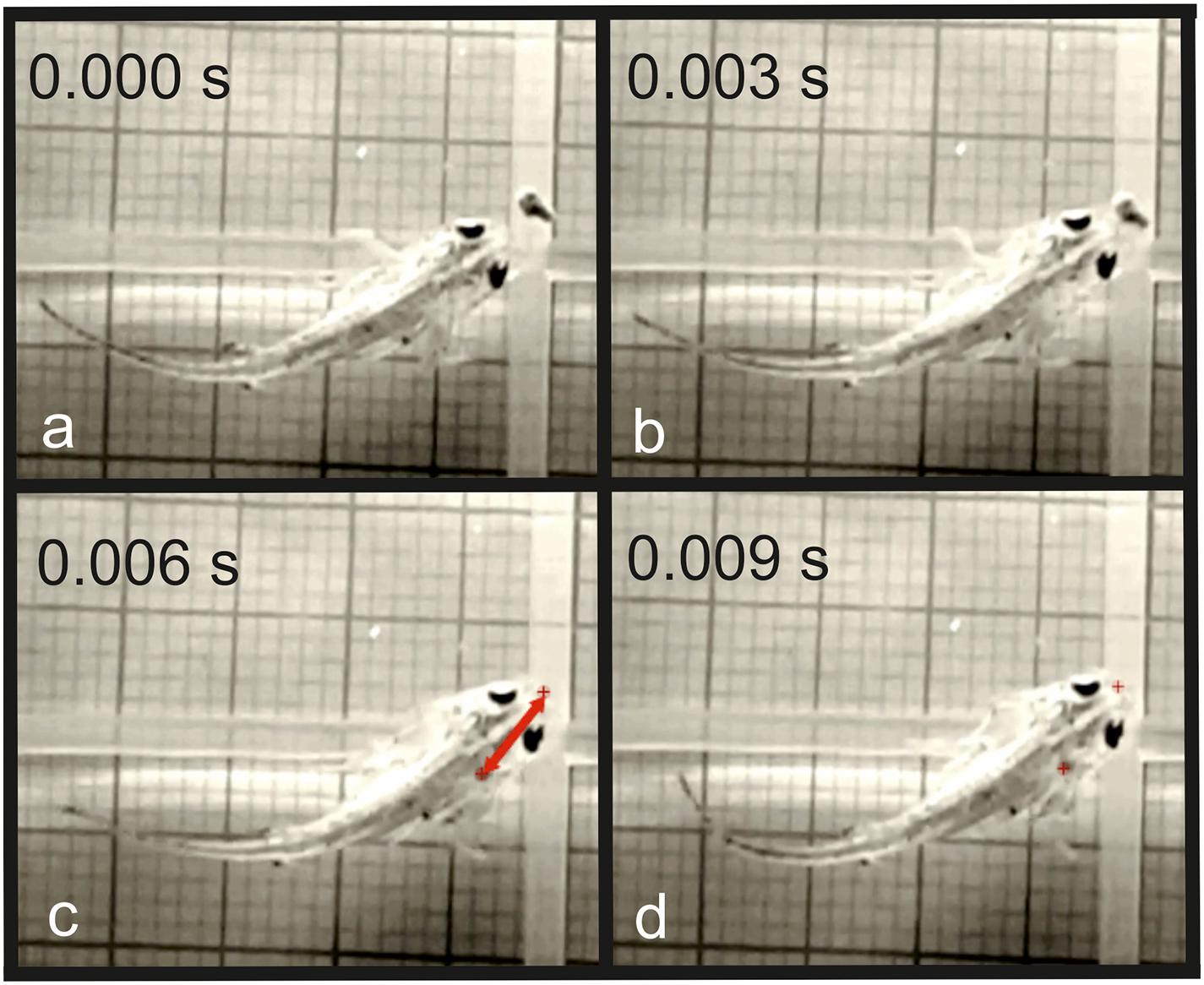
Figure 5. Inertial suction feeding event in T. dobrogicus larvae. (a) Start of mouth opening; (b) start of the movement of the food toward the predator; (c) the food item is ingested in the oropharyngeal cavity; (d) end of the forward movement of the predator; the red crosses indicate the initial and the final position of the food item; the red arrow indicates the distance traveled by the food item during ingestion.
When the particles landed at a distance larger than 74% of the body length, the larvae reacted in two different manners. In most cases, the animals did not react to the stimulus at all. In 17 cases however, the larvae swam toward the food item and positioned their snouts like in the strike demonstrated on Figure 5, hence no further prey capture occurred.
In the pre-metamorphosis larvae from stage 50, the newts approached the prey to a very close distance and then stopped. The active prey capture started by simultaneously activation of the tail and the limbs, followed by the start of the gape opening (Figure 6). In adult Danube crested newts, during the start of the ingestion, we detected a simultaneous activation of the limbs and tail prior jaw opening (Table 3 and Figure 2). The kinematical data measured in adults did not differ significantly between the specimens and sexes (Table 3).
