- Department of Earth Sciences, University of Oxford, Oxford, United Kingdom
Terrestrial ecosystems during the Pennsylvanian (late Carboniferous) and Cisuralian (early Permian) are usually described in the literature as being dominated by synapsids, the mammal-line amniotes. The pelycosaurs (a paraphyletic grouping of synapsid families) have been considered more speciose, abundant, and ecologically diverse than contemporary reptile-line amniotes. However, this dominance has never been subjected to quantitative testing accounting for sampling bias. Moreover, in recent years the amniote phylogeny has undergone numerous revisions, with suggestions that varanopids and recumbirostran microsaurs fall within reptiles, and that diadectomorphs may be pelycosaurian-grade synapsids. An examination of local species richness (alpha diversity) of synapsids and reptiles during the Pennsylvanian and Cisuralian at different spatial scales shows that these taxonomic revisions have substantial impacts on relative diversity patterns of synapsids and reptiles. Synapsids are only found to be consistently more diverse through the early Permian when using the “traditional” taxonomy. The recent taxonomic updates produce diversity estimates where reptile diversity is consistent with, or in some cases higher than that of synapsids. Moreover, biases in preservation may affect patterns. Where preservation favors smaller vertebrates, e.g., Richards Spur, South Grandfield, reptiles overwhelmingly dominate. If smaller vertebrates are expected to make up the bulk of amniote diversity, as they do in the present day, such lagerstätten may be more representative of true diversity patterns. Therefore, the dominance of pelycosaurs during this interval should be reconsidered, and this interval may be considered the First Age of Reptiles.
Introduction
Amniotes, the vertebrates with the amniotic egg that gives their reproduction complete independence from water, first appear in the fossil record about 315 million years ago (Carroll, 1964) and rapidly diversified into a great diversity of species and ecologies (Sahney et al., 2010; Dunne et al., 2018) that is seen in the present day. This earliest record at Joggins contains representatives of both lineages from the basal divergence of amniotes into Synapsida (mammal-line amniotes) and Reptilia, or Sauropsida (the lineage which produced reptiles and birds; hereafter referred to as Reptilia or reptiles) (Carroll, 1964; Reisz, 1972; Müller and Reisz, 2006), and estimates of divergence times based on molecular data and tip dating analyses indicate the split occurred between 315 and 330 million years ago (e.g., Crottini et al., 2012; Dos Reis et al., 2015; Laurin et al., 2018; Marjanović, 2019; Ford and Benson, 2020), with a median estimate of 318 derived from the TimeTree database1.
The earliest diversification of amniotes during the Pennsylvanian (late Carboniferous) and Cisuralian (early Permian) are characterized by faunas that are generally considered synapsed-dominated. During this interval, a paraphyletic grouping of basally diverging synapsed families known as pelycosaurs (non-therapsid synapsids) have been described in the published literature since 1940 as dominating in terms of abundance, species richness and morphological diversity (e.g., Romer and Price, 1940; Kemp, 2006; Sahney et al., 2010; Benton, 2012; Brocklehurst et al., 2013; Brocklehurst and Fröbisch, 2018a; Mann and Reisz, 2020) with reptiles being considered the less diverse lineage until the radiation of archosauromorphs in the Mesozoic (Bakker, 1977; Benton et al., 2004; Ezcurra and Butler, 2018). By the end of the Carboniferous, synapsids are suggested to have outnumbered reptiles approximately two to one, in terms of both raw taxonomic counts and lineage counts from timescaled phylogenies (Reisz, 2003; Modesto et al., 2015).
The idea of pelycosaur dominance during the Pennsylvanian and Cisuralian is so prevalent in the literature, to the extent that this interval in earth’s history has sometimes been referred to in the popular literature as “The First Age of Mammals” (e.g., Panciroli, 2021), that it is easy to forget that there has been very little assessment of this pattern. There have been no quantitative examinations of the global amniote record from this time comparing the diversities of reptiles and synapsids while accounting for sampling and preservation bias. It has been recently suggested that this pattern may be a due to biases in either preservation or collection: larger synapsids may be either easier to find or preserve than smaller, more fragmentary reptiles (Modesto et al., 2015). The reptiles named from the Carboniferous are smaller, but more complete than the synapsids, potentially indicating only the most complete reptiles are collected or described, whereas larger synapsed material may be considered informative even when more fragmentary (Modesto et al., 2015). An alternative suggestion was, however, provided in this same study: that the signal was genuine and reflected the earlier adoption of herbivory in synapsids and their radiation into this new niche (Modesto et al., 2015). Mann and Reisz (2020) alternatively suggested that elongation of neural spines, a trait limited to synapsids until the Mesozoic, may have contributed to the success of synapsids relative to reptiles, whether as a thermoregulatory device giving a metabolic advantage or as a mode of intraspecific recognition aiding reproductive success.
The question of synapsed dominance during the Pennsylvanian and Cisuralian is complicated by the recent revisions to early amniote phylogeny, calling into question which clades should be assigned to each of the two lineages. What might be called the “conventional wisdom” of basal amniote divergences had, before 2017, remained relatively stable since the 1990s (Laurin and Reisz, 1995; Laurin and Reisz, 1997), stabilizing on the relationships established in early studies incorporating cladistic principles and parsimony analyses (Benton, 1985; Gauthier et al., 1988). Early phylogenetic studies cemented the basal dichotomy between synapsids and reptiles and the assignment of individual clades to one or the other of these two lineages remained consistent until the mid-2010s (Figure 1A). There has been debate surrounding the relationships within the two lineages (e.g., Müller and Reisz, 2006; Benson, 2012; Brocklehurst et al., 2016; Laurin and Piñeiro, 2017; MacDougall et al., 2018) and individual species have sometimes been moved between synapsids and reptiles (e.g., Reisz and Modesto, 2007; Reisz et al., 2010; Ford and Benson, 2019; Mann et al., 2020), but on the whole the clade, Synapsida or Reptilia, to which the Pennsylvanian and Cisuralian lineages have been assigned has been consistent. It should be noted that, in the interval between the early studies of the 1980s and 1990s and more recent analyses described below, there have very few analyses incorporating broad sampling of both synapsids and reptiles; large-scale analyses have tested relationships within synapsids, diapsids or parareptiles, with inclusion of clades within those groups assumed along the lines established in the early studies. In recent years, however, there have been several more inclusive analyses that have led to several revisions to this “conventional wisdom.”
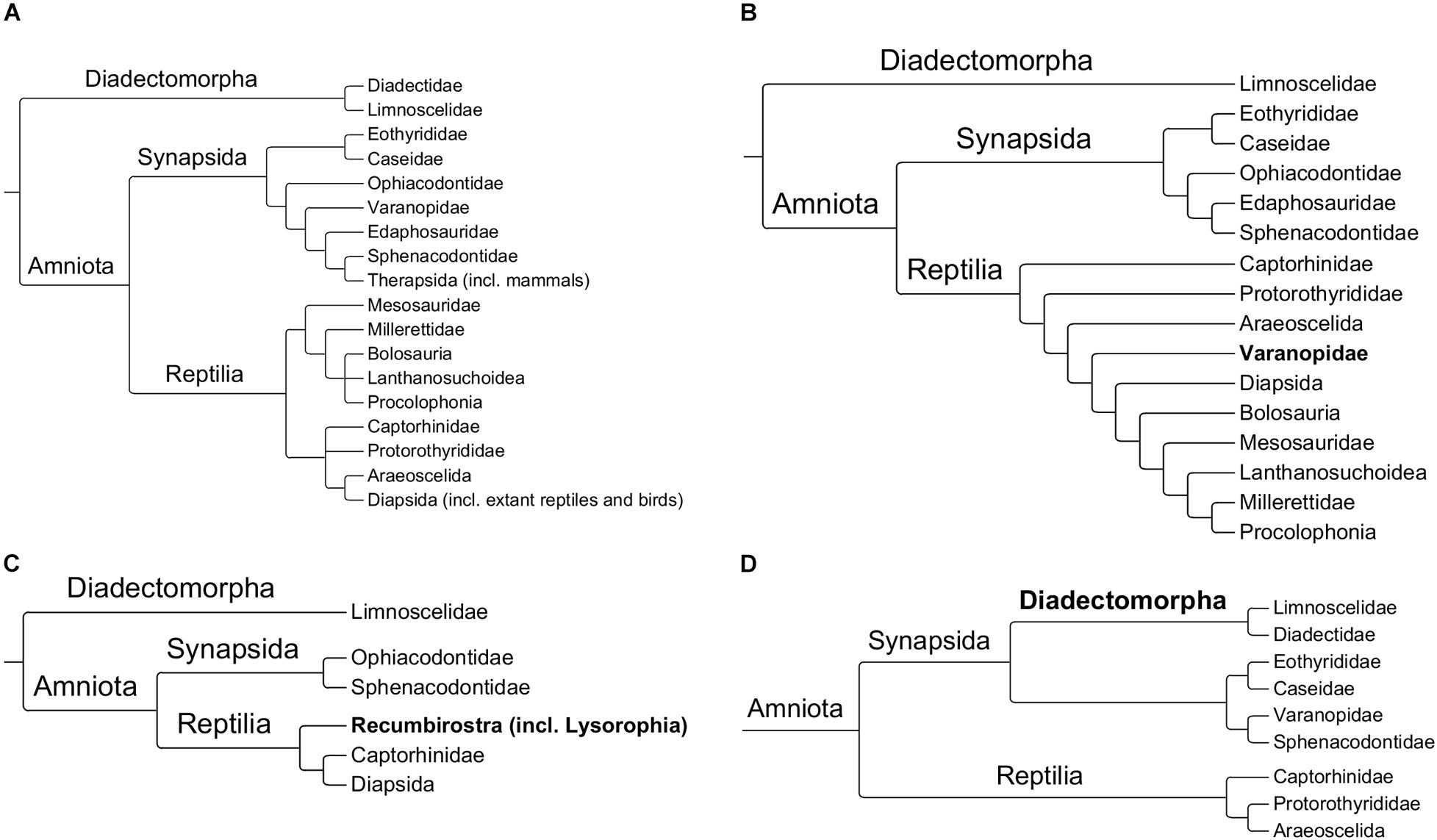
Figure 1. Summaries of four hypotheses of early amniote relationships. (A) Pre-2017 relationships (based on Müller and Reisz, 2006; MacDougall and Reisz, 2014; Brocklehurst and Fröbisch, 2018a; Brocklehurst et al., 2018a); (B) Varanopids within reptiles (based on Ford and Benson, 2020); (C) Recumbirostrans and lysorophians within reptiles (based on Pardo et al., 2017); (D) Diadectomorphs within synapsids (based on Klembara et al., 2020).
