- 1Rudi Drent Chair in Global Flyway Ecology, Groningen Institute for Evolutionary Life Sciences, University of Groningen, Groningen, Netherlands
- 2Department of Coastal Systems, NIOZ Royal Netherlands Institute for Sea Research, Texel, Netherlands
- 3U.S. Geological Survey, Alaska Science Center, Anchorage, AK, United States
In a 1998 paper entitled “Guts don’t fly: small digestive organs in obese bar-tailed godwits,” Piersma and Gill (1998) showed that the digestive organs were tiny and the fat loads huge in individuals suspected of embarking on a non-stop flight from Alaska to New Zealand. It was suggested that prior to migratory departure, these godwits would shrink the digestive organs used during fuel deposition and boost the size and capacity of exercise organs to optimize flight performance. Here we document the verity of the proposed physiomorphic changes by comparing organ sizes and body composition of bar-tailed godwits Limosa lapponica baueri collected in modesty midway during their fueling period (mid-September; fueling, n = 7) with the previously published data for godwits that had just departed on their trans-Pacific flight (October 19; flying, n = 9). Mean total body masses for the two groups were nearly identical, but nearly half of the body mass of fueling godwits consisted of water, while fat constituted over half of total body mass of flying godwits. The two groups also differed in their fat-free mass components. The heart and flight muscles were heavier in fueling godwits, but these body components constituted a relatively greater fraction of the fat-free mass in flying godwits. In contrast, organs related to digestion and homeostasis were heavier in fueling godwits, and most of these organ groups were also relatively larger in fueling godwits compared to flying godwits. These results reflect the functional importance of organ and muscle groups related to energy acquisition in fueling godwits and the consequences of flight-related exertion in flying godwits. The extreme physiomorphic changes apparently occurred over a short time window (≤1 month). We conclude that the inferences made on the basis of the 1998 paper were correct. The cues and stimuli which moderate these changes remain to be studied.
Introduction
The bodies of individual animals are not only in constant material and energy flux, but also constantly changing in mass and composition as individuals mature and age and make adjustments to external conditions like weather and food. Such changes may also reflect seasonally changing “goals and ambitions” – whether these relate to reproduction, molt or migration (Piersma and van Gils, 2011). The greater the variability in external demands in response to the manifold seasonal challenges, the greater the corporeal changes with respect to physiological and morphological phenotypic traits one come to expect. The impressive individual bodily changes during long-distance migration of birds discussed here, along with the fast morphological changes in snakes as they go from a fasting state to one of digesting large prey (Secor et al., 1994), have served as starting points in the field (Piersma and Lindström, 1997). To capture the simultaneous physiological (i.e., nervous, endocrine, and other biochemical states) and organ-level transformations shown by animals responding to changing context, Oudman et al. (2016) used the term “physiomorphic” as a unifying term. Among the great diversity of avian life forms, the extreme endurance migrants (Piersma, 2011; Conklin et al., 2017) would be expected to embody the most extensive physiomorphic changes.
Here we describe the physiomorphic restructuring in extreme endurance migrant birds during their transition from fueling to flight. In a 1998 paper entitled “Guts don’t fly: small digestive organs in obese bar-tailed godwits,” Piersma and Gill (1998) showed that in individual bar-tailed godwits Limosa lapponica baueri (Naumann, 1836) suspected of embarking on a non-stop flight from Alaska to New Zealand, the digestive organs were tiny and the fat load huge. Lacking a comparison with fueling birds, it was suggested that shortly prior to migratory departure, godwits would shrink the digestive organs used during fuel deposition and boost the size and capacity of exercise organs to optimize flight performance. Such patterns have meanwhile been established in other shorebird migration systems (Piersma et al., 1999; Landys-Ciannelli et al., 2003; Hua et al., 2013). In this contribution, we present the compositional analysis of a new sample of fueling godwits in comparison with the published in-flight sample, which now substantiates the suggestions made by Piersma and Gill (1998) about organ-restructuring for migration.
Study System
Bar-tailed godwits of the baueri subspecies breed in western Alaska and migrate south on a non-stop 10-day flight across the Pacific Ocean and spend the northern winter in eastern Australia and New Zealand; returning in spring to the Alaska breeding grounds in two long endurance flights interrupted by a refueling period in the Yellow Sea (Gill et al., 2009; Conklin et al., 2011; Battley et al., 2012). Before their southward migration, bar-tailed godwits stage at sites in Alaska along the coasts of the Yukon–Kuskokwim Delta and Alaska Peninsula estuaries from mid-July through October (Gill et al., 1981; Gill and Handel, 1990; Gill and McCaffery, 1999) and time their departure with favorable wind conditions (see Gill et al., 2009, 2014 for details). The initiation of southbound migration from Alaska to Australia and New Zealand varies annually but typically commences in late August or early September (McCaffery et al., 2006; McCaffery and Gill, 2020). Most adult godwits precede juveniles, such that adults typically begin arriving at non-breeding destinations in Australia and New Zealand in early or mid-September, while most juveniles do not arrive prior to October (T. Habraken and A. Riegen personal communication).
Materials and Methods
The samples used in our comparisons derive from two sites on the Alaska Peninsula. The first sample consists of nine juveniles (all males) salvaged after colliding with a large (12-m diameter) radar dome near Cold Bay, Alaska, at 23:30 h on October 19, 1987. Cold Bay is located near the tip of the ∼500-km-long Alaska Peninsula. Given the location (3–5 km inland of nearest intertidal habitat), time of day and season, and prevailing weather when the dome-strike occurred, it is believed that this sample represent godwits actively embarking upon southbound migration; see Piersma and Gill (1998) for details. The second sample of bar-tailed godwits (also all juveniles; two females and five males) was collected September 17–18, 2005 at Egegik Bay, a sampling period timed 2–4 weeks prior to the expected onset of southbound migration for juveniles. Egegik Bay is located ∼450 km northeast of Cold Bay, and has been identified as an important site for bar-tailed godwits during fall (boreal) migration (Gill and McCaffery, 1999). The juvenile godwits treated here thus represent a sample of birds (Egegik Bay) in the fueling annual-cycle stage of their migration, characterized by hyperphagia and possibly still some active body growth (Battley and Conklin, 2010), compared with a sample (Cold Bay) in the flight stage of their long-distance migration across the Pacific. Typically, bar-tailed godwit eggs hatch in the second week of June through the first week of July in Alaska (McCaffery and Gill, 2020). Assuming an average hatch date of June 21 for the birds in our sample, the Egegik Bay godwits would thus be approximately 3 months old when collected and the Cold Bay godwits approximately 4 months old.
