- 1Guangdong Provincial Key Laboratory of Animal Molecular Design and Precise Breeding, School of Life Science and Engineering, Foshan University, Foshan, China
- 2National Engineering Laboratory for Animal Breeding, Key Laboratory of Animal Genetics, Breeding and Reproduction, Ministry of Agriculture, College of Animal Science and Technology, China Agricultural University, Beijing, China
Coprolites (mummified or fossilized feces), belonging to the group of ichnofossils, are fossilized remains of feces produced by animals. Various types of data from coprolites provide detailed evidence of the producer’s condition, like diet, intestinal microbiome, virus infection and parasites diseases. In addition, the palaeoenvironment information relevant to producers’ ecological niche can be drawn from taphonomy details the coprolites mirrored. At present, the phylogenetic clues of the producer’s population can be determined by advanced molecular biotechnologies. With the integration of multiple methods and techniques, coprolite has been widely accepted as an ideal material to study the diet, evolution, and palaeoenvironment of producers. In this paper, we reviewed the history of coprolite research, enumerated and interpreted the data recovered from coprolites, and explained their research value to palaeocoprology and evolutionary biology. Finally, we summarized the current directions of coprolite research and looked into its future prospects.
Introduction
Coprolites are fossilized feces of animals that belong to the group of ichnofossils (Callen and Cameron, 1960; Heizer and Napton, 1969; Bryant, 1974; Reinhard and Bryant, 1992; Hunt et al., 1994). As excellent biological and environmental archives, coprolites are still underutilized for obtaining better knowledge regarding their producers’ condition and the corresponding palaeoenvironment. The macroscopic and microscopic components of coprolites provide significant types of information. Macroscopic inclusions of coprolites, such as animal bones, hair and feathers could provide broad indications for the diets of producers, including digestive efficiency, feeding strategies and their trophic levels in the food chain. Meanwhile, the microscopic components of coprolites, such as pollen, bacteria, fungi, and archaea are essential for qualitative analyses of ancient gastrointestinal microbiomes, as well as quantitative analysis of the vegetation in the palaeoenvironment. Pathogenic flora and parasites in coprolites will be constructive in understanding virus infection and parasite diseases of producers. In particular, the DNA and RNA fragments sustaining in coprolites have become an additional genetic information source for the phylogenetic analysis of the producers, especially for extinct organisms.
Starting from the description of coprolites, we illustrate the progress of coprolites research for reproducing a complete lifelike scene of the producers in the palaeoenviroment, then summarize the technical points and difficulties of coprolite research at present, and conclude with a summary of the prospects of coprolites research.
The Discovery of Coprolites
According to the palaeontological and archeological literature, the term coprolite was first coined by the British geologist William Buckland in 1829 and applied to describe mineralized dinosaur feces in the Lyme Regis (Buckland, 1829). The term “coprolite” consists of two Greek words, Kopros and Litos, which represent feces/excreta and stone/rock, respectively (Reinhard and Bryant, 1992; Ford and O’Connor, 2002). In addition, the term palaeofaece is also used to refer to coprolite (Watson and Yarnell, 1966).
The Definition and Characteristics of Coprolites
Origin of Coprolites
Coprolites are usually produced by mammals, reptiles, annelids, mollusks, coelenterates, and arthropods. In addition to the wild areas where animal remains are often found, coprolites are also excavated from latrines, sewers, and cesspits by the human that can be traced back to the Quaternary period (Rocha et al., 2006; Smith, 2013; Sistiaga et al., 2014b; Chessa et al., 2020; Sabin et al., 2020).
Physical Traits of Coprolites
Although the physical traits of fresh feces are often dependent on the foods the producers ingested (Gilmour and Skinner, 2012), having undergone abundant chemical and microbial changes during thousands of years of burial, the physical traits of coprolites are quite different from those of fresh feces. However, there is no standard model or method for the morphological classification of coprolites, except for a few explicit terms, such as overall shape, outline, size, continuity, pliability, matrix color, and large inclusions of coprolites. By screening the results of previous studies (Neumayer, 1904; Häntzschel et al., 1968; Jain, 1983; McAllister, 1985; Jouy-Avantin et al., 2003; Hunt et al., 2012; Laojumpon et al., 2012; Barrios-de Pedro et al., 2018), the morphologies of coprolites could be classified as six main types including discoidal, spiral, round, rod-like, kidney-shaped, and irregular (Figure 1). Interestingly, spiral coprolites are believed to be the most distinctive group of coprolites. Since Neumayer first introduced a systematic terminology for spiral coprolites based on coprolites from the Early Permian of Texas in 1904 (Neumayer, 1904), more detailed terminologies for describing spiral coprolites have been proposed (Jain, 1983; McAllister, 1985; Hunt et al., 2012; Laojumpon et al., 2012). For instance, the number of spirals visible on the lateral aspect of the coprolites referred to as spiral counts (McAllister, 1985), the number of whorls (Jain, 1983), or the number of coils (Laojumpon et al., 2012). Other studies have also proposed specific morphology descriptions of coprolites (Jouy-Avantin et al., 2003; Barrios-de Pedro et al., 2018). Coprolites from the Las Hoyas site (Barrios-de Pedro et al., 2018), a well-known Early Cretaceous site located in Spain, were delimited according to a dichotomous-key method based on three points: (1) the presence/absence of spiral marks, (2) the morphology of coprolite ends, and (3) the overall shape, outline, diameter, and constrictions. Subsequently, 12 morphotypes, including spiral, circular, irregular, elongated, rosary, and so on were distinguished.
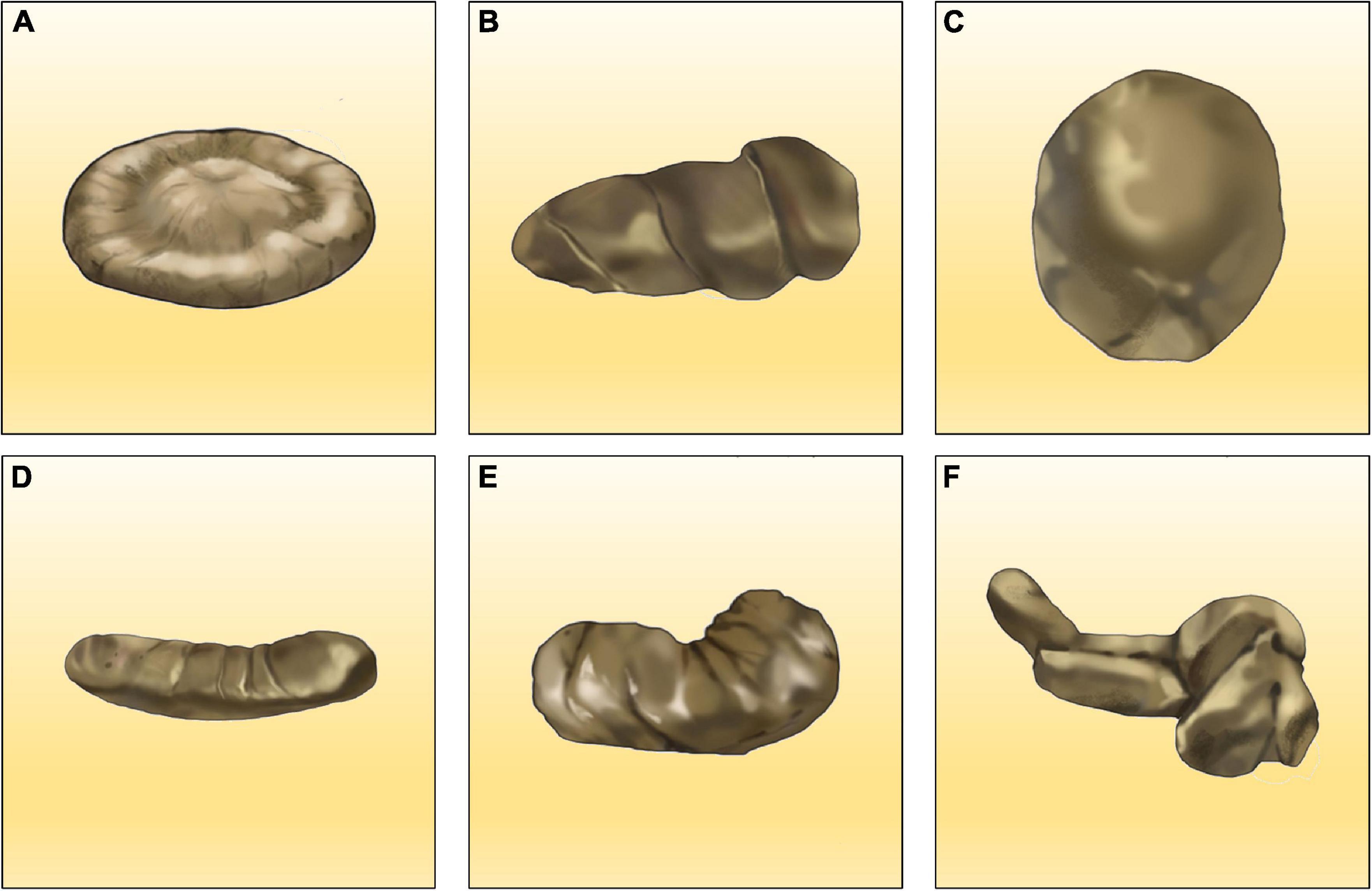
Figure 1. Classification of coprolites by morphology. (A) Discoidal; (B) spiral; (C) round; (D) rod-like; (E) kidney-shaped; (F) irregular.
