- 1Laboratory of Socioecology and Social Evolution, Department of Biology, KU Leuven, Leuven, Belgium
- 2Laboratório de Biologia e Ecologia de Abelhas – Instituto de Ciências Biológicas, Universidade Federal do Pará – Rua Augusto Corrêa, Belém, Brazil
- 3Polytechnic School, Universidade do Vale do Rio dos Sinos, São Leopoldo, Brazil
- 4Vale Institute of Technology – Sustainable Development, Rua Boaventura de Silva, Belém, Brazil
- 5Exponent, Bellevue, WA, United States
- 6Vale Institute of Technology – Mining, Ouro Preto, Brazil
- 7Institute of Exact and Natural Sciences, Federal University of Pará, Belém, Brazil
- 8Computing Department, Universidade Federal de Ouro Preto, Ouro Preto, Brazil
- 9Nativo Bees, Brisbane, QLD, Australia
- 10Embrapa Amazônia Oriental, Belém, Brazil
- 11School of Information Communication Technology, Griffith University, Gold Coast, QLD, Australia
- 12Instituto de Biociências, Universidade de São Paulo, São Paulo, Brazil
Bees play a key role in ecosystem services as the main pollinators of numerous flowering plants. Studying factors influencing their foraging behavior is relevant not only to understand their biology, but also how populations might respond to changes in their habitat and to the climate. Here, we used radio-frequency identification tags to monitor the foraging behavior of the neotropical stingless bee Melipona fasciculata with special interest in drifting patterns i.e., when a forager drifts into a foreign nest. In addition, we collected meteorological data to study how abiotic factors affect bees’ activity and behavior. Our results show that only 35% of bees never drifted to another hive nearby, and that factors such as temperature, humidity and solar irradiation affected the bees drifting rates and/or foraging activity. Moreover, we tested whether drifting levels would decrease after marking the nest entrances with different patterns. However, contrary to our predictions, there was an increase in the proportion of drifting, which could indicate factors other than orientation mistakes playing a role in this behavior. Overall, our results demonstrate how managed bee populations are affected by both nearby hives and climate factors, offering insights on their biology and potential commercial application as crop pollinators.
Introduction
Stingless bees are a highly diverse group of social bees comprising more than 500 species native to the tropical and subtropical regions of the world (Grüter, 2020a). They form perennial colonies composed of hundreds to thousands of workers that are common visitors of many flowering plants, including several crop species (Heard, 1999; Michener, 2007). Some species of stingless bees are already successfully managed in small scale, notably those from the genus Melipona that have been traditionally used for honey production in the Americas, with several other stingless bee genera used in Africa, Asia and Oceania (Cortopassi-Laurino et al., 2006; Quezada-Euán et al., 2018; Orr et al., 2021). However, despite their great potential to be used as commercial pollinators (Cruz et al., 2005; Del Sarto et al., 2005; Slaa et al., 2006; Bispo dos Santos et al., 2009; Hikawa and Miyanaga, 2009; Nunes-Silva et al., 2013; Caro et al., 2017; Silva-Neto et al., 2019; Giannini et al., 2020; Layek et al., 2021), the large scale application of stingless bee species with this purpose is not yet as developed as for example honeybees and bumblebees (Roubik, 1995; Ramírez et al., 2018; Roubik et al., 2018). This could be a direct consequence of a general lack of knowledge about their biology and natural history. It is therefore important to understand basic aspects of their biology such as foraging activity patterns, as well as their viability to be managed prior to any potential application of stingless bee populations.
Studying the foraging patterns of bees can help to not only increase the knowledge about these important providers of ecosystem services, but also to better formulate beekeeping strategies such as colony density and proximity to both natural areas and crops. Bees tend to forage nearby their hives (Basari et al., 2018), usually ranging from a few meters to about 2 km away from their natal nests (Van Nieuwstadt and Iraheta, 1996; Araujo et al., 2004; Kuhn-Neto et al., 2009; Nunes-Silva et al., 2019). In natural conditions, colonies of a single species are usually located somewhat distant from each other with densities ranging between 0.014-16 hives/ha (Eltz et al., 2002; Silva and Ramalho, 2016). However, there is usually a large number of colonies aggregated next to each other in managed populations, resulting in increased competition for resources and high levels of orientation mistakes or “drifting” when foragers return to their hives. There is a wide variation in the rates of drifting behavior between species and/or the type of environment, being generally higher in managed in contrast to natural populations. For example, in bumblebees, drifting rates vary between 2.7% in natural or semi natural conditions to 28% in greenhouses (Birmingham and Winston, 2004; Takahashi et al., 2010; Zanette et al., 2014), while in honeybees drifting ranges from 1–5% in natural populations up to 42% in apiaries (Pfeiffer and Crailsheim, 1998; Nanork et al., 2005, 2007; Chapman et al.,2009a,b,c). This behavior can have major consequences to colony health since diseases and pathogens may spread across hives via drifted workers (Bordier et al., 2017; Nolan and Delaplane, 2017). Hence, it is a crucial factor to be considered in terms of both honey production and crop pollination.
As yet, many aspects related to foraging patterns and drifting behavior remain poorly understood in stingless bees. In this study, we used state-of-the-art radio frequency identification (RFID) tags to monitor the foraging behavior of the stingless bee Melipona fasciculata over with special interest in the drifting patterns between colonies. In particular, we tested how marking the colony entrances with different geometric patterns affected the drifting behavior. In addition, we tested if the position of the colonies had an influence in terms of the direction of the drifting rates and, finally, we correlated the data collected with the RFID system with meteorological data to understand how abiotic climatic factors affected both the bees’ lifespan and drifting rates.
Materials and Methods
Study Species and Experimental Design
This study was performed with the stingless bee Melipona fasciculata, which has its natural distribution in the northern region of Brazil (Pedro, 2014). The colonies used in the experiment were located at the meliponary of Eastern Amazon Embrapa, in an environment consisting of a mosaic of agricultural crops, forest remnants, and human habitations, where worker bees could forage freely on their expected range of about 2.5 km (Van Nieuwstadt and Iraheta, 1996; Araujo et al., 2004; Kuhn-Neto et al., 2009; Nunes-Silva et al., 2019). The climate at the site is characterized as tropical with daily mean precipitation of at least 60 mm throughout the year (Alvares et al., 2013).
To analyze the foraging and drifting patterns of M. fasciculata, eight experimental colonies were housed in identical hives designed for stingless bees and located in a shed consisting of two parallel rows with four colonies each that were placed 15 cm apart (Figure 1A). A plastic tube that extended the colony entrance and allowed the positioning of the antennae and microcomputer of the RFID system was placed in the front of the hive. The entire system was enclosed inside a box that protected entrance tubes from direct light in order to not disturb the forager’s behavior (Figure 1B). Young worker bees that were not yet foraging were randomly sampled from each hive to receive the RFID tags. Bees were tagged every week for 9 consecutive sessions with 40 workers tagged per week, amounting to 360 bees per colony and 2,880 in total. The process consisted in collecting the young workers in the early morning (8:00–9:00) and placing them in a tube with maximum 5 workers per tube prior to tagging them with the RFIDs. The RFID-tags were then glued with cyanoacrylate adhesive onto the worker thorax (Figure 2B) and, after all bees were marked and the glue sufficiently dried, they were returned to their original hive. Workers from M. fasciculata tolerated well the RFID-tags glued on their thorax without any apparent disturbance to their flight behavior (Nunes-Silva et al., 2019; Gomes et al., 2020; Costa et al., 2021). Finally, after 42 days of the beginning of the experiment, colonies received simple geometrical individual black and white markings made with electrical tape at their entrances to test whether foragers would then improve recognition of their own hive and drift to fewer foreign hives, i.e., make fewer orientation mistakes. The experiment ran for another 78 days after the marking of the colony entrances until the activity of tagged bees was no longer observed. Despite the unbalanced number of days before and after marking the colony entrances, the number of worker bees tracked during each period was similar (n = 1496 workers during control and n = 1,203 during the experimental period).
