- 1Past Landscape Dynamics Laboratory, Institute of Geography and Spatial Organization, Polish Academy of Sciences, Warszawa, Poland
- 2Climate Change Ecology Research Unit, Institute of Geoecology and Geoinformation, Adam Mickiewicz University, Poznań, Poland
- 3Institute of Nuclear Physics, Polish Academy of Sciences, Kraków, Poland
- 4Institute of Geological Sciences, Polish Academy of Sciences, Warszawa, Poland
- 5Institute of Botany and Landscape Ecology, Greifswald, Germany
- 6Department of Environmental Resources and Geohazards, GIS and Cartography Workgroup, Institute of Geography and Spatial Organization, Polish Academy of Sciences, Warszawa, Poland
Global warming has compelled to strengthen the resilience of European forests. Due to repeated droughts and heatwaves, weakened trees become vulnerable to insect outbreaks, pathogen invasions, and strong winds. This study combines high-resolution analysis of a 100-year-old high-resolution peat archive synthesized from the Martwe peatland in Poland with remote sensing data. We present the first REVEALS based vegetation reconstruction in a tornado-hit area from Poland on the background of previous forest management in monocultural even-aged stands – Tuchola Pinewoods. During the 20th century, the pine monocultures surrounding the peatland were affected by clear-cutting and insect outbreaks. In 2012, a tornado, destroyed ca. 550 ha of pine forest around the peatland. The palynological record reflects these major events of the past 100 years as well as changes in forest practices. Our study showed the strong relationships between the decrease of Pinus sylvestris (Scots pine) in palynological record as well as planting patterns after the tornado. Moreover, past forestry practices [such as domination of Pinus sylvestris, the collapse of Picea abies (Norway spruce), low share of Betula spec. (birch) due to Pinus sylvestris promotion and probable also to a lesser by removal of Betula as a “forest weed,” and low plant coverage of tree species due to clear-cutting and cutting after insect outbreaks] were well identified in the proxy record. In monocultures managed over decades, the reconstruction of vegetation may be challenging due to changes in the age composition of the Pinus sylvestris stands. We found that through historical, remote sensing, and paleoecological data, the dynamics of disturbances such as insect outbreaks and tornadoes, as well as the changing perceptions of local society about forests, can be determined.
Introduction
Forest disturbance by storms, including tornadoes, is a rare but important problem that needs to be considered in the development of forest management strategies (Dobrowolska, 2015; Szmyt and Dobrowolska, 2016). Over the past decades, the frequency of extreme events such as storms and tornadoes has increased across Europe (Seidl et al., 2014) and is forecasted to increase further in the future (Senf and Seidl, 2021). Atmospheric phenomena that can damage forests, such as strong winds and tornadoes, are difficult to predict. However, it is important to understand the complex interactions between the damage caused by extreme events, the resilience of forest communities, climate change, and forest management due to the increased extent of forest destruction by the wind in recent years in Europe (Gardiner et al., 2010; Seidl et al., 2014; Gregow et al., 2017). Moreover, observations from the last decades indicate that the future will witness more extreme events that will not just affect the forests. These events include heatwaves, which will increase the possibility of fires (Ummenhofer and Meehl, 2017; Brando et al., 2019). The European monocultures are expected to be more sensitive to drought, and hence, the exposure of forests to pathogens, insect outbreaks, and strong winds (Leuschner and Ellenberg, 2017; Seidl et al., 2017, 2020).
Wind disturbances were more frequent in the European forests in the last decades (Seidl et al., 2017). During 1950–2010, more than 130 separate storms occurred, causing significant damage to forests (Gardiner et al., 2010). Huge economic losses resulted from cyclone Gudrun in 2005 and extratropical cyclones in Sweden in 1969 (wood loss of 42.2 mln m3), Lothar and Martin storm in 15 European states in 1990 (more than 240 mln m3), and cyclone Klaus in France, Spain, Portugal, and Italy in 2009 (43.1 mln m3) (Jantz, 1971; Gardiner et al., 2010). Furthermore, tornadoes of F2 or higher intensity can harm and uproot trees (Fujita, 1971). Across Europe, 9,529 tornadoes have been recorded between 1800 and 2014 CE (Groenemeijer and Kühne, 2014; European Severe Weather Database, 2020), with the actual number predicted to be higher (Shikhov and Chernokulsky, 2018).
Our study is focused on the Tuchola Pinewoods, which are one of the largest forest complexes in Poland. The current forest composition includes pine monocultures (plantations) in even-aged stands, introduced in the second half of the 18th century by Prussian forestry (Gietkowski, 2009). At present, Pinus sylvestris (Scots pine) is the dominant species, occupying more than 95% of the forest area, and is accompanied by other species such as Betula (birch) (1.8%), Quercus (oak) (1.1%), Alnus glutinosa (black alder) (1.0%), and Picea abies (Norway spruce) (0.5%) (State Forests data, 2020).
Studies report that forests have been affected by fires, insect outbreaks, strong winds, and tornados in the last 100 years (Karasiewicz, 1926; Koziński, 2007; Gietkowski, 2009; Słowiński et al., 2019). The Tuchola Pinewoods showed markedly low resistance to insect outbreaks. The most severe outbreak in this forest and Poland, in general, was that of Panolis flammea (pine beauty) in 1922–1924 (Mokrzecki, 1928; Broda, 2000, 2010). Due to the outbreak, most of the Notecka Forest and the Tuchola Pinewoods were cut down (Koehler, 1974; Ankudo-Jankowska, 2003; Broda, 2003). During 1978–1985, weather anomalies with cold winters and changes in water conditions triggered the outbreak of Lymantria monacha (black arches), which was the largest recorded in the history of the Polish State Forests (since its founding in 1924) (Sliwa, 1989; Jablonski, 2015). This outbreak affected the northern and western parts of Poland, including the Tuchola Pinewoods.
On 14 July 2012, the Tuchola Pinewoods have been hit by one of the most destructive tornadoes in Poland, which had an intensity of F3 (Taszarek et al., 2016). The tornado caused one fatality and 10 injuries, and within minutes, around 550 ha of the Tuchola Pinewoods in the Trzebciny District and 105 buildings were damaged. The track of the tornado was 20-km long and 800-m wide at maximum. On 11/12 August 2017, a hurricane hit the Tuchola Pinewoods. It destroyed forests up to an area of ca. 80,000 ha (9.8 mln m3) in 60 forest districts in northwestern Poland (Trębski, 2019).
In Poland, about 350 tornadoes have occurred between 2000 and 2019 (European Severe Weather Database, 2020). Moreover, 37 deadly tornadoes were known from earlier periods, for example, in Turzyn (1829), Tuchola (1871), Rowiska (1926), and Rawa Mazowiecka (1958) (Taszarek, 2016; Taszarek and Gromadzki, 2017). Each year, an average of 8–14 tornadoes hits Poland. The country is also affected by tornadoes of very high intensity (F4 on the Fujita scale) once every one or two decades (Taszarek, 2016).
Martwe peatland, located within the area deforested by the July 2012 tornado, offers the rare opportunity to study the imprint of a tornado in the paleoecological (pollen) record. We moreover use this archive to study the representation of other forest changes during the past 100 years, including further catastrophic events such as large-scale disasters and clear-cutting. To this end we attempt quantitative interpretation of the pollen record using the REVEALS model and compare the results with archival data from the area 4 km radius around the lake.
Here, we used a Sphagnum peatland as a natural archive that is often used to reconstruct long-term environmental and climate changes of the past based on paleoecological research (Tobolski, 2000; Charman, 2007; Słowiński et al., 2014). Paleoecological archives, such as peat, provide information about the past landscape changes, regional and local vegetation, climate, fires, and human history (Booth et al., 2004; Mitchell et al., 2007; Lamentowicz et al., 2015; Payne et al., 2015). In particular, pollen analysis serves as a proxy to reconstruct local- and regional-scale vegetation (van Geel, 1978; Słowiński et al., 2015; Kołaczek et al., 2018) and infer the history of forest management (Słowiński et al., 2019; Lamentowicz et al., 2020; Schafstall et al., 2020). Pollen percentage values do not perfectly represent past vegetation composition because pollen production and dispersal differ among plant taxa so strong pollen producers are over-represented while weak pollen producers are under-represented. We applied the REVEALS model (Sugita, 2007) to reduce this bias in the pollen data.
Furthermore, peatlands have been used extensively as archives of the past direct and indirect disturbances such as deforestation, clear-cutting, drainage, land use, pollution, fragmentation, fire, floods, or storms (Ireland and Booth, 2012; Ireland et al., 2012; Marcisz et al., 2019; Swindles et al., 2019; Łuców et al., 2020). However, they have never been used so far to explore past tornado events. Therefore, the aim of this study, is to present the first tornado-related paleoecological record from a monoculture in Poland on the background of previous forest management (clear-cutting, cutting after insect outbreaks), through vegetation reconstruction of a 100-year-old peat core, based on pollen analysis and the REVEALS model (Sugita, 2007) as well as on remote sensing data. We used the pollen analysis for the better understanding disturbances recorded in pine dominated forests in periods that devoid of historical data.
Materials and Methods
Study Site
The study site is the Martwe peatland in NW Poland (a partly floating mat on the Martwe lake) and its neighboring area within a radius of 4 km (Figure 1B). The peatland, which is located in the middle of a Pine monoculture forest, surrounds a small dystrophic lake. This lake is overgrown by Sphagnum floating mat (Martwe peatland) (Figure 1C), from which the peat core was obtained. The forest in this area has existed continuously for centuries, however, since after the first partition of Poland (1772 CE), a large-scale transformation of forests to pine monocultures by Prussian forestry was recorded. It was exactly connected with the introduction of the decree “On the management of the Tuchola Forest” issued by King Frederick of Prussia in 1782 (Jaszczak, 2008; Jażdżewski, 2008). As a result, eight administrative areas were created in the forested area of Tuchola Forest, whose first purpose was to make a detailed map of the forest area in order to estimate the potential for timber harvesting. The study site is located in Trzebciny Forest District in the southern part of the Tuchola Pinewoods (53°37′07.0″N, 18°12′09.0″E, 109.4 m a.s.l.; Figure 1). It is situated in the outwash plain of the Wda River, which developed during the Pomeranian phase of the Vistulian glaciation (Błaszkiewicz et al., 2015). The Martwe lake was formed by the melting of a buried ice block (Kordowski et al., 2010; Słowiński, 2010; Słowiński et al., 2015). The entire peatland as well as the lake (3.56 ha) is protected at the national level as Nature Reserve. It is assumed that acidification of the lake and the development of floating mat have been triggered by the cultivation of Pinus sylvestris monoculture about 200 years ago (Gietkowski, 2009; Kordowski et al., 2010). The maximum depth of the lake in the central part is about 3 m, while the peat layer has a thickness of 20–100 cm (Kordowski et al., 2010). The climate data from the meteorological station in Chojnice (about 45 km from the study site) obtained for the years 1951–2017 reveal that the warmest month is July with a temperature of 17.1°C and the coldest is January with –2.5°C (Institute of Meteorology and Water Management - National Research Institute, 2019). The average annual temperature of the study site is 7.3°C, and the average annual precipitation ranges between 550 and 600 mm.
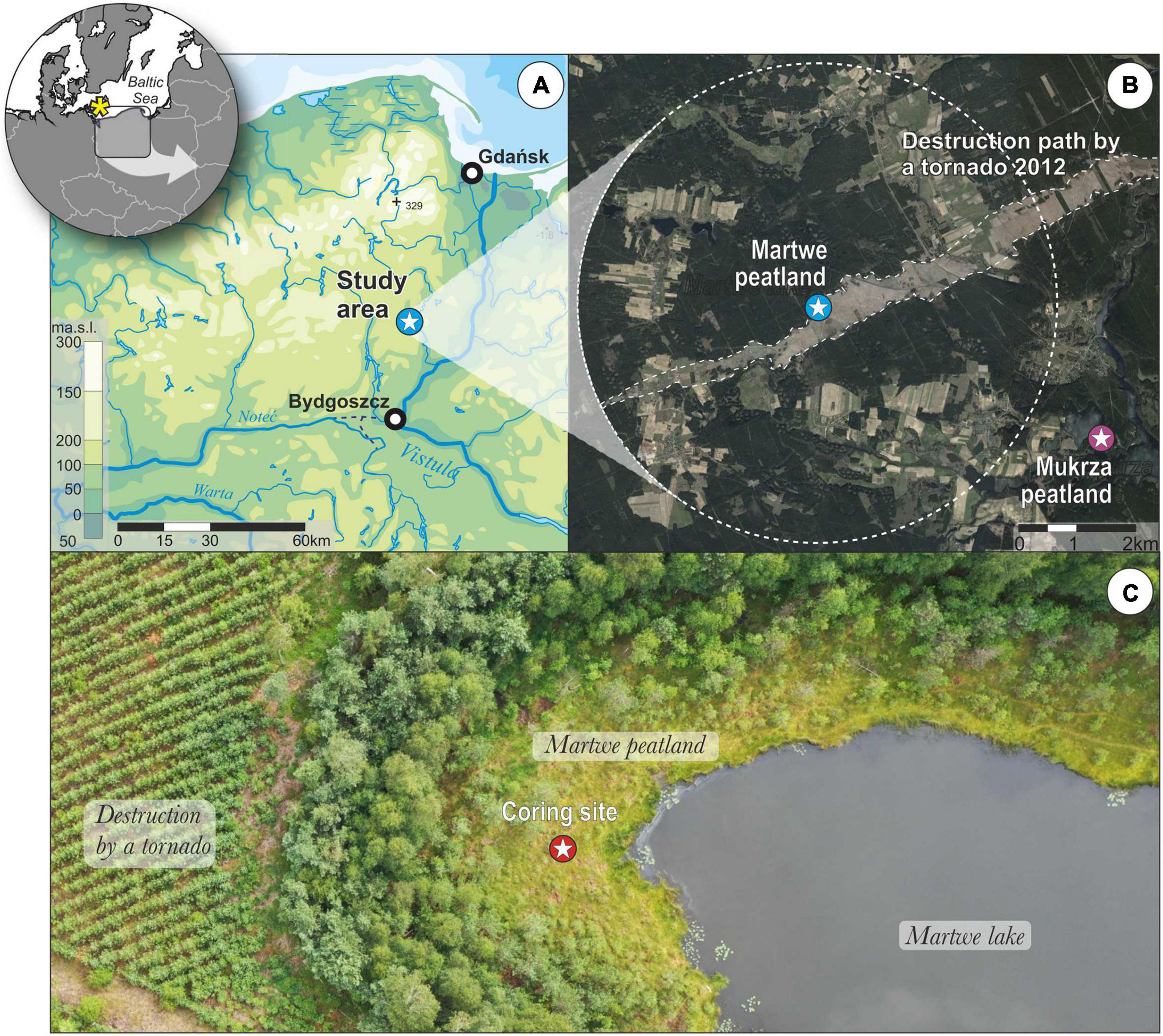
Figure 1. Setting of the study site. (A) Location on the Europe and Poland maps. (B) Location of the tornado path from 2012 in the southern part of the Tuchola Pinewoods. The Martwe peatland is in the center of map. Its neighboring area within the 4-km radius is marked in a white circle. The Martwe peatland is indicated by blue color, and Mukrza peatland (the nearest well-dated paleoecological site) by magenta color. (C) Coring site from the Martwe peatland (Sphagnum floating mat on the Martwe lake) and drone picture from July 2020.