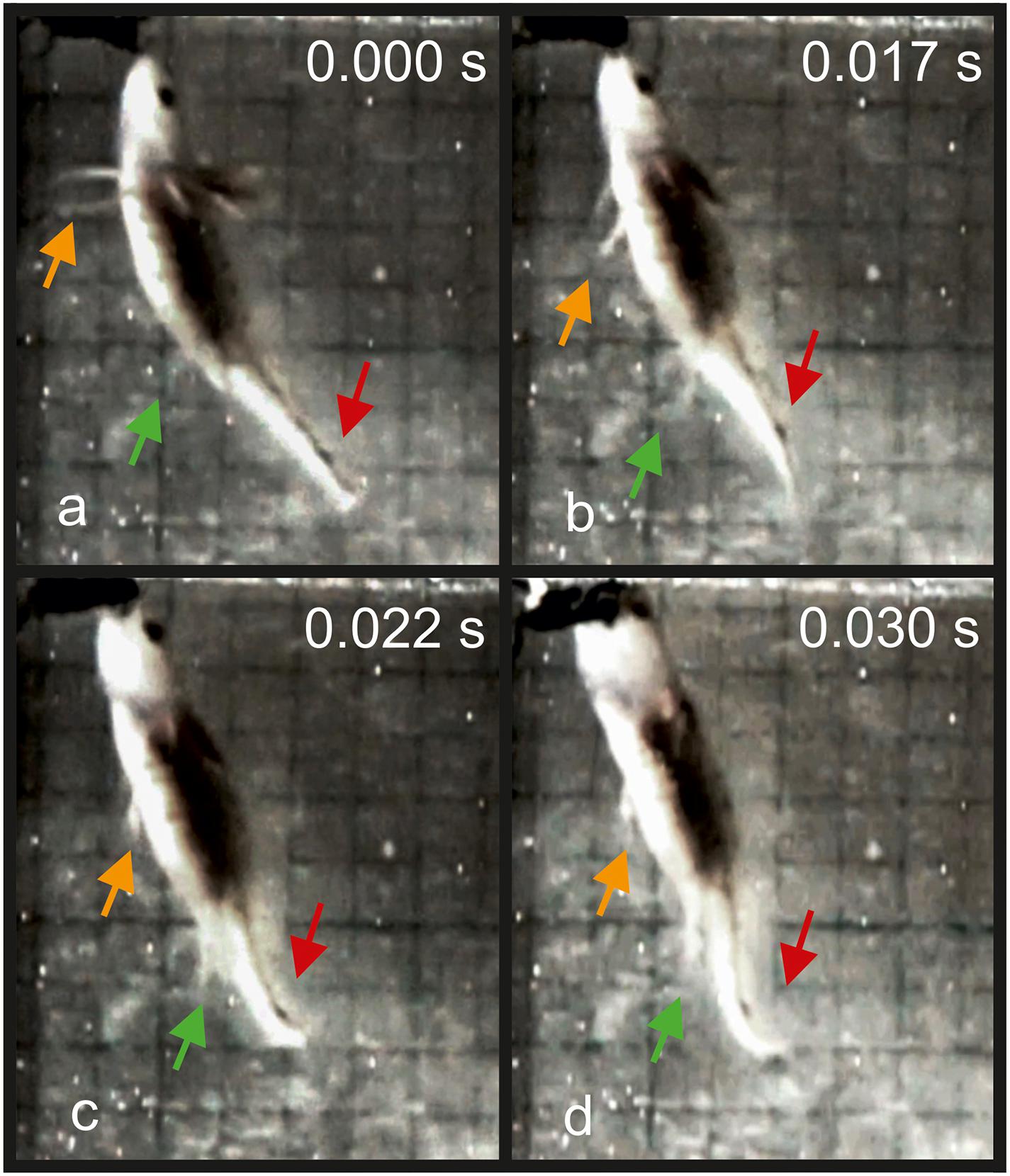
Figure 6. Kinematics of the prey capture in a T. dobrogicus larvae stage 50. (a) Start of the approach phase; (b) start of jaw opening (notice the change in the position of the limbs and the tail); (c) end of limb adduction; (d) reaching peak gape. The yellow arrow indicates the position of the fore-limb metapodium; the green arrow indicates the position of the hind-limb metapodium; the red arrow indicates the undulation of the tail.
Discussion
Based on our results, we propose a theoretical framework concerning the evolution of the integration of the motor programs of the locomotor and feeding apparatus in newts. Only shortly before metamorphosis, the limbs became operational and this impacted the feeding strategy of the developing larvae to large scale. The young larvae in T. dobrogicus are operating predominantly on the bottom and have more or less a two dimensional hunting field. Only after the development of operational limbs in the late pre-metamorphic phases, the larvae started to exploit the whole 3-D volume of the water basin and were able to attack prey from multiple directions (see Figure 6). In the younger stages of the development in T. dobrogicus, the feeding strategy is impacted by the poor potential of the larvae to perform any forms of ram feeding (for overview see Heiss et al., 2018; Montuelle and Kane, 2019).