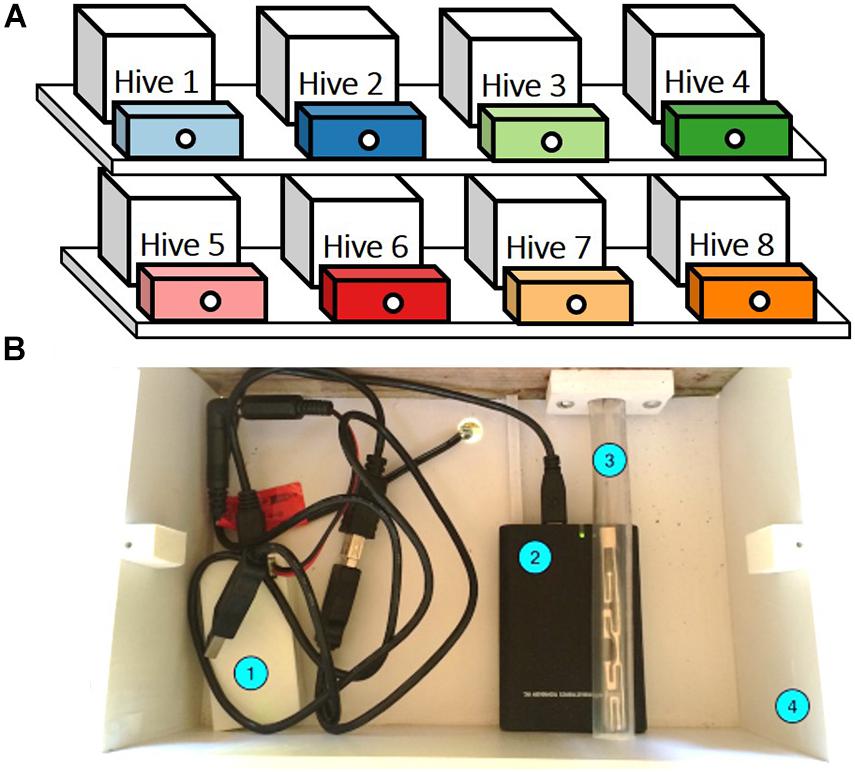
Figure 1. (A) Experimental setup scheme not to scale with four hives in the top shelf and four in the bottom shelf. The RFID systems were placed in a plastic box at the hive entrances. (B) Inside view of the entrance box containing the RFID system. 1, box containing the Intel Edison and Breakout Board; 2, RU-824 antenna; 3, tube connecting the hive to the entrance, and 4, protective plastic casing.
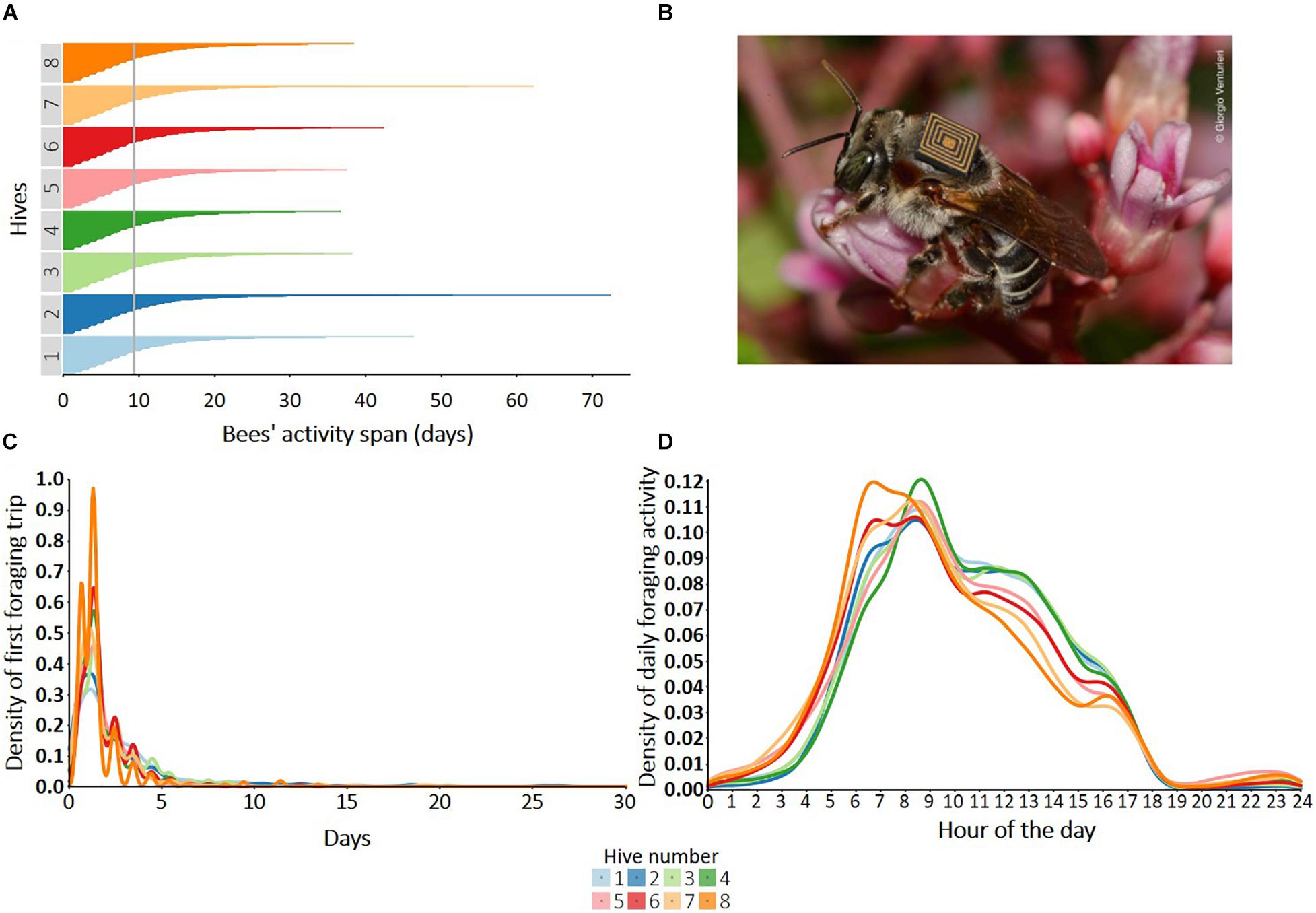
Figure 2. (A) Workers extranidal activity span based on the difference between the last reading on the RFID system and the day they were tagged. Different colonies are plotted on the y axis with every row corresponding to an individual bee sorted by activity span. The vertical gray line represents the mean activity of 9.3 days. (B) Picture of a M. fasciculata forager with a RFID tag on her thorax. Photo by GV. (C) Kernel density estimate plot of the bees’ first foraging trip calculated as the difference between the tagging data and the first record on the reader. (D) Kernel density estimate plot calculated by the overall frequency of reads per colony per day displaying the bees’ activity throughout the day. Peak activity was recorded between 5:00 to 10:00 in all colonies.