Current Forest Composition and Potential Vegetation
In the study area, the forests are currently dominated by Pinus sylvestris forests (Figures 2A,C). The map of potential natural vegetation suggests a greater variety of trees species (Figure 2B; Matuszkiewicz et al., 1995), with mixed oak-pine forests being dominant (Querco-Pinetum), accompanied by oak-lime-hornbeam forests (Tilio-Carpinetum, poor) and swampy ash-alder wood forests (Frazino-Alnetum [Circaeo-Alnetum]). Leucobryo-Pinetum, Peucedano-Pinetum, and Carici elongate-Alnetum associations occupy a small area of the forests.
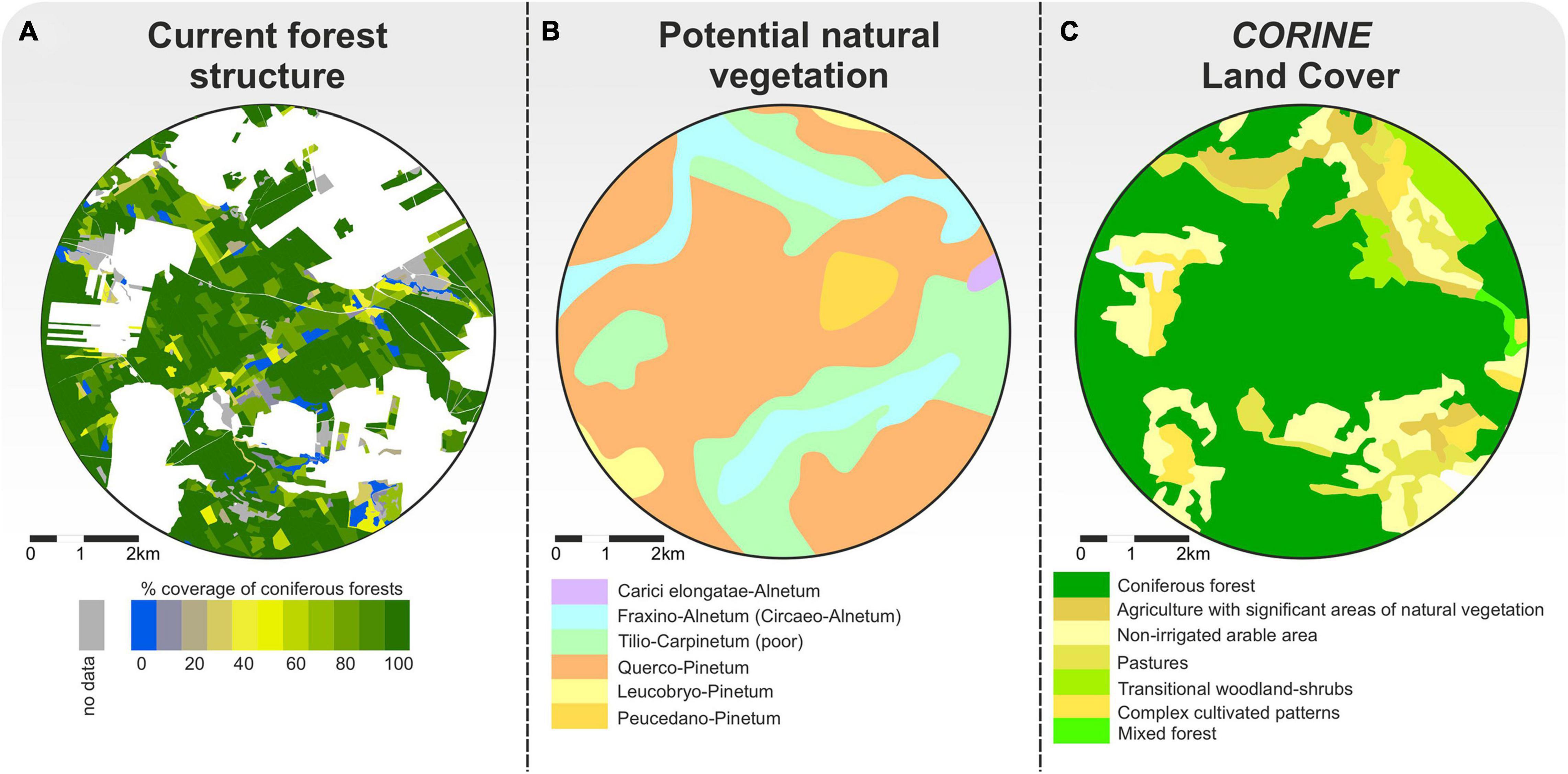
Figure 2. Maps of the area within the 4-km radius from the Martwe peatland (Sphagnum floating mat on the Martwe lake). (A) Current forest structure. The map was developed for the year 2020. (B) Differentiation of potential natural vegetation (Matuszkiewicz et al., 1995). (C) CORINE Land Cover. The map was developed for the year 2018. The Martwe peatland is located in the center of each map.
Peat Record
Four peat monoliths were extracted from the Martwe peatland in spring 2016 using a Wardenaar sampler (chamber dimension: 10 cm × 10 cm × 100 cm) (Wardenaar, 1987). The most representative peat core was selected for this study. For reconstruction covering the last 100 years, we analyzed a 47-cm-long part of one peat monolith (MAR1, 67 cm) from the southern part of the Martwe peatland, which was affected by a tornado from 2012. Pollen analysis was performed to reconstruct changes in the composition of forests over the last 100 years. Pollen samples (1 cm3) were collected continuously at 0.5-cm intervals (from 4 to 11 cm) and 1-cm intervals (1–3 and 12–47 cm), and prepared using standard laboratory procedures for palynological analysis (Berglund and Ralska-Jasiewiczowa, 1986). Pollen grains were identified using atlases (Moore et al., 1991; Beug, 2004) under a microscope at 400× and 1,000× magnifications. The figure of the simplified percentage pollen diagram was prepared using the Tilia program (version 2.0.41) (Grimm, 1992; Supplementary Figure 1). The pollen records from the Martwe peatland was compared with pollen record from Mukrza peatland, which is ca. 5 km southeast of the Martwe peatland. Original data come from the doctoral dissertation of Milena Obremska (Obremska, 2006; Lamentowicz and Obremska, 2010).
To extract past vegetation cover from pollen data we applied REVEALS using the REVEALSinR function from the discover R package (Theuerkauf et al., 2016). REVEALS is a correction factor approach, i.e., bias in pollen data is removed by dividing pollen counts by two corrections factors, i.e., pollen productivity estimates (PPEs) to account for differential pollen production and the dispersal-deposition factor K which accounts for differential pollen dispersals. We selected the Lagrangian-stochastic model to calculated K factors. As yet no PPEs are available from Poland, we used a preliminary PPE data set, which is based on the application of the ROPES model on a number of lake pollen records from northern Poland and Germany (Supplementary Table 1; Theuerkauf and Couwenberg, 2018). ROPES is a quantitative method for translating single pollen records into past vegetation composition without PPEs as an input parameter. The method requires pollen counts and pollen accumulation rate data. Besides reconstructed vegetation composition, ROPES also estimates PPEs. Stratigraphic diagrams were prepared using C2 (Juggins, 2003).
Chronology
The six samples of Sphagnum stems were dated using 14C AMS method in Poznań Radiocarbon Laboratory (laboratory code: Poz; Table 1). The Bayesian age-depth model based on these dates was constructed to determine the absolute chronology the age-depth model was constructed using OxCal 4.3 software Bronk Ramsey, 1995, 2006), by applying the P_Sequence function, assuming k0 = 0.9, log10(k/k0) = 1, and interpolation = 0.5 cm (Bronk Ramsey, 2008; Ramsey and Lee, 2013). IntCal20 (Reimer et al., 2020) and BombNH1 (Hua et al., 2013) 14C atmospheric curves were used as the calibration sets. For better readability, μ (mean) values are used to reflect the modeled age derived from the age-depth model.
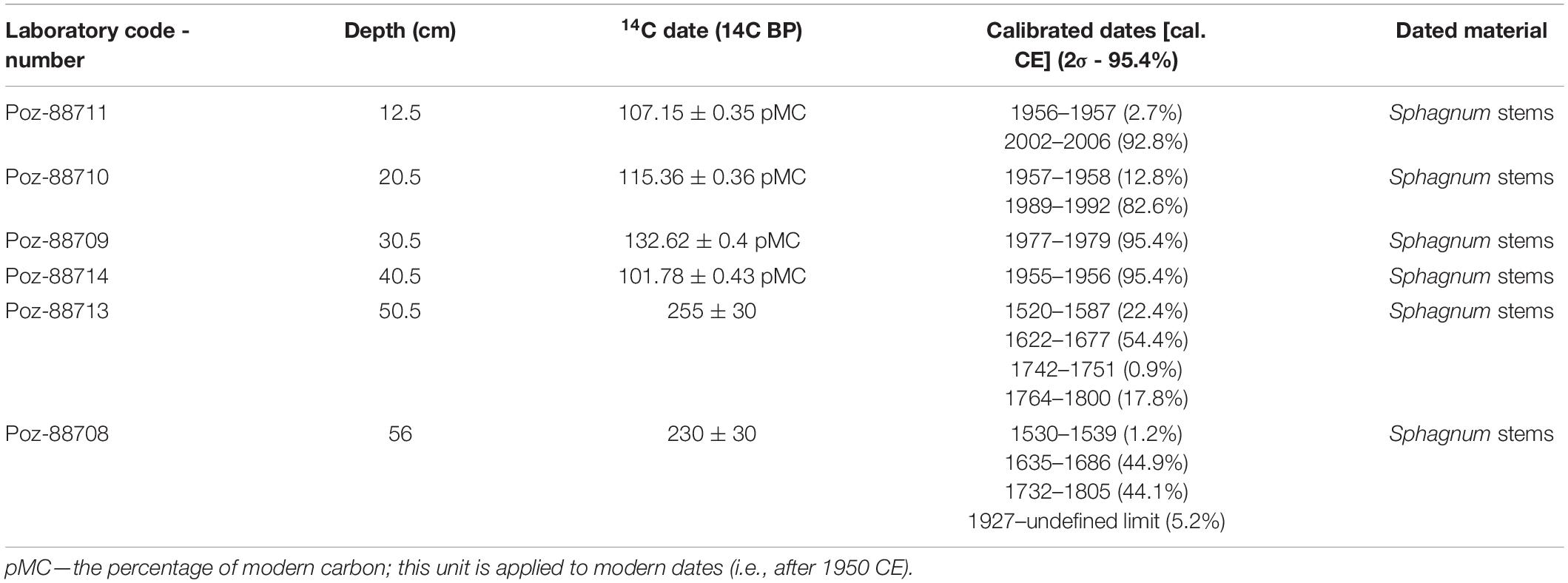
Table 1. 14C dating results from the Martwe peatland with the calibration and description of the dated plant macrofossils.
The topmost peat profile (53 cm) was dated using the 210Pb method. A samples for the analysis were processed at the Institute of Nuclear Physics, Polish Academy of Sciences, in Kraków. The activity of 210Pb was determined as the activity of its daughter radionuclide 210Po (half-life 138 days), which is in radioactive equilibrium with 210Pb. A total of 52 peat samples, weighing 0.35–0.63 g, were spiked with 208Po as a yield tracer and digested using a concentrated mixture of HNO3, HCl, and H2O2. Then, the solution was treated with 0.5 M HCl. Finally, a thin alpha-spectrometric Po source was prepared by spontaneous electrodeposition onto a silver plate after reduction of Fe3+ with ascorbic acid (Flynn, 1968; Fernández et al., 2012; Lee et al., 2014). The 210Po activities were measured using Alpha Duo spectrometer with Ortec detectors.
Excess 210Pb (unsupported) was calculated as the difference between the total activity concentration and supported activity concentration of 210Pb. The supported activity was calculated from the mean 210Pb activity concentration for the bottom layers (56 ± 5 Bq/kg). The age–depth relationships in a part of the peat core were estimated using two alternative dating models: Constant Rate of Supply (CRS) and Constant Flux Constant Sedimentation (CF/CS) (Sanchez-Cabeza and Ruiz-Fernández, 2012). The total unsupported inventory of 210Pb was calculated to be 2,800 ± 350 Bq/m2. The value was then corrected based on the extrapolation of the exponential equation to eliminate a systematic deviation of CRS dates toward erroneously old ages—the so-called “old-date error” (Binford, 1990; Tylmann et al., 2016).