In newts with seasonal shifts of terrestrial and aquatic life stages, the specialization of the feeding apparatus for underwater prey capture may be constrained. The seasonal change of feeding media (water vs air) is not related only to shifts in the motocontrol programs of the feeding apparatus (suction vs. lingual or jaw prehension), but may lead even to reorganization of the oropharyngeal morphology (Heiss et al., 2013a, 2015, 2016, 2017, 2018; Van Wassenbergh and Heiss, 2016). These authors provide a detailed analysis of the feeding ecology and the functional morphology of the feeding apparatus in the adults of the Alpine newt (Ichthiosaura alpestris) and the Smooth newt (Lissotriton vulgaris). Heiss and De Vylder (2016) report on aquatic suction feeding in the predominantly terrestrial living Himalayan newt (Tylototriton verrucosus). It seems that the Smooth newt (at least in aquatic phase) and the Himalayan newt use predominantly inertial suction for prey capture. The data provided for the aquatic feeding kinematics in the Balkan-Anatolian crested newt (Triturus ivanbureschi) also indicate on the predominant use of inertial suction in this species (Lukanov et al., 2016). The alpine newt, when fed underwater during the terrestrial life stage, is moving forward during suction and the angles of the joints in the forelimb are changing during ingestion (see Heiss et al., 2013a). The involvement of the limb movement in ingestion indicates a similar feeding behavior as described here for the adults in the Danube crested newt. In adult T. dobrogicus, the ingestion stage starts with the simultaneous movement of the fore- and the hind-limbs, in combination with tail undulation (Figure 2). The locomotor apparatus is active until the start of the fast closing gape phase (see Bramble and Wake, 1985), indicating that the adult Danube crested newt use a form of compensatory suction (sensu Aerts et al., 2001) during food ingestion.
Even small differences in the shape and the volume of the oropharynx may largely impact the efficiency of the suction performance (see Lauder, 1979). A larger oropharyngeal space contributes to the execution of powerful suction (see Lemell et al., 2002; Heiss et al., 2013b). The relatively small skull and the bidirectional water suction mode which is used by the adult Danube newts do not allow them to engulf a large amount of water - the bow wave has to be compensated by a lounge of the predator toward the prey. Thus, it is possible that the suction mechanism in T. dobrogicus does not allow the adults to rely on inertial suction and the activity of the locomotor system has to be integrated in the ingestion phase to support successful prey capture. The timing coordination in the activation of the locomotor system and the feeding apparatus is rather uniform and does not indicate significant differences concerning the sexes in the adults in T. dobrogicus.
Our results demonstrate a major difference in the feeding modes used by the young larvae and the adults in the Danube crested newt. In larvae we observed two scenarios of successful feeding depending on food position – a pure inertial suction, when the food was offered close to the snout, and the swimming/stop/suction behavior when the food was offered further from the predator. In the second case, the larvae swam by tail undulations toward the offered food until the snout was positioned at a very close distance to the particle. Then the locomotion apparatus was deactivated and the feeding apparatus was activated during the ingestion stage. The larvae, however, were not motionless during the ingestion stage and moved forward, despite the fact that the locomotor apparatus was not functioning. Such forward movement of the larvae during the ingestion phase was described by Deban and Olson (2002). These authors studied the ingestion mechanism of a tiny pipid frog larvae and found that in Hymenochirus boettgeri, the prey is captured by the extension of a tubular formed mouth that approaches the food. This way the predatory larvae reduces the bow wave - a remarkable convergence to feeding apparati in some teleost species (Osse, 1985). The larvae of H. boettgeri moved only slightly forward during ingestion. For larvae that rely on inertial suction (sensu Aerts et al., 2001), but lack protrudable snouts, they have to engulf more water and the generated momentum is greater – contributing to the forward movement of the whole predator (see Muller and Osse, 1984). In other words, the larvae “suck themselves forward” (Deban and Olson, 2002).
The body form impacts the efficiency of the swimming and steering in the amphibian larvae. In the evolution of the amphibians, the larvae have lost the sturdy plates in form of skeletal fin elements characteristic for the bony fishes and their body execute significant lateral undulation in low Re numbers swimming (Wassersug and Hoff, 1985). The tadpoles show a high yaw rotation during swimming (Wassersug and Hoff, 1985). The bauplan of the salamander larvae is not principally different concerning the hydrodynamics and the streamlining is additionally impacted by the external bushy gills (Sanderson and Kupferberg, 1999). According to Blight (1976), the main difference of the swimming mode in salamander larvae compared to fish, is the high amplitude to which the larvae rotate laterally their heads in swimming (larvae wobble). The yaw of the head during swimming is not significant for the feeding efficiency in most anuran larvae, because they are mostly greasers or rely on filtration (Wassersug and Hoff, 1985). In predators the estimation of distance is crucial for successful prey capture. The highly specialized carnivorous larvae of H. boettgeri possess large eyes which are directed forwards (Deban and Olson, 2002). To reduce yaw in prey capture, these larvae use only the most posterior part of their tail to propel themselves. This results in lack of lateral oscillation of the head (Wassersug and Hoff, 1985). The swimming mode in the young larvae of T. dobrogicus is not contributing to the stabilization of the head. Unlike in H. boettgeri, the tail undulation is initiated in the midsection of the body and the whole tail is involved in swimming (Figures 7a,b). The high lateral displacement of the head prevents from exact aiming of the mouth toward the prey during active swimming. During the food approach phase, the snout tip is constantly redirected left and right off-target due to the wobbling (Figures 7b,c). Directly before the ingestion, the locomotor activity stops and the snout is correctly aimed (Figure 7d).