RFID-System Setup
This study was conducted using the Radio Frequency Identification System Ultra Small Package Tag (USPT) developed by Hitachi Chemical (Endou et al., 2014). The system consisted in a single antenna placed below the colony entrance tube connected to an Intel Edison micro-computer to store data (Figure 1B). Each tag was recorded with an individual ID that included the bee number and her colony of origin prior to being glued onto the bees. Therefore, whenever a tagged bee passed through the entrance tube both worker ID and time of the day were recorded. A caveat of the experimental design was that our system consisted of only one reader per colony, hence the signal sent to the computer did not inform the directionality of the bee’s movement (toward or away from the hive) which was then mitigated during data analysis. Moreover, guard bees staying by the colony entrance would have repeated readings over a short period of time. Hence, only signals that were at least 180 s apart were included in the analysis to resolve this issue. Thresholds between 60 s and 5 min are consistently used to filter RFID foraging data in Hymenoptera (Lach et al., 2015; Dosselli et al., 2016; Susanto et al., 2018; Santoro et al., 2019). This threshold was adopted during data filtering in order to reflect only the extranidal flight activity. We opted for this threshold because, while it might misrepresent short patrolling flights, it prevents overestimating their foraging activity.
Data Analysis
All statistical analyses were carried out using the R software (R Core Team, 2020). Data filtering and merging RFID and meteorological data was performed using a custom R script (available on data repository). Extranidal activity span of foragers was calculated based on the difference between the last recorded data and the date the bees were tagged (Decourtye et al., 2011; Tenczar et al., 2014; Perry et al., 2015; Dosselli et al., 2016; Santoro et al., 2019). First foraging trip was calculated with the difference between the first trip recorded and the tagging date and the kernel density estimates were calculated based on the smoothed histogram using the “geom_density” function in the R package ggplot2. Likewise, the daily foraging activity were also calculated using the density function in the package ggplot2. To analyze the influence that both biotic and abiotic factors have on the observed drifting rates we used a model selection approach using the package glmulti to select the best set of explanatory variables based on the models Akaike’s Information Criterion. The selected best model had drifting numbers coded as the dependent variable with activity span, hive ID, number of days to begin foraging as well as several meteorological factors coded as covariates with a Poisson error distribution. We then used the same approach to select a model with the bees’ lifespan coded as the dependent variable but used a quasipoisson error distribution do deal with overdispersion detected in this model. In addition, we tested whether the proportion of drifters present on the colonies was different before and after marking the colony entrances by fitting a binomial GLMM with the proportion of foragers that drifted to an unrelated colony as the dependent variable, colony marking (before or after) as a fixed factor and hive ID and an observation-level random effect variable to cope with overdispersion as random factors. Finally, we tested whether drifters had any preference on the direction they would drift. To this end, we ran a binomial GLM with the direction of the drifting event (i.e., horizontal and vertical) as the dependent variable, both the natal hives ID and the host hives ID as cofactors and individual IDs as a random factor. When appropriate, models were tested for temporal autocorrelation, which was not observed in the data. The R script used in the analyses as well as the original datasets are publicly available in the data repository (Oliveira et al., 2021). All models presented in the results section are Poisson GLMs unless otherwise specified. In addition, Wald Z Scores are shortened to Z throughout the text.
Results
Bees Extranidal Activity
Our results show that the tagged workers were active on average for 9.3 days, ranging from a minimum of 1.2 to a maximum of 72.5 days after being tagged (Figure 2A). In addition, bees began foraging on average 2 days after being tagged, with some more extreme cases where workers only started foraging after 25 days and beyond, as registered by the first reading of their tags at the colony entrance (Figure 2C). Foragers were active throughout the day, with most activity being recorded during the early morning hours (between 5:00–10:00), reaching the peak activity at 9:00 and then decreasing until 18:00 (Figure 2D). Furthermore, our data show that workers in colony four showed significantly less activity, while in colony one and two significantly more activity than the average was recorded (colony four: Z = −7.602, p < 0.001; colony one: Z = 2.373, p = 0.047; colony two: Z = 5.478, p < 0.001). Figure 3 illustrates the reconstructed foraging activity of all 2,880 bees during the 4-month study period.
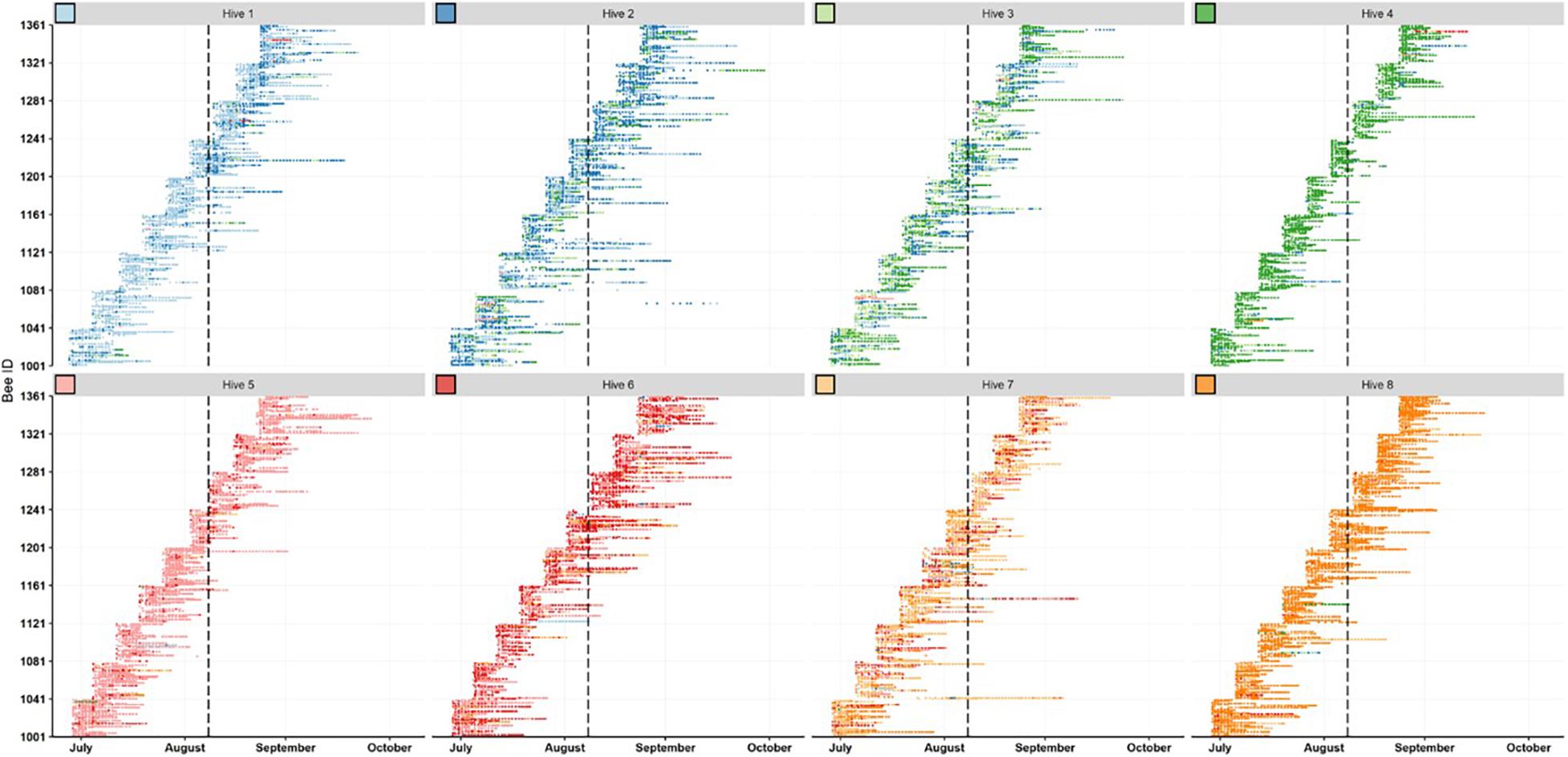
Figure 3. Foraging activity span of all bees during the 4-month study period. Each row represents the RFID scans of an individual bee, with the specific colors corresponding to their respective natal hives. Therefore, bees that matched the color with their nest of origin are natal workers whereas different colors within a hive represent the activity of foreign drifter workers. In total 2,880 bees were tagged in nine separate sessions demonstrated by the ladder-like appearance along the y axis. That is, at every session 40 tagged bees were introduced per colony. The vertical dashed line shows when the colony entrances were marked, 42 days after the beginning of the experiment. The experimental period ran for another 78 days until no tagged bees could be observed in the nest.