Compositional Analysis
The specimens from Cold Bay and Egegik Bay were preserved and processed similarly. Two of the nine godwits from Cold Bay died upon impact with the radar dome, while the remaining seven surviving godwits were euthanized by chest compression 10 h after impact in view of the gravity of their injuries. Subsequent analysis determined no difference between the body composition of godwits that died upon impact and those that were euthanized after 10 h (see Piersma and Gill, 1998 for details). At Egegik Bay, godwits were collected by REG by shooting. Birds not killed instantly were immediately euthanized by chest compression. Godwits from both sites were weighed within an hour after death [using a spring scale (±5 g) and electronic balance (±1 g) for the flighted birds from Cold Bay and the fueling birds from Egegik Bay, respectively], and measurements of the exposed culmen and diagonal tarsus were collected [both using calipers (±0.1 mm)]) [note that we also measured wing chord (±1 mm), but as the measurements of wing length of the two samples were based on different methods, we refrained from using this measure of body size]. After the collection of external measurements, godwits from both sites were sealed in airtight plastic bags and frozen at −20°C.
Compositional analysis for both groups of godwits was performed similarly and consisted of dissecting birds into relevant organ and muscle groups; see Piersma and Gill (1998) and Landys-Ciannelli et al. (2003) for details related to specimens from Cold Bay and Egegik Bay, respectively. Birds from both sites were first plucked of feathers. Next, for birds from Cold Bay, the right flight muscle (including the pectoralis and supracoracoideus muscles; see Piersma and Dietz, 2007), heart, gizzard, esophagus and proventriculus, liver, left kidney, and gut were removed and weighed wet to the nearest 0.01 g. The length of the gut was measured to the nearest 1.0 mm, then gently flushed with water and reweighed empty; the gizzard and proventriculus were similarly emptied and reweighed.
To determine the compositional analysis of godwits from Cold Bay, a ground homogenate was made for each bird by passing the entire carcass (minus contents from the gut, gizzard, and proventriculus) multiple times through a food grinder (see Brown and Fredrickson, 1986). From each godwit, a random sample of the homogenate (∼10% of bird body weight) was collected and dried in a vacuum at 100°C for 15 h to determine water content. The lipid content of each sample was determined using Soxhlet extraction with petroleum ether following procedures described by AOAC (1984). Results of these analyses were expressed as proportions of fresh body mass from which we determined total fat, water, and fat-free dry masses for each specimen.
For godwit specimens from Egegik Bay, the heart, stomach (gizzard and proventriculus), liver, both kidneys, gut, and pectoralis and supracoracoideus muscles (from both sides) were dissected from each bird. These organs and muscles were weighed wet to the nearest 0.01 g. As with the birds from Cold Bay, the length of the gut was measured to the nearest 1.0 mm, then gently flushed with water and reweighed empty. The stomach was similarly emptied and reweighed. Each of these discrete organ and muscle groups was then dried along with the carcass at 60°C for ≥24 h and reweighed. These component pieces were next individually wrapped in filter paper and placed in a Soxhlet apparatus from which lipids were extracted using petroleum ether. Samples were again dried at 60°C for ≥24 h and weighed. From each Egegik Bay specimen we subtracted the dry mass from the wet mass to determine the water mass, and the fat-free dry mass from the dry mass to determine the fat mass. The sex of all specimens was determined based on gonadal inspection during dissections.
Statistical Analyses
We summed the values for the supracoracoideus and pectoralis muscles for bar-tailed godwits from Egegik Bay to determine the mass of each bird’s total flight muscle and then doubled the values of right flight muscle and left kidney from godwits from Cold Bay to standardize values between the two sample sites. For sake of comparison to previous studies (i.e., Piersma et al., 1999; Battley et al., 2000; Landys-Ciannelli et al., 2003), we summed the values of gizzard, esophagus and proventriculus from Cold Bay godwits to match the value of stomach mass from Egegik Bay godwits. Note, however, that Piersma and Gill (1998) reported values for gizzard alone.
Bar-tailed godwits exhibit sexual size dimorphism, with females averaging larger than males (Piersma and Jukema, 1990; Conklin et al., 2011). We corrected for sex-specific differences in the mass of organs and muscle groups by comparing values between the fueling birds from Egegik Bay and the flighted birds from Cold Bay using analysis of variance with sex as an additive factor. However, as we detected no significant differences in comparisons between females (n = 2) and males (n = 14) in our analyses, we combined the sexes in graphical comparisons between the two samples. As the mean body masses were nearly identical for the two sites, relative values would have been almost equivalent to what we present.
Due to unequal sample sizes in our groups, we fit analysis of variance models using type II sums of squares, and tested underlying model assumptions of normality and equality of variance using standard diagnostics (Faraway, 2002). We conducted all analyses using R software (R Core Team, 2021) and considered differences to be biologically meaningful at α = 0.05. Summaries are presented as mean ± standard deviation unless otherwise noted.
Results
There were few structural differences in body components between Egegik and Cold Bay birds. The average body mass of bar-tailed godwits fueling at Egegik Bay (379.0 ± 44.6 g, n = 7; the two females weighed 442 and 325 g, with an average of 383.5 g, similar to the five males who averaged 377.2 ± 35.5 g, range 326–417 g) was not statistically significantly different from that of birds at Cold Bay at the start of their migratory flight (366.9 ± 26.7 g, n = 9; Figure 1A; F1,13 = 0.3, p = 0.62). Likewise, the length of the exposed culmen did not differ significantly between the two groups (F1,13 = 3.3, p = 0.09; 80.0 ± 8.6 mm for Egegik Bay males, 102.8 ± 12.5 mm for Egegik Bay females, and 71.9 ± 6.8 mm for Cold Bay males), although the diagonal tarsus was longer in godwits from Egegik Bay (55.8 ± 3.1 mm for males, 60.0 ± 1.6 mm for females) than Cold Bay (52.9 ± 1.2 mm; F1,13 = 6.4, p = 0.03).