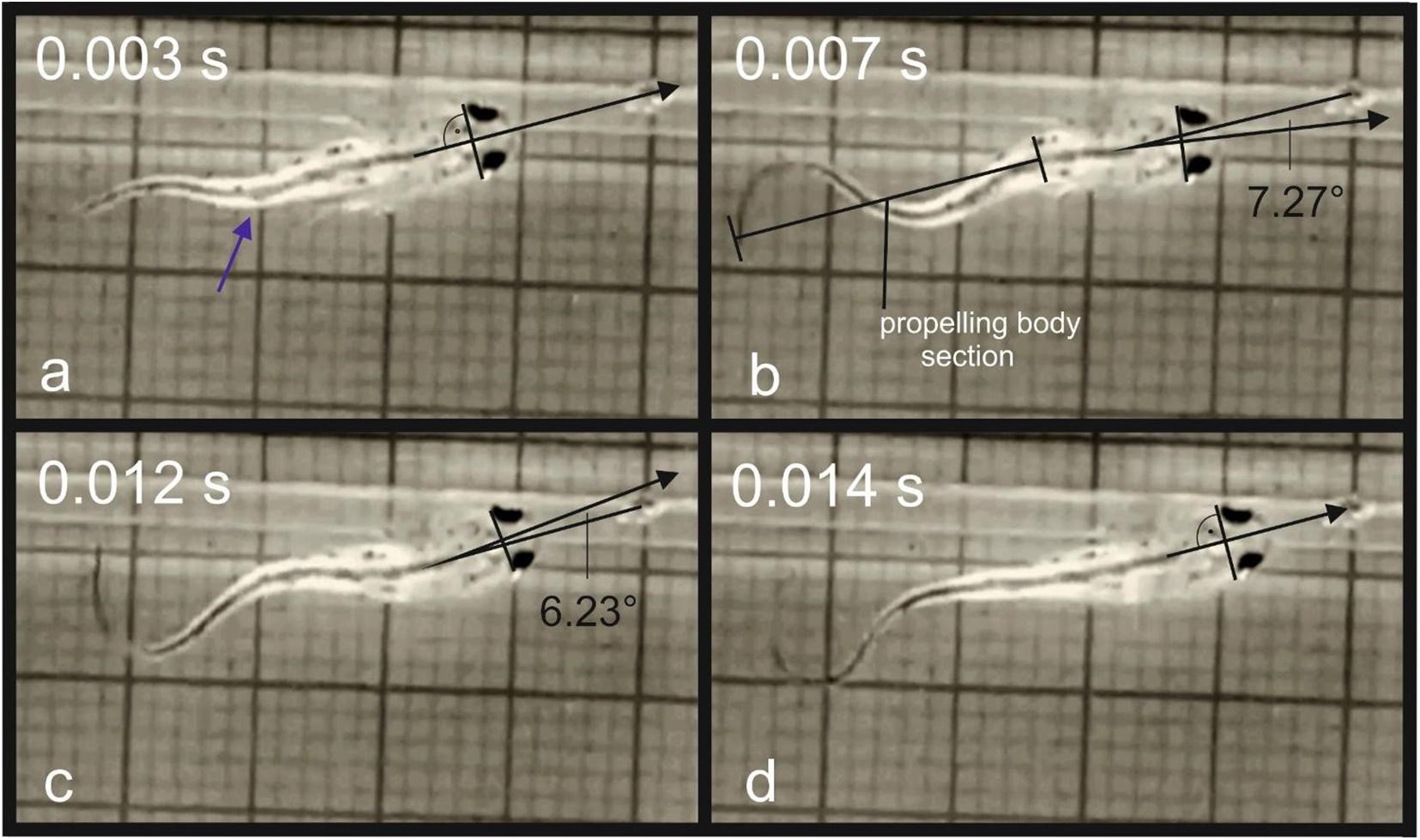
Figure 7. Movement of the tip of the snout during a food uptake event in T. dobrogicus larvae: (a) start of the approach phase (the blue arrow indicates the point where undulation was initially generated); (b) displacement of the snout tip to the right from the correct direction due to head yaw; (c) displacement of the snout tip to the left from the correct direction due to head yaw; (d) stop of the tale undulation.
The young larvae of T. dobrogicus have to approach the food item to a very close distance for a successful grasp - this occurs as the locomotion system transports the predator near the prey. These larvae do not use the tail (or the legs) during the food ingestion. That indicates a strict succession in the execution of the motor control programs of the locomotor and the feeding apparati. Both systems were switched in succession – the locomotor system was active during the prey approach phase and the feeding apparatus was activated in the following ingestion phase. A very interesting point is, that when the larvae had to travel a distance of over 74% body length toward the prey, they successfully took the position of final head fixation (see Lemell and Weisgram, 1997), but the activity of the feeding apparatus was not triggered to start the ingestion stage. This may indicate that the motor programs of the locomotor system and the feeding system are time correlated. The execution of the ingestion may start only within a defined period of time after the activation of the locomotor system, as a reaction to the prey detection in a particular strike. Such a coordination may prevent the energy loss from feeding attempt which could be potentially unsuccessful. We were not able to test that hypothesis, since due to technical limitations, we were forced to use food pellets and not living prey in our experiments with the “young larvae”. In case of active movements of the prey after the predator approached it, a new feeding situation could occur and the larvae may lounge a new attack in reaction to the new stimulus. As Triturus adults are known to feed on immobile prey (see Careddu et al., 2020), a topic for further research is the detection of the ontogenetic stage at which the animals switch their mode of reaction concerning the mobility of the prey.