Factors Affecting Drifting Behavior and Foraging Activity
Throughout the study period, 64.1% of all tagged workers drifted to at least one other colony, with 36.6% drifting to only one, 19.1% to two, 7.6% to three foreign colonies, and the percentage decreasing below 1% as the number of foreign colonies increased up to a maximum of seven colonies, i.e., all non-natal experimental colonies (Figure 4A). It is interesting to note that the majority of the drifting events was in the horizontal plane, that is, workers mostly drifted to colonies on their left or right rather than above or below their natal hives (Binomial GLMM, Z > 8.568, p < 0.001 for all colonies, Figure 4B). Moreover, colonies placed on both edges produced fewer drifters than colonies placed between other hives. For instance, foragers in colonies two, three, six and seven showed significantly higher levels of drifting behavior (hive two: Z = 11.880, p < 0.001; hive three: Z = 11.499, p < 0.001; hive six: Z = 4.125, p < 0.001 and hive seven: Z = 3.514, p = 0.001) while hives four, five and eight had significant fewer drifters (hive four: Z = −5.746, p < 0.001; hive five: Z = −5.250, p < 0.001 and hive eight: Z = −9.716, p < 0.001), with hive one being not significant (Z = 0.181, p = 0.936). Furthermore, drifting rates were positively correlated with workers’ lifespan Z = 18.113, p < 0.001) and the sooner bees began foraging after being tagged the higher the observed drifting rates (Z = 7.494, p < 0.001).
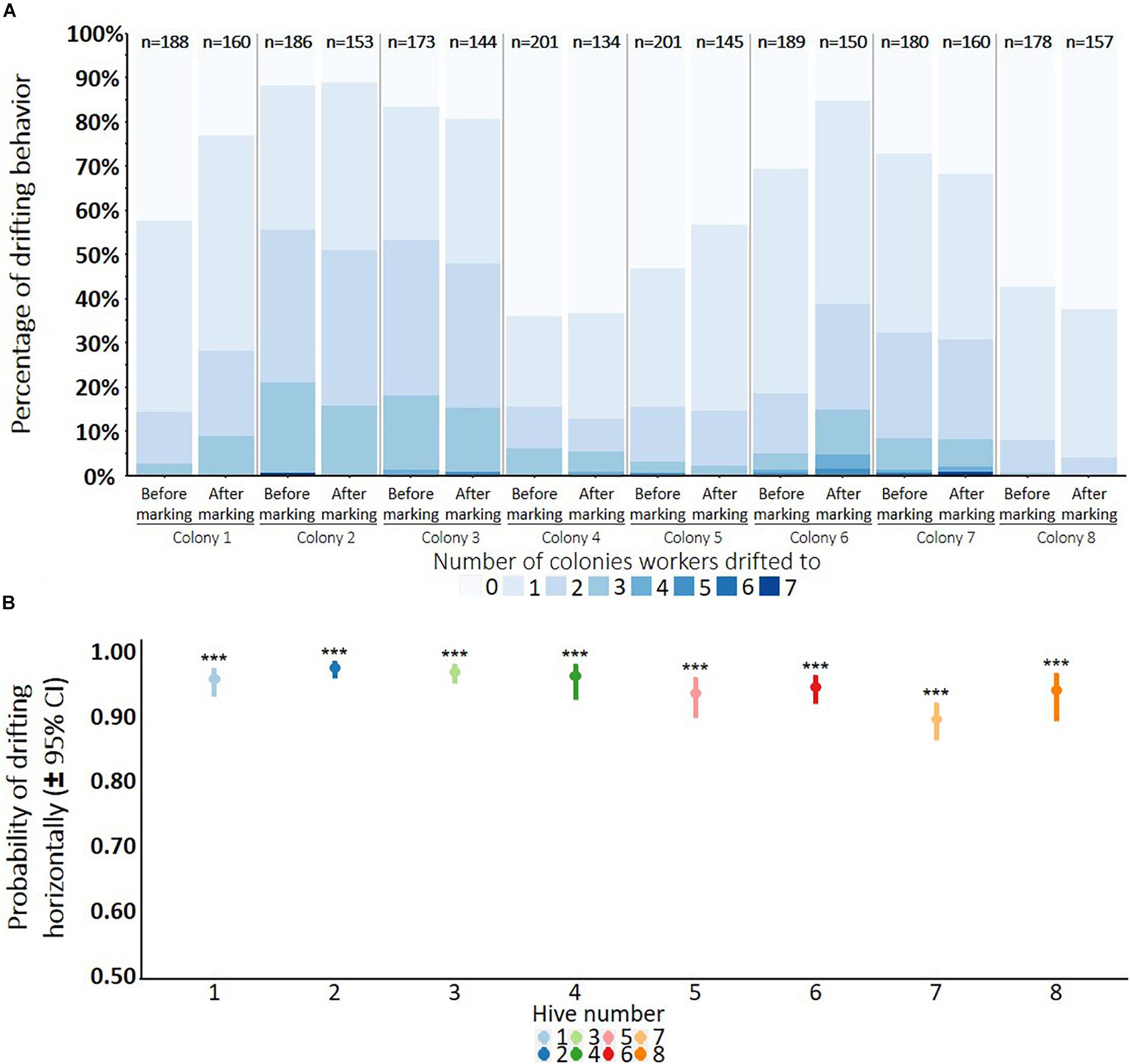
Figure 4. (A) Percentages of drifting events to different foreign colonies during the experimental period before and after marking the colony entrances. Hives placed in the middle sections of the shelves presented higher rates of drifting behavior with some drifters visiting all seven non-natal hives. n shows the number of tagged worker drifters per colony per period. (B) Drifting events were mostly in the horizontal plane, with on average 96% of the drifting events being to colonies placed on the same shelf than the natal hives.
In addition to biotic factors, several meteorological factors influenced the levels of drifting behavior, with dew point positively affecting the drifting rates (Z = 3.205, p = 0.001), while solar irradiation (Z = −2.804, p = 0.005), maximum relative humidity (Z = −2.883, p = 0.002) and minimum daily temperature (Z = −2.722, p = 0.006) were negatively correlated to the drifting rates.
