- 1Swiss Ornithological Institute, Sempach, Switzerland
- 2Michael-Otto-Institut im NABU, Bergenhusen, Germany
- 3Independent Researcher, Gendrey, France
- 4Institut Pluridisciplinaire Hubert Curien, Department of Ecology, Physiology and Ethology, Strasbourg, France
Human recreational activities increase worldwide in space and frequency leading to higher rates of encounter between humans and wild animals. Because wildlife often perceive humans as predators, this increase in human disturbance may have negative consequences for the individuals and also for the viability of populations. Up to now, experiments on the effects of human disturbance on wildlife have mainly focused on individual behavioral and stress-physiological reactions, on breeding success, and on survival. However, the effects on other physiological parameters and trans-generational effects remain poorly understood. We used a low-intensity experimental disturbance in the field to explore the impacts of human disturbance on telomere length in great tit (Parus major) populations and found a clear effect of disturbance on telomere length. Adult males, but not females, in disturbed plots showed shorter telomere lengths when compared to control plot. Moreover, variation in telomere length of adult great tits was reflected in the next generation, as we found a positive correlation between telomere length of the chicks and of their fathers. Given that telomere length has been linked to animal lifespan, our study highlights that activities considered to be of little concern (i.e., low levels of disturbance) can have a long-lasting impact on the physiology and survival of wild animals and their next generation.
Introduction
Outdoor recreational activities are increasing worldwide, and areas that were previously only rarely accessed and disturbed are now being exposed to an ever-greater amount of human visitation (Balmford et al., 2009; Buckley, 2009; Hammitt et al., 2015). This increases the frequency of human-wildlife encounters, which raises concerns among researchers and conservationists about the potential consequences for wildlife. Wild animals often perceive humans as predators (Frid and Dill, 2002) and accordingly activate physiological and behavioral reactions, which may have negative consequences, such as offspring abandonment or energy loss (Cott, 1969; Weimerskirch et al., 2002; Tablado and Jenni, 2017).
Most studies investigating the impact of human disturbance on wild animals focused on the behavioral and physiological reactions to human stressors, such as flushing behavior after a human approach (Stankowich and Blumstein, 2005; Blumstein, 2006; Møller, 2010; Bötsch et al., 2018a) or levels of baseline and stress-induced glucocorticoids in animals exposed to anthropogenic disturbance (Walker et al., 2006; French et al., 2010; Bonier, 2012; Muehlenbein et al., 2012). Other studies have investigated how disturbance affects further biological parameters such as reproduction (Carney and Sydeman, 1999; Ellenberg et al., 2006; Strasser and Heath, 2013), survival (Ruhlen et al., 2003; López-Roig and Serra-Cobo, 2014; Gibson et al., 2018), or movement and habitat use (Tablado et al., 2016; Bötsch et al., 2017, 2018b; Doherty et al., 2021). Some researchers have also examined potential transgenerational effects of human disturbance transmitted via maternal hormones or antibodies to their offspring (Bertin et al., 2008; Bötsch et al., 2020). However, the impact of human disturbance on the DNA, such as on telomere length or attrition, remains still largely unexplored in wild animals (but see Angelier et al., 2018; Injaian et al., 2019).
Telomeres are highly repetitive sections of DNA that cap the ends of chromosomes in most eukaryote species and serve to maintain chromosome integrity (Blackburn, 1991), but shorten with cell replication and oxidative stress (Olovnikov, 1973; Von Zglinicki, 2002; Stewart et al., 2003). Individuals with longer telomeres for their age are generally found to have better survival prospects, both in humans (reviewed by Boonekamp et al., 2013) and other vertebrates, mainly mammals and birds (reviewed by Wilbourn et al., 2018). Explaining between and within individual variation in telomere length therefore has become an important topic in the study of life histories and can be used as an indicator of longevity.
Telomere length and accelerated telomere attrition have been previously linked to stress in human and non-human vertebrates (Monaghan, 2014; Bateson, 2016; Oliveira et al., 2016; Coimbra et al., 2017; Chatelain et al., 2020; Rentscher et al., 2020). Although the exact mechanisms underlying this link are still being studied, it appears that glucocorticoids could mediate the association between environmental stressors and telomere dynamics (Angelier et al., 2018; Casagrande et al., 2020; Powolny et al., 2020). When exposed to stressors, vertebrates react by mounting a series of behavioral and physiological stress responses, among which there is an increase in glucocorticoid secretion (Sapolsky et al., 2000; Tablado and Jenni, 2017). Glucocorticoids enhance metabolic rates and energy mobilization for coping with the stressors, and these usually result in higher oxidative stress levels leading to DNA damage and telomere shortening (Casagrande et al., 2020; Chatelain et al., 2020). Other mechanisms through which glucocorticoids have been suggested to affect telomere length include reduced antioxidant defenses through genomic effects and the modulation of telomerase activity (Kratschmar et al., 2012; Angelier et al., 2018).
Frequent encounters with humans, which often increase circulating glucocorticoids (Tablado and Jenni, 2017), could act as stressors and, therefore, are likely to have a direct impact on telomere length in wildlife. A second way in which human disturbance could affect telomere length of wild populations would be indirectly through generating stressful environments, which tend to be occupied by low-quality individuals with shorter telomeres that are displaced from better environments. Human disturbance could have thus, have both direct effects on telomere length, for example, through inducing high levels of glucocorticoids in blood (Angelier et al., 2018; Casagrande et al., 2020), and/or indirect effects, through altering settlement patterns (Bötsch et al., 2017), in that disturbed areas are used mainly by low-quality individuals with shorter telomeres. Some studies have investigated the effect of traffic noise on telomere length (Meillère et al., 2015; Injaian et al., 2019); however, to our knowledge no previous study has assessed the impact of the disturbance caused through the mere presence of humans on wildlife telomere dynamics.
The aim of this study was to experimentally test whether human recreational activities affect telomere length in wild bird populations. For this purpose, we compared telomere length of free-living adult great tits (Parus major) which had been exposed to an experimental disturbance treatment during the early breeding season with telomere length of adults hardly exposed to humans. Moreover, we examined whether potential variations in telomere length experienced by disturbed adults would be reflected in their offspring. We hypothesized that our experimental disturbance would lead to shorter telomere length in adults, through direct or indirect effects, and that these telomere length patterns would appear also in the next generation, through a correlation between telomere length in the chicks and in their parents. Given the negative association between telomere length and survival (Wilbourn et al., 2018), studies like ours are essential to understand the magnitude of the potential long-term effects of human disturbance on wildlife.
Materials and Methods
Study Area and Experimental Design
Our study took place in the Forêt domaniale de Chaux near Dole in eastern France (47°05′N, 05°40′E) in 2015. This mixed deciduous forest covers 200 km2 and consists mainly of pedunculate oaks (Quercus robur) and European hornbeam (Carpinus betulus). We selected 12 plots of well-structured, mature forest, with large old trees, some understory and dead wood, in which no timber harvesting happened during the study period. The plots were at least 9 km from the nearest town (Dole, 23,000 inhabitants) and the frequency of human recreational activities was extremely low (primarily in autumn during mushroom collection and hunting). During our fieldwork (daily from March to June, 50 h per week) we rarely saw people off-trail within the plots (less than one person per month) and we saw approximately one person per week on the gravel roads bordering the study plots.
In February 2015 we mounted 210 nestboxes (Schwegler, B1, with 32 mm entrance diameter) for small cavity nesters (mainly tit species) in the twelve plots. As the plots had different sizes (mean 9.2 ha, range: 7.5–13 ha), we adjusted the number of nest boxes per plot, so that the nest box density per plot was approximately the same and did not exceed the natural breeding density of tits [1.9 boxes per hectare, 60 m minimal distance between neighboring boxes (Krebs, 1971)].
Each plot was divided into two halves, called split-plots, one receiving the disturbance treatment and the other serving as control. The applied treatment was a human disturbance/intrusion event applied in early spring during territory settlement of the focal birds. A group of two to three people walked through the split-plot on a regular mower-pattern (distance between walking lines 20 m), which allowed a homogenous disturbance of the entire split-plots. The direction of the mower-pattern walks was turned 90° and the starting point and time of day was varied between successive disturbance events to avoid animals getting used to the disturbance. The group carried a loudspeaker (Hama, smartphone speaker, power 3W) which continuously played human conversation (discussions, interviews, or TV-news-presentations in different languages) at an average human-conversation volume level of approximately 60 dB at 1 m distance (Byrne et al., 1994; Hacki, 1996). The goal was to mimic human outdoor recreational activities, namely hiking through forests in a small group. The plots were well separated from each other with a minimal distance of 600 m to prevent confounding effects of neighboring treatments (for more details see Bötsch et al., 2017). Each plot was bordered on one side by a gravel road (closed to the public for cars) which was at the border of the disturbed split-plot in six plots and at the border of the control split-plot in the other six plots. In each split-plot, the disturbance events lasted about 45 min and occurred one to three (mean 2.3) times per day during daytime from March 7 to April 22 (in total 105 disturbance events during 46 days in each split-plot). Afterward, the plots were only entered for nest box checks and catching of the adults. From the end of April, all 210 nestboxes were checked every second week to determine nestbox occupation. Nestboxes occupied by great tits were checked daily to determine the precise hatching date and the number of hatchlings. To minimize the risk of brood abandonment, we caught the adults at the earliest 8 days after the chicks hatched.