The larvae changed their feeding strategy at the developmental stage 50. The kinematics in this pre-metamorphosis stage (see Bernabò and Brunelli, 2019) resembles that of the adult specimens. The limbs and the tail were involved in the prey ingestion mode and the prey capture starts with the activation of the locomotor apparatus - the gape opened with a delay relative to the limb and tail activation. The involvement of the functional limbs and the tail in the food ingestion process allowed for a principal change in the execution of the prey capture. The larvae switched from inertial suction to a form of compensatory suction (Heiss et al., 2018) with a component of lounge of the whole body toward the prey. This shift may allow the larvae for hunting of larger and more agile prey, which in turn increase feeding efficiency and may result in improvement of the general fitness of the specimens.
From a biomechanical perspective (see Neenan et al., 2014), one of the crucial factors for the shift in the feeding mode during the ontogeny in T. dobrogicus is the development of movable limbs. In the post 50-stage the limbs can be stretched before the strike in a manner to stabilize the position of the head toward the prey for attack from many possible directions. Possibly, the limbs are also contributing to development of thrust, however at the late larval stages the main momentum was presumably gained by the tail. We suggest that during the prey capture, the forward thrust is delivered predominantly by the tail and the correct lounge direction was maintained with the support of the lateral appendages. During the ingestion, the function of the limbs may be crucial for preventing head yaw and in securing precise aiming of the gaping mouth toward the prey. This function of the lateral appendages may converge to the function of the paired fins in teleost food uptake (Wainwright, 1983; Westneat et al., 2004; Higham, 2007).
The ontogenetic shift may impact the feeding behavior to a large degree in newts (see Schwarz et al., 2020c). Even specimens from closely following ontogenetic phases, like late larval phases and early metamorphosed newts may differ in the execution of some feeding stages. In the case of I. alpestris, the development of the tongue during the metamorphosis has a deep impact on the food processing mode of the newt (Schwarz et al., 2020c). The drastic change in the feeding mode of the pre-metamorphic larvae and adults compared to the younger larvae in T. dobrogicus indicates the evolutionary development of a defined relation in the activity of the locomotor system and the control of the feeding apparatus. We propose that in newts, the interaction between the control execution in both systems switches from successive (body movement – feeding) into integrated (body movement – body movement and feeding) during ontogeny. Our results indicate that this shift in feeding behavior occurrs prior to metamorphosis, and can be crucial for maintaining continuous feeding through metamorphosis. In the genus Triturus, during the postembryonic phase (i.e., the brief period between hatching and feeding) the larvae rely on the rest of the yolk sac. The further development is convoyed by crucial changes in the skeletal morphology (Fahrbach and Gerlach, 2018) and presumably in the skull kinetics, which allows the larvae to include larger and more mobile prey in their diet. Further studies are needed to unravel the levels of integration in the movements of the locomotor system and the hyoid apparatus (as the main generator of suction forces) in Triturus larvae. An important aspect for the in-depth understanding of the ontogenetic shift in the feeding motorics would be the direct comparison of the 3D movements of components of the feeding apparatus in larvae and adults.
Data Availability Statement
The original contributions presented in the study are included in the article/supplementary material, further inquiries can be directed to the corresponding author/s.
Ethics Statement
The animal study was reviewed and approved by the ethical committee of Ovidius University of Constanţa, Danube Delta Biosphere Reserve Administration.
Author Contributions
NN prepared the concept for the manuscript, performed the experiments, digitized the films, calculated variables, provided tables and figures, and wrote the manuscript. KY digitized the films, provided tables and figures, and wrote the manuscript. ST prepared the concept for the manuscript, performed the experiments, calculated variables, provided tables and figures, and wrote the manuscript. TK performed the experiments, calculated variables, provided tables and figures, and wrote the manuscript. DD calculated variables, provided tables and figures, and wrote the manuscript. DC prepared the concept for the manuscript, provided tables and figures, and wrote the manuscript. All authors contributed to the article and approved the submitted version.
Funding
This work was supported by the project ANTREPRENORDOC, in the frame work of Human Resources Development Operational Program 2014–2020, financed from the European Social Fund under the contract number 36355/23.05.2019 HRD OP/380/6/13 – SMIS Code: 123847, the fund for support of publication at “Konstantin Preslavsky” University of Shumen, the Research Fund of the “Konstantin Preslavsky” University of Shumen (Grant Nos. RD-08-104/30.01.2020 and RD-08-67/25.01.2021).
Conflict of Interest
The authors declare that the research was conducted in the absence of any commercial or financial relationships that could be construed as a potential conflict of interest.
Acknowledgments
The comments of two reviewers contributed significantly to the improvement of the manuscript.