Remote Sensing Methods
The land-use changes in the study area (neighboring area within a radius of 4 km) were reconstructed using archival material and the following maps: (1) German topographic maps—Messtischblatt (Meßtischblätter) in the scale of 1:25,000 [sheet: Lonsk (2375) from 1932 CE]; (2) Topographic Map of the Military Geographical Institute—Tactical Map of Poland in the scale of 1:100,000 (sheet P34 S26 Tuchola, released in 1933 CE, based on a photo from 1928 CE); (3) postwar topographic maps in the scale of 1:25,000 published in the 1980s CE; (4) overview map of the stands of the Osie Forest District, Szarłata area, in the scale of 1:20,000 (state on 1975); and (5) potential natural vegetation map of Poland in the scale of 1:300,000 (sheet A2) (Matuszkiewicz et al., 1995). All historic maps used for the analysis were calibrated and geo-referenced. Their spatial accuracy was ca. 30 m or better. The current state of the forest was determined using aerial orthophotomaps. Information layers with age, type, and tree species were obtained from the Forest Data Bank of the Polish State Forests (pl. Bank Danych o Lasach – Lasy Państwowe), which is part of the Numerical Forest Map. The Forest Data Bank provides detailed information about the modern forest. Data about open land were obtained from CORINE Land Cover, topographic maps in the scale of 1:10,000, and aerial photographs. Private forests were not included in the study. At present, their share in the total forest area is insignificant but it was considerable in the past in Tuchola Pinewoods (Pączewski, 1924). All maps were prepared using ArcGIS software.
Results and Interpretation
Chronology
The age-depth model revealed that the model agreement (Amodel) was 59%, which is almost equal to the recommended minimum (60%) (Bronk Ramsey, 2008). As most of the dates showed an individual agreement of >30% and all dates represented the period after the year 1945, we decided to accept the model (Figure 3 and Table 1). The fragment of the profile that was studied (0–47 cm) spanned a period of ca. 1892–2016. For the period after 1945, the maximum error of modeled age reached 4.3 years, whereas for the period between ca. 1891 and 1945 the age uncertainty ranged between 10.6 and 30 years.
The results showed that 210Pb and 14C chronologies were inconsistent for the lower part of the profile after applying the two models (CF/CS and CRS) and correcting for the lower part of the 210Pb date profile (Supplementary Files). For the section between 35.5 and 25.5 cm, the chronologies did not overlap even when maximum uncertainties were taken into account and both models differed from each other by ca. 15–20 years. Finally, we chose the absolute chronology based on 14C dates as it spans a longer time interval. However, it must be mentioned that the chronology for the period before the year 1945 (below 41 cm) should be treated with caution as it is encumbered by relatively high uncertainty.
Forest Composition Over the Last Century
The results of the pollen analysis of the 47-cm peat core and descriptions of the maps of plantings in 1900–2016 CE within the 4-km radius of the Martwe peatland are presented together for common zones (A–D) (Figures 4, 5). Zonation was based on changes in regional vegetation between ca. 1900 CE and 2016, data on planting areas (km2) within the 4-km radius of the peatland, and historical events such as a tornado that occurred on 14 July 2012 in Tuchola Pinewoods and local outbreaks of Panolis flammea during 1922–1924 CE and Lymantria monacha during 1979–1982 (Mokrzecki, 1928; Sliwa, 1989; Broda, 2000).
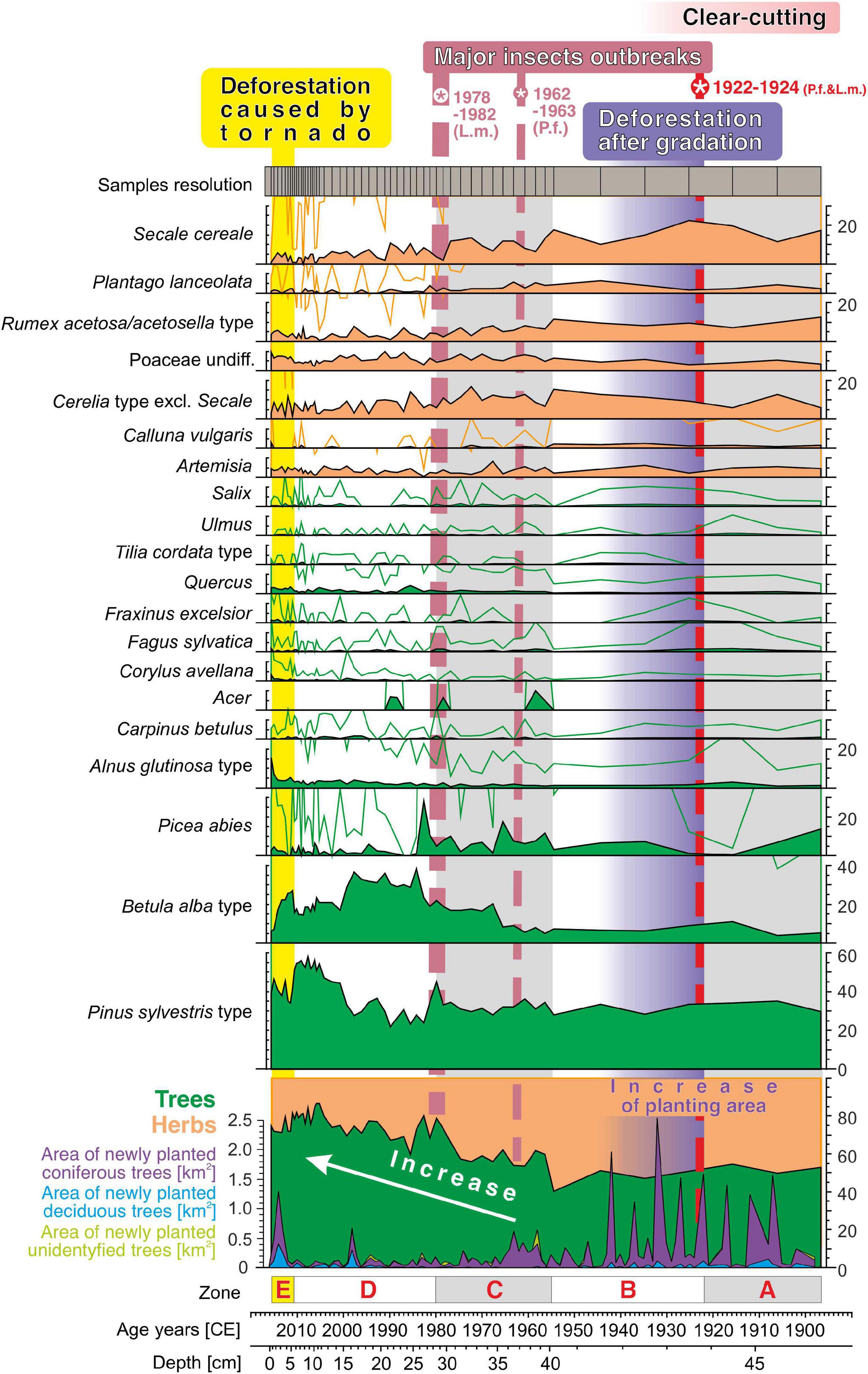
Figure 4. REVEALS-reconstructed abundance of major plant taxa in the surroundings of the Martwe peatland (Sphagnum floating mat on the Martwe lake) (×10 magnification). The figure also includes the area of newly planted (km2) coniferous, deciduous, and unidentified trees within the 4-km radius from the peatland, major local outbreak events (Lymantria monacha—L.m.; Panolis flammea—P.f.), and a description of the main stages.
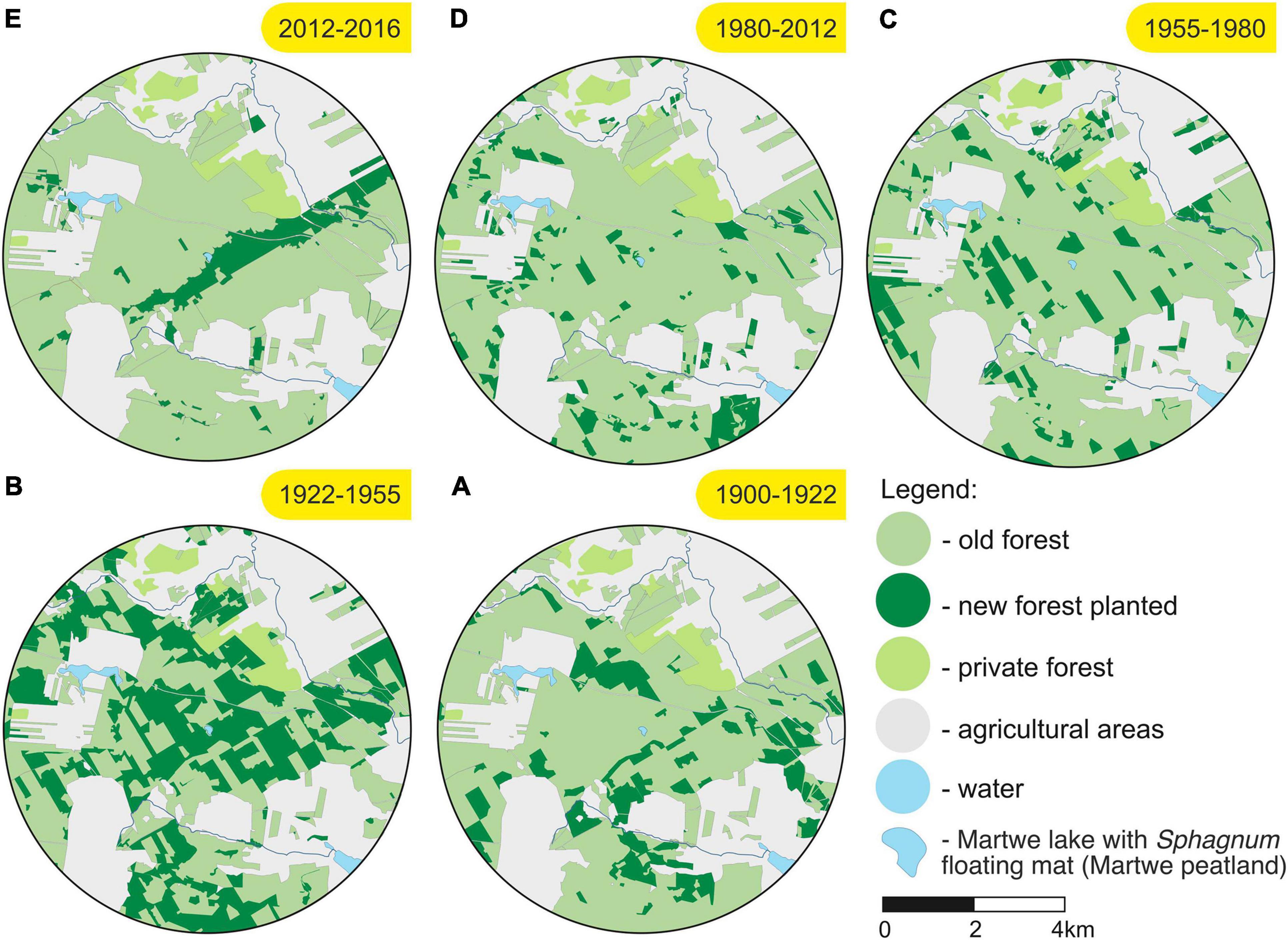
Figure 5. Maps of the subsequent plantings in 1900–2018 CE within the 4-km radius from the Martwe peatland (Sphagnum floating mat on the Martwe lake). The peatland is located in the center of each map. The letters (A–E) correspond to the palynological zones. The term “old forest” in the map refers to an already wooded area.
For the interval between ca. 1900 and 1922 CE (zone A, 47–44 cm), the pollen-based reconstruction suggested that the forest covered on average ca. 52% of the surroundings of the Martwe peatland (Figure 4). Pinus sylvestris was found to be the most abundant taxon (ca. 29.7–34.9%), while Betula spec. was rarer (ca. 3.8–10.8%). Picea abies had covered more than 10% at the beginning of zone A but then its abundance declined to almost zero. Secale cereale (11.4–19.7%) and other cereals (5.8–12.8%) were the most abundant open land taxa, followed by Rumex acetosa/acetosella (ca. 7.1–12.6%), Poaceae (grasses) (ca. 3.5–5.6%), Artemisia (ca. 4.6–5.5%), and Plantago lanceolata (ca. 2.2–4.2%). The lower forest cover (pollen data) in the peat core accompanied with an increase in the planting area (Figure 5A). The pollen data reflected high openness with simultaneous afforestation, which might be interpreted by the lag between tree planting and the onset of pollen production. Historical map data showed that ca. 5.6 km2 of the surface area within the 4-km radius of the peatland (i.e., ca. 10% of the area) was afforested between 1900 and 1922 CE (Figure 5A). Most plantings were made beyond 1 km from the peatland, and the plantations included ca. 90.2% coniferous, ca. 9.1% deciduous, and ca. 0.7% unidentified trees. The plantings probably suggests previous clear cutting within these areas (assuming the forest has been continuously maintained over the last 100 years in the area) and/or afforestation of new areas (for example, agricultural fields) (Koziński, 2007). Tuchola Forest became the largest center of wood production at the end of the 19th century. Therefore, in the planting area, there may have been felling of trees for industrial purposes in the earlier period and/or afforestation after clear-cutting for war (Broda, 2010).
The reconstructed plant abundances are roughly similar in zone B (1922–1955 CE, 44–40 cm). The forest cover remained at an average of ca. 47% (Figure 4), with Pinus sylvestris covering ca. 31% and Betula spec. covering ca. 6–9% on average. Picea abies clearly recovered from the decline in zone A, covering ca. 5% in zone B. The reconstructed cover of Secale cereale is ca. 15–22%, that of other cereals ca. 9–16%, that of Poaceae ca. 5%. Between 1922 and 1955 CE, trees were newly planted on ca. 13.3 km2 (ca. 26.5% of the study area), mainly as large plantations and also in the vicinity of the Martwe lake (Figure 4B). Again, more than 93.1% of the trees planted were coniferous, mainly Pinus sylvestris, maintaining pine monocultures (ca. 6.1% deciduous and ca. 0.8% unidentified trees). Much of the plantations likely compensated for clear-cutting following World War II, and especially disturbances caused by insect outbreaks from the beginning of the 20th century (1922–1924 CE) (Stieber and Bartz, 1923; Bartz and Ziółkowski, 1924; Mokrzecki, 1928; Ziółkowski and Bartz, 1928; Andrzejewski and Bartz, 1929; Broda, 2010; Słowiński et al., 2019). Widespread large-scale clear-cutting in the Tuchola Pinewoods was interspersed with insect outbreaks in these times (Fudała, 1985; Sukovata and Kolk, 2000), resulting in high economic losses. Between 1922 and 1925 CE, the influence of the insect outbreaks was the strongest, and the outbreaks mainly included that of Panolis flammea (moth and its caterpillars feed on Pinus needles), and to a lesser extent Lymantria monacha, which affected the Trzebciny Forest District (forest district in which the lake is located) (Mokrzecki, 1928; Fudała, 1985; Broda, 2000; Załoga, 2014). In this case, an increase in plantings was noted (Figure 5B). The clear-cutting caused by insect outbreaks led to the removal of trees and reforestation, mainly of Pinus sylvestris, in very large areas of Trzebciny Forest District (Figure 5B).