Sample Collection
For the telomere analysis of the adult great tits we used the red blood cells (erythrocytes) from a small blood sample (sampling technique see below), whereas for the chicks some growing body feathers were used. Note that telomere lengths in feathers and blood has been previously shown to be correlated (Kärkkäinen et al., 2020). When the chicks were at least 8 days old, we started to catch adults while they were feeding their chicks by closing the entrance hole of the nestbox manually from the outside. Adults were quickly taken out of the box to take a first blood sample within 3 min after closing the entrance hole. This allowed determining baseline corticosterone levels, as corticosterone increases quickly after 3 min (Romero and Romero, 2002). The blood sample (up to 30 μl) was taken by vein puncturing with a 0.3 mm needle and the effluent blood was collected with a heparinized capillary and stored in Eppendorf tubes in the cool. Blood samples were centrifuged in the field for 5 min at 8,000 rpm (Hettich, EBA 3S) and plasma and cells were stored separately in liquid nitrogen or deep freezers (−20°C) until analysis. We measured the bird’s tarsus length, weighed, and sexed them according to plumage characters (adults only). All birds were ringed with the official French aluminum rings (C.R.B.P.O., Centre de Recherches sur la Biologie des Populations d’Oiseaux). To measure the reactivity of the hypothalamic–pituitary–adrenal axis (HPA) with a standardized capture and handling protocol (Wingfield et al., 1994), the birds were kept in a cloth bag and a second blood sample was taken on the other wing 30 min after capture (stress-induced corticosterone level).
Nestlings were weighed and ringed when they were about 14 days old (13–15 days). The number of ringed nestlings per brood at this age reflects the number of fledglings, because nestling mortality during the last days in the nest is very low (Linden et al., 1992; Oddie, 2000). For genetic sexing and the telomere analysis, a few small feathers from the underside were plucked and stored in Eppendorf tubes, immediately frozen and then stored in liquid nitrogen or deep freezers (−20°C) until analysis.
From a total of 140 great tit broods in the 12 plots, we selected 10 first broods from control split-plots and 10 first broods from disturbed split-plots. Of these 20 broods, we had obtained both blood samples (one for baseline and one for stress-level corticosterone measures) from both adults. On average 9.7 chicks per brood fledged from these 20 broods. Due to financial constraints we had to randomly select six chicks from each brood for the telomere analysis.
The study was approved by the local authorities and the French ringing scheme C.R.B.P.O. (permit number 2014157-0012 of the Direction Régionale de l’Environnement, de l’Aménagement et du Logement de Franche-Comté and permit number 15006 for 2014–2016 from the C.R.B.P.O., for details see also Bötsch et al., 2017).
Laboratory Analyses: Telomere Length, Genetic Sexing, and Corticosterone
Telomere length was measured in DNA extracted (2.5 years after sample collection) either from red blood cells in adults [applying the Nucleospin Blood Quickpure kit (Macherey-Nagel, Düren, Germany to stress-level blood samples)] or from feathers in chicks [using the Nucleospin tissue kit (Macherey Nagel, Düren, Germany)] with a quantitative real-time amplification (qPCR) previously used in birds (Criscuolo et al., 2009). The qPCR were done immediately after DNA extraction using a Bio-Rad CFX384 system (Biorad Hercules, United States). Previous to qPCR analyses, DNA quantity and quality were assessed based on spectrophotometer absorbance (Nanodrop 1000 Thermo Scientific, ratios A260/A280 and A260/A230) and gel-migration. The qPCR telomere measurement produces a relative telomere length expressed as the T/S ratio between telomere (T) and control genomic sequence (S) amplifications. T and S values were calculated following (Pfaffl, 2001), based on the number of amplification cycles of each sequence, controlled for the efficiencies of each reaction. Individual T/S ratios are expressed relatively to a randomly chosen individual, for which the value is set at 1 (golden sample). We used the P. major (great tit) EGR1 (ZENK), early growth response 1 gene (Gene ID: 107210829) as a control gene, with the primers ZENK1: (5′-TACATGTGCCATGGTTTTGC-3′) and ZENK2: (5′-AAGTGCTGCTCCCAAAGAAG-3′). The primers used for the telomere sequence amplification were: Tel1b (5′-CGGTTTGTTTGGGTTTGGGTTTGGGTTTGGGTTTGGGTT-3′) andTel2b (5′-GGCTTGCCTTACCCTTACCCTTACCCTT ACCCTTACCCT-3′). Both qPCR (control gene and telomere) was performed in a total volume of 10 μL. Primer concentrations in the final mix were 100 nM for telomere and 500 nM for the control gene. We used 2 ng DNA per reaction and GoTaq qPCR mix (Promega, Madison, WI, United States). All samples were measured in one run of qPCR, containing one plate for the telomere sequence amplification and one plate for the ZENK gene amplification. Real-time amplification of TL and Zenk sequences was performed in separate 384 well plates filled by a calibrated automated liquid handling workstation (Epmotion, Eppendorf, Montesson, France). All samples were run in duplicates. We run all chick and adult samples on the same plate. We choose randomly one sample among the chicks (MES 74,10) and one sample among the adults (Mes 180575) for golden samples. For each tissue type (blood = adults or feather = chicks), a serial dilution was carried out to evaluate the efficiency (E) of the qPCR reaction. For adults ES = 100.2%, r2 = 0.976 and ET = 99.3%, r2 = 0.982 and for chicks ES = 100%, r2 = 0.997 and ET = 99.7%, r2 = 0.982. The reaction conditions were: 95°C for 2 min – followed by 30 (telomere) or 40 (control) cycles of 95°C for 15 s, 56°C for 30 s, and 72°C for 90 s. All amplifications ended by a dissociation curve analysis to check for unspecific signals. Intra-run coefficients of variation for chicks were 1.07 ± 0.12% (Cq S), 1.69 ± 0.13% (Cq T), and 10.40 ± 1.00% (T/S ratio) and intra-run coefficients of variation for adults were 1.03 ± 0.1% (Cq S), 0.95 ± 0.14% (Cq T), and 7.13 ± 0.95 (T/S ratio). Following Lindrose et al. (2021), we also calculate the intra-class correlation (ICC) to assess repeatability of T/S ratio, which was of 0,785 (chicks) and 0,899 (adults). Sex determination was also done on DNA extracted, following an adapted method from Griffiths et al. (1998).
With the following enzyme immunoassay (EIA) the corticosterone concentrations of all the samples (in triplicates for 5 μl plasma, or duplicates if less than 5 μl plasma were available) were determined (Munro and Stabenfeldt, 1984; Müller et al., 2006). The sample was diluted in 195 μl of water and corticosterone was extracted from this solution with 4 ml dichloromethane and after evaporation re-dissolved with 200 μl phosphate-buffer. The anti-corticosterone antibody (Chemicon International, AB1297) was diluted with the coating buffer to the final dilution of 1:8,000. HRP (horseradish peroxidase) conjugated to corticosterone at a final dilution of 1:400,000 served for labeling the enzyme, while ABTS [2,2′-azino-bis(3-ethylbenzothiazoline-6-sulphonic acid)] was the substrate. A standard curve ran in parallel on each plate was used for calculating the corticosterone concentrations in the samples. For an internal control a chicken plasma sample with known corticosterone concentrations (was ran in duplicate on each plate). Intra-assay variation was 4.5% and inter-assay variation 5.4%. The detection (quantitation) threshold was at 0.026 ng ml–1, if a measure was under the threshold we re-measured the sample and diluted it less strongly to reach the detection limit. From the triplicate/duplicate measures the mean was computed for further analysis.
Statistical Analyses
With the aim of investigating the effect of human disturbance on adult telomere length we applied a linear mixed model (LMM) to test for the effect of experimental disturbance (treatment: disturbed vs control split-plots) on adult T/S ratio, which followed a normal distribution. We also accounted for the effect of sex (as main effect and in interaction with treatment), other stressors (i.e., presence/absence of a gravel road bordering the split-plot) and timing of reproduction (expressed as hatching date). We were not able to account for the effect of adult age, because age was not available for all adults.