The analysis of Pardo et al. (2017) suggested several novel relationships within early tetrapods, including rendering the lepospondyl amphibians polyphyletic. This analysis found Recumbirostra and Lysorophia, two lineages normally included within lepospondyls (Ruta et al., 2003; Ruta and Coates, 2007; Marjanović and Laurin, 2019; Klembara et al., 2020), to belong to Reptilia (Figure 1C). The assignment of recumbirostrans to reptiles is not without precedent; early studies of microsaurs (the group to which recumbirostrans are usually assigned) debated whether they should be considered amphibians or reptiles (Romer, 1950; Vaughn, 1962; Gregory, 1965), but Pardo et al. (2017) represents the first quantitative cladistic analysis supporting such a relationship.
Ford and Benson (2019), in a re-examination of the early reptile Orovenator, found that adding this taxon to an existing character/taxon matrix (Reisz et al., 2010) had the effect of moving the entire family Varanopidae, normally assigned to the synapsids (Romer and Price, 1940; Reisz et al., 2010) into the reptiles. This new position for Varanopidae received further support when using a new character/taxon matrix with a broader sampling of early synapsids and reptiles (Ford and Benson, 2020; Figure 1B). The unconventional position of varanopids outside of synapsids had already been hinted at in an analysis by MacDougall et al. (2018) but received little attention. Their analysis was focused on parareptiles, but included several putative synapsed outgroups to test the impact of characters relating to temporal fenestration. The two varanopid taxa included did not form a clade with the other synapsids, but instead formed a polytomy at the base of Reptilia. This novel position for varanopids has been suggested to indicate greater diversity of reptiles in the early Permian than was previously recognized (Ford and Benson, 2020).
A final point of contention is the position of Diadectomorpha. Unlike the clades discussed in the previous paragraphs, their position does not represent a recent overturning of established relationships, but rather a persistent point of contention over the last 20 years. Diadectomorphs have usually been considered to be the sister to the amniotes (Panchen and Smithson, 1988; Laurin and Reisz, 1997, 1999; Pardo et al., 2017), and are often used as specified outgroups in analyses of amniotes (e.g., Müller and Reisz, 2006; Benson, 2012). However, there have been intermittent phylogenetic analyses that have recovered them within the synapsids (Berman, 2000, 2013; Marjanović and Laurin, 2019; Klembara et al., 2020; Figure 1D). Berman (2013), in particular, provided an extensive list of characteristics uniting diadectomorphs with synapsids, although their cladistic analysis relied on many tips coded above the genus level (Synapsida itself was coded as a single tip taxon).
These new relationships, if borne out by future study, have the potential to overturn many of our assumptions on early amniote evolution. In this study, we show how incorporating the novel positions suggested for varanopids, recumbirostrans and lysorophians into analyses of early amniote diversity (species richness) produces estimates of reptile diversity more consistent with those of synapsids, challenging the assumption that synapsids dominated during the Pennsylvanian and Cisuralian. Moreover, consideration of the preservation biases and the effect of lagerstätten suggest that any apparent dominance of synapsids during this time may well be artifactual.
Materials and Methods
Dataset
A dataset was formulated containing occurrences within each formation of amniotes, diadectomorphs, microsaurs and lysorophians, occurring between their origin in the Carboniferous and the end of the Cisuralian. The data was derived primarily from the Paleobiology Database (PBDB;2) and supplemented from the published literature and observations from museum collections. The PBDB data was downloaded in July 2020, and so taxa published after this were not added to the dataset.
Analysis of Diversity Through Time
The interval of time under study was divided into time bins, using the Formation Binning approach (Dean et al., 2020). This approach uses the top and bottom ages of formations to assess the most suitable position for the interval boundaries, rather than using bins from the international commission of stratigraphy that are largely based on marine faunal turnover. This method was implemented in R v3.6.1 (R Core Team, 2017) using the functions provided by Dean et al. (2020).
Due to recent studies suggesting that global estimates of diversity are more representative of spatial patterns in sampling than genuine patterns of species richness (Close et al., 2020a, b), this study will focus on more local (alpha) diversity patterns. Within each time bin, the formations were grouped into bioregions. These are areas of endemicity, defined by the taxa present in each, and so their boundaries better represent barriers to dispersal instead of arbitrarily defined boundaries based on geography, geology or sampling e.g., continents, basins, localities (Brocklehurst and Fröbisch, 2018b). The bioregions were defined with the approach of Brocklehurst and Fröbisch (2018b), where formations within each time bin are grouped using two cluster analyses, one based on taxonomic distances, and one based on geographic distances. Clusters shared between the two analyses represent bioregions. This approach allows diversity to be assessed at different spatial scales, defined by the geographic cluster node height, since patterns of diversity will vary at different scales (Palmer and White, 1994; Rosenweig, 1995; Whittaker et al., 2001; Field et al., 2009; Keil et al., 2012; Brocklehurst and Fröbisch, 2018b; Brocklehurst et al., 2018b).
In this study, taxonomic distances between formation were calculated using the modified Forbes metric (Alroy, 2015), computed in R using the script provided in Brocklehurst et al. (2018a). Geographic distances were based on palaeocoordinates derived from the PBDB and calculated using functions in the R package letsR (Vilela and Villalobos, 2015). Diversity within each time bin was analyzed at two spatial scales: a local scale (formations within 100 km of each other united into their bioregions) and a regional scale (formations within 1,000 km of each other united into their bioregions). In the former, the boundaries between bioregions will be more reflective of local environmental/habitat variability, while in the latter geophysical boundaries will be of greater importance.
Within each bioregion, diversity was assessed using shareholder quorum subsampling (SQS) (Alroy, 2010; Chao and Jost, 2012). This approach accounts for sampling heterogeneity by subsampling occurrences to fixed levels of coverage, rather than sample size as in rarefaction approaches. The analysis was carried out in R using functions from the package iNEXT (Hsieh et al., 2016). A coverage quorum of 0.9 was applied, following research suggesting diversity estimates below this coverage become imprecise (Brocklehurst et al., 2018a; Close et al., 2018).
As far as possible, taxa were defined to the species level, but attempts were made to include occurrences that could not be assigned to that taxonomic resolution. If an occurrence was assigned to a higher taxon, and no occurrences of species within that higher taxon are known from that bioregion, then the higher taxon was considered a unique species within that bioregion.
The above procedures were carried out on eight different taxonomic schemes (Table 1), representing different combinations of taxonomic assignments of the disputed clades (varanopids, diadectomorphs, recumbirostrans and lysorophians. Note that while the dataset contains a broader sampling of microsaurs, only recumbirostrans and lysorophians are tested within reptiles; others are included in the dataset to aid future analysis in case of further revisions to microsaurs (see “Discussion”). Within each bioregion, all taxa were assigned to either Reptilia or Synapsida based on the taxonomic scheme in use, and the species richness of each within the bioregion was calculated.
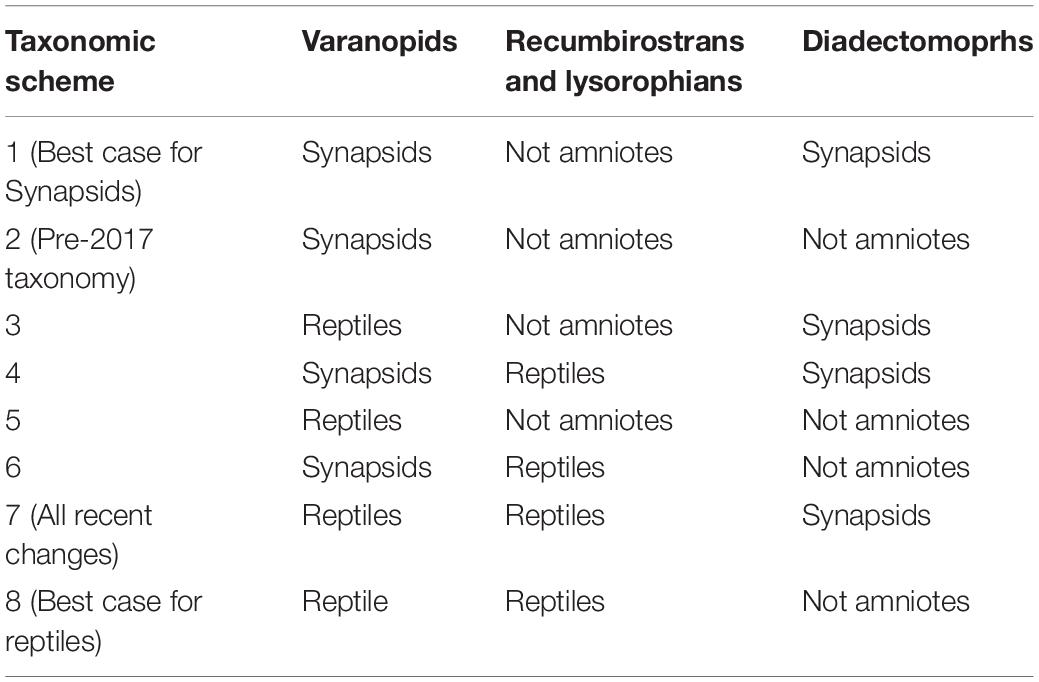
Table 1. The eight taxonomic schemes used when calculating reptile and synapsed diversity, indicating to which clade the disputed lineages are assigned.
Analysis of Diversity in Richards Spur and South Grandfield
Richards Spur is an Artinskian aged (Woodhead et al., 2010) locality in Oklahoma representing the most diverse tetrapod-bearing locality currently known from the early Permian, with more than 30 taxa currently described (MacDougall et al., 2016). The site represents a cave deposit, with clay and mudstone filling fissures in limestone (Sullivan et al., 2000). Being a cave deposit, unlike many of the lower Permian formations in North America, the mode of preservation is more favorable toward smaller taxa (Muñoz-Durán and Van Valkenburgh, 2006; Jass and George, 2010; Brown et al., 2019). Richards Spur was therefore analyzed separately, to provide a comparison of the diversity of reptiles and synapsids at small sizes.