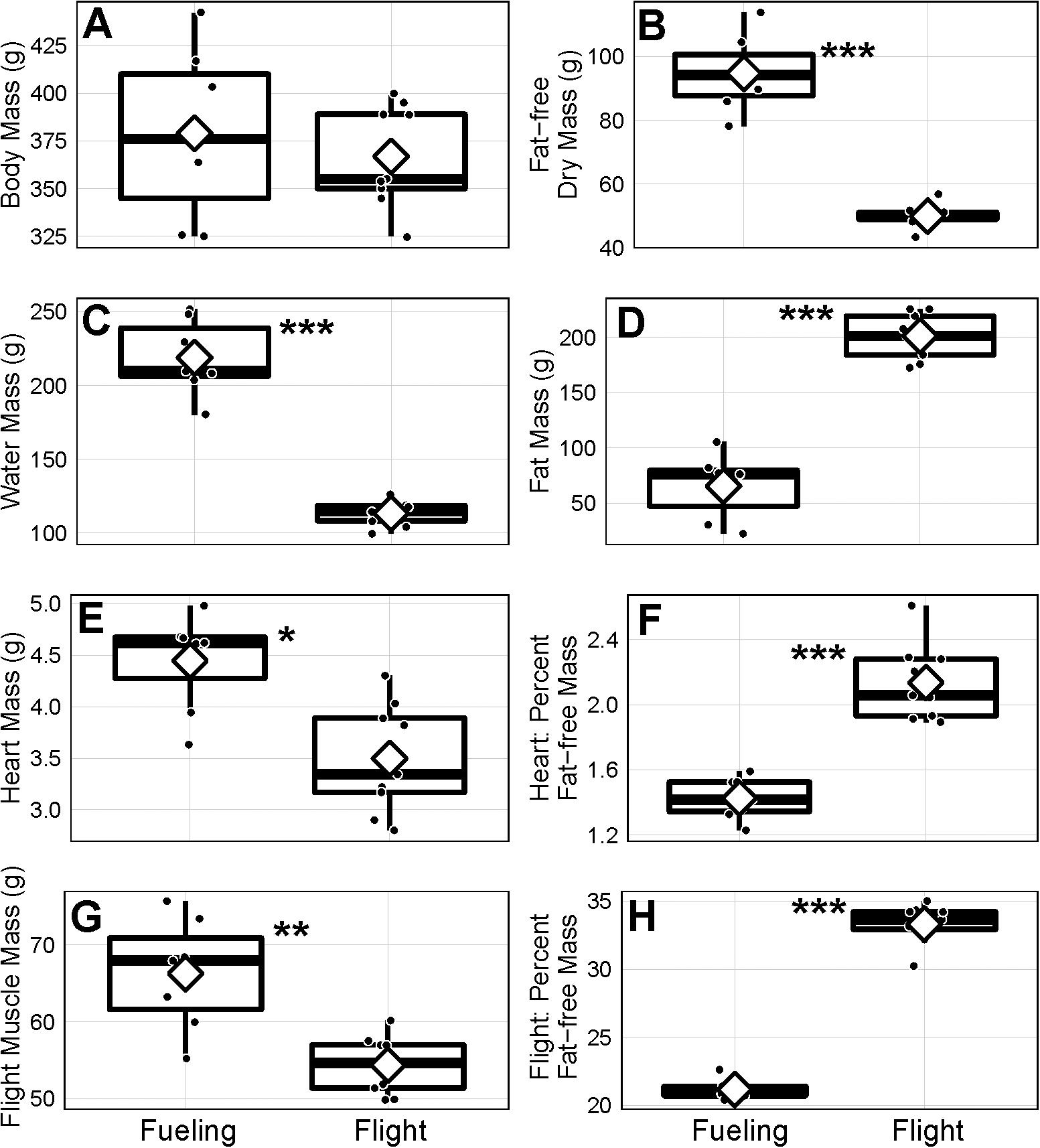
Figure 1. Mass (A–E,G) and mass relative to fat-free mass (F,H) of body components of juvenile bar-tailed godwits sampled in Alaska, United States, during the fueling (n = 2 females and 5 males) and flight (n = 9 males) annual-cycle stages of their first southward trans-Pacific migration. Horizontal lines represent the median, diamonds the mean, black circles the actual values, boxes the 25th and 75th percentiles, and whiskers the range of values. Statistically significant group differences based on analysis of variance analyses controlling for effects of sex represented by *p ≤ 0.05, **p ≤ 0.01, and ***p ≤ 0.001.
The similar body mass values stand in contrast to their composition, with the fueling birds consisting of 25.1% fat-free dry tissue, 58.0% water, and 17.0% fat, while the corresponding values for migrating birds from Cold Bay were 13.6% fat-free dry tissue, 30.9% water, and 54.8% fat (Figure 2). The mass of fat-free dry tissue (Figure 1B; F1,13 = 110.5, p < 0.001), water (Figure 1C; F1,13 = 102.2, p < 0.001), and fat (Figure 1D; F1,13 = 89.7, p < 0.001) all differed between the two groups. As expected (Piersma and van Brederode, 1990), water content of the fat-free mass of the two groups was similar (fueling group: 69.8%, flight group: 69.4%). In the flight group, fat-free dry mass (49.9 ± 3.5 g) was 52.7% that of the fueling group (94.7 ± 11.9 g). There was one additional source of heterogeneity, however. In the flight group from Cold Bay, the two dying on impact showed a water content of fat-free mass of 70.4%, whilst the value for the seven that were held for 10 h before euthanization was 68.0%, i.e., they lost a little water.