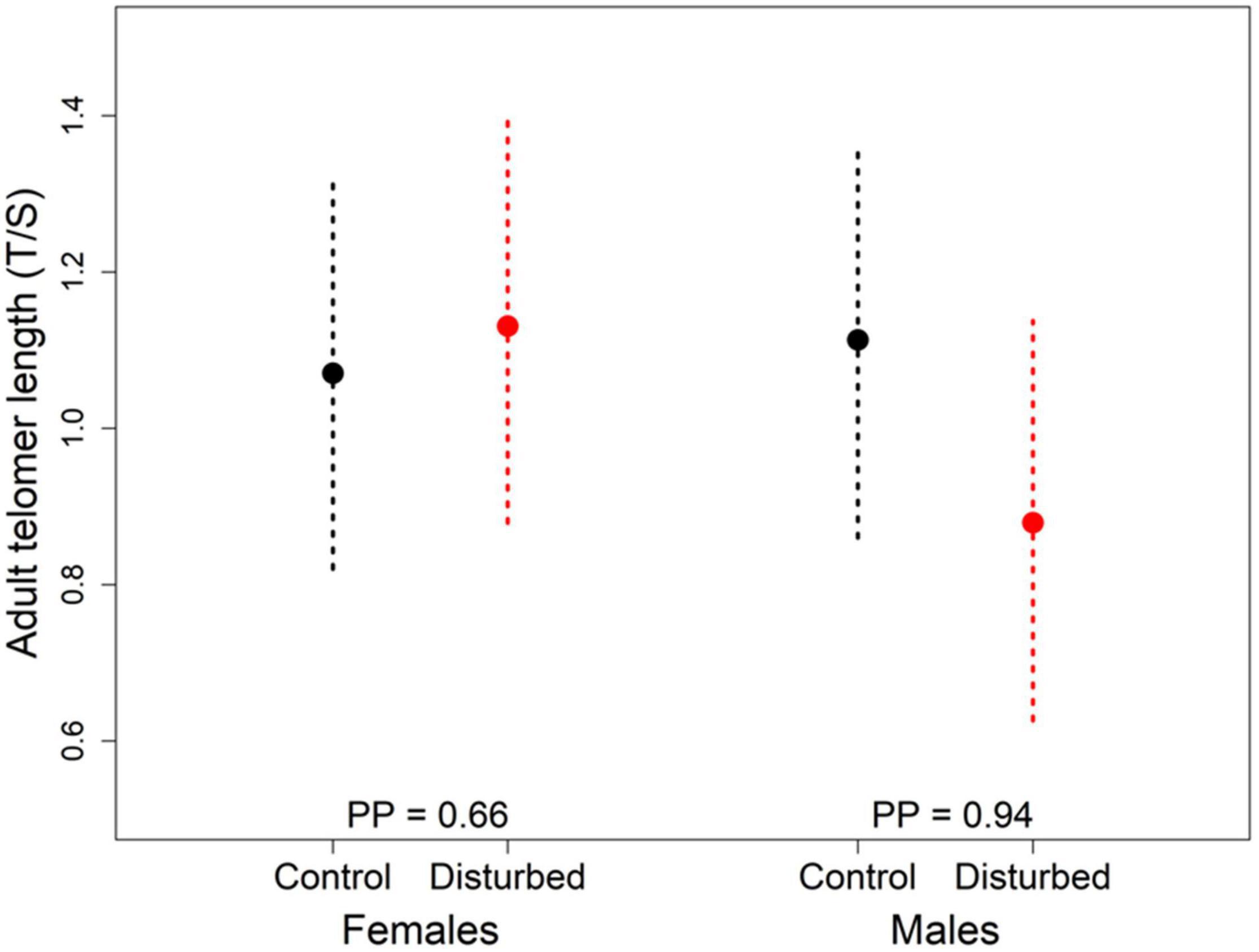
Figure 1. Effect of disturbance and sex on adult telomere length (T/S ratio). Represented are the posterior means ± 95% CrI (N = 40). Posterior probabilities (PP; from 0.5 to 1) indicate the support for a true difference between the two factor levels.
In a second step, we explored some of the potential mechanisms through which the stress caused by experimental disturbance could have influenced telomere length. For this purpose, we performed additional LMMs to examine how disturbed and control split-plots differed in number of hatchlings produced, adult body condition [calculated as the Scaled Mass Index (SMI; Peig and Green, 2009) using body mass and tarsus length], and in adults baseline levels of corticosterone (i.e., baseline corticosterone) and in the stress-induced adrenocortical response (i.e., stress corticosterone levels). As response variables for those four models we used the number of hatchlings per nest, adult body condition, baseline corticosterone (log-transformed), and stress-induced levels of corticosterone. As explanatory variables we included treatment alone and in interaction with sex, presence/absence of road, and chickage (i.e., age of the nestlings). In the model investigating the determinants of number of hatchlings, we were not able to include sex, since there is only one value per breeding pair, and chickage was replaced by hatching date. In the models referring to corticosterone levels we also accounted for sampling time (seconds after capture of birds) as linear term in the case of baseline corticosterone and both as linear and quadratic form in the case of stress-induced corticosterone to control for the point of the stress-response curve in which the samples were taken.
Finally, after investigating the impact of disturbance on adult telomere length and the potential mechanisms underlying this effect, we investigated the link between telomere length of the adults and of their nestlings. We thus performed a LMM with nestling telomere length (i.e., log-transformed nestling T/S ratio) as response variable and tested for the effect of fathers’ telomere length (father T/S ratio) and mothers’ telomere length (mother T/S ratio). As explanatory variables, we also accounted for other potentially effects such as that of number of hatchlings, nestling sex, hatching date, and nestling body condition (calculated in the same way as adult body condition).
In all aforementioned models we accounted for the potential non-independence of the data within plots and nest boxes by including plot-ID and nest-box-ID as random factors. The only exception was the model where we tested the effect of disturbance on the number of hatchlings, in which there was no need to include nest-box-ID as random term since there is only one value per nest. All analyses were done using the “lme4” package (Bates et al., 2014) in R v. 3.6.0 (R Core Team, 2019) using a Bayesian framework. Parameter estimates (and their 95% credible intervals; CrI) were calculated by simulating random samples (n = 10,000) from the joint posterior distribution of the model parameters using the function sim from the R-package arm (Gelman and Su, 2015). In order to assess the impact of the explanatory variables we calculated the posterior probability (PP) of the hypotheses that these explanatory variables had either a positive or a negative influence on the response variables, based on the posterior distributions of the model parameters. The higher the probability (from 0.5 to 1), the stronger the support for a given effect. For the categorical variables treatment, sex and road, we calculated the PP of the hypotheses that there were differences between disturbed and control treatment, between females and males, and between split-plots with or without bordering gavel road, respectively. The larger the PPs (0.5 to 1), the greater the support for the difference between categories.
Results
We found an effect of experimental disturbance on adult telomere length, although it varied according to sex. While the probability that telomeres are shorter in disturbed than in control plots is 0.94 for males, there is only a low probability (0.66) that female telomere lengths differs between treatments (Figure 1 and Table 1). Adult telomere length was neither related to the presence of a gravel road bordering the split-plot nor to hatching date (Table 1).
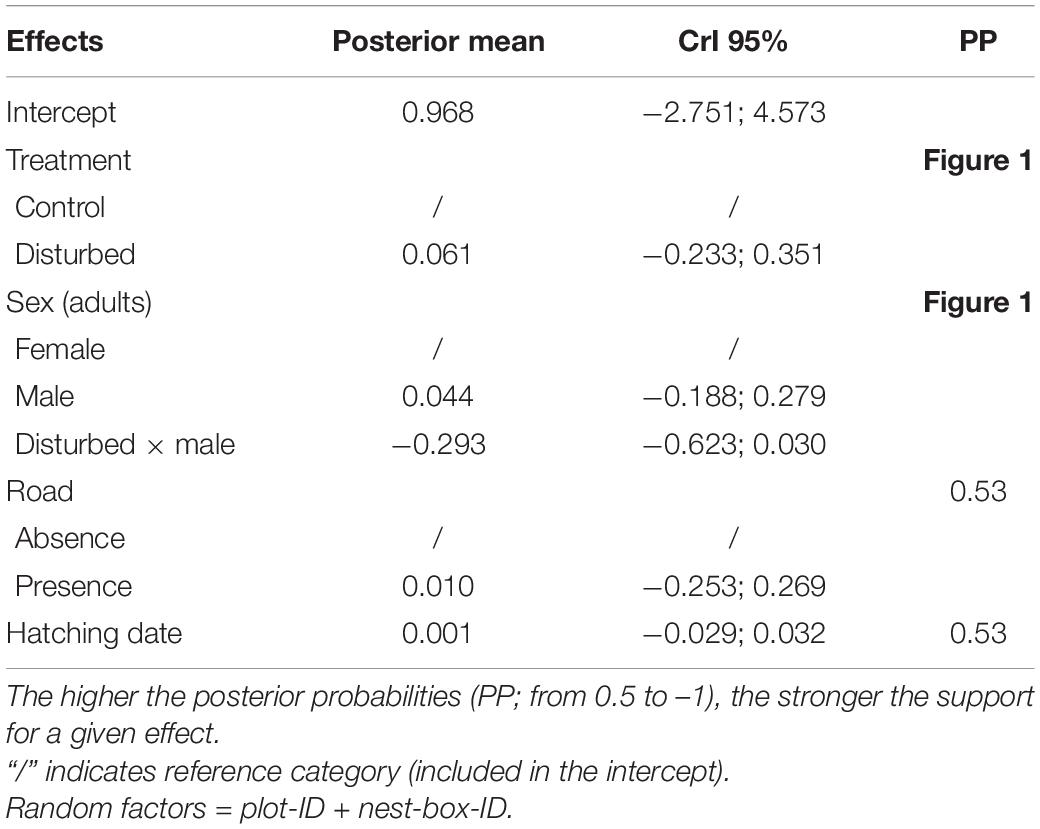
Table 1. Parameter estimates and corresponding 95% credible intervals (CrI) of the model examining the effect of experimental disturbance, sex, presence of gravel road, and hatching date on adult telomere length (N = 40).
When comparing parameters between disturbed and control split-plots that could hint on the potential underlying mechanisms through which disturbance leads to differences in telomere length, we observed that the number of hatchlings and body condition of the adults were lower in disturbed split-plots than in control split-plots, after controlling for the presence of a gravel road, and hatching date or chick age, respectively (Figures 2A,B and Table 2). We also found that baseline corticosterone was higher in males in disturbed split-plots compared to control split-plots, whereas there was no difference in females (Figure 2C and Table 2). On the other hand, there was no association between disturbance and stress-induced corticosterone levels in either sex (Figure 2D and Table 2).