Diversity of reptiles and synapsids within Richards Spur was again calculated using SQS. However, as a single locality is being analyzed, specimens were subsampled rather than occurrences. Abundance data was drawn from the PBDB and the published literature (Supplementary Data 3). Diversity was calculated at a range of coverage quora, at intervals of 0.05 between 0.4 and 0.95, to examine the impact of varying sampling intensity. Also, due to concerns surrounding the performance of SQS when abundance distributions are highly uneven (Close et al., 2018; Alroy, 2020; see “Discussion”), a second method more robust to these issues was used to test the results: Squares (Alroy, 2018).
Richards Spur contains numerous varanopid species, but only four recumbirostrans and some fragmentary unnamed diadectid specimens (Reisz and Sutherland, 2001). It was therefore decided not to be worth calculating diversity with all eight taxonomic schemes, but to test only a “best case for synapsids” (the varanopids and diadectid specimens included within synapsids and recumbirostrans not included), and a “best case for reptiles (both varanopids and recumbirostrans included within reptiles).
South Grandfield is another well-sampled Cisuralian locality (Kungurian age) containing a diverse array of tetrapods, including good preservation of several small taxa (Daly, 1973). Although the small number of species and large number of singletons (at least when recumbirostrans are not included) makes this locality unsuitable for examination via the species accumulation curves using SQS, squares was used to examine the diversity of reptiles and synapsids within the two taxonomic schemes, again using abundance data drawn from the PBDB and literature (Supplementary Data 4).
Results
Diversity Through Time
While altering the taxonomic scheme employed varies the relative diversities of synapsids and reptiles, the overall trends of species richness remain consistent (Figures 2, 3). The earliest interval where sampling is sufficient to assess diversity of both reptiles and synapsids is the Moscovian (Pennsylvanian). Median synapsed diversity is stable through the latter stages of the Carboniferous, before rising gradually to a late Artinskian peak, visible when examining diversity at local scales (at regional scales the late Artinskian does not have the spatial extent of sampling to examine diversity in this time bin). Median reptile diversity also remains relatively stable in the Pennsylvanian, before rising to an early Artinskian peak, visible at both local and regional scales. During the late Kungurian, synapsed diversity at both local and regional scales falls sharply. At local scales reptile diversity remains stable, but a decline is observed in their regional diversity.
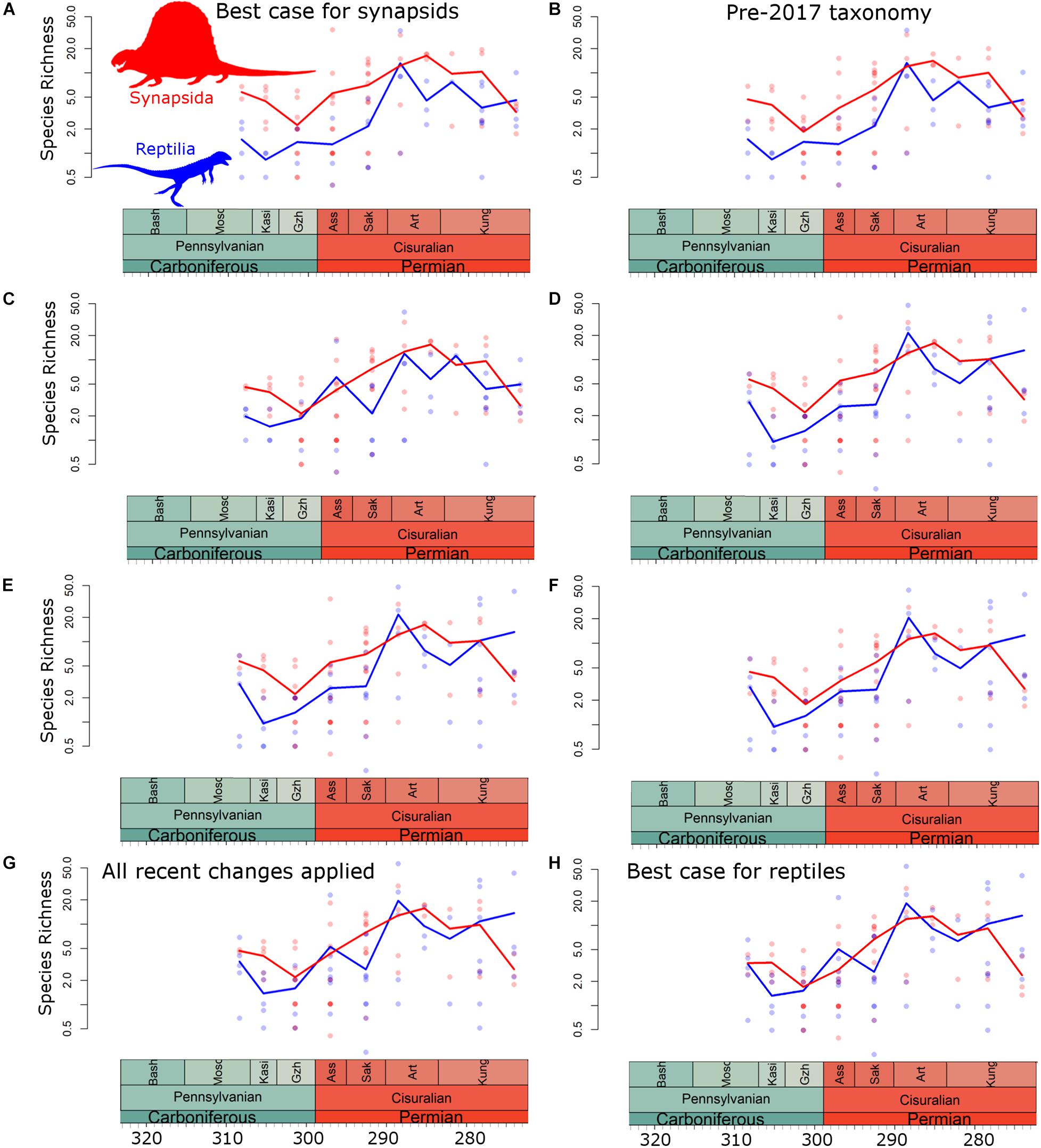
Figure 2. Diversity estimates at the local scale. Points represent diversity within each bioregion (Blue = reptiles; Red = synapsids). Curve is median diversity within each time bin. (A) Taxonomic scheme 1; (B) Taxonomic scheme 2; (C) Taxonomic scheme 3; (D) Taxonomic scheme 4; (E) Taxonomic scheme 5; (F) Taxonomic scheme 6; (G) Taxonomic scheme 7; (H) Taxonomic scheme 8.
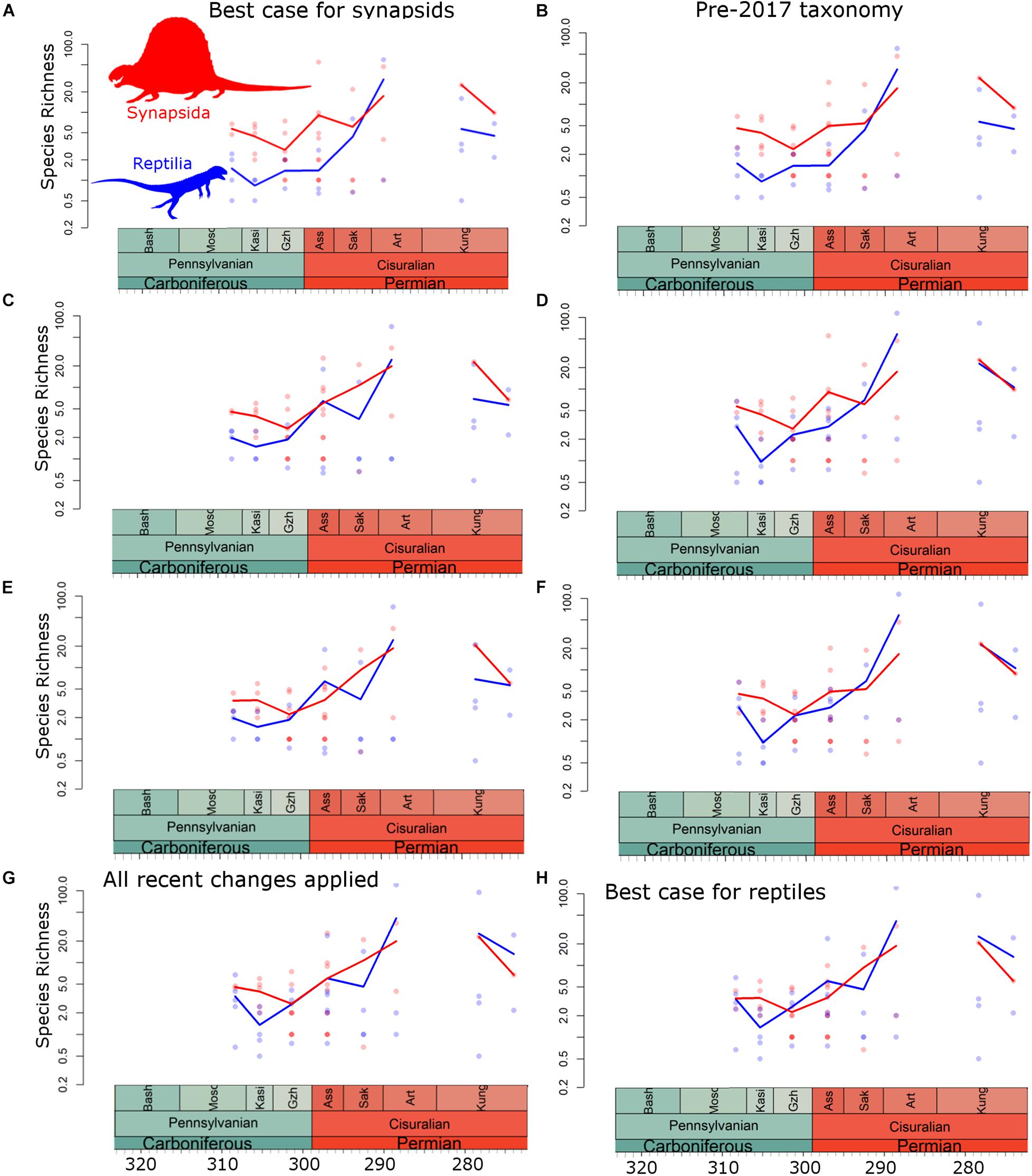
Figure 3. Diversity estimates at the regional scale. Points represent diversity within each bioregion (Blue = reptiles; Red = synapsids). Curve is median diversity within each time bin. (A) Taxonomic scheme 1; (B) Taxonomic scheme 2; (C) Taxonomic scheme 3; (D) Taxonomic scheme 4; (E) Taxonomic scheme 5; (F) Taxonomic scheme 6; (G) Taxonomic scheme 7; (H) Taxonomic scheme 8.