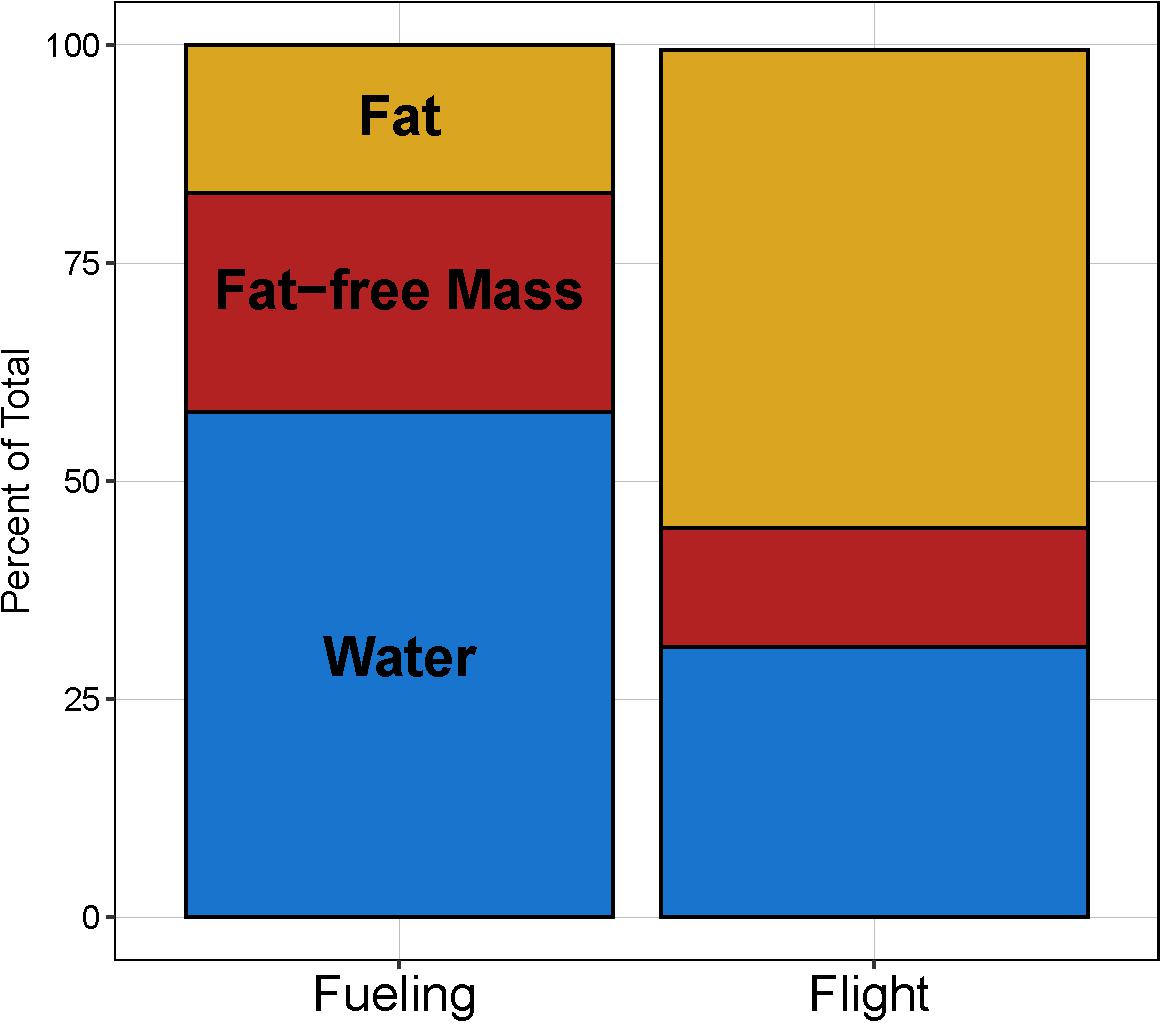
Figure 2. Average composition [percent of total mass comprised by water (blue), fat-free tissues (red), and fat (yellow)] of juvenile bar-tailed godwits sampled in Alaska, United States, during the fueling (September 17–18; n = 2 females and 5 males) and flight (October 19; n = 9 males) annual-cycle stages of their first southward trans-Pacific migration.
The proportionately low fat-free dry mass values (Figure 2) in the flight group resulted from reductions in the size of all measured body parts compared to those in the fueling group. This included the fresh mass of the primary exercise organs, the heart (Figure 1E; F1,13 = 8.4, p = 0.01) and the flight muscles (Figure 1G; F1,13 = 11.4, p = 0.004), which were heavier in bar-tailed godwits sampled during fueling than during flight. Nevertheless, as a fraction of the overall fat-free mass, the mass of heart (Figure 1F) and flight muscles (Figure 1H) showed proportional increases during the transition from fueling to flight (F1,13 = 40.3, p < 0.001 for heart, F1,13 = 333.5, p < 0.001 for flight muscle).
For the organs related to digestive and homeostatic functions, the fresh mass of liver (Figure 3A; F1,13 = 128.3, p < 0.001), kidneys (Figure 3C; F1,13 = 36.5, p < 0.001), stomach (Figure 3E; F1,13 = 18.5, p = 0.03), and intestine (Figure 3H; F1,13 = 135.4, p < 0.001) were all greater in fueling than in flying godwits. The length of the intestine was also longer in fueling godwits compared to flying godwits (Figure 3G; F1,13 = 62.2, p < 0.001). However, in contrast to the sizes of heart and flight muscle, the relative masses of these organs (as a percentage of overall fat-free mass) had become smaller in flying compared with fueling godwits [Figure 3B (F1,13 = 78.3, p < 0.001) for liver; Figure 3D (F1,13 = 6.8, p = 0.02) for kidney; F1,13 = 141.0, p < 0.001 for intestine]. The exception here was the mass of the stomach, which was relatively heavier in flying godwits (Figure 3F; F1,13 = 4.2, p = 0.02).