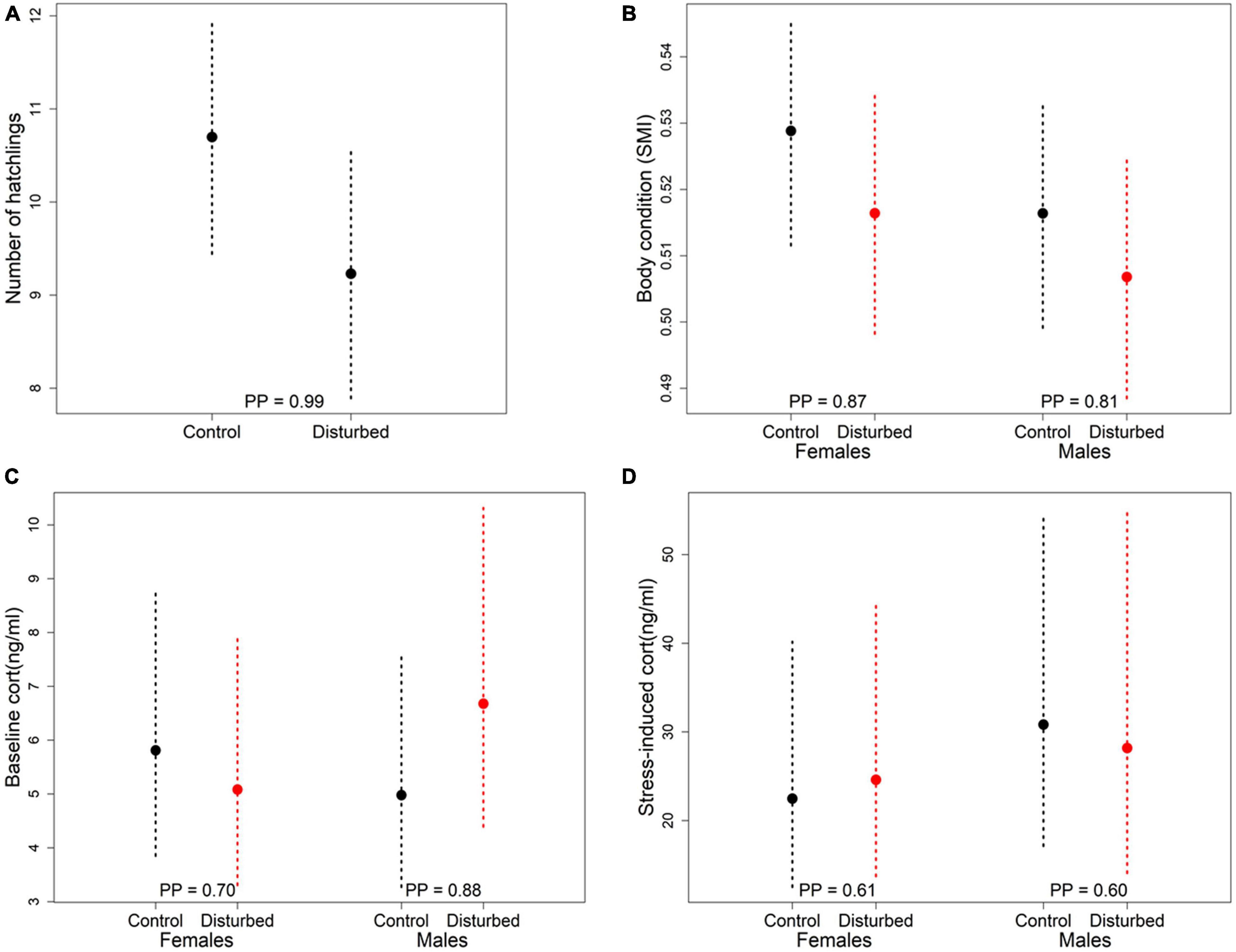
Figure 2. Comparison of number of hatchlings (A), adult body condition (B), adult baseline corticosterone (C), and stress-induced corticosterone (D) between split-plots with or without experimental disturbance. Values correspond to posterior means ± 95% CrI (N = 40, except for number of hatchlings, where N = 20). Posterior probabilities (PP; from 0.5 to 1) represent the likelihood that the differences between factor levels are true.
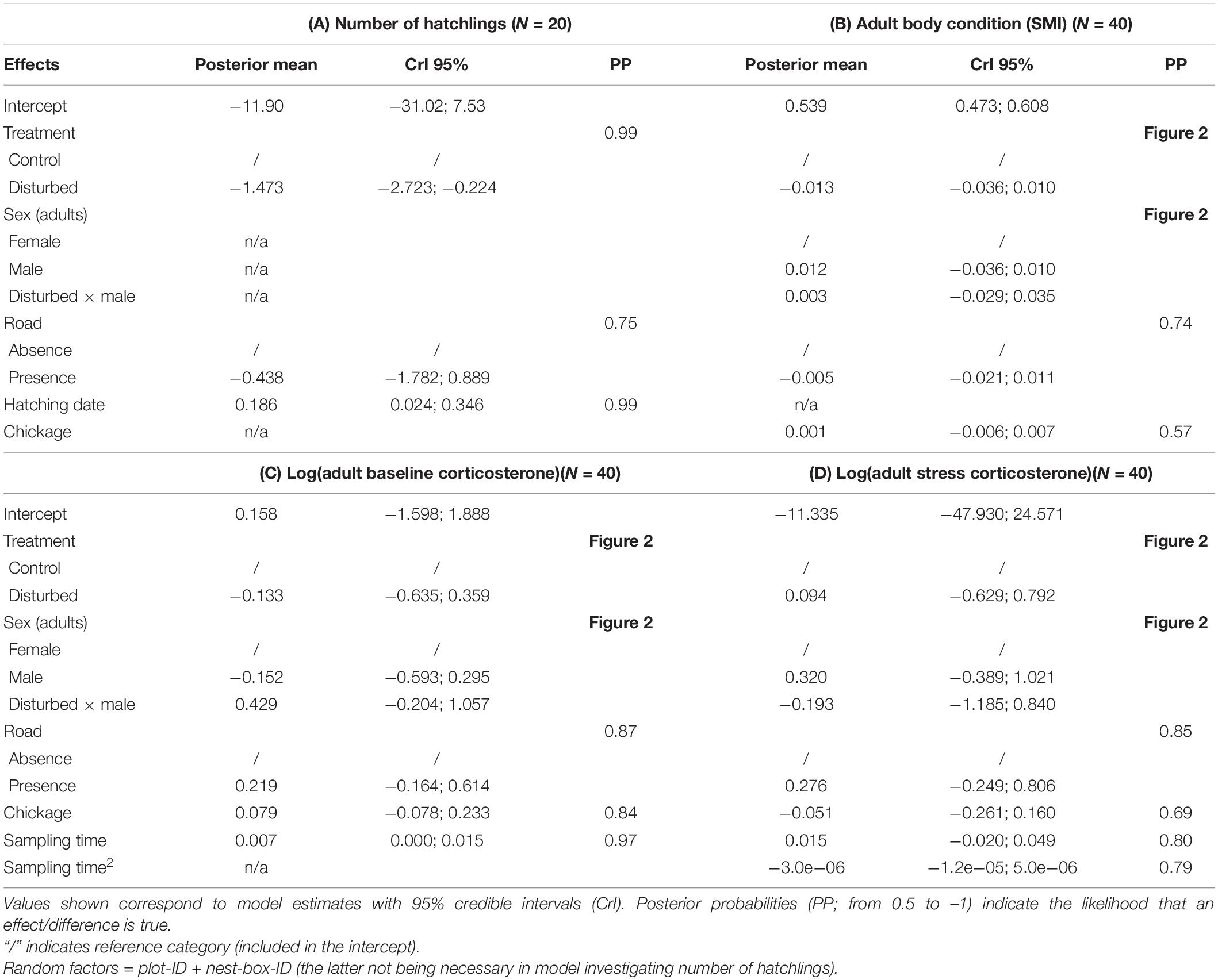
Table 2. Factors related to number of hatchlings (A), adult body condition (B), adult baseline corticosterone (C), and adult stress-induced corticosterone (D).
Finally, the exploration of the correlation between parental telomere length and nestling telomere length, revealed that nestling telomere length was positively related to father telomere length, whereas there was a weaker support for a correlation between nestling telomere length and mother telomere length (Figures 3A,B and Table 3). There seems to be also a relationship between chick telomere length and number of hatchlings and hatching date. Chick telomere length decreased with increasing number of siblings (Figure 3C and Table 3) and was longer at later hatching dates (Figure 3D and Table 3).