References
Aerts, P., van Damme, J., and Herrel, A. (2001). Intrinsic mechanics and control of fast cranio-cervical movements in aquatic feeding turtles. Amer. Zool. 41, 1299–1310. doi: 10.1093/icb/41.6.1299
Bels, V., and Whishaw, I. Q. (2019). Feeding in Vertebrates: Evolution, Morphology, Behavior, Biomechanics. Switzerland: Springer, doi: 10.1007/978-3-030-13739-7
Bernabò, I., and Brunelli, E. (2019). Comparative morphological analysis during larval development of three syntopic newt species (Urodela: Salamandridae). Eur. Zool. J. 86, 38–53. doi: 10.1080/24750263.2019.1568599
Blight, A. R. (1976). Undulatory swimming with and without waves of contraction. Nature 264, 352–354. doi: 10.1038/264352a0
Bramble, D. M., and Wake, D. B. (1985). “Feeding mechanisms of lower tetrapods,” in Functional Vertebrate Morphology, Vol. 13, eds M. Hildebrand, D. M. Bramble, K. F. Liem, and D. B. Wake (Cambridge, MA: Harvard University Press), 230–261.
Careddu, G., Carlini, N., Romano, A., Rossi, L., Calizza, E., Caputi, S., et al. (2020). Diet composition of the Italian crested newt (Triturus carnifex) in structurally different artificial ponds based on stomach contents and stable isotope analyses. Aquat. Conserv.: Mar. Freshw. Ecosyst. 30, 1505–1520. doi: 10.1002/aqc.3383
Carreño, C. A., and Nishikawa, K. C. (2010). Aquatic feeding in pipid frogs: the use of suction for prey capture. J. Exp. Biol. 213, 2001–2008. doi: 10.1242/jeb.043380
Catania, K. (2014). The shocking predatory strike of the electric eel. Science 346, 1231–1234. doi: 10.1126/science.1260807
Catania, K. (2019). The astonishing behavior of electric eels. Front. Integr. Neurosc. 13:23. doi: 10.3389/fnint.2019.00023
Cundall, D., Fernandez, E., and Irish, F. (2017). The suction mechanism of the pipid frog, Pipa pipa (Linnaeus, 1758). J. Morphol. 278, 1229–1240. doi: 10.1002/jmor.20707
Deban, S. M. (2003). “Constraint and convergence in the evolution of salamander feeding,” in Vertebrate Biomechanics and Evolution, eds J. Gasc, A. Casinos, and V. Bels (Oxford: BIOS Scientific Publishers), 163–180.
Deban, S. M., and Olson, W. M. (2002). Suction feeding by a tiny predatory tadpole. Nature 420, 41–42. doi: 10.1038/420041a
Deban, S. M., O’Reilly, J. C., and Nishikawa, K. C. (2001). The evolution of the motor control of feeding in Amphibians. Amer. Zool. 41, 1280–1298. doi: 10.1093/icb/41.6.1280
Deban, S. M., and Wake, D. B. (2000). “Aquatic feeding in salamanders,” in Feeding: Form, Function, and Evolution in Tetrapod Vertebrates, ed. K. Schwenk (San Diego, CA: Academic Press), 65–94.
Furtula, M., Todorović, B., Simić, V., and Ivanović, A. (2009). Interspecific differences in early life-history traits in crested newts (Triturus cristatus superspecies, Caudata, Salamandridae) from the Balkan Peninsula. J. Nat. Hist. 43, 469–477. doi: 10.1080/00222930802585794
Heiss, E., Aerts, P., and Van Wassenbergh, S. (2015). Flexibility is everything: prey capture throughout the seasonal habitat switches in the smooth newt Lissotriton vulgaris. Org. Divers. Evol. 15, 127–142. doi: 10.1007/s13127-014-0187-1
Heiss, E., Aerts, P., and Van Wassenbergh, S. (2018). Aquatic-terrestrial transitions of feeding systems in vertebrates: a mechanical perspective. J. Exp. Biol. 221:jeb154427. doi: 10.1242/jeb.154427
Heiss, E., and De Vylder, M. (2016). Dining dichotomy: aquatic and terrestrial prey capture behavior in the Himalayan newt Tylototriton verrucosus. Biol. Open 5, 1500–1507. doi: 10.1242/bio.020925
Heiss, E., Handschuh, S., Aerts, P., and Van Wassenbergh, S. (2016). Musculoskeletal architecture of the prey capture apparatus in salamandrid newts with multiphasic lifestyle: does anatomy change during the seasonal habitat switches? J. Anat. 228, 757–770. doi: 10.1111/joa.12445
Heiss, E., Handschuh, S., Aerts, P., and Van Wassenbergh, S. (2017). A tongue for all seasons: extreme phenotypic flexibility in salamandrid newts. Sci. Rep. 7:1006. doi: 10.1038/s41598-017-00674-y
Heiss, E., Aerts, P., and Van Wassenbergh, S. (2013a). Masters of change: seasonal plasticity in the prey-capture behavior of the Alpine newt Ichthyosaura alpestris (Salamandridae). J. Exp. Biol. 216, 4426–4434. doi: 10.1242/jeb.091991
Heiss, E., Natchev, N., Gumpenberger, M., Weissenbacher, A., and Van Wassenbergh, S. (2013b). Biomechanics and hydrodynamics of prey capture in the Chinese giant salamander reveal a high-performance jaw-powered suction feeding mechanism. J. R. Soc. Interface 10:20121028. doi: 10.1098/rsif.2012.1028
Heiss, E., Schwarz, D., and Konow, N. (2019). Chewing or not? Intraoral food processing in a salamandrid newt. J. Exp. Biol. 222:jeb189886. doi: 10.1242/jeb.189886
Higham, T. E. (2007). Feeding, fins and braking maneuvers: locomotion during prey capture in centrarchid fishes. J. Exp. Biol. 210, 107–117. doi: 10.1242/jeb.02634
Lauder, G. V. (1979). Feeding mechanisms in primitive teleosts and in the halecomorph fish Amia calva. J. Zool. 187, 543–578. doi: 10.1111/j.1469-7998.1979.tb03386.x
Lauder, G. V., and Reilly, S. M. (1988). Functional design of the feeding mechanism in salamanders: causal bases of ontogenetic changes in function. J. Exp. Biol. 134, 219–233.