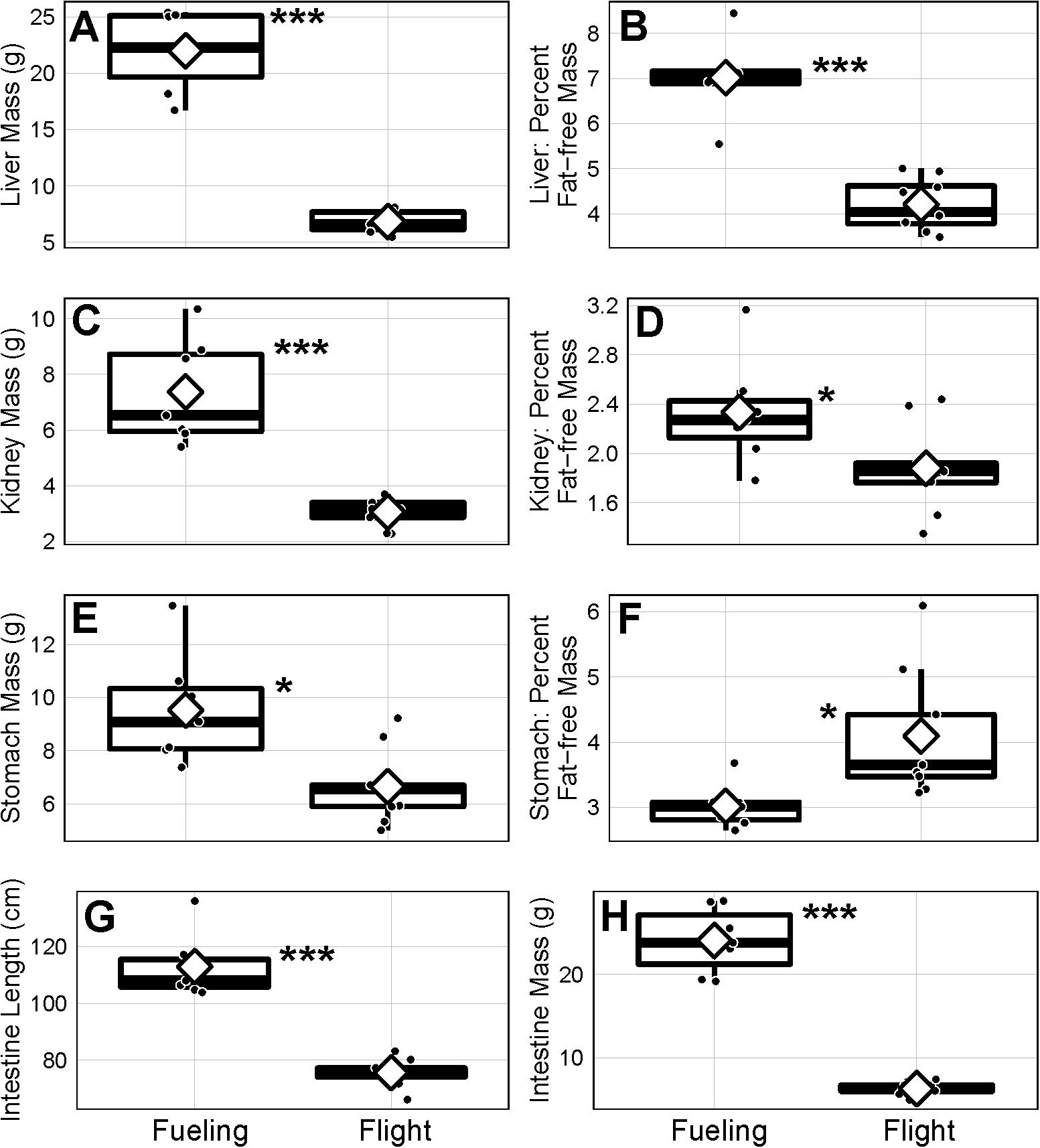
Figure 3. Mass (A,C,E,H), mass relative to fat-free mass (B,D,F), and length (G) of body components of juvenile bar-tailed godwits sampled in Alaska, United States, of body components of juvenile bar-tailed godwits sampled in Alaska, United States, during the fueling (n = 2 females and 5 males) and flight (n = 9 males) annual-cycle stages of their first southward trans-Pacific migration. Horizontal lines represent the median, diamonds the mean, black circles the actual values, boxes the 25th and 75th percentiles, and whiskers the range of values. Statistically significant group differences based on analysis of variance analyses controlling for effects of sex represented by *p ≤ 0.05, **p ≤ 0.01, and ***p ≤ 0.001.
Discussion
Although the mean total body masses of the fueling and flying groups were similar, fat contributed a small fraction of 17% to the bodies of fueling godwits but comprised over half in the flying godwits. Interestingly, not only were the organs related to digestion and homeostasis heavier in fueling godwits, so were heart and flight muscles; although the latter two components constituted a relatively greater fraction of the fat-free mass of flying godwits. The sampling of the juvenile bar-tailed godwits occurred a month earlier at Egegik Bay than in Cold Bay. Although we do not know their precise ages, we do know that the older Cold Bay birds died in migratory flight (Piersma and Gill, 1998), whereas the birds from Egegik Bay were collected whilst foraging on intertidal flats on the known staging grounds (Gill and Handel, 1990; Gill and McCaffery, 1999). The differences in composition between the fueling and flying groups should reflect the difference in the annual-cycle stage during which they were sampled rather than differences in age.
In previous studies on body composition and organ sizes in (re-)fueling red knots Calidris canutus and bar-tailed godwits based on sequential sampling, the absolute sizes of flight muscles and heart always increased in the week before take-off on long-distance migratory flights (Piersma et al., 1999; Landys-Ciannelli et al., 2003; Hua et al., 2013). Preparations also included increases in hematocrit (Piersma et al., 1996; Landys-Ciannelli et al., 2002), the correlated increases in the viscosity of the blood apparently necessitating a stronger, bigger pump (i.e., the heart; Piersma et al., 1999). In the present study, the increases in relative flight muscle mass are consistent with an increasing power requirement for similar flight performance as weight load increases (Lindström et al., 2000; Dietz et al., 2007). Yet, the decreases in absolute (rather than relative) size of heart and flight muscle mass in fueling vs. flying juvenile baueri godwits shown here are unexpected. The relative increase in heart size may still allow adequate blood circulation due to a structurally smaller “working body” (expressed in fat-free mass), as the enormous fat load at the start of flight would be poorly vascularized. The small flight muscles relative to body mass inevitably lead to reductions in take-off speed and flight maneuverability, thus increasing predation danger in the birds that are ready to depart (Burns and Ydenberg, 2002; van den Hout et al., 2010) should aerial predators be around. As argued before (Piersma, 1998), the outcomes of these trade-offs indicate the extreme pressures on the “economic design” (Yang et al., 2013) necessitated by the trans-Pacific non-stop flights of 11,000 km or more (Gill et al., 2009; Battley et al., 2012).