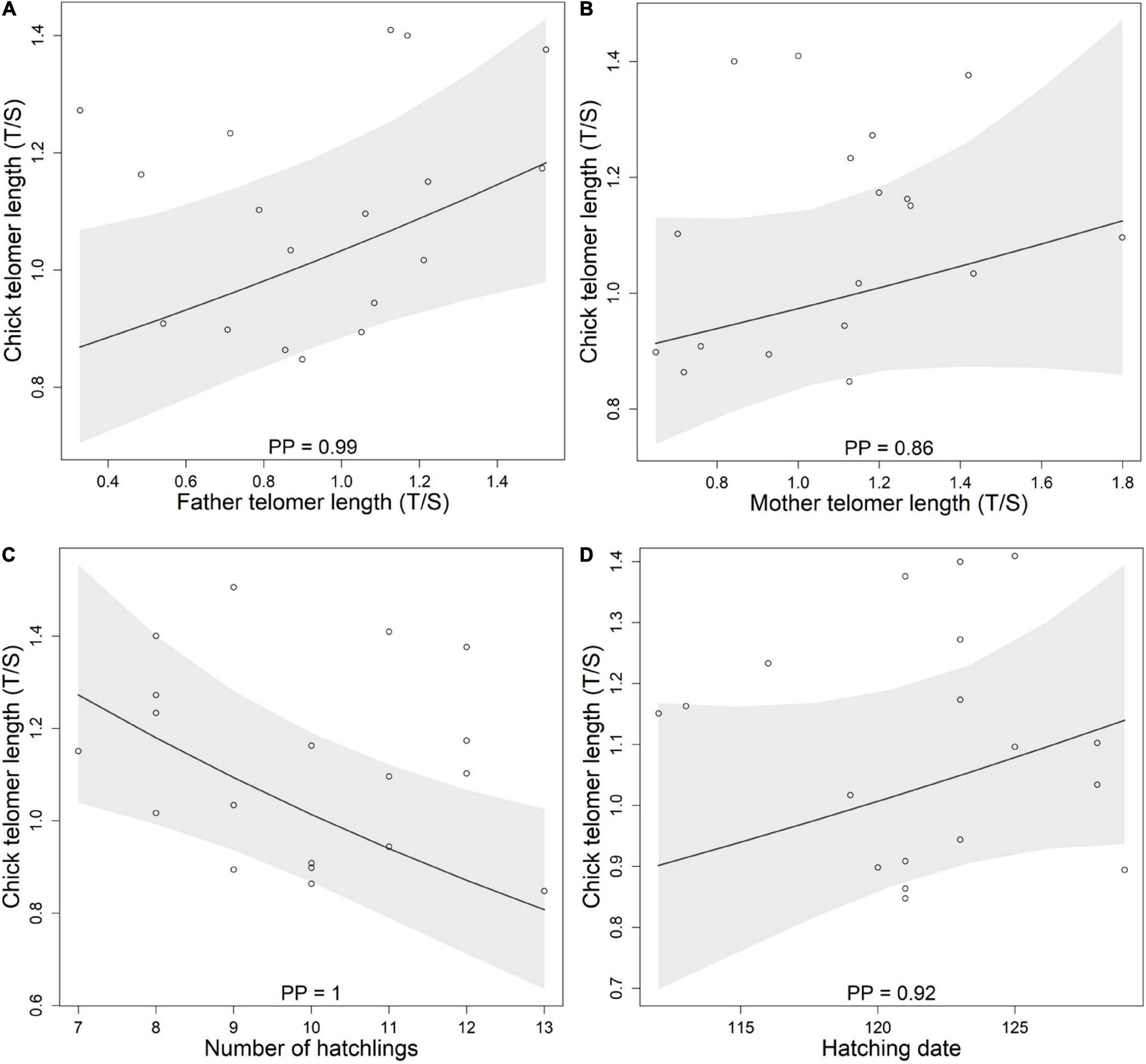
Figure 3. Nestling telomere length (T/S) in relation to father (A) and mother (B) telomere length (T/S), to number of hatchlings (C), and to hatching date (D). Represented are posterior means ± 95% CrI (N = 116). Dots refer to the raw data averaged by nest. Posterior probabilities (PP; from 0.5 to 1) indicate the likelihood that the relationships observed are real.
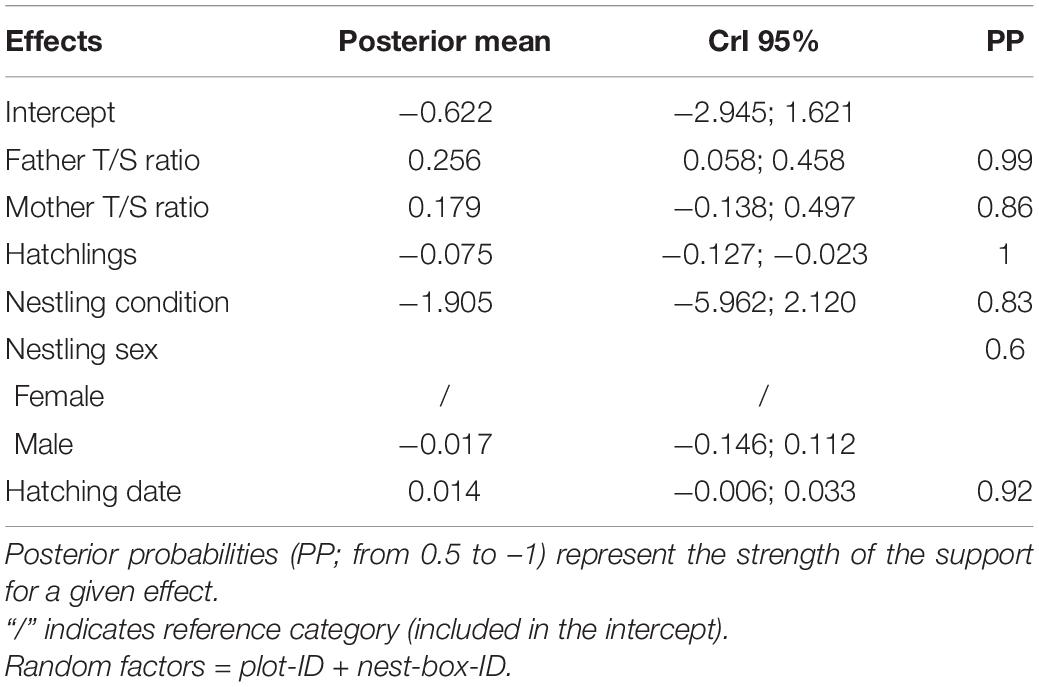
Table 3. Model estimates and corresponding 95% credible intervals (CrI) of the model investigating the relationship between father and mother telomere length and nestling telomere length, while accounting for other covariates (N = 116).
Discussion
Our findings suggest that human presence can indeed lead to shorter telomere lengths in wildlife. We found that adult male great tits exposed to an experimental human disturbance (i.e., mimicking hikers) tended to have shorter telomere length than adult males in control split-plots, although a similar effect for females was not detectable. This coincides with previous studies showing how stressors, whether natural (predators and food scarcity) or anthropogenic (noise, light pollution, and proximity of roads) promote telomere attrition, thus resulting in telomere length reductions (Salmón et al., 2016; Injaian et al., 2019; Chatelain et al., 2020; Grunst A. et al., 2020; Grunst M.L. et al., 2020; Caccavo et al., 2021).
The mechanisms through which our experimental disturbance led to shorter telomere lengths could be several, entailing both direct and indirect effects, which are not mutually exclusive. The mere presence of humans, which wild animals often perceive as predators (Frid and Dill, 2002), may lead to increases in circulating corticosterone (Müller et al., 2006; Strasser and Heath, 2013; Soldatini et al., 2015), which is directly linked to increased oxidative stress (both through exacerbation of free radical production and decrease of enzymatic antioxidant capacity) and to reduced telomerase activity (Liu and Mori, 1999; Choi et al., 2008; Costantini et al., 2008; Haussmann and Marchetto, 2010). All of this leads, therefore, to higher telomere attrition and shorter telomere lengths (Casagrande et al., 2020; Chatelain et al., 2020). This is supported by the higher levels of baseline corticosterone we found in males in disturbed split-plots compared to males in control sites. Along the same line, the repeated exposure to human disturbance may be the cause of the lower body condition shown by adult great tits in the disturbed split-plots; body mass/condition has also been previously associated to decreased telomere lengths (Barrett et al., 2013; Rollings et al., 2017).
Indirect effects of disturbance could also play a role in determining the telomere length patterns. Given that the experimental disturbance took place early in the breeding season (i.e., during territory settlement and egg-laying), our treatment could have had an influence in the quality of the males occupying disturbed vs undisturbed split-plots. If disturbed split-plots had been occupied mainly by low quality individuals and/or less experienced great tits, which could not compete with higher quality adults for the undisturbed sites, this would result in a non-random territory settlement, which could also, at least partially, explain the lower body condition, higher baseline levels and shorter telomere lengths found in males from disturbed split-plots. This explanation is supported by previous studies that have shown that even low-intensity human disturbance may affect bird territory settlement (Bötsch et al., 2017, 2018b).
We found that disturbed split-plots were occupied more often by first-year breeders (birds in their second calendar year) than older birds (15 vs 2; that is, 8 females and 7 males vs 1 female and 1 male older breeders; plus 3 of undetermined age). In contrast, undisturbed split-plots were occupied equally by the two age classes: 7 vs 7 (i.e., 5 female and 2 male first-year breeders vs 3 female and 4 male older breeders; plus 6 individuals of undetermined age). Because telomere length generally decreases with age (Hall et al., 2004; Monaghan and Haussmann, 2006), the shorter telomere length of males in disturbed split-plots cannot readily be explained by the higher proportion of first-breeders.
Our results also show a lower number of hatchlings per nest in disturbed than in control split-plots. This could again be explained by both direct and indirect effects. Our experimental disturbance, which lasted until the egg-laying period could have resulted in stressed mothers laying lower-quality eggs and in interruptions during incubation, both negatively associated to number of hatchlings (Blomqvist et al., 1997; Krist, 2011). Indirect effects would involve disturbance leading to settlement of lower quality adults in the disturbed split-plots, which produce less or lower quality eggs, which in turn results also in a lower number of hatchlings (Coulson and Porter, 1985; Kärkkäinen et al., 2019). Independently of the mechanism through which human disturbance might have led to lower number of hatchlings, the smaller brood sizes in the disturbed split-plots, which may translate into lower parental effort and stress during the chick-rearing phase (Smith et al., 1988; Bonier et al., 2011), might have helped buffering the negative effects of the disturbance itself in adult females. This could at least partially explain why the effect of disturbance on telomere length is higher in males than females. Another complementary explanation for this sex-specific response of telomere length to disturbance would be the fact that females have higher amounts of antioxidants, especially during the breeding period, and therefore can be more resistant than males to oxidative damage and telomere shortening, as has been shown in some birds and mammals (López-Arrabé et al., 2018; Viblanc et al., 2018).