Chemical Contents of Coprolites
Due to the process of diagenesis, the chemical inclusions of coprolites vary from different preserved phases and places. For example, fresh components such as calcite, aragonite, quartz limonite, siderite, and pyrite of feces are replaced by carbonate or phosphate minerals during the formation of coprolites. Specifically, coprolites from sites protected by caves and rock shelters exhibit the best preservation (Reinhard et al., 2019). The petrification caused by the deterioration and displacement is necessary for fresh feces to transform into coprolites. However, the mineralization process is usually affected by the minerals such as calcium in the soil, which is able to alter the microcrystalline calcium phosphate of coprolites and result in incorrect determination in the biochemical investigation of coprolites.
Technological Advances of Coprolite Research
By systematically integrating discrete data provided by the inclusions, coprolites present valuable information about the producers and their palaeoenvironments as well as their correlation. Since the first report on coprolites, studies on coprolites have gone through three stages. From 1829 to the 1970s, coprolites research expanded from simple morphological analysis to diet analysis based on chemical experiments such as immersion, which helped establish a prototype of palaeocoprology (Callen and Cameron, 1960; Callen, 1963, 1965; Callen and Martin, 1969). From the 1970s to the early twenty first century, the molecular potential of coprolites has been examined deeper owing to the development of various molecular biology methods, such as the extraction and cloning of aDNA (Poinar et al., 1998, 2001; Hofreiter et al., 2000). The third stage began in the 2000s, when high-throughput sequencing, different fields of archeology, molecular biology, environmental ecology, and geology were weaved together for coprolite research (Tito et al., 2012; Wood et al., 2016; Green and Speller, 2017). To date, the subdisciplines and applications of coprolite researches have incorporated aspects of morphology, macroscopic content, stable isotope and biomarker analyses, microbiome analysis/palaeomicrobiology, ancient DNA, palaeobotany, palaeovirology, and parasitology. Using coprolites as brushes, inclusions as pigments, we are able to draw information about the palaeoenvironment and producers at the time and place.
Morphological Study
Describing the morphological characteristics of coprolites is the first step to completing the portrait of the coprolite. Although in Shillito et al.’s (2011) opinion, the morphological characteristics of coprolites are formed by the types of foods consumed, the way of foods moved through the digestive tract, and the health and age of the producers, only in a few cases the producers can be distinguished by the external appearance of coprolites. In the early stage, without the ability to identify the internal components, researchers attributed certain coprolite specimens to small hominids based on their size and shape (Reinhard and Bryant, 1992). With the development of technologies to discriminate the interference from other organisms, the specific characteristics of coprolites were used to discriminate the interference from other organisms. Moreover, the appearance of the coprolites could not only illuminate the information of the producers but also show the influence of other organisms’ activities on the coprolites during the long burial process, providing additional information about the palaeoenvironment at the time. For example, the small scrapes, tracks, and grooves on coprolites in shallow-marine strata of Åsen indicated a manipulation by other organisms (presumably coprophagous organisms) after deposition, which could be evidence of benthic activity and resource recycling in the Late Cretaceous marine ecosystem (Eriksson et al., 2011). Although there is still no convincing consensus on how to describe the appearance of coprolites, a full morphological record is recommended before the downstream destructive analysis.
Faunal Study
After characterizing the appearance of coprolites, faunal macroscopic analyses on coprolite inclusions are helpful to disclose more detailed features coprolites, especially information about prey animals of the carnivore coprolites (Horwitz and Goldberg, 1989; Chin, 2002; Bajdek et al., 2016). With thin-section analysis and targeted microscopes observation on carnivorous tetrapod’s coprolite from the Late Permian Vyazniki site of Russia, bone fragments, hair-like structures, invertebrate remains, and possible invertebrate eggs were found. Then depending on the digestive corrosion degrees of bone remains, the coprolites are proposed coming from therapsid carnivores and archosauromorphs or other non-therapsid carnivores, respectively. And the hair-like structures found in one Upper Permian coprolite suggested that the latest Permian therapsids probably had hair-like integuments or hair suits. All above information derived from coprolites portrays a typical carnivore’s image with therapsids and hair suits at that time (Bajdek et al., 2016). Meanwhile, much of the palaeoecological information relies on well-preserved organic remains, which comprise food residues through the early lithification of the feces, a process thought to be facilitated by bacterial autolithification and high phosphate content (Hollocher et al., 2001; Chin et al., 2003; Qvarnström et al., 2016, 2019c).
Scanning electron microscopy (SEM) coupled with energy-dispersive X-ray spectroscopy (EDS) and propagation phase-contrast synchrotron microtomography (PPC-SRμCT) are powerful techniques that could help study various inclusions in the entire coprolite by performing non-destructive imaging in three dimensions (Sanz et al., 2016; Qvarnström et al., 2017, 2019a,2019b,2019c; Zatoń et al., 2017; Moreau et al., 2020; Rummy et al., 2021). By using these techniques, the morphology and macroscopic inclusions of three coprolites collected from the early Kimmeridgian (Hypselocyclum Zone) intertidal deposits were explored. And the scanning results suggested the remains of ostracods, bivalves, gastropods, and crustaceans as well as possible bristles from polychaete worms, providing the first direct evidence of filter-feeding pterosaurs that deliberately targeted minute organisms (Qvarnström et al., 2019b).
Analysis of Stable Isotopes and Other Biomarkers
The isotopic composition of carbon and nitrogen (i.e., 13C/12C ratio, δ15N, etc.) in animals closely represents the dietary composition and the trophic level of the producers in the food chain (DeNiro and Epstein, 1978; Vogel, 1978; Minagawa and Wada, 1984; van der Merwe, 1986; Sealy et al., 1987; Lee-Thorp et al., 1989). Stable isotope analyses are expected to provide key information on primary diet and digestion mechanism, especially for herbivorous producers (Ghosh et al., 2003; Harrison, 2011; Sistiaga et al., 2014a). For example, the average 13C/12C ratio of titanosaurs’ coprolites from the Cretaceous Lameta Formation in central India suggested a primary C3 plant diet for titanosaurs (Ghosh et al., 2003). The δ15N value of coprolites indicated that an active gut fermentation process, which is a common digestive mechanism of modern large herbivorous mammals, was rare in titanosaurs (Ghosh et al., 2003).