When using taxonomic schemes 1 (“best case for synapsids”) and 2 (pre-2017 taxonomy), synapsids are found to be more diverse than reptiles throughout much of Pennsylvanian and Cisuralian, with higher median diversity at both local and regional scales (Figures 2A,B, 3A,B). There are only two time bins where median reptile diversity exceeds that of synapsids when using taxonomic schemes 1 and 2: the early Artinskian and, at local but not regional scales, the latest Kungurian. As more of the recent taxonomic revisions are incorporated, however, median reptile diversity is pushed closer to that of synapsids (Figures 2C–G, 3C–G). In taxonomic schemes 7 (all recent taxonomic changes applied) and 8 (“best case for reptiles”), median diversities of reptiles and synapsids are consistent throughout the Pennsylvanian and Cisuralian (Figures 2G,H, 3G,H). In fact, at local scales during the latest Kungurian, reptile diversity substantially exceeds that of synapsids when using these taxonomic schemes (Figures 2G,H).
Diversity Within Richards Spur and South Grandfield
When using the taxonomic scheme most favorable to synapsids, at low levels of coverage synapsed diversity at Richards Spur substantially exceeds that of reptiles (Figure 4A). However, at higher levels of coverage (above 0.75), reptile diversity is found to be higher (Figure 4A). When using the taxonomic scheme most favorable to reptiles, reptile diversity in Richards Spur is found to exceed that of synapsids at all levels of coverage (Figure 4B). In both taxonomic schemes, analysis using squares produced higher diversity of reptiles than synapsids at Richards Spur. When using the best case for synapsids, synapsed diversity calculated with squares is 8, reptile diversity 17.84. When using the best case for reptiles, synapsed diversity calculated with squares is 3, reptile diversity 26.31. At South Grandfield, analysis using squares also produced higher diversity of reptiles than synapsids regardless of the taxonomic scheme. When using the worst case for reptiles, reptile diversity calculated using squares is 6.39; when using the best case for reptiles it is 10.61. Synapsid diversity using both schemes is 2.71.
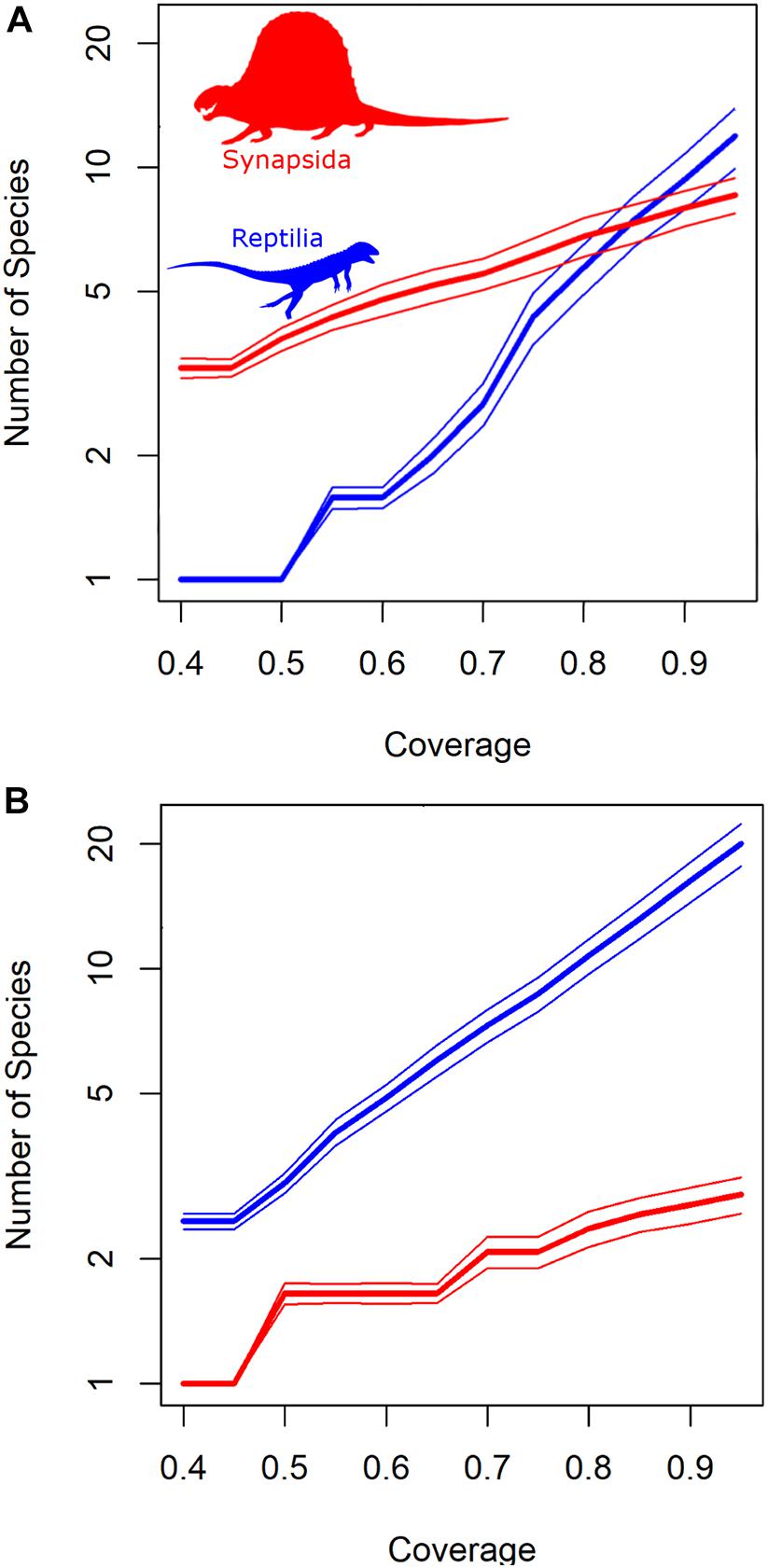
Figure 4. Diversity of reptiles and synapsids within Richards Spur. Thick lines represent diversity estimate of reptiles (blue) and synapsids (red) when subsampling to a particular coverage. (A) Taxonomic scheme 1; (B) Taxonomic scheme 8.
Discussion
Global Diversity Patterns of Synapsids and Reptiles
The recent revisions of amniote phylogeny will doubtless be subject to further debate and modification, and there may well be other clades whose position will come under scrutiny. It is therefore not worth discussing at this point in time which of the global curves of diversity through time is most likely to represent the true signal. It is more useful to examine general patterns across the different datasets, which will indicate what we are still able to say with confidence, and what work is most important in the future.
First, the relative diversity patterns during the latest Carboniferous and earliest Cisuralian are most strongly affected by the assignment of the varanopids to either synapsids or reptiles. The Asselian stage documents a substantial increase in varanopid diversity in North America, particularly west of the Hueco seaway. This shallow inland seaway separated the formations in Texas and Oklahoma from those in New Mexico, Colorado, Arizona, and Utah (Lucas et al., 2011), and there are noticeable differences in the faunas on either side (Brocklehurst and Fröbisch, 2018b; Brocklehurst et al., 2018b). The Cutler Group of New Mexico has a considerable diversity of varanopids, including Ruthiromia (Eberth and Brinkman, 1983), two species of Aerosaurus (Romer, 1937; Langston and Reisz, 1981), and potentially Nitosaurus [(Romer, 1937), although this taxon’s affinity and validity is uncertain (Reisz, 1986)]. There is also an indeterminate varanopid in the Placerville localities of Colorado (Lewis and Vaughn, 1965; Brocklehurst et al., 2016). Their assignment to reptiles pushes median reptile diversity during the Gzhelian and Asselian close to that of synapsids and alters the timing and rapidity of the reptile radiation (although note Maddin et al. (2020) discuss how ontogenetic changes in varanopids may lead to taxonomic oversplitting). When varanopids are assigned to Synapsida, reptile diversity remains low in the initial stages of the Cisurialian, and the rise to their Artinskian peak is rapid (Figures 2A,B,D,F, 3A,B,D,F). When they are assigned to Reptilia, reptile diversity rises gradually through the early stages if the Cisuralian (Figures 2C,E,G,H, 3C,E,G,H).
The global diversity estimates also provide an interesting perspective on the latest Cisuralian amniote diversity. The late Kungurian and early Roadian were intervals of substantial change in tetrapod faunas, with a decline in the pelycosaurs and amphibians that had thrived during the early Permian, and a radiation of therapsid synapsids and procolophonian parareptiles (Kemp, 2006; Benson and Upchurch, 2013; Brocklehurst et al., 2013, 2017). Substantial ecological shifts also occurred, with the establishment of herbivore-dominated ecosystems and increasingly complex food webs with more trophic levels (Olson, 1966), as well as increases in faunal provinciality (Brocklehurst and Fröbisch, 2018b). This transition has been suggested to have been accompanied by a mass extinction, dubbed Olson’s Extinction (Sahney and Benton, 2008). Substantial debate does surround this event, it having been argued to be an artifact of spatial patterns of sampling (Benson and Upchurch, 2013), incorrect dating of formations (Lucas, 2017, 2018), and inappropriate time-binning methods (Lucas, 2017). However, thorough study of these issues still supports the extinction as a genuine event (Brocklehurst et al., 2017; Brocklehurst, 2018, 2020).
All diversity curves presented here indicate a substantial decrease in synapsed diversity during the latest Kungurian (Figures 2, 3). However, the pattern in reptiles is more complex and the signal varies depending on the spatial scale examined. At the local scale, reptile diversity remains stable between the middle and late Kungurian (Figure 2), whereas at regional scales there is a decrease (Figure 3). This pattern would indicate a more cosmopolitan reptile fauna developing during the late Kungurian relative to that of synapsids. When amniote-bearing localities are combined into larger bioregions, the number of synapsed species in each region increases, indicating different faunas are being combined. This is not the case in reptiles, potentially indicating a broader range of environmental tolerances; different local-scale bioregions have similar taxa present. A similar conclusion has been drawn from event-based biogeography analysis (Brocklehurst et al., 2018b).