Lauder, G. V., and Reilly, S. M. (1994). “Amphibian feeding behavior: comparative biomechanics and evolution,” in Biomechanics of Feeding in Vertebrates, eds V. Bels, M. Chardon, and P. Vandwalle (Berlin: Springer), 163–195. doi: 10.1007/978-3-642-57906-6_7
Lauder, G. V., and Shaffer, H. B. (1986). Functional design of the feeding mechanism in lower vertebrates: unidirectional and bidirectional flow systems in the tiger salamander. Zoo. J. Linn. Soc. 88, 277–290. doi: 10.1111/j.1096-3642.1986.tb01191.x
Lauder, G. V., and Shaffer, H. B. (1988). Ontogeny of functional design in tiger salamanders (Ambystoma tigrinum): are motor patterns conserved during major morphological transformations? J. Morphol. 197, 249–268. doi: 10.1002/jmor.1051970302
Lemell, P., Lemell, C., Snelderwaard, P., Gumpenberger, M., Wochesländer, R., and Weisgram, J. (2002). Feeding patterns of Chelus fimbriatus (Pleurodira: Chelidae). J. Exp. Biol. 205, 1495–1506.
Lemell, P., and Weisgram, J. (1997). Feeding patterns of Pelusios castaneus. Neth. J. Zool. 47, 429–441.
Lukanov, S., Tzankov, N., Handshuh, S., Heiss, E., Naumov, B., and Natchev, N. (2016). On the amphibious food uptake and prey manipulation behavior in the Balkan-Anatolian crested newt (Triturus ivanbureschi, Arntzen and Wielstra, 2013). Zoology 119, 224–231. doi: 10.1016/j.zool.2016.02.002
Mendes-Júnior, R. N. G., Sá-Oliveira, J. C., Vasconcelos, H. C. G., Costa-Campos, C. E., and Araújo, A. S. (2020). Feeding ecology of electric eel Electrophorus varii (Gymnotiformes: Gymnotidae) in the Curiaú River Basin, Eastern Amazon. Neotrop. Ichthyol. 18:e190132. doi: 10.1590/1982-0224-2019-0132
Michel, K. B., Heiss, E., Aerts, P., and Van Wassenbergh, S. (2015). A fish that uses its hydrodynamic tongue to feed on land. Proc. R. Soc. B. 282:20150057. doi: 10.1098/rspb.2015.0057
Montuelle, S. J., and Kane, E. A. (2019). “Food capture in vertebrates: a complex integrative performance of the cranial and postcranial systems,” in Feeding in Vertebrates, Fascinating Life Sciences, eds V. Bels and I. Q. Whishaw (Cham: Springer), 71–137. doi: 10.1007/978-3-030-13739-7_4
Muller, M., and Osse, J. W. M. (1984). Hydrodynamics of suction feeding in fish. Trans. Zool. Soc. Lond. 37, 51–135.
Neenan, J. M., Ruta, M., Clack, J. A., and Rayfield, E. J. (2014). Feeding biomechanics in Acanthostega and across the fish–tetrapod transition. Proc. R. Soc. B. 281:20132689. doi: 10.1098/rspb.2013.2689
O’Reilly, J. C., Deban, S. M., and Nishikawa, K. C. (2002). “Derived life history characteristics constrain the evolution of aquatic feeding behavior in adult amphibians,” in Topics in Functional and Ecological Vertebrate Morphology, eds P. Aerts, K. D’Aout, A. Herrel, and R. Van Damme (Maastricht: Shaker Publishing), 153–190.