Fully consistent with the earlier findings (Piersma et al., 1999; Landys-Ciannelli et al., 2003; Hua et al., 2013) were the decreases, both in absolute and relative terms, of the digestive and processing organs such as liver, kidney, stomach, and intestine (with relative stomach mass showing a small increase, Figure 3F). This makes sense as, during the non-stop endurance flights of more than a week, there is no need to maintain any machinery other than that for active flight, provided that the ecological conditions (food and danger from predators) at the ports of call in Australia and New Zealand are permissive to “fully depleted” arriving birds (see discussion in Piersma et al., 2021). To illuminate the ecological and physiological constraints on endurance performance, information on the “design” consequences of ecological context during arrival and physiological knowledge on the requirement to liberate amino acids from proteinaceous tissue for repair work during the non-stop flights (Battley et al., 2000; Guglielmo et al., 2001), are now in urgent need of further study. This would include answers to questions on the apparent “defense” of stomach size in the transformation to endurance flight, reminiscent of similar organ “behavior” in starving red knots (Dietz and Piersma, 2007), and the striking lack of mass loss in the stomachs of great knots Calidris tenuirostris during northward non-stop flights of 7,000 km from northwest Australia to the Yellow Sea when most other organs except the brain lost mass (Battley et al., 2000).
All these changes lead to individual avian bodies which in the course of 2–4 weeks do not change in mass, but strongly increase in fat load, culminating in 58% fat in the sample of bar-tailed godwits colliding in flight with the radar dome at Cold Bay (Figure 2). As fat has an energy density four times higher than wet protein (i.e., the building material of organs and muscles; Jenni and Jenni-Eiermann, 1998), over the period of transformation the energy content of the godwits continues to increase. Assuming that the phenology of migration was the same in both years so that a 4-week period realistically separates the two sampling events, the observed increase in fat mass from 65.5 to 201.4 g (i.e., 135.9 g) would mean an additional daily requirement of 4.85 g fat/day, which would mean a daily additional energy requirement of 4.85/0.85 (=assimilation efficiency; Kersten and Piersma, 1987) × 40 kJ (=energy density per g fat), or 228 kJ/day. This would add a 58% increase over the maintenance requirement (ca. 400 kJ/day according to Scheiffarth et al., 2002). Thus, during the 28 days of staging following on from the moment of sampling at Egegik Bay, in order to “pay” for a linear increase in fat stores staging bar-tailed godwits would still need to keep eating over 50% more than what would be required to simply maintain energy balance.
This raises a new question regarding the seasonal progression of fat storage and the shrinking of digestive machinery, changes that probably do not happen in linear ways. Specifically, when in the departure process do the various physiomorphic transformations take place and what cues them? For example, if birds reach some set point of fuel and then cue on a synoptic weather event to begin the transition, they likely have 2–5 days over which to at least reduce the digestive machinery. During this period, the flight muscles may also be tuned to the impending endurance challenge. The prolonged circuitous bouts of flight seen at Egegik Bay and Nelson Lagoon which were only observed in the few days before departures (R.E. Gill personal observation), raise the question whether these flights provide the required training to shape the flight machinery. It is also possible that, instead, the flights function in a more social context related to “coordinating” departures of birds in similar condition (Piersma et al., 1990).
More questions remain, as the inferences on the bodily transition were made on the basis of comparisons of juveniles before their first trans-Pacific flight rather than experienced adults. In view of the similar water percentages in the fat-free fraction of the body, it is unlikely that tissue maturation (see Starck and Ricklefs, 1998) contributed to some of the reduction in overall water mass between fueling and flight samples. Nevertheless, this leaves open the option that some of the increase in fat mass between sampling periods could be due to completion of growth and consequent reallocation from growth to energy storage. We now need comparative work on adults to illuminate these questions.
The experimental finding that captive and relatively immobile shorebirds can build flight muscles without exercise (Dietz et al., 1999), nicely predates Hoppeler’s (2016) conclusion that “Muscle bulk and composition can be changed without invoking endurance or strength training paradigms, depending on the needs of a species. Available signaling networks can be invoked by any adequate internal or external cue.” Thus, whereas for exercise physiologists it may not come as a surprise that bar-tailed godwits can do what they do, the precise time path of physiomorphic preparation for the extreme endurance exercise required for trans-Pacific and other long-distance migrations still needs to be revealed. Positioned in ecological context, with reference to food availability, access to food resources, time-activity budgets, diets, and the danger of predation, they may lead to a better appreciation on the creative evolutionary interface between organisms and environment.
Data Availability Statement
The datasets presented in this study can be found in online repositories. The names of the repository/repositories and accession number(s) can be found below: Data used in this study are publicly available from the U.S. Geological Survey (https://doi.org/10.5066/P9GIQ8J2).
Ethics Statement
The animal study was reviewed and approved by the Alaska Department of Fish and Game and the USFWS Migratory Bird Permit Office.
Author Contributions
TP and RG conceptualized the study. RG collected the data. DR analyzed the data. All authors wrote the manuscript and approved the final manuscript.
Funding
Funding was provided by the Ecosystems Mission Area of the U.S. Geological Survey.
Conflict of Interest
The authors declare that the research was conducted in the absence of any commercial or financial relationships that could be construed as a potential conflict of interest.
Acknowledgments
Serendipity played a large role in bringing this story together. Ben Weinrick, Cold Bay Long Range Radar site technician had the foresight to contact Chris Dau, the local U.S. Fish and Wildlife Service (retired) biologist, within minutes of when the godwits collided with the dome. Had Weinrick waited until the morning, scavenging foxes and gulls would have undoubtedly found the birds. Dau, in turn, realized not only the significance of the birds in terms of documenting trans-oceanic migration but also the importance of recording timely meristic data from fresh specimens. And we of course owe special thanks to Leigh H. Fredrickson, University of Missouri-Columbia (Emeritus) for his lab’s detailed compositional analyses of the Cold Bay specimens. Anne Dekinga helped us during the collection of the Egegik birds and their laboratory analysis in Netherlands. Any use of trade, product, or firm names is for descriptive purposes only and does not imply endorsement by the U.S. Government.