During the experimental period colony entrances received individual markings to test whether foragers would then improve recognition of their own hive and drift to fewer foreign hives, i.e., make fewer orientation mistakes. Intriguingly, we observed an increase in the proportion of drifting events after marking the hive entrances, with 63.9% of tagged bees drifting before and 68.7% after the hive entrances were marked (Binomial GLMM, Z = 2.508, p = 0.012), which could indicate factors other than mere orientation mistakes playing a role in drifting behavior (Figure 4A).
Finally, it was possible to observe that meteorological factors were also correlated with the workers extranidal activity, whereby maximum daily atmospheric pressure and temperature, precipitation as well as dew point temperature were positively correlated with the bees activity span (max atmospheric pressure: Z = 6.521, p < 0.001; max temperature, Z = 5.236, p < 0.001; precipitation, Z = 3.020, p = 0.002; max dew point: Z = 2.504, p = 0.012; min dew point: (Z = 4.710, p < 0.001). Conversely, minimum daily temperature and atmospheric pressure, humidity and wind speed negatively affecting the bees’ activity (min temperature: Z = −8.834, p < 0.001; min atmospheric pressure, Z = −6.922, p < 0.001; max humidity: Z = −4.235, p < 0.001; min humidity: Z = −3.156, p = 0.001; and wind speed: Z = −9.901, p < 0.001). Table 1 summarizes the relationship of abiotic factors with both drifting behavior and foraging activity.
Discussion
By reconstructing their daily foraging activity of the stingless bee M. fasciculata, we could observe that bees forage during the entire day, with the average peak activity per colony at 9:00 in the morning (Figure 2D). In contrast, studies using similar RFID technology show that both honeybees and bumblebees have their peak foraging activity at noon, even during a permanent daylight condition in the arctic circle (Stelzer and Chittka, 2010; Susanto et al., 2018). Similarly to honeybees, stingless bee workers perform different tasks along their lives, from taking care of the young and cleaning the colony soon after emerging, to carrying out more dangerous tasks such as defending the hive and foraging toward the end of their lives with some degree of specialization in certain tasks (Mateus et al., 2019). In the congeneric M. beecheii it was shown that some foragers collected mostly pollen whereas some others were specialized in foraging for nectar, with great impact both in their daily activity and lifespan. Nectar foragers were active during the entire day but died approximately 3 days after they began foraging, while pollen foragers were only active for 1–3 h in the early morning but lived on average 9 days after they started foraging (Biesmeijer and Tóth, 1998). These patterns could explain the differences observed in our experiments, where we detected a wide variation in their activity span (1.2–72.5 days). Despite the average extranidal activity span observed in our experiment being seemingly short (9.3 days), it is in line with a similar experiment performed with RFID system in honeybees in which individuals in control colonies lived on average 12.2 days after being introduced to the hives (Thompson et al., 2016). In addition, even though we did not quantify the precise age of the workers, Melipona bees usually start foraging around 25–33 days after emergence (Biesmeijer and Tóth, 1998; Mateus et al., 2019), hence we can estimate the life expectance of the bees in our experiments approximately between 25 and 105 days, which is consistent with what is found in the literature for M. fasciculata and other species of this genus (Grüter, 2020b).
For most social insects, life-threatening challenges increase when workers leave the security of their nests and start their foraging activity. Outside the nest they face an increased chance of predation, death by the elements (e.g., storms) or by exhaustion (Roubik, 1982; Visscher and Dukas, 1997; Gomes et al., 2015). Indeed, we observed some abiotic factors having strong effects on the bees’ activity, notably temperature, atmospheric pressure, and wind speed. An increase in the average daily maximum temperature by one degree during the bee’s lifespan corresponded to an increased activity span of 1.7 days. On the other hand, an increase in the minimum daily temperature had the opposite effect, decreasing the bee’s activity span by 3.3 days. A possible explanation for this observation is that while bees benefit from higher temperatures during daily foraging activity, the same was not true when they were inside their hives during the night, when the minimum temperatures were recorded. A similar pattern was observed for average maximum and minimum atmospheric pressure, where the maximum recorded values had a positive effect whereas minimum values had a negative effect on the bees’ foraging activity span. Finally, average recorded wind speed had a negative impact on their survival, likely by impairing the bee’s flight ability (Crall et al., 2017). Even though further studies are still needed to fully comprehend how climate factors affect the bees’ behavior, our results show that this species is highly susceptible to variations in climate factors with relatively small fluctuations having a significant impact in their lifespan, demonstrating that even small changes in the future climate might cause notable implications in their extranidal activities.
In terms of the drifting behavior, our results show that 64% of the tagged workers drifted to at least one foreign hive, and that some of them were recorded entering all seven foreign hives (Figure 3A). Bees use a combination of landmarks and polarized light to orient themselves in the environment, naturally experiencing some degree of error while returning to their nests (Rossel and Wehner, 1982; Kraft et al., 2011; Kheradmand and Nieh, 2019). The overall low density of nests in natural conditions likely sustain no strong selective pressure for higher accuracy. Nevertheless, high rates of drifting behavior are not uncommonly observed in apiaries (Free, 1958; Free and Spencer-Booth, 1961; Pfeiffer and Crailsheim, 1998), which is likely caused by the high density of hives next to each other, resulting in a larger proportion of orientation mistakes. This happens particularly when resources are abundant and guard bees become more permissive to the infiltration of non-nestmates in their hives (Pfeiffer and Crailsheim, 1998; Downs and Ratnieks, 2000). Although the levels of drifting behavior observed are likely mainly due to orientation mistakes, nest robbing or social parasitism cannot be completely ruled out, since we observed an increased proportion of drifting behavior after marking the colony entrances which presumably increased the bees ability to recognize their own colony (Plowright et al., 1995). In fact, worker social parasitism is well documented in both honeybees (Nanork et al., 2005, 2007; Chapman et al.,2009a,b, c) and bumblebees (Birmingham and Winston, 2004; Birmingham et al., 2004; Takahashi et al., 2009; Zanette et al., 2014), as well as in other social insect species including wasps (Oliveira et al., 2016). An alternative explanation for the increased proportion of drifting after marking the colony entrances could be linked with bees merely not recognizing their hive entrances. Nevertheless, this is unlikely since about half of the tagged bees (n = 1203) began foraging only after the experimental manipulation i.e., they had no prior interaction with the unmarked colony entrances. Whether workers indeed actively drift into foreign colonies and how they manage to avoid being detected as non-nestmates and attacked by guards still deserves further study.
An interesting outcome of our experimental design is the fact the nearly all drifting events took place horizontally, i.e., foragers drifted almost exclusively to colonies placed in the same shelf as their natal hive rather than above or below, and that hives placed in the center of the rows produced more drifters, similarly to what is observed in honeybees (Pfeiffer and Crailsheim, 1998). In contrast, drifting occurred preferentially in the vertical plane in two species of bumblebees, with colonies placed on top significantly receiving more drifter workers (Birmingham and Winston, 2004). These differences in the orientation of the drifting behavior are possibly due to particularities in nesting biology since bumblebees usually nest underground (Kells and Goulson, 2003), while honeybees and most stingless bee species built their nests in tree cavities or branches (Roubik, 2006; Hepburn et al., 2014). Therefore, honeybees and stingless bees would benefit more from higher accuracy in finding their nests in the vertical plane whereas bumblebees would have a stronger selective pressure toward accuracy in the vertical plane. Our finding demonstrates that the spatial distribution of colonies has important management implications for stingless bee populations. Furthermore, our results also suggest that other factors other than the position of the hives played a role in the rates of drifting behavior. In one hand, the average dew point temperature was observed to be positively correlated to the drifting levels, possible because most foraging activity happens in the early morning hours and a higher temperature overall could be linked with higher metabolic activity. On the other hand, factors like solar irradiation, maximum humidity and minimum daily temperatures were shown to negatively impact drifting rates. These factors are usually linked with lower foraging activity (de Figueiredo-Mecca et al., 2013), which could explain the reduced rates of drifting merely as an outcome of fewer foraging trips.