While ichnotaxa are not considered in this analysis, the tetrapod footprint record provides support for decreasing diversity of synapsids during the Kungurian, and their being superseded in abundance by the reptiles. The Kungurian stage is roughly contemporary with the Erpetopus ichno-biochron (Voigt and Lucas, 2018). This biochron is characterized by a diverse array of trackways usually assigned to reptilian taxa, in particular Erpetopus, Varanopus and Hyloidichnus, while the Dimetropus tracks abundant in lower stratigraphic levels and usually associated with pelycosaurian grade-synapsids are considerably rarer (Haubold and Lucas, 2003; Voigt and Lucas, 2018; Marchetti et al., 2019). The footprint record provides a less spatially restricted sample that the body-fossil record, providing data on this reptilian diversification in North America, Europe and North Africa, in which reptile tracks make up between 50 and 100% of ichnoassociations (Marchetti et al., 2019).
Apparent reptile diversity during Olson’s Extinction is particularly influenced by the exclusion or inclusion of recumbirostrans and lysorophians. At local scales it is this which determines whether late Kungurian reptile diversity is similar to that of synapsids or substantially higher. At regional scales it determines whether reptile diversity is substantially lower than that of synapsids or is more similar. These two lineages are among the most diverse and abundant taxa in the Clear Fork group and to a lesser extent the Hennessey Formation, the set of formations that most clearly documents Olson’s extinction (see Supplementary Data 1). In fact, all lineages previously assigned to microsaurs show an increase in abundance and richness at this time, underscoring the necessity for further taxonomic revision of this group. Phylogenetic analysis and anatomical revisions of taxa are increasingly indicating that “Microsauria” is a polyphyletic assemblage of taxa, with many showing similarities in morphology with amniotes (e.g., Szostakiwskyj et al., 2015; Pardo et al., 2017; Mann et al., 2019, 2020; Marjanović and Laurin, 2019). A recent example is Asaphestera from Joggins (Bashkirian age) previously assigned to tuditanid microsaurs, but recently found to be a chimera of which part was reassigned to Synapsida, making it the earliest known member of that lineage (Mann et al., 2020).
While there will doubtless be further debate regarding the taxonomy of amniotes, and which lineages should be assigned to reptiles or synapsids, what is apparent from these analyses is that many of the recent taxonomic revisions are pushing the diversity of reptiles closer to that of synapsids during the Pennsylvanian and Cisuralian. Even adding diadectomorphs to synapsed diversity does not substantially increase the median diversity in most time bins. When using a taxonomy incorporating all recent revisions, including the addition of diadectomorphs to synapsids, there is little difference in median diversity of reptiles and synapsids throughout the Pennsylvanian and Cisuralian (Figures 2G, 3G). If these recent revisions are further supported by future research, the apparent dominance of pelycosaurs during the Cisuralian may have to be reconsidered.
Richards Spur, South Grandfield, and the Importance of Lagerstätten
The majority of the data on Cisuralian tetrapods comes from North America, in particular the Texas Red Beds (Romer and Price, 1940; Kemp, 2006; Benson and Upchurch, 2013; Brocklehurst et al., 2013). These formations are heavily biased in their mode of preservation toward larger taxa (Behrensmeyer, 1988), a bias that will favor the preservation of synapsids over reptiles. While synapsids reached large sizes early in their evolution, with multiple lineages independently evolving sizes of greater than 40 kg during the Carboniferous (Reisz and Fröbisch, 2014; Brocklehurst and Brink, 2017; Brocklehurst and Fröbisch, 2018a), reptiles did not reach such sizes until the latest Cisuralian with the evolution of Moradisaurinae (Brocklehurst, 2016).
The mode of preservation in the cave deposits at Richards Spur provides a unique taphonomic window into the small vertebrates present during the Cisuralian (MacDougall et al., 2016). Richards Spur contains a considerably more diverse reptile fauna than elsewhere in the Cisuralian and is partially responsible for the early Artinskian peak in reptile diversity observed in the global analysis. There is an unparalleled diversity and abundance of captorhinids (deBraga et al., 2019), with the genus Captorhinus being represented by hundreds of specimens (Richards, 2016). There is also a substantial parareptile diversity (MacDougall et al., 2016), including lineages not found elsewhere until the middle Permian (MacDougall and Reisz, 2014).
Richards Spur’s unusual combination of taxa and different relative abundances of species compared to contemporary localities has been explained by it being an upland locality, while most early Permian formations represent lowland fluival/lacustrine environments (Sullivan et al., 2000). However, there are two key issues that make Richards Spur an extremely important data point. First, lagerstätten (areas of exceptional preservation) frequently preserve assemblages of taxa atypical of non-lagerstätten formations (Walker et al., 2020). This is likely due to the unique modes of preservation in such localities, that often selectively preserve small delicate taxa that are usually easily destroyed by taphonomic processes, but in the environments that characterize many lagerstätten are easier to bury rapidly than larger taxa (Brocklehurst et al., 2012; Dean et al., 2016; Brown et al., 2019). Selective preservation of smaller taxa is characteristic of cave deposits (Muñoz-Durán and Van Valkenburgh, 2006; Jass and George, 2010; Brown et al., 2019) like Richards Spur.
The second point that must be remembered is that, in modern ecosystems, most species are small. The greater species richness of small animals is observed across a wide range of taxa, both vertebrate and invertebrate (e.g., May, 1978; Brown and Nicoletto, 1991; McClain, 2004), and is underpinned by theoretical considerations (Hutchinson and MacArthur, 1959), so there is no reason to expect that different patterns were prevalent in Paleozoic ecosystems. Therefore, the differences observed between the faunas preserved in lagerstätten and those in other formations should not be interpreted as the lagerstätten being aberrant. Instead, formations that selectively preserve smaller taxa should be considered more representative of the true diversity patterns in an ecosystem.
Thus, Richards Spur, instead of representing an aberrant fauna, could represent a crucial insight into the organisms that would be expected to make up the majority of the early Permian fauna, but are less well-sampled in the majority of available localities due to the size-based preservation biases. South Grandfield (Hennessey Formation, Kungurian in age), which represents a lowland fluvial system (Daly, 1973) is another locality that contains unusually good preservation quality of small taxa, and so is also useful in this context.
Analyses of reptile and synapsed diversity within Richards Spur support reptiles as being more diverse, whether using a taxonomic scheme favorable to reptiles or synapsids. It is true that, when using the scheme favorable to synapsids, synapsed diversity is found to be higher when subsampling to lower coverage levels (Figure 4A), but this is likely an artifact of a bias affecting the SQS method. SQS is heavily influenced by the shape of the relative abundance distribution (Close et al., 2018; Alroy, 2020): when it is uneven, with a small number of hyper-abundant taxa easily sampled, and most others being rare, it is easier to reach low coverage quora after sampling a small number of taxa. The extreme abundance of Captorhinus aguti at Richards Spur is likely confounding the estimates of reptile diversity in this way. When subsampling to higher coverage levels that produce more robust results (Brocklehurst et al., 2018a; Close et al., 2018) and when using the Squares method that is more resilient to this bias (Alroy, 2018, 2020), reptiles are found to be more diverse at Richards Spur no matter which taxonomic scheme is employed (Figure 4).
South Grandfield, which represents a lowland fluvial system but also shows unusual preservation quality of small taxa, preserves a similar fauna to that of Richards Spur: a great abundance of captorhinid reptiles, with large synapsids forming a relatively minor component of the fauna (Daly, 1973). Reptile diversity within South Grandfield is here found to be higher than that of synapsids whether or not the numerous recumbirostran species known from the locality are included within Reptilia.
Pennsylvanian Largestätte, also with preservation modes favoring of small taxa, could also be argued to show a greater emphasis on reptile diversity, although most of these contain better sampling of non-amniote tetrapods and did not contain the coverage of both synapsids and reptiles necessary to perform the species-accumulation analyses applied to Richards Spur. The Linton and Nýřany tetrapod assemblages of Ohio and Czechia, respectively (both of Moscovian age) contain only a single synapsed (Archaeothyris) but multiple reptiles and numerous microsaurs (Carroll and Baird, 1972; Reisz, 1975; Hook and Baird, 1986, 1988). The Garnett Quarry (of Kasimovian age) contains a greater observed species richness of synapsids, but by far the most abundant taxon is the diapsid Petrolacosaurus (Peabody, 1952; Wehrbein, 2017).
While this study focusses on the Pennsylvanian and Cisuralian, the interval previously thought dominated by pelycosaurian-grade synapsids, it is worth discussing the middle and late Permian (Guadalupian and Changhsingian) in the context of this lagerstätten effect. Reptile and synapsed diversity estimates have been compared during these intervals within the most productive tetrapod-bearing basin of that time: the Karoo of South Africa. Synapsids (then mostly represented by the therapsids rather than pelycosaurs) were found to be consistently more diverse than the reptiles (Fröbisch, 2013). However, this basin, and many other contemporary tetrapod-bearing basins, again show preservation heavily selective toward larger, more robust specimens (Kammerer, 2016), perhaps again biasing diversity estimates in favor of synapsids. A formation which provides a better record of small-bodied amniotes is Mezen in Russia, of early Guadalupian age (Golubev, 2015). Mezen’s depositional environment is thought to have been repeated, low-current shallow flooding events, which overtook small amniotes but allowed larger taxa to escape (Efremov, 1940). This fauna, where small taxa have been preferentially preserved, is again overwhelmingly dominated by reptiles. More than 70% of specimens from Mezen are parareptiles (see Supplementary Text and Data in Brocklehurst et al., 2017), while therapsid synapsids are rare (Lozovsky, 2005; Brocklehurst et al., 2017). There are also abundant varanopids at Mezen (Reisz and Berman, 2001; Anderson and Reisz, 2004), previously considered pelycosaurian-grade synapsids, but now potentially assigned to reptiles (Ford and Benson, 2019, 2020). While it must be noted that Mezen is geographically separated from other contemporary Russian localities, potentially providing an alternative explanation for the unusual fauna (Ivakhnenko, 2001), it is also possible that Mezen has allowed the preservation of the abundant small-bodied taxa that were prevalent elsewhere but less easily preserved (see Supplementary Text in Brocklehurst et al., 2017). In Mezen further corroboration is found of the signal found in Richards Spur: the selective preservation of smaller taxa, which we expect to make up the bulk of diversity, produces a reptile-dominated fauna.