References
AOAC. (1984). Official Methods of Analysis, 14th Edn. Washington: Association of Official Analytical Chemists.
Battley, P. F., and Conklin, J. R. (2010). Post-fledging growth in a migrating juvenile bar-tailed godwit. Wader Study Group Bull. 117, 186–187.
Battley, P. F., Piersma, T., Dietz, M. W., Tang, S., Dekinga, A., and Hulsman, K. (2000). Empirical evidence for differential organ reductions during trans-oceanic bird flight. Proc. R. Soc. Lond. B Biol. Sci. 267, 191–196. doi: 10.1098/rspb.2000.0986
Battley, P. F., Warnock, N., Tibbitts, T. L., Gill, R. E. Jr., Piersma, T., Hassell, C. J., et al. (2012). Contrasting extreme long-distance migration patterns in bar-tailed godwits Limosa lapponica. J. Avian Biol. 43, 21–32. doi: 10.1111/j.1600-048x.2011.05473.x
Brown, P. W., and Fredrickson, L. H. (1986). Body and organ weights, and carcass composition of breeding female white-winged scoters. Wildfowl 38, 103–107.
Burns, J. G., and Ydenberg, R. C. (2002). The effects of wing loading and gender on the escape flights of least sandpipers (Calidris minutilla) and western sandpipers (Calidris mauri). Behav. Ecol. Sociobiol. 52, 128–136. doi: 10.1007/s00265-002-0494-y
Conklin, J. R., Battley, P. F., Potter, M. A., and Ruthrauff, D. R. (2011). Geographic variation in morphology of Alaska-breeding bar-tailed godwits (Limosa lapponica) is not maintained on their nonbreeding grounds in New Zealand. Auk 128, 363–373. doi: 10.1525/auk.2011.10231
Conklin, J. R., Senner, N. R., Battley, P. F., and Piersma, T. (2017). Extreme migration and the individual quality spectrum. J. Avian Biol. 48, 19–36. doi: 10.1111/jav.01316
Dietz, M. W., and Piersma, T. (2007). Red knots give up flight capacity and defend food processing capacity during winter starvation. Funct. Ecol. 21, 899–904. doi: 10.1111/j.1365-2435.2007.01290.x
Dietz, M. W., Piersma, T., and Dekinga, A. (1999). Body-building without power training: endogenously regulated pectoral muscle hypertrophy in confined shorebirds. J. Exp. Biol. 202, 2831–2837. doi: 10.1242/jeb.202.20.2831
Dietz, M. W., Piersma, T., Hedenström, A., and Brugge, M. (2007). Intraspecific variation in avian pectoral muscle mass: constraints on maintaining maneouvrability with increasing body mass. Funct. Ecol. 21, 317–326. doi: 10.1111/j.1365-2435.2006.01234.x
Faraway, J. J. (2002). Practical Regression and Anova using R. Available online at: http://www.cran.r-project.org/doc/contrib/Faraway-PRA.pdf (accessed March 3, 2021).
Gill, R. E. Jr., Douglas, D. C., Handel, C. M., Tibbitts, T. L., Hufford, G., and Piersma, T. (2014). Hemispheric-scale wind selection facilitates bar-tailed godwit circum-migration of the Pacific. Anim. Behav. 90, 117–130. doi: 10.1016/j.anbehav.2014.01.020
Gill, R. E. Jr., and Handel, C. M. (1990). The importance of subarctic intertidal habitats to shorebirds: a study of the central Yukon-Kuskokwim Delta, Alaska. Condor 92, 709–725. doi: 10.2307/1368690
Gill, R. E. Jr., and McCaffery, B. J. (1999). Bar-tailed godwits Limosa lapponica in Alaska: a population estimate from the staging grounds. Wader Study Group Bull. 88, 49–54.
Gill, R. E. Jr., Petersen, M. R., and Jorgensen, P. D. (1981). Birds of the northcentral Alaska Peninsula, 1976-1980. Arctic 34, 286–306.
Gill, R. E. Jr., Tibbitts, T. L., Douglas, D. C., Handel, C. M., Mulcahy, D. M., Gottschalck, J. C., et al. (2009). Extreme endurance flights by landbirds crossing the Pacific Ocean: ecological corridor rather than barrier? Proc. R. Soc. Lond. B Biol. Sci. 276, 447–457. doi: 10.1098/rspb.2008.1142
Guglielmo, C. G., Piersma, T., and Williams, T. D. (2001). A sport-physiological perspective on bird migration: evidence for flight-induced muscle damage. J. Exp. Biol. 204, 2683–2690. doi: 10.1242/jeb.204.15.2683
Hoppeler, H. (2016). Molecular networks in skeletal muscle plasticity. J. Exp. Biol. 219, 205–213. doi: 10.1242/jeb.128207
Hua, N., Piersma, T., and Ma, Z. (2013). Three-phase fuel deposition in a long-distance migrant, the red knot (Calidris canutus piersmai), before the flight to High Arctic breeding grounds. PLoS One 8:e62551. doi: 10.1371/journal.pone.0062551
Jenni, L., and Jenni-Eiermann, S. (1998). Fuel supply and metabolic constraints in migrating birds. J. Avian Biol. 29, 521–528. doi: 10.2307/3677171
Kersten, M., and Piersma, T. (1987). High levels of energy expenditure in shorebirds; metabolic adaptations to an energetically expensive way of life. Ardea 75, 175–187. doi: 10.5253/arde.v75.p175
Landys-Ciannelli, M. M., Jukema, J., and Piersma, T. (2002). Blood parameter changes during stopover in a long-distance migratory shorebird, the bar-tailed godwit Limosa lapponica taymyrensis. J. Avian Biol. 33, 451–455. doi: 10.1034/j.1600-048x.2002.03051.x
Landys-Ciannelli, M. M., Piersma, T., and Jukema, J. (2003). Strategic size changes of internal organs and muscle tissue in the bar-tailed godwit during fat storage on a spring stopover site. Funct. Ecol. 17, 151–159. doi: 10.1046/j.1365-2435.2003.00715.x
Lindström, Å, Kvist, A., Piersma, T., Dekinga, A., and Dietz, M. W. (2000). Avian pectoral muscle size rapidly tracks body mass changes during flight, fasting and fuelling. J. Exp. Biol. 203, 913–919. doi: 10.1242/jeb.203.5.913
McCaffery, B. J., and Gill, R. E. (2020). “Bar-tailed godwit (Limosa lapponica), version 1.0,” in Birds of the World, ed. S. M. Billerman (Ithaca: Cornell Lab of Ornithology).