Stingless bees present great potential to be used in commercial crop pollination (Heard, 1999; Slaa et al., 2006; Giannini et al., 2020; Layek et al., 2021). Indeed, Melipona bees have been demonstrated to be efficient pollinators of many economically important fruits and vegetables (Cruz et al., 2005; Bispo dos Santos et al., 2009; Nunes-Silva et al., 2013; Caro et al., 2017; Silva-Neto et al., 2019). A recent study using the RFID technology with the stingless bee M. fasciculata showed that workers of this species can return to their nests from distances up to 10 km away from their hives (Nunes-Silva et al., 2019), suggesting that these bees could be well suited for pollination of large scale plantations as well.
Overall, this study presents data on the foraging activity and drifting patterns of the stingless bee M. fasciculata, showing the impact of the presence of hives nearby as well as several abiotic factors on both their lifespan and the rates of drifting behavior. This is an important step toward a better understanding of stingless bees’ biology, providing insights on how some factors might affect their application as pollinators in crops as well as in natural areas.
Data Availability Statement
The original contributions presented in the study are included in the article/supplementary material, further inquiries can be directed to the corresponding author/s.
Author Contributions
VI-F, FC, and RJ had the original idea. FC, HA, RJ, LC, GP, and GV performed the experiments. PS provided the RFID system for the experiments. RO analyzed the data and wrote the first draft of the manuscript. All authors revised and approved the final version of the manuscript.
Funding
This project was funded by the Brazilian National Council for Scientific and Technological Development CNPq, grant 444384/2018-9; and by individual research grants to VLIF (CNPq 312250/2018-5), RF (CNPq 301616/2017-5) and RCO (Research Foundation Flanders FWO research Grant 1502119N and postdoctoral Grant 12R9619N).
Conflict of Interest
The authors declare that the research was conducted in the absence of any commercial or financial relationships that could be construed as a potential conflict of interest.
Publisher’s Note
All claims expressed in this article are solely those of the authors and do not necessarily represent those of their affiliated organizations, or those of the publisher, the editors and the reviewers. Any product that may be evaluated in this article, or claim that may be made by its manufacturer, is not guaranteed or endorsed by the publisher.
Acknowledgments
We would like to thank Elisângela Rêgo for helping to tag the bees.
References
Alvares, C. A., Stape, J. L., Sentelhas, P. C., de Moraes Gonçalves, J. L., and Sparovek, G. (2013). Köppen’s climate classification map for Brazil. Meteorologische Zeitschrift 22, 711–728. doi: 10.1127/0941-2948/2013/0507
Araujo, E. D., Costa, M., Chaud-Netto, J., and Fowler, H. G. (2004). Body size and flight distance in stingless bees (Hymenoptera: Meliponini): Inference of flight range and possible ecological implications. Braz. J. Biol. 64, 563–568. doi: 10.1590/s1519-69842004000400003
Basari, N., Ramli, S. N., and Mohd Khairi, N. S. (2018). Food reward and distance influence the foraging pattern of stingless bee, Heterotrigona itama. Insects 9:138. doi: 10.3390/insects9040138
Biesmeijer, J., and Tóth, E. (1998). Individual foraging, activity level and longevity in the stingless bee Melipona beecheii in costa rica (Hymenoptera, apidae, meliponinae). Ins. soc. 45, 427–443. doi: 10.1007/s000400050099
Birmingham, A. L., Hoover, S. E., Winston, M. L., and Ydenberg, R. C. (2004). Drifting bumble bee (Hymenoptera: Apidae) workers in commercial greenhouses may be social parasites. Can. J. Zool. 82, 1843–1853. doi: 10.1139/z04-181
Birmingham, A. L., and Winston, M. L. (2004). Orientation and drifting behaviour of bumblebees (Hymenoptera: Apidae) in commercial tomato greenhouses. Can. J. Zool. 82, 52–59. doi: 10.1139/z03-201
Bispo dos Santos, S. A., Roselino, A. C., Hrncir, M., and Bego, L. R. (2009). Pollination of tomatoes by the stingless bee Melipona quadrifasciata and the honey bee Apis mellifera (Hymenoptera, Apidae). Gen. Mol. Res. 8:751. doi: 10.4238/vol8-2kerr015
Bordier, C., Pioz, M., Crauser, D., Le Conte, Y., and Alaux, C. (2017). Should I stay of should I go: Honeybee drifting behaviour as a function of parasitism. Apidologie 48, 286–297. doi: 10.1007/s13592-016-0475-1
Caro, A., Moo-Valle, H., Alfaro, R., and Javier, Q. E. (2017). Pollination services of africanized honey bees and native melipona beecheii to buzz-pollinated annatto (Bixa orellana l.) in the neotropics. Agric. For. Entomol. 19, 274–280. doi: 10.1111/afe.12206
Chapman, N. C., Beekman, M., and Oldroyd, B. P. (2009c). Worker reproductive parasitism and drift in the western honeybee Apis mellifera. Behav. Ecol. Sociobiol. 64, 419–427. doi: 10.1007/s00265-009-0858-7
Chapman, N. C., Higgs, J. S., Wattanachaiyingcharoen, W., Beekman, M., and Oldroyd, B. P. (2009b). Worker reproductive parasitism in naturally orphaned colonies of the asian red dwarf honey bee, Apis florea. Ins. soc. 57, 163–167. doi: 10.1007/s00040-009-0061-x
Chapman, N. C., Nanork, P., Gloag, R. S., Wattanachaiyingcharoen, W., Beekman, M., and Oldroyd, B. P. (2009a). Queenless colonies of the asian red dwarf honey bee (Apis florea) are infiltrated by workers from other queenless colonies. Behav. Ecol. 20, 817–820. doi: 10.1093/beheco/arp065
Core Team, R. (2020). R: A Language and Environment for Statistical Computing. Austria: R Foundation for Statistical Computing.
Cortopassi-Laurino, M., Imperatriz-Fonseca, V. L., Roubik, D. W., Dollin, A., Heard, T., Aguilar, I., et al. (2006). Global meliponiculture: Challenges and opportunities. Apidologie 37, 275–292. doi: 10.1051/apido:2006027
Costa, L., Nunes-Silva, P., Galaschi-Teixeira, J., Arruda, H., Veiga, J., Pessin, G., et al. (2021). RFID-tagged Amazonian stingless bees confirm that landscape configuration and nest re-establishment time affect homing ability. Ins soc. 68, 1–8. doi: 10.1007/978-3-319-90306-4_147-1
Crall, J. D., Chang, J. J., Oppenheimer, R. L., and Combes, S. A. (2017). Foraging in an unsteady world: Bumblebee flight performance in field-realistic turbulence. Interface Focus 7:20160086. doi: 10.1098/rsfs.2016.0086
Cruz, D. O., Freitas, B. M., Silva, L. A., Silva, E. M. S., and Bomfim, I. G. A. (2005). Pollination efficiency of the stingless bee Melipona subnitida on greenhouse sweet pepper. Pesqu. Agropecu. Bras. 40, 1197–1201. doi: 10.1590/s0100-204x2005001200006
de Figueiredo-Mecca, G., Bego, L. R., and do Nascimento, F. S. (2013). Foraging behavior of Scaptotrigona depilis (Hymenoptera, Apidae, Meliponini) and its relationship with temporal and abiotic factors. Sociobiology 60, 267–282.