In addition, molecular biomarker (such as 5β-stanols) analysis highlighted a significant potential in palaeocoprology to identify the specific diet of producers, for instance, omnivorous, carnivorous or herbivorous diet (Lin et al., 1978; Knights et al., 1983; Bull et al., 2005; Baeten et al., 2011; Gill and Bull, 2012). The steroidal biomarker analysis of a 14,000 years old putative human coprolite from Paisley Cave, which yielded the predominant presence of 5β-stigmastanol, and therefore reversely supported that the putative human coprolite was from a herbivore, and the presence of palaeoindian mitochondrial DNA in coprolites was inferred to be contamination (Sistiaga et al., 2014a). Beyond that, bile acids, testosterone, and estrogen levels also help to identify the producers (Rhode, 2003; Zhang et al., 2020; Porru et al., 2021).
However, studies have shown that microbial activities around ancient specimens could change the isotopic signal and confound archeological results (Reinhard and Bryant, 1992), suggesting that they may affect the results of palaeocoprology research. Meanwhile, stable isotope and other biomarker analyses in palaeocoprology may be feeble when there is no clear knowledge of the stable isotope ecology and biophysiology of extinct animals and ancient animals.
Microbiome Analysis/Palaeomicrobiology
DNA of micro-eukaryotes, bacteria, and archaea, acquired from coprolites can be purified and sequenced to roughly reconstruct the oral and gut microflora of producers. Meanwhile, by combining the microbial information derived from coprolites with the results of anatomical adaptations, gut contents, and feeding traces, a general picture about the producers’ feeding and predating behavior, gut microbiota, group structure, and other characteristics can be derived (Tito et al., 2012; Santiago-Rodriguez et al., 2013; Appelt et al., 2014; Cano et al., 2014; Dentzien-Dias et al., 2017). Pre-Columbia coprolites from two cultures, Huecoid and Saladoid, were evaluated for their bacterial, fungal, and archaeal content (Santiago-Rodriguez et al., 2013). The results showed that Proteobacteria, Bacteroidetes, and methanogens were present in the coprolite specimens from both cultures. However, Basidiomycetes were the most notable fungi in Huecoid samples and Ascomycetes predominated in Saladoid samples, which suggested a difference in the dietary habits, possibly resulting from the cultural difference. The mineralization process of coprolites can also be explained by the bacterial content of coprolites. By examining coprolites from herbivorous dinosaurs (Hollocher et al., 2001), it was revealed that bacteria within the capillaries induced initial coprolites mineralization and generated barriers that protected organic residues from subsequent destruction.
Due to the full exposure to the environment, it is not easy to distinguish whether the microbiota is from the internal matrix of coprolite or the surrounding environment. Therefore, an optimal metagenomic analyses workflow aiming at coprolites is needed. Comfortingly, various improvements have been carried out, such as coproID, which uses a combination of host and microbial DNA to identify the producers of coprolites (Borry et al., 2020).
Ancient DNA
Ancient DNA (aDNA), the genetic material isolated from ancient organisms, has various sources in coprolites, including producers, faunal organisms that producers preyed on, and the organisms activated in the place where coprolites were buried. It is difficult to perform the aDNA studies at the beginning because inhibitors may hamper the PCR reaction, such as lignin and Maillard reaction products found in coprolites (Poinar et al., 1998). In 1996, ancient human DNA was recovered for the first time from four coprolites at two sites in western North America (Sutton et al., 1996). For years, various protocols were sprung up to effectively against inhibitors and extract aDNA successfully, and the combination of soil DNA extraction method and aDNA extraction method has been proved as an optimal approach to extract aDNA from coprolites (Karpinski et al., 2017; Hagan et al., 2020). With the development of high-throughput sequencing and metagenomic analyses, aDNA analyses of coprolites have been dramatically enhanced and broadened to provide more information about the producers. Using a modified PowerSoil DNA isolation kit, researchers performed metagenomic analyses on a fourteenth century human coprolite and found seventeen bacterial species representing the environment and organisms known to be gut inhabitants in Belgium (Appelt et al., 2014).
Even more, well-preserved coprolites are able to provide sufficient authigenic DNA to carry out mitogenomic phylogenesis and evolution of the producers (Speller et al., 2010; Palacio et al., 2017). A nearly complete mammoth mitochondrial genome (81.6%) at an average coverage depth of 8.1 × from a coprolite at Bechan Cave was reconstructed, which proved the utility of aDNA in coprolites (Karpinski et al., 2017). In another study (Palacio et al., 2017), large amounts of bovine aDNA were obtained and assembled to a complete mitochondrial genome from a cave hyena’s coprolite. And the result showed the mitochondrial genome sequence from the coprolite was belonged to the clade of ancient bison specimens and closely related to the extant European bison, proving Bison schoetensacki as a sister species of Bison bonasus.
Palaeobotanical Analyses
Meanwhile, coprolites contain abundant botanical remains, including pollen, seeds and fibrous materials, which could be informative to generate a regional map of plant distribution in specific environments and to recover the trophic structure of the producers. In general, palaeobotanical analyses in this area are set up to specify the plant remains in coprolites. Pollen spectra from animal feces have been supposed the optimal analog of local vegetation compared to sediments dominated by wind-pollinated pollen species (Carrión, 2002). In the mid-1960s, pollen analysis was first applied to human coprolites (Martin and Sharrock, 1964). In the 1970s, the classification method combining phytophagous diet and environmental pollen was established (Bryant, 1974; Williams-Dean, 1978; Scott, 1979; Clary, 1983; Reinhard and Bryant, 1992). Thereafter, optical microscopes and other microhistological techniques on macrobotanical remains such as plant leaves, seeds, and stems as well as pollens, were used in palaeobotanical analyses of coprolites (Bryant, 1977; Greig, 1981; Akeret and Rentzel, 2001; Carrión et al., 2001, 2007; Baeten et al., 2011; Kühn et al., 2013; Wood and Wilmshurst, 2013; Welker et al., 2014; Velázquez et al., 2015; Tosto et al., 2016; Taylor et al., 2020). Along with ecological niche models (ENMs) (Peterson et al., 2011), which identify the relationships between species presence records and biotic conditions at certain sites, pollen analysis of coprolites can be used to predict habitat suitability of producers under past climatic conditions. By combining pollen analysis, macrofossil analysis and aDNA analysis on the coprolites from the extinct Balearic mountain goat Myotragus balearicus (M. balearicus), it was concluded that M. balearicus was probably a browser that ate mostly Buxus balearica (B. balearica) (Welker et al., 2014). Accompanied with ENMs, authors supposed that the extinction of M. balearicus was related to the decline and regional extinction of B. balearica (Welker et al., 2014).
However, it is important to distinguish whether the pollen was ingested intentionally by producers or carried from the environment by the wind and insects, which would result in misleading interpretation about pollen in coprolites. On account of this, taking the concentration and relative proportion of grains as an indicator to identify the intentional ingestion of pollen was recommended. To be specific, a high frequency of pollen from specific taxa implies intentional ingestion of specific flowers, seeds or foliage rather than a wind- and insect-pollinated origination (Shillito et al., 2011).