Conclusion
The assumption that pelycosaurian-grade synapsids dominated terrestrial ecosystems in terms of their species richness has become extremely prevalent in the published literature, making it easy to forget that there has never been a robust analysis of the relative diversity patterns of the two amniote clades. While a global analysis of reptile and synapsed diversity during the Pennsylvanian and Cisuralian does support pelycosaur dominance when using a “traditional” taxonomy, recent revisions in the amniote phylogeny push reptile diversity closer to that of synapsids. In fact, if, as argued above, the selective preservation of smaller taxa in lagerstätten are more representative of true diversity patterns, then the patterns observed in the majority of formations may be misleading. Areas of exceptional preservation such as the Richards Spur fauna are better representative of true diversity patterns. In this context, reptiles should be considered to dominate early Permian ecosystems regardless of one’s opinion on amniote phylogeny. The Carboniferous and Early Permian, therefore, should not be considered a pelycosaur-dominate fauna, and the “Age of Reptiles,” rather than commencing in the Mesozoic as popular science suggests (e.g., Colbert, 1965; Delgado, 1993; Witton, 2017), may actually have begun with this clade’s earliest diversification.
Data Availability Statement
The original contributions presented in the study are included in the article/Supplementary Material, further inquiries can be directed to the corresponding author/s.
Author Contributions
NB conceived the project, generated the dataset, analyzed the data, and wrote the manuscript.
Funding
NB’s research was supported by Deutsche Forschungsge- meinschaft grant no. BR 5724/1-1p.
Conflict of Interest
The author declares that the research was conducted in the absence of any commercial or financial relationships that could be construed as a potential conflict of interest.
Publisher’s Note
All claims expressed in this article are solely those of the authors and do not necessarily represent those of their affiliated organizations, or those of the publisher, the editors and the reviewers. Any product that may be evaluated in this article, or claim that may be made by its manufacturer, is not guaranteed or endorsed by the publisher.
Acknowledgments
The author would like to thank Arjan Mann, Jason Pardo, and David Ford for helpful comments and discussion. Comments of two reviewers greatly improved the quality of the manuscript. This study is Paleobiology Database Official Publication Number 403. The author would also like to thank all who uploaded data to the PBDB, including B. Allen, E. Dunne, T. Leibrecht, and R. Whatley.
Supplementary Material
The Supplementary Material for this article can be found online at: https://www.frontiersin.org/articles/10.3389/fevo.2021.669765/full#supplementary-material
Footnotes
References
Alroy, J. (2010). Geographical, environmental and intrinsic biotic controls on Phanerozoic marine diversification. Palaeontology 53, 1211–1235. doi: 10.1111/j.1475-4983.2010.01011.x
Alroy, J. (2015). A new twist on a very old binary similarity coefficient. Ecology 96, 575–586. doi: 10.1890/14-0471.1
Alroy, J. (2018). Limits to species richness in terrestrial communities. Ecol. Lett. 21, 1781–1789. doi: 10.1111/ele.13152
Alroy, J. (2020). On four measures of taxonomic richness. Paleobiology 46, 158–175. doi: 10.1017/pab.2019.40
Anderson, J. S., and Reisz, R. R. (2004). Pyozia mesenensis, a new, small varanopid (Synapsida, Eupelycosauria) from Russia: “pelycosaur” diversity in the Middle Permian. J. Vertebr. Paleontol. 24, 173–179. doi: 10.1671/1940-13
Bakker, R. T. (1977). “Tetrapod mass extinctions—a model of the regulation of speciation rates and immigration by cycles of topographic diversity,” in Developments in Palaeontology and Stratigraphy, Vol. 5, ed. A. Hallam (Amsterdam: Elsevier), 439–468. doi: 10.1016/s0920-5446(08)70334-0
Behrensmeyer, A. K. (1988). Vertebrate preservation in fluvial channels. Palaeogeogr. Palaeoclimatol. Palaeoecol. 63, 183–199. doi: 10.1016/0031-0182(88)90096-x
Benson, R. B. (2012). Interrelationships of basal synapsids: cranial and postcranial morphological partitions suggest different topologies. J. Syst. Palaeontol. 10, 601–624. doi: 10.1080/14772019.2011.631042
Benson, R. B., and Upchurch, P. (2013). Diversity trends in the establishment of terrestrial vertebrate ecosystems: interactions between spatial and temporal sampling biases. Geology 41, 43–46. doi: 10.1130/g33543.1
Benton, M. J. (1985). Classification and phylogeny of the diapsid reptiles. Zool. J. Linn. Soc. 84, 97–164. doi: 10.1111/j.1096-3642.1985.tb01796.x
Benton, M. J. (2012). No gap in the Middle Permian record of terrestrial vertebrates. Geology 40, 339–342. doi: 10.1130/g32669.1
Benton, M. J., Tverdokhlebov, V. P., and Surkov, M. V. (2004). Ecosystem remodelling among vertebrates at the Permian–Triassic boundary in Russia. Nature 432, 97–100. doi: 10.1038/nature02950
Berman, D. S. (2000). Origin and early evolution of the amniote occiput. J. Paleontol. 74, 938–956. doi: 10.1666/0022-3360(2000)074<0938:oaeeot>2.0.co;2
Brocklehurst, N. (2016). Rates and modes of body size evolution in early carnivores and herbivores: a case study from Captorhinidae. PeerJ 4:e1555. doi: 10.7717/peerj.1555
Brocklehurst, N. (2018). An examination of the impact of Olson’s extinction on tetrapods from Texas. PeerJ 6:e4767. doi: 10.7717/peerj.4767
Brocklehurst, N. (2020). Olson’s gap or Olson’s extinction? A Bayesian tip-dating approach to resolving stratigraphic uncertainty. Proc. R. Soc. B 287:20200154. doi: 10.1098/rspb.2020.0154
Brocklehurst, N., and Brink, K. S. (2017). Selection towards larger body size in both herbivorous and carnivorous synapsids during the Carboniferous. Facets 2, 68–84. doi: 10.1139/facets-2016-0046
Brocklehurst, N., and Fröbisch, J. (2018a). A reexamination of Milosaurus mccordi, and the evolution of large body size in Carboniferous synapsids. J. Vertebr. Paleontol. 38:e1508026. doi: 10.1080/02724634.2018.1508026
Brocklehurst, N., and Fröbisch, J. (2018b). The definition of bioregions in palaeontological studies of diversity and biogeography affects interpretations: Palaeozoic tetrapods as a case study. Front. Earth Sci. 6:200. doi: 10.3389/feart.2018.00200
Brocklehurst, N., Day, M. O., and Fröbisch, J. (2018a). Accounting for differences in species frequency distributions when calculating beta diversity in the fossil record. Methods Ecol. Evol. 9, 1409–1420. doi: 10.1111/2041-210x.13007
Brocklehurst, N., Day, M. O., Rubidge, B. S., and Fröbisch, J. (2017). Olson’s extinction and the latitudinal biodiversity gradient of tetrapods in the Permian. Proc. R. Soc. B 284:20170231. doi: 10.1098/rspb.2017.0231
Brocklehurst, N., Dunne, E. M., Cashmore, D. D., and Fröbisch, J. (2018b). Physical and environmental drivers of Paleozoic tetrapod dispersal across Pangaea. Nat. Commun. 9:5216.
Brocklehurst, N., Kammerer, C. F., and Fröbisch, J. (2013). The early evolution of synapsids, and the influence of sampling on their fossil record. Paleobiology 39, 470–490. doi: 10.1666/12049
Brocklehurst, N., Reisz, R. R., Fernandez, V., and Fröbisch, J. (2016). A re-description of ‘Mycterosaurus’ smithae, an early Permian eothyridid, and its impact on the phylogeny of pelycosaurian-grade synapsids. PLoS One 11:e0156810. doi: 10.1371/journal.pone.0156810
Brocklehurst, N., Upchurch, P., Mannion, P. D., and O’Connor, J. (2012). The completeness of the fossil record of Mesozoic birds: implications for early avian evolution. PLoS One 7:e39056. doi: 10.1371/journal.pone.0039056
Brown, E. E., Cashmore, D. D., Simmons, N. B., and Butler, R. J. (2019). Quantifying the completeness of the bat fossil record. Palaeontology 62, 757–776. doi: 10.1111/pala.12426
Brown, J. H., and Nicoletto, P. F. (1991). Spatial scaling of the species composition – body masses of North-American land mammals. Am. Nat. 138, 1478–1512. doi: 10.1086/285297
Carroll, R. L. (1964). The earliest reptiles. Zool. J. Linn. Soc. 45, 61–83. doi: 10.1111/j.1096-3642.1964.tb00488.x
Carroll, R. L., and Baird, D. (1972). Carboniferous stem-reptiles of the family Romeriidae. Bull. Mus. Comp. Zool. 14, 321–363.
Chao, A., and Jost, L. (2012). Coverage-based rarefaction and extrapolation: standardizing samples by completeness rather than size. Ecology 93, 2533–2547. doi: 10.1890/11-1952.1
Close, R. A., Benson, R. B., Alroy, J., Carrano, M. T., Cleary, T. J., Dunne, E. M., et al. (2020a). The apparent exponential radiation of Phanerozoic land vertebrates is an artefact of spatial sampling biases. Proc. R. Soc. B 287:20200372. doi: 10.1098/rspb.2020.0372
Close, R. A., Benson, R. B., Saupe, E. E., Clapham, M. E., and Butler, R. J. (2020b). The spatial structure of Phanerozoic marine animal diversity. Science 368, 420–424. doi: 10.1126/science.aay8309
Close, R. A., Evers, S. W., Alroy, J., and Butler, R. J. (2018). How should we estimate diversity in the fossil record? Testing richness estimators using sampling-standardised discovery curves. Methods Ecol. Evol. 9, 1386–1400. doi: 10.1111/2041-210x.12987
Crottini, A., Madsen, O., Poux, C., Strauß, A., Vieites, D. R., and Vences, M. (2012). Vertebrate time-tree elucidates the biogeographic pattern of a major biotic change around the K–T boundary in Madagascar. Proc. Natl. Acad. Sci. U.S.A. 109, 5358–5363. doi: 10.1073/pnas.1112487109
Daly, E. (1973). A lower permian vertebrate fauna from southern Oklahoma. J. Paleontol. 47, 562–589.