Additionally, we observed that variations in telomere length of adult great tits were reflected in the next generation as we found a positive correlation between telomere length of the chicks and their fathers. This agrees with recent studies showing that telomere length is highly heritable in wild bird populations (Vedder et al., 2021). Interestingly, we did not find enough support for a correlation between telomere length of chicks and mothers, which agrees with some previous research suggesting that telomere length of nestlings might be paternally inherited [Criscuolo et al., 2017; Bouwhuis et al., 2018; although see for example, Horn et al. (2011) and Asghar et al. (2015)]. Cross-fostering experiments would be necessary to separate the genetic, epigenetic and environmental components of the correlation between chick’s and parents’ telomere length (Soler et al., 2003; McCarty, 2017).
Finally, telomere length of the chicks was negatively correlated with brood size (i.e., number of hatchlings), probably reflecting the negative influence that competition with nest-mates over resources has on nestlings’ oxidative stress and telomere length (Boonekamp et al., 2014; Reichert et al., 2015; Costanzo et al., 2016). Telomere length of chicks also correlated with hatching date, with nestlings hatched from later broods having longer telomere lengths, when compared to earlier hatching broods. This is likely a consequence of earlier broods overlapping more with the experimental disturbance, which ended on the 22nd of April, than later broods (i.e., hatching dates ranging from the 22nd of April until the 9th of May).
Conclusion
In conclusion, even though our study does not allow disentangling direct from indirect effects of disturbance, it still highlights how even low levels of disturbance, such as small groups of hikers, may have an impact on telomere length of wild animal populations and their next generations. Moreover, given the association between telomere length and lifespan and survival (Heidinger et al., 2012; Tricola et al., 2017; Wilbourn et al., 2018; Vedder et al., 2021), disturbance-driven changes in telomere length could thus have important long-term consequences at the population level (Dupoué et al., 2017). Therefore, management measures such as restrictions guiding outdoor recreational activities and the creation of protected core areas without human access are essential for the long-term conservation of biodiversity.
Data Availability Statement
The original contributions presented in the study are publicly available. This data can be found here: doi: 10.5281/zenodo.5760347.
Ethics Statement
The animal study was reviewed and approved by C.R.B.P.O. (permit number 2014157-0012 of the Direction Régionale de l’Environnement, de l’Aménagement et du Logement de Franche-Comté and permit number 15006 for 2014–2016 from the C.R.B.P.O).
Author Contributions
ZT participated in the study design, analyzed the data, and wrote the manuscript. YB helped with designing the study, collecting the data, laboratory work, and wrote the manuscript. TP participated in the study design and worked on manuscript drafts. SM and SZ did the laboratory telomere analyses and helped with revision and writing of the manuscripts. SJ-E introduced the corticosterone assays and worked on the manuscript drafts. LJ helped with designing and manuscript writing. All authors contributed to manuscript revision, read, and approved the submitted version.
Conflict of Interest
The authors declare that the research was conducted in the absence of any commercial or financial relationships that could be construed as a potential conflict of interest.
Publisher’s Note
All claims expressed in this article are solely those of the authors and do not necessarily represent those of their affiliated organizations, or those of the publisher, the editors and the reviewers. Any product that may be evaluated in this article, or claim that may be made by its manufacturer, is not guaranteed or endorsed by the publisher.
Acknowledgments
First of all we thank all the collaborators in the field which did the hard disturbance-work in the forest (in alphabetical order): C. Arnace, M. Crespo Ballester, B. Garde, L. Gillespie, H. Lemke, F. Leugger, N. Mortier, J. Nouri, M. Quetstroey, A. Rouméas, J. Vasseur. We thank M. Romanski and J. L. Dessolin from the Office National des Forêts (ONF), as well as B. Dorbani from the DREAL (Direction Régionale de l’Environnement, de l’Aménagement et du Logement de Franche-Comté) for allowing us to do this study in the Forêt domaniale de Chaux. We also thank B. Almasi and J. Olano-Marin for the support in the laboratory in Sempach. For the telomere analyses, we were financially supported by the Observatoire des Sciences de l’Univers Terre Homme Environnement Temps Astronomie (OSU THETA Bourgogne-Franche Comté).
References
Angelier, F., Costantini, D., Blévin, P., and Chastel, O. (2018). Do glucocorticoids mediate the link between environmental conditions and telomere dynamics in wild vertebrates? A review. Gen. Comp. Endocrinol. 256, 99–111. doi: 10.1016/j.ygcen.2017.07.007
Asghar, M., Bensch, S., Tarka, M., Hansson, B., and Hasselquist, D. (2015). Maternal and genetic factors determine early life telomere length. Proc. R. Soc. B Biol. Sci. 282:20142263. doi: 10.1098/rspb.2014.2263
Balmford, A., Beresford, J., Green, J., Naidoo, R., Walpole, M., and Manica, A. (2009). A global perspective on trends in nature-based tourism. PLoS Biol. 7:e1000144. doi: 10.1371/journal.pbio.1000144
Barrett, E. L., Burke, T. A., Hammers, M., Komdeur, J., and Richardson, D. S. (2013). Telomere length and dynamics predict mortality in a wild longitudinal study. Mol. Ecol. 22, 249–259. doi: 10.1111/mec.12110
Bates, D., Mächler, M., Bolker, B., and Walker, S. (2014). Fitting linear mixed-effects models using lme4. J. Stat. Softw. 67, 1–48.
Bateson, M. (2016). Cumulative stress in research animals: telomere attrition as a biomarker in a welfare context? BioEssays 38, 201–212. doi: 10.1002/bies.201500127
Bertin, A., Richard-Yris, M.-A., Houdelier, C., Lumineau, S., Möstl, E., Kuchar, A., et al. (2008). Habituation to humans affects yolk steroid levels and offspring phenotype in quail. Horm. Behav. 54, 396–402. doi: 10.1016/j.yhbeh.2008.04.012
Blomqvist, D., Johansson, O. C., and Götmark, F. (1997). Parental quality and egg size affect chick survival in a precocial bird, the lapwing Vanellus vanellus. Oecologia 110, 18–24. doi: 10.1007/s004420050128
Blumstein, D. T. (2006). Developing an evolutionary ecology of fear: how life history and natural history traits affect disturbance tolerance in birds. Anim. Behav. 71, 389–399. doi: 10.1016/j.anbehav.2005.05.010
Bonier, F. (2012). Hormones in the city: endocrine ecology of urban birds. Horm. Behav. 61, 763–772. doi: 10.1016/j.yhbeh.2012.03.016
Bonier, F., Moore, I. T., and Robertson, R. J. (2011). The stress of parenthood? Increased glucocorticoids in birds with experimentally enlarged broods. Biol. Lett. 7, 944–946. doi: 10.1098/rsbl.2011.0391
Boonekamp, J. J., Mulder, G., Salomons, H. M., Dijkstra, C., and Verhulst, S. (2014). Nestling telomere shortening, but not telomere length, reflects developmental stress and predicts survival in wild birds. Proc. R. Soc. B Biol. Sci. 281, 20133287. doi: 10.1098/rspb.2013.3287
Boonekamp, J. J., Simons, M. J., Hemerik, L., and Verhulst, S. (2013). Telomere length behaves as biomarker of somatic redundancy rather than biological age. Aging Cell 12, 330–332. doi: 10.1111/acel.12050
Bötsch, Y., Gugelmann, S., Tablado, Z., and Jenni, L. (2018a). Effect of human recreation on bird anti-predatory response. PeerJ 6:e5093. doi: 10.7717/peerj.5093
Bötsch, Y., Tablado, Z., Scherl, D., Kéry, M., Graf, R. F., and Jenni, L. (2018b). Effect of recreational trails on forest birds: human presence matters. Front. Ecol. Evol. 6:175. doi: 10.3389/fevo.2018.00175
Bötsch, Y., Tablado, Z., Almasi, B., and Jenni, L. (2020). Human recreation decreases antibody titre in bird nestlings: an overlooked transgenerational effect of disturbance. J. Exp. Biol. 223:jeb210930. doi: 10.1242/jeb.210930
Bötsch, Y., Tablado, Z., and Jenni, L. (2017). Experimental evidence of human recreational disturbance effects on bird-territory establishment. Proc. R. Soc. B Biol. Sci. 284:20170846. doi: 10.1098/rspb.2017.0846
Bouwhuis, S., Verhulst, S., Bauch, C., and Vedder, O. (2018). Reduced telomere length in offspring of old fathers in a long-lived seabird. Biol. Lett. 14:20180213. doi: 10.1098/rsbl.2018.0213
Byrne, D., Dillon, H., Tran, K., Arlinger, S., Wilbraham, K., Cox, R., et al. (1994). An international comparison of long-term average speech spectra. J. Acoust. Soc. Am. 96, 2108–2120. doi: 10.1121/1.410152
Caccavo, J. A., Raclot, T., Poupart, T., Ropert-Coudert, Y., and Angelier, F. (2021). Anthropogenic activities are associated with shorter telomeres in chicks of Adélie penguin (Pygoscelis adeliae). Polar Biol. 44, 1391–1399.