In the period 1955 to 1980 CE (zone C, 40–28.5 cm), the reconstructed forest cover increases from ca. 50% to ca. 80%, mainly because of an increase in the cover of Betula spec. from ca. 5% to ca. 22%, particularly after 1965 CE (Figure 4). The cover of Pinus sylvestris remains at about ca. 33%. The reconstructed cover of Picea abies shows large variations from ca. 1.4% to ca. 17.4%, and at least periodic higher values than before. For most herbal taxa, including Secale cereale and other cereals, the reconstructed cover in zone C is somewhat lower that before. Only the cover of Poaceae is somewhat higher than before, arriving at ca. 4.7% to ca. 8.6%. Between 1955 and 1980 CE, ca. 5 km2 of the area was afforested, i.e., significantly less than during previous periods (Figure 5C). As before, most planted trees have been coniferous (ca. 90%), mainly pine (Figure 5A). Although archival data do not indicate prominent changes in forest cover, our pollen-based reconstruction still suggests an increase in forest cover, i.e., particularly a higher cover of Betula spec. and Picea abies. We consider two likely explanations for this mismatch. First, Betula spec. may have truly expanded within existing, pine dominated forests, e.g., due to changes in forest management or nutrient availability. Secondly, Betula spec. may have expanded in the vicinity of the sample site. In this case, higher pollen deposition of Betula spec. a is result of high (extra) local pollen deposition.
Zone D (28.5–5.5 cm, 1980 and 2012 CE ± 2 years) is characterized by the highest reconstructed forest cover (ca. 67.2–86.7%, Figure 4). Within the zone, the cover of Betula spec. is highest between 1980 and 2000 CE (ca. 30%) while the cover of Pinus sylvestris is highest between 2000 and 2012 CE (ca. 50%). Moreover, at the beginning of zone D, a peak in the cover of Picea abies (ca. 28%) is observed. After ca. 1980, the reconstructed cover remains much lower. This decline likely represents the outbreak of Lymantria monacha between 1979 and 1982 in the Trzebciny District (Sliwa, 1989; Załoga, 2014; Jablonski, 2015). Our reconstruction also suggested some expansion in Alnus glutinosa to ca. 2.6% on average. Other deciduous taxa showed only slight changes. The cover of open taxa and, including Cerealia type excl. Secale, Secale cereale, Plantago lanceolota, and Rumex acetosa/acetosella, is lower than before. Between 1980 and 2012 CE, an area of ca. 3.6 km2 has been afforested within 4 km distance from lake Martwe, i.e., a smaller area than before (Figure 5C). Among the newly planted trees, the proportion of deciduous trees is higher than before (>30%) whereas the proportion of coniferous trees is lower (>60%) (Figure 5A).
Our reconstruction suggests a prominent role of Betula spec. in the forests of the study area at least between 1980 and 2000 CE. Currently the forests in the Trzebciny District are clearly dominated by Pinus sylvestris (ca. 91%) whereas Betula spec. plays only a minor role (ca. 3%) (Kasprzyk, 2021). The literature/maps indicate that the cover of Betula spec. was not substantially higher between 1980 and 2000. The higher reconstructed cover may be an artifact of high (extra)local pollen deposition, i.e., pollen deposition from Betula spec. trees growing at or close to the core location. We cannot evaluate whether the increase in the reconstructed cover of Betula spec. since 1965 is fully attributable to such effect, or whether it represents some true regional expansion of Betula spec. Such expansion may have been triggered by changes in forest management and grazing intensity. Betula could be actively removed as a “forest weed” until the mid-20th century (our results) Grus, 1891, 1897; Dec, 1945). Also, in the past Betula was limited by intense grazing (Supplementary Figures 2, 3; Mokrzecki, 1928; Broda, 2000) and insect outbreaks. In previous centuries, the forest was used more by humans (Supplementary Figures 2, 3).
Finally, our reconstruction indicates very recent changes in forest composition (zone E, 2012 ±1 year to 2016 CE, 5.5–1 cm, Figure 4). The cover of Pinus sylvestris is somewhat lower (40%), which can be mainly related to forest tornado damage in 2012 CE (Figure 1; Taszarek et al., 2016; Kaleta, 2017). For Betula spec., a short increase to ca. 26% was observed, followed by a steady decline to ca. 6%. For Alnus glutinosa, a distinct increase to ca. 16% is indicated after 2014 CE. Other tree taxa showed no prominent changes. Both the high cover of Betula spec. around 2012 CE and the high cover of Alnus glutinosa in recent years are unrealistically high, forest inventories show much lower cover. Hence, the high values likely are an artifact of (extra) local pollen deposition at the coring site. Among the herbs, some increase was noted particularly for the grasses, compared to the end of zone D. Between 2012 and 2016 CE, ca. 2.6% of the area within 4 km from the study site was afforested (Figure 5E), with ca. 67.3% coniferous and ca. 32.7% deciduous trees (Figure 2A). The afforested areas included those bordering the peatland to the south and east. Most trees were newly planted following the tornado in 2012 in the devastated areas (Figures 1, 4D; Stopiński, 2012; Taszarek et al., 2016). The tornado had mostly destroyed ca. 80-year-old Pinus sylvestris trees planted after an outbreak of Panolis flammea from 1922 and 1924 (Figure 4; Mokrzecki, 1928; Broda, 2003).
Discussion
Legacy of Forest Management
The forests of the study area (Figures 4, 5) are mainly pine monocultures introduced by humans for timber harvesting (Mokrzecki, 1928; Gietkowski, 2009). The current vegetation clearly differs from the potential natural vegetation, which is richer in deciduous tree taxa (Figure 5). Human impact on the vegetation in the Tuchola region is well recognizable since the beginning of the Middle Ages (Milecka and Szeroczyńska, 2005; Noryśkiewicz, 2006), whereas the origin of the Pinus sylvestris monocultures dates back to the Prussian Partition (Gietkowski, 2009; Figure 6). Large-scale transformation of forests was started by Prussia soon after the first partition of Poland in 1772 CE (Gietkowski, 2009; Słowiński et al., 2019). Some effects of this transformation were clear-cutting and introduction of pine monocultures on poor soils developed on Wda and Brda sandy outwash plains (Dysarz, 1998; Gietkowski, 2009; Supplementary Figure 1). Paleoecological studies conducted on the lakes and peatlands in the Tuchola forest have confirmed strong human-induced changes in the composition of the forest over the last 300 years Lamentowicz et al., 2007, 2013; Dietze et al., 2019; Słowiński et al., 2019).
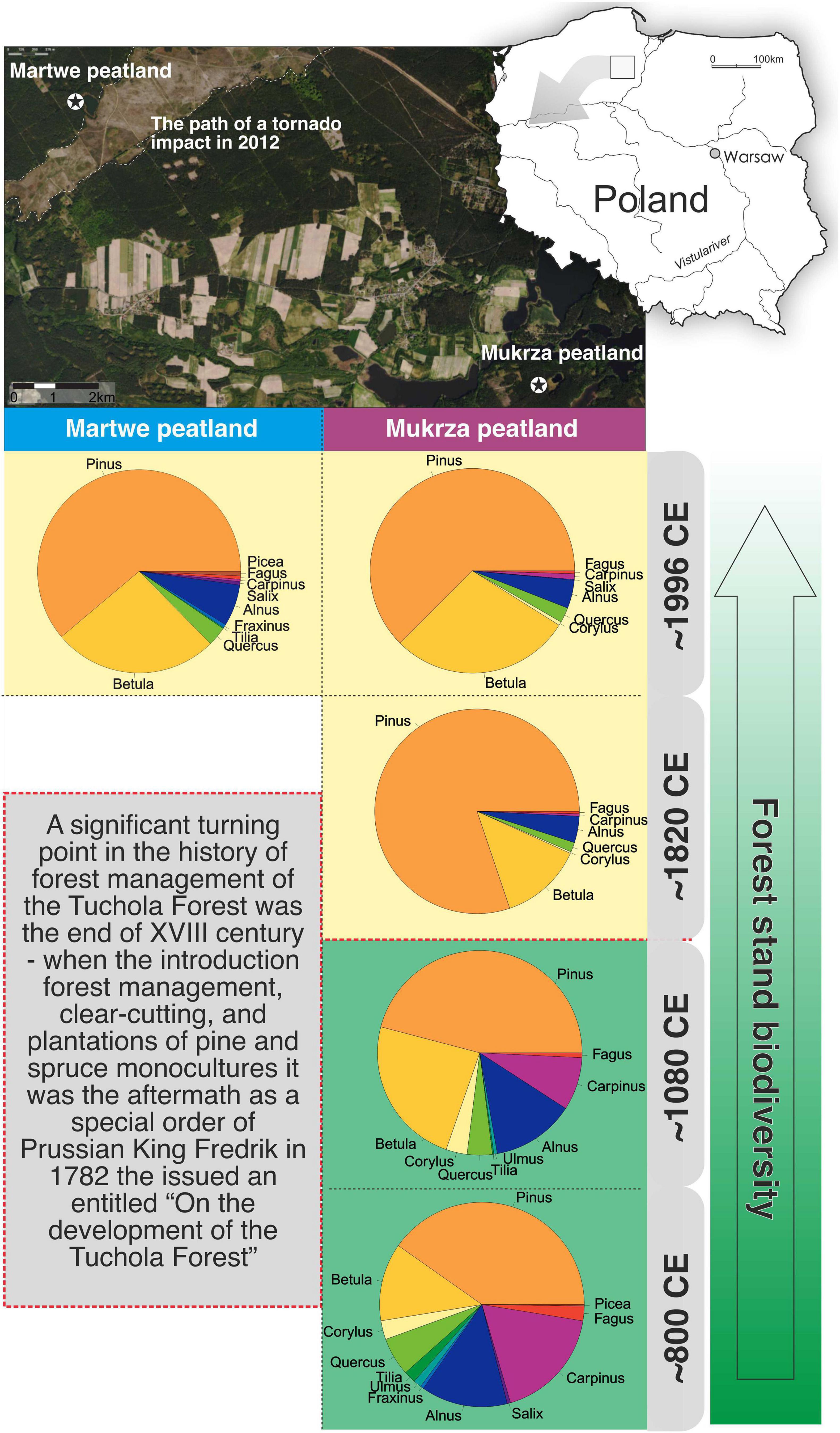
Figure 6. Relative abundance of arboreal pollen (AP, standard and untransformed pollen values) in the past based on a pollen record from the Mukrza peatland and comparison of records from the Martwe peatland. The figure present four phases of forest transformation by human activities in the Tuchola Pinewoods: (a) around 800 CE, before the end of the Migration Period, seminatural vegetation forest composition; (b) early Medieval Period, beginning of the 12th century, low human activity; (c) beginning of the 19th century, the period after the introduction of pine monoculture; and (d) current forest vegetation composition, end of the 20th century. The zones are based on a study of the Mukrza peatland by Obremska (2006).
The forest management, as well as the perception of the forest by managers and local communities, has changed through time. This is most clearly visible in the species composition of the forests that were managed before the last centuries in the entire Tuchola Pinewoods (Broda, 2000). Due to changes in the management and transformation of forests into a pine monoculture, the perception of local people and foresters toward pine monoculture and particular tree species has also been affected Karasiewicz, 1922, 1926; Mokrzecki, 1928; Supplementary Figures 1, 2). The most prominent example is Betula spec. which has been considered a forest pest (Grus, 1897) and hence was simply removed from the undergrowth (Grus, 1897; Dec, 1945). Correspondingly, our reconstruction showed a low cover of Betula spec. until World War II. After the war, Betula spec. became more abundant because Betula strips were introduced as a so-called green belt surrounding pine monocultures to better control forest fires (Forest Fire Protection Manual). Moreover, in the past, local communities were allowed to use the forest for the grazing of sheep and cows and to collect needles and cones (Mokrzecki, 1928; Broda, 2000). These activities largely removed the forest undergrowth, including Betula, and prevented ground fires. However, these activities also removed nutrients, and as a result, caused degradation of forests and increased their susceptibility to insect attacks (Broda, 2000).
Methodological Implications – Archival Materials Meet Paleoecology
The present comparison of a pollen-based land-cover reconstruction with archival data shows similarities but also differences, which points at limitations of either approach. Most importantly, the land-cover reconstruction suggests an increase in forest cover since about 1950 CE, while archival data show a stable pattern of forest and open land. The primary cause for that mismatch may be the changing age structure of pine plantations. Our pollen-based reconstruction assumes that pollen productivity of all taxa is a constant. In reality, however, pollen productivity is variable and influenced, e.g., by the age of a forest stand. Before 1950 CE, the area of newly planted pine forests was high, following large scale harvesting and insect outbreaks. Hence, the area of young pine forests, which still produce low or little pollen was high. After 1950 CE, the situation was more stable and the proportion of older forests with high pollen production likely increased. Besides age, pollen production may also be influenced by nutrient availability. Until the mid 20th century, forest grazing and the removal of organic matter probably has reduced nutrient availability, which may have reduced tree growth and pollen productivity. Moreover, over the past decades, atmospheric fertilization has enabled accelerated tree growth (Pretzsch et al., 2014), and may have also affected pollen production.