Dean, C. D., Chiarenza, A. A., and Maidment, S. C. (2020). Formation binning: a new method for increased temporal resolution in regional studies, applied to the Late Cretaceous dinosaur fossil record of North America. Palaeontology 63, 881–901. doi: 10.1111/pala.12492
Dean, C. D., Mannion, P. D., and Butler, R. J. (2016). Preservational bias controls the fossil record of pterosaurs. Palaeontology 59, 225–247. doi: 10.1111/pala.12225
deBraga, M., Bevitt, J. J., and Reisz, R. R. (2019). A new captorhinid from the Permian cave system near Richards spur, Oklahoma, and the taxic diversity of Captorhinus at this locality. Front. Earth Sci. 7:112. doi: 10.3389/feart.2019.00112
Dos Reis, M., Thawornwattana, Y., Angelis, K., Telford, M. J., Donoghue, P. C., and Yang, Z. (2015). Uncertainty in the timing of origin of animals and the limits of precision in molecular timescales. Curr. Biol. 25, 2939–2950. doi: 10.1016/j.cub.2015.09.066
Dunne, E. M., Close, R. A., Button, D. J., Brocklehurst, N., Cashmore, D. D., Lloyd, G. T., et al. (2018). Diversity change during the rise of tetrapods and the impact of the ‘Carboniferous rainforest collapse’. Proc. R. Soc. B 285:20172730. doi: 10.1098/rspb.2017.2730
Eberth, D. A., and Brinkman, D. (1983). Ruthiromia elcobriensis, a new pelycosaur from El Cobre Canyon, New Mexico. Breviora 474, 1–26.
Efremov, J. A. (1940). Die mesen-fauna der permischen reptilien. Neues Jahrb. Mineral. Geol. Paläontol. Beilage Band Abt. B 84, 379–466.
Ezcurra, M. D., and Butler, R. J. (2018). The rise of the ruling reptiles and ecosystem recovery from the Permo-Triassic mass extinction. Proc. R. Soc. B 285:20180361. doi: 10.1098/rspb.2018.0361
Field, R., Hawkins, B. A., Cornell, H. V., Currie, D. J., Diniz-Filho, J. A. F., Guégan, J. F., et al. (2009). Spatial species-richness gradients across scales: a meta-analysis. J. Biogeogr. 36, 132–147. doi: 10.1111/j.1365-2699.2008.01963.x
Ford, D. P., and Benson, R. B. (2019). A redescription of Orovenator mayorum (Sauropsida, Diapsida) using high-resolution μ CT, and the consequences for early amniote phylogeny. Pap. Palaeontol. 5, 197–239. doi: 10.1002/spp2.1236
Ford, D. P., and Benson, R. B. (2020). The phylogeny of early amniotes and the affinities of parareptilia and varanopidae. Nat. Ecol. Evol. 4, 57–65. doi: 10.1038/s41559-019-1047-3
Fröbisch, J. (2013). Vertebrate diversity across the end-Permian mass extinction—separating biological and geological signals. Palaeogeogr. Palaeoclimatol. Palaeoecol. 372, 50–61. doi: 10.1016/j.palaeo.2012.10.036
Gauthier, J., Kluge, A. G., and Rowe, T. (1988). Amniote phylogeny and the importance of fossils. Cladistics 4, 105–209. doi: 10.1111/j.1096-0031.1988.tb00514.x
Golubev, V. K. (2015). Dinocephalian stage in the history of the Permian tetrapod fauna of Eastern Europe. Paleontol. J. 49, 1346–1352. doi: 10.1134/s0031030115120059
Gregory, J. T. (1965). Microsaurs and the origin of captorhinomorph reptiles. Am. Zool. 5, 277–286. doi: 10.1093/icb/5.2.277
Haubold, H., and Lucas, S. G. (2003). Tetrapod footprints of the lower Permian Choza formation at Castle Peak, Texas. Paläontol. Z. 77, 247–261. doi: 10.1007/bf03006940
Hook, R. W., and Baird, D. (1986). The Diamond coal mine of Linton, Ohio, and its Pennsylvanian-age vertebrates. J. Vertebr. Paleontol. 6, 174–190. doi: 10.1080/02724634.1986.10011609
Hook, R. W., and Baird, D. (1988). An overview of the Upper Carboniferous fossil deposit at Linton, Ohio. Ohio J. Sci. 88, 55–60.
Hsieh, T. C., Ma, K. H., and Chao, A. (2016). iNEXT: an R package for rarefaction and extrapolation of species diversity (Hill numbers). Methods Ecol. Evol. 7, 1451–1456. doi: 10.1111/2041-210x.12613
Hutchinson, G. E., and MacArthur, R. H. (1959). A theoretical ecological model of size distributions among species of animals. Am. Nat. 93, 117–125. doi: 10.1086/282063
Ivakhnenko, M. F. (2001). Tetrapods from the east european placket—Late Paleozoic natural territorial complex. Tr. Paleontol. Inst. Ross. Akad. Nauk 283, 1–200.
Jass, C. N., and George, C. O. (2010). An assessment of the contribution of fossil cave deposits to the Quaternary paleontological record. Quat. Int. 217, 105–116. doi: 10.1016/j.quaint.2009.11.008
Kammerer, C. F. (2016). A new taxon of cynodont from the Tropidostoma assemblage zone (upper Permian) of South Africa, and the early evolution of Cynodontia. Pap. Palaeontol. 2, 387–397. doi: 10.1002/spp2.1046
Kemp, T. S. (2006). The origin and early radiation of the therapsid mammal-like reptiles: a palaeobiological hypothesis. J. Evol. Biol. 19, 1231–1247. doi: 10.1111/j.1420-9101.2005.01076.x
Keil, P., Schweiger, O., Kühn, I., Kunin, W. E., Kuussaari, M., Settele, J., et al. (2012). Patterns of beta diversity in Europe: the role of climate, land cover and distance across scales. J. Biogeogr. 39, 1473–1486. doi: 10.1111/j.1365-2699.2012.02701.x
Klembara, J., Hain, M., Čerňanský, A., Berman, D. S., and Henrici, A. C. (2020). Anatomy of the neural endocranium, parasphenoid and stapes of Diadectes absitus (Diadectomorpha) from the early Permian of Germany based on the high-resolution X-ray microcomputed tomography. Anat. Rec. 303, 2977–2999. doi: 10.1002/ar.24376
Langston, W. Jr., and Reisz, R. R. (1981). Aerosaurus wellesi, new species, a varanopseid mammal-like reptile (Synapsida: Pelycosauria) from the Lower Permian of New Mexico. J. Vertebr. Paleontol. 1, 73–96. doi: 10.1080/02724634.1981.10011881
Laurin, M., and Piñeiro, G. H. (2017). A reassessment of the taxonomic position of mesosaurs, and a surprising phylogeny of early amniotes. Front. Earth Sci. 5:88. doi: 10.3389/feart.2017.00088
Laurin, M., and Reisz, R. R. (1995). A reevaluation of early amniote phylogeny. Zool. J. Linn. Soc. 113, 165–223. doi: 10.1006/zjls.1995.0007
Laurin, M., and Reisz, R. R. (1997). “A new perspective on tetrapod phylogeny,” in Amniote Origins: Completing the Transition to Land, eds S. S. Sumida and K. L. M. Martin (San Diego, CA: Academic Press), 9–59. doi: 10.1016/b978-012676460-4/50003-2
Laurin, M., and Reisz, R. R. (1999). A new study of Solenodonsaurus janenschi, and a reconsideration of amniote origins and stegocephalian evolution. Can. J. Earth Sci. 36, 1239–1255. doi: 10.1139/e99-036
Laurin, M., Lapauze, O., and Marjanović, D. (2018). What do ossification sequences tell us about the origin of extant amphibians? bioRxiv [Preprint] doi: 10.1101/352609
Lewis, G. E., and Vaughn, P. P. (1965). Early Permian vertebrates from the cutler formation of the Placerville Area, Colorado. Geol. Surv. Prof. Pap. 500C, 1–50.
Lozovsky, V. R. (2005). Olson’s gap or Olson’s bridge, that is the question. N. M. Mus. Nat. Hist. Sci. Bull. 30, 179–184.
Lucas, S. G., Celeskey, M., and Sundstrom, M. (2011). Traces of a Permian Seacoast: Prehistoric Trackways National Monument. Albuquerque, NM: New Mexico Museum of Natural History and Science.
Lucas, S. G. (2017). Permian tetrapod extinction events. Earth Sci. Rev. 170, 31–60. doi: 10.1016/j.earscirev.2017.04.008
Lucas, S. G. (2018). Permian tetrapod biochronology, correlation and evolutionary events. Geol. Soc. London Spec. Publ. 450, 405–444. doi: 10.1144/sp450.12
MacDougall, M. J., and Reisz, R. R. (2014). The first record of a nyctiphruretid parareptile from the early Permian of North America, with a discussion of parareptilian temporal fenestration. Zool. J. Linn. Soc. 172, 616–630. doi: 10.1111/zoj.12180
MacDougall, M. J., Modesto, S. P., Brocklehurst, N., Verrière, A., Reisz, R. R., and Fröbisch, J. (2018). Commentary: a reassessment of the taxonomic position of mesosaurs, and a surprising phylogeny of early amniotes. Front. Earth Sci. 6:99. doi: 10.3389/feart.2018.00099
MacDougall, M. J., Tabor, N. J., Woodhead, J., Daoust, A. R., and Reisz, R. R. (2016). The unique preservational environment of the early Permian (Cisuralian) fossiliferous cave deposits of the Richards Spur locality, Oklahoma. Palaeogeogr. Palaeoclimatol. Palaeoecol. 475, 1–11. doi: 10.1016/j.palaeo.2017.02.019
Maddin, H. C., Mann, A., and Hebert, B. (2020). Varanopid from the Carboniferous of Nova Scotia reveals evidence of parental care in amniotes. Nat. Ecol. Evol. 4, 50–56. doi: 10.1038/s41559-019-1030-z
Mann, A., and Reisz, R. R. (2020). Antiquity of “Sail-Backed” neural spine hyper-elongation in mammal forerunners. Front. Earth Sci. 8:83. doi: 10.3389/feart.2020.00083
Mann, A., Gee, B. M., Pardo, J. D., Marjanović, D., Adams, G. R., Calthorpe, A. S., et al. (2020). Reassessment of historic ‘microsaurs’ from Joggins, Nova Scotia, reveals hidden diversity in the earliest amniote ecosystem. Pap. Palaeontol. 6, 605–625. doi: 10.1002/spp2.1316
Mann, A., Pardo, J. D., and Maddin, H. C. (2019). Infernovenator steenae, a new serpentine recumbirostran from the ‘Mazon Creek’ Lagerstätte further clarifies lysorophian origins. Zool. J. Linn. Soc. 187, 506–517. doi: 10.1093/zoolinnean/zlz026
Marchetti, L., Voigt, S., Lucas, S. G., Francischini, H., Dentzien-Dias, P., Sacchi, R., et al. (2019). Tetrapod ichnotaxonomy in eolian paleoenvironments (Coconino and De Chelly Formations, Arizona) and late Cisuralian (Permian) sauropsid radiation. Earth Sci. Rev. 190, 148–170. doi: 10.1016/j.earscirev.2018.12.011
Marjanović, D. (2019). Recalibrating the transcriptomic timetree of jawed vertebrates. bioRxiv [Preprint] doi: 10.1101/2019.12.19.882829
Marjanović, D., and Laurin, M. (2019). Phylogeny of Paleozoic limbed vertebrates reassessed through revision and expansion of the largest published relevant data matrix. PeerJ 6:e5565.