Osse, J. W. M. (1985). “Jaw protrusion, an optimization of the feeding apparatus of teleosts?,” in Architecture in Living Structure, eds G. A. Zweers and P. Dullemeijer (Dordrecht: Springer), doi: 10.1007/978-94-009-5169-3_10
Parker, M. S. (1994). Feeding ecology of stream-dwelling pacific giant salamander larvae (Dicamptodon tenebrosus). Copeia 3, 705–718. doi: 10.2307/1447187
Reilly, S. M., and Lauder, G. V. (1992). Morphology, behavior, and evolution: comparative kinematics of aquatic feeding in salamanders. Brain Behav. Evol. 40, 182–196. doi: 10.1159/000113911
Sanderson, S. L., and Kupferberg, S. J. (1999). “Development and evolution of aquatic larval feeding mechanisms,” in The Origin and Evolution of Larval Forms, eds B. K. Hall and M. H. Wake (San Diego, CA: Acad. Press), 301–377. doi: 10.1016/B978-012730935-4/50011-0
Schwarz, D., Gorb, S. N., Kovalev, A., Konow, N., and Heiss, E. (2020a). Flexibility of intraoral food processing in the salamandrid newt Triturus carnifex: effects of environment and prey type. J. Exp. Biol. 223:jeb232868. doi: 10.1242/jeb.232868
Schwarz, D., Konow, N., Roba, Y. T., and Heiss, E. (2020b). A salamander that chews using complex, three-dimensional mandible movements. J. Exp. Biol. 223:jeb.220749. doi: 10.1242/jeb.220749
Schwarz, D., Konow, N., Porro, L. B., and Heiss, E. (2020c). Ontogenetic plasticity in cranial morphology is associated with a change in the food processing behavior in Alpine newts. Front. Zool. 17:34. doi: 10.1186/s12983-020-00373-x
Schwenk, K. (2000). “Tetrapod feeding in the context of vertebrate morphology,” in Feeding: Form, Function, and Evolution in Tetrapod Vertebrates, ed. K. Schwenk (San Diego, CA: Academic Press), 3–20.
Schwenk, K., and Rubega, M. (2005). “Diversity of vertebrate feeding systems,” in Physiological and Ecological Adaptations to Feeding in Vertebrates, eds J. M. Starck and T. Wang (New Hampshire: Science Publishers), 1–41.
Scott, W. B., and Tibbo, S. N. (1968). Food and feeding habits of Swordfish, Xiphias gladius, in the Western North Atlantic. J. Fish. Res. Bd. Canada 25, 903–919. doi: 10.1139/f68-084
Shimose, T., Shono, H., Yokawa, K., Saito, H., and Tachihara, K. (2006). Food and feeding habits of blue marlin, Makaira nigricans, around Yonaguni Island, southwestern Japan. Bull. Mar. Sci. 79, 761–775.
Stillwell, C. E., and Kohler, N. E. (1985). Food and feeding ecology of the swordfish Xiphias gladius in the western North Atlantic Ocean with estimates of daily ration. Mar. Ecol. Prog. Ser. 22, 239–247.
Stojanov, A., Tzankov, N., and Naumov, B. (2011). Die Amphiben und Reptilien Bulgariens. Frankfurt: Chimaira.
Van Wassenbergh, S., and Heiss, E. (2016). Phenotypic flexibility of gape anatomy fine-tunes the aquatic prey-capture system of newts. Sci. Rep. 6:29277. doi: 10.1038/srep29277
Wainwright, S. A. (1983). “To bend a fish,” in Fish Biomechanics, eds P. W. Webb and D. Weihs (New York, NY: Praeger), 68–91.
Wassersug, R. J., and Hoff, K. (1985). The kinematics of swimming in anuran larvae. J. Exp. Biol. 119, 1–30.
Westneat, M. W., Thorsen, D. H., Walker, J. A., and Hale, M. E. (2004). Structure, function, and neural control of pectoral fins in fishes. IEEE J. Ocean. Eng. 29, 674–683. doi: 10.1109/JOE.2004.833207
Yordanova, K., Lukanov, S., and Natchev, N. (2017). “Preliminary data indicate hard-wired coordination between locomotor and feeding systems in the Marsh frog (Pelophylax ridibundus Pallas, 1771) when catching prey on the water surface,” in Proceedings of the 5th Student Scientific Conference “Ecology and Environment”, (Shumen: Konstantin Preslavsky University Press), 30–31.
Keywords: locomotion, feeding, coordination, larvae, water-land transition, urodela
Citation: Natchev N, Yordanova K, Topliceanu S, Koynova T, Doichev D and Cogălniceanu D (2021) Ontogenetic Changes of the Aquatic Food Uptake Mode in the Danube Crested Newt (Triturus dobrogicus Kiritzescu 1903). Front. Ecol. Evol. 9:641657. doi: 10.3389/fevo.2021.641657
Received: 06 January 2021; Accepted: 15 March 2021;
Published: 09 April 2021.
Edited by:
Julia L. Molnar, New York Institute of Technology, United StatesReviewed by:
Anthony Herrel, Muséum National d’Histoire Naturelle, FranceAna Ivanović, University of Belgrade, Serbia
Copyright © 2021 Natchev, Yordanova, Topliceanu, Koynova, Doichev and Cogălniceanu. This is an open-access article distributed under the terms of the Creative Commons Attribution License (CC BY). The use, distribution or reproduction in other forums is permitted, provided the original author(s) and the copyright owner(s) are credited and that the original publication in this journal is cited, in accordance with accepted academic practice. No use, distribution or reproduction is permitted which does not comply with these terms.
*Correspondence: Nikolay Natchev, bmF0Y2hldkBzaHUuYmc=