Palaeovirology and Palaeoparasitology
Based on good preservation conditions, well-preserved remains of pathogens and intestinal parasites in archaeological materials, the health status of the organisms can be constructed, such as gastrointestinal infection. As far as we know now, the first evidence of a prehistoric parasite, a whipworm (Trichuris trichiura) egg, came from the study on the colonic contents of an Inca mummy (Pizzi and Schenone, 1954). Thereafter, palaeovirological and palaeoparasitological studies on coprolites have been widely performed to reveal the history of the host-parasite relationship, zoonosis, and phylogenetic changes in enteric protozoa (Taglioretti et al., 2015). Microscopic examination and enzyme-linked immunosorbent assays (ELISA) are common tools used in parasitological research on coprolites (Morrow and Reinhard, 2016; Nunes et al., 2017). Remnants of intestinal protozoans and helminths, such as the uninucleated cysts of Entamoeba sp., and the presence of Cryptosporidium sp. in the coprolites of M. balearicus, allowed further clarification of the reason for the extinction of M. balearicus (Nunes et al., 2017). The viral aDNA obtained from the coprolites of two pre-Columbian indigenous cultures were used to deduce different ancient diet-related information. The results confirmed that rodents such as Isolobodon portoricensis (hutía) and Heteropsomys insulans (spiny rat) were normal dietary components of the two pre-Columbians (Turvey et al., 2007; Rivera-Perez et al., 2015). Despite the dietary habits, parasites in coprolite contain clues about where parasites originated from and how they transmitted. Taenia spp. are frequently found in human coprolites from areas where beef is commonly eaten, but rarely found in pre-Columbian New World sites where beef is not available, suggesting the first human infections with this parasite is probably caused by beef (Gonçalves et al., 2003). However, it should be noted that the virus and parasite found exactly in human-derived coprolites retain two possible sources, one is the consumption of infected animals from the mouth, another is the infection by soil, water around the infected animals. Five sets of factors affect the preservation of parasite eggs: abiotic, contextual, anthropogenic, organismal, and ecological. Among these, the abiotic factor acts as the key role which refers to temperature, soil, pH, humidity, and other non-living parameters (Morrow et al., 2016). Therefore, the environmental and the man-made conditions mentioned above are decisive in the palaeovirological and parasitological studies on coprolites. Correspondingly, a deep metagenomic sequencing is recommended to distinguish the exact origination of virus and parasite in coprolites (Shillito et al., 2011).
Applications of Coprolites Analyses
With the integration of multiple methods and techniques, coprolite has been widely accepted as an ideal material to study the diet, evolution, and palaeoenvironment of producers.
To Describe the Taphonomic Features and Sedimentological Properties
The taphonomic features of coprolites, including arrangement direction and distribution density, could be used to interpret sedimentological properties in the specified stratum, as well as the defecation speed, posture, and other behavioral habits concerning producers. If the coprolites group shows a regular linear arrangement and uniform distribution, it can be inferred that the producers were making a regular directional movement during defecation, which usually reflects a comfortable and normal state of the producers at that time. Abundant coprolites found at the Las Hoyas site were verified as taphonomically autochthonous because their taphonomic features were consistent with the feces produced and deposited in an aquatic ecosystem (Barrios-de Pedro et al., 2018). Upon integration with multiple biochemical data and morphological types, the ecosystem information surrounding the producers can be uncovered more clearly and macroscopically. A study of coprolites from the Pisdura in India showed the advantages of combining morphological methods with chemical and palaeobotanical methods (Khosla et al., 2015). In detail, the SEM with macerated fractions of Pisdura coprolites revealed that seven ostracod taxa and the different plant remains were included in coprolites, such as a probable Gabonisporites vigourouxii globular spore. And optical emission spectroscopy showed a high level of calcium phosphate in coprolites. Combined with a variety of lithotypes indicating fluctuating lake levels, it is revealed that the coprolites were buried under Fluvio-lacustrine positional conditions and the producers were omnivores.
To Disclose the Dietary, Sanitary Culture, and Husbandry Development
The inclusion of data from coprolites generally provides a unique perspective on the diet of producers, such as dietary, sanitary culture, and husbandry development. By combining pollen and aDNA analyses on coprolites from New Zealand purebred dogs, Wood et al. (2016) revealed their predominant diet of marine fish and plant matter, which overlapped with the typical diets of early local indigenous people in New Zealand. Remains of fish heads found in dogs’ coprolites raised a presumption that the fish heads were thrown to dogs by humans who ate fish bodies only (Tolar and Galik, 2019), which suggested a close relationship between the dogs and humans at 1,000–750 years ago. The 16S/18S rDNA and shotgun metagenomics sequencing of pre-Columbian coprolite assemblies from two different sites, the Huecoid and Saladoid, indicated distinctly different microbiota, viromes, and diets of people from two sites, which further supported the Huecoid and Saladoid were distinct cultures with their own set of characteristics (Cano et al., 2014; Rivera-Perez, 2017). A comparative study on the microbiome data from coprolites to modern environmental microbiome including soil, compost as well as the gut, oral and skin of the nearby habitants have been deployed previously. The results showed a striking compositional similarity between the coprolites microbiome and rural communities, which implied a huge change in response to the lifestyle change from rural to modern cosmopolitan cultures (Tito et al., 2012). By combining palaeoparasitological and palaeobotanical analyses on fourteen human coprolites from three transiting chronological periods in Lluta Valley, de Souza et al. (2018) recovered an increasing frequency, diversity and number of parasites eggs in coprolites with civilization development, which supported an increasing severe sanitary condition and sanitary concepts improvements of the local people with the Inca expansion in the Lluta Valley. Moreover, thin-section analyses on coprolites-containing samples were also expected to illustrate the animal feeding and husbandry regimes at the respective site. The plant macro- and micro- remains analyses against Lycopodium spore tablets were deployed on coprolites-containing samples from two late Neolithic sites in Germany, and the results showed different plant remains at different layers, providing evidence for distinct animal husbandry systems for these two sites (Kühn et al., 2013).
To Trace the Evolutionary History of Producers
Currently, the archaeological analyses and aDNA interpretation on the bone and dental calculus remains are able to provide plenty of morphological and genetic information on the evolutionary history of organisms. Nevertheless, the various data extracted from the coprolites, in particular those of microbiome, pollen, aDNA, parasites, etc. show how the producers have evolved and what intestinal characteristics were retained or emerged in the long, having great power regarding the understanding of evolution. Based on the analyses of pollen and macrofossils of coprolites from the extinct Balearic mountain goat Myotragus balearicus, it has been suggested that the extinction of M. balearicus was highly related to the decline and regional extinction of the plant species B. balearica, which formed a major component of its diet (Welker et al., 2014). By comparative analysis on aDNA from turkey’s bones and coprolites, a unique domesticated breed in precontact Southwestern America was identified (Speller et al., 2010). Furthermore, by integrating high-throughput sequencing on 18S rRNA, microfossil analysis on pollen and Sanger sequencing on parasites of the avain coprolites in New Zealand, an extinct mycophagy-diet moa (Aves, Dinornithiformes) was found parasite-host coextinction between the moa and several parasite species were deduced, which caused a broken ecological interaction and impacted the historical ecosystem processes (Boast et al., 2018).
To Recover the Palaeoenvironment
Especially for herbivores, identification of inclusions in their dung can indicate regional vegetation distribution, providing further information of the ecological interactions among various organisms and guidance for contemporary conservation and ecosystem restoration efforts (Carrión et al., 2007; Boast et al., 2018; Wang et al., 2018; Zhang et al., 2019). Biomarker analysis and detrended correspondence analysis (DCA) between pollen and non-pollen palynomorphs (NPPs) were performed on dog coprolites from a Chinese Neolithic site, Tianluoshan. And the biomarker analysis suggested the dogs with a plant-rich diet closely relating to humans while the pollen and NPPs results indicated three main internal vegetation types, indicating the vegetation patterns of the main areas affected by human activities in Tianluoshan (Zhang et al., 2019). For a decade, broader palaeobotanical analyses were applied to identify stomatal traits of coprolites to provide evidence of environmental factors in specific areas in the past. Based on the inseparable relationship between the stomatal density of plants and CO2 concentration, the stomatal density and index of epidermal fragments of B. sempervirens found in the coprolites were examined and compared with the living related species (Rivera et al., 2014). The results indicated that ancient B. sempervirens had a higher stomatal density and index than those of extant B. balearica and B. sempervirens species, providing palaeobotanical evidence of a change in atmospheric CO2 concentration since the mid-Holocene period in the Mediterranean basin (Rivera et al., 2014). In the study about dog coprolites from the Late Neolithic pile-dwelling site in Slovenia, abundant birch, goosefoot, turnip, water chestnut, and flax were found, suggesting the ecological conditions around the site as marshy ground with a slow-flowing river or a lake (Tolar and Galik, 2019).