McClain, C. R. (2004). Connecting species richness, abundance and body size in deep-sea gastropods. Glob. Ecol. Biogeogr. 13, 327–334. doi: 10.1111/j.1466-822x.2004.00106.x
Modesto, S. P., Scott, D. M., MacDougall, M. J., Sues, H.-D., Evans, D. C., and Reisz, R. R. (2015). The oldest parareptile and the early diversification of reptiles. Proc. R. Soc. B 282:20141912. doi: 10.1098/rspb.2014.1912
Müller, J., and Reisz, R. R. (2006). The phylogeny of early eureptiles: comparing parsimony and Bayesian approaches in the investigation of a basal fossil clade. Syst. Biol. 55, 503–511. doi: 10.1080/10635150600755396
Muñoz-Durán, J., and Van Valkenburgh, B. (2006). The Rancholabrean record of Carnivora: taphonomic effect of body size, habitat breadth, and the preservation potential of caves. Palaios 21, 424–430. doi: 10.2110/palo.2002.p05-078r
Olson, E. C. (1966). Community evolution and the origin of mammals. Ecology 47, 291–302. doi: 10.2307/1933776
Palmer, M. W., and White, P. S. (1994). Scale dependence and the species-area relationship. Am. Nat. 144, 717–740. doi: 10.1086/285704
Panchen, A. L., and Smithson, T. R. (1988). “The relationships of the earliest tetrapods,” in The Phylogeny and Classification of the Tetrapods, Amphibians, Reptiles, Birds, Vol. 1, ed. M. J. Benton (Oxford: Clarendon Press), 1–32.
Pardo, J. D., Szostakiwskyj, M., Ahlberg, P. E., and Anderson, J. S. (2017). Hidden morphological diversity among early tetrapods. Nature 546, 642–645. doi: 10.1038/nature22966
Peabody, F. E. (1952). Petrolacosaurus kansensis lane, a pennstlvanian reptile from Kansas. Univ. Kansas Paleontol. Contrib. Vertebr. 1, 1–41.
R Core Team (2017). R: A Language and Environment for Statistical Computing. Vienna: R Foundation for Statistical Computing.
Reisz, R. (1975). Pennsylvanian pelycosaurs from Linton, Ohio and Nýřany, Czechoslovakia. J. Paleontol. 49, 522–527.
Reisz, R. (2003). Cotylosaur phylogeny and the initial diversification of amniotes. J. Vertebr. Paleontol. 23:89A.
Reisz, R. R. (1972). Pelycosaurian reptiles from the middle Pennsylvanian of North America. Bull. Mus. Comp. Zool. 144, 27–61.
Reisz, R. R. (1986). Pelycosauria. Handbuch der Palaoherpetologie, Vol. 17. Stuttgart: Gustav Fischer Verlag.
Reisz, R. R., and Berman, D. S. (2001). The skull of Mesenosaurus romeri, a small varanopseid (Synapsida: Eupelycosauria) from the Upper Permian of the Mezen River Basin, northern Russia. Ann. Carnegie Mus. 70, 113–132.
Reisz, R. R., and Fröbisch, J. (2014). The oldest caseid synapsid from the Late Pennsylvanian of Kansas, and the evolution of herbivory in terrestrial vertebrates. PLoS One 9:e94518. doi: 10.1371/journal.pone.0094518
Reisz, R. R., and Modesto, S. P. (2007). Heleosaurus scholtzi from the Permian of South Africa: a varanopid synapsid, not a diapsid reptile. J. Vertebr. Paleontol. 27, 734–739. doi: 10.1671/0272-4634(2007)27[734:hsftpo]2.0.co;2
Reisz, R. R., and Sutherland, T. E. (2001). A diadectid (Tetrapoda: Diadectomorpha) from the lower Permian fissure fills of the Dolese Quarry, near Richards Spur, Oklahoma. Ann. Carnegie Mus. 70, 133–142.
Reisz, R. R., Laurin, M., and Marjanović, D. (2010). Apsisaurus witteri from the Lower Permian of Texas: yet another small varanopid synapsid, not a diapsid. J. Vertebr. Paleontol. 30, 1628–1631. doi: 10.1080/02724634.2010.501441
Richards, E. J. (2016). The Ontogenetic Osteohistology of the Eureptile Captorhinus aguti (Reptilia: Captorhinidae) and the Community Histology of the Early Permian Fissure-Fill Fauna Dolese Quarry, Richards Spur, Oklahoma. Toronto, ON: University of Toronto.
Romer, A. S. (1937). New genera and species of pelycosaurian reptiles. Proc. N. Engl. Zool. Club 16, 89–96.
Romer, A. S. (1950). The nature and relationships of the Paleozoic microsaurs. Am. J. Sci. 248, 628–654. doi: 10.2475/ajs.248.9.628
Romer, A. S., and Price, L. I. (1940). Review of the Pelycosauria. Boulder, CO: Geological Society of America.
Rosenweig, M. L. (1995). Species Diversity in Space and Time. Cambridge: Cambridge University Press.
Ruta, M., and Coates, M. I. (2007). Dates, nodes and character conflict: addressing the lissamphibian origin problem. J. Syst. Palaeontol. 5, 69–122. doi: 10.1017/s1477201906002008
Ruta, M., Jeffery, J. E., and Coates, M. I. (2003). A supertree of early tetrapods. Proc. R. Soc. B 270, 2507–2516. doi: 10.1098/rspb.2003.2524
Sahney, S., and Benton, M. J. (2008). Recovery from the most profound mass extinction of all time. Proc. R. Soc. B 275, 759–765. doi: 10.1098/rspb.2007.1370
Sahney, S., Benton, M. J., and Falcon-Lang, H. J. (2010). Rainforest collapse triggered Carboniferous tetrapod diversification in Euramerica. Geology 38, 1079–1082. doi: 10.1130/g31182.1
Sullivan, C., Reisz, R. R., and May, W. J. (2000). Large dissorophoid skeletal elements from the lower Permian Richards Spur fissures, Oklahoma, and their paleoecological implications. J. Vertebr. Paleontol. 20, 456–461. doi: 10.1671/0272-4634(2000)020[0456:ldseft]2.0.co;2
Szostakiwskyj, M., Pardo, J. D., and Anderson, J. S. (2015). Micro-CT study of Rhynchonkos stovalli (Lepospondyli, Recumbirostra), with description of two new genera. PLoS One 10:e0127307. doi: 10.1371/journal.pone.0127307
Vaughn, P. P. (1962). The Paleozoic microsaurs as close relatives of reptiles, again. Am. Midland Nat. 67, 79–84. doi: 10.2307/2422819
Vilela, B., and Villalobos, F. (2015). letsR: a new R package for data handling and analysis in macroecology. Methods Ecol. Evol. 6, 1229–1234. doi: 10.1111/2041-210x.12401
Voigt, S., and Lucas, S. G. (2018). Outline of a Permian tetrapod footprint ichnostratigraphy. Geol. Soc. London Spec. Publ. 450, 387–404. doi: 10.1144/sp450.10
Walker, F. M., Dunhill, A. M., and Benton, M. J. (2020). Variable preservation potential and richness in the fossil record of vertebrates. Palaeontology 63, 313–329. doi: 10.1111/pala.12458
Wehrbein, R. (2017). Comparisons of Paleoenvironments, Taxa, and Taphonomy of the Late Carboniferous Garnett and Hamilton Quarry Localities, Eastern Kansas. Kansas, MO: Emporia State University.
Whittaker, R. J., Willis, K. J., and Field, R. (2001). Scale and species richness: towards a general, hierarchical theory of species diversity. J. Biogeogr. 28, 453–470. doi: 10.1046/j.1365-2699.2001.00563.x
Keywords: amniote, reptile, alpha diversity, species richness, body size, preservation
Citation: Brocklehurst N (2021) The First Age of Reptiles? Comparing Reptile and Synapsid Diversity, and the Influence of Lagerstätten, During the Carboniferous and Early Permian. Front. Ecol. Evol. 9:669765. doi: 10.3389/fevo.2021.669765
Received: 19 February 2021; Accepted: 12 July 2021;
Published: 29 July 2021.
Edited by:
Sean P. Modesto, Cape Breton University, CanadaReviewed by:
Stuart Sumida, California State University, San Bernardino, United StatesJason D. Pardo, University of Calgary, Canada
Copyright © 2021 Brocklehurst. This is an open-access article distributed under the terms of the Creative Commons Attribution License (CC BY). The use, distribution or reproduction in other forums is permitted, provided the original author(s) and the copyright owner(s) are credited and that the original publication in this journal is cited, in accordance with accepted academic practice. No use, distribution or reproduction is permitted which does not comply with these terms.
*Correspondence: Neil Brocklehurst, bmVpbC5icm9ja2xlaHVyc3RAZWFydGgub3guYWMudWs=