Carney, K. M., and Sydeman, W. J. (1999). A review of human disturbance effects on nesting colonial waterbirds. Waterbirds Int. J. Waterbird Biol. 22, 68–79. doi: 10.2307/1521995
Casagrande, S., Stier, A., Monaghan, P., Loveland, J. L., Boner, W., Lupi, S., et al. (2020). Increased glucocorticoid concentrations in early life cause mitochondrial inefficiency and short telomeres. J. Exp. Biol. 223:jeb222513. doi: 10.1242/jeb.222513
Chatelain, M., Drobniak, S. M., and Szulkin, M. (2020). The association between stressors and telomeres in non-human vertebrates: a meta-analysis. Ecol. Lett. 23, 381–398. doi: 10.1111/ele.13426
Choi, J., Fauce, S. R., and Effros, R. B. (2008). Reduced telomerase activity in human T lymphocytes exposed to cortisol. Brain Behav. Immun. 22, 600–605. doi: 10.1016/j.bbi.2007.12.004
Coimbra, B. M., Carvalho, C. M., Moretti, P. N., Mello, M. F., and Belangero, S. I. (2017). Stress-related telomere length in children: a systematic review. J. Psychiatr. Res. 92, 47–54. doi: 10.1016/j.jpsychires.2017.03.023
R Core Team (2019). R: A Language and Environment for Statistical Computing. Vienna: R Foundation for Statistical Computing.
Costantini, D., Fanfani, A., and Dell’Omo, G. (2008). Effects of corticosteroids on oxidative damage and circulating carotenoids in captive adult kestrels (Falco tinnunculus). J. Comp. Physiol. B 178, 829–835. doi: 10.1007/s00360-008-0270-z
Costanzo, A., Parolini, M., Bazzi, G., Khoriauli, L., Santagostino, M., Possenti, C. D., et al. (2016). Brood size, telomere length, and parent-offspring color signaling in barn swallows. Behav. Ecol. 28, 204–211.
Coulson, J., and Porter, J. (1985). Reproductive success of the kittiwake Rissa tridactyla: the roles of clutch size, chick growth rates and parental quality. Ibis 127, 450–466.
Criscuolo, F., Bize, P., Nasir, L., Metcalfe, N. B., Foote, C. G., Griffiths, K., et al. (2009). Real-time quantitative PCR assay for measurement of avian telomeres. J. Avian Biol. 40, 342–347.
Criscuolo, F., Zahn, S., and Bize, P. (2017). Offspring telomere length in the long lived Alpine swift is negatively related to the age of their biological father and foster mother. Biol. Lett. 13:20170188. doi: 10.1098/rsbl.2017.0188
Doherty, T. S., Hays, G. C., and Driscoll, D. A. (2021). Human disturbance causes widespread disruption of animal movement. Nat. Ecol. Evol. 5, 513–519. doi: 10.1038/s41559-020-01380-1
Dupoué, A., Rutschmann, A., Le Galliard, J. F., Clobert, J., Angelier, F., Marciau, C., et al. (2017). Shorter telomeres precede population extinction in wild lizards. Sci. Rep. 7:16976. doi: 10.1038/s41598-017-17323-z
Ellenberg, U., Mattern, T., Seddon, P. J., and Jorquera, G. L. (2006). Physiological and reproductive consequences of human disturbance in Humboldt penguins: the need for species-specific visitor management. Biol. Conserv. 133, 95–106.
French, S. S., DeNardo, D. F., Greives, T. J., Strand, C. R., and Demas, G. E. (2010). Human disturbance alters endocrine and immune responses in the Galapagos marine iguana (Amblyrhynchus cristatus). Horm. Behav. 58, 792–799. doi: 10.1016/j.yhbeh.2010.08.001
Frid, A., and Dill, L. M. (2002). Human-Caused disturbance stimuli as a form of predation risk. Conserv. Ecol. 6:11.
Gelman, A., and Su, Y.-S. (2020). Arm: Data Analysis Using Regression and Multilevel/Hierarchical Models. R package version 1.11-2. Available online at: https://CRAN.R-project.org/package=arm
Gibson, D., Chaplin, M. K., Hunt, K. L., Friedrich, M. J., Weithman, C. E., Addison, L. M., et al. (2018). Impacts of anthropogenic disturbance on body condition, survival, and site fidelity of nonbreeding Piping Plovers. Condor Ornithol. Appl. 120, 566–580.
Griffiths, R., Double, M. C., Orr, K., and Dawson, R. J. (1998). A DNA test to sex most birds. Mol. Ecol. 7, 1071–1075. doi: 10.1046/j.1365-294x.1998.00389.x
Grunst, A., Grunst, M., Bervoets, L., Pinxten, R., and Eens, M. (2020). Proximity to roads, but not exposure to metal pollution, is associated with accelerated developmental telomere shortening in nestling great tits. Environ. Pollut. 256:113373. doi: 10.1016/j.envpol.2019.113373
Grunst, M. L., Grunst, A. S., Pinxten, R., and Eens, M. (2020). Anthropogenic noise is associated with telomere length and carotenoid-based coloration in free-living nestling songbirds. Environ. Pollut. 260:114032. doi: 10.1016/j.envpol.2020.114032
Hacki, T. (1996). Comparative speaking, shouting and singing voice range profile measurement: physiological and pathological aspects. Logoped. Phoniatr. Vocol. 21, 123–129. doi: 10.3109/14015439609098879
Hall, M. E., Nasir, L., Daunt, F., Gault, E. A., Croxall, J. P., Wanless, S., et al. (2004). Telomere loss in relation to age and early environment in long-lived birds. Proc. R. Soc. Lond. B Biol. Sci. 271, 1571–1576. doi: 10.1098/rspb.2004.2768
Hammitt, W. E., Cole, D. N., and Monz, C. A. (2015). Wildland Recreation: Ecology and Management. Hoboken, NJ: John Wiley & Sons.
Haussmann, M. F., and Marchetto, N. M. (2010). Telomeres: linking stress and survival, ecology and evolution. Curr. Zool. 56, 714–727. doi: 10.1093/czoolo/56.6.714
Heidinger, B. J., Blount, J. D., Boner, W., Griffiths, K., Metcalfe, N. B., and Monaghan, P. (2012). Telomere length in early life predicts lifespan. Proc. Natl. Acad. Sci. U.S.A. 109, 1743–1748. doi: 10.1073/pnas.1113306109
Horn, T., Robertson, B. C., Will, M., Eason, D. K., Elliott, G. P., and Gemmell, N. J. (2011). Inheritance of telomere length in a bird. PLoS One 6:e17199. doi: 10.1371/journal.pone.0017199
Injaian, A. S., Gonzalez-Gomez, P. L., Taff, C. C., Bird, A. K., Ziur, A. D., Patricelli, G. L., et al. (2019). Traffic noise exposure alters nestling physiology and telomere attrition through direct, but not maternal, effects in a free-living bird. Gen. Comp. Endocrinol. 276, 14–21. doi: 10.1016/j.ygcen.2019.02.017
Kärkkäinen, T., Bize, P., and Stier, A. (2020). Correlation in telomere lengths between feathers and blood cells in pied flycatchers. J. Avian Biol. 51:e02300.
Kärkkäinen, T., Teerikorpi, P., Panda, B., Helle, S., Stier, A., and Laaksonen, T. (2019). Impact of continuous predator threat on telomere dynamics in parent and nestling pied flycatchers. Oecologia 191, 757–766. doi: 10.1007/s00442-019-04529-3
Kratschmar, D. V., Calabrese, D., Walsh, J., Lister, A., Birk, J., Appenzeller-Herzog, C., et al. (2012). Suppression of the Nrf2-dependent antioxidant response by glucocorticoids and 11β-HSD1-mediated glucocorticoid activation in hepatic cells. PLoS One 7:e36774. doi: 10.1371/journal.pone.0036774
Krebs, J. R. (1971). Territory and breeding density in the Great Tit. Parus major L. Ecology 52, 2–22.
Krist, M. (2011). Egg size and offspring quality: a meta-analysis in birds. Biol. Rev. 86, 692–716. doi: 10.1111/j.1469-185X.2010.00166.x
Linden, M., Gustafsson, L., and Part, T. (1992). Selection on fledging mass in the collared flycatcher and the great tit. Ecology 73, 336–343.
Lindrose, A. R., McLester-Davis, L. W., Tristano, R. I., Kataria, L., Gadalla, S. M., Eisenberg, D. T., et al. (2021). Method comparison studies of telomere length measurement using qPCR approaches: a critical appraisal of the literature. PLoS One 16:e0245582. doi: 10.1371/journal.pone.0245582
Liu, J., and Mori, A. (1999). Stress, aging, and brain oxidative damage. Neurochem. Res. 24, 1479–1497.
López-Arrabé, J., Monaghan, P., Cantarero, A., Boner, W., Pérez-Rodríguez, L., and Moreno, J. (2018). Sex-specific associations between telomere dynamics and oxidative status in adult and nestling pied flycatchers. Physiol. Biochem. Zool. 91, 868–877. doi: 10.1086/697294
López-Roig, M., and Serra-Cobo, J. (2014). Impact of human disturbance, density, and environmental conditions on the survival probabilities of pipistrelle bat (Pipistrellus pipistrellus). Popul. Ecol. 56, 471–480. doi: 10.1007/s10144-014-0437-2
McCarty, R. (2017). Cross-fostering: elucidating the effects of gene× environment interactions on phenotypic development. Neurosci. Biobehav. Rev. 73, 219–254. doi: 10.1016/j.neubiorev.2016.12.025
Meillère, A., Brischoux, F., Ribout, C., and Angelier, F. (2015). Traffic noise exposure affects telomere length in nestling house sparrows. Biol. Lett. 11:20150559. doi: 10.1098/rsbl.2015.0559
Møller, A. P. (2010). Interspecific variation in fear responses predicts urbanization in birds. Behav. Ecol. 21, 365–371.