Our reconstruction suggests widespread expansion of Betula spec. during the second half of the 20th century, likely because forest grazing ended and more nutrients were available. Betula spec. did not expand in previously open areas but obviously in the existing pine forests, as understory element and/or along forest margins. The forests would hence produce, besides Pinus sylvestris pollen, an increasing amount of Betula spec. pollen. In other words, the pollen deposition of tree taxa, mainly Pinus sylvestris and Betula spec., increases, although the forest cover is unchanged. Hence, a second cause for the mismatch between the pollen-based forest cover and archival pollen cover may be the expansion of Betula spec. in Pinus sylvestris forests.
Alternatively, the possibly too high reconstructed cover of Betula spec. particularly between 1980 and 2000, may be attributable to (extra) local pollen deposition. The REVEALS approach is suited for pollen records from large lakes, which well represents regional vegetation composition. Pollen deposition in small lakes and peatlands, like lake Martwe, may instead be much influenced by (extra) local pollen deposition from nearby vegetation and hence represent a mixture of regional and local scale vegetation composition. Application of more suited local scale methods, such as LOVE or Marco Polo, was impossible because of a missing regional reference site (Sugita, 2007; Mrotzek et al., 2017).
Overall, our results underline that interpretation of pollen records from the recent past is far from simple, mainly because the pollen production of trees and herbs is variable due to changes in land management, atmospheric fertilization and also the climate. Further comparisons of archival and pollen-based reconstructions would be helpful to better understand the effects. To this end, records of pollen accumulation rates, e.g., from varved lakes, would be particularly useful because they avoid the mutual interdependence of pollen percentage data.
Long-Term Environmental Consequences of the Forest Plantations – Archival Materials Meet Paleoecology
The pollen signal from the Martwe peat core (MAR1), along with the remote sensing data, clearly show the land-use changes that were caused by the clear-cutting of pine monocultures and insect outbreaks in the 20th century as well as a recent disaster—the tornado in 2012 (Figures 4, 5). Especially, a good correspondence was observed between the changes in Pinus sylvestris pollen signals with archival materials and the cartographic data of the tornado event. The last dramatic event (and other massive wind throws that occurred in the region) and historical events like insect outbreaks during the last 100 years in Tuchola Pinewoods confirm the high vulnerability of these types of ecosystems to various disturbances like winds, pathogens, and fires (Mokrzecki, 1928; Seidl et al., 2017). Similar changes were observed in the forest composition during the last 100 years in the Mukrza peatland (Figures 1, 6). The pollen records of both peatland are compared in Figure 6. It can be noticed that the relative abundance of arboreal pollen was similar—90.8% in Martwe and 87.5 in Mukrza peat—in ca. 1996 CE (standard and untransformed pollen values). The relative abundance of Pinus sylvestris was ca. 55.9% and ca. 54.7%, respectively (Figure 6). Studies of forest plantations in various regions of the world show that forest homogenization increases the vulnerability of the emerging novel ecosystem (Słowiński et al., 2019; Matusick et al., 2020). Monocultures of Pinus sylvestris cause acidification and depletion of soils, which not only results in reduced productivity (Bialy, 1999; Baltodano, 2000; Rutkowska, 2019; Steckel et al., 2020), but also has a negative impact on the biodiversity of forests (Gustafsson et al., 2012; Fedrowitz et al., 2014) and leads to acidification of wetlands (Lamentowicz and Mitchell, 2007). Furthermore, the long-term process of restoration influences soil biodiversity (Fedrowitz et al., 2014) and, as a consequence, the resistance of Scots pine in Tuchola Pinewoods. Due to the transformation of mixed-forest (mainly removal of hornbeam, oak, ash, and alder forests) into pure Pinus sylvestris monoculture (Figure 6; Miotk-Szpiganowicz, 1990; Boiński, 1993), the Tuchola Pinewoods are more vulnerable to disturbances such as insect outbreaks, fires, or winds (Słowiński et al., 2019).
Across Poland, pine forest monocultures have usually been established on sandy soils (Matuszkiewicz, 1999). The susceptibility of pine forest in our study area represents a climate-related hazard. Due to maintaining pine monocultures, the management of Tuchola Pinewoods is not focused on the natural regeneration of forests (Figure 6). The current challenge is to find optimal solutions for forest regeneration and adapt its species composition to changing habitat (Tomaś and Jagodziński, 2019). As a consequence of delay in the adaptation of tree composition to new conditions caused by climate changes due to the long-life cycle of trees (Jagodziński, 2020), Picea abies and Betula forests die out in many regions of Poland (Boczoń et al., 2018; Skrzecz and Perlińska, 2018). Although the European forest policy and management widely promote the shift from coniferous monocultures to mixed stands of coniferous and broadleaved species, these suggestions are either not introduced in most of the forested areas or not applied appropriately (Figure 7; Zerbe, 2002; Kint et al., 2006; Knoke et al., 2008; Zhang et al., 2012; Thurm et al., 2016; del Río et al., 2017). In the last decade, several studies confirmed that the replacement of monocultures by mixed forests increases their resistance to sudden climate shifts (Thurm et al., 2016; Leuschner and Ellenberg, 2017) and disturbances such as insect outbreaks, fires, and pathogen invasion. Nevertheless, it has been highlighted that natural recovery leads to higher biodiversity as well as a more resilient and productive ecosystem (Liang et al., 2016; Leuschner and Ellenberg, 2017). Based on the forecasts of threats to individual tree species in Europe, Dyderski et al. (2018) clearly emphasized that the so-called pioneering tree species like Betula pendula, Larix decidua, Picea abies, and Pinus sylvestris will be increasingly affected by climate change-related issues such as increases in disturbances and insect outbreaks (Seidl et al., 2014). The authors underline that this constitutes a serious threat to both forest management and nature conservation due to the fact that Pinus sylvestris occupies about 67% of the forest area of the country (Dyderski et al., 2018). The cultivation of Picea abies and Pinus sylvestris forest was adequate in the past two centuries; however, with progressive global warming, the measurable benefits might be difficult to achieve. Droughts cause permanent changes in forest composition and conditions (Jagodziński, 2020), and thus contribute to the weakening of trees in the forests. Our study documented an increased abundance of deciduous forests (Figure 4) in the last two decades. During that time, the planting of deciduous forest accounted for a high percentage, which was not observed earlier (Figure 4). However, there is still a large difference in relation to the species composition from the Medieval Period (Figure 6; Obremska and Lamentowicz, 2006).
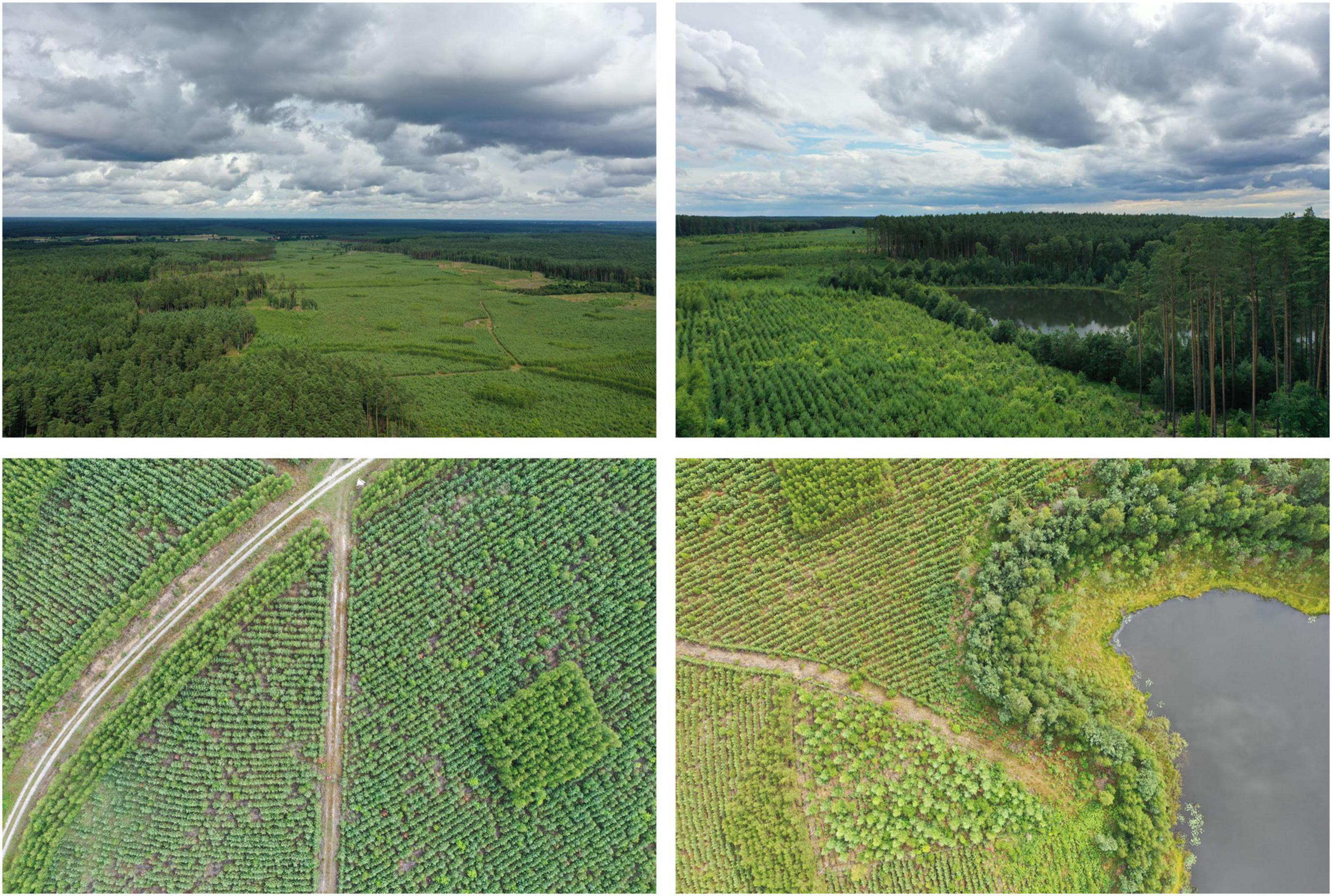
Figure 7. Drone pictures of the forest planted after forest tornado damage from 2012 with characteristic Betula pendula islands and Betula pendula trees along the roads. In the background – Martwe lake with Sphagnum floating matt (Martwe peatland).
The changes in the structure and composition of the forest during the last 100 years were compared using pollen records and archive maps. The results (Figures 4, 5) showed that the size and location of tree plantings in the last 100 years allowed the reconstruction of considerable land-use changes. We recorded a strong relationship between the pollen spectra of Pinus sylvestris and the planting process, and consequently, forest tornado damage by the tornado in 2012 (Figures 4, 5, 8). Data combination proves that paleoecology with archival materials and cartographic data complement each other well, especially in the case of heavily economically exploited monoculture leading to critical transitions. The pollen pattern of Pinus sylvestris, along with the archival materials and cartographic data between 1900 and 1943 CE, indicates clear cutting on a wide scale. Moreover, the location and size of plantings and later clear-cutting suggest dynamic changes in land use until 1965 CE, especially in the first half of the 20th century, while the period between 1965 and 2012 CE was characterized by a clear slowdown in changes. We have planned to use precise data about the location and size of plantings in further paleoecological research to study the impact of deforestation and clear-cutting on the functioning of wetland ecosystems (Słowiński et al., 2017; Łuców et al., 2018).
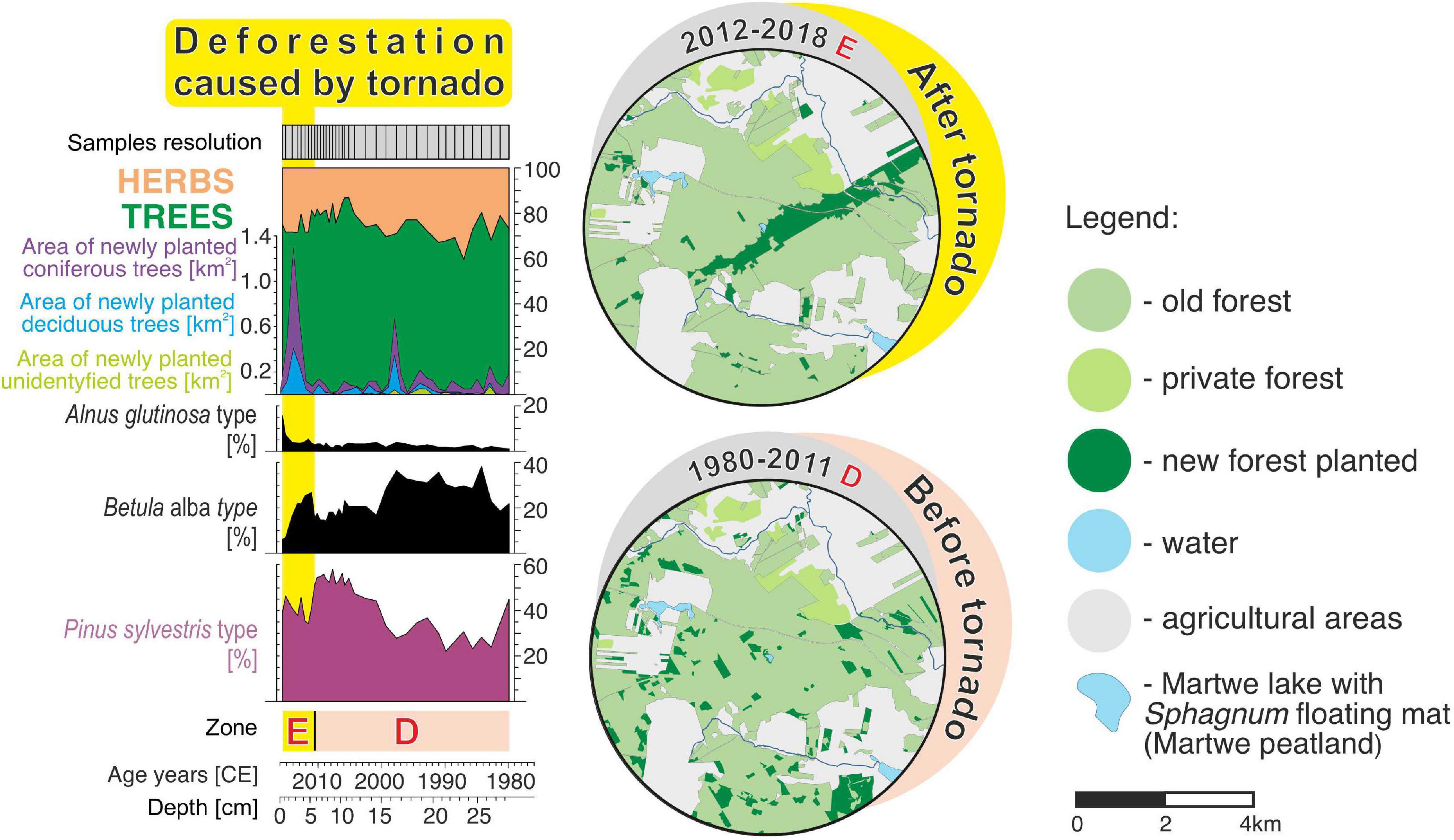
Figure 8. Summary diagram of REVEALS-reconstructed plant abundances and remote sensing data after and before the tornado event from 2012. Maps of the area within the 4-km radius from the Martwe peatland (Sphagnum floating mat on the Martwe lake).