Discussion
Whilst believed more reliable than other archaeological materials from the same site and time (Hofreiter et al., 2001; Carrión, 2002), coprolites have received less attention than bones and dental calculus. Over the years practiced, palaeocoprology has been proven to be a bond for interdisciplinary fields by gathering various data from coprolites to understand the dietary structure, physical condition, genesis evolution, and phylogenetic status of producers. Accompanying with information about the ecological process, culture, and social evolution at the time, coprolites can further mirror every nuance of what has happened to the producers and environment in general at the time and place.
Due to the continuous microbial activities and deposition of other substances around coprolites as well as the change in taphonomic conditions such as temperature, humidity, and oxygen concentration, the non-contamination downstream analysis is a great challenge (Appelt et al., 2016). In addition, an important issue in archaeozoology is the use of extant animal materials, such as bones, teeth, and feces, as control samples to interpret the results from the fossil record. The following problem is that excellent records of a large number of reference individuals from controlled populations are difficult to obtain, especially for wild and extinct animals. Comfortingly, interdisciplinary technologies and tools have been deployed in the research of palaeocoprology to solve problems related to diet, evolution, and palaeoecology. In this situation, future research efforts should focus on the scientific and normative collection, transportation, preservation of coprolite, and the establishment of standardized procedures to remove contamination in subsequent experiments as well as to make a clear assessment of their negative effects on palaeocoprology, and eliminate them as much as possible. Another step is expected to expand collaboration between archaeozoology, biology, and ecology to build more reliable frames of references, and more standardized experimental designs and methods. With the increasing discovery of coprolite-related ancient key sites around the world, coprolite researches will have greater value in the era of multi-interdisciplinary collaboration.
Author Contributions
HX and XBZ designed this project. LY, XZ, XBZ, and HX drafted the manuscript. All authors contributed critically to the drafts and gave final approval for publication.
Funding
We acknowledge funding from the National Natural Science Foundations of China (31961133031), the Foundation for Distinguished Young Talents (2019KQNCX167) and Creative Team in Higher Education of Guangdong (2019KCXTD006), the Guangdong Provincial Key Laboratory of Animal Molecular Design and Precise Breeding (2019B030301010), and the Scientific Research Fund for Postdoc of Foshan City (BKS209071). The funding bodies contributed nothing to the study design, data analyses, data interpretation, or manuscript preparation.
Conflict of Interest
The authors declare that the research was conducted in the absence of any commercial or financial relationships that could be construed as a potential conflict of interest.
Publisher’s Note
All claims expressed in this article are solely those of the authors and do not necessarily represent those of their affiliated organizations, or those of the publisher, the editors and the reviewers. Any product that may be evaluated in this article, or claim that may be made by its manufacturer, is not guaranteed or endorsed by the publisher.
References
Akeret, Ö, and Rentzel, P. (2001). Micromorphology and plant macrofossil analysis of cattle dung from the Neolithic lake shore settlement of Arbon Bleiche 3. Geoarchaeology 16, 687–700. doi: 10.1002/gea.1016
Appelt, S., Armougom, F., Le Bailly, M., Robert, C., and Drancourt, M. (2014). Polyphasic analysis of a middle ages coprolite microbiota, Belgium. PLoS One 9:e88376. doi: 10.1371/journal.pone.0088376
Appelt, S., Drancourt, M., and Le Bailly, M. (2016). Human coprolites as a source for paleomicrobiology. Microbiol. Spectr. 4, 59–74. doi: 10.1128/microbiolspec.PoH-0002-2014
Baeten, J., Marinova, E., De Laet, V., Degryse, P., De Vos, D., and Waelkens, M. (2011). Faecal biomarker and archaeobotanical analyses of sediments from a public latrine shed new light on ruralisation in Sagalassos, Turkey. J. Archaeol. Sci. 39, 1143–1159. doi: 10.1016/j.jas.2011.12.019
Bajdek, P., Qvarnström, M., Owocki, K., Sulej, T., Sennikov, A., Golubev, V., et al. (2016). Microbiota and food residues including possible evidence of pre-mammalian hair in Upper Permian coprolites from Russia. Lethaia 49, 455–477. doi: 10.1111/let.12156
Barrios-de Pedro, S., Poyato-Ariza, F., Moratalla, J., and Buscalioni, Á (2018). Exceptional coprolite association from the early Cretaceous continental Lagerstätte of Las Hoyas, Cuenca, Spain. PLoS one 13:e0196982. doi: 10.1371/journal.pone.0196982
Boast, A., Weyrich, L., Wood, J., Metcalf, J., Knight, R., and Cooper, A. (2018). Coprolites reveal ecological interactions lost with the extinction of New Zealand birds. Proc. Natl. Acad. Sci. U.S.A. 115, 1546–1551. doi: 10.1073/pnas.1712337115
Borry, M., Cordova, B., Perri, A., Wibowo, M., Honap, T., Ko, J., et al. (2020). CoproID predicts the source of coprolites and paleofeces using microbiome composition and host DNA content. PeerJ 8:e9001. doi: 10.7717/peerj.9001
Bryant, V. (1974). Prehistoric diet in southwest Texas: the coprolite evidence. Am. Antiq. 39, 407–420. doi: 10.2307/279430
Bryant, V. (1977). A 16,000 year pollen record of vegetational change in central Texas. Palynology 1, 143–156. doi: 10.1080/01916122.1977.9989156
Buckland, W. (1829). XII.—On the discovery of coprolites, or fossil fæces, in the Lias at Lyme Regis, and in other Formations. Trans. Geol. Soc. Lond. 2, 223–236. doi: 10.1144/transgslb.3.1.223
Bull, I., Elhmmali, M., Perret, V., Matthews, W., Roberts, D., and Evershed, R. (2005). “Biomarker evidence of faecal deposition in archaeological sediments at Çatalhöyük,” in Inhabiting Çatalhöyük: Reports from the 1995-99 Seasons, ed. I. Hodder (Cambridge: McDonald Institute Monographs).
Callen, E. (1963). “Diet as revealed by coprolites,” in Science in Archaeology, eds D. Brothwell and E. Higgs (New York, NY: Basic Books, Inc).
Callen, E. (1965). Food habits of some pre-Columbian Mexican Indians. Econ. Bot. 19, 335–343. doi: 10.1007/BF02904803
Callen, E., and Martin, P. (1969). Plant remains in some coprolites from Utah. Am. Antiq. 34:329. doi: 10.2307/278417
Cano, R., Rivera-Perez, J., Toranzos, G., Santiago-Rodriguez, T., Narganes-Storde, Y., Chanlatte-Baik, L., et al. (2014). Paleomicrobiology: revealing fecal microbiomes of ancient indigenous cultures. PLoS one 9:e106833. doi: 10.1371/journal.pone.0106833
Carrión, J. (2002). A taphonomic study of modern pollen assemblages from dung and surface sediments in arid environments of Spain. Rev. Palaeobot. Palynol. 120, 217–232. doi: 10.1016/S0034-6667(02)00073-8
Carrión, J., Riquelme, J., Navarro, C., and Munuera, M. (2001). Pollen in hyaena coprolites reflects late glacial landscape in southern Spain. Palaeogeogr. Palaeoclimatol. Palaeoecol. 176, 193–205. doi: 10.1016/S0031-0182(01)00338-8
Carrión, J., Scott, L., Arribas, A., Fuentes, N., Gil-Romera, G., and Montoya, E. (2007). Pleistocene landscapes in central Iberia inferred from pollen analysis of hyena coprolites. J. Q. Sci. 22, 191–202. doi: 10.1002/jqs.1024
Chessa, D., Murgia, M., Sias, E., Deligios, M., Mazzarello, V., Fiamma, M., et al. (2020). Metagenomics and microscope revealed T. trichiura and other intestinal parasites in a cesspit of an Italian nineteenth century aristocratic palace. Sci. Rep. 10:12656. doi: 10.1038/s41598-020-69497-8
Chin, K. (2002). Analyses of coprolites produced by carnivorous vertebrates. Paleontol. Soc. Pap. 8, 43–50. doi: 10.1017/S1089332600001042
Chin, K., Eberth, D., Schweitzer, M., Rando, T., Sloboda, W., and Horner, J. (2003). Remarkable preservation of undigested muscle tissue within a Late Cretaceous tyrannosaurid coprolite from Alberta, Canada. Palaios 18, 286–294. doi: 10.1669/0883-1351(2003)018<0286:RPOUMT>2.0.CO;2
Clary, K. (1983). Prehistoric Coprolite Remains From Chaco Canyon, New Mexico: Inferences for Anasazi Diet and Subsistence. Albuquerque, NM: University of New Mexico.