Decourtye, A., Devillers, J., Aupinel, P., Brun, F., Bagnis, C., Fourrier, J., et al. (2011). Honeybee tracking with microchips: A new methodology to measure the effects of pesticides. Ecotoxicology 20, 429–437. doi: 10.1007/s10646-011-0594-4
Del Sarto, M. C. L., Peruquetti, R. C., and Campos, L. A. O. (2005). Evaluation of the neotropical stingless bee Melipona quadrifasciata (Hymenoptera: Apidae) as pollinator of greenhouse tomatoes. J. Econ. Entomol. 98, 260–266. doi: 10.1093/jee/98.2.260
Dosselli, R., Grassl, J., Carson, A., Simmons, L. W., and Baer, B. (2016). Flight behaviour of honey bee (Apis mellifera) workers is altered by initial infections of the fungal parasite Nosema apis. Sci. Rep. 6, 1–11.
Downs, S. G., and Ratnieks, F. L. W. (2000). Adaptive shifts in honey bee (Apis mellifera l.) guarding behavior support predictions of the acceptance threshold model. Behav. Ecol. 11, 326–333. doi: 10.1093/beheco/11.3.326
Eltz, T., Bruhl, C. A., van der Kaars, S., and Linsenmair, E. K. (2002). Determinants of stingless bee nest density in lowland dipterocarp forests of sabah, Malaysia. Oecologia 131, 27–34. doi: 10.1007/s00442-001-0848-6
Endou, T., and Ishizaka, Hironori, Oota, Masahiko, Tasaki, Kouji, Hosoi, Hiroyuki, Company, H. C. (eds) (2014). RFID Tag and Automatic Recognition System. United States: WIPO.
Free, J. (1958). The drifting of honey-bees. J. Agricult. Sci. 51, 294–306. doi: 10.1017/s0021859600035103
Free, J., and Spencer-Booth, Y. (1961). Further experiments on the drifting of honey-bees. J. Agricult. Sci. 57, 153–158. doi: 10.1017/s0021859600047626
Giannini, T. C., Alves, D. A., Alves, R., Cordeiro, G. D., Campbell, A. J., Awade, M., et al. (2020). Unveiling the contribution of bee pollinators to brazilian crops with implications for bee management. Apidologie 51, 1–16.
Gomes, P. A., Suhara, Y., Nunes-Silva, P., Costa, L., Arruda, H., Venturieri, G., et al. (2020). An Amazon stingless bee foraging activity predicted using recurrent artificial neural networks and attribute selection. Sci. Rep. 10, 1–12.
Gomes, R. L. C., Menezes, C., and Contrera, F. A. L. (2015). Worker longevity in an Amazonian Melipona (Apidae, Meliponini) species: Effects of season and age at foraging onset. Apidologie 46, 133–143. doi: 10.1007/s13592-014-0309-y
Heard, T. A. (1999). The role of stingless bees in crop pollination. Annu. Rev. Entomol. 44, 183–206. doi: 10.1146/annurev.ento.44.1.183
Hepburn, H., Pirk, C., and Duangphakdee, O. (2014). Honeybee nests Composition, structure, function. Springer 978, 642–648.
Hikawa, M., and Miyanaga, R. (2009). Effects of pollination by Melipona quadrifasciata (Hymenoptera: Apidae)on tomatoes in protected culture. Appl. Entomol. Zool. 44, 301–307. doi: 10.1303/aez.2009.301
Kells, A. R., and Goulson, D. (2003). Preferred nesting sites of bumblebee queens (hymenoptera: Apidae) in agroecosystems in the UK. Biol. Conserv. 109, 165–174. doi: 10.1016/s0006-3207(02)00131-3
Kheradmand, B., and Nieh, J. C. (2019). The role of landscapes and landmarks in bee navigation: A review. Insects 10:342. doi: 10.3390/insects10100342
Kraft, P., Evangelista, C., Dacke, M., Labhart, T., and Srinivasan, M. (2011). Honeybee navigation: Following routes using polarized-light cues. Philos. Trans. R. Soc. B Biol. Sci. 366, 703–708. doi: 10.1098/rstb.2010.0203
Kuhn-Neto, B., Contrera, F. A., Castro, M. S., and Nieh, J. C. (2009). Long distance foraging and recruitment by a stingless bee, Melipona mandacaia. Apidologie 40, 472–480. doi: 10.1051/apido/2009007
Lach, L., Kratz, M., and Baer, B. (2015). Parasitized honey bees are less likely to forage and carry less pollen. J. Invertebr. Pathol. 130, 64–71. doi: 10.1016/j.jip.2015.06.003
Layek, U., Kundu, A., Bisui, S., and Karmakar, P. (2021). Impact of managed stingless bee and western honey bee colonies on native pollinators and yield of watermelon: A comparative study. Ann. Agricult. Sci. 66, 38–45. doi: 10.1016/j.aoas.2021.02.004
Mateus, S., Ferreira-Caliman, M., Menezes, C., and Grüter, C. (2019). Beyond temporal-polyethism: Division of labor in the eusocial bee melipona marginata. Ins. soc. 66, 317–328. doi: 10.1007/s00040-019-00691-2
Nanork, P., Chapman, N. C., Wongsiri, S., Lim, J., Gloag, R. S., and Oldroyd, B. P. (2007). Social parasitism by workers in queenless and queenright Apis cerana colonies. Mol. Ecol. 16, 1107–1114. doi: 10.1111/j.1365-294x.2006.03207.x
Nanork, P., Paar, J., Chapman, N. C., Wongsiri, S., and Oldroyd, B. P. (2005). Entomology: Asian honeybees parasitize the future dead. Nature 437:829. doi: 10.1038/437829a
Nolan, M. P., and Delaplane, K. S. (2017). Distance between honey bee Apis mellifera colonies regulates populations of Varroa destructor at a landscape scale. Apidologie 48, 8–16. doi: 10.1007/s13592-016-0443-9
Nunes-Silva, P., Costa, L., Campbell, A. J., Arruda, H., Contrera, F. A. L., Teixeira, J. S. G., et al. (2019). Radiofrequency identification (RFID) reveals long-distance flight and homing abilities of the stingless bee Melipona fasciculata. Apidologie 51, 240–253. doi: 10.1007/s13592-019-00706-8
Nunes-Silva, P., Hrncir, M., da Silva, C. I., Roldão, Y. S., and Imperatriz-Fonseca, V. L. (2013). Stingless bees, melipona fasciculata, as efficient pollinators of eggplant (solanum melongena) in greenhouses. Apidologie 44, 537–546. doi: 10.1007/s13592-013-0204-y
Oliveira, R. C., Andrés León, Contrera, F., Arruda, H., Jaffé, R., Costa, L., et al. (2021). Foraging and drifting patterns of a highly eusocial neotropical stingless bee assessed by radio-frequency identification tags. Mendeley Data [Preprint]. doi: 10.21203/rs.3.rs-162274/v1
Oliveira, R. C., Oi, C. A., Vollet-Neto, A., and Wenseleers, T. (2016). Intraspecific worker parasitism in the common wasp, Vespula vulgaris. Anim. Behav. 113, 79–85. doi: 10.1016/j.anbehav.2015.12.025
Orr, M. C., Hughes, A. C., Chesters, D., Pickering, J., Zhu, C. D., and Ascher, J. S. (2021). Global patterns and drivers of bee distribution. Curr. Biol. 31:e4.