Conclusion
We present the first tornado-related paleoecological record from a monoculture in Poland on the background of previous forest management (clear-cutting, cutting after insect outbreaks), through vegetation reconstruction of a 100-year-old peat core, based on pollen analysis and the REVEALS model as well as on remote sensing data. Results of this study confirm that an event such as a tornado (forest tornado damage) may be recorded in a peat core. The forest tornado damage from 2012 was characterized by a decrease in Pinus sylvestris cover as a result of the destruction of the forest by a tornado and the increase of Betula spec. cover. The combination of paleoecological and remote sensing data allowed us to present the forest management practices during the last 100 years. The past forestry practices (like clear-cutting and cutting after insect outbreaks, also to a lesser extent an active removal of Betula as a “forest weed”) are well identified in the proxy record. Our study shows that the observed monocultural stands lacked resistance to strong winds, but they were weakened most possibly also by droughts, and susceptible to insect outbreaks. Although the monocultures quickly replaced the disturbed stands, they did not result in any change in management, and as a result, only Pinus sylvestris was newly planted repeatedly. It is only in the last two decades that a change occurred in management and deciduous species such as oak and hornbeam were introduced into monocultures. This trend should be maintained to achieve the species composition and percentage share as that of 300 years ago to make the forest less sensitive to wind and better adapted to climate change.
Data Availability Statement
The original contributions presented in the study are included in the article/Supplementary Material, further inquiries can be directed to the corresponding author.
Author Contributions
MS contributed to the conception and design of the study. MS, ML, KM, and DŁ collected the peat core. DŁ wrote the first draft of the manuscript. PK performed the pollen analyses. ST performed the maps analysis. MT performed the REVEALS analysis. PK, ML, EŁ, and MS contributed to the chronology. DŁ, MS, and ST contributed to the figures and tables. All authors contributed to edit and revision of the manuscript and approved the submitted version.
Funding
The research was financed by the Polish National Science Centre (Grant No. 2015/17/B/ST10/03430). MT and KM activities were funded respectively by the ULAM NAWA scholarship PPN/ULM/2019/1/00350 and 2020/39/D/ST10/00641, respectively.
Conflict of Interest
The authors declare that the research was conducted in the absence of any commercial or financial relationships that could be construed as a potential conflict of interest.
Publisher’s Note
All claims expressed in this article are solely those of the authors and do not necessarily represent those of their affiliated organizations, or those of the publisher, the editors and the reviewers. Any product that may be evaluated in this article, or claim that may be made by its manufacturer, is not guaranteed or endorsed by the publisher.
Acknowledgments
We thank the reviewers (LF and MN), as well as the editor Gianluca Piovesan for their comments and suggestions that allowed us to improve the quality of the manuscript. This research is a part of the Ph.D. thesis of DL titled “Disturbances of Sphagnum peatlands in Central-Eastern Europe” at Adam Mickiewicz University, Poznań (Poland). We would like to thank the Polish State Forests for sharing the databases which are part of the Numerical Forest Map. We are grateful to Jan Matuszkiewicz for allowing us to use the map of the potential natural vegetation of Poland.
Supplementary Material
The Supplementary Material for this article can be found online at: https://www.frontiersin.org/articles/10.3389/fevo.2021.747976/full#supplementary-material
Supplementary Figure 1 | Simplified percentage pollen diagram from the Martwe peatland (×10 magnification).
Supplementary Figure 2 | A postcard from around 1900 CE showing the life of the inhabitants of Tuchola Pinewoods and their integration with the forest (from the collection of the Muzeum Pomorza, www.muzeumpomorza.pl) and wood run-off down the Wda River.
Supplementary Figure 3 | A postcard from around 1900 CE showing the life of the inhabitants of Tuchola Pinewoods and their integration with the forest (from the collection of the Muzeum Pomorza, www.muzeumpomorza.pl) as well as the life of the inhabitants of pine monocultures.
Supplementary Table 1 | Parameters (fall speed, PPE, and PPE error) of pollen used to prepare REVEALS reconstruction.
Supplementary Files | Absolute chronologies derived from 14C and 210Pb dates from the Martwe peat profile (MAR-1).
References
Andrzejewski, M., and Bartz, E. (1929). Rynek Drzewny i Budowlany: Organ dla Handlu i Przemysłu Drzewnego Oraz Budowlanego (Eng. The Timber and Construction Market: An Authority for the Wood and Construction Trade and Industry): Oficjalny Organ Związku Przemysłowców Drzewnych w Poznaniu: Oficjalny Organ Giełdy Drzewnej w Bydgoszczy. Poznań: Poznań, 14.
Ankudo-Jankowska, A. (2003). Gospodarka lasów państwowych na terenie Województwa Poznańskiego i Pomorskiego w okresie międzywojennym (eng. Economy of the state forests in the Poznań and Pomeranian Voivodeships in the interwar period). Stud. Mater. Ośrodka Kult. Leśnej 5, 53–75.
Baltodano, J. (2000). “Monoculture forestry: a critique from an ecological perspective,” in Proceedings of the Tree trouble: A Compilation of Testimonies on the Negative Impact of Large-Scale Monoculture Tree Plantations Prepared for the 6th COP of the FCCC. Hague: Friends of the Earth International.
Bartz, E., and Ziółkowski, J. (1924). Rynek Drzewny i Przegląd Leśniczy : Organ dla Handlu i Przemysłu Drzewnego (eng. Market Wood and Forestry Review: A Body for Trade and the Wood industry). Poznań: Rynek drzewny w Poznaniu, 24.
Berglund, B. E., and Ralska-Jasiewiczowa, M. (1986). “Pollen analysis and pollen diagrams,” in Handbook of Holocene Paleoecology and Paleohydrology, ed. B. E. Berglund (Toronto, ON: Wiley & Sons Ltd.), 455–484.
Beug, H.-J. (2004). Leitfaden der Pollenbestimmung für Mitteleuropa und Angrenzende Gebiete. München: Verlag Dr. Friedrich Pfeil.
Bialy, K. (1999). Dowolnosc wyrozniania typow siedliskowych lasu i projektowanie skladow docelowych drzewostanow w obrebie gleb bielicoziemnych (eng. Freedom to distinguish forest habitat types and designing the composition of target stands within the subterranean soils). Sylwan 143, 65–72.
Binford, M. W. (1990). Calculation and uncertainty analysis of 210 Pb dates for PIRLA project lake sediment cores. J. Paleolimnol. 3, 253–267.
Błaszkiewicz, M., Piotrowski, J. A., Brauer, A., Gierszewski, P., Kordowski, J., Kramkowski, M., et al. (2015). Climatic and morphological controls on diachronous postglacial lake and river valley evolution in the area of Last Glaciation, northern Poland. Quat. Sci. Rev. 109, 13–27. doi: 10.1016/j.quascirev.2014.11.023
Boczoń, A., Kowalska, A., Ksepko, M., and Sokołowski, K. (2018). Climate warming and drought in the Bialowieza forest from 1950–2015 and their impact on the dieback of Norway Spruce Stands. Water 10:1502. doi: 10.3390/w10111502
Booth, R. K., Jackson, S. T., and Gray, C. E. D. (2004). Paleoecology and high-resolution paleohydrology of a kettle peatland in upper Michigan. Quat. Res. 61, 1–13. doi: 10.1016/j.yqres.2003.07.013
Brando, P. M., Paolucci, L., Ummenhofer, C. C., Ordway, E. M., Hartmann, H., Cattau, M. E., et al. (2019). Droughts, wildfires, and forest carbon cycling: a pantropical synthesis. Annu. Rev. Earth Planet. Sci. 47, 555–581.
Broda, J. (2000). Historia Leśnictwa w Polsce (eng. History of Forestry in Poland). Wydaw. Akademii Rolniczej im. Augusta Cieszkowskiego. Poznań: Wydawnictwo Akademii Rolniczej.
Broda, J. (2003). Gradacje strzygoni choinowki w Lasach Panstwowych Wielkopolski i Pomorza Gdanskiego w okresie międzywojennym (eng. Gradations of christmas tree cutters in the state forests of Greater Poland and Pomerania Gdańsk in the interwar period). Stud. Mater. Ośrodka Kultury Leśnej 5, 77–94.
Broda, J. (2010). Lasy Państwowe Województwa Pomorskiego w Okresie Międzywojennym (eng. State Forests of the Pomeranian Voivodeship in the Interwar Period). Regionalna Dyrekcja Lasów państwowych w Toruniu Broda 2006, Lasy Panìstwowe Wojewoìdztwa Pomorskiego w Okresie międzywojennym. Toruń: Regionalna Dyrekcja Lasów Państwowych w Toruniu.
Bronk Ramsey, C. (1995). Radiocarbon calibration and analysis of stratigraphy: the OxCal program. Radiocarbon 37, 425–430.
Bronk Ramsey, C. (2006). OxCal Program v 4.0 [Software and Documentation]. Available online at: http://c14.arch.ox.ac.uk/oxcal/hlp_contents.html (accessed 2020).
Bronk Ramsey, C. (2008). Deposition models for chronological records. Quat. Sci. Rev. 27, 42–60. doi: 10.1016/j.quascirev.2007.01.019
Charman, D. J. (2007). Summer water deficit variability controls on peatland water-table changes: implications for Holocene palaeoclimate reconstructions. Holocene 17, 217–227. doi: 10.1177/0959683607075836
Dec, J. (1945). Lasy Brzozowe w Walce ze Szkodliwymi Owadami. Nakładem Oddziału Związku Zawodowego Pracowników Leśnych i Tartacznych Okręgu Toruńskiego. Toruń: nakł. Oddziału Zwia̧zków Zawodowych Pracowników Leśnych i Tartacznych Okrȩgu Toruńskiego.
del Río, M., Pretzsch, H., Ruíz-Peinado, R., Ampoorter, E., Annighöfer, P., Barbeito, I., et al. (2017). Species interactions increase the temporal stability of community productivity in Pinus sylvestris–Fagus sylvatica mixtures across Europe. J. Ecol. 105, 1032–1043. doi: 10.1111/1365-2745.12727
Dietze, E., Brykała, D., Schreuder, L. T., Jażdżewski, K., Blarquez, O., Brauer, A., et al. (2019). Human-induced fire regime shifts during 19th century industrialization: a robust fire regime reconstruction using northern Polish lake sediments. PLoS One 14:e0222011. doi: 10.1371/journal.pone.0222011
Dobrowolska, D. (2015). Forest regeneration in northeastern Poland following a catastrophic blowdown. Can. J. For. Res. 45, 1172–1182. doi: 10.1139/cjfr-2014-0507
Dyderski, M. K., Paź, S., Frelich, L. E., and Jagodziński, A. M. (2018). How much does climate change threaten European forest tree species distributions? Glob. Change Biol. 24, 1150–1163. doi: 10.1111/gcb.13925
Dysarz, R. (1998). Zarys Geomorfologii i Typy Krajobrazu Naturalnego w Północnej Części Borów Tucholskich in Park Narodowy Bory Tucholskie. Stan poznania przyrody na tle Kompleksu leśnego Bory Tucholskie (eng. Outline of Geomorphology and Types of Natural Landscape in the Northern Part of Bory Tucholskie in Bory Tucholskie National Park. The State of Knowledge of Nature Against the Background of the Bory Tucholskie Forest Complex), eds J. Banaszak and K. Tobolski (Bydgoszcz: Uczeln. WSP) 9–16.
European Severe Weather Database (2020). Selected Data from the Database. Available online at: https://www.eswd.eu/ (accessed May 05, 2020)
Fedrowitz, K., Koricheva, J., Baker, S. C., Lindenmayer, D. B., Palik, B., Rosenvald, R., et al. (2014). Can retention forestry help conserve biodiversity? A meta-analysis. J. Appl. Ecol. 51, 1669–1679. doi: 10.1111/1365-2664.12289
Fernández, R. D., Catalán, J. R. M., Arenas, R., Abati, J., Gerdes, A., and Fernández-Suárez, J. (2012). U–Pb detrital zircon analysis of the lower allochthon of NW Iberia: age constraints, provenance and links with the Variscan mobile belt and Gondwanan cratons. J. Geol. Soc. 169, 655–665. doi: 10.1144/jgs2011-146
Flynn, W. W. (1968). The determination of low-levels of polonium-210 in environmental materials. Anal. Chem. Acta 43, 221–227. doi: 10.1016/s0003-2670(00)89210-7
Fudała, A. (1985). Uwagi o gradacji brudnicy mniszki Lymantria monacha (L) w latach 1794-1984 (eng. Notes on the gradation of the nun moth Lymantria monacha (L) in 1794-1984). Wiadomości. Entomol. 6, 1–2.