de Souza, M., da Silva, L., Silva-Pinto, V., Mendez-Quiros, P., de Miranda Chaves, S., and Mayo Iñiguez, A. (2018). New paleoparasitological investigations from the pre-inca to hispanic contact period in northern Chile. Acta Trop. 178, 290–296. doi: 10.1016/j.actatropica.2017.11.021
DeNiro, M., and Epstein, S. (1978). Influence of diet on the distribution of carbon isotopes in animals. Geochim. Cosmochim. Acta 42, 495–506. doi: 10.1016/0016-7037(78)90199-0
Dentzien-Dias, P., Poinar, G., and Francischini, H. (2017). A new actinomycete from a Guadalupian vertebrate coprolite from Brazil. Hist. Biol. 29, 770–776. doi: 10.1080/08912963.2016.1241247
Eriksson, M., Lindgren, J., Chin, K., and Månsby, U. (2011). Coprolite morphotypes from the Upper Cretaceous of Sweden: novel views on an ancient ecosystem and implications for coprolite taphonomy. Lethaia 44, 455–468. doi: 10.1111/j.1502-3931.2010.00257.x
Ford, T., and O’Connor, B. (2002). Coprolite mining in England. Geol. Today 18, 178–181. doi: 10.1046/j.0266-6979.2003.00363.x
Ghosh, P., Bhattacharya, S., Sahni, A., Kar, R., Mohabey, D., and Ambwani, K. (2003). Dinosaur coprolites from the Late Cretaceous (Maastrichtian) Lameta Formation of India: isotopic and other markers suggesting a C3 plant diet. Cretac. Res. 24, 743–750. doi: 10.1016/j.cretres.2003.08.002
Gill, F., and Bull, I. (2012). Lipidanalysis of vertebrate coprolites. New Mexico Mus. Nat. Hist. Sci. Bull. 57, 93–98.
Gilmour, R., and Skinner, M. (2012). Forensic scatology: preliminary experimental study of the preparation and potential for identification of captive carnivore scat. J. Forensic Sci. 57, 160–165. doi: 10.1111/j.1556-4029.2011.01912.x
Gonçalves, M., Araújo, A., and Ferreira, L. (2003). Human intestinal parasites in the past: new findings and a review. Memórias Inst. Oswaldo Cruz 98, 103–118. doi: 10.1590/S0074-02762003000900016
Green, E., and Speller, C. (2017). Novel substrates as sources of ancient DNA: prospects and hurdles. Genes 8:180. doi: 10.3390/genes8070180
Greig, J. (1981). The investigation of a medieval barrel-latrine from Worcester. J. Archaeol. Sci. 8, 265–282. doi: 10.1016/0305-4403(81)90003-0
Hagan, R., Hofman, C., Hübner, A., Reinhard, K., Schnorr, S., and Lewis, C. Jr., et al. (2020). Comparison of extraction methods for recovering ancient microbial DNA from paleofeces. Am. J. Phys. Anthropol. 171, 275–284. doi: 10.1002/ajpa.23978
Häntzschel, W., El-Baz, F., and Amstutz, G. (1968). Coprolites an Annotated Bibliography. Boulder, Co: The Geological Society of America.
Harrison, T. (2011). “Coprolites: taphonomic and paleoecological implications,” in Paleontology and geology of Laetoli: Human evolution in context, ed. T. Harrison (Dordrecht: Springer). doi: 10.1007/978-90-481-9956-3_14
Heizer, R., and Napton, L. (1969). Biological and cultural evidence from prehistoric human coprolites. Science 165, 563–568. doi: 10.1126/science.165.3893.563
Hofreiter, M., Poinar, H., Spaulding, W., Bauer, K., Martin, P., Possnert, G., et al. (2000). A molecular analysis of ground sloth diet through the Last Glaciation. Mol. Ecol. 9, 1975–1984. doi: 10.1046/j.1365-294X.2000.01106.x
Hofreiter, M., Serre, D., Poinar, H., Kuch, M., and Pääbo, S. (2001). Ancient DNA. Nat. Rev. Genet. 2, 353–359. doi: 10.1038/35072071
Hollocher, T., Chin, K., Hollocher, K., and Kruge, M. (2001). Bacterial residues in coprolite of herbivorous dinosaurs: role of bacteria in mineralization of feces. Palaios 16, 547–565. doi: 10.1669/0883-1351(2001)016<0547:BRICOH>2.0.CO;2
Horwitz, L., and Goldberg, P. (1989). A study of Pleistocene and Holocene hyaena coprolites. J. Archaeol. Sci. 16, 71–94. doi: 10.1016/0305-4403(89)90057-5
Hunt, A., Chin, K., and Lockley, M. (1994). “The palaeobiology of vertebrate coprolites,” in The palaeobiology of Trace Fossils, ed. S. K. Donovan (Chichester: Johns Hopkins University Press, Wiley).
Hunt, A., Lucas, S., Milàn, J., and Spielmann, J. (2012). Descriptive terminology of coprolites and recent feces. Vertebrate Coprolites. New Mexico Mus. Nat. Hist. Sci. Bull. 57, 153–160.
Jain, S. (1983). Spirally coiled “coprolites” from the Upper Triassic Maleri formation, India. Palaeontology 26, 813–829.
Jouy-Avantin, F., Debenath, A., Moigne, A., and Moné, H. (2003). A standardized method for the description and the study of coprolites. J. Archaeol. Sci. 30, 367–372. doi: 10.1006/jasc.2002.0848
Karpinski, E., Mead, J., and Poinar, H. (2017). Molecular identification of paleofeces from Bechan Cave, southeastern Utah, USA. Q. Int. 443, 140–146. doi: 10.1016/j.quaint.2017.03.068
Khosla, A., Chin, K., Alimohammadin, H., and Dutta, D. (2015). Ostracods, plant tissues, and other inclusions in coprolites from the Late Cretaceous Lameta Formation at Pisdura, India: taphonomical and palaeoecological implications. Palaeogeogr. Palaeoclimatol. Palaeoecol. 418, 90–100. doi: 10.1016/j.palaeo.2014.11.003
Knights, B., Dickson, C., Dickson, J., and Breeze, D. (1983). Evidence concerning the Roman military diet at Bearsden, Scotland, in the 2nd century AD. J. Archaeol. Sci. 10, 139–152. doi: 10.1016/0305-4403(83)90048-1
Kühn, M., Maier, U., Herbig, C., Ismail-Meyer, K., Le Bailly, M., and Wick, L. (2013). Methods for the examination of cattle, sheep and goat dung in prehistoric wetland settlements with examples of the sites Alleshausen-Täschenwiesen and Alleshausen-Grundwiesen (around cal. 2900 BC) at Lake Federsee, south-west Germany. Environ. Archaeol. 18, 43–57. doi: 10.1179/1461410313Z.00000000017
Laojumpon, C., Matkhammee, T., Wathanapitaksakul, A., Suteethorn, V., Suteethorn, S., Lauprasert, K., et al. (2012). Preliminary report on coprolites from the Late Triassic of Thailand. Vertebrate Coprolites. New Mexico Mus. Nat. Hist. Sci. Bull. 57, 207–213.