Monaghan, P. (2014). Organismal stress, telomeres and life histories. J. Exp. Biol. 217, 57–66. doi: 10.1242/jeb.090043
Monaghan, P., and Haussmann, M. F. (2006). Do telomere dynamics link lifestyle and lifespan? Trends Ecol. Evol. 21, 47–53. doi: 10.1016/j.tree.2005.11.007
Muehlenbein, M. P., Ancrenaz, M., Sakong, R., Ambu, L., Prall, S., Fuller, G., et al. (2012). Ape conservation physiology: fecal glucocorticoid responses in wild Pongo pygmaeus morio following human visitation. PLoS One 7:e33357. doi: 10.1371/journal.pone.0033357
Müller, C., Jenni-Eiermann, S., Blondel, J., Perret, P., Caro, S. P., Lambrechts, M., et al. (2006). Effect of human presence and handling on circulating corticosterone levels in breeding blue tits (Parus caeruleus). Gen. Comp. Endocrinol. 148, 163–171. doi: 10.1016/j.ygcen.2006.02.012
Munro, C. J., and Stabenfeldt, G. (1984). Development of a microtitre plate enzyme immunoassay for the determination of progesterone. J. Endocrinol. 101, 41–49. doi: 10.1677/joe.0.1010041
Oddie, K. R. (2000). Size matters: competition between male and female great tit offspring. J. Anim. Ecol. 69, 903–912. doi: 10.1046/j.1365-2656.2000.00438.x
Oliveira, B. S., Zunzunegui, M. V., Quinlan, J., Fahmi, H., Tu, M. T., and Guerra, R. O. (2016). Systematic review of the association between chronic social stress and telomere length: a life course perspective. Ageing Res. Rev. 26, 37–52. doi: 10.1016/j.arr.2015.12.006
Olovnikov, A. M. (1973). A theory of marginotomy: the incomplete copying of template margin in enzymic synthesis of polynucleotides and biological significance of the phenomenon. J. Theor. Biol. 41, 181–190. doi: 10.1016/0022-5193(73)90198-7
Peig, J., and Green, A. J. (2009). New perspectives for estimating body condition from mass/length data: the scaled mass index as an alternative method. Oikos 118, 1883–1891. doi: 10.1111/j.1600-0706.2009.17643.x
Pfaffl, M. W. (2001). A new mathematical model for relative quantification in real-time RT–PCR. Nucleic Acids Res. 29:e45. doi: 10.1093/nar/29.9.e45
Powolny, T., Bassin, N., Crini, N., Fourel, I., Morin, C., Pottinger, T., et al. (2020). Corticosterone mediates telomere length in raptor chicks exposed to chemical mixture. Sci. Total Environ. 706:135083. doi: 10.1016/j.scitotenv.2019.135083
Reichert, S., Criscuolo, F., Zahn, S., Arrivé, M., Bize, P., and Massemin, S. (2015). Immediate and delayed effects of growth conditions on ageing parameters in nestling zebra finches. J. Exp. Biol. 218, 491–499. doi: 10.1242/jeb.109942
Rentscher, K. E., Carroll, J. E., and Mitchell, C. (2020). Psychosocial stressors and telomere length: a current review of the science. Annu. Rev. Public Health 41, 223–245. doi: 10.1146/annurev-publhealth-040119-094239
Rollings, N., Uhrig, E. J., Krohmer, R. W., Waye, H. L., Mason, R. T., Olsson, M., et al. (2017). Age-related sex differences in body condition and telomere dynamics of red-sided garter snakes. Proc. R. Soc. B Biol. Sci. 284:20162146. doi: 10.1098/rspb.2016.2146
Romero, L. M., and Romero, R. C. (2002). Corticosterone responses in wild birds: the importance of rapid initial sampling. Condor 104, 129–135. doi: 10.1650/0010-5422(2002)104[0129:criwbt]2.0.co;2
Ruhlen, T. D., Abbott, S., Stenzel, L. E., and Page, G. W. (2003). Evidence that human disturbance reduces Snowy Plover chick survival. J. Field Ornithol. 74, 300–304. doi: 10.1648/0273-8570-74.3.300
Salmón, P., Nilsson, J. F., Nord, A., Bensch, S., and Isaksson, C. (2016). Urban environment shortens telomere length in nestling great tits, Parus major. Biol. Lett. 12:20160155. doi: 10.1098/rsbl.2016.0155
Sapolsky, R. M., Romero, L. M., and Munck, A. U. (2000). How do glucocorticoids influence stress responses? Integrating permissive, suppressive, stimulatory, and preparative actions. Endocr. Rev. 21, 55–89. doi: 10.1210/edrv.21.1.0389
Smith, H. G., Källander, H., Fontell, K., and Ljungström, M. (1988). Feeding frequency and parental division of labour in the double-brooded great tit Parus major. Behav. Ecol. Sociobiol. 22, 447–453. doi: 10.1007/BF00294983
Soldatini, C., Albores-Barajas, Y. V., Tagliavia, M., Massa, B., Fusani, L., and Canoine, V. (2015). Effects of human disturbance on cave-nesting seabirds: the case of the storm petrel. Conserv. Physiol. 3:cov041. doi: 10.1093/conphys/cov041
Soler, J. J., Moreno, J., and Potti, J. (2003). Environmental, genetic and maternal components of immunocompetence of nestling pied flycatchers from a cross-fostering study. Evol. Ecol. Res. 5, 259–272.
Stankowich, T., and Blumstein, D. T. (2005). Fear in animals: a meta-analysis and review of risk assessment. Proc. R. Soc. B Biol. Sci. 272, 2627–2634. doi: 10.1098/rspb.2005.3251
Stewart, S. A., Ben-Porath, I., Carey, V. J., O’Connor, B. F., Hahn, W. C., and Weinberg, R. A. (2003). Erosion of the telomeric single-strand overhang at replicative senescence. Nat. Genet. 33, 492–496. doi: 10.1038/ng1127
Strasser, E. H., and Heath, J. A. (2013). Reproductive failure of a human-tolerant species, the A merican kestrel, is associated with stress and human disturbance. J. Appl. Ecol. 50, 912–919.
Tablado, Z., and Jenni, L. (2017). Determinants of uncertainty in wildlife responses to human disturbance. Biol. Rev. 92, 216–233. doi: 10.1111/brv.12224
Tablado, Z., Revilla, E., Dubray, D., Saïd, S., Maillard, D., and Loison, A. (2016). From steps to home range formation: species-specific movement upscaling among sympatric ungulates. Funct. Ecol. 30, 1384–1396.
Tricola, G. M., Simons, M. J. P., Atema, E., Boughton, R. K., Brown, J. L., Dearborn, D. C., et al. (2017). The rate of telomere loss is related to maximum lifespan in birds. Philos. Trans. R. Soc. B Biol. Sci. 373:20160445. doi: 10.1098/RSTB.2016.0445
Vedder, O., Moiron, M., Bichet, C., Bauch, C., Verhulst, S., Becker, P. H., et al. (2021). Telomere length is heritable and genetically correlated with lifespan in a wild bird. Mol. Ecol. doi: 10.1111/mec.15807
Viblanc, V. A., Schull, Q., Roth, J. D., Rabdeau, J., Saraux, C., Uhlrich, P., et al. (2018). Maternal oxidative stress and reproduction: testing the constraint, cost and shielding hypotheses in a wild mammal. Funct. Ecol. 32, 722–735. doi: 10.1111/1365-2435.13032
Walker, B. G., Boersma, P. D., and Wingfield, J. C. (2006). Habituation of adult Magellanic penguins to human visitation as expressed through behavior and corticosterone secretion. Conserv. Biol. J. Soc. Conserv. Biol. 20, 146–154. doi: 10.1111/j.1523-1739.2005.00271.x
Weimerskirch, H., Shaffer, S. A., Mabille, G., Martin, J., Boutard, O., and Rouanet, J. L. (2002). Heart rate and energy expenditure of incubating wandering albatrosses: basal levels, natural variation, and the effects of human disturbance. J. Exp. Biol. 205, 475–483. doi: 10.1242/jeb.205.4.475
Wilbourn, R. V., Moatt, J. P., Froy, H., Walling, C. A., Nussey, D. H., and Boonekamp, J. J. (2018). The relationship between telomere length and mortality risk in non-model vertebrate systems: a meta-analysis. Philos. Trans. R. Soc. B Biol. Sci. 373:20160447. doi: 10.1098/rstb.2016.0447
Keywords: human disturbance, outdoor recreation, telomere length, corticosterone, stress hormones, transgenerational effects, body condition, oxidative stress
Citation: Tablado Z, Bötsch Y, Powolny T, Massemin S, Zahn S, Jenni-Eiermann S and Jenni L (2022) Effect of Human Disturbance on Bird Telomere Length: An Experimental Approach. Front. Ecol. Evol. 9:792492. doi: 10.3389/fevo.2021.792492
Received: 10 October 2021; Accepted: 24 November 2021;
Published: 10 January 2022.
Edited by:
Carlos Alonso Alvarez, National Museum of Natural Sciences, Spanish National Research Council (CSIC), SpainReviewed by:
Tiia Kärkkäinen, University of Turku, FinlandMarco Parolini, University of Milan, Italy
Copyright © 2022 Tablado, Bötsch, Powolny, Massemin, Zahn, Jenni-Eiermann and Jenni. This is an open-access article distributed under the terms of the Creative Commons Attribution License (CC BY). The use, distribution or reproduction in other forums is permitted, provided the original author(s) and the copyright owner(s) are credited and that the original publication in this journal is cited, in accordance with accepted academic practice. No use, distribution or reproduction is permitted which does not comply with these terms.
*Correspondence: Zulima Tablado, enV0YWxAeWFob28uZXM=