Fujita, T. T. (1971). Proposed Characterization of Tornadoes and Hurricanes by Area and Intensity (No. NASA-CR-125545). Chicago, IL: University of Chicago.
Gardiner, B., Blennow, K., Carnus, J.-M., Fleischer, P., Ingemarson, F., Landmann, G., et al. (2010). Destructive Storms in European forests: Past and Forthcoming Impacts. Joensuu: European Forest Institute.
Gietkowski, T. (2009). Zmiany lesistości Borów Tucholskich w latach 1938 – 2000, (Temporal change of forest area in Tuchola Piewoods region between 1938 and 2000). Prom. Geogr. Bydgostien. 4, 149–162.
Gregow, H., Laaksonen, A., and Alper, M. (2017). Increasing large scale windstorm damage in Western, Central and Northern European forests, 1951–2010. Sci. Rep. 7:46397. doi: 10.1038/srep46397
Groenemeijer, P., and Kühne, T. (2014). A climatology of tornadoes in Europe: results from the European Severe weather database. Month. Weather Rev. 142, 4775–4790.
Grus, R. (1891). Brzoza, jej Opisanie, Uprawa i Znaczenie w Gospodarstwie Leśnym (eng. Birch, its Description, Cultivation and Importance in a Forest Holding). Nakładem Autor, Drugiem N. Kamińskiego i Spółki. Poznań: N. Kamińskiego i Spółki.
Grus, R. (1897). Uprawa i Pielęgnowanie Lasu; Rady dla Rolników i Leśników (eng. Forest Cultivation and Maintenance; Advice for Farmers and Foresters). Bibioteka Rolnicza II. Lwów: Jakubowski & Zadurowicz.
Gustafsson, L., Baker, S. C., Bauhus, J., Beese, W. J., Brodie, A., Kouki, J., et al. (2012). Retention forestry to maintain multifunctional forests: a world perspective. BioScience 62, 633–645.
Hua, Q., Barbetti, M., and Rakowski, A. Z. (2013). Atmospheric radiocarbon for the period 1950-2010. Radiocarbon 55, 2059–2072. doi: 10.1016/j.dib.2018.10.040
Institute of Meteorology and Water Management - National Research Institute (2019). Available online at: https://meteomodel.pl (accessed May, 2019).
Ireland, A. W., and Booth, R. K. (2012). Upland deforestation triggered an ecosystem state-shift in a kettle peatland. J. Ecol. 100, 586–596.
Ireland, A. W., Booth, R. K., Hotchkiss, S. C., and Schmitz, J. E. (2012). Drought as a trigger for rapid state shifts in kettle ecosystems: implications for ecosystem responses to climate change. Wetlands 32, 989–1000. doi: 10.1007/s13157-012-0324-6
Jablonski, T. (2015). Występowanie i zwalczanie leśnych foliofagów-trendy i prognozy (eng. Occurrence and control of forest foliophages - trends and forecasts. Post. Tech. Leśnictwie 132, 13–19.
Jagodziński, M. (2020). Every Drop Counts (Liczy się każda kropla). Echa leśne. Available online at: http://www.idpan.poznan.pl/images/Echa_Le%C5%9Bne_-_Liczy_si%C4%99_ka%C5%BCda_kropla.pdf?fbclid=IwAR2dhF0weIv8FSM4giHd8szpHCsj6D03IfPoCx8bVoVRD_6e5yHskLy9gX8 (accessed July 06, 2020).
Jaszczak, R. (2008). Urządzanie lasu w Polsce do 1939 roku. Część III – urządzanie lasu na ziemiach polskich w zaborze austriackim i pruskim. Forest management in Poland until 1939. Part III - forest management in Poland in the Austrian and Prussian Partitions. Sylwan 159, 3–10.
Jażdżewski, K. (2008). Administracja lasami państwowymi na obszarze RDLP w Gdańsku w latach 1815-1867. Administration of state forests in the area of RDSF in Gdańsk in 1815-1867. Sylwan 152, 56–61.
Juggins, S. (2003). C2 User Guide. Software for Ecological and Palaeoecological Data Analysis and Visualisation. Newcastle upon Tyne: University of Newcastle.
Kaleta, J. (2017). Największy Kataklizm w Gistorii Lasów PAÑSTWOWYCH (eng. The Greatest Cataclysm in the History of the STATE Forests). Available online at: https://www.krosno.lasy.gov.pl/aktualnosci/-/asset_publisher/1M8a/content/najwiekszy-kataklizm-w-historii-lasow-panstwowych#.XwcOCefgoUE (accessed July 06, 2020).
Karasiewicz, K. (1922). Przewodnik po Borach Tucholskich (eng. Guide to Bory Tucholskie.). Krajoznawczego: Nakładem Polskiego Tow.
Karasiewicz, K. (1926). Bory Tucholskie (eng. Tuchola Pineforest). “Ruch”. Kolejowych: Polskie Towarzystwo Księg.
Kasprzyk, K. (2021). Warunki Przyrodniczo-LEŚNE (eng. Natural and Forest Conditions). Available online at: https://trzebciny.torun.lasy.gov.pl/lasy-nadlesnictwa#.YZ8yyC-2x1t (accessed May 05, 2021).
Kint, V., Geudens, G., Mohren, G., and Lust, N. (2006). Silvicultural interpretation of natural vegetation dynamics in ageing Scots pine stands for their conversion into mixed broadleaved stands. For. Ecol. Manag. 223, 363–370. doi: 10.1016/j.foreco.2005.11.018
Knoke, T., Ammer, C., Stimm, B., and Mosandl, R. (2008). Admixing broadleaved to coniferous tree species: a review on yield, ecological stability and economics. Eur. J. For. Res. 127, 89–101. doi: 10.1007/s10342-007-0186-2
Kołaczek, P., Gałka, M., Apolinarska, K., Płóciennik, M., Gąsiorowski, M., Brooks, S. J., et al. (2018). A multi-proxy view of exceptionally early postglacial development of riparian woodlands with Ulmus in the Dniester River valley, western Ukraine. Rev. Palaeobot. Palynol. 250, 27–43. doi: 10.1016/j.revpalbo.2017.12.001
Kordowski, J., Brykała, D., Bartczak, A., Kaczmarek, H., and Słowiński, M. (2010). Antropogeniczne i Naturalne Przemiany środowiska Geograficznego Województwa Kujawsko-Pomorskiego: Wybrane Przykłady (eng. Anthropogenic and Natural Transformations of the Geographical Environment of the Kuyavian-Pomeranian Voivodeship: Selected Examples). Warszawa: IGiPZ PAN.
Koziński, G. (2007). Model Przestrzenny Szkód Wywołanych Przez Wiatr w Drzewostanach borów Tucholskich (eng. Spatial Model of Wind Damage in Forest Stands of Bory Tucholskie). Maszynopis Rozprawy Doktorskiej. Toruń: Uniwersytet Mikołaja Kopernika w Toruniu. Wydział Biologii i Nauki o Ziemi.
Lamentowicz, M., and Mitchell, E. A. D. (2007). “Testate amoebae as ecological and palaeohydrological indicators in peatlands - The polish experience,” in Proceeding of the International Conference W3M “Wetlands: Monitoring, Modelling and Management”, Wierzba, Poland, 22-25 September 2005, ed. T. Okruszko (Leiden: Taylor & Francis), 85–90.
Lamentowicz, M., and Obremska, M. (2010). A rapid response of testate amoebae and vegetation to inundation of a kettle hole mire. J. Paleolimnol. 43, 499–511. doi: 10.1007/s10933-009-9347-2
Lamentowicz, M., Kajukało-Drygalska, K., Kołaczek, P., Jassey, V. E., Gąbka, M., and Karpińska-Kołaczek, M. (2020). Testate amoebae taxonomy and trait diversity are coupled along an openness and wetness gradient in pine-dominated Baltic bogs. Eur. J. Protistol. 73:125674. doi: 10.1016/j.ejop.2020.125674
Lamentowicz, M., and Lamentowicz, Ł, and Payne, R. (2013). Towards quantitative reconstruction of peatland nutrient status from fens. Holocene 23, 1659–1663.
Lamentowicz, M., Mueller, M., Gałka, M., Barabach, J., Milecka, K., Goslar, T., et al. (2015). Reconstructing human impact on peatland development during the past 200 years in CE Europe through biotic proxies and X-ray tomography. Quat. Int. 357, 282–294. doi: 10.1016/j.quaint.2014.07.045
Lamentowicz, M., Tobolski, K., and Mitchell, E. A. D. (2007). Palaeoecological evidence for anthropogenic acidification of a kettle-hole peatland in northern Poland. Holocene 17, 1185–1196. doi: 10.1177/0959683607085123
Lee, S.-H., Ji, W., Lee, W.-S., Koo, N., Koh, I. H., Kim, M.-S., et al. (2014). Influence of amendments and aided phytostabilization on metal availability and mobility in Pb/Zn mine tailings. J. Environ. Manag. 139, 15–21. doi: 10.1016/j.jenvman.2014.02.019
Leuschner, C., and Ellenberg, H. (2017). Ecology of Central European Non-Forest Vegetation: Coastal to Alpine, Natural to Man-Made Habitats: Vegetation Ecology of Central Europe, Vol. II. Berlin: Springer.
Liang, J., Crowther, T. W., Picard, N., Wiser, S., Zhou, M., Alberti, G., et al. (2016). Positive biodiversity-productivity relationship predominant in global forests. Science 354:aaf8957. doi: 10.1126/science.aaf8957
Łuców, D., Lamentowicz, M., Kołaczek, P., Łokas, E., Lane, C., Tjallingii, R., et al. (2018). An impact of past catastrophic deforestations on the hydrology of Sphagnum peatland in Northern Poland. EGU Gen. Assemb. Conf. Abstr. 20:1017.
Łuców, D., Lamentowicz, M., Obremska, M., Arkhipova, M., Kittel, P., Łokas, E., et al. (2020). Disturbance and resilience of a Sphagnum peatland in western Russia (Western Dvina Lakeland) during the last 300 years: a multiproxy, high-resolution study. Holocene 30, 1552–1566.
Marcisz, K., Lamentowicz, M., Gałka, M., Colombaroli, D., Adolf, C., and Tinner, W. (2019). Responses of vegetation and testate amoeba trait composition to fire disturbances in and around a bog in central European lowlands (northern Poland). Quat. Sci. Rev. 208, 129–139. doi: 10.1016/j.quascirev.2019.02.003
Matusick, G., Hudson, S. J., Garrett, C. Z., Samuelson, L. J., Kent, J. D., Addington, R. N., et al. (2020). Frequently burned loblolly–shortleaf pine forest in the southeastern United States lacks the stability of longleaf pine forest. Ecosphere 11:e03055.
Matuszkiewicz, W. (1999). “Szata roślinna (eng. Vegetable cover),” in Geografia Polski Środowisko Przyrodnicze, ed. L. Starkel (Warszawa: Wydawnictwo Naukowe PWN), 427–475.
Matuszkiewicz, W., Faliński, J. B., Kostrowicki, A. S., Matuszkiewicz, J. M., Olaczek, R., and Wojterski, T. (eds) (1995). Potencjalna Roślinność Naturalna Polski. Mapa przeglądowa 1:300 000 (Potential natural Vegetation of Poland. Outline Map 1: 300,000). Arkusze 1-12. Warszawa: IGiPZ PAN.
Milecka, K., and Szeroczyńska, K. (2005). Changes in macrophytic flora and planktonic organisms in lake Ostrowite, Poland as a response of climatic and trophy fluctuations. Holocene 15, 74–84.
Miotk-Szpiganowicz, G. (1990). Historia Lasów Borów Tucholskich i ich Antropogeniczne Przemiany, [w:] K. Tobolski (red). Paleoekologia i Paleolimnologia Postglacjału niżu Polskiego (na Przykładzie środkowej Wielkopolski i Borów Tucholskich) (eng. Paleoecology and Palaeolimnology of the Post-Glacial Polish Lowlands (On the Example of Central Wielkopolska and Bory Tucholskie)). Warszawa, SGGW-AR, 20–28.
Mitchell, E. A. D., Charman, D. J., and Warner, B. G. (2007). Testate amoebae analysis in ecological and paleoecological studies of wetlands: past, present and future. Biodiv. Conserv. 17, 2115–2137. doi: 10.1007/s10531-007-9221-3
Mokrzecki, Z. (1928). Strzygonia Choinówka. Panolla Llammea Schiff.) Monografia. Warszawa: Związek Zawod. Leśników w Rzplitej Polskiej.
Moore, P. D., Webb, J. A., and Collinson, M. E. (1991). Pollen Analysis. Hoboken, NJ: Blackwell Scientific Publication.
Mrotzek, A., Couwenberg, J., Theuerkauf, M., and Joosten, H. (2017). MARCO POLO – a new and simple tool for pollen-based stand-scale vegetation reconstruction. Holocene 27, 321–330. doi: 10.1177/0959683616660171
Noryśkiewicz, A. (2006). Historia Cisa w Okolicy Wierzchlasu w Świetle Analizy Pyłkowej. Toruń: Instytut Archeologii UML w Toruniu, Towarzystwo Przyjaciół Dolnej Wisły.
Obremska, M. (2006). Dynamics of Sediments Accumulation in Area Influenced by Żur Dam Lake (Tuchola Pinewoods) (In Polish: Dynamika Akumulacji Biogenicznej w Obszarze Oddzialywania Zalewu Żur (Bory Tucholskie). Ph.D. thesis. Adama Mickiewicza: Uniwersytet im. Adama Mickiewicza.
Obremska, M., and Lamentowicz, M. (2006). “An impact of Żur dam lake on the peat accumulation process in a kettle-hole mire in Tuchola Pinewoods (In Polish: wpływ zalewu Żurskiego na procesy akumulacji osadów torfowiska kotłowego Mukrza w Borach Tucholskich),” in Conference: Przemiany środowiska Geograficznego Polski Północno-Zachodniej, Ogólnopolska Konferencja Naukowa Poświęcona Pamięci Profesora Bogumiła Krygowskiego, Poznań 20-21 marca 2006, eds A. Kostrzewski and J. Czerniawska, Poznań, 151.