Lee-Thorp, J., Sealy, J., and van der Merwe, N. (1989). Stable carbon isotope ratio differences between bone collagen and bone apatite, and their relationship to diet. J. Archaeol. Sci. 16, 585–599. doi: 10.1016/0305-4403(89)90024-1
Lin, D., Connor, W., Napton, L., and Heizer, R. (1978). The steroids of 2000-year-old human coprolites. J. Lipid Res. 19, 215–221. doi: 10.1016/S0022-2275(20)41560-3
Martin, P., and Sharrock, F. (1964). Pollen analysis of prehistoric human feces: a new approach to ethnobotany. Am. Antiq. 30, 168–180. doi: 10.2307/278848
McAllister, J. (1985). Reevaluation of the formation of spiral coprolites. Univ. Kansas Paleontol.l Contrib. Pap. 114, 1–12.
Minagawa, M., and Wada, E. (1984). Stepwise enrichment of 15N along food chains: further evidence and the relation between δ15N and animal age. Geochim. Cosmochim. Acta 48, 1135–1140. doi: 10.1016/0016-7037(84)90204-7
Moreau, J., Trincal, V., Nel, A., Simon-Coinçon, R., Sallé, V., Le Couls, M., et al. (2020). Hidden termite coprolites revealed by Synchrotron microtomography inside Eocene-Oligocene filled wood-borings from the Malzieu Basin, Lozère, southern France. Lethaia 53, 106–117. doi: 10.1111/let.12344
Morrow, J., Newby, J., Piombinomascali, D., and Reinhard, K. (2016). Taphonomic considerations for the analysis of parasites in archaeological materials. Int. J. Paleopathol. 13, 56–64. doi: 10.1016/j.ijpp.2016.01.005
Morrow, J., and Reinhard, K. (2016). Cryptosporidium parvum Among Coprolites from La Cueva de los Muertos Chiquitos (600–800 CE), Rio Zape Valley, Durango, Mexico. J. Parasitol. 102, 429–436. doi: 10.1645/15-916
Nunes, V., Alcover, J., Silva, V., Cruz, P., Machado-Silva, J., and de Araújo, A. (2017). Paleoparasitological analysis of the extinct Myotragus balearicus Bate 1909 (Artiodactyla, Caprinae) from Mallorca (Balearic Islands, Western Mediterranean). Parasitol. Int. 66, 7–11. doi: 10.1016/j.parint.2016.11.009
Palacio, P., Berthonaud, V., Guérin, C., Lambourdière, J., Maksud, F., Philippe, M., et al. (2017). Genome data on the extinct Bison schoetensacki establish it as a sister species of the extant European bison (Bison bonasus). BMC Evol. Biol. 17:48. doi: 10.1186/s12862-017-0894-2
Peterson, A., Soberón, J., Pearson, R., Anderson, R., Martínez-Meyer, E., Nakamura, M., et al. (2011). Ecological Niches and Geographic Distributions (MPB-49). New Jursey, NJ: Princeton University Press. doi: 10.23943/princeton/9780691136868.001.0001
Pizzi, T., and Schenone, H. (1954). Hallazgo de huevos de Trichuris trichiura en contenido intestinal de un cuerpo arqueológico incaico. Bol. Chileno Parasitol. 9, 73–75.
Poinar, H., Hofreiter, M., Spaulding, W., Martin, P., Stankiewicz, B., Bland, H., et al. (1998). Molecular coproscopy: dung and diet of the extinct ground sloth Nothrotheriops shastensis. Science 281, 402–406. doi: 10.1126/science.281.5375.402
Poinar, H., Kuch, M., Sobolik, K., Barnes, I., Stankiewicz, A., Kuder, T., et al. (2001). A molecular analysis of dietary diversity for three archaic Native Americans. Proc. Natl. Acad. Sci. U.S.A. 98, 4317–4322. doi: 10.1073/pnas.061014798
Porru, E., Giorgi, E., Turroni, S., Helg, R., Silani, M., Candela, M., et al. (2021). Bile acids and oxo-metabolites as markers of human faecal input in the ancient Pompeii ruins. Sci. Rep. 11:3650. doi: 10.1038/s41598-021-82831-y
Qvarnström, M., Niedźwiedzki, G., Tafforeau, P., Žigaitë, Ž, and Ahlberg, P. (2017). Synchrotron phase-contrast microtomography of coprolites generates novel palaeobiological data. Sci. Rep. 7:2723. doi: 10.1038/s41598-017-02893-9
Qvarnström, M., Niedźwiedzki, G., and Žigaitë, Ž (2016). Vertebrate coprolites (fossil faeces): an underexplored Konservat-Lagerstätte. Earth Sci. Rev. 162, 44–57. doi: 10.1016/j.earscirev.2016.08.014
Qvarnström, M., Ahlberg, P., and Niedźwiedzki, G. (2019a). Tyrannosaurid-like osteophagy by a Triassic archosaur. Sci. Rep. 9:925. doi: 10.1038/s41598-018-37540-4
Qvarnström, M., Elgh, E., Owocki, K., Ahlberg, P., and Niedźwiedzki, G. (2019b). Filter feeding in Late Jurassic pterosaurs supported by coprolite contents. PeerJ 7:e7375. doi: 10.7717/peerj.7375
Qvarnström, M., Wernström, J., Piechowski, R., Tałanda, M., Ahlberg, P., and Niedźwiedzki, G. (2019c). Beetle-bearing coprolites possibly reveal the diet of a Late Triassic dinosauriform. R. Soc. Open Sci. 6:181042. doi: 10.1098/rsos.181042
Reinhard, K., and Bryant, V. (1992). Coprolite analysis: a biological perspective on archaeology. Archaeol. Method Theory 4, 245–288.
Reinhard, K., Camacho, M., Geyer, B., Hayek, S., Horn, C., Otterson, K., et al. (2019). Imaging coprolite taphonomy and preservation. Archaeol. Anthropol. Sci. 11, 6017–6035. doi: 10.1007/s12520-019-00946-w
Rhode, D. (2003). Coprolites from Hidden Cave, revisited: evidence for site occupation history, diet and sex of occupants. J. Archaeol. Sci. 30, 909–922. doi: 10.1016/S0305-4403(02)00270-4
Rivera, L., Baraza, E., Alcover, J., Bover, P., Rovira, C., and Bartolomé, J. (2014). Stomatal density and stomatal index of fossil Buxus from coprolites of extinct Myotragus balearicus Bate (Artiodactyla, Caprinae) as evidence of increased CO2 concentration during the late Holocene. Holocene 24, 876–880. doi: 10.1177/0959683614530445
Rivera-Perez, J. (2017). Metagenomic Paleomicrobiology Characterizes Fecal Microbiomes and Viromes of Pre-Columbian Cultures from Vieques, Puerto Rico. San Juan, PR: University of Puerto Rico.