Pedro, S. R. M. (2014). The stingless bee fauna in Brazil (Hymenoptera: Apidae). Sociobiology 61, 348–354.
Perry, C. J., Søvik, E., Myerscough, M. R., and Barron, A. B. (2015). Rapid behavioral maturation accelerates failure of stressed honey bee colonies. Proc. Natl. Acad. Sci. U. S. A. 112, 3427–3432. doi: 10.1073/pnas.1422089112
Pfeiffer, K. J., and Crailsheim, K. (1998). Drifting of honeybees. Ins. soc. 45, 151–167. doi: 10.1007/s000400050076
Plowright, C., O’Connell, C. E., Roberts, L. J., and Reid, S. L. (1995). The use of proximal and distal cues in nest entrance recognition by bumble bees. J. Apic. Res. 34, 57–64. doi: 10.1080/00218839.1995.11100888
Quezada-Euán, J. J. G., Nates-Parra, G., Maués, M. M., Roubik, D. W., and Imperatriz-Fonseca, V. L. (2018). The economic and cultural values of stingless bees (Hymenoptera: Meliponini) among ethnic groups of tropical America. Sociobiology 65, 534–557. doi: 10.13102/sociobiology.v65i4.3447
Ramírez, V. M., Ayala, R., and González, H. D. (2018). Crop Pollination by Stingless Bees. In: Pot-Pollen in Stingless Bee Melittology. Germany: Springer.
Rossel, S., and Wehner, R. (1982). The bee’s map of the e-vector pattern in the sky. Proc. Natl. Acad. Sci. U. S. A. 79, 4451–4455. doi: 10.1073/pnas.79.14.4451
Roubik, D., Heard, T., and Kwapong, P. (2018). “Stingless bee colonies and pollination” in The Pollination of Cultivated Plants. A Compendium for Practitioners. (Ed). D. W. Roubik (United Nation: Food and Agricultural organization of the United Nations). 2, 39–64.
Roubik, D. W. (1982). Seasonality in colony food storage, brood production and adult survivorship: Studies of Melipona in tropical forest (Hymenoptera: Apidae). J. Kans. Entomol. Soc. 55, 789–800.
Roubik, D. W. (1995). Pollination of Cultivated Plants in the Tropics. Pollination of Cultivated Plants in the Tropics. United Nation: Food and Agriculture Organization.
Roubik, D. W. (2006). Stingless bee nesting biology. Apidologie 37, 124–143. doi: 10.1051/apido:2006026
Santoro, D., Hartley, S., and Lester, P. J. (2019). Behaviourally specialized foragers are less efficient and live shorter lives than generalists in wasp colonies. Sci. Rep. 9, 1–10.
Silva, M. D., and Ramalho, M. (2016). The influence of habitat and species attributes on the density and nest spacing of a stingless bee (Meliponini) in the Atlantic rainforest. Sociobiology 63, 991–997. doi: 10.13102/sociobiology.v63i3.1037
Silva-Neto, C. D. M. E., Ribeiro, A. C. C., Gomes, F. L., Melo, APCd, Oliveira, GMd, Faquinello, P., et al. (2019). The stingless bee mandaçaia (Melipona quadrifasciata Lepeletier) increases the quality of greenhouse tomatoes. J. Apic. Res. 58, 9–15. doi: 10.1080/00218839.2018.1494913
Slaa, E. J., Chaves, L. A. S., Malagodi-Braga, K. S., and Hofstede, F. E. (2006). Stingless bees in applied pollination: Practice and perspectives. Apidologie 37, 293–315. doi: 10.1051/apido:2006022
Stelzer, R. J., and Chittka, L. (2010). Bumblebee foraging rhythms under the midnight sun measured with radiofrequency identification. BMC Biol. 8:93. doi: 10.1186/1741-7007-8-93
Susanto, F., Gillard, T., De Souza, P., Vincent, B., Budi, S., Almeida, A., et al. (2018). Addressing RFID misreadings to better infer bee hive activity. IEEE Access 6, 31935–31949. doi: 10.1109/access.2018.2844181
Takahashi, J.-I., Martin, S. J., Ono, M., and Shimizu, I. (2009). Male production by non-natal workers in the bumblebee, Bombus deuteronymus (hymenoptera: Apidae). J. Ethol. 28, 61–66. doi: 10.1007/s10164-009-0155-y
Takahashi, J.-I., Martin, S. J., Ono, M., and Shimizu, I. (2010). Male production by non-natal workers in the bumblebee, Bombus deuteronymus (hymenoptera: Apidae). J. Ethol. 28, 61–66. doi: 10.1007/s10164-009-0155-y
Tenczar, P., Lutz, C. C., Rao, V. D., Goldenfeld, N., and Robinson, G. E. (2014). Automated monitoring reveals extreme interindividual variation and plasticity in honeybee foraging activity levels. Anim. Behav. 95, 41–48. doi: 10.1016/j.anbehav.2014.06.006
Thompson, H., Coulson, M., Ruddle, N., Wilkins, S., and Harkin, S. (2016). Thiamethoxam: Assessing flight activity of honeybees foraging on treated oilseed rape using radio frequency identification technology. Environ. Toxicol. Chem. 35, 385–393. doi: 10.1002/etc.3183
Van Nieuwstadt, M., and Iraheta, C. R. (1996). Relation between size and foraging range in stingless bees (Apidae, Meliponini). Apidologie 27, 219–228. doi: 10.1051/apido:19960404
Visscher, P., and Dukas, R. (1997). Survivorship of foraging honey bees. Ins. soc. 44, 1–5. doi: 10.1007/s000400050017
Keywords: stingless bee, radio-frequency identification, Melipona fasciculata, foraging activity, drifting behavior
Citation: Oliveira RC, Contrera FAL, Arruda H, Jaffé R, Costa L, Pessin G, Venturieri GC, de Souza P and Imperatriz-Fonseca VL (2021) Foraging and Drifting Patterns of the Highly Eusocial Neotropical Stingless Bee Melipona fasciculata Assessed by Radio-Frequency Identification Tags. Front. Ecol. Evol. 9:708178. doi: 10.3389/fevo.2021.708178
Received: 11 May 2021; Accepted: 15 July 2021;
Published: 04 August 2021.
Edited by:
Isabel Marques, University of Lisbon, PortugalReviewed by:
M. N. Kuperman, Bariloche Atomic Centre (CNEA), ArgentinaJose Octavio Macias-Macias, University of Guadalajara, Mexico
William G. Meikle, Agricultural Research Service, United States Department of Agriculture, United States
Copyright © 2021 Oliveira, Contrera, Arruda, Jaffé, Costa, Pessin, Venturieri, de Souza and Imperatriz-Fonseca. This is an open-access article distributed under the terms of the Creative Commons Attribution License (CC BY). The use, distribution or reproduction in other forums is permitted, provided the original author(s) and the copyright owner(s) are credited and that the original publication in this journal is cited, in accordance with accepted academic practice. No use, distribution or reproduction is permitted which does not comply with these terms.
*Correspondence: Ricardo Caliari Oliveira, cmljYXJkby5vbGl2ZWlyYUBldm9iaW8uZXU=
†These authors have contributed equally to this work