McCaffery, B. J., Handel, C. M., Gill, R. E. Jr., and Ruthrauff, D. R. (2006). The blind men and the elephant: concerns about the use of juvenile proportion data. Stilt 50, 194–204.
Oudman, T., Bijleveld, A. I., Kavelaars, M. M., Dekinga, A., Cluderay, J., Piersma, T., et al. (2016). Diet preferences as the cause of individual differences rather than the consequence. J. Anim. Ecol. 85, 1378–1388. doi: 10.1111/1365-2656.12549
Piersma, T. (1998). Phenotypic flexibility during migration: optimization of organ size contingent on the risks and rewards of fueling and flight? J. Avian Biol. 29, 511–520. doi: 10.2307/3677170
Piersma, T. (2011). Why marathon migrants get away with high metabolic ceilings: towards an ecology of physiological restraint. J. Exp. Biol. 214, 295–302. doi: 10.1242/jeb.046748
Piersma, T., and Dietz, M. W. (2007). Twofold seasonal variation in the supposedly constant, species-specific, ratio of upstroke to downstroke flight muscles in red knots Calidris canutus. J. Avian Biol. 38, 536–540. doi: 10.1111/j.2007.0908-8857.04253.x
Piersma, T., Everaarts, J. M., and Jukema, J. (1996). Build-up of red blood cells in refuelling bar-tailed godwits in relation to individual migratory quality. Condor 98, 363–370. doi: 10.2307/1369154
Piersma, T., and Gill, R. E. Jr. (1998). Guts don’t fly: small digestive organs in obese bar-tailed godwits. Auk 115, 196–203. doi: 10.2307/4089124
Piersma, T., Gill, R. E. Jr., Ruthrauff, D. R., Guglielmo, C. G., Conklin, J. R., and Handel, C. M. (2021). The Pacific as the world’s greatest theater of bird migration: extreme migrants and the potential for insightful biological comparisons. Ornithology, manuscript in review
Piersma, T., Gudmundsson, G. A., and Lilliendahl, K. (1999). Rapid changes in the size of different functional organ and muscle groups during refueling in a long-distance migrating shorebird. Physiol. Biochem. Zool. 72, 405–415. doi: 10.1086/316680
Piersma, T., and Jukema, J. (1990). Budgeting the flight of a long-distance migrant: changes in nutrient reserve levels of bar-tailed godwits at successive spring staging sites. Ardea 78, 315–337. doi: 10.5253/arde.v78.p315
Piersma, T., and Lindström, Å (1997). Rapid reversible changes in organ size as a component of adaptive behaviour. Trends Ecol. Evol. 12, 134–138. doi: 10.1016/s0169-5347(97)01003-3
Piersma, T., and van Brederode, N. E. (1990). The estimation of fat reserves in coastal waders before their departure from northwest Africa in spring. Ardea 78, 221–236.
Piersma, T., and van Gils, J. A. (2011). The Flexible Phenotype. A Body-Centred Integration of Ecology, Physiology and Behaviour. Oxford: Oxford University Press.
Piersma, T., Zwarts, L., and Bruggemann, J. H. (1990). Behavioural aspects of the departure of waders before long-distance flights: flocking, vocalizations, flight paths and diurnal timing. Ardea 78, 157–184.
R Core Team (2021). R: A Language and Environment for Statistical Computing. Vienna: R Foundation for Statistical Computing. Available online at: https://www.r-project.org/ (accessed January 28, 2021).
Scheiffarth, G., Wahls, S., Ketzenberg, C., and Exo, K.-M. (2002). Spring migration strategies of two populations of bar-tailed godwits, Limosa lapponica, in the Wadden Sea: time minimizers or energy minimizers? Oikos 96, 346–354. doi: 10.1034/j.1600-0706.2002.960216.x
Secor, S. M., Stein, E. D., and Diamond, J. (1994). Rapid upregulation of snake intestine in response to feeding: a new model of intestinal adaptation. Am. J. Physiol. 266, G695–G705.
Starck, J. M., and Ricklefs, R. E. (eds) (1998). Avian Growth and Development: Evolution within the Altricial-Precocial Spectrum. New York: Oxford University Press.
van den Hout, P. J., Mathot, K. J., Maas, L. R. M., and Piersma, T. (2010). Predator escape tactics in birds: linking ecology and aerodynamics. Behav. Ecol. 21, 16–25. doi: 10.1093/beheco/arp146
Keywords: body composition, exercise, fat stores, migration, organ, shorebird
Citation: Piersma T, Gill RE Jr and Ruthrauff DR (2021) Physiomorphic Transformation in Extreme Endurance Migrants: Revisiting the Case of Bar-Tailed Godwits Preparing for Trans-Pacific Flights. Front. Ecol. Evol. 9:685764. doi: 10.3389/fevo.2021.685764
Received: 25 March 2021; Accepted: 17 May 2021;
Published: 17 June 2021.
Edited by:
Arne Hegemann, Lund University, SwedenReviewed by:
Lukas Jenni, Swiss Ornithological Institute, SwitzerlandPierrick Bocher, Université de la Rochelle, France
Copyright © 2021 Piersma, Gill and Ruthrauff. This is an open-access article distributed under the terms of the Creative Commons Attribution License (CC BY). The use, distribution or reproduction in other forums is permitted, provided the original author(s) and the copyright owner(s) are credited and that the original publication in this journal is cited, in accordance with accepted academic practice. No use, distribution or reproduction is permitted which does not comply with these terms.
*Correspondence: Theunis Piersma, dGhldW5pcy5waWVyc21hQG5pb3oubmw=