Rivera-Perez, J., Cano, R., Narganes-Storde, Y., Chanlatte-Baik, L., and Toranzos, G. (2015). Retroviral DNA sequences as a means for determining ancient diets. PLoS One 10:e0144951. doi: 10.1371/journal.pone.0144951
Rocha, G., Harter-Lailheugue, S., Le Bailly, M., Araújo, A., Ferreira, L., Serra-Freire, N., et al. (2006). Paleoparasitological remains revealed by seven historic contexts from “Place d’Armes”, Namur, Belgium. Memórias Inst Oswaldo Cruz 101, 43–52. doi: 10.1590/S0074-02762006001000008
Rummy, P., Halaclar, K., and Chen, H. (2021). The first record of exceptionally-preserved spiral coprolites from the Tsagan-Tsab formation (lower cretaceous), Tatal, western Mongolia. Sci. Rep. 11:7891. doi: 10.1038/s41598-021-87090-5
Sabin, S., Yeh, H., Pluskowski, A., Clamer, C., Mitchell, P., and Bos, K. (2020). Estimating molecular preservation of the intestinal microbiome via metagenomic analyses of latrine sediments from two medieval cities. Philos. Trans. R. Soc. B 375:20190576. doi: 10.1098/rstb.2019.0576
Santiago-Rodriguez, T., Narganes-Storde, Y., Chanlatte, L., Crespo-Torres, E., Toranzos, G., Jimenez-Flores, R., et al. (2013). Microbial communities in pre-Columbian coprolites. PLoS one 8:e65191. doi: 10.1371/journal.pone.0065191
Sanz, M., Daura, J., Égüez, N., and Brugal, J. (2016). Not only hyenids: A multi-scale analysis of Upper Pleistocene carnivore coprolites in Cova del Coll Verdaguer (NE Iberian Peninsula). Palaeogeogr. Palaeoclimatol. Palaeoecol. 443, 249–262. doi: 10.1016/j.palaeo.2015.11.047
Scott, L. (1979). Dietary inferences from Hoy House coprolites: a palynological interpretation. Kiva 44, 257–281. doi: 10.1080/00231940.1979.11757920
Sealy, J., van der Merwe, N., Thorp, J., and Lanham, J. (1987). Nitrogen isotopic ecology in southern Africa: implications for environmental and dietary tracing. Geochim. Cosmochim. Acta 51, 2707–2717. doi: 10.1016/0016-7037(87)90151-7
Shillito, L., Bull, I., Matthews, W., Almond, M., Williams, J., and Evershed, R. (2011). Biomolecular and micromorphological analysis of suspected faecal deposits at Neolithic Çatalhöyük, Turkey. J. Archaeol. Sci. 38, 1869–1877. doi: 10.1016/j.jas.2011.03.031
Sistiaga, A., Berna, F., Laursen, R., and Goldberg, P. (2014a). Steroidal biomarker analysis of a 14,000 years old putative human coprolite from Paisley Cave, Oregon. J. Archaeol. Sci. 41, 813–817. doi: 10.1016/j.jas.2013.10.016
Sistiaga, A., Mallol, C., Galván, B., and Summons, R. (2014b). The Neanderthal meal: a new perspective using faecal biomarkers. PLoS One 9:e101045. doi: 10.1371/journal.pone.0101045
Smith, D. (2013). Defining an indicator package to allow identification of ‘cesspits’ in the archaeological record. J. Archaeol. Sci. 40, 526–543. doi: 10.1016/j.jas.2012.06.014
Speller, C., Kemp, B., Wyatt, S., Monroe, C., Lipe, W., Arndt, U., et al. (2010). Ancient mitochondrial DNA analysis reveals complexity of indigenous North American turkey domestication. Proc. Natl. Acad. Sci. U.S.A. 107, 2807–2812. doi: 10.1073/pnas.0909724107
Sutton, M., Malik, M., and Ogram, A. (1996). Experiments on the determination of gender from coprolites by DNA analysis. J. Archaeol. Sci. 23, 263–267. doi: 10.1006/jasc.1996.0023
Taglioretti, V., Fugassa, M., and Sardella, N. (2015). Parasitic diversity found in coprolites of camelids during the Holocene. Parasitol. Res. 114, 2459–2464. doi: 10.1007/s00436-015-4442-y
Taylor, A., Hutson, J., Bryant, V., and Jenkins, D. (2020). Dietary items in Early to Late Holocene human coprolites from Paisley Caves, Oregon, USA. Palynology 44, 12–23. doi: 10.1080/01916122.2018.1530699
Tito, R., Knights, D., Metcalf, J., Obregon-Tito, A., Cleeland, L., Najar, F., et al. (2012). Insights from characterizing extinct human gut microbiomes. PLoS One 7:e51146. doi: 10.1371/journal.pone.0051146
Tolar, T., and Galik, A. (2019). A study of dog coprolite from Late Neolithic Pile-Dwelling Site in Slovenia. Archaeol. Discov. 7:20. doi: 10.4236/ad.2019.71002
Tosto, A., Burry, L., Arriaga, M., and Civalero, M. (2016). Archaeobotanical study of Patagonian Holocene coprolites, indicators of diet, cultural practices and space use. J. Archaeol. Sci. 10, 204–211. doi: 10.1016/j.jasrep.2016.09.017
Turvey, S., Oliver, J., Narganes Storde, Y., and Rye, P. (2007). Late Holocene extinction of Puerto Rican native land mammals. Biol. Lett. 3, 193–196. doi: 10.1098/rsbl.2006.0585
van der Merwe, N. (1986). Carbon isotope ecology of herbivores and carnivores. Palaeoecol. Afr. Surrounding Islands 17, 123–131.
Velázquez, N., Burry, L., and Fugassa, M. (2015). Palynological analysis of extinct herbivore dung from Patagonia, Argentina. Q. Int. 377, 140–147. doi: 10.1016/j.quaint.2015.05.012
Vogel, J. (1978). Isotopic assessment of the dietary habits of ungulates. South Afr. J. Sci. 74:298.
Wang, X., White, S., Balisi, M., Biewer, J., Sankey, J., Garber, D., et al. (2018). First bone-cracking dog coprolites provide new insight into bone consumption in Borophagus and their unique ecological niche. eLife 7:e34773. doi: 10.7554/eLife.34773.023
Watson, P., and Yarnell, R. (1966). Archaeological and paleoethnobotanical investigations in salts cave, mammoth cave national park, Kentucky. Am. Antiq. 31, 842–849. doi: 10.2307/2694457
Welker, F., Duijm, E., van der Gaag, K., van Geel, B., de Knijff, P., van Leeuwen, J., et al. (2014). Analysis of coprolites from the extinct mountain goat Myotragus balearicus. Q. Res. 81, 106–116. doi: 10.1016/j.yqres.2013.10.006
Williams-Dean, G. (1978). Ethnobotany and Cultural Ecology of Prehistoric Man in Southwest Texas. College Station, TX: Texas A&M University.
Wood, J., Crown, A., Cole, T., and Wilmshurst, J. (2016). Microscopic and ancient DNA profiling of Polynesian dog (kurî) coprolites from northern New Zealand. J. Archaeol. Sci. 6, 496–505. doi: 10.1016/j.jasrep.2016.03.020
Wood, J., and Wilmshurst, J. (2013). Pollen analysis of coprolites reveals dietary details of heavy-footed moa (Pachyornis elephantopus) and coastal moa (Euryapteryx curtus) from Central Otago. New Zealand J. Ecol. 37, 151–155.
Zatoń, M., Broda, K., Qvarnström, M., Niedźwiedzki, G., and Ahlberg, P. (2017). The first direct evidence of a Late Devonian coelacanth fish feeding on conodont animals. Sci. Nat. 104:26. doi: 10.1007/s00114-017-1455-7
Zhang, Y., van Geel, B., Gosling, W. D., McMichael, C., Jansen, B., Absalah, S., et al. (2019). Local vegetation patterns of a Neolithic environment at the site of Tianluoshan, China, based on coprolite analysis. Rev. Palaeobot. Palynol. 271:104101. doi: 10.1016/j.revpalbo.2019.104101
Keywords: coprolite, diet, palaeocoprology, ecological niche, evolution
Citation: Yang L, Zhang X, Zhao X and Xiang H (2022) The Technological Advance and Application of Coprolite Analysis. Front. Ecol. Evol. 9:797370. doi: 10.3389/fevo.2021.797370
Received: 18 October 2021; Accepted: 17 December 2021;
Published: 07 January 2022.
Edited by:
Anderson Santos, Rio de Janeiro State University, BrazilReviewed by:
Maciej Tomasz Krajcarz, Institute of Geological Sciences, Polish Academy of Sciences (PAN), PolandMarcelo Alfredo Reguero, Argentine Antarctic Institute (IAA), Argentina
Copyright © 2022 Yang, Zhang, Zhao and Xiang. This is an open-access article distributed under the terms of the Creative Commons Attribution License (CC BY). The use, distribution or reproduction in other forums is permitted, provided the original author(s) and the copyright owner(s) are credited and that the original publication in this journal is cited, in accordance with accepted academic practice. No use, distribution or reproduction is permitted which does not comply with these terms.
*Correspondence: Xingbo Zhao, emh4YkBjYXUuZWR1LmNu; Hai Xiang, dmFteWx1b0AxMjYuY29t