Pączewski, L. (1924). Lasy, Przemysł i Handel Drzewny w Polsce (eng. Forests, Industry and Timber Trade in Poland). Instytut Wydawniczy. Warszawa: Bibljoteka Polska.
Payne, R. J., Malysheva, E., Tsyganov, A., Pampura, T., Novenko, E., Volkova, E., et al. (2015). A multi-proxy record of Holocene environmental change, peatland development and carbon accumulation from Staroselsky Moch peatland, Russia. Holocene 26, 314–326. doi: 10.1177/0959683615608692
Pretzsch, H., Biber, P., Schütze, G., Uhl, E., and Rötzer, T. (2014). Forest stand growth dynamics in Central Europe have accelerated since 1870. Nat. Commun. 5:4967. doi: 10.1038/ncomms5967
Ramsey, C. B., and Lee, S. (2013). Recent and planned developments of the program OxCal. Radiocarbon 55, 720–730. doi: 10.1017/s0033822200057878
Reimer, P. J., Austin, W. E., Bard, E., Bayliss, A., Blackwell, P. G., Ramsey, C. B., et al. (2020). The IntCal20 Northern Hemisphere radiocarbon age calibration curve (0–55 cal kBP). Radiocarbon 62, 725–757. doi: 10.1017/rdc.2020.41
Rutkowska, P. (2019). Przebieg Procesu Bielicowania w Cyklu Uprawy Monokultur Sosnowych w Wybranych Obszarach Piaszczystych Polski Północnej (eng. The Course of the Podzolic Process in the Cycle of Pine Monoculture Cultivation in Selected Sandy Areas of Northern Poland). Doctoral dissertation. Toruń: Uniwersytet Mikolaja Kopernika w Toruniu.
Sanchez-Cabeza, J., and Ruiz-Fernández, A. (2012). 210Pb sediment radiochronology: an integrated formulation and classification of dating models. Geochim. Cosmochim. Acta 82, 183–200.
Schafstall, N., Whitehouse, N., Kuosmanen, N., Svobodová-Svitavská, H., Saulnier, M., Chiverrell, R. C., et al. (2020). Changes in species composition and diversity of a montane beetle community over the last millennium in the High Tatras, Slovakia: implications for forest conservation and management. Palaeogeogr. Palaeoclimatol. Palaeoecol. 555:109834. doi: 10.1016/j.palaeo.2020.109834
Seidl, R., Honkaniemi, J., Aakala, T., Aleinikov, A., Angelstam, P., Bouchard, M., et al. (2020). Globally consistent climate sensitivity of natural disturbances across boreal and temperate forest ecosystems. Ecography 43, 967–978. doi: 10.1111/ecog.04995
Seidl, R., Schelhaas, M.-J., Rammer, W., and Verkerk, P. J. (2014). Increasing forest disturbances in Europe and their impact on carbon storage. Nat. Clim. Change 4:806. doi: 10.1038/nclimate2318
Seidl, R., Thom, D., Kautz, M., Martin-Benito, D., Peltoniemi, M., Vacchiano, G., et al. (2017). Forest disturbances under climate change. Nat. Clim. Change 7, 395–402.
Senf, C., and Seidl, R. (2021). Storm and fire disturbances in Europe: distribution and trends. Global Change Biol. 27, 3605–3619. doi: 10.1111/gcb.15679
Shikhov, A., and Chernokulsky, A. (2018). A satellite-derived climatology of unreported tornadoes in forested regions of northeast Europe. Remote Sens. Environ. 204, 553–567. doi: 10.1016/j.rse.2017.10.002
Skrzecz, I., and Perlińska, A. (2018). Current problems and tasks of forest protection in Poland. Folia Forest. Polon. 60, 161–172. doi: 10.2478/ffp-2018-0016
Sliwa, E. (1989). Przebieg masowego pojawu brudnicy mniszki (Lymantria monacha L.) i jej zwalczania w Polsce w latach 1978-1985 oraz regeneracja aparatu asymilacyjnego w uszkodzonych drzewostanach (eng. The course of the mass outbreak of the nun moth (Lymantria monacha L.) and its control in Poland in 1978-1985 and the regeneration of the assimilation apparatus in damaged stands). Prace Inst. Badaw. Leśnic. 710, 3–120.
Słowiński, M. (2010). Macrofossil reconstruction of Preboreal wetland formed on dead ice block: a case study of the Borzechowo mire in East Pomerania, Poland. Stud. Quat. 27, 3–10.
Słowiński, M., Błaszkiewicz, M., Brauer, A., Noryśkiewicz, B., Ott, F., and Tyszkowski, S. (2014). The role of melting dead ice on landscape transformation in the early Holocene in Tuchola Pinewoods, North Poland. Quat. Int. 388, 64–75.
Słowiński, M., Błaszkiewicz, M., Brauer, A., Noryśkiewicz, B., Ott, F., and Tyszkowski, S. (2015). The role of melting dead ice on landscape transformation in the early Holocene in Tuchola Pinewoods, North Poland. Quat. Int. 388, 64–75.
Słowiński, M., Lamentowicz, M., Łuców, D., Barabach, J., Brykała, D., Tyszkowski, S., et al. (2019). Paleoecological and historical data as an important tool in ecosystem management. J. Environ. Manag. 236, 755–768. doi: 10.1016/j.jenvman.2019.02.002
Słowiński, M., Lamentowicz, M., Łuców, D., Kołaczek, P., Tjallingii, R., Noryśkiewicz, A. M., et al. (2017). Tornado Project-The Impact of Catastrophic Deforestation on the Lake and Peatland Ecosystems of the Tuchola Pinewoods, Northern Poland. Vienna: EGU General Assembly, 10168.
State Forests data (2020). Available online at: www.lasy.gov.pl/en (accessed November, 2020).
Steckel, M., Del Río, M., Heym, M., Aldea, J., Bielak, K., Brazaitis, G., et al. (2020). Species mixing reduces drought susceptibility of Scots pine (Pinus sylvestris L.) and oak (Quercus robur L., Quercus petraea (Matt.) Liebl.)–Site water supply and fertility modify the mixing effect. For. Ecol. Manag. 461:117908.
Stieber, K., and Bartz, E. (1923). Rynek Drzewny i Przegląd Leśniczy : Organ dla Handlu i Przemysłu Drzewnego (eng. Market Wood and Forestry Review: a Body for Trade and the Wood Industry). Poznań: Rynek drzewny w Poznaniu, 33.
Stopiński, M. (2012). Trąba Powietrzna Spustoszyła lasy (Eng. The Tornado Ravaged the Forests). Biuletyn Regionalnej Dyrekcji Lasów państwowych w Toruniu 3/64. Toruń: Regionalna Dyrekcja Lasów Pañstwowych w Toruniu.
Sugita, S. (2007). Theory of quantitative reconstruction of vegetation I: pollen from large sites REVEALS regional vegetation composition. Holocene 17, 229–241. doi: 10.1177/0959683607075837
Sukovata, L., and Kolk, A. (2000). Wplyw Defoliacji Drzew i Drzewostanow Sosnowych Spowodowanej Przez Strzygonie Choinowke [Panolis Flammea Den. et Schiff.] Na ich Przezywalnosc w Krajach Srodkowej i Wschodniej Europy (eng. Effect of Defoliation of Pine Trees and Stands Caused by Christmas Tree Shrubs [Panolis Flammea Den. et Schiff.] on their Viability in the Countries of Central and Eastern Europe). Warszawa: Sylwa.
Swindles, G. T., Morris, P. J., Mullan, D. J., Payne, R. J., Roland, T. P., Amesbury, M. J., et al. (2019). Widespread drying of European peatlands in recent centuries. Nat. Geosci. 12, 922–928.
Szmyt, J., and Dobrowolska, D. (2016). Spatial diversity of forest regeneration after catastrophic wind in northeastern Poland. iFor. Biogeosci. For. 9, 414.
Taszarek, M. (2016). Charakterystyka Występowania Burz Oraz trąb Powietrznych na Obszarze Polski (Eng. Characteristics of the Occurrence of Storms and Whirlwinds in the Territory of Poland). Poznan: Adam Mickiewicz in Poznan.
Taszarek, M., and Gromadzki, J. (2017). Deadly tornadoes in Poland from 1820 to 2015. Month. Weather Rev. 145, 1221–1243.
Taszarek, M., Czernecki, B., Walczakiewicz, S., Mazur, A., and Kolendowicz, L. (2016). An isolated tornadic supercell of 14 July 2012 in Poland—A prediction technique within the use of coarse-grid WRF simulation. Atmos. Res. 178, 367–379. doi: 10.1016/j.atmosres.2016.04.009
Theuerkauf, M., and Couwenberg, J. (2018). ROPES reveals past land cover and PPEs from single pollen records. Front. Earth Sci. 6:14.
Theuerkauf, M., Couwenberg, J., Kuparinen, A., and Liebscher, V. (2016). A matter of dispersal: revealsinr introduces state-of-the-art dispersal models to quantitative vegetation reconstruction. Veg. Hist. Archaeobot. 25, 541–553. doi: 10.1007/s00334-016-0572-0
Thurm, E. A., Uhl, E., and Pretzsch, H. (2016). Mixture reduces climate sensitivity of Douglas-fir stem growth. For. Ecol. Manag. 376, 205–220.
Tobolski, K. (2000). Przewodnik do Oznaczania Torfów i Osadów Jeziornych (eng. Guide to the Determination of Peat and Lake Sediment). Warszawa: PWN.
Tomaś, Ł, and Jagodziński, A. M. (2019). Przebudowa Drzewostanów (Eng. Reconstruction of Stands). Warszawa: ACADEMIA-magazyn Polskiej Akademii Nauk, 94–97.
Trębski, K. (2019). Największy Kataklizm w Historii Lasów państwowych. Dyrekcja Lasów państwowych (Eng. The greatest cataclysm in the history of the State Forests. Directorate of State Forests). Available online at: https://www.lasy.gov.pl/pl/informacje/aktualnosci/najwieksza-taka-kleska-w-historii-polskich-lasow (accessed June 02, 2020).
Tylmann, W., Bonk, A., Goslar, T., Wulf, S., and Grosjean, M. (2016). Calibrating 210Pb dating results with varve chronology and independent chronostratigraphic markers: problems and implications. Quat. Geochronol. 32, 1–10. doi: 10.1016/j.quageo.2015.11.004
Ummenhofer, C. C., and Meehl, G. A. (2017). Extreme weather and climate events with ecological relevance: a review. Philos. Trans. R. Soc. Lond. B Biol. Sci. 372:20160135. doi: 10.1098/rstb.2016.0135
van Geel, B. (1978). A palaeoecological study of Holocene peat bog sections in Germany and the Netherlands. Review of Palaeobotany and Palynology 25, 1–120.
Wardenaar, E. C. P. (1987). A new hand tool for cutting peat profiles. Can. J. Bot. 65, 1772–1773. doi: 10.1139/b87-243
Załoga, K. (2014). Ochrona Lasu (Forest Protection). Available online at: https://trzebciny.torun.lasy.gov.pl/ochrona-lasu#.YZ80Dy-2x1u (accessed July 06, 2020).
Zerbe, S. (2002). Restoration of natural broad-leaved woodland in Central Europe on sites with coniferous forest plantations. For. Ecol. Manag. 167, 27–42. doi: 10.1016/s0378-1127(01)00686-7
Zhang, Y., Chen, H. Y., and Reich, P. B. (2012). Forest productivity increases with evenness, species richness and trait variation: a global meta-analysis. J. Ecol. 100, 742–749. doi: 10.1111/j.1365-2745.2011.01944.x
Ziółkowski, J., and Bartz, E. (1928). Rynek Drzewny i Budowlany : Organ Dla Handlu i Przemysłu Drzewnego Oraz Budowlanego (eng. The Timber and Construction Market: An Authority for the Wood and Construction Trade and Industry): Oficjalny Organ Związku Przemysłowców Drzewnych w Poznaniu : Oficjalny Organ Giełdy Drzewnej w Bydgoszczy. Poznań: Rynek drzewny w Poznaniu, 18.
Keywords: tornado, peatland, paleoecology, pollen analysis - REVEALS, monocultures, insect outbreaks
Citation: Łuców D, Lamentowicz M, Kołaczek P, Łokas E, Marcisz K, Obremska M, Theuerkauf M, Tyszkowski S and Słowiński M (2021) Pine Forest Management and Disturbance in Northern Poland: Combining High-Resolution 100-Year-Old Paleoecological and Remote Sensing Data. Front. Ecol. Evol. 9:747976. doi: 10.3389/fevo.2021.747976
Received: 27 July 2021; Accepted: 18 November 2021;
Published: 15 December 2021.
Edited by:
Gianluca Piovesan, University of Tuscia, ItalyReviewed by:
Lee E. Frelich, University of Minnesota Twin Cities, United StatesMats Niklasson, Swedish University of Agricultural Sciences, Sweden
Copyright © 2021 Łuców, Lamentowicz, Kołaczek, Łokas, Marcisz, Obremska, Theuerkauf, Tyszkowski and Słowiński. This is an open-access article distributed under the terms of the Creative Commons Attribution License (CC BY). The use, distribution or reproduction in other forums is permitted, provided the original author(s) and the copyright owner(s) are credited and that the original publication in this journal is cited, in accordance with accepted academic practice. No use, distribution or reproduction is permitted which does not comply with these terms.
*Correspondence: Dominika Łuców, ZG9taW5pa2EubHVjb3dAdHdhcmRhLnBhbi5wbA==
†ORCID: Dominika Łuców, orcid.org/0000-0001-9966-7478; Mariusz Lamentowicz, orcid.org/0000-0003-0429-1530; Piotr Kołaczek, orcid.org/0000-0003-2552-8269; Edyta Łokas, orcid.org/0000-0001-9996-6523; Katarzyna Marcisz, orcid.org/0000-0003-2655-9729; Milena Obremska, orcid.org/0000-0002-3465-1894; Martin Theuerkauf, orcid.org/0000-0002-4033-3040; Sebastian Tyszkowski, orcid.org/0000-0001-7861-5492; Michał Słowiński, orcid.org/0000-0002